- 1Departamento de Química, Faculdade de Filosofia Ciências e Letras de Ribeirão Preto (FFCLRP), Universidade de São Paulo, Ribeirão Preto, Brazil
- 2UNESP, National Institute for Alternative Technologies of Detection, Toxicological Evaluation and Removal of Micropollutants and Radioactives (INCT-DATREM), Institute of Chemistry, Araraquara, Brazil
Ever-increasing biofuel production has raised the supply of glycerol, an abundant waste from ethanolic fermentation and transesterification, for biodiesel production. Glycerol can be a starting material for sustainable production of 1,3-propanediol (1,3 PD), a valued polymer subunit. Here, we compare how Clostridium pasteurianum DSMZ 525, a well-known 1,3-PD-producer, and the non-solventogenic Clostridium beijerinckii Br21 perform during glycerol fermentation. Fermentative assays in 80-, 390-, or 1,100-mM glycerol revealed higher 1,3-PD productivity by DSMZ 525 compared to Br21. The highest 1,3-PD productivities by DSMZ 525 and Br21 were obtained in 390 mM glycerol: 3.01 and 1.70 mM h−1, respectively. Glycerol uptake by the microorganisms differed significantly: C. beijerinckii Br21 consumed 41.1, 22.3, and 16.3%, while C. pasteurianum consumed 93, 44.5, and 14% of the initial glycerol concentration in 80, 390, and 1,100 mM glycerol, respectively. In 1,100 mM glycerol, C. beijerinckii Br21 growth was delayed. Besides 1,3-PD, we detected butyrate and acetate during glycerol fermentation by both strains. However, at 80 mM glycerol, C. beijerinckii Br21 formed only butyrate as the by-product, which could help downstream processing of the 1,3-PD fermentation broth. Therefore, C. beijerinckii Br21, an unexplored biocatalyst so far, can be used to convert glycerol to 1,3-PD and can be applied in biofuel biorefineries.
Introduction
Glycerol (propane-1,2,3-triol) is a by-product of ethanolic fermentation and biodiesel transesterification. Glycerol generation represents 10% of the weight of the transesterification reaction products from biodiesel manufacture Checa et al., 2020). In 2021, biodiesel production worldwide will generate ~1,600 million tons of residual glycerol (OECD/FAO, 2020).
In Brazil, the percentage of mandatory biodiesel addition to fossil diesel was increased from 10 to 12% in 2020, which represents ~ 800 million tons of residual glycerol (Analysis of Biofuels' Current Outlook, 2020). In addition, during ethanolic fermentation, 3–5% carbon from ethanol is diverted to glycerol generation (Chen and Liu, 2016; Mutton et al., 2019). Thus, the ever-growing demand for biofuels has increased the amount of glycerol as the by-product. Converting glycerol to a value-added product helps to make bioethanol and/or biodiesel biorefinery feasible (Chen and Liu, 2016).
Glycerol has numerous applications. Depending on its purity, it is used for pharmaceutical, cosmetic, and energy purposes. In biotechnological processes, glycerol can be employed as a carbon source and be converted to 1,3-propanediol (1,3-PD), a polymer backbone obtained by fermentation catalyzed mainly by the genera Clostridium and Klebsiella (Gungormusler et al., 2011; Chen and Liu, 2016; Uprety et al., 2017). Among clostridia, Clostridium pasteurianum (Zhang et al., 2021) and Clostridium butirycum (Martins et al., 2020) are the most studied species. The well-known clostridial glycerol conversion to 1,3-PD comprises two interdependent stages: the oxidative stage, when energy for growth is gained, and the reductive stage, when reduced coenzymes are oxidized upon 1,3-PD evolution (Gungormusler et al., 2011; Uprety et al., 2017). During the oxidative stage, glycerol is oxidized by glycerol dehydrogenase and phosphorylated by dihydroxyacetone kinase, forming dihydroxyacetone phosphate, which is followed by glycolysis (Uprety et al., 2017; Zabed et al., 2019). During the metabolic pathways that depend on reducing equivalents (NAD(P)H), organic acids and solvents are generated as glycerol oxidation by-products. During the clostridial reductive stage, glycerol dehydratase (GDHt) dehydrates glycerol, generating 3-hydroxypropionaldehyde (3-HPA). 1,3 Propanediol oxidoreductase (PDDH) then reduces 3-HPA to 1,3-PD using dissipating reducing equivalents gained during the oxidative stage (Uprety et al., 2017; Zabed et al., 2019).
