- 1Department of Agricultural Sciences, Biotechnology and Food Science, Cyprus University of Technology, Limassol, Cyprus
- 2Department of Dairy Science and Quality Management, Faculty of Food Sciences, University of Warmia and Mazury, Olsztyn, Poland
Thermal treatment has always been the processing method of choice for food treatment in order to make it safe for consumption and to extend its shelf life. Over the past years non-thermal processing technologies are gaining momentum and they have been utilized especially as technological advancements have made upscaling and continuous treatment possible. Additionally, non-thermal treatments are usually environmentally friendly and energy-efficient, hence sustainable. On the other hand, challenges exist; initial cost of some non-thermal processes is high, the microbial inactivation needs to be continuously assessed and verified, application to both to solid and liquid foods is not always available, some organoleptic characteristics might be affected. The combination of thermal and non-thermal processing methods that will produce safe foods with minimal effect on nutrients and quality characteristics, while improving the environmental/energy fingerprint might be more plausible.
Introduction
Milk and dairy products are an important and major source of nutritional compounds for consumers (Pereira et al., 2014; Ortega et al., 2019). Food scandals due to bacterial contamination leading to food poisoning incidents, including dairy products, has always been a major concern to the food industry. Although thermal treatment, is nowadays the most common process for producing goods and ensuring food safety, it affects negatively the functionality and the quality of several food components (Bandla et al., 2012; Pal et al., 2015; Liepa et al., 2016; Aydar et al., 2020; Coolbear et al., 2022; Huppertz and Nieuwenhuijse, 2022).
Currently, consumers are more cautious about the foodstuff they purchase. New trends are rising, regarding nutritious products with proven health benefits and produced by environmentally-friendly methods (Knorr et al., 2011; Aydar et al., 2020; Minj and Anand, 2020; Asaithambi et al., 2021). At the same time, the industrial sector is searching for non-invasive processes, regarding the quality aspects and nutritional degradation time, in order to fulfil consumer's demands (Valdramidis and Koutsoumanis, 2016; de Toledo Guimarães et al. Guimarães et al., 2018; Aydar et al., 2020; Chakka et al., 2021).
Consequently, conventional methods using thermal energy are becoming less popular and non-thermal technologies are emerging (Jermann et al., 2015; Valdramidis and Koutsoumanis, 2016; Baboli et al., 2020). Novel non-thermal technologies instead of thermal energy, they require electrical, electromagnetic, light and mechanical forces (Rodriguez-Gonzalez et al., 2015). In general, heat treatment is a less sustainable processing technique compared to novel emerging technologies due to high costs, energy and water consumption (Guimarães et al., 2021). Often, they are defined as the technologies which are effective at ambient and sublethal temperatures (Cullen et al., 2012) without exposing the product directly to heat (Chacha et al., 2021).
Non-thermal technologies have been shown to be efficient for microbial inactivation while preserving the functionality and the nutritional quality (Pereira and Vicente, 2010; Jermann et al., 2015) of milk and milk products (Shrivastava and Banerjee, 2017; Shabbir et al., 2020). Furthermore, de Toledo Guimarães et al. Guimarães et al. (2018), highlights that non-thermal technologies could create opportunities for innovative and nutritious products within the dairy sector.
In order though, for a product to be accepted by the EU legislation as “Novel”, it should comply to the EU Regulation (EU) 2015/2283 of the European Parliament where the products deriving from non-thermal fall under the scope of “novel” of article 3.2a if the product is“(vii)… resulting from a production process not used for food production within the Union before 15 May 1997, which gives rise to significant changes in the composition or structure of a food, affecting its nutritional value, metabolism or level of undesirable substances”. In case the abovementioned is applicable, the company needs to apply for authorization.
The most investigated non-thermal processes during the last decade are: (a) Ultraviolet processing (UV-C), (b) High-Pressure Homogenization (HPH) and High-Pressure Processing (HPP), (c) Cold Plasma, (d) Ultrasound, (e) Pulsed electric fields (PEF) and (f) Membrane Filtration (MF) (see Table 1 and Figure 1).
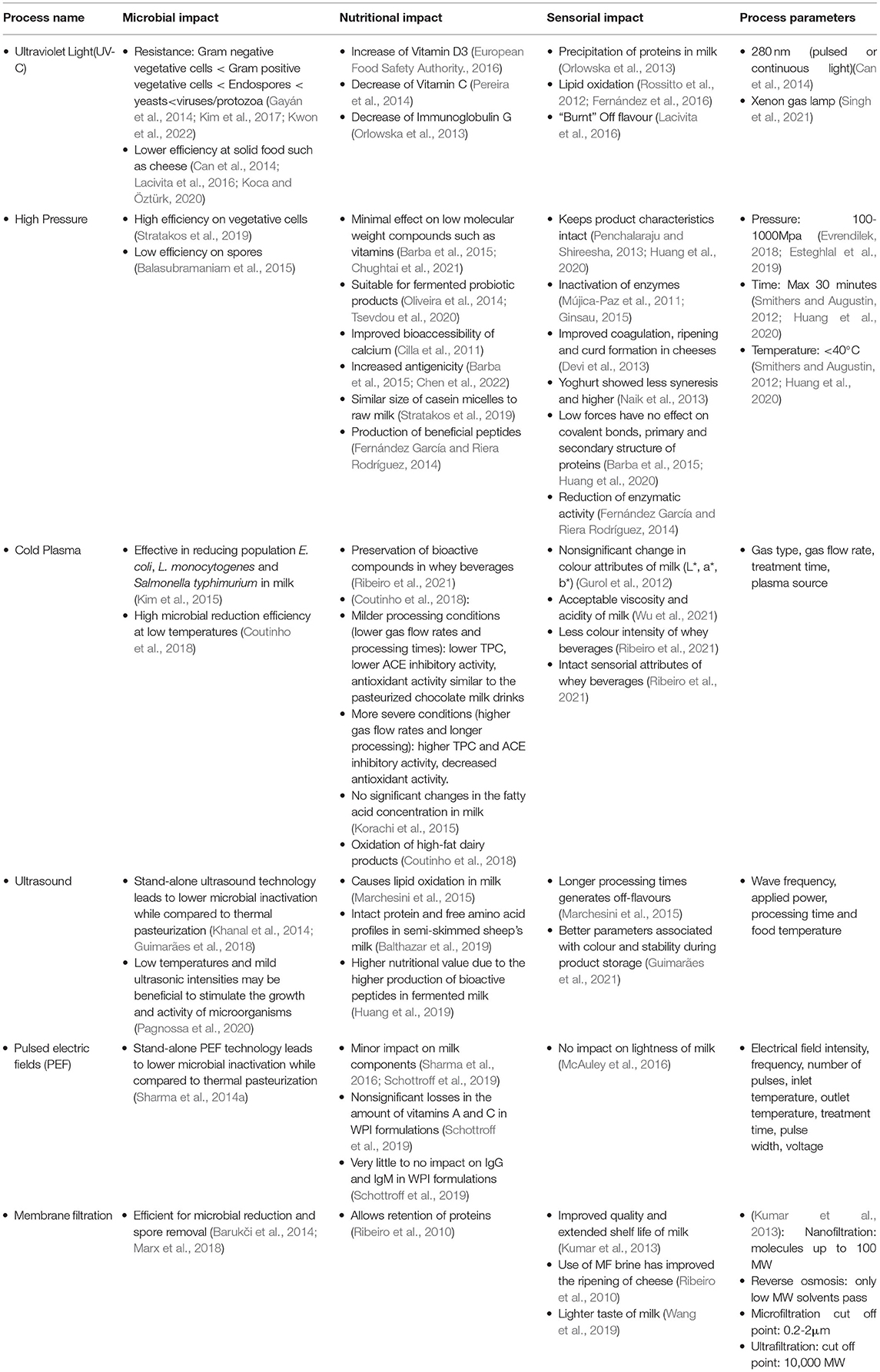
Table 1. The effect of non-thermal technologies on microbial, nutritional and sensorial aspects in dairy products.
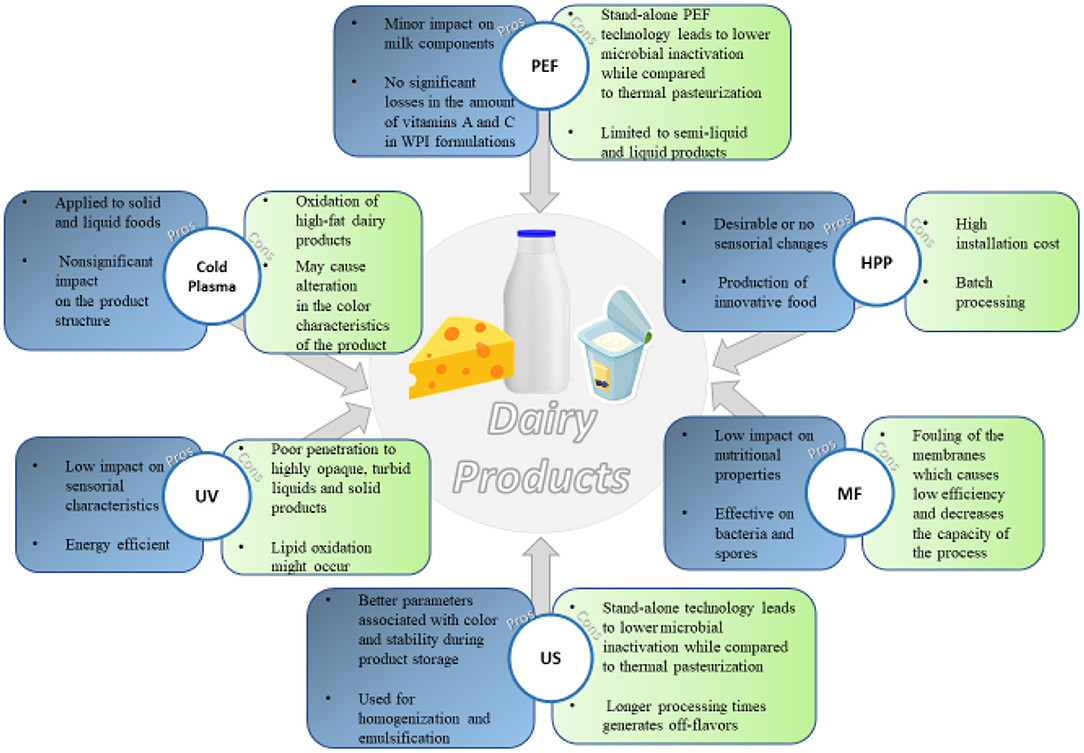
Figure 1. Pros and cons of application of emerging nonthermal processing to dairy products: PEF, pulsed electric fields; HPP, high pressure processing; UV, ultraviolet light; MF, membrane filtration; US, ultrasound.
The aim of this review is to give an overview of the effects on microbial safety, organoleptic characteristics, nutritional quality and functionality, of the abovementioned novel processes. Furthermore, advantages and disadvantages will be discussed and examples of consumer acceptance will be given.
Non-Thermal Processes
Ultraviolet Light
UV light is a non-ionizing irradiation (Beauchamp and Lacroix, 2012), which is consisted of 3 spectrums: UV-A spectrum from 315 to 400 nm, UV-B from 280 to 315 nm and UV-C which ranges from 200 to 280 nm (Shabbir et al., 2020). It is considered as a non-toxic and environmentally friendly technique, which uses physical energy (Delorme et al., 2020). The procedure can run under continuous or pulsed light (Can et al., 2014), where light photons are being absorbed by the product (Rodriguez-Gonzalez et al., 2015).
The mechanism can be photochemical where DNA and RNA chemical changes occur, photothermal in case of long duration of pulses which increases the temperature inactivating the bacterial cells and/or photophysical through damages on the structure of the cells (Can et al., 2014). Different lamps can be used such as mercury lamps and pulsed light (Rodriguez-Gonzalez et al., 2015). Can et al. (2014), mentions that pulsed light treatments are more efficient than the continuous UV light. The pulses from these lamps, are created by the compression of electrical energy delivered by a xenon gas lamp (Can et al., 2014; Singh et al., 2021).
Besides the type of the lamp, the efficiency of the treatment depends on many factors such as the surface of the product (Koca and Öztürk, 2020), the opacity (Müller et al., 2011; Cilliers et al., 2014; Ward et al., 2019) and the turbidity of the liquid (Orlowska et al., 2014). The higher the value of the opacity and the more turbid it is, the less the penetration of the light. Milk for example has high content of colloidal and suspended solids, due to this it is highly turbid and opaque (Shabbir et al., 2020), thereafter the penetration of the light is low and higher doses are required.
Other factors influencing are the intensity of the light, flow patterns, geometry of the equipment (e.g. tube diameter), temperature, time, number of passes, calculation of the doses and the type of microorganisms present (Gayán et al., 2014; Fan et al., 2017; Pendyala et al., 2021). Generally, the pH, water content and the absorbance coefficient of the medium should be taken into account when using a UV-C system (Gayán et al., 2011; Singh et al., 2021).
Besides, the intrinsic factors mentioned above, the growth phase, the conditions of the process, prior stresses and the conditions after the process play major role to the effectiveness (Gayán et al., 2014). Due to the dependence of the efficiency on many parameters the comparison of the data between papers on UV-C technology is very difficult.
Effect on Product Safety
UV-C spectrum has been proven to damage the DNA of microorganisms and prevent their replication (Choudhary and Bandla, 2012; Morales-de la Peña et al., 2019; Ward et al., 2019). Proteins, absorb light around 280nm which disrupts the membrane's integrity (Kim et al., 2017), where others such that 260 nm is the most efficient one because it's the wavelength where DNA absorbs (Beauchamp and Lacroix, 2012).
Once the light is absorbed by the microorganism's DNA, the production of photoproducts begins such as cyclobutane pyrimidine dimers, which prevent replication and stop the transcription, leading to the cell inactivation and death (Gayán et al., 2014). This way, UV-C light is capable of inactivating microorganisms (Rossitto et al., 2012; Pereira et al., 2014; Shin et al., 2016; Dhahir et al., 2021; Papademas et al., 2021). UV-C applied in rose and chocolate milk appeared to be more efficient for microbial reduction, than pasteurization (Kayalvizhi et al., 2021).
