- Fish Nutrition Research Laboratory, Department of Zoology, Aligarh Muslim University, Aligarh, India
Groundnut oil (GO) is one of the most widely available vegetable oils (VOs) in India and throughout the world, with a global production of 6.12 million metric tons in the year 2020–2021. GO contains phytochemicals and antioxidants with a longer shelf life. Because of these benefits, GO can produce durable, low-cost, and sustainable aquaculture feeds. To evaluate the nutritional efficacy and possibility of replacing fish oil (FO) with GO, this experiment was carried out to test the effects of partial or total substitution of dietary FO by GO on the growth performance, carcass composition, antioxidant capacity, lysozyme activity, muscle fatty acid composition, and filet nutritional quality in Labeo rohita fingerling. Induced bred healthy rohu fingerlings (4.84 ± 0.13 g) were fed six isonitrogenous (400 g kg−1) and isolipidic (97 g kg−1) casein- and gelatin-containing purified diets, wherein the FO was gradually replaced by 0, 20, 40, 60, 80, and 100% GO. Fishes were stocked randomly in triplicate groups of 30 fish per tank and fed at 08:00, 12:00, and 16:00 h to apparent satiety for 8 weeks. The results showed that FO replacement with GO did not affect the growth but decreased the eicosapentaenoic (EPA), docosahexaenoic acid (DHA) levels, and n-3/n-6 ratio in the muscle of rohu fingerlings. The antioxidant capacity and lysozyme activity improved up to 60% replacement of FO with GO in diet and then declined (P > 0.05) upon further inclusion of GO in diets. Although the atherogenicity, thrombogenicity indices, and hypocholesterolemic/hypercholesterolemic (H/H) ratio did not change significantly among all the muscle samples, the highest filet lipid quality (FLQ) value was found in fish receiving a 100% FO diet. Further inclusion of dietary GO decreased the filet H/H ratio and FLQ value. In summary, replacing FO with GO at a higher level had negative consequences on the filet nutritional quality of rohu fingerlings. Therefore, FO can be replaced by GO in formulated feeds to a level of 60% without hampering the growth, antioxidant capacity, and lysozyme activity and to avoid degrading the nutritional quality of fish filet.
Introduction
Aquatic foods from marine and freshwater play an important role in the nutrition of more than 3.3 billion people globally (FAO, 2020). Global food fish consumption increased at an average annual rate of 3.1% from 1961 to 2017, a rate almost two times that of the annual world population growth (1.6%) and higher than that of all the other animal protein foods, which increased by 2.1% per year (SOFIA, 2020). Fish oil (FO), obtained from wild catch fish, is a good source of long-chain polyunsaturated fatty acids (n-3 LC-PUFA), mainly 20:5n-3 (eicosapentaenoic acid, EPA) and 22:6n-3 (docosahexaenoic acid, DHA). EPA and DHA are considered essential for cell membrane function and maintenance. These LC-PUFAs also play an essential role in preventing neurodegenerative and arteriosclerosis diseases, as well as cardiovascular and cerebrovascular diseases in humans (Golden et al., 2021). However, traditional capture fisheries may no longer be the world's most important provider of food fish. Global fish production in 2018 reached about 179 million tons, and the world aquaculture production was 114.5 million tons (valued at USD 250 billion), accounting for 63.7% of the total production (FAO, 2020). With the capture fishery being stagnant, aquaculture is the only means to increase production. Currently, aquaculture in India is based on a semi-intensive level and needs to be intensified. This could be done by using nutritionally balanced formulated feeds with locally available feed ingredients, which may increase aquaculture production and profitability, especially when reared in high densities. To formulate a nutritionally balanced, cost-effective, and eco-friendly diet for intensive aquaculture of a candidate fish, the information on nutrient requirements is essential. Among the nutrients required for physiological functions, dietary lipids are essential to maintain the normal growth and health of fish.
Lipids have two prominent roles: to provide metabolic energy for growth and homeostasis and as a source of phospholipids and essential fatty acids such as EPA and DHA. Like humans, fish also need to obtain EPA and DHA through their diet (NRC, 2011). Both EPA and DHA play important roles in accelerating fish growth and neural development, regulating fish metabolism, and improving the immunity of the organism (Yu et al., 2019). The stagnation of capture fishery has led to the shortage of FO production, a main source of EPA and DHA, which has increased its price. The high cost of FO has vast implications for aquafeed and aquaculture production (Sankian et al., 2019), making it economically unsustainable. Also, the presence of LC-PUFAs in FO, especially EPA and DHA, has a huge tendency to lipid peroxidation, leading to oxidative stress. Thus, finding economically viable alternatives to reduce reliance on FO in aquaculture is imperative. Reducing the utilization of FO in feed formulations while ensuring the availability of appropriate proportions of n-3 LC-PUFA in the final product is a challenge. Vegetable oils (VOs), rich in C18 PUFA, have been considered the most sustainable alternatives to FO due to their wide range of availability and low price. VOs have lower dioxin concentrations, leading to a less environmental impact. Also, VOs are less susceptible to lipid peroxidation (Ayisi et al., 2019). Thus, VOs are proven to be excellent and sustainable alternatives to FO in aquafeed for freshwater fish species. However, VOs lack essential fatty acids like LC-PUFA (Sankian et al., 2019), which are required in the filets for a healthier product and for the health of human consumers. As freshwater fishes have a high capacity to convert C18 PUFAs to C20/22 PUFAs, such as arachidonic acid (AA), EPA, and DHA (Ren et al., 2012), the deficiency of LC-PUFA can be satisfied. Biosynthesis involves the desaturation and elongation of C18 PUFAs (alpha-linolenic acid, ALA, and linoleic acid, LA), and Δ6 desaturase and Elovl5 elongase are the critical enzymes in the biosynthetic pathway of LC-PUFA (Tocher et al., 2004). The desaturation and elongation capacity varies with the fish species (Monroig et al., 2011). The effects of the incorporation of dietary VOs in aquaculture feeds have been previously worked out in many fish species, such as Psetta maxima (Regost et al., 2003), European sea bass, Dicentrarchus labrax (Mourente et al., 2005), rohu, Labeo rohita (Karanth et al., 2009), iridescent shark, Pangasius hypophthalmusturbot (Asdari et al., 2011), blunt snout bream, Megalobrama amblycephala (Li et al., 2015), gibel carp, Carassius auratus gibelio (Zhou et al., 2016), hybrid grouper, Epinephelus fuscoguttatus × E. lanceolatus (An et al., 2020), common carp, Cyprinus carpio (Ren et al., 2020), golden pompano, Trachinotus ovatus (Guo et al., 2021), turbot, Scophthalmus maximus (Xu et al., 2021), tiger puffer, Takifugu rubripes (Liao et al., 2021), and blackhead seabream, Acanthopagrus schlegelii (Zhu et al., 2022).
Mostly used VOs in aquaculture feeds are linseed, soybean, rapeseed, palm, olive, groundnut, and sunflower oil (Ayisi et al., 2019). Although all the above oils are consumed by human beings, a comparatively huge availability of groundnut oil (GO) in Asian countries, especially China and India, makes it a preferred ingredient to use in aquafeeds. It is considered a potential substitution for FO. GO, derived from groundnut (Arachis hypogaea), is one of the most widely available VOs in India. The crop is planted in two cycles and harvested in March and October, thus making it available throughout the year. In the year 2020–2021, the global production of GO amounted to 6.12 million metric tons (Shahbandeh, 2021), and its production is increasing. India was the second largest producer of GO in the year 2020–2021 after China, with 1.27 million metric tons of GO production (USDA, 2022). Owing to its huge availability, it is considered an excellent candidate to replace FO in fish feeds. Also, the price of GO is 1,000 USD/ton against the price of FO, which is 2,000 USD/ton (Kesbiç et al., 2016; Acar and Türker, 2018). GO has a very stable flavor due to a comparatively lower amount of linoleic acid, known for causing deterioration of oil flavor. In addition, it has a longer shelf life due to the higher proportion of saturated fatty acids. GO also contains a high level of PUFA (NRC, 2011). The major fatty acids of GO are 10% palmitic (C16:0), 45% oleic (C18:1n-9), and 30% linoleic (C18:2n-6) acid with alpha-linolenic acid (C18:3n-9) present in traces (Dernekbasi et al., 2020). It also contains up to 6% LC-PUFA, such as 20:0, 22:0, and 24:0, as compared to other VOs (Dernekbasi et al., 2020). Thus, GO is a suitable alternative feedstuff to FO. The effects of the incorporation of dietary GO in aquaculture feeds have been previously worked out in many fish species, such as pikeperch, Sander lucioperca (Kowalska et al., 2010), African catfish, Clarias gariepinus (Ochang, 2012), catfish, Heterobranchus longifilis (Babalola and Apata, 2012), Nile tilapia, Oreochromis niloticus (Sagne et al., 2013; Erondu et al., 2021), common carp, C. carpio (Yildirim et al., 2013), Mozambique tilapia, Oreochromis mossambicus (Demir et al., 2014), two-banded sea bream, Diplodus vulgaris (Kesbiç et al., 2016), rainbow trout, Oncorhynchus mykiss (Acar and Türker, 2018), and European sea bass, Dicentrarchus labrax (Dernekbasi et al., 2020).