The wavelength emission and UV dose are the most important parameters for the microbial inactivation. The wavelength emission depends on the UV lamp used. Both factors are influenced by the strain, species and type of the microorganism (cell thickness). Furthermore, the pH, water activity, absorption coefficient and turbidity affect the efficiency (Gayán et al., 2014). The higher the turbidity and opacity, the lower the effectiveness of the light on microorganisms (Pereira et al., 2014; Ward et al., 2019; Shabbir et al., 2020). There are studies mentioning that in the case of milk, it appears to be less effective, something which is preventing its use on an industrial level (Choudhary and Bandla, 2012; Delorme et al., 2020). Choudhary and Bandla (2012), suggested that a solution to this, is to pass the liquid products as a thin layer.
Vegetative bacteria are the most sensitive to UV-C treatment, yeasts are more resistant and the most resistant ones are viruses and protozoa, depending on the surrounding factors mentioned above (Gayán et al., 2014). Regarding vegetative bacteria, Gram positive bacteria are more resistant towards the UV-C inactivation probable due the outer layer of glycoproteins and liposaccharides (Kim et al., 2017). Beauchamp and Lacroix (2012), showed that Listeria monocytogenes (Gram positive bacteria) was more resistant to UV light than Escherichia coli (Gram negative bacteria).
As for the spore forming bacteria, according to Pendyala et al. (2021), UV-C light is efficient to endospores of Bacillus cereus, but they are more resistant than vegetative cells due to their coating (Gayán et al., 2014; Kwon et al., 2022). Koca and Öztürk (2020), suggested that the microbial survival and growth after the process should be monitored, in case of cell reparation through photoreactivation. The same study expressed, that quantification via plate count is not sufficient, due to presence of viable but not culturable bacteria.
Consequently, for ensuring microbial inactivation and food safety through a UV-C system, there is the need for an indicator showing the efficiency of the process like the alkaline phosphatase for the heat pasteurization (Delorme et al., 2020), shelf life tests should occur and further analysis on damaged cells and their reparation after the process should be done.
Effect on Nutritional Quality and Functional Properties
An example, of beneficial effect deriving from non-thermal technologies, is the UV-C treatment and increasing of vitamin D3. EFSA's opinion on UV-C treated milk mentions that: “UV-treated milk is comparable to non-UV-treated milk, except for the vitamin D3 content”. It was found that cow milk was containing traces of vitamin D3, while UV treated milk contained a significant amount of the specific vitamin (0.5–3.2 μg/100 g in whole milk and 1.5 μg/100 g in semi-skimmed milk) (European Food Safety Authority., 2016). The effect of UV-C treatment on vitamins in milk is depended on the intensity of the light, the initial amount of vitamin present in milk and the number of passages through the system (Guneser and Yuceer, 2012). Using higher fluence could lead to quality loss and nutrient degradation, such a loss of Vitamin C (Orlowska et al., 2013). Pereira et al. (2014) studied the effect of UV-C in colostrum and proved that the treatment led to lower concentration of Immunoglobulin G.
The application to solid foods, such as cheese, can be difficult because of the low penetration. It was shown that after 4 days of extended shelf life, the microbial population of UV-C treated Fiordilatte cheese was the same as the initial one (Lacivita et al., 2016). This could be due to the inactivation of bacteria only at the surface of the cheese (Koca and Öztürk, 2020).
Can et al. (2014), studied the effectiveness of pulsed UV light on packed and unpacked hard American cheeses. The authors concluded that the treatment is efficient for Listeria monocytogenes and Penicillium roqueforti for both packed and unpacked cheeses. Penicillium roqueforti showed higher resistance to UV light. After moderate (30 s at 8 cm) and extreme (60 s at 5 cm) conditions though, lipid peroxidation was observed and the values for a* and b* had changed significantly. Mild conditions (5s at 13cm) provided no differences in color or lipid oxidation for all samples. The centimeters were indicating the distance of the cheese for the UV lamp. Significant differences in a* and b* values were shown also by (Koca and Öztürk, 2020) in kashar fresh cheese although panellists did not identify the colour difference.
Effect on Organoleptic Properties
The organoleptic profile, as the efficiency of the system, is influenced by the equipment used, the dosage, exposure and passages. The lamp of the system for example, plays an important role when treating food with UV-C. Orlowska et al. (2013), showed that there was precipitation of proteins in milk stored at 4°C for 4 days when using HIP lamp (novel mercury-free UV lamp) and polychromatic medium pressure mercury lamps (MPM). At the same study they concluded, that a promising alternative for the mercury lamps are pulsed UV lamps.
Moreover, in Fiordilatte cheese, the sample treated with 6.0 kJ/m2 (highest exposure) was identified as the worst quality and characterized as “burnt”, but had the most extended shelf life (3 days). The odour though was found to be the same for a week and the pH remained stable through time (Lacivita et al., 2016). Some authors expressed their concern about off flavours which may be produced, because of the free radical oxidation which might be initiated (Choudhary and Bandla, 2012; Delorme et al., 2020). Additionally, Rossitto et al. (2012), via a triangle test for UV treated bovine milk, at 880 J/L and 1760 J/L, identified differences in comparison to the control and those differences were mostly associated with lipid oxidation. Panellists reported significant differences in odour and flavour in Manchego and Gouda sliced cheeses treated with UV pulsed light of 4.2 and 8.4 J/cm2 compared to the non-treated samples. Mild treatment (lower than 4 J/cm2) had no effect on the sensory characteristics (Fernández et al., 2016).
The volatile profile analysis in UV-C treated cheese, showed that aldehydes and hydrocarbons were significantly different compared to the control, probably due to lipid oxidation at higher than 4 J/cm2 (Fernández et al., 2016). Amino acids concentrations were significantly different, too (Fernández et al., 2016). Koca and Öztürk (2020), revealed also the off flavours due to the specific compounds and the “burnt” flavour. When fresh kashar cheese was UV treated though, no changes in lipid oxidation, pH value and moisture changes were observed (Keklik et al., 2019). Another study on fresh kashar cheese, observed no significant differences in ash, pH, fat, protein and hardness for fresh kashar cheese, in comparison to the control sample (non-treated) up to 9.63kJ/m2 dosage, except that the peroxide levels in this study, were significantly different in between the samples until 0.96 kJ/m2. The accumulation though, was not stable after that dose. The score in kashar cheese treated with dose and more 1.93 kJ/m2 was decreasing as the dose was increasing while the samples were still acceptable at the highest dose (9.63 kJ/m2) (Koca and Öztürk, 2020).
Sustainability
The specific process is considered as friendly towards the environment and effective in terms of cost (Pendyala et al., 2021). The electrical energy per order (EEO) is usually used to measure the cost efficiency of UV system. The specific factor is affected by the lamp and the geometry of the reactor (Ward et al., 2019). Ward et al. (2019), calculated the energy needed for the inactivation of Escherichia coli, Salmonella typhimurium and Listeria monocytogenes in skim milk and it would require 0.172 kWh energy per m3/log; 0.21 kWh energy per m3/log and 0.196 kWh energy per m3/log respectively.
High Pressure
The specific treatment is mentioned in papers as High Pressure Processing (HPP), High Hydrostatic Pressure (HHP) or Ultra High Pressure Processing (UHPP) (Pal et al., 2015; Rodriguez-Gonzalez et al., 2015; Muntean et al., 2016; Chughtai et al., 2021). It can be applied in a wide range of food products (Ginsau, 2015; Parekh et al., 2017). Some distinguish HPP with HHP mentioning that HPH involves high turbulence, velocity and shear forces, while HPP uses isostatic pressure (Penchalaraju and Shireesha, 2013).
HP is based on hydrostatic pressure which increases the free energy (Heinz and Buckow, 2010; Goyal et al., 2013; Naik et al., 2013) and functions in batches (Huang et al., 2014). The system includes a pressure chamber, pressurizing system and a temperature chamber (supporting unit) (Naik et al., 2013; Pal et al., 2015). It is used on sealed products (closed systems) which are travelling through a vessel containing a liquid medium (Naik et al., 2013; Pal et al., 2015; Huang et al., 2020), usually water, and reaches pressures from 100-1000MPa (Goyal et al., 2013; Shrivastava and Banerjee, 2017; Evrendilek, 2018; Esteghlal et al., 2019; Huang et al., 2020).
The process lasts for maximum 30 min and the chamber temperature is usually kept below 40°C (Smithers and Augustin, 2012; Huang et al., 2020). The parameters defining HPP are: Temperature, Pressure and Exposure Time (Naik et al., 2013; Pal et al., 2015; Liepa et al., 2016, 2018).
In order to commercialize HPP in dairy industries, understanding the effect on proteins in single and mixed systems is a prerequisite (Devi et al., 2013). The principles describing the functionality of the process are mentioned below:
• Le Chatelier's principle (Naik et al., 2013; Barba et al., 2015).
• Microscopic Ordering Principle (Naik et al., 2013; Balasubramaniam et al., 2015).
• Isostatic principle (Naik et al., 2013; Huang et al., 2020).
Effect on Product Safety
The main purpose of the specific process was the homogenization of fat globules, but today it is proven that it can reduce the microbial population of food, while maintaining the sensory characteristics (Smithers and Augustin, 2012). Besides, changes in the conformation of the matrix itself, HPP disrupts the membranes of bacteria leading to the inactivation of pathogens (Martínez-Rodríguez et al., 2012; Huang et al., 2014; Shabbir et al., 2020), depending on the type of microorganism (Penchalaraju and Shireesha, 2013; Huang et al., 2014, 2020). Thereafter, HPP can provide products with extended shelf life and higher quality (Mújica-Paz et al., 2011; Ginsau, 2015).
Pressure of 600MPa for 3 minutes led to more than 5 log reduction for Listeria monocytogenes, Escherichia coli and Salmonella spp. in milk (Stratakos et al., 2019). Moreover, the specific application reduced TVC, Enterobacteriaceae, LAB, and Pseudomonas spp. and prolonged the microbiological shelf life of milk by 7 days compared to pasteurized milk (Stratakos et al., 2019). Even though, the specific process is efficient for microbial reduction, when used alone it has no effect or limited effect on the spores (Mújica-Paz et al., 2011; Penchalaraju and Shireesha, 2013; Balasubramaniam et al., 2015; Ginsau, 2015; Stratakos et al., 2019; Huang et al., 2020), while other studies mention that there are ways to achieve the inactivation of spores (Shao et al., 2010; Gao et al., 2011; Balasubramaniam et al., 2015), depending on the temperature applied (Penchalaraju and Shireesha, 2013). The high resistance of spores to pressure, could be due to the multiple layers and the low water activity of the cortex (Parekh et al., 2017).
Effect on Nutritional Quality and Functional Properties
Authors have proved that HPP preserves product characteristics keeping their original matrix almost intact (Penchalaraju and Shireesha, 2013; Huang et al., 2020). Some believe that this is due to the non-thermal, limited effect on covalent bonds (Penchalaraju and Shireesha, 2013; Huang et al., 2014, 2020; Barba et al., 2015).
The effect of HPP, on low molecular weight molecules such as vitamins and volatile compounds is minimal (Heinz and Buckow, 2010; Naik et al., 2013; Huang et al., 2014; Barba et al., 2015; Pal et al., 2015; Chughtai et al., 2021). It has been shown that HPP could be suitable for certain fermented probiotic products (Tsevdou and Taoukis, 2011; Oliveira et al., 2014; Tsevdou et al., 2020). Cilla et al. (2011), showed that the bio-accessibility of calcium in milk-based beverages treated with HPP was higher than the thermally treated samples.
Further examples, proving the beneficial effects, are the processing of human donor milk using HPP which leads to preservation of adipokines, growth factor, and higher values of lactoferrin, Immunoglobulin (IgG) when compared to pasteurization (62.5°C for 30min) (Wesolowska et al., 2018). Also, it seems that HPP could alter allergies in food such as β-Lactoglobulin in bovine milk by increasing antigenicity (Barba et al., 2015; Chen et al., 2022).
Last but not least, it is s believed that the specific process could improve the functionality of the ingredients in milk such as proteins leading to better foaming and emulsifying properties (Chawla and Patil, 2011; Pal et al., 2015; Bogahawaththa et al., 2018; Esteghlal et al., 2019).
Effect on Organoleptic Properties
Besides microorganisms, HPP can inactivate enzymes (Mújica-Paz et al., 2011; Ginsau, 2015; Chughtai et al., 2021) and as a result, the product can maintain its organoleptic profile during shelf life (Mújica-Paz et al., 2011; Smithers and Augustin, 2012). For example, Alkaline phosphatase is inactivated at 800MPa for 8 minutes (Pal et al., 2015).
Devi et al. (2013), concluded that HPP can evidently improve coagulation, cheese ripening and curd formation in cheeses, while yoghurt showed less syneresis and ice cream was melting slower than the conventional products. Increased firmness was observed in HPP yoghurts which might explain the milder effect of syneresis, which could be related to increased interaction surface (Naik et al., 2013).
Due to the milder effect on the product's components, HPP can extend shelf life. It has been shown to extend the shelf life in cheeses (Evert-Arriagada et al., 2014) and accelerate the ripening procedure by influencing the enzymatic activity and altering the casein micelles (Martínez-Rodríguez et al., 2012; Naik et al., 2013; Shabbir et al., 2020). HPP milk treated casein micelles had similar size to raw milk when compared to pasteurized milk (Stratakos et al., 2019).
Some authors mention that there is no effect on the primary of secondary structure of proteins (Pal et al., 2015; Huang et al., 2020). Whereas contradictory, others suggest, that in milk the disruption depends on the pressure, which in high forces could lead to irreversible denaturation of proteins and disruption of casein micelles up to their primary structure (Goyal et al., 2013; Liepa et al., 2016; Stratakos et al., 2019). Regarding α-lactalbumin and bovine serum albumin, no denaturation was observed in skim milk for pressures up to 400MPa (Anema, 2022).
Research done by Evert-Arriagada et al. (2014), on cheeses deriving from fresh pasteurized cow milk, showed higher modulus values, higher stress and lower strain values compared to the controls which indicates more resistance to deformation, lower fracturability and lower deformability respectively. On the same research, application of 500MPa resulted in increased yellow colour and a decrease on lightness during 7 days storage compared to the controls. Panellists found differences between the controls and the pressurized cheeses only for the firmness (HPP cheeses were firmer), while the preference mean score for fresh cheeses and for a shelf life of 22 days was the same.
Another study showed that the L* value indicating lightness, was higher for pasteurized milk compared to raw and HPP milk, while the L* value of HPP milk was closer to the raw milk. The colour is related to fat globules and in the specific study was shown that the average size of fat globules was smaller in pasteurized milk than in HPP and raw milk (Stratakos et al., 2019).
Sustainability
Products produced by HPP can exceed 10 billion US dollars (Huang et al., 2014). The limitation of HPH at industrial level, is the high cost and low capacity at pressures higher than 100MPa (Smithers and Augustin, 2012). As a high capital investment is needed, while the throughput is limited, leading to higher production costs in comparison to thermal treatments (Penchalaraju and Shireesha, 2013; Huang et al., 2020). Hence, it could be used at smaller scale for high value products (Smithers and Augustin, 2012) and the energy consumption is much lower than thermal process requiring cooling afterwards (Asaithambi et al., 2021). Mújica-Paz et al. (2011), mention that the high investment cost has been overcame by increasing the size of the equipment and the capacity of the vessel used. On the other hand, (Shabbir et al., 2020), mention that the cost per L or Kg is US$0.05–0.5 which is lower than thermal treatment.
Cold Plasma
Among other non-thermal processing technologies, cold plasma is relatively new technique applied in food-based research (Sharma and Singh, 2020). The cold plasma processing is reported to be effective in microbial and enzyme inactivation (Segat et al., 2016). Furthermore, there were several attempts undertaken to evaluate the effect of this technology on quality parameters of dairy products like milk (Korachi et al., 2015), and dairy-based beverages (Coutinho et al., 2019; Ribeiro et al., 2021). However, the impact of cold plasma on the physicochemical properties and retention of bioactive compounds providing health benefits in dairy products has not been extensively studied (Silveira et al., 2019).
Plasma is the fourth state of matter. It is an ionized gas composed of free electrons, ions, atoms and molecules in the ground or excited states. Its nature is quasi-neutral due to the existence of an equal number of negative and positive charges carried by different species (Sharma and Singh, 2020). Based on degree of ionization and the thermodynamic equilibrium between the constituent species of plasma, two plasma classes are distinguished i.e. thermal and nonthermal (cold) plasma (Pankaj et al., 2018). Plasma characterized by highly ionized species, which are in thermodynamic equilibrium with each other is so called thermal plasma. Its temperature reaches up to 104 K and is uniform across all constituent species. Whereas, when the temperature of electrons is very high, but the ions and unionized species are around room temperature, there is thermodynamic nonequilibrium between them, resulting in cold plasma type (Surowsky et al., 2015).
The generation of cold plasma can be achieved using various kinds of apparatus, for example dielectric barrier discharges (DBD), high voltage pulsed discharge, corona glow discharges, gliding arc discharge, plasma jet, radio frequency discharges and atmospheric glow discharges (Sharma and Singh, 2020). Depending on generation technique used, various reactive species are produced, usually from electronically and vibrationally excited nitrogen and oxygen. Moreover, the type of gas used determines the type of reactive species produced. Mostly used gases are nitrogen, hydrogen, oxygen, argon, air and their mixtures. The reactive species react with surfaces resulting in modifications (Annor, 2019). Antimicrobial action of plasma is based on cell membrane damaging/electroporation, followed by leakage of cell constituents, cell shrinkage, dagame of DNA, etching effect and morphological changes (Rathod et al., 2021). The general effectiveness of plasma for inactivation of microorganisms depends on gas type and flow, time of treatment, and electric current intensity. Moreover, the higher the gas flow rate and longer processing times the higher the number of collisions and the possibility of reactive species acting on the microbes, resulting in more efficient decontamination (Silveira et al., 2019).
Effect on Product Safety
The effectiveness of cold plasma on the microbial inactivation of food has been already established. Kim et al. (2015) evaluated the microbial characteristics of milk treated with encapsulated DBD plasma generated in a plastic container (250 W, 15 kHz, ambient air) and applied to milk samples for periods of 5 and 10 min. The total aerobic bacterial count in the untreated sample was 0.98 log CFU/ml. No viable cells were detected in milk sample after plasma processing. Moreover, the encapsulated DBD plasma significantly reduced the number of pathogens (Escherichia coli, Listeria monocytogenes and Salmonella Typhimurium) inoculated in the milk samples. The 10 min treatment resulted in a bacterial counts reduction by 2.40 log CFU/ml (Kim et al., 2015). In another study of Gurol et al. (2012), the time dependent effect of atmospheric corona discharge generated with 9 kV of AC power supply on Escherichia coli (ATCC 25922) dispersed in whole, semi skimmed and skimmed milk was evaluated. Plasma was applied at time intervals of 0, 3, 6, 9, 12, 15 and 20 min. Three minutes of plasma treatment resulted in a significant 54% reduction in the population of E. coli cells regardless the fat content in milk. The initial bacterial population of 7.78 log CFU/ml in whole milk was reduced to 3.63 log CFU/ml after 20 min of plasma processing.
The initial pathogens concentration in food subjected to cold plasma treatment is of great importance, while higher microbe concentration decreases the inactivation effect of this treatment. Most probably, when more microorganisms are present in food sample, they form clusters of cells which decreases the ability of plasma active ingredients to reach each cell (Coutinho et al., 2018).
To measure the effectiveness of pasteurization process in dairy industry, the activity of alkaline phosphatase (ALP) is routinely evaluated. ALP is an enzyme naturally present in milk of mammals and its activity in pasteurized milk is an indication of insufficient pasteurization process. Therefore, ALP would be a good model enzyme for evaluating the efficiency of atmospheric pressure cold plasma against inactivation of enzymes endogenous to milk (Segat et al., 2016). Results of (Segat et al., 2016) demonstrated that 98% ALP inactivation (equally effective as achieved with HTST or LTLT pasteurization treatments), was obtained with atmospheric pressure cold plasma treatment for 300 s (DBD plasma setup). However, the study was conducted in model buffer media, and the results need to be verified in milk samples.
Effect on Nutritional and Functional Properties
The effects of cold plasma technology on nutritional and functional properties of milk and dairy-based beverages were investigated (Korachi et al., 2015; Coutinho et al., 2019; Ribeiro et al., 2021). The study performed by Ribeiro et al. (2021) revealed that cold plasma-treated (a corona discharge plasma, ambient air at ~17°C) whey beverages presented higher concentration of the bioactive compounds (antioxidant activity, and inhibition activity against ACE, α-amylase, and α-glucosidase) than the pasteurized product. The cold plasma exposure time of 15 min was suggested to increase the nutritional value of the prebiotic whey beverages.
According to Coutinho et al. (2019), the cold plasma process parameters (i.e. time and gas flow) have a significant impact on the bioactivity of chocolate milk drinks. The milder processing conditions (lower gas flow rates and processing times) resulted in chocolate milk drinks with lower total phenolic content (TPC) and ACE inhibitory activity while maintaining the antioxidant activity similar to the pasteurized product. Whereas, more severe conditions (higher gas flow rates and longer processing), resulted in higher TPC and ACE inhibitory activity with a decrease in antioxidant activity. Considering the effect of cold plasma on chemical composition of dairy products, Korachi et al. (2015) reported no significant changes in the fatty acid concentration of plasma-processed (corona discharge setup) milk compared to fresh sample. Abovementioned results demonstrate that the selection of the suitable cold plasma parameters allows obtaining dairy-based products with improved nutritional quality when compared to the pasteurized product.
Effect on Organoleptic Properties
Several studies have reported the effect of cold plasma processing on the sensorial properties of dairy products. The analyses of milk samples treated with atmospheric plasma corona discharge system (9 and 20 min of 9 kV plasma treatment) revealed that this treatment does not cause any significant change to the colour values (L*, a*, b*) (Gurol et al., 2012). In another study of Wu et al. (2021), the values of colour, viscosity and acidity of milk showed an acceptable physicochemical property with DBD treatment at 70 V (120 s) and 80 V (120 s). However, in the study of Ribeiro et al. (2021), using cold plasma system (the power source of 15 kV; 0-15 min), the colour intensity of whey beverages was decreased by plasma treatment when compared with the untreated product. Same authors also revealed that cold plasma treatment has no negative influence on sensory characteristics of whey beverages. On the other hand, some sources highlight the limitation of cold plasma processing. Plasma treatment applied to high-fat dairy foods can lead to oxidation. Thus, the sensory characteristics must be evaluated in detail in the future research (Coutinho et al., 2018).
In general, the sensorial attributes of cold plasma-treated foods are well preserved. In some cases, negative effects were seen as well with respect to flavour and aroma. The detrimental effects can be neglected by further optimization and control of experimental conditions. Cold plasma technology has a better control on conditions over thermal processing (Rathod et al., 2021).
Sustainability
The features highlighting the sustainability of plasma processing are on-demand production of the acting agent, the absence of water, solvents and residues. It is also a resource-efficient technology. Plasma treatment is environmentally safe—the active species disappear after the plasma power is turned off (Coutinho et al., 2018). Considering the applicability of cold plasma in food industry, it generates lower costs when compared to thermal treatment (Hernández-Hernández et al., 2019). To lower costs of cold plasma generation, it is important to avoid using costly noble gases. For this reason, plasma sources capable of ionizing air at large gaps will be suitable. Furthermore, plasma sources operating at line frequency rather than radio-frequency power sources could help manage costs. Currently, plasma systems are not commercially available as a decontaminating tool in the food industry and still need further exploration (Coutinho et al., 2018).
Ultrasounds
Ultrasounds refers to sound waves above the frequency of human hearing (> 20 kHz) (Arvanitoyannis et al., 2017). Application of ultrasounds in food processing can be divided according to the wave's frequency ranges, in low frequency (16–100 kHz) and high frequency ultrasound (100 kHz−1 MHz). The high intensity ultrasound (HIUS) is mainly studied for processing of dairy products. Ultrasounds as a standalone technology are not effective in reducing microorganism counts at low temperatures, thus the use of ultrasounds as a non-thermal technology is not a well-studied area of dairy products (Khanal et al., 2014; Guimarães et al., 2018). There are limited studies on application of ultrasounds with no heat treatment (Marchesini et al., 2015) or with controlled low temperature (Gao et al., 2014; Khanal et al., 2014). Increased temperatures and ultrasonic intensities for milk processing may cause changes in sensory and physicochemical properties (Marchesini et al., 2015; Guimarães et al., 2018). The main applications for HIUS in dairy products include the homogenization, emulsification, lactose crystallization, extraction, hydrolysis and microbial inactivation (Guimarães et al., 2018).
The driving force in ultrasound technique in milk is acoustic cavitation caused by the ultrasonic waves passing the liquid milk. The size of cavities becomes large in successive cycles and finally produces acoustic cavitation bubbles. Thousands of bubbles are formed, and a violent collapsing occur in very short time (millisecond scale) by expanding the attractive forces between the molecules in food system (Chandrajith et al., 2018). There are three different mechanism of ultrasound action, namely:
• mechanical damage of the cell wall induced by gradients of pressure generated during the collapse of the cavitation bubbles within or near the cells,
• the micro-streaming inside the cell,
• the formation of chemical compounds during cavitation that interacts with the structure of cell wall causing its disintegration (Hernández-Hernández et al., 2019).
Considering a constant wave frequency, among main factors influencing the efficiency of HIUS, there is treatment intensity, which is a result of the applied power, processing time and food temperature (Guimarães et al., 2021).
Effect on Product Safety
The inactivation of Enterobacter aerogenes in skim milk using low-frequency (20 kHz) and high-frequency (850 kHz) ultrasonication was investigated by Gao et al. (2014). For the low-frequency ultrasound treatment, the log reduction was found to decrease linearly with an increase in sonication time. High-frequency ultrasound was not able to inactivate E. aerogenes in milk even when powers as high as 50 W for 60 min were used. In different study on milk performed by Marchesini et al. (2015), the effect of eight different combinations of ultrasound amplitude (70 and 100%) and duration (50, 100, 200 and 300 s) for their lethal capacity against Escherichia coli, Pseudomonas fluorescens, Staphylococcus aureus and Debaryomyces hansenii was evaluated. The strongest treatment (100% × 300 s) led to a population reduction of 4.61, 2.75, and 2.09 for D. hansenii, P. fluorescens and E. coli. S. aureus was found to be resistant to the strongest treatment, showing only an irrelevant log reduction.
These results suggest that decontamination effect of ultrasounds depends on bacteria type and processing parameters like frequency, power and treatment time. Many authors highlight that the ultrasound technology applied alone, using controlled low temperature, is not enough to effectively reduce the number of microorganisms and ensure the food safety (Guimarães et al., 2021). Moreover, low temperatures and mild ultrasonic intensities may be beneficial to stimulate the growth and activity of microorganisms (Pagnossa et al., 2020). Thus, it is suggested to combine the ultrasounds with heat or pressure, which affect the physicochemical properties of the cell membranes and improve the cavitation effects, enhancing the microbial inactivation (Silva, 2020; Guimarães et al., 2021; Akdeniz and Akalin, 2022). Additionally, the choice of using HIUS to reduce microbial load instead of conventional heating, must be precisely, since inefficient ultrasonic treatments may not inactivate but stimulate spore forming bacteria in dairy products (Lim et al., 2019).
Effect on Nutritional and Functional Properties
Ultrasound applied as a milk processing technology is responsible for the increase in the lipid oxidation in milk (Marchesini et al., 2015). Ultrasonication (20 kHz, 78 and 104 W/4–8 min <69°C) of semi-skimmed sheep's milk does not influence the protein and free amino acid profiles (Balthazar et al., 2019).
Among the reported advantages of ultrasounds applied instead of thermal treatment to reduce the number of microorganisms in multicomponent dairy beverages, there are the concurrent fat homogenization and kinetic stabilization (Guimarães et al., 2019; Monteiro et al., 2020). The application of ultrasounds has been further extended to probiotic food development. Many studies have investigated the application of HIUS in the fermentation of milk and noted an increase in probiotic culture activity and consequent reduction in fermentation time. Its impact is connected with the changes in bacterial probiotic cells treated with HIUS, releasing β-galactosidase, accelerating the transgalactosylation, and lactose hydrolysis fermentation (Huang et al., 2019; Asaithambi et al., 2021). Moreover, as reported by Huang et al. (2019), the treated fermented milk also shows higher nutritional value due to the higher production of bioactive peptides. The pulsed ultrasounds (on-time 100 s and off-time 10s; 28 kHz; 100 W/L/30 min) cause almost 50% increase in peptide content in fermented skim milk.