Indian freshwater aquaculture is mainly dominated by carp species (Rasal and Sundaray, 2020). Among these, Indian major carps (IMCs), catla, Catla catla, rohu, L. rohita, and mrigal, Cirrhinus mrigala, are the most important and prime cultivable fish species in India (Surasani et al., 2021) due to their high customer demand and economic value. IMCs contribute more than 70% of freshwater production in India (DoF, 2020). Out of all these carps, rohu is very popular due to its high growth rate, taste, and public preference. It consists of almost 35% of India's total cultured fish production (Mir et al., 2017). It can grow up to 800–1,000 g in a year. Rohu is also a candidate aquaculture species in India, Bangladesh, Myanmar (Burma), and Pakistan (Rasal and Sundaray, 2020). With the increasing market demand for rohu, there is a dire need to intensify its culture, requiring nutritionally balanced and low-cost aquaculture feeds. Much information on dietary protein (Debnath et al., 2012), amino acid (Abidi and Khan, 2004, 2007, 2010, 2011), vitamin (Rahman et al., 2018; Tandel et al., 2019; Inayat et al., 2020), and mineral (Musharraf and Khan, 2018, 2019a,b, 2021) requirements of this species is available. However, very scarce information is available for this species regarding the FO substitution with VOs. Because of the enormous availability and low price of GO, the objectives of the present study were to evaluate the possible effects of dietary FO substitution with GO on the growth performance of rohu fingerlings. In addition to the growth performance, feed utilization, fatty acid profiles, filet quality, and immune response were also studied.
Materials and Methods
Preparation of Experimental Diets
Six experimental, purified, isonitrogenous (400 g kg−1 crude protein), isolipidic (97 g kg−1 crude lipid) diets were formulated wherein the FO was gradually replaced by 0, 20, 40, 60, 80, and 100% GO. The diets were designated as 0 GO, 20 GO, 40 GO, 60 GO, 80 GO, and 100 GO (Table 1). Unrefined GO was obtained from a local factory for the replacement trial. Dietary lipid was fixed at 97 g kg−1 in the experimental diets as per the requirement worked out for fingerlings of L. rohita (Siddiqua and Khan, 2022). The fatty acid compositions of the diets are depicted in Table 2. Casein and gelatine (fat-free) were used as sources of protein and dextrin as carbohydrate. The compositions of mineral and vitamin mixtures were as per Halver (2002). The diets were prepared as per the method reported earlier (Abdel-Hameid et al., 2017). Gelatin was dissolved in distilled water by stirring and heating the bowl on a double-boiling heating system followed by adding and cooking casein at 80°C. When the mixture cooled down, the remaining ingredients, including oils, were mixed for about 15 min in a blender (Hobart Corporation, Troy, OH, USA) and then vitamin and mineral premixes were added with continuous mixing at 40°C. Lastly, carboxymethyl cellulose was mixed. The dough thus produced was forced through the 2-mm die of an extruder. The strands thus obtained were dried at 40°C to reduce the moisture content to less than 100 g kg−1. The strands were crumbled to the required size (500 μm), packed in several small packets to meet the weekly quantitative needs, and preserved at −4°C.
Experimental Fish Maintenance
For this experiment, fingerlings of Labeo rohita were collected from the hatchery of the College of Fisheries, G. B. Pant University of Agriculture and Technology, Pantnagar. Fish were stocked in cylindrical (water volume 600 L, 1.22 m in diameter, and 0.91 m in height) tanks after giving prophylactic treatment in KMnO4 (1:3,000) solution, and adapted by feeding H440 dry diet (Halver, 2002) for 2 weeks before proceeding for the feeding trial. The experiment was conducted in the wet laboratory of the Department of Zoology, Aligarh Muslim University, Aligarh, India.
Experimental Design and Feeding Trial
Rohu fingerlings (6.58 ± 0.21 cm, 4.84 ± 0.13 g) were randomly stocked in 18 circular (water volume 55 L) polyvinyl tanks at the rate of 30 fish in triplicate groups supplied with flow-through water at the rate of 1–1.5 L min−1. The fish were fed by hand to near satiety three times at 8:00, 12:00, and 16:00 h. A photoperiod of 12-h light and 12-h dark was maintained. The leftover feed after each feeding was collected instantly after satiety was obtained and recorded for the calculations of feed conversion ratio. The weight gain was noted fortnightly on a balance (Precisa 120A, Oerlikon AG, Zurich, Switzerland) after anesthetizing in tricaine methanesulfonate solution (MS-222, 100 mg L−1, Sigma, St. Louis, MO, USA). On the sampling day, the fish were not fed to avoid stress. The experiment was conducted for 8 weeks.
Water Quality Parameter Analysis
Water quality parameters were measured weekly (APHA, 1992). The water temperature ranged from 25.6 to 28.7°C, and the dissolved oxygen ranged from 6.45 to 7.37 mg L−1. Total ammonia nitrogen, alkalinity, free carbon dioxide, and pH were recorded in the range of 0.22–0.41 mg L−1, 64.9–78.1 mg L−1, 6.1–10.3 mg L−1, and 7.2–7.5, respectively.
Sample Collection and Chemical Analyses
After starving for 24 h, 30 fish were randomly collected (4.84 ± 0.13 g), their length and weight were recorded, and the condition factor (CF) was calculated. These fish were then killed with an anesthetic overdose (200 mg L−1) of MS-222 and pooled. Ten subsamples were used to assess the initial body composition, the hepatosomatic index (HSI), and the viscerosomatic index (VSI). When the trial ended, 10 fish from each replicate of the groups were collected and anesthetized with MS-222 (100 mg L−1), and blood samples from the caudal vein were collected. To collect serum, blood samples were left to settle for about 10 min in a slanted position for clotting at room temperature. The samples were centrifuged (3,000 × g, 4°C, 10 min), and serum was collected and preserved at −20°C for further analysis. The length and weight of five fish from each replicate group were recorded to calculate the CF. The liver and viscera of these fish were dissected, and the weight was recorded to calculate the HSI and VSI. After that, muscle samples from another five fish were immediately harvested and preserved at −20°C for the analysis of fatty acid composition. The proximate composition of the remaining fish from each replicate (n = 3 × 5) group was analyzed.
Proximate Composition Analysis
The proximate composition of the test diets and the initial and final body was analyzed using the AOAC (2015) methods. The moisture content was determined in a hot air oven at 102 ± 1°C (Yorko Instruments, New Delhi, India). The crude protein (N × 6.25) was determined in an automatic analyzer (Kjeltec TecatorTM Technology 2300, Hoganas, Sweden). The crude fat was assessed by extracting in a solvent (Socs Plus SCS 4, Pelican Equipments, Chennai, India), and ash was determined by burning the sample in a furnace at 650°C for 12 h (S.M. Scientific Instrument Pvt. Ltd., Jindal Company, New Delhi, India). The gross energy was determined in a ballistic bomb calorimeter (CBB 330 010L, Gallenkamp, Loughborough, UK).
Oxidation and Antioxidant Parameters Assay
Serum subsamples (n = 3 × 5) were subjected to biochemical analyses of malondialdehyde (MDA), superoxide dismutase (SOD), catalase (CAT), and glutathione peroxidase (GPx). The method adopted by Buege and Aust (1978) was used to determine the MDA concentration. SOD was assayed by following the method of Misra and Fridovich (1972), CAT activity was determined by the method of Takahara et al. (1960), and GPx by the method of Rotruck et al. (1973). Serum MDA concentration was expressed in nmol ml−1, whereas SOD, CAT, and GPx activities were expressed in U ml−1.
Lysozyme Activity Analysis
Lysozyme activity of serum was determined following the turbidimetric method (Hultmark et al., 1980), as adopted by Wang et al. (2015). A serum sample (20 μL) with 0.1 M sodium phosphate buffer (pH 6.4) was added to the suspension of 1.2 mL Micrococcus lysodeikticus. The absorbance was read in a spectrophotometer at 530 nm after 0.5 and 4.5 min. One unit of lysozyme activity is defined as the amount of sample causing a decrease in absorbance of 0.001 min−1 at 530 nm compared with the control. The lysozyme activities were expressed in U ml−1.
Fatty Acid Profile Assay
The muscle fatty acid composition was analyzed following Metcafe et al. (1966) procedures with some modifications. About 50–100 mg of freeze-dried muscle samples were added into a 20-mL tube with a lid. After that, 1 ml diethyl ether was added and mixed. Then, 1 mL 0.5% methanolic potassium hydroxide (1 N) was added, continuously shaken for 10 min, and heated in a water bath at 75°C for 20 min. Hydrogen chloride (1 ml, 1 N) was added, cooled, and then heated in a water bath at 75°C for another 20 min. The above test was carried out previously to ensure that all fatty acids are esterified. After that, 2–3 mL petroleum ether was added, shaken continuously for about 1 min, and allowed to get separated into two layers. The upper layer containing fatty acid methyl esters was separated, decanted, and dried in a water bath for about 20 min. Lastly, 0.5 mL n-heptane was added to the tube. Finally, all the samples were quantified by a gas chromatograph–mass spectrometer (GC–MS) (Shimadzu QP-2010 Plus coupled with Thermal Desorption System TD 20). The percent of each fatty acid was determined as the proportion of the area under the peak in question to the total area of all peaks.