Currently, there are no ideal ultrasonic powers or in general treatment parameters for each application, since other factors (sample volume, temperature, product type) greatly influence the results. Overall, the technology of ultrasound has been proven as a promising tool for the development of probiotic foods (Asaithambi et al., 2021; Guimarães et al., 2021).
Effect on Organoleptic Properties
Although high intensity ultrasound applied for longer processing times has the potential to reduce (to some extend) microbial load in milk, the treatment may generate off-odours under certain conditions. Riener et al. (2009) reported that extended sonication (acoustic power output of 400 W, frequency 24 kHz, processing time 2.5–20 min, temp. 45°C) of milk could generate volatile organic compounds that may be responsible for a “rubbery” aroma. Authors has related these compounds to both free radical-induced lipid oxidation resulting from the decomposition of unsaturated fatty acid hydroperoxides and to pyrolysis reactions inside the cavitating bubbles. The milk sensorial deterioration due to the formation of off-flavours was also observed by Marchesini et al. (2015) for sonication (constant frequency of 24 kHz, a 400 W ultrasonic processor) applied for longer than 100 s.
On the other hand, the advantage of ultrasound applied to milk are better parameters associated with colour and stability during product storage (Guimarães et al., 2021). Moreover, non-thermal processing by HIUS seems to be an interesting technology for dairy based beverages production, since it presents some additional effects, like better disruption of milk components and decreased viscosity and consistency (Guimarães et al., 2018).
In general, the effects of HIUS on the studied dairy products are difficult to understand, due to the complexity of tested products and varieties of applied parameters. Further studies in respect to the effect of ultrasound on dairy based products must be evaluated before commercialization.
Sustainability
Ultrasound is considered as a green technology (Alves de Aguiar Bernardo et al., 2021). It is reported to meet process requirements as simplicity and scale up (Chemat and Khan, 2011; Kadam et al., 2015; Arvanitoyannis et al., 2017). According to Chemat and Khan (2011), one of the advantages of ultrasound over standard heat treatment are significant energy savings. The use of ultrasound in industry for milk treatment is likely to occur as a continuous flow system (CFS). It is composed of an ice bath, heat exchanger and an ultrasonic reaction cell coupled with two transducers (Alves de Aguiar Bernardo et al., 2021).
Pulsed Electric Fields
The majority of researches on application of PEF in dairy industry is focused on microbial inactivation or shelf-life (Guerrero-Beltrán et al., 2010; Bermúdez-Aguirre et al., 2012; Zhao et al., 2013; Simonis et al., 2019) and recent data concerning the effect of PEF on physicochemical and functional properties of different dairy products is rather scarce. Cow's milk is the material which is most often subjected to PEF-treatment (Soltanzadeh et al., 2020). However, there are some results on how PEF affect other dairy products i.e. whey (Simonis et al., 2019) whey protein formulations (Schottroff et al., 2019) or goat's milk (Mohamad et al., 2020).
Pulsed electric field technology was proven to have a sublethal effect on inactivation of microorganism (Zhao et al., 2013), to influence some enzymes (Sharma et al., 2014b) and to restore (to some extent) the physicochemical and functional characteristics of raw dairy products while compared with thermal treatment (Sharma et al., 2016; Schottroff et al., 2019). To date, the mechanism of PEF-induced inactivation of microorganisms has not been clearly identified. Nevertheless, the commonly accepted and investigated phenomenon involved in the destruction and inactivation of microbes is electroporation of their cell membrane (Buckow et al., 2014). The PEF treatment causes the change in transmembrane potential and initiates the pore formation in the membrane of microbial cell membranes. It results in the cell contents diffusing into their surroundings and may lead to the death of living cells. A typical PEF system is composed of a treatment chamber and a high-voltage pulse generator. A liquid product is pumped into the chamber between two electrodes (high-voltage and a grounded electrode) and subjected to electrical pulses (1-100 μs) of high-voltage electric fields ~15–60 kV/cm applied for few milliseconds (Hernández-Hernández et al., 2019; Alirezalu et al., 2020). When the electric field is applied, current flows into the food sample and is transferred to each point because of the presence of charged molecules (Shabbir et al., 2021). Square wave pulses (monopolar, bipolar) and exponentially decaying pulses are the most common shapes of wave generated during PEF treatment. It is reported, that better lethal effect can be achieved with bipolar square wave pulses which cause a charge reversal across the cell membrane inducing more effective cellular damage (Sharma et al., 2014c). Depending on electric field strength, treatment time and number of pulses the cell damage may be reversible or irreversible. The lethal effect can be achieved only with irreversible electroporation.
Effect on Product Safety
Milk as the potential application target of PEF has been the subject of many research papers (Walkling-Ribeiro et al., 2011; Zhao et al., 2013; Sharma et al., 2014a), where the effect of PEF on common pathogens related with foodborne illnesses was evaluated. It is confirmed that the application of stand-alone PEF technology leads to lower microbial inactivation while compared to thermal pasteurization. The effectiveness of milk treatment with PEF is limited due to the presence of fat and proteins (casein) which can serve as protectors of bacterial cell during treatment (Sharma et al., 2014a).
Walkling-Ribeiro et al. (2011), noted 2.0, 2.1, 2.3, and 2.5 log10 CFU/mL reduction in microbial load of bovine skim milk (inoculated with native milk microorganisms) for respective electric field strength and treatment times of 16 kV/cm for 2105 μs, 20 kV/cm for 1454 μs, 30 kV/cm for 983 μs and 42 kV/cm for 612 μs. This effect was accomplished with monopolar exponential decay pulses with a pulse width of 1.5 μs for milk temperature ranging from 16°C at the inlet to PEF chamber to 40-49°C at the outlet. Samples were collected after cooling PEF-treated milk to 10°C. In comparison with PEF, thermal pasteurization (75°C for 24s) conducted in the same studies, resulted in milk microflora inactivation of 4.6 log10 CFU/ml.
Another studies of Zhao et al. (2013), investigating the effectiveness of square-wave pulses (the pulse repetition rate of 200 Hz and pulse width of 2 μs) at temperature lower than 35°C, proved the occurrence of sublethally injured microbial cells (E. coli, S. aureus and L. monocytogenes) after PEF treatment of inoculated whole milk, indicating that electroporation was not sufficient to effectively reduce number of vegetative bacteria. Moreover, the proportion of sublethally injured microbial cells under stress of PEF depended on the type of bacteria and treatment time. Authors Zhao et al. (2013) observed that L. monocytogenes is the most resistant strain with less than 2 log10 reduction achieved with PEF at 30 kV/cm for 200 μs. Whereas, the same treatment parameters cause around 2.8 log10 cycles reduction of S. aureus and >3 log10 cycles of E. coli. In summary, the Gram-negative cells are more sensitive to PEF. A higher degree of inactivation of same cells can be achieved with PEF treatments (30 kV/cm) applied for time range between 200 and 600 μs with the maximum inactivation level obtained after 600 μs (~5 log10 cycles of reduction for E.coli).
The control of enzymatic activity as mean to preserve milk quality and to extend its shelf-life is of great importance when subjecting milk to PEF treatment. The changes in enzymes induced by PEF may be influenced by factors like enzyme type, its structure, the presence of cofactors or the degree of denaturation. The main effect associated with PEF action is the change in enzyme's conformation (Alirezalu et al., 2020). However, similarly to limited microbial reduction, endogenous milk enzymes (alkaline phosphatase and lactoperoxidase) are nonsignificantly influenced by PEF at low temperatures (Buckow et al., 2014; Sharma et al., 2014b; McAuley et al., 2016).
As shown by scientific reports published in the last decade, the degree of inactivation obtained during PEF treatment of milk must be improved to achieve higher bacterial reduction, enzyme inactivation and greater efficiency compared to thermal pasteurization. Some issues still need to be optimized and resolved before PEF technology can be transferred to commercial scale.
Effect on Nutritional and Functional Properties
Pulsed electric fields technology has a minor impact on food components, thus PEF-treated products retain a more fresh-like character with higher concentration of valuable compounds. Considering vitamins, there are no significant losses in the amount of vitamin A and vitamin C in 2 % whey protein isolate (WPI) formulations subjected to PEF processing (32 kV/cm, pulse width of 3 μs, inlet temperature <40°C, pH 4–7). Additionally, HPLC elution profile indicated that these vitamins do not undergo chemical modifications during the treatment with PEF (Schottroff et al., 2019). As revealed in the same study, PEF treatment has also very little to no impact on the concentration of immunoglobulins (IgM and IgG) present in WPI formulations. Another research study by Mohamad et al. (2020) concerning changes induced by PEF (20–40 kV/cm, monopolar square wave pulses of 5 and 10 μs treatment time, pulse width of 8 μs) in goat milk showed reduction in the total saturated fatty acids (ΣSFAs) and total polyunsaturated fatty acids (ΣPUFAs) and increase in total monounsaturated fatty acids (ΣMUFAs). Authors explained that the disintegration of fat and triacylglycerol breakdown from the fat globule membrane, together with heterogeneous metal catalysis oxidation could cause the degradation of certain fatty acids in this particular experiment and further research is needed. Nevertheless, researchers highlighted that applied PEF treatment had minor effects on short-chain fatty acids and medium-chain fatty acids of goat milk and this technique can be recommended to increase goat milk quality at the industrial level.
Effect on Organoleptic Properties
The effect of PEF treatment on dairy liquids' sensory properties has not been widely studied to this date. There are few research papers published in the last decade concerning this topic. They are focused on the effects of PEF on the volatile profiles of milk evaluated by gas chromatography/mass spectrometry (GC-MS) (Zhang et al., 2011) and assessment of color changes in same media examined with colorimetric analysis (McAuley et al., 2016).
The study of Zhang et al. (2011) revealed that PEF treatment (15-30 kV/cm for 800 μs, bipolar square-wave pulses with width of 2 μs, the pulse repetition rate of 200 Hz and temperature <40°C) results in increase in aldehydes and does not alter methyl ketones of milk, while heat treatment (75°C for 15s) induces an increase in both compounds. Additionally, no significant differences in composition of alcohols (ethanol, 3-methyl-butanol, pentanol, hexanol, and 2-ethyl-hexanol), acids (butanoic acid, acetic acid, octanoic acid, hexanoic acid, and decanoic acid), and lactones (δ-dodecalactone and δ-decalactone) were detected among raw, heat-treated and PEF-treated milk. The exceptions were 2(5H)-furanone (caramel odour) detected only in PEF-treatment milk and not identified odorant described as “fatty and waxy” detected in PEF-treated sample and not detected in thermal treated sample. Considering odour intensity, these two compounds are classified as clearly perceived odours which indicates that sensory profile of PEF-treated milk may be slightly different from that of raw or pasteurized milk. However, as mentioned by authors, further investigation is necessary to understand the formation mechanism of volatile compounds induced by PEF.
Similarly, to minor effect on volatile compounds, colour analysis of PEF-treated milk (30 kV/cm for 22 μs) conducted by McAuley et al. (2016) revealed that PEF generates marginal changes in lightness of this product.
Sustainability
Pulsed electric fields treatment is considered as environment-friendly technology that can be applied in food processing for microorganism/enzyme inactivation and recovery of bioactive compounds (Li and Farid, 2016). However, further development in PEF equipment is necessary to lower the energy demand to achieve results compared to standard pasteurization and transfer PET to the industrial level as competitive technology (Alirezalu et al., 2020). PEF is still too complex to easily upscale it from pilot to industrial scale (Arshad et al., 2020). It is stated that PEF treatment would cost more to process the same amount of liquid food in comparison with other emerging technologies like membrane filtration and ultraviolet radiation or even a conventional heat processing (Alirezalu et al., 2020).
Membrane Filtration
Membrane filtration has been applied in dairy industry since 1960s (Ribeiro et al., 2010; Kumar et al., 2013) and is based on separating compounds by blocking some (retentate) and allowing permeance of other (permeate). The liquid product travels through a membrane by hydrostatic pressure (transmembrane pressure) (Skrzypek and Burger, 2010; Kumar et al., 2013; Shabbir et al., 2020). The membranes are defined by the size of their pores and their cut off; Microfiltration (MF), Ultrafiltration (UF), Nanofiltration (NF), Reverse Osmosis (RO) (Ribeiro et al., 2010; Kumar et al., 2013).
During MF the particles passing through the membrane are of sizes 0.2–2μm and in comparison to UF the pressure is much lower (Kumar et al., 2013). UF uses semi permeable membranes where only water and low molecular weight compounds can pass through it, with a cut off of 10,000 MW (Molecular Weight). RO is a process which uses high pressures and only low molecular weight solvents can pass through the pores. NF can be assumed as a type of RO, allowing only monovalent ions to pass from the membranes by using a cut off of 100 MW The retained part is called “retentate” or “concentrate” and the part which passes through the membrane is known as “permeate” (Kumar et al., 2013).
Cellulose acetate membranes are usually used due to their low cost and the low percentage of fouling (Shabbir et al., 2020). Cross flow electro membranes are also known for their low cost and easy scale up (Leeb et al., 2014). The use in dairy industry is mostly oriented towards fractionation of fat/proteins, demineralization, extension of milk's shelf life and whey purification/processing (Kumar et al., 2013). Whey purification is essential for protecting the environment, as most of the whey/brine is waste. By filtrating, minerals and other hazardous compounds are not released in the environment (Kumar et al., 2013). Further examples are the MF usage to reduce somatic cells in milk (Ribeiro et al., 2010; Wang et al., 2019) and RO already being used for the production of concentrated milk (Skrzypek and Burger, 2010).
Effect on Product Safety
Microfiltration has been proved to be efficient procedure for microbial and spore removal by many studies (Ribeiro et al., 2010; Tomasula et al., 2011; Barukči et al., 2014; Fernández García and Riera Rodríguez, 2014; Shrivastava and Banerjee, 2017; Griep et al., 2018; Marx et al., 2018; Wang et al., 2019). Some authors mention that using only MF for microbial safety, is not recommended as spores and some pathogenic bacteria may survive and grow during the shelf life of products such as milk (Fernández García and Riera Rodríguez, 2014). Others suggest, that microfiltration by ceramic membranes, is a safe way and non-invasive way of purification (Skrzypek and Burger, 2010).