Calculation of Growth Parameters, Biometric Indices, and Diet Peroxidation Index
Absolute weight gain (g fish−1) = Final body weight (g fish−1) – Initial body weight (g fish−1),
Specific growth rate (SGR; % day−1) = ln final body weight (g) – ln initial body weight (g)/No. of days of the experiment × 100,
Feed conversion ratio (FCR) = Dry feed fed (g)/wet weight gain (g),
Percent survival = Final number of fish/initial number of fish × 100,
Hepatosomatic index (HSI%) = Liver weight (g)/body weight (g) × 100,
Viscerosomatic index (VSI%) = Viscera weight (g)/body weight (g) × 100,
Condition factor (CF) = Body weight (g)/body length (cm)3 × 100,
PIn (Peroxidation index) = 0.025 × (percentage of monoenoics) + 1 × (percentage of dienoics) + 2 × (percentage of trienoics) + 4 × (percentage of tetraenoics) + 6 × (percentage of pentaenoics) + 8 × (percentage of hexaenoics) (Yuan et al., 2019).
Calculation of Filet Nutritional Indices
The fatty acid composition of muscle was used to determine the nutritional parameters of lipids. The following equations were used to calculate filet nutritional quality indices. The n-3/n-6, n-6/n-3, PUFA/SFA, and EPA/ARA ratios were also calculated.
AI (atherogenicity) = [12:0 + (4 × 14:0) + 16:0] / [∑MUFA + ∑n-3 + ∑n-6] (Ulbricht and Southgate, 1991),
TI (thrombogenicity) = (14:0 + 16:0 + 18:0) / [0.5 × (∑MUFA + ∑n-6) + (3 × ∑n-3) + (∑n-3/∑n-6)] (Ulbricht and Southgate, 1991),
H/H index (hypocholesterolemic/hypercholesterolemic FA ratio) = (18:1n-9 + 18:2n-6 + 18:3n-3 + 20:4n-6 + 20:5n-3 + 22:5n-3 + 22:6n-3) / (14:0 + 16:0) (Santos-Silva et al., 2002),
Filet lipid quality (FLQ) = (20:5n-3 + 22:6n-3)/ ∑total FA (Abrami et al., 1992).
Statistical Analyses
The growth responses were subjected to a one-way analysis of variance (Sokal and Rohlf, 1981). The data were tested for normality using the Shapiro–Wilk test and the homogeneity of variance using Levene's test. Tukey's honest significant difference (HSD) test was performed to analyze the mean comparisons at the P < 0.05 level. The statistical analyses were carried out using SPSS 20.0 (SPSS, USA). Principal component analysis (PCA) (Wold et al., 1987) was used as an unsupervised pattern for the statistical procedure that converts a set of observations of possibly correlated variables into a set of values of linearly uncorrelated variables (principal components) using orthogonal transformation. The main information in the variables is expressed by a lower number of variables called principal components (PC1 and PC2). The PCA was performed on the data matrix of the fatty acid composition of the muscle using statistical software (Origin version 9.1, Origin Software, San Clemente, CA).
Results
Growth Response, Conversion Efficiency, Proximate Composition, and Biometric Indices
The effects of replacing FO with GO on the growth performance of rohu fingerlings are summed up in Table 3. Dietary GO inclusion had no negative impact (P > 0.05) on the growth performance. The absolute weight gain (AWG), specific growth rate (SGR), and feed conversion ratio (FCR) ranged between 21.65 and 23.78 g fish−1, 3.01% and 3.20% day−1, and 1.31 and 1.68, respectively. No mortality was recorded in all the groups. The whole-body protein was recorded highest (P < 0.05) in fish receiving a 100% FO diet (0 GO), followed by 20, 40, and 60% replacement of FO in diets. The lowest body protein was evident in the fish receiving diets replacing 80 and 100% FO. The whole-body fat content showed the reverse trend (Table 4). HSI and VSI were recorded highest (P < 0.05) in fish receiving a diet with 100% replacement of FO (100 GO) followed by a diet containing 80% replacement of FO (80 GO). The lowest values for HSI and VSI were recorded from fish fed a 100% FO diet (0 GO), followed by 20% (20 GO), 40% (40 GO), and 60% (60 GO) replacement of FO diets. However, the condition factor remained significantly unchanged (P > 0.05) in the fish receiving all the diets.
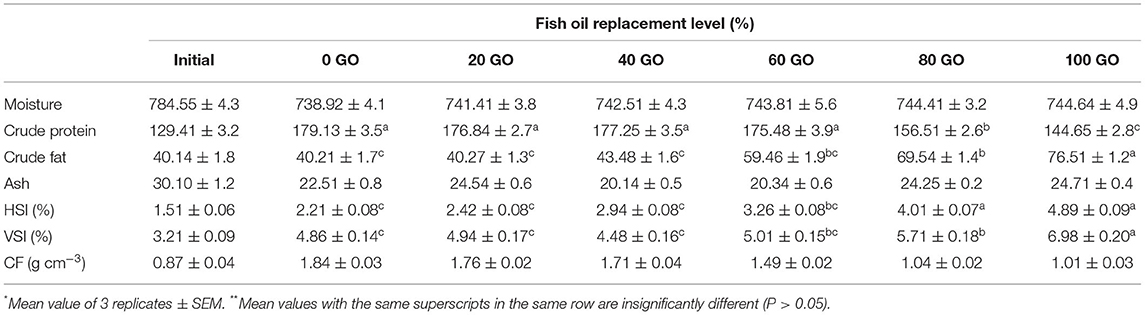
Table 4. Carcass compositions (g kg−1, wet basis), and biological indices of fingerling Labeo rohita fed different experimental diets *,**.
Serum Antioxidant and Non-specific Immune Status
Serum antioxidant activities of rohu fingerlings fed test diets are depicted in Table 5. Serum SOD, CAT, and GPx values did not change significantly (P > 0.05) up to 60% replacement of FO in diet (60 GO) and then declined with the lowest activity noted in fish fed diet with 100% replacement of FO (100 GO). The highest MDA activity (P < 0.05) was noted in fish fed a 100% FO (0 GO) diet, whereas the activity decreased upon further inclusion of dietary GO. The highest serum lysozyme activity (P < 0.05) was noted in fish fed diet with 60% replacement of FO (60 GO), followed by 40% (40 GO) and 0% (100 GO) FO replacement diets. The lowest lysozyme activity was recorded from fish receiving a 100% GO (100 GO)-containing diet.
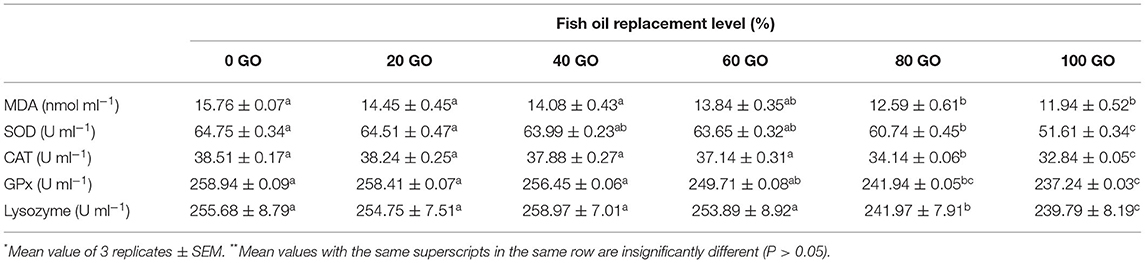
Table 5. Serum antioxidant capacity and lysozyme activity of fingerling Labeo rohita fed different experimental diets*,**.
Muscle Fatty Acid Compositions
The muscle fatty acid profile of rohu fingerlings fed different diets is depicted in Table 6. The highest proportion of SFA and MUFA (P < 0.05) were noted in fish fed a 100% GO diet (100 GO). No significant difference (P > 0.05) was recorded in the percentage of ALA in the muscle of fish fed all the diets, whereas muscle LA was recorded highest in fish fed 100% GO (100 GO) diet followed by 80% GO (80 GO) diet. Muscle EPA, DHA, and n-3/n-6 ratio were recorded highest (P < 0.05) in fish fed a 100% FO diet (0 GO), and then the value declined upon inclusion of GO in diets with the lowest value recorded from fish receiving a 100% GO (100 GO) diet. The muscle fatty acid composition data of fish fed all the diets were subjected to principal component analysis (PCA), and the result is shown in Figure 1. The bi-plot of the first two principal components accounted for 99.63% of total variance with PC1 (main axis 1) of 89.32% and PC2 (main axis 2) of 10.31%, respectively. The bi-plot also revealed that LA, OA, EPA, and DHA were significant for explaining the variations among all the samples.
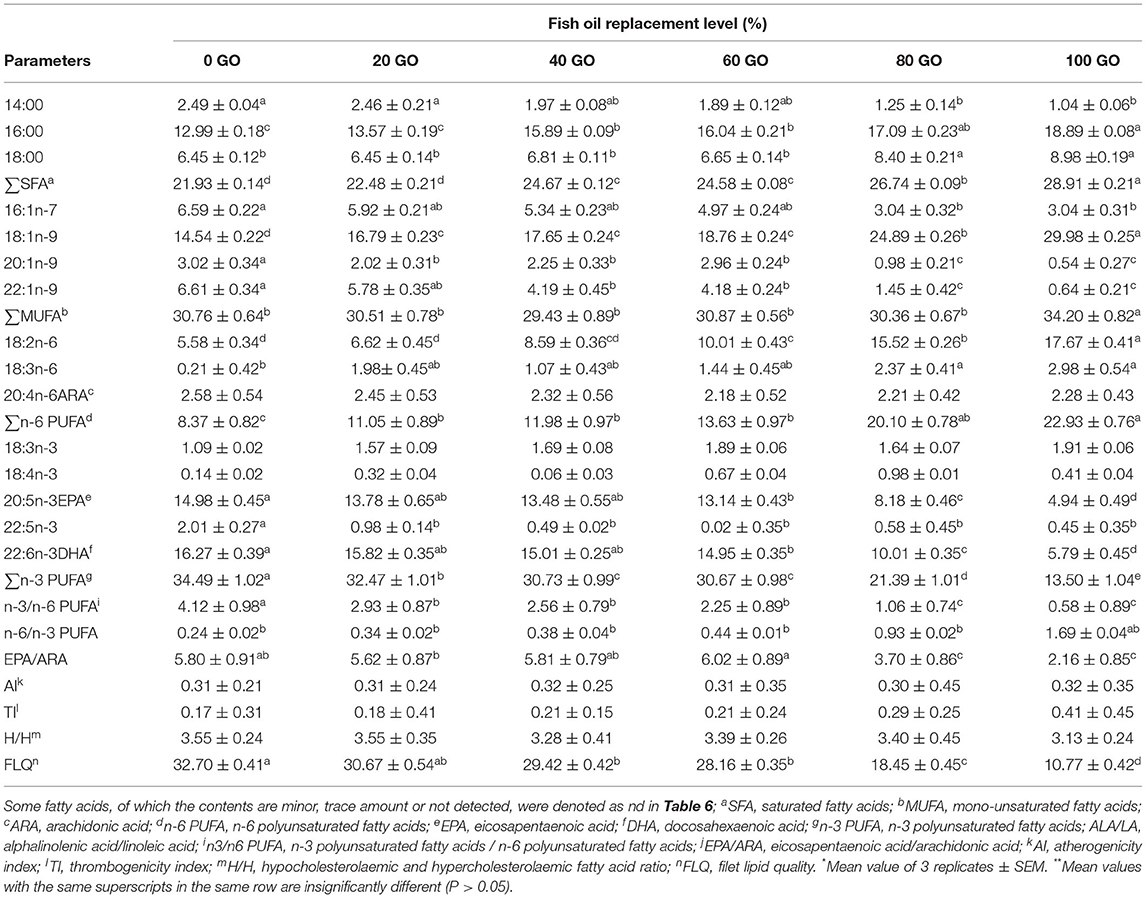
Table 6. Muscle fatty acid compositions of fingerling Labeo rohita fed different experimental diets (% total fatty acids)*,**.
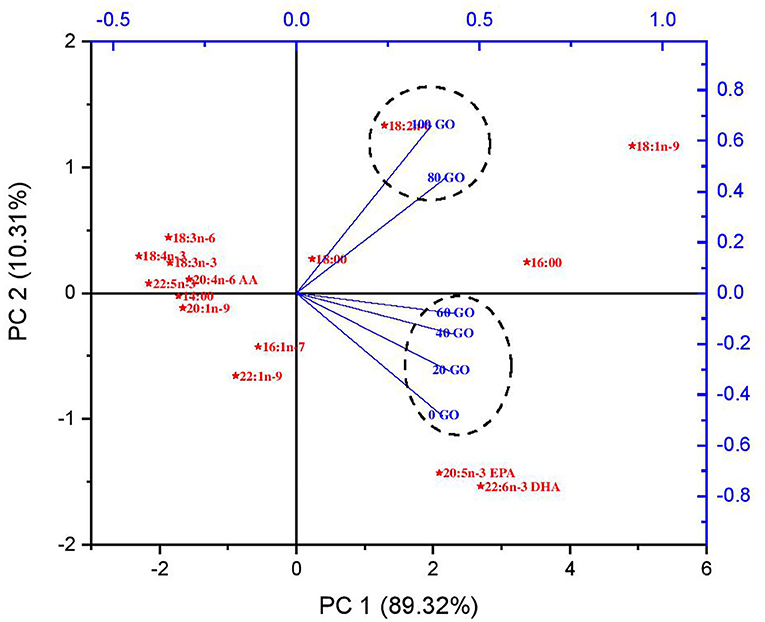
Figure 1. Principal component analysis of muscle fatty acids of rohu fingerling fed on different experimental diets.
Nutritional Quality Indices of Fish Filet
The nutritional quality indices of fish filet fed different diets are shown in Table 6 and Figure 2. AI, TI, and H/H indices in the muscle of rohu fingerlings did not differ among treatments (P > 0.05). However, the highest (P < 0.05) FLQ value was noted in the filet of fish fed a 100% FO diet and then the value declined upon further inclusion of GO in the diet. EPA/ARA ratio remained significantly unchanged up to 60% replacement of FO (60 GO) and further inclusion of dietary GO resulted in a significant decrease (P < 0.05) in EPA/ARA ratio (GO and 100 GO). PUFA/SFA ratio did not significantly differ (P > 0.05) among all the treatments. The highest n-6/n-3 ratio was noted in fish receiving a 100% GO (100 GO) diet, whereas the lowest value was recorded from fish receiving 100% FO diet (0 GO).
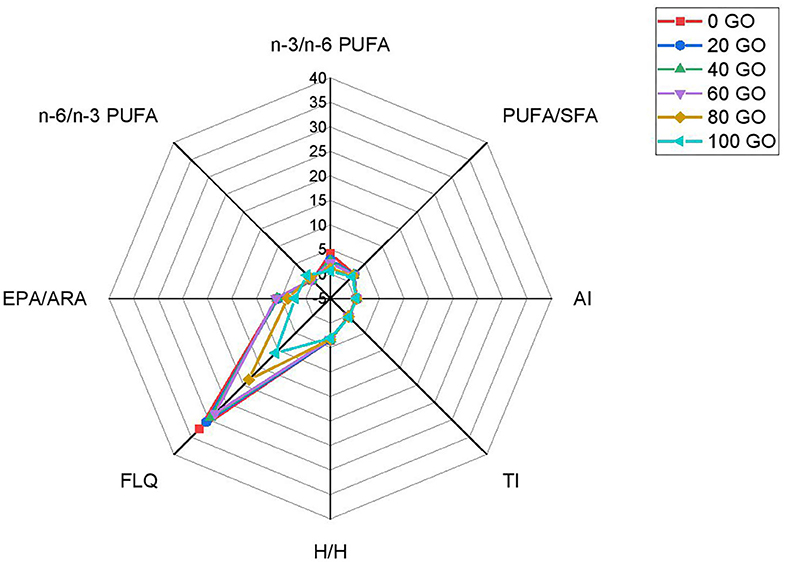
Figure 2. Radar plot of the indices of nutrition quality of filet fatty acids of rohu fingerling fed on different experimental diets. Values are the mean of triplicate groups of fish.
Discussion
Due to the limited availability and escalating cost, the search for FO alternatives is presently the main priority in the aquafeed industry. The effectiveness of dietary lipids in promoting the growth and filet quality relies upon the content and type of fatty acids present in the dietary lipid rather than the amount of lipid used in the diet. Herbivorous or omnivorous freshwater fish can convert ALA and LA into AA, EPA, and DHA (Sargent et al., 2002), but this activity can differ among fish species due to the variation in the ability to desaturase and elongase the fatty acid chains. Growth performances are used to evaluate the effect of the nutrient in the diet (NRC, 2011). In this study, the replacement of FO with GO in the diets had no adverse impact on the growth performance of L. rohita fingerlings. However, the significant differences in filet lipid quality of fish fed diets replacing FO more than 60% were recorded, thus indicating that FO could be replaced by GO in diets of L. rohita fingerlings, either partially or totally. Previous studies where dietary FO replacement by different VOs did not affect the growth performance of freshwater fish include catla, C. catla (Priya et al., 2005), Nile tilapia, O. niloticus (Ochang, 2012; Ayisi et al., 2018), rohu, L. rohita (Karanth et al., 2009), common carp, C. carpio (Ren et al., 2012), freshwater catfish, H. longifilis (Babalola and Apata, 2012), pikeperch, S. lucioperca (Kowalska et al., 2010), darkbarbel catfish, Pelteobagrus vachelli (Jiang et al., 2013), Mozambique tilapia, O. mossambicus (Demir et al., 2014), gibel carp, Carassius auratus gibelio (Zhou et al., 2016), and mandarin fish, Siniperca scherzeri (Sankian et al., 2019). This is because once the requirement of essential fatty acids is satisfied, a significant proportion of FO is spared by VOs without hampering the growth, feed consumption, and utilization efficiency. Studies also show that higher levels of n-6 series of essential fatty acids than n-3 FAs are acceptable for the growth of freshwater fish (NRC, 2011; Erondu et al., 2021). No significant differences in FCR of L. rohita fingerlings fed diets replacing FO with GO at different levels were observed, indicating that fish have a similar capacity for digesting and utilizing both FO and GO.
The whole-body crude lipid and crude protein content is a vital index to evaluate the nutritional quality of fish. In this study, fish fed diets with 80% and 100% FO replacement levels exhibited higher whole-body lipid than fish fed other diets, whereas whole-body crude protein exhibited the reverse pattern. This might have happened due to the presence of excessive SFAs in the above-mentioned diets. This might have decreased the absorption of fatty acids (Morais et al., 2005; Li et al., 2019), resulting in lipid deposition (Leamy et al., 2013). The highest HSI and VSI recorded in the fish fed with the above-mentioned diets point to the fact that the higher concentration of dietary SFA present in those diets resulted in the increase of lipid deposition in the hepatic and visceral regions of fish. This result was also in agreement with findings in African catfish, H. longifilis (Babalola and Apata, 2012), large yellow croaker, Larimichthys crocea (Li et al., 2019), and European sea bass, Dicentrarchus labrax (Dernekbasi et al., 2020), where inclusion of dietary VOs in a higher level resulted in increased lipid deposition in the fish body.