Tetra pack International SA in Switzerland is already using Bactocatch for microbial removal in skim milk using 1.4 μm cross flow transmembrane ceramic membrane (Ribeiro et al., 2010; Caplan and Barbano, 2013). Furthermore, IsofluxTM ceramic membranes are efficient for the production of ESL milk in combination with heat, they can remove spores and keep the flux constant and are used widely (Skrzypek and Burger, 2010).
Effect on Nutritional Quality and Functional Properties
Membrane filtration is already a technique for the production of ESL milk (Ribeiro et al., 2010; Caplan and Barbano, 2013; Kumar et al., 2013; Panopoulos et al., 2020). It is also used for the fractionation of dairy proteins which by hydrolyzation lead to the production of beneficial peptides. Besides fractionation of proteins, the enzymatic activity can be reduced (Fernández García and Riera Rodríguez, 2014). There is the need though for higher fractionation efficiency and higher throughput (Leeb et al., 2014).
Skrzypek and Burger (2010) concluded that for IsofluxTM ceramic membranes are suitable for the production of ESL in combination with heat. MF can reduce the temperature of UHT milk by 20°C and in combination with pasteurization can provide a shelf life of 30 days stored at 4-6°C (Fernández García and Riera Rodríguez, 2014). Also, it allows the retention of proteins and their commercialization (Ribeiro et al., 2010).
The balance between ensuring safety and maintaining nutritional quality for ESL milk is very difficult (Fernández García and Riera Rodríguez, 2014). As mentioned before the constant flux is also difficult to maintain, but membranes of 1.4 μm can work for continuous flux, no rejection of precious compounds such as proteins and achieve microbial reduction. The major drawback is that fat is around the same size as microbes which leads to fast fouling and therefore low performance of the system (Fernández García and Riera Rodríguez, 2014).
Consequently, they are promising for the production of innovative products as the nutritional value of the products is not affected and they can be used for protein fractionation. Last but not least, they can be used for brine purification as it assures microbiological safety and does not affect the proteins and salts in brine (Skrzypek and Burger, 2010).
Effect on Organoleptic Properties
For the production of cheeses, the milk derived from MF is considered appropriate, due to the optimization of its components and its microbial premium quality. Regarding milk fat processing, membranes are being used to produce milk cream with improved quality and extended shelf life. RO can concentrate milk without affecting any of its components and remove 70% of its water without heating being involved, while the UF permeate consists of only water and lactose (Kumar et al., 2013).
The use of brine deriving from MF has been proved to produce ripening cheeses of higher quality compared to the brine deriving from heating (Ribeiro et al., 2010). Wang et al. (2019), performed triangle tests of MF and non-MF skim milk in order to identify differences during shelf life. At 5 days refrigeration the random panellists could not identify any differences, while at 24 days refrigeration there was a significant difference between the samples. The differences were based on “lighter taste”, “less sweet” and “less creamy” and the authors justified these observations by the reduced solids in the MF milk. Trained panellists did not find any differences during the 24 days of shelf life.
Finally, Kumar et al. (2013), concluded that the use of membranes in the dairy industry provided products with higher quality and created space for innovation, while Ribeiro et al. (2010), stated that sensory characteristics of milk are improved.
Sustainability
The cost for installing equipment for an extended shelf life (ESL) milk product produced by microfiltration is lower to the cost of ultra-high temperature (UHT). For installing a microfiltration of capacity 25,000L/h with a cream heater is about 600,000€, while a UHT ESL installation will be around 1,000,000 EUR for the same capacity (Skrzypek and Burger, 2010).
Consumer Acceptance
The terminology is important for the communication with the consumers. Consumers need to be educated about the benefits of non-thermal technologies (Shrivastava and Banerjee, 2017; Coutinho et al., 2021), as they believe them to be overestimated and it is too risky to replace them, so they are not willing to pay more than the conventional ones (Coutinho et al., 2021). Hence consumers still tend to reject novel products by non-thermal technologies (Tuorila and Hartmann, 2020).
Studies suggest that informing consumers about novel non-thermal processes and their labelling could be a solution to increase the intention of buying the specific products (Jaeger et al., 2015; Coutinho et al., 2021; Schiano and Drake, 2021). They require simple, short and understandable labels stating the benefits of the novel technologies (Jaeger et al., 2015). On the other hand, consumers are still cautious about unknown processing terms (Schiano and Drake, 2021) and the industry recognizes that labelling of the novel technologies could be a barrier for their adoption due to that (Meijer et al., 2021). It seems that some novel technologies are more acceptable than others due to the familiarity of the terms. HPP (pressure) seems to be more acceptable than PEF (pulses) (Olsen et al., 2010). HPP was associated with more positive comments in comparison to PEF (Jaeger et al., 2015).
Delorme et al. (2021), presented three different stimuli of Prato cheese to Brazilian consumers, where the label was differing. The label of the first one was “Sliced Prato cheese” which was the conventional one, the second one was “Sliced Prato cheese treated with UV light” and the third one was the same with the second but had an explanation of the benefits of UV light. The results showed that even though there was no significant difference in the perceived safety and quality, the “Sliced Prato cheese treated with UV light” without further information, had lower acceptance than the conventional, even though the consumers were characterized by innovativeness. The study revealed that consumers need to be informed about novel technologies in order to accept them (Delorme et al., 2021). Generally, studies for the consumer acceptance of UV-C treated dairy are very limited (Rossitto et al., 2012; Delorme et al., 2021).
Another example related to terminology, is a study done by Jaeger et al. (2015), in which consumers associated “Micro Electric Pulse” with more neutral reactions, compared to the term “Pulsed Electric Field”, even though it was describing the same technology. The same study revealed that young people are more open to the non- conventional treatments while the elderly associate them with “more expensive”.
Mújica-Paz et al. (2011), highlights that HPP due to the preservation of high nutritional value and quality, the microbial safety and the lack of preservatives directing closer to the term natural, has great potential for high consumer acceptance. Consumers are still not aware of the beneficial effects of non-thermal technologies which can influence their acceptance (Mújica-Paz et al., 2011) and they will be the ones assuring the success of a product (Olsen et al., 2010).
Parekh et al. (2017), states that consumers are willing to buy a product only of its safe and to pay more if they are characterized as premium quality (Murphy et al., 2016). It was shown that HPP is the second most applicable non-thermal technology around the world after microwaving (Jermann et al., 2015). The survey showed that the participants were driven mostly by the added-value and premium quality. Brazilian consumers (72.3%) were willing to buy a chocolate milk drink processed by cold plasma if the product was sold at the same or lower price from the conventional one (Coutinho et al., 2021).
In terms of cold plasma processing, it was reported that despite reduced consistency of whey beverages subjected to cold plasma treatment, the consumer acceptance of cold plasma treated product was similar to the untreated one (Ribeiro et al., 2021). This result is important from the commercialization point of view, as it demonstrates that it is possible to formulate plasma-treated whey beverages that maintain the acceptance of the untreated products.
Future Trends
Over the last decade a number of studies have been published regarding novel processes. The next step, is scaling-up of these processes, to eliminate their drawbacks and to connect to consumer acceptance. In order to industrialize the processes, their efficacy and safety parameters should be established, therefore, industries need to understand the mechanisms of action, determine the critical control points of the whole production line, ensure quality and implement risk and cost analysis in order to scale up the processes. The industry should be directed towards cost effectiveness and have data on the specific topic compared to the thermal treatment (Chakka et al., 2021).
As an example, the efficacy of thermal processes is easy to monitor, as heat processing cause changes in milk enzymatic activity; i.e. alkaline phosphatase activity (ALP) in pasteurized milk is an indication of insufficient pasteurization process (Segat et al., 2016). Such a fast indicator needs to be established for the novel non-thermal technologies for a rapid assessment of the process efficacy in producing a safe product (Delorme et al., 2020).
The challenge associated with these novel technologies is that their mode of action is based on many product parameters the affect processing i.e. for the case of the UV-C technology surface, opacity and turbidity of the product, intensity and dose of the light, species of the microorganism are related to end-product safety (Pereira et al., 2014; Fan et al., 2017; Pendyala et al., 2021). As a result, specific studies should take place on each system and on each product in order to specify the conditions to ensure that the product is safe for consumption. Moreover, higher product penetration and efficiency needs to be guaranteed to counteract the high investment cost (Can et al., 2014; Koca and Öztürk, 2020).
The effects of HIUS technology are also very dependent on the processing parameters, which affect the acoustic intensity or the total acoustic energy received by the food. The ultrasonication area still lack a consensus in the presentation of processing parameters and the form of calculation of the real applied acoustic energy in order to be able to compare results of different studies. Thus, further studies should be focused on the determination of processing parameters for each type of dairy product. In addition, it is necessary to determine the inactivation kinetics of different pathogens by ultrasound in variety of dairy products. The aim of the future research would be the application of quantitative risk analysis models, which could be used as a part of food quality management system (Asaithambi et al., 2021; Guimarães et al., 2021).
In terms of PEF processing, extra capital expenses of commercial PEF equipment and processes over traditional heat treatment will need to be balanced by a premium-priced product (Soltanzadeh et al., 2020). Moreover, data obtained from the laboratory-scale trials (usually batch-type with the laminar flow) should be compared with results collected from the industrial scale. As processes differ in uniformity and conditions, generalization of data to commercial-scale is often not feasible and reliable (Buckow et al., 2010). Therefore, up-scaling of PEF-equipment for dairy applications remains a challenge and should be a topic of future studies. Same applies for HPP, Cold Plasma and Ultrasound (Coutinho et al., 2018; Chakka et al., 2021).
Along with the abovementioned, consumers need to be informed adequately in order for commercial products derived from these novel technologies, to be accepted (Ribeiro et al., 2022). Generally, studies for consumer acceptance of novel processes are very limited. Usually, consumers are sceptic of unknown words such as irradiation (Chacha et al., 2021). In order for the consumers to accept such technologies they need to familiarized with such terms (Tuorila and Hartmann, 2020; Coutinho et al., 2021; Schiano and Drake, 2021).
Summing up, future studies should focus on the cost efficiency of the production, on ensuring the safety of food especially regarding inactivation of spores and informing the consumers about the advantages of these non-thermal technologies in order to accept them.
Author Contributions
PP contributed to conception and design of the study and edited the draft. IN and JT wrote the first draft of the manuscript and contributed to the design of the study. All authors contributed to the article and approved the submitted version.
Conflict of Interest
The authors declare that the research was conducted in the absence of any commercial or financial relationships that could be construed as a potential conflict of interest.
Publisher's Note
All claims expressed in this article are solely those of the authors and do not necessarily represent those of their affiliated organizations, or those of the publisher, the editors and the reviewers. Any product that may be evaluated in this article, or claim that may be made by its manufacturer, is not guaranteed or endorsed by the publisher.