During the normal cellular metabolism, sequential reduction in molecular oxygen generates ROS in animals, resulting in cell and tissue damage as they are carcinogenic, mutagenic, and highly toxic (Nayak et al., 2021). Under normal conditions, a balance between the production and removal of ROS is maintained. However, when an imbalance between the generation and removal of ROS happens, the antioxidant defense mechanism gets stimulated to cope with oxidative stress (Guillou et al., 2010). The antioxidant defense system of fish counteracts free radicals, protecting the cells from oxidative damage. This defense system includes enzymatic antioxidants such as SOD, which accelerates the dismutation rate of superoxide radicals, into oxygen and hydrogen peroxide, H2O2 as the first line of enzymatic antioxidant defense against free radicals, CAT, which catalyzes the reduction of lipid peroxides and H2O2 to molecular oxygen and water, completing the detoxification process imitated by SOD and GPx, which, along with glutathione as a hydrogen donor, reduces all the organic lipid peroxides (Jin et al., 2017), and non-enzymatic antioxidants, such as glutathione and vitamins C and E (Nayak et al., 2021). However, oxidative stress happens when the antioxidant defense system fails to protect cells and tissues from ROS. Lipid peroxidation is the oxidative deterioration of membrane lipids by ROS. Oxidation and breakdown of the fatty acids of membrane lipids that contain more than two methylene interrupted double bonds, resulting in the production of MDA, an important metabolite considered an indicator of oxidative damage caused by ROS (Yuan et al., 2019). In the current experiment, the lowest SOD, CAT, and GPx activities were observed in fish fed a 100% GO diet, whereas the highest was observed in fish fed diet with 100% FO (0 GO), followed by 20, 40, and 60% FO replacement diets. This signifies that feeding rohu fingerlings with GO up to 60% can decrease the peroxidative damage by removing excessive ROS. The decline in SOD and CAT activities (Table 5) is related to the decrease in the dietary n-3 LC-PUFA concentration as evident in Table 2. Serum MDA level was recorded highest in the fish receiving a 100% FO diet. A significant decline in serum MDA level was noted as dietary GO level increased in all the diets indicating a reduced susceptibility of the fish to fatty acid peroxidation. This could also signify that supplementing diets with 100% replacement of FO with GO helps prevent the MDA accumulation effectively, thus suppressing lipid peroxidation.
Lysozyme plays a vital role in non-specific immunity (Zhang et al., 2017). In fish, it is considered to be more important than specific immunity because the latter requires a longer time in specific cellular activations and to produce antibodies. It is distributed widely in the mucus, serum, gill, and intestinal tract mucus of the fish body. Lysozyme has antiviral, anti-inflammatory, and antibacterial activities. Lysozyme is the first line of defense against bacterial pathogens. Fish immunity is regulated by dietary fatty acid composition, including serum lysozyme and alternative complement activity (Yu et al., 2020). In the present experiment, lysozyme activity did not change significantly in diets replacing FO with GO up to 60% and then declined by further replacement of FO with GO at 80 and 100%. Serum lysozyme activity was also found to be unaffected by FO substitution with VOs up to a certain level in gilthead sea bream, Sparus aurata (Montero and Izquierdo, 2010), common carp, C. carpio (Yildirim et al., 2013), Eurasian perch, Perca fluvitalis (Geay et al., 2015), and Nile tilapia, O. niloticus (Ayisi et al., 2018).
The fatty acid composition of cultured fish is directly influenced by the diet (Barriviera et al., 2021; Zhu et al., 2022). The muscle fatty acid profile of rohu fingerlings was significantly affected by the dietary inclusion of GO. Fish receiving a 100% FO diet resulted in the highest EPA and DHA level in fish muscle among all the treatments. However, EPA and DHA levels declined with the replacement of FO in diets. The lowest EPA and DHA level was recorded from fish fed a 100% GO diet. A significantly higher ratio of muscle n-3/n-6 was noted in fish receiving a diet containing 100% FO. Although the replacement of FO with GO lowered the muscle n-3/n-6 ratio, it is still higher than their respective diet levels. This is because freshwater fish can bio-convert C18 PUFA of both n-3 and n-6 to their corresponding LC-PUFA via a series of desaturation and elongation reactions (Karanth et al., 2009; NRC, 2011; Sankian et al., 2019).
The contents of PUFA/SFA and H/H ratios and AI and TI values were used as tools to evaluate the nutritional quality of meat (Chen and Liu, 2020). In relation to human health, an n-6/n-3 PUFA ratio lower than 4 and a PUFA/SFA ratio higher than 0.45 (Department of Health Social Security, Diet and Cardiovascular Disease, 1984) are recommended. With the decrease in the PUFA/SFA ratio, cholesterol levels in the blood increase, leading to the risk of disease. In this study, the n-6/n-3 ratios were lower than 4 and PUFA/SFA ratios were >0.45 in all the muscle samples, indicating that the muscle of rohu fingerlings met the requirements for healthy human nutrition. The consumption of a balanced n-6/n-3 ratio is important as high levels of n-6 fatty acid lead to health problems, such as coronary artery diseases, obesity, and type 2 diabetes. In contrast, n-3 fatty acids have health benefits in reducing the risk of cardiovascular and Alzheimer's disease and anti-inflammatory properties. The desaturation and elongation of ALA and LA to EPA and ARA, respectively, are mediated by the same enzymes, generating a preference over the substrate with greater availability (Tocher, 2003). Thus, EPA/ARA ratio is a vital filet nutritional indicator. Moreover, EPA and ARA both are precursors of bioactive mediators including eicosanoids, which are the indicators of inflammatory processes, being produced from EPA more efficiently and bearing anti-inflammatory action (Gonçalves et al., 2021). In the present experiment, EPA/ARA ratio remained significantly unchanged up to 60% replacement of FO (60 GO). Further replacement of dietary FO with GO resulted in a significant decrease (P < 0.05) in EPA/ARA ratio (80 GO and 100 GO), indicating that 60% replacement of FO with GO is optimum for rohu fingerlings.
The H/H ratio reflects the influence of fatty acids on cholesterol metabolism. Fish flesh with a higher H/H value is suitable for human consumption (Santos-Silva et al., 2002). Also, the larger the filet lipid quality (FLQ) value, the better it is. AI represents arteriosclerosis, a tendency for clot formation in the blood vessels (Ulbricht and Southgate, 1991). TI characterizes the thrombogenic potential of fatty acids (Ulbricht and Southgate, 1991). It is the relation between pro-thrombogenic fatty acids (C12:0, C14:0, and C16:0 acids) and antithrombogenic fatty acids (MUFAs, n-3, and n-6 FAs). Both the AI and TI indices indicate the potential of platelet aggregation in blood. Therefore, lower indices have positive effects on coronary artery disease prevention. In the present study, no significant changes (P > 0.05) in the AI, TI, and H/H indices were noted among all the treatments (Figure 2) and their values ranged between 0.30 and 0.32, 0.17 and 0.41, and 3.14 and 3.55, respectively. These values are similar to the studies conducted by Linhartová et al. (2018) on some freshwater carp species. Fish fed with a 100% FO diet resulted in the highest FLQ value (32.70 ± 1.02) in fish muscle (Table 6). However, the replacement of FO with GO in diets declined the FLQ value with the lowest value recorded in fish fed diet containing 100% GO (Table 6, Figure 2).
PCA was used to observe the clustering trends of the muscle fatty acid profiles in rohu fingerlings fed with different experimental diets. The bi-plot helped to evaluate the fatty acids responsible for causing the differences among samples. Among the samples, 18:1n-9, 18:2n-6, 20:5n-3, and 22:6 n-3 fatty acids were responsible to cause the differences. The bi-plot showed that diets with the replacement of FO with GO at 20% (20 GO), 40% (40 GO), and 60% (60 GO) levels are positively correlated and clustered together with a diet containing 100% FO (0 GO). However, diets with further replacement of FO with GO at 80% and 100% levels (80 GO and 100 GO) are negatively correlated with a diet containing 100% FO. Also, the bi-plot showed that the presence of 18:2n-6 fatty acids in the muscle of fish fed 80 GO and 100 GO diets might have resulted in increased lipid deposition. Therefore, it can be concluded that FO can be replaced up to 60% (60 GO) in the diet without changing the fatty acid composition in fish muscle.
Conclusion
In conclusion, the present study demonstrates that FO could be replaced with GO without distinct compromise in growth performance and feed utilization in rohu fingerlings. However, the nutritional and health benefits of feeding L. rohita diets with higher GO levels (80 and 100% replacement of FO) are reduced due to the decrease in EPA and DHA levels. Antioxidant capacity and lysozyme activity were improved up to 60% replacement of FO with GO in the diet. Although the AI, TI, and H/H values did not change among all the muscle samples, the highest FLQ and PI values were evident in fish receiving a 100% FO diet. In summary, replacing FO with GO at higher levels than 60% had negative consequences on the nutritional quality of rohu fingerlings. Therefore, FO could be replaced by GO in formulated feeds at a moderate level of 60% without hampering the growth and filet nutritional quality of the final product.
Data Availability Statement
The original contributions presented in the study are included in the article/supplementary material, further inquiries can be directed to the corresponding author.
Ethics Statement
The animal study was reviewed and approved by all the experimental procedures including the animal experimentation were approved by the Institutional Ethical Committee of the Department of Biochemistry, Aligarh Muslim University, Aligarh, India (Registration Number. 714/02/a/CPCSEA).
Author Contributions
MK provided expert assistance and is a scientific advisor for designing this study. He also contributed to the drafting of the article. KS conducted the feeding trial, substantially contributed to the analysis, writing of the manuscript, statistical analysis, and interpretation of the data. All authors contributed to the article and approved the submitted version.
Funding
The financial endorsement provided by the University Grants Commission, India (Grant No. F-15-1/2012), to KS is cordially mentioned.
Conflict of Interest
The authors declare that the research was conducted in the absence of any commercial or financial relationships that could be construed as a potential conflict of interest.
Publisher's Note
All claims expressed in this article are solely those of the authors and do not necessarily represent those of their affiliated organizations, or those of the publisher, the editors and the reviewers. Any product that may be evaluated in this article, or claim that may be made by its manufacturer, is not guaranteed or endorsed by the publisher.
Acknowledgments
We are appreciative of the Chairperson of the Department of Zoology, Aligarh Muslim University, Aligarh, for permitting the use of certain laboratory facilities. We also express our gratitude to Dr. Ajay Kumar, in-charge of GCMS, Advanced Instrumentation Research Facility, Jawaharlal Nehru University, New Delhi 110067, for letting us carry out the analysis of fatty acid profile of the samples.
References
Abdel-Hameid, N. A. H., Zehra, S., and Khan, M. A. (2017). Dietary copper requirement of fingerling Channa punctatus (Bloch) based on growth, feed conversion, blood parameters and whole body copper concentration. Aquac. Res. 48, 2787–2797. doi: 10.1111/are.13112
Abidi, S. F., and Khan, M. A. (2004). Dietary histidine requirement of fingerling Indian major carp, Labeo rohita (hamilton). Isr. J. Aquac. Bamid. 56, 200–208. doi: 10.46989/001c.20380
Abidi, S. F., and Khan, M. A. (2007). Dietary leucine requirement of fingerling Indian major carp, Labeo rohita (Hamilton). Aquac. Res. 38, 478–486. doi: 10.1111/j.1365-2109.2007.01687.x
Abidi, S. F., and Khan, M. A. (2010). Growth, protein retention and body composition of fingerling Indian major carp, rohu, Labeo rohita (Hamilton), fed diets with various levels of lysine. J. World Aquac. Soc. 41, 791–799. doi: 10.1111/j.1749-7345.2010.00421.x
Abidi, S. F., and Khan, M. A. (2011). Total Sulphur amino acid requirement and cystine replacement value for fingerling rohu, Labeo rohita: effects on growth, nutrient retention and body composition. Aquac. Nutr. 17, e583–e594. doi: 10.1111/j.1365-2095.2010.00799.x
Abrami, G., Natiello, F., Bronzi, P., McKenzie, D., Bolis, L., and Agradi, E. (1992). A comparison of highly unsaturated fatty acid levels in wild and farmed eels (Anguilla anguilla). Comp. Biochem. Physiol. Part B. 101, 79–81. doi: 10.1016/0305-0491(92)90161-J
Acar, Ü., and Türker, A. (2018). Response of Rainbow trout (Oncorhynchus mykiss) to unrefined peanut oil diets: Effect on growth performance, fish health and fillet fatty acid composition. Aquac. Nutr. 24, 292–299. doi: 10.1111/anu.12559
An, W., Dong, X., Tan, B., Wu, M., Zhang, S., Chi, S., et al. (2020). Effects of dietary vegetable oil on growth performance, digestive capacity, antioxidant capacity and expression of immune-related genes in the hybrid grouper (Epinephelus fuscoguttatus female x E. lanceolatus male). Aquac. Nutr. 26, 1–16. doi: 10.1111/anu.13149
AOAC. (2015). Official Methods of Analysis of Official Analytical Chemists. 18th ed. Arlington VA: Association of Official Analytical Chemists Inc.
APHA. (1992). Standard Methods for the Examination of Water and Waste-Water. 18th ed. Washington DC: American Public Health Association. p. 1268.
Asdari, R., Aliyu-Palko, M., Hashim, R., and Ramachandran, S. (2011). Effects of different dietary lipid sources in the diet for Pangasius hypophthalmus juvenile on growth performance, nutrient utilization, body indices and muscle and liver fatty acid composition. Aquac. Nutr. 17, 44–53. doi: 10.1111/j.1365-2095.2009.00705.x
Ayisi, C. L., Zhao, J. I., and Apraku, A. (2019). Consequences of replacing fish oil with vegetable oils in fish. J. Anim. Res. Nutr. 4, 1–3.
Ayisi, C. L., Zhao, J. L., Apraku, A., and Hua, X. M. (2018). Replacing fish oil with palm oil: Effects on mRNA expression of fatty acid transport genes and signalling factors related to lipid metabolism in Nile tilapia (Oreochromis niloticus). Aquac. Nutr. 24, 1822–1833. doi: 10.1111/anu.12821
Babalola, T. O., and Apata, D. F. (2012). Effect of dietary palm oil on growth and carcass composition of Heterobranchus longifilis fingerlings. J. Cent. Eur. Agric. 13, 782–791. doi: 10.5513/JCEA01/13.4.1130
Barriviera, V.R., Tsujii, K.M., dos Santos, L.D., Furuya, L.B., Panaczevicz, P.A.P., Miranda, J.A.G., et al. (2021). Substitution of soybean oil with linseed oil on growth performance, fatty acid profile and texture attributes in large Nile tilapia, Oreochromis niloticus reared under cold suboptimal temperature. Aquac. Res. 52, 5136–5145. doi: 10.1111/are.15381
Buege, J. A., and Aust, S. D. (1978). Microsomal lipid peroxidation. Methods Enzymol. 52, 302–310. doi: 10.1016/S0076-6879(78)52032-6
Chen, J., and Liu, H. (2020). Nutritional indices for assessing fatty acids: a mini-review. Int. J. Mol. Sci. 21, 5695. doi: 10.3390/ijms21165695
Debnath, D., Pal, A. K., Sahu, N. P., Yengkokpam, S., and Baruah, K. (2012). Protein requirement of Labeo rohita fingerlings based on growth and ammonia excretion. J. Inland Fish. Soc. India. 44, 12–20.
Demir, O., Türker, A., Acar, Ü., and Kesbiç, O. S. (2014). Effects of dietary fish oil replacement by unrefined peanut oil on the growth, serum biochemical and hematological parameters of Mozambique tilapia juveniles (Oreochromis mossambicus). Turk. J. Fish. Aquacult. Sci. 14, 887–892.
Department of Health and Social Security Diet and Cardiovascular Disease. (1984). in Report on health and social subjects. London: HMSO. Vol. 28, p. 443–456.
DernekbasI, S, Karayücel, I., Karatas, E., and Akyüz, A. P. (2020). Potential of using peanut oil as alternative to fish oil for European Seabass diets (Dicentrarchus Labrax) in recirculated systems. Alinteri J. Agr. Sci. 36, 109–121. doi: 10.47059/alinteri/V36I1/AJAS21017
DoF. (2020). Hand Book on Fisheries Statistics. New Delhi: Department of Fisheries, Ministry of Fisheries, Animal Husbandry and Dairying, Government of India.
Erondu, E. S., Akpoilih, B. U., and John, F. S. (2021). Total replacement of dietary fish oil with vegetable lipid sources influenced growth performance, whole body composition, and protein retention in Nile tilapia (Oreochromis niloticus) fingerlings. J. Appl. Aquac. 1–20. doi: 10.1080/10454438.2021.1960230
FAO (2020). The State of World Fisheries and Aquaculture. Rome, Italy: Sustainability in Action. p. ca9229en.