References
Akdeniz, V., and Akalin, A. S. (2022). Recent advances in dual effect of power ultrasound to microorganisms in dairy industry: activation or inactivation. Critic. Rev. Food Sci. Nutr. 62, 889–904. doi: 10.1080/10408398.2020.1830027
Alirezalu, K., Munekata, P. E., Parniakov, O., Barba, F. J., Witt, J., Toepfl, S., et al. (2020). Pulsed electric field and mild heating for milk processing: a review on recent advances. J. Sci. Food Agric. 100, 16–24. doi: 10.1002/jsfa.9942
Alves de Aguiar Bernardo, Y., Kaic Alves do Rosario, D., and Adam Conte-Junior, C. (2021). Ultrasound on milk decontamination: potential and limitations against foodborne pathogens and spoilage bacteria. Food Rev. Int. 1–14. doi: 10.1080/87559129.2021.1906696
Anema, S. G.. (2022). Denaturation of α-lactalbumin and bovine serum albumin in pressure-treated reconstituted skim milk. Food Chem. Adv. 1, 100002. doi: 10.1016/j.focha.2021.100002
Annor, G. A.. (2019). “Cold plasma effects on the nutritional, textural and sensory characteristics of fruits and vegetables, meat, and dairy products.” in Effect of Emerging Processing Methods on the Food Quality, eds S. Roohinejad, M. Koubaa., R. Greiner, and K. Mallikarjunan (Berlin: Springer, Cham). doi: 10.1007/978-3-030-18191-8_7
Arshad, R. N., Abdul-Malek, Z., Munir, A., Buntat, Z., Ahmad, M. H., Jusoh, Y. M., et al. (2020). Electrical Systems for Pulsed Electric Field Applications in the Food Industry: An Engineering Perspective. Trends Food Sci. Technol. 104, 1–13. doi: 10.1016/j.tifs.2020.07.008
Arvanitoyannis, I. S., Kotsanopoulos, K. V., and Savva, A. G. (2017). Use of ultrasounds in the food industry–methods and effects on quality, safety, and organoleptic characteristics of foods: a review. Crit. Rev. Food Scie. Nutr. 57, 109–28. doi: 10.1080/10408398.2013.860514
Asaithambi, N., Singh, S. K., and Singha, P. (2021). Current status of non-thermal processing of probiotic foods: A review. J. Food Eng. 303, 110567. doi: 10.1016/j.jfoodeng.2021.110567
Aydar, E. F., Tutuncu, S., and Ozcelik, B. (2020). Plant-based milk substitutes: Bioactive compounds, conventional and novel processes, bioavailability studies, and health effects. J. Funct. Foods, 70, 103975. doi: 10.1016/j.jff.2020.103975
Baboli, Z. M., Williams, L., and Chen, G. (2020). Rapid pasteurization of apple juice using a new ultrasonic reactor. Foods. 9, 801. doi: 10.3390/foods9060801
Balasubramaniam, V. M. B., Martínez-Monteagudo, S. I., and Gupta, R. (2015). Principles and application of high pressure-based technologies in the food industry. Ann. Rev. Food Sci. Technol. 6, 435–462. doi: 10.1146/annurev-food-022814-015539
Balthazar, C. F., Santillo, A., Guimarães, J. T., Bevilacqua, A., Corbo, M. R., Caroprese, M., et al. (2019). Ultrasound processing of fresh and frozen semi-skimmed sheep milk and its effects on microbiological and physical-chemical quality. Ultrasonics Sonochem. 51, 241–248. doi: 10.1016/j.ultsonch.2018.10.017
Bandla, S., Choudhary, R., Watson, D. G., and Haddock, J. (2012). UV-C treatment of soymilk in coiled tube UV reactors for inactivation of Escherichia coli W1485 and Bacillus cereus endospores. LWT - Food Sci. Technol. 46, 71–76. doi: 10.1016/j.lwt.2011.10.024
Barba, F. J., Terefe, N. S., Buckow, R., Knorr, D., and Orlien, V. (2015). New opportunities and perspectives of high pressure treatment to improve health and safety attributes of foods. A review. Food Res. Int. 77, 725–742. doi: 10.1016/j.foodres.2015.05.015
Barukči,ć, I., BoŽani,ć, R., and Kulozik, U. (2014). Effect of pore size and process temperature on flux, microbial reduction and fouling mechanisms during sweet whey cross-flow microfiltration by ceramic membranes. Int. Dairy J. 39, 8–15. doi: 10.1016/j.idairyj.2014.05.002
Beauchamp, S., and Lacroix, M. (2012). Resistance of the genome of Escherichia coli and Listeria monocytogenes to irradiation evaluated by the induction of cyclobutane pyrimidine dimers and 6-4 photoproducts using gamma and UV-C radiations. Radiat. Phys. Chem. 81, 1193–1197. doi: 10.1016/j.radphyschem.2011.11.007
Bermúdez-Aguirre, D., Dunne, C. P., and Barbosa-Cánovas, G. V. (2012). Effect of processing parameters on inactivation of bacillus cereus spores in milk using pulsed electric fields. Int. Dairy J. 24, 13–21. doi: 10.1016/j.idairyj.2011.11.003
Bogahawaththa, D., Buckow, R., Chandrapala, J., and Vasiljevic, T. (2018). Comparison between thermal pasteurization and high pressure processing of bovine skim milk in relation to denaturation and immunogenicity of native milk proteins. Innov. Food Sci. Emerg. Technol. 47, 301–308. doi: 10.1016/j.ifset.2018.03.016
Buckow, R., Chandry, P. S., Ng, S. Y., McAuley, C. M., and Swanson, B. G. (2014). Opportunities and challenges in pulsed electric field processing of dairy products. Int. Dairy J. 34, 199–212. doi: 10.1016/j.idairyj.2013.09.002
Buckow, R., Schroeder, S., Berres, P., Baumann, P., and Knoerzer, K. (2010). Simulation and evaluation of pilot-scale pulsed electric field (PEF) processing. J. Food Eng. 101, 67–77. doi: 10.1016/j.jfoodeng.2010.06.010
Can, F. O., Demirci, A., Puri, V. M., and Gourama, H. (2014). Decontamination of hard cheeses by pulsed UV light. J. Food Protection, 77, 1723–1731. doi: 10.4315/0362-028X.JFP-13-559
Caplan, Z., and Barbano, D. M. (2013). Shelf life of pasteurized microfiltered milk containing 2% fat1. J. Dairy Sci., 96, 8035–8046. doi: 10.3168/jds.2013-6657
Chacha, J. S., Zhang, L., Ofoedu, C. E., Suleiman, R. A., Dotto, J. M., Roobab, U., et al. (2021). Revisiting non-thermal food processing and preservation methods-action mechanisms, pros and cons: A technological update (2016-2021). Foods 10, 1430. doi: 10.3390/foods10061430
Chakka, A. K., Sriraksha, M. S., and Ravishankar, C. N. (2021). Sustainability of emerging green non-thermal technologies in the food industry with food safety perspective: a review. Lwt, 151, 112140. doi: 10.1016/j.lwt.2021.112140
Chandrajith, V. G. G., Karunasena, G. A. D. V., and Vithanage, R. (2018). Effect of non-thermal processing techniques on milk components and dairy products: mini review. Int. J. Food Sci. Nutr. 3, 157–159.
Chawla, R., and Patil, G. R. (2011). High hydrostatic pressure technology in dairy processing: a review, J. food Sci. Technol. 48, 260–268. doi: 10.1007/s13197-010-0180-4
Chemat, F., and Khan, M. K. (2011). Applications of ultrasound in food technology: processing, preservation and extraction. Ultrason. Sonochem. 18, 813–835. doi: 10.1016/j.ultsonch.2010.11.023
Chen, G., Wu, C., Xiaojie, C., Zhennai, Y., and Huqing, Y. (2022). Studying the effects of high pressure – temperature treatment on the structure and immunoreactivity of β-lactoglobulin using experimental and computational methods. Food Chem. 372, 131226. doi: 10.1016/j.foodchem.2021.131226
Choudhary, R., and Bandla, S. (2012). Ultraviolet Pasteurization for Food Industry. International J. Food Sci. Nutr. Eng., 2, 12–15. doi: 10.5923/j.food.20120201.03
Chughtai, M. F. J., Farooq, M. A., Ashfaq, S. A., Khan, S., Khaliq, A., Antipov, S., et al. (2021). Role of pascalization in milk processing and preservation: a potential alternative towards sustainable food processing. Photonics. 8, 498. doi: 10.3390/photonics8110498
Cilla, A., Lagarda, M. J., Alegría, A., de Ancos, B., Cano, M. P., Sánchez-Moreno, C., et al. (2011). Effect of processing and food matrix on calcium and phosphorous bioavailability from milk-based fruit beverages in Caco-2 cells. Food Res. Int., 44, 3030–3038. doi: 10.1016/j.foodres.2011.07.018
Cilliers, F. P., Gouws, P. A., Koutchma, T., Engelbrecht, Y., Adriaanse, C., and Swart, P. (2014). A microbiological, biochemical and sensory characterisation of bovine milk treated by heat and ultraviolet (UV) light for manufacturing Cheddar cheese. Innov. Food Sci. Emerg. Technol. 23, 94–106. doi: 10.1016/j.ifset.2014.03.005
Coolbear, T., Janin, N., Traill, R., and Shingleton, R. (2022). Heat-induced changes in the sensory properties of milk. Int. Dairy J., 126, 105199. doi: 10.1016/j.idairyj.2021.105199
Coutinho, N. M., Silveira, M. R., Fernandes, L. M., Moraes, J., Pimentel, T. C., Freitas, M. Q., et al. (2019). Processing chocolate milk drink by low-pressure cold plasma technology. Food Chem. 278, 276–283. doi: 10.1016/j.foodchem.2018.11.061
Coutinho, N. M., Silveira, M. R., Guimarães, J. T., Fernandes, L. M., Pimentel, T. C., Silva, M. C., et al. (2021). Are consumers willing to pay for a product processed by emerging technologies? The case of chocolate milk drink processed by cold plasma. LWT. 138, 110772. doi: 10.1016/j.lwt.2020.110772
Coutinho, N. M., Silveira, M. R., Rocha, R. S., Moraes, J., Ferreira, M. V. S., Pimentel, T. C., et al. (2018). Cold Plasma Processing of Milk and Dairy Products. Trends Food Sci. Technol. 74, 56–68. doi: 10.1016/j.tifs.2018.02.008
Cullen, P. J., Tiwari, B. K., and Valdramidis, V. P. (2012). “Status and trends of novel thermal and non-thermal technologies for fluid foods,” in Novel Thermal and Non-Thermal Technologies for Fluid Foods, eds P. J. Cullen, B. K. Tiwari, and V. Valdramidis (Academic Press), 1–6. doi: 10.1016/B978-0-12-381470-8.00001-3
de Toledo Guimarães, J., Silva, E. K., de Freitas, M. Q., de Almeida Meireles, M. A., and da Cruz, A. G. (2018). Non-thermal emerging technologies and their effects on the functional properties of dairy products. Curr Opin. Food Sci. 22, 62–66. doi: 10.1016/j.cofs.2018.01.015
Delorme, M. M., Guimarães, J. T., Coutinho, N. M., Balthazar, C. F., Rocha, R. S., Silva, R., et al. (2020). Ultraviolet radiation: an interesting technology to preserve quality and safety of milk and dairy foods. Trends Food Sci. Technol. 102, 146–154. doi: 10.1016/j.tifs.2020.06.001
Delorme, M. M., Pimentel, T. C., Freitas, M. Q., da Cunha, D. T., Silva, R., Guimarães, J. T., et al. (2021). Consumer innovativeness and perception about innovative processing technologies: A case study with sliced Prato cheese processed by ultraviolet radiation. Int. J. Dairy Technol. 74, 768–777. doi: 10.1111/1471-0307.12807
Devi, A. F., Buckow, R., Hemar, Y., and Kasapis, S. (2013). Structuring dairy systems through high pressure processing. J. Food Eng. 114, 106–122. doi: 10.1016/j.jfoodeng.2012.07.032
Dhahir, N., Feugang, J., Witrick, K., Park, S., White, S., and AbuGhazaleh, A. (2021). The effect of different ultraviolet-C light doses on microbial reduction and the components of camel milk. Food Sci. Technol. Int. 27, 99–111. doi: 10.1177/1082013220935230
Esteghlal, S., Gahruie, H. H., Niakousari, M., Barba, F. J., Bekhit, A. E., Mallikarjunan, K., et al. (2019). Bridging the knowledge gap for the impact of non-thermal processing on proteins and amino acids. Foods 8, 262. doi: 10.3390/foods8070262
European Food Safety Authority. (2016). Safety of UV-treated milk as a novel food pursuant to Regulation (EC) No 258/97. EFSA J. 14, 4370. doi: 10.2903/j.efsa.2016.4370
Evert-Arriagada, K., Hernández-Herrero, M. M., Guamis, B., and Trujillo, A. J. (2014). Commercial application of high-pressure processing for increasing starter-free fresh cheese shelf-life. LWT Food Sci. Technol. 55, 498–505. doi: 10.1016/j.lwt.2013.10.030
Evrendilek, G. A.. (2018). Effects of high pressure processing on bioavaliability of food components. J. Nutr. and Food Sci. 8, 676. doi: 10.4172/2155-9600.1000676
Fan, X., Huang, R., and Chen, H. (2017). Application of ultraviolet C technology for surface decontamination of fresh produce. Trends Food Sci. Technol. 70, 9–19. doi: 10.1016/j.tifs.2017.10.004
Fernández García, L., and Riera Rodríguez, F. A. (2014). Combination of microfiltration and heat treatment for ESL milk production: Impact on shelf life. J. Food Eng. 128, 1–9. doi: 10.1016/j.jfoodeng.2013.11.021
Fernández, M., Hospital, X. F., Arias, K., and Hierro, E. (2016). Application of Pulsed Light to Sliced Cheese: Effect on Listeria Inactivation, Sensory Quality and Volatile Profile. Food Bioprocess Technol. 9, 1335–1344. doi: 10.1007/s11947-016-1721-2
Gao, S., Hemar, Y., Lewis, G. D., and Ashokkumar, M. (2014). Inactivation of enterobacter aerogenes in reconstituted skim milk by high- and low-frequency ultrasound. Ultrason. Sonochem. 21, 2099–2106. doi: 10.1016/j.ultsonch.2013.12.008
Gao, Y., Qiu, W., Wu, D., and Fu, Q. (2011). Assessment of clostridium perfringens spore response to high hydrostatic pressure and heat with nisin. Applied BioChem. BioTechnol. 164, 1083–1095. doi: 10.1007/s12010-011-9196-0
Gayán, E., Condón, S., and Álvarez, I. (2014). Biological aspects in food preservation by ultraviolet light: a review. Food Bioprocess Technol. 7, 1–20. doi: 10.1007/s11947-013-1168-7
Gayán, E., Monfort, S., Álvarez, I., and Condón, S. (2011). UV-C inactivation of Escherichia coli at different temperatures. Innov. Food Sci. Emerg. Technol., 12, 531–541. doi: 10.1016/j.ifset.2011.07.008
Ginsau, M. A.. (2015). High pressure processing: a novel food preservation technique. IOSR J. Environmental Sci. Toxicol Food Technol. 9, 109–113. doi: 10.9790/2402-0951109113