Geay, F., Mellery, J., Tinti, E., Douxfils, J., Larondelle, Y., Mandiki, S. N. M., et al. (2015). Effects of dietary linseed oil on innate immune system of Eurasian perch and disease resistance after exposure to Aeromonas salmonicida achromogen. Fish Shellfish Immunol. 47, 782–796. doi: 10.1016/j.fsi.2015.10.021
Golden, C. D., Koehn, J. Z., Shepon, A., Passarelli, S., Free, C. M., Daniel, F. V., et al. (2021). Aquatic foods to nourish nations. Nature 598, 315–320. doi: 10.1038/s41586-021-03917-1
Gonçalves, L. U., Cortegano, C. A. A., Lorenz, E. K., Cyrino, J. E. P., and Barone, R. S. C. (2021). Effects of dietary linolenic acid to linoleic acid ratio on growth performance, proximate composition and fatty acid contents of pacu (Piaractus mesopotamicus). Aquac. Res. 52, 6667–77. doi: 10.1111/are.15536
Guillou, H., Zadravec, D., Martin, P. G., and Jacobsson, A. (2010). The key roles of elongases and desaturases in mammalian fatty acid metabolism: insights from transgenic mice. Prog. Lipid Res. 49, 186–199. doi: 10.1016/j.plipres.2009.12.002
Guo, H., Chen, C., Yan, X., Li, Y., Wen, X., You, C., et al. (2021). Effects of different dietary oil sources on growth performance, antioxidant capacity and lipid deposition of juvenile golden pompano Trachinotus ovatus. Aquaculture 530, 735923 doi: 10.1016/j.aquaculture.2020.735923
Halver, J. E. (2002). The Vitamins. In: Halver, J.E., Hardy, R.W. (Eds.). Fish Nutrition, 3rd ed. San Diego, CA: Academic Press. p. 61–141. doi: 10.1016/B978-012319652-1/50003-3
Hultmark, D., Steiner, H., Rasmuson, T., and Boman, H. G. (1980). Insect immunity, purification and properties of three inducible bactericidal proteins from hemolymph of immunized pupae of Hyalophora cecropia. Eur. J. biochem. 106, 7–16. doi: 10.1111/j.1432-1033.1980.tb05991.x
Inayat, M., Abbas, F., Razzaq, S., Hafeez-ur-Rehman, M., Javid, A., Hussain, A., et al. (2020). Effect of Vitamin D on the Growth, Haematological and Bio-Chemical Profile of Labeo rohita. Agric. Sci. Digest. 40, 418–423. doi: 10.18805/ag.D-226
Jauncey, K. (1982). The effects of varying dietary protein level on the growth, food conversion, protein utilization and body composition of juvenile tilapias (Sarotherodon mossambicus). Aquaculture 27, 43–54. doi: 10.1016/0044-8486(82)90108-9
Jiang, X., Chen, L., Qin, J., Qin, C., Jiang, H., and Li, E. (2013). Effects of dietary soybean oil inclusion to replace fish oil on growth, muscle fatty acid composition, and immune responses of juvenile darkbarbel catfish, Pelteobagrus vachelli. Afr. J. Agric. Res. 8, 1492–1499. doi: 10.5897/AJAR12.156
Jin, M., Yuan, Y., Lu, Y., Ma, H., Sun, P., Li, Y., et al. (2017). Regulation of growth, tissue fatty acid composition, biochemical parameters and lipid related genes expression by different dietary lipid sources in juvenile black seabream, Acanthopagrus schlegelii. Aquaculture 479, 25–37. doi: 10.1016/j.aquaculture.2017.05.017
Karanth, S., Sharma, P., Pal, A. K., and Venkateshwarlu, G. (2009). Effect of different vegetable oils on growth and fatty acid profile of Rohu (Labeo rohita, Hamilton); Evaluation of a return fish oil diet to restore human cardio-protective fatty acids. Asian-Aust. J. Anim. Sci. 22, 565–575. doi: 10.5713/ajas.2009.80301
Kesbiç, O. S., Acar, Ü., Yigit, M., Bulut, M., Gültepe, N., and Yilmaz, S. (2016). Unrefined Peanut oil as a Lipid source in diets for juveniles of two-banded Seabream Diplodus vulgaris N. Am. J. Aquac. 78, 64–71. doi: 10.1080/15222055.2015.1095829
Kowalska, A., Zakes, Z., Jankowska, B., and Siwicki, A. (2010). Impact of diets with vegetable oils on the growth, histological structure of internal organs, biochemical blood parameters and proximate composition of pikeperch Sander lucioperca (L.). Aquaculture 301, 69–71. doi: 10.1016/j.aquaculture.2010.01.028
Leamy, A. K., Egnatchik, R. A., and Young, J. D. (2013). Molecular mechanisms and the role of saturated fatty acids in the progression of non-alcoholic fatty liver disease. Prog. Lipid Res. 52, 65–174. doi: 10.1016/j.plipres.2012.10.004
Li, T. X., Ji, R., Cui, K., Chen, Q., Chen, Q., Fang, W., et al. (2019). High percentage of dietary palm oil suppressed growth and antioxidant capacity and induced the inflammation by activation of TLR-NF-κB signaling pathway in large yellow croaker (Larimichthys crocea). Fish Shellfish Immunol. 87, 600–608. doi: 10.1016/j.fsi.2019.01.055
Li, Y., Zhao, Y. T., Zhang, Y. K., Liang, X., Zhang, Y., and Gao, J. (2015). Growth performance, fatty acid composition, peroxisome proliferator-activated receptors gene expressions, and antioxidant abilities of blunt snout bream, Megalobrama amblycephala, fingerlings fed different dietary oil sources. J. World Aquacult. Soc. 46, 395–408. doi: 10.1111/jwas.12203
Liao, Z., Sun, Z., Bi, Q., Gong, Q., Sun, B., Wei, Y., et al. (2021). Application of the fish oil-finishing strategy in a lean marine teleost, tiger puffer (Takifugu rubripes). Aquaculture 534, 736306. doi: 10.1016/j.aquaculture.2020.736306
Linhartová, Z., Krejsa, J., Zajíc, T., Másílko, J., Sampels, S., and Mráz, J. (2018). Proximate and fatty acid composition of 13 important freshwater fish species in central Europe. Aquac Int. 26, 695–711. doi: 10.1007/s10499-018-0243-5
Metcafe, L. D., Schmitz, A. A., and Pelka, J. R. (1966). Rapid preparation of fatty acid esters from lipids for gas chromatographic analysis. Anal. Chem. 38, 514–515. doi: 10.1021/ac60235a044
Mir, I. N., Sahu, N. P., Pal, A. K., and Makesh, M. (2017). Synergistic effect of L-methionine and fucoidan rich extract in eliciting growth and non-specific immune response of Labeo rohita fingerlings against Aeromonas hydrophila. Aquaculture 479, 396-403. doi: 10.1016/j.aquaculture.2017.06.001
Misra, H. P., and Fridovich, I. (1972). The role of superoxide anion in the antioxidation of epinephrine and a simple assay for superoxide dismutase. J. Biol. Chem. 217, 3170–3175. doi: 10.1016/S0021-9258(19)45228-9
Monroig, Ó., Li, Y., and Tocher, D. R. (2011). Delta-8 desaturation activity varies among fatty acyl desaturases of teleost fish: high activity in delta-6 desaturases of marine species. Comp. Biochem. Physiol. B. 159, 206–213. doi: 10.1016/j.cbpb.2011.04.007
Montero, D., and Izquierdo, M. S. (2010). “Welfare and health of fish fed vegetable oils as alternative lipid sources to fish oil”, in: Fish Oil Replacement and Alternative Lipid Sources in Aquaculture Feeds. Florida, USA: CRC Press, Taylor and Francis Group, Boca Raton. p. 439–485. doi: 10.1201/9781439808634-c14
Morais, S., Koven, W., Rønnestad, I., Dinis, M. T., and Conceição, L. E. (2005). Dietary protein: lipid ratio and lipid nature affects fatty acid absorption and metabolism in a teleost larva. Br. J. Nutr. 93, 813–820. doi: 10.1079/BJN20051378
Mourente, G., Good, J., and Bell, J. (2005). Partial substitution of fish oil with rapeseed, linseed and olive oils in diets for European sea bass (Dicentrarchus labrax L.): Effects on flesh fatty acid composition, plasma prostaglandins E2 and F2α, immune function and effectiveness of a fish oil finishing diet. Aquac. Nutr. 11, 25–40. doi: 10.1111/j.1365-2095.2004.00320.x
Musharraf, M., and Khan, M. A. (2018). Dietary phosphorus requirement of fingerling Indian major carp, Labeo rohita (Hamilton). J. World Aquacult. Soc. Aquaculture 496, 96–104. doi: 10.1016/j.aquaculture.2018.07.013
Musharraf, M., and Khan, M. A. (2019a). Dietary zinc requirement of fingerling Indian major carp, Labeo rohita (Hamilton). Aquaculture 503, 489–498. doi: 10.1016/j.aquaculture.2019.01.039
Musharraf, M., and Khan, M. A. (2019b). Requirement of fingerling Indian major carp, Labeo rohita (Hamilton) for dietary iron based on growth, whole body composition, haematological parameters, tissue iron concentration and serum antioxidant status. Aquaculture 504, 148–157. doi: 10.1016/j.aquaculture.2019.02.002
Musharraf, M., and Khan, M. A. (2021). Dietary manganese requirement of fingerling Indian major carp, Labeo rohita (Hamilton) estimated by growth, tissue manganese concentration and hepatic manganese-superoxide dismutase activity. Aquaculture 736734. doi: 10.1016/j.aquaculture.2021.736734
Nayak, S., Dash, S. N., Patnaik, L., Pati, S. S., and Priyadarshini, P. (2021). Lipid peroxidation and antioxidant levels in Anabas testudineus (Bloch) under naphthalene (PAH) stress. Aquac. Res. 52, 5739–5749. doi: 10.1111/are.15450
NRC (2011). Nutrient Requirements of Fish and Shrimp. Washington DC: National Academies Press. p. 376.
Ochang, S. N. (2012). Substitution of fish oil with groundnut oil in diets of the African Catfish, Clarias gariepinus: Effects on growth, body composition, haematology and sensory quality. J. Eng. Sci. Technol. 1, 10–18.
Priya, K., Pal, A. K., Sahu, N. P., and Mukherjee, S. C. (2005). Effect of dietary lipid sources on growth, enzyme activities and immuno-hematological parameters in Catla catla fingerlings. Asian-Aust. J. Anim. Sci. 18(11), 1609-1616. doi: 10.5713/ajas.2005.1609
Rahman, M. M., Rahman, R., and Chowdhury, M. M. (2018). Evaluation of the effects of dietary Vitamin C, E and Zinc supplementation on growth performances and survival rate of Rohu, Labeo rohita (Hamilton, 1822). IOSR-JAVS. 11, 68–74.