Goyal, A., Sharma, V., Upadhyay, N., Sihag, M., and Kaushik, R. (2013). High pressure processing and its impact on milk proteins: a review. research and reviews. J. Dairy Sci. Technol. 2, 12–20. Retrieved from http://www.stmjournals.com/sci/index.php?journal=RRJoDSTandpage=articleandop=viewandpath%5B%5D=366%5Cn
Griep, E. R., Cheng, Y., and Moraru, C. I. (2018). Efficient removal of spores from skim milk using cold microfiltration: spore size and surface property considerations. J. Dairy Sci. 101, 9703–9713. doi: 10.3168/jds.2018-14888
Guerrero-Beltrán, J. Á., Sepulveda, D. R., Góngora-Nieto, M. M., Swanson, B., and Barbosa-Cánovas, G. V. (2010). Milk thermization by Pulsed Electric Fields (PEF) and Electrically Induced Heat. J. Food Eng. 100, 56–60. doi: 10.1016/j.jfoodeng.2010.03.027
Guimarães, J. T., Scudino, H., Ramos, G. L., Oliveira, G. A., Margalho, L. P., Costa, L. E., et al. (2021). Current applications of high-intensity ultrasound with microbial inactivation or stimulation purposes in dairy products. Curr Opin. Food Sci. 42, 140–147. doi: 10.1016/j.cofs.2021.06.004
Guimarães, J. T., Silva, E. K., Alvarenga, V. O., Costa, A. L. R., Cunha, R. L., Sant'Ana, A. S., et al. (2018). Physicochemical changes and microbial inactivation after high-intensity ultrasound processing of prebiotic whey beverage applying different ultrasonic power levels. Ultrason. Sonochem. 44, 251–260. doi: 10.1016/j.ultsonch.2018.02.012
Guimarães, J. T., Silva, E. K., Ranadheera, C. S., Moraes, J., Raices, R. S. L., Silva, M. C., et al. (2019). Effect of high-intensity ultrasound on the nutritional profile and volatile compounds of a prebiotic soursop whey beverage. Ultrasonics Sonochem. 55, 157–164. doi: 10.1016/j.ultsonch.2019.02.025
Guneser, O. N. U. R., and Yuceer, Y. K. (2012). Effect of ultraviolet light on water- and fat-soluble vitamins in cow and goat milk. J. Dairy Sci., 95, 6230–6241. doi: 10.3168/jds.2011-5300
Gurol, C., Ekinci, F. Y., Aslan, N., and Korachi, M. (2012). Low temperature plasma for decontamination of E. Coli in Milk. Int. J. Food Microbiol. 157, 1–5. doi: 10.1016/j.ijfoodmicro.2012.02.016
Heinz, V., and Buckow, R. (2010). Food preservation by high pressure. J. Fur Verbraucherschutz Und Lebensmittelsicherheit, 5, 73–81. doi: 10.1007/s00003-009-0311-x
Hernández-Hernández, H. M., Moreno-Vilet, L., and Villanueva-Rodríguez, S. J. (2019). Current status of emerging food processing technologies in latin america: novel non-thermal processing. Innov. Food Sci. Emerg. Technol. 58, 102233. doi: 10.1016/j.ifset.2019.102233
Huang, G., Chen, S., Tang, Y., Dai, C., Sun, L., Ma, H., et al. (2019). Stimulation of low intensity ultrasound on fermentation of skim milk medium for yield of yoghurt peptides by Lactobacillus paracasei. Ultrasonics Sonochem. 51, 315–324. doi: 10.1016/j.ultsonch.2018.09.033
Huang, H. W., Hsu, C. P., and Wang, C. Y. (2020). Healthy expectations of high hydrostatic pressure treatment in food processing industry. J. Food and Drug Analysis, 28, 1–13. doi: 10.1016/j.jfda.2019.10.002
Huang, H. W., Lung, H. M., Yang, B. B., and Wang, C. Y. (2014). Responses of microorganisms to high hydrostatic pressure processing. Food Control, 40, 250–259. doi: 10.1016/j.foodcont.2013.12.007
Huppertz, T., and Nieuwenhuijse, H. (2022). Constituent fouling during heat treatment of milk: a review. Int. Dairy J. 126, 105236. doi: 10.1016/j.idairyj.2021.105236
Jaeger, H., Knorr, D., Szab,ó, E., Hámori, J., and Bánáti, D. (2015). Impact of terminology on consumer acceptance of emerging technologies through the example of PEF technology. Innov. Food Sci. Emerg. Technol., 29, 87–93. doi: 10.1016/j.ifset.2014.12.004
Jermann, C., Koutchma, T., Margas, E., Leadley, C., and Ros-Polski, V. (2015). Mapping trends in novel and emerging food processing technologies around the world. Innov. Food Sci. Emerg. Technol., 31, 14–27. doi: 10.1016/j.ifset.2015.06.007
Kadam, S. U., Tiwari, B. K., Álvarez, C., and O'Donnell, C.P. (2015). Ultrasound applications for the extraction, identification and delivery of food proteins and bioactive peptides. Trends Food Sci. Technol. 46, 60–67. doi: 10.1016/j.tifs.2015.07.012
Kayalvizhi, V., Sowmiya, S., Priya, T. S., and Antony, U. (2021). Processing flavoured milk by thermal and non thermal methods. Asian J. Dairy Food Res. 40, 129–135. doi: 10.18805/ajdfr.DR-1561
Keklik, N. M., Elik, A., Salgin, U., Demirci, A., and Koçer, G. (2019). Inactivation of Staphylococcus aureus and Escherichia coli O157:H7 on fresh kashar cheese with pulsed ultraviolet light. Food Sci. Technol. Int., 25, 680–691. doi: 10.1177/1082013219860925
Khanal, S. N., Anand, S., Muthukumarappan, K., and Huegli, M. (2014). Inactivation of thermoduric aerobic sporeformers in milk by ultrasonication. Food Control 37, 232–239. doi: 10.1016/j.foodcont.2013.09.022
Kim, D. K., Kim, S. J., and Kang, D. H. (2017). Bactericidal effect of 266 to 279 nm wavelength UVC-LEDs for inactivation of Gram positive and Gram negative foodborne pathogenic bacteria and yeasts. Food Res. Int., 97, 280–287. doi: 10.1016/j.foodres.2017.04.009
Kim, H. J., Yong, H. I., Park, S., Kim, K., Choe, W., and Jo, C. (2015). Microbial safety and quality attributes of milk following treatment with atmospheric pressure encapsulated dielectric barrier discharge plasma. Food Control 47, 451–456. doi: 10.1016/j.foodcont.2014.07.053
Knorr, D., Froehling, A., Jaeger, H., Reineke, K., Schlueter, O., and Schoessler, K. (2011). Emerging technologies in food processing. Ann. Rev. Food Sci. Technol. 2, 203–235. doi: 10.1146/annurev.food.102308.124129
Koca, N., and Öztürk, M. U. (2020). Application of batch system ultraviolet light on the surface of kashar cheese, a kind of pasta-filata cheese: effects on mould inactivation, lipid oxidation, colour, hardness and sensory properties. J. Dairy Res. 87, 493–497. doi: 10.1017/S0022029920001119
Korachi, M., Ozen, F., Aslan, N., Vannini, L., Guerzoni, M. E., Gottardi, D., et al. (2015). Biochemical changes to milk following treatment by a novel, cold atmospheric plasma system. Int. Dairy J. 42, 64–69. doi: 10.1016/j.idairyj.2014.10.006
Kumar, P., Sharma, N., Ranjan, R., Kumar, S., Bhat, Z. F., and Jeong, D. K. (2013). Perspective of membrane technology in dairy industry: a review. Asian-Australasian J. Animal Sci. 26, 1347–1358. doi: 10.5713/ajas.2013.13082
Kwon, S. W., Kwon, E. A., Hong, Y. G., and Kim, S. S. (2022). Germination of Bacillus cereus ATCC 14579 spore at various conditions and inactivation of the germinated cells with microwave heating and UVC treatment in milk samples. LWT. 154, 112702. doi: 10.1016/j.lwt.2021.112702
Lacivita, V., Conte, A., Manzocco, L., Plazzotta, S., Zambrini, V. A., Del Nobile, M. A., et al. (2016). Surface UV-C light treatments to prolong the shelf-life of Fiordilatte cheese. Innov. Food Sci. Emerg. Technol. 36, 150–155. doi: 10.1016/j.ifset.2016.06.010
Leeb, E., Holder, A., Letzel, T., Cheison, S. C., Kulozik, U., and Hinrichs, J. (2014). Fractionation of dairy based functional peptides using ion-exchange membrane adsorption chromatography and cross-flow electro membrane filtration. Int. Dairy J. 38, 116–123. doi: 10.1016/j.idairyj.2013.12.006
Li, X., and Farid, M. (2016). A review on recent development in non-conventional food sterilization technologies. J. Food Eng. 182, 33–45. doi: 10.1016/j.jfoodeng.2016.02.026
Liepa, M., Zagorska, J., and Galoburda, R. (2016). High-pressure processing as novel technology in dairy industry: a review. Res. for Rural Develop. 1, 76–83.
Liepa, M., Zagorska, J., Galoburda, R., and Kostascuka, S. (2018). Effect of high-pressure processing on microbial quality of skimmed milk. Proc Latvian Acad Sci. Sect B Nat Exact Appl Sci., 72, 118–122. doi: 10.2478/prolas-2018-0019
Lim, S. Y., Benner, L. C., and Clark, S. (2019). Neither thermosonication nor cold sonication is better than pasteurization for milk shelf life. J. Dairy Sci. 102, 3965–3977. doi: 10.3168/jds.2018-15347
Marchesini, G., Fasolato, L., Novelli, E., Balzan, S., Contiero, B., Montemurro, F., et al. (2015). Ultrasonic Inactivation of Microorganisms: a Compromise between lethal capacity and sensory quality of milk. Innov. Food Sci. Emerg. Technol. 29, 215–221. doi: 10.1016/j.ifset.2015.03.015
Martínez-Rodríguez, Y., Acosta-Muñiz, C., Olivas, G. I., Guerrero-Beltrán, J., Rodrigo-Aliaga, D., and Sepúlveda, D. R. (2012). High Hydrostatic Pressure Processing of Cheese. Comprehensive Rev. Food Sci. Food Safety, 11, 399–416. doi: 10.1111/j.1541-4337.2012.00192.x
Marx, M., Bernauer, S., and Kulozik, U. (2018). Manufacturing of reverse osmosis whey concentrates with extended shelf life and high protein nativity. Int. Dairy J., 86, 57–64. doi: 10.1016/j.idairyj.2018.06.019
McAuley, C. M., Singh, T. K., Haro-Maza, J. F., Williams, R., and Buckow, R. (2016). Microbiological and physicochemical stability of raw, pasteurised or pulsed electric field-treated milk. Innov. Food Sci. Emerg. Technol. 38, 365–373. doi: 10.1016/j.ifset.2016.09.030
Meijer, G. W., Lähteenmäki, L., Stadler, R. H., and Weiss, J. (2021). Issues surrounding consumer trust and acceptance of existing and emerging food processing technologies. Crit. Rev. Food Sci. Nutr. 61, 97–115. doi: 10.1080/10408398.2020.1718597
Minj, S., and Anand, S. (2020). Whey Proteins and Its Derivatives: Bioactivity, Functionality, and Current Applications. Dairy, 1, 233–258. doi: 10.3390/dairy1030016
Mohamad, A., Shah, N. N. A. K., Sulaiman, A., Mohd Adzahan, N., and Aadil, R. M. (2020). Impact of the pulsed electric field on physicochemical properties, fatty acid profiling, and metal migration of goat milk. J. Food Process Preserv. 44. doi: 10.1111/jfpp.14940
Monteiro, S. H., Silva, E. K., Guimarães, J. T., Freitas, M. Q., Meireles, M. A. A., and Cruz, A. G. (2020). High-intensity ultrasound energy density: How different modes of application influence the quality parameters of a dairy beverage. Ultrasonics Sonochem. 63, 104928. doi: 10.1016/j.ultsonch.2019.104928
Morales-de la Peña, M., Welti-Chanes, J., and Martín-Belloso, O. (2019). Novel technologies to improve food safety and quality. Curr Opin. Food Sci., 30, 1–7. doi: 10.1016/j.cofs.2018.10.009
Mújica-Paz, H., Valdez-Fragoso, A., Samson, C. T., Welti-Chanes, J., and Torres, A. (2011). High-pressure processing technologies for the pasteurization and sterilization of foods. Food Bioprocess Technol. 4, 969–985. doi: 10.1007/s11947-011-0543-5
Müller, A., Stahl, M. R., Graef, V., Franz, C. M., and Huch, M. (2011). UV-C treatment of juices to inactivate microorganisms using Dean vortex technology. J. Food Eng., 107, 268–275. doi: 10.1016/j.jfoodeng.2011.05.026
Muntean, M.-V., Marian, O., Barbieru, V., Cătunescu, G. M., Ranta, O., Drocas, I., et al. (2016). High pressure processing in food industry—characteristics and applications. Agricult Agricult Sci. Procedia, 10, 377–383. doi: 10.1016/j.aaspro.2016.09.077
Murphy, S. C., Martin, N. H., Barbano, D. M., and Wiedmann, M. (2016). Influence of raw milk quality on processed dairy products: how do raw milk quality test results relate to product quality and yield? J. Dairy Sci., 99, 10128–10149. doi: 10.3168/jds.2016-11172
Naik, L., Sharma, R., and Gaare, R. (2013). Application of high pressure processing technology for dairy food preservation—future perspective: a review. J. Animal Prod Adv. 3, 232. doi: 10.5455/japa.20120512104313
Oliveira, M. M., De, A., ugusto, P. E. D., Cruz, A. G., and Da Cristianini, M. (2014). Effect of dynamic high pressure on milk fermentation kinetics and rheological properties of probiotic fermented milk. Innov. Food Sci. Emerg. Technol, 26, 67–75. doi: 10.1016/j.ifset.2014.05.013
Olsen, N. V., Grunert, K. G., and Sonne, A. M. (2010). Consumer acceptance of high-pressure processing and pulsed-electric field: a review. Trends Food Sci. Technol. 21, 464–472. doi: 10.1016/j.tifs.2010.07.002
Orlowska, M, Koutchma, T., Grapperhaus, M., Gallagher, J., Schaefer, R., and Defelice, C. (2013). Continuous and pulsed ultraviolet light for nonthermal treatment of liquid foods. part 1: effects on quality of fructose solution, apple juice, and milk. Food Bioprocess Technol. 6, 1580–1592. doi: 10.1007/s11947-012-0779-8
Orlowska, M., Koutchma, T., Kostrzynska, M., Tang, J., and Defelice, C. (2014). Evaluation of mixing flow conditions to inactivate Escherichia coli in opaque liquids using pilot-scale Taylor—Couette UV unit. J. Food Eng., 120, 100–109. doi: 10.1016/j.jfoodeng.2013.07.020
Ortega, R. M., Ortega, A. I. J., Sánchez, J. M. P., Soto, E. C., Vizuete, A. A., and López-Sobaler, A. M. (2019). Nutritional value of dairy products and recommended daily consumption. Nutricion Hospitalaria 36, 25–29. doi: 10.20960/nh.02803
Pagnossa, J. P., Rocchetti, G., Ribeiro, A. C., Piccoli, R. H., and Lucini, L. (2020). Ultrasound: beneficial biotechnological aspects on microorganisms-mediated processes: Ultrasound: beneficial aspects on microorganisms processes. Curr. Opin. Food Sci. 31, 24–30. doi: 10.1016/j.cofs.2019.10.006
Pal, M., Desta, A., Worldemariam, T., and Karanfil, O. (2015). Use of high pressure technology on milk and milk products. Academia.Edu, 42, 2013–2015. Retrieved from https://www.academia.edu/download/39449404/October_2015_article.pdf
Pankaj, S. K., Wan, Z., and Keener, K. M. (2018). Effects of cold plasma on food quality: a review. Foods 7, 4. doi: 10.3390/foods7010004
Panopoulos, G., Moatsou, G., Psychogyiopoulou, C., and Moschopoulou, E. (2020). Microfiltration of ovine and bovine milk: effect on microbial counts and biochemical characteristics. Foods 9, 284. doi: 10.3390/foods9030284
Papademas, P., Mousikos, P., and Aspri, M. (2021). Optimization of uv-c processing of donkey milk: An alternative to pasteurization? Animals, 11, 1–10. doi: 10.3390/ani11010042
Parekh, S. L., Aparnathi, K. D., and Sreeja, V. (2017). High pressure processing: a potential technology for processing and preservation of dairy foods. Int. J. Curr Microbiol. Applied Sci., 6, 3526–3535. doi: 10.20546/ijcmas.2017.612.410
Penchalaraju, M., and Shireesha, B. (2013). Preservation of foods by HPP A review. J Sci. Res. Tech, 1, 30–38. Retrieved from http://www.indjsrt.com
Pendyala, B., Patras, A., Sudhir Gopisetty, V. V., and Sasges, M. (2021). UV-C inactivation of microorganisms in a highly opaque model fluid using a pilot scale ultra-thin film annular reactor: Validation of delivered dose. J. Food Eng., 294, 110403. doi: 10.1016/j.jfoodeng.2020.110403
Pereira, R. N., and Vicente, A. A. (2010). Environmental impact of novel thermal and non-thermal technologies in food processing. Food Res. Int., 43, 1936–1943. doi: 10.1016/j.foodres.2009.09.013
Pereira, R. V., Bicalho, M. L., Machado, V. S., Lima, S., Teixeira, A. G., Warnick, L. D., et al. (2014). Evaluation of the effects of ultraviolet light on bacterial contaminants inoculated into whole milk and colostrum, and on colostrum immunoglobulin G. J. Dairy Sci., 97, 2866–287. doi: 10.3168/jds.2013-7601
Rathod, N. B., Kahar, S. P., Ranveer, R. C., and Annapure, U. S. (2021). Cold plasma an emerging nonthermal technology for milk and milk products: a review. Int. J. Dairy Technol. 74, 615–626. doi: 10.1111/1471-0307.12771
Ribeiro, H. S., Janssen, J. J., Kobayashi, I., and Nakajima, M. (2010). Membrane emulsification for food applications. Membrane technology. Membranes Food Appl. 3, 129–166. doi: 10.1002/9783527631384.ch7
Ribeiro, K. C., Coutinho, N. M., Silveira, M. R., Rocha, R. S., Arruda, H. S., Pastore, G. M., et al. (2021). Impact of cold plasma on the techno-functional and sensory properties of whey dairy beverage added with xylooligosaccharide. Food Res. Int. 142, 110232. doi: 10.1016/j.foodres.2021.110232
Ribeiro, N. G., Xavier-Santos, D., Campelo, P. H., Guimarães, J. T., Pimentel, T. C., Duarte, M. C. K., et al. (2022). Dairy foods and novel thermal and non-thermal processing: A bibliometric analysis. Innov. Food Sci. Emerg. Technol. 76, 102934. doi: 10.1016/j.ifset.2022.102934
Riener, J., Noci, F., Cronin, D. A., Morgan, D. J., and Lyng, J. G. (2009). Effect of high intensity pulsed electric fields on enzymes and vitamins in bovine raw milk. Int. J. Dairy Technol. 62, 1–6. doi: 10.1111/j.1471-0307.2008.00435.x
Rodriguez-Gonzalez, O., Buckow, R., Koutchma, T., and Balasubramaniam, V. M. (2015). Energy requirements for alternative food processing technologies-principles, assumptions, and evaluation of efficiency. Compr. Rev. Food Sci. Food Safety, 14, 536–554. doi: 10.1111/1541-4337.12142
Rossitto, P. V., Cullor, J. S., Crook, J., Parko, J., Sechi, P., and Cenci-Goga, B. T. (2012). Effects of UV irradiation in a continuous turbulent flow uv reactor on microbiological and sensory characteristics of cow's milk. J. Food Protect. 75, 2197–2207. doi: 10.4315/0362-028X.JFP-12-036
Schiano, A. N., and Drake, M. A. (2021). Consumer understanding of fluid milk and cheese processing and composition. J. Dairy Sci., 104, 8644–8660. doi: 10.3168/jds.2020-20057
Schottroff, F., Gratz, M., Krottenthaler, A., Johnson, N. B., Bedard, M. F., and Jaeger, H. (2019). Pulsed electric field preservation of liquid whey protein formulations – influence of process parameters, PH, and protein content on the inactivation of listeria innocua and the retention of bioactive ingredients. J. Food Eng. 243, 142–152. doi: 10.1016/j.jfoodeng.2018.09.003
Segat, A., Misra, N. N., Cullen, P. J., and Innocente, N. (2016). Effect of Atmospheric Pressure Cold Plasma (ACP) on activity and structure of alkaline phosphatase. Food Bioprod Process. 98, 181–188. doi: 10.1016/j.fbp.2016.01.010
Shabbir, M. A., Ahmed, H., Maan, A. A., Rehman, A., Afraz, M. T., Iqbal, M. W., et al. (2021). Effect of non-thermal processing techniques on pathogenic and spoilage microorganisms of milk and milk products. Food Sci. Technol. 41, 279–294.
Shabbir, M. A., Haassan, A., Abid Aslam, M., Rehman, A., Afraz, M. T., Iqbal, M. W., et al. (2020). Effect of non-thermal processing techniques on pathogenic and spoilage microorganisms of milk and milk products. Food Sci. Technol. 41, 279–294. doi: 10.1590/fst.05820
Shao, Y., Zhu, S., Ramaswamy, H., and Marcotte, M. (2010). Compression heating and temperature control for high-pressure destruction of bacterial spores: an experimental method for kinetics evaluation. Food Bioprocess Technol. 3, 71–78. doi: 10.1007/s11947-008-0057-y
Sharma, P., Bremer, P., Oey, I., and Everett, D. W. (2014a). Bacterial inactivation in whole milk using pulsed electric field processing. Int. Dairy J. 35, 49–56. doi: 10.1016/j.idairyj.2013.10.005
Sharma, P., Oey, I., Bremer, P., and Everett, D. W. (2014b). Reduction of bacterial counts and inactivation of enzymes in bovine whole milk using pulsed electric fields.” Int. Dairy J., 39, 146–56. doi: 10.1016/j.idairyj.2014.06.003
Sharma, P., Oey, I., and Everett, D. W. (2014c). Effect of pulsed electric field processing on the functional properties of bovine milk. Trends Food Sci. Technol. 35, 87–101. doi: 10.1016/j.tifs.2013.11.004
Sharma, P., Oey, I., and Everett, D. W. (2016). Thermal properties of milk fat, xanthine oxidase, caseins and whey proteins in pulsed electric field-treated bovine whole milk. Food Chem. 207, 34–42. doi: 10.1016/j.foodchem.2016.03.076
Sharma, S., and Singh, R. K. (2020). Cold plasma treatment of dairy proteins in relation to functionality enhancement. Trends Food Sci. Technol. 102, 30–36. doi: 10.1016/j.tifs.2020.05.013
Shin, J., Kim, S., Kim, D., and Kang, D. (2016). Fundamental characteristics of deep-UV light-emitting diodes and their application to control foodborne pathogens. Appl Environ Microbiol. 82, 2–10. doi: 10.1128/AEM.01186-15
Shrivastava, S. L., and Banerjee, S. (2017). Recent trends in milk processing—a short review. Approaches Poultry, Dairy Vet. Sci. 2, 11–13. doi: 10.31031/APDV.2017.02.000527
Silva, F. V.. (2020). Ultrasound assisted thermal inactivation of spores in foods: Pathogenic and spoilage bacteria, molds and yeasts. Trend Food Sci. Technol. 105, 402–415. doi: 10.1016/j.tifs.2020.09.020
Silveira, M. R., Coutinho, N. M., Esmerino, E. A., Moraes, J., Fernandes, L. M., Pimentel, T. C., et al. (2019). Guava-flavored whey beverage processed by cold plasma technology: bioactive compounds, fatty acid profile and volatile compounds. Food Chem. 279, 120–127. doi: 10.1016/j.foodchem.2018.11.128
Simonis, P., Kersulis, S., Stankevich, V., Sinkevic, K., Striguniene, K., Ragoza, G., et al. (2019). Pulsed electric field effects on inactivation of microorganisms in acid whey. Int. J. Food Microbiol. 291, 128–134. doi: 10.1016/j.ijfoodmicro.2018.11.024
Singh, H., Bhardwaj, S. K., Khatri, M., Kim, K. H., and Bhardwaj, N. (2021). UVC radiation for food safety: An emerging technology for the microbial disinfection of food products. Chemical Eng. J., 417, 128084. doi: 10.1016/j.cej.2020.128084
Skrzypek, M., and Burger, M. (2010). Isoflux® ceramic membranes—practical experiences in dairy industry. Desalination, 250, 1095–1100. doi: 10.1016/j.desal.2009.09.116
Smithers, G. W., and Augustin, M. A. (Eds). (2012). Advances in Dairy Ingredients (79th ed.). Hoboken: Wiley. doi: 10.1002/9781118448205
Soltanzadeh, M., Peighambardoust, S. H., Gullon, P., Hesari, J., Gullón, B., Alirezalu, K., et al. (2020). Quality Aspects and Safety of Pulsed Electric Field (PEF) processing on dairy products: a comprehensive review. Food Rev. Int. 1–22. doi: 10.1080/87559129.2020.1849273
Stratakos, A. C., Inguglia, E. S., Linton, M., Tollerton, J., Murphy, L., Corcionivoschi, N., et al. (2019). Effect of high pressure processing on the safety, shelf life and quality of raw milk. Innov. Food Sci. Emerg. Technol. 52, 325–333. doi: 10.1016/j.ifset.2019.01.009
Surowsky, B., Schlüter, O., and Knorr, D. (2015). Interactions of non-thermal atmospheric pressure plasma with solid and liquid food systems: a review. Food Eng. Reviews 7, 82–108. doi: 10.1007/s12393-014-9088-5
Tomasula, P. M., Mukhopadhyay, S., Datta, N., Porto-Fett, A., Call, J. E., Luchansky, J. B., et al. (2011). Pilot-scale crossflow-microfiltration and pasteurization to remove spores of Bacillus anthracis (Sterne) from milk. J. Dairy Sci., 94, 4277–4291. doi: 10.3168/jds.2010-3879
Tsevdou, M., Ouli-Rousi, M., Soukoulis, C., and Taoukis, P. (2020). Impact of high-pressure process on probiotics: Viability kinetics and evaluation of the quality characteristics of probiotic yoghurt. Foods. 9:360. doi: 10.3390/foods9030360
Tsevdou, M., and Taoukis, P. (2011). Effect of non-thermal processing by high hydrostatic pressure on the survival of probiotic microorganisms: study on Bifidobacteria spp. Anaerobe, 17, 456–458. doi: 10.1016/j.anaerobe.2011.06.007
Tuorila, H., and Hartmann, C. (2020). Consumer responses to novel and unfamiliar foods. Curr Opin. Food Sci. 33, 1–8. doi: 10.1016/j.cofs.2019.09.004
Valdramidis, V. P., and Koutsoumanis, K. P. (2016). Challenges and perspectives of advanced technologies in processing, distribution and storage for improving food safety. Curr Opin. Food Sci. 12, 63–69. doi: 10.1016/j.cofs.2016.08.008
Walkling-Ribeiro, M., Rodríguez-González, O., Jayaram, S., and Griffiths, M. W. (2011). Microbial inactivation and shelf life comparison of ‘cold' hurdle processing with pulsed electric fields and microfiltration, and conventional thermal pasteurisation in skim milk. Int. J. Food Microbiol. 144, 379–386. doi: 10.1016/j.ijfoodmicro.2010.10.023
Wang, D., Fritsch, J., and Moraru, C. I. (2019). Shelf life and quality of skim milk processed by cold microfiltration with a 1.4-μm pore size membrane, with or without heat treatment. J. Dairy Sci., 102, 8798–8806. doi: 10.3168/jds.2018-16050
Ward, D. M., Patras, A., Kilonzo-Nthenge, A., Yannam, S. K., Pan, C., Xiao, H., et al. (2019). UV-C treatment on the safety of skim milk: effect on microbial inactivation and cytotoxicity evaluation. J. Food Process Eng., 42, e12944. doi: 10.1111/jfpe.12944
Wesolowska, A., Sinkiewicz-Darol, E., Barbarska, O., Strom, K., Rutkowska, M., Karzel, K., et al. (2018). New achievements in high-pressure processing to preserve human milk bioactivity. Front Pediatr. 6, 323. doi: 10.3389/fped.2018.00323
Wu, X., Luo, Y., Zhao, F., Murad, M. S., and Mu, G. (2021). Influence of dielectric barrier discharge cold plasma on physicochemical property of milk for sterilization. Plasma Process. Polym. 18, 1900219. doi: 10.1002/ppap.201900219
Zhang, S., Yang, R., Zhao, W., Hua, X., Zhang, W., and Zhang, Z. (2011). Influence of pulsed electric field treatments on the volatile compounds of milk in comparison with pasteurized processing. J. Food Sci. 76, C127–C132. doi: 10.1111/j.1750-3841.2010.01916.x
Keywords: non-thermal dairy, safety, quality, UV-C, mild-processing, microbiology, functionality
Citation: Neoκleous I, Tarapata J and Papademas P (2022) Non-thermal Processing Technologies for Dairy Products: Their Effect on Safety and Quality Characteristics. Front. Sustain. Food Syst. 6:856199. doi: 10.3389/fsufs.2022.856199
Received: 16 January 2022; Accepted: 18 April 2022;
Published: 19 May 2022.
Edited by:
Sajid Maqsood, United Arab Emirates University, United Arab EmiratesReviewed by:
Veronica Ortiz Alvarenga, Federal University of Minas Gerais, BrazilAdriano Gomes Cruz, Adriano Gomes da Cruz, Brazil
Vesna Radovanović, Educons University, Serbia
Copyright © 2022 Neoκleous, Tarapata and Papademas. This is an open-access article distributed under the terms of the Creative Commons Attribution License (CC BY). The use, distribution or reproduction in other forums is permitted, provided the original author(s) and the copyright owner(s) are credited and that the original publication in this journal is cited, in accordance with accepted academic practice. No use, distribution or reproduction is permitted which does not comply with these terms.
*Correspondence: Photis Papademas, cGhvdGlzLnBhcGFkZW1hcyYjeDAwMDQwO2N1dC5hYy5jeQ==