Rasal, K. D., and Sundaray, J. K. (2020). Status of genetic and genomic approaches for delineating biological information and improving aquaculture production of farmed rohu, Labeo rohita (Ham, 1822). Rev. Aquac. 12, 2466–2480. doi: 10.1111/raq.12444
Regost, C., Arzel, J., Robin, J., Rosenlund, G., and Kaushik, S. J. (2003). Total replacement of fish oil by soybean or linseed oil with a return to fish oil in turbot (Psetta maxima): 1. Growth performance, flesh fatty acid profile, and lipid metabolism. Aquaculture 217, 465–482. doi: 10.1016/S0044-8486(02)00259-4
Ren, H. T., Yu, J. H., Xu, P., and Tang, Y. K. (2012). Influence of dietary fatty acids on muscle fatty acid composition and expression levels of Δ6 desaturase-like and Elovl5-like elongase in common carp (Cyprinus carpio var. Jian). Comp. Biochem. Physiol. B. 163, 184–192. doi: 10.1016/j.cbpb.2012.05.016
Ren, H. T., Zhang, G. Q., Huang, Y., and Gao, X. C. (2020). Effects of different dietary lipid sources on fatty acid composition and gene expression in common carp. Czech J. Anim. Sci. 65, 51–57. doi: 10.17221/248/2019-CJAS
Rotruck, J. T., Pope, A. L., Ganther, H. E., Swanson, A. B., Hafeman, D. G., and Hoekstra, W. G. (1973). Selenium: biochemical role as a component of glutathione peroxidase. Science 179, 588–590. doi: 10.1126/science.179.4073.588
Sagne, M., Loum, A., Fall, J., Ndong, D., Diouf, M., Sarr, A., et al. (2013). Effects of different types of oils on growth performance, survival and carcass composition of Nile tilapia (Oreochromis niloticus). J. Biol. Life Sci. 4, 1–12. doi: 10.5296/jbls.v4i2.3041
Sankian, Z., Khosravi, S., Kim, Y. O., and Lee, S. M. (2019). Total replacement of dietary fish oil with alternative lipid sources in a practical diet for mandarin fish, Siniperca scherzeri, juveniles. Fish Aquat. Sci. 22, 8. doi: 10.1186/s41240-019-0123-6
Santos-Silva, J., Bessa, R. J. B., and Santos-Silva, F. (2002). Effect of genotype, feeding system and slaughter weight on the quality of light lambs. II. Fatty acid composition of meat. Livest. Prod. Sci. 77, 187–194. doi: 10.1016/S0301-6226(02)00059-3
Sargent, J. R., Tocher, D. R., and Bell, J. G. (2002). The lipids. In: Halver, J.E., Hardy, R.W. (Eds.), Fish Nutrition. Elsevier, San Diego: Academic Press. p. 181–257. doi: 10.1016/B978-012319652-1/50005-7
Shahbandeh, M. (2021). Peanut oil: global production volume 2012/13-2020/21. Available online at: https://www.statista.com/statistics/613483/peanut-oil-production-volume worldwide/#statistic Container (accessed January 22, 2022).
Siddiqua, K. S., and Khan, M. A. (2022). Effects of dietary lipid levels on growth, feed utilization, RNA/DNA ratio, digestive tract enzyme activity, non-specific immune response and optimum inclusion in feeds for fingerlings of rohu, Labeo rohita (Hamilton). Aquaculture 554, 738114. doi: 10.1016/j.aquaculture.2022.738114
SOFIA. FAO. (2020). The State of World Fisheries and Aquaculture. In Sustainability in action. Rome, Italy. p. ca9229en.
Surasani, V. K. R., Raju, C. V., Sofi, F. R., and Shafiq, U. (2021). Utilization of protein isolates from rohu (Labeo rohita) processing waste through incorporation into fish sausages; quality evaluation of the resultant paste and end product. J. Sci. Food Agric. 102, 1263–1270. doi: 10.1002/jsfa.11464
Takahara, S., Hamilton, B. H., Nell, J. V., Kobra, T. Y., Ogawa, Y., and Nishimura, E. T. (1960). Hypocatalasemia: a new genetic carried state. J. Clin. Investi. 29, 610–619. doi: 10.1172/JCI104075
Tandel, R. S., Sharma, A., Dash, P., Bhat, R. A. H., and Deo, A. (2019). Dietary vitamin C supplementation on growth and haemato-biochemical parameters of Labeo rohita (Ham.) fingerlings. J. Entomol. Zool. Stud. 7, 72–79.
Tocher, D. R. (2003). Metabolism and functions of lipids and fatty acids in teleost fish. Rev. Fish. Sci. Aquac. 11, 107–184. doi: 10.1080/713610925
Tocher, D. R., Fonseca-Madrigal, J., Dick, J. R., Ng, W., Bell, J. G., and Campbell, P. J. (2004). Effects of water temperature and diet containing palm oil on fatty acid desaturation and oxidation in hepatocytes and intestinal enterocytes of rainbow trout (Onchorhynchus mykiss). Comp. Biochem. Physiol. B. 137, 49–63. doi: 10.1016/j.cbpc.2003.10.002
Ulbricht, T. L. V., and Southgate, D. A. T. (1991). Coronary heart disease: Seven dietary factors. Lancet 338, 985–992. doi: 10.1016/0140-6736(91)91846-M
USDA. (2022). Oilseeds: World Markets and Trade In: United States Department of Agriculture Foreign Agricultural Service. Available online at: https://public.govdelivery.com/accounts/USDAFAS/subscriber/new (accessed January 20, 2022).
Wang, L. G., Li, E. C., Qin, J. G., Du, Z. Y., Yu, N., Kong, Y. Q., et al. (2015). Effect of oxidized fish oil and α-tocopherol on growth, antioxidation status, serum immune enzyme activity and resistance to Aeromonas hydrophila challenge of Chinese mitten crab Eriocheir Sinensis. Aquac. Nutr. 21, 414–424. doi: 10.1111/anu.12171
Wold, S., Esbensen, K., and Geladi, P. (1987). Principal component analysis. Chemometr. Intell. Lab. 2, 37–52. doi: 10.1016/0169-7439(87)80084-9
Xu, H., Bi, Q., Liao, Z., Sun, B., Jia, L., Wei, Y., et al. (2021). Long-term alternate feeding between fish oil and terrestrially sourced oil-based diets mitigated the adverse effects of terrestrially sourced oils on turbot fillet quality. Aquaculture 531, 735974. doi: 10.1016/j.aquaculture.2020.735974
Yildirim, O., Acar, U., Turker, A. A., Sunar, M. C., and Yilmaz, S. (2013). Effects of partial or total replacement of fish oil by unrefined peanut oil on growth and chemical composition of common carp (Cyprinus carpio). Isr. J. Aquac-Bamid. 65, 919–915.
Yu, J., Li, S., Chang, J., Niu, H., Hu, Z., and Han, Y. (2020). Effects of dietary LNA/LA ratios on growth performance, tissue fatty acid composition and immune indices in Manchurian trout, Brachymystax lenok. Aquac. Res. 51, 4495–4506. doi: 10.1111/are.14794
Yu, J. H., Li, S. G., Chang, J., Niu, H. X., Hu, Z. F., and Han, Y. (2019). Effect of variation in the dietary ratio of linseed oil to fish oil on growth, body composition, tissues fatty acid composition, flesh nutritional value and immune indices in Manchurian trout, Brachymystax lenok. Aquacult Nutr. 25, 377–387. doi: 10.1111/anu.12863
Yuan, Y., Wang, X., Jin, M., Sun, P., and Zhou, Q. (2019). Influence of different lipid sources on growth performance, oxidation resistance and fatty acid profiles of juvenile swimming crab, Portunus trituberculatus. Aquaculture 508, 147-158. doi: 10.1016/j.aquaculture.2019.04.068
Zhang, Y., Sun, Z., Wang, A., Ye, C., and Zhu, X. (2017). Effects of dietary protein and lipid levels on growth, body and plasma biochemical composition and selective gene expression in liver of hybrid snakehead (Channa maculata ♀ × Channa argus ♂) fingerlings. Aquaculture 468, 1–9. doi: 10.1016/j.aquaculture.2016.09.052
Zhou, L., Han, D., Zhu, X., Yang, Y., Jin, J., and Xie, S. (2016). Effects of total replacement of fish oil by pork lard or rapeseed oil and recovery by a fish oil finishing diet on growth, health and fish quality of gibel carp (Carassius auratus gibelio). Aquac Res. 47, 2961–2975. doi: 10.1111/are.12748
Zhu, T., Shen, Y., Li, X., Pan, T., Luo, J., Lu, J., et al. (2022). Effects of an alternating linseed oil-fish oil feeding strategy on growth, fatty acid restoration and expression of lipid related genes in black seabream (A. schlegelii). Aquaculture 547, 737456. doi: 10.1016/j.aquaculture.2021.737456
Keywords: growth performance, fatty acid composition, groundnut oil, filet lipid quality, fingerling, Labeo rohita
Citation: Siddiqua KS and Khan MA (2022) Replacement of Fish Oil With Groundnut Oil for Developing Sustainable Feeds for Labeo rohita Fingerling. Front. Sustain. Food Syst. 6:862054. doi: 10.3389/fsufs.2022.862054
Received: 25 January 2022; Accepted: 23 May 2022;
Published: 23 June 2022.
Edited by:
Saleem Mustafa, Universiti Malaysia Sabah, MalaysiaReviewed by:
Dr.Shamna Nazeemashahul, Central Institute of Fisheries Education (ICAR), IndiaDomitila Kyule, Kenya Marine and Fisheries Research Institute, Kenya
Copyright © 2022 Siddiqua and Khan. This is an open-access article distributed under the terms of the Creative Commons Attribution License (CC BY). The use, distribution or reproduction in other forums is permitted, provided the original author(s) and the copyright owner(s) are credited and that the original publication in this journal is cited, in accordance with accepted academic practice. No use, distribution or reproduction is permitted which does not comply with these terms.
*Correspondence: Mukhtar A. Khan, a2hhbm11a2h0YXJAeWFob28uY29t