A mixture of organic acids is usually produced from glycerol during the Clostridium oxidative stage (Sarma et al., 2012). The maximum 1,3-PD yield is 0.6 mol per mol of glycerol when acetic and butyric acids are the by-products of glycerol oxidation (Equation 1).
Typically, Clostridium beijerinckii is a solventogenic clostridial species, i.e., it ferments substrates by initially producing organic acids, and then it reabsorbs the acids to generate the solvents acetone, butanol, and ethanol in the so-called ABE fermentation (Zhao et al., 2011). Nevertheless, in our laboratory, we have isolated the non-solventogenic C. beijerinckii Br21 strain (Fonseca et al., 2016, 2019), which cannot reabsorb organic acids to produce the solvents. This causes the organic acids, especially butyric acid, to accumulate in the medium, and ABE fermentation does not occur (Fonseca et al., 2020). A non-solventogenic C. beijerinckii strain has never been studied for 1,3-PD production from glycerol. Supposedly, lack of solventogenesis by strain Br21 would help it to save reducing equivalents required for 1,3-PD evolution.
Therefore, this investigation explores the potential of the non-solventogenic C. beijerinckii Br21 to produce 1,3-PD from glycerol. We compare the well-known 1,3-PD-producer C. pasteurianum DSMZ 525 with our isolate C. beijerinckii Br21 in terms of the glycerol fermentation by-product profile and shed some light on glycerol metabolism by a non-solventogenic C. beijerinckii strain.
Materials and Methods
Microorganisms
The microorganisms C. beijerinckii Br21 GenBank KT626859, a non-solventogenic strain (Fonseca et al., 2019), and C. pasteurianum DSMZ 525, the strain obtained from the culture collection of Deutsche Sammlung von Mikroorganismen und Zellkulturen, were used.
Cells were maintained as a glycerol (20%, w v−1) stock, which was prepared after the cells were grown to an optical density of 0.8, and were stored at −80°C. Before the fermentative assays, the stock cultures were transferred to a fresh reinforced Clostridium medium (RCM, Sigma-Aldrich) to activate the cells.
Culture Medium
Culture media with 80, 390, or 1,000 mM glycerol were prepared. The media consisted of glycerol, yeast extract (1.0 g L−1), Na2HPO4 (5.0 g L−1), KH2PO4 (1.0 g L−1), NaCl (1.0 g L−1), MgSO4.7H2O (0.1 g L−1), FeSO4 (0.045 g L−1), 1.0 mL L−1 resazurin solution (1.0 g L−1), and 2.0 mL L−1 trace elements solution containing H3BO3 (2.85 g L−1), MnSO4.4H2O (2.03 g L−1), and FeCl3 (0.167 g L−1) (Chen et al., 2005). The medium pH was adjusted to 7.0. Nitrogen gas was bubbled through 100-mL flasks filled with 56 mL of medium for 3 min to ensure anaerobiosis. The flasks were sealed and autoclaved at 121°C and 1 atm for 20 min.
Batch Fermentation Assays
Both microorganisms stored at −80°C were activated before the fermentation assays. The stored microorganism suspension was resuspended in RCM in 50-mL flasks filled with 15 mL of RCM and cultivated at 35°C and 125 rpm for 24 h. The pre-inoculum was prepared by resuspending 4 mL of the activated culture in 100-mL flasks containing 56 mL of medium and incubated at 35°C and 125 rpm overnight. The volume of the last culture was calculated so that the initial optical density (OD) at 600 nm would be 0.5 at the beginning of the fermentation assays. With a syringe, the inoculum was introduced into the fermentative flasks, which had already been bubbled with nitrogen gas during medium preparation (section Culture Medium) to ensure anaerobiosis. An OD of 0.5 at 600 nm represents a cell concentration of 0.01 mg mL−1 (Fonseca et al., 2016). Fermentations were carried out at 35°C and 125 rpm for 25 h.
Periodically, 2 mL of the sample was taken with a syringe under sterile conditions for pH and OD (600 nm) measurements. Samples were centrifuged at 13,400 rpm for 1.5 min, filtered through a 0.22-μm nylon filter, and stored at −18°C until analytical measurements were accomplished.
Kinetic Parameters
The substrate conversion factor in cells (YX/S) was calculated as the ratio of the variation in cell concentration (X) at the beginning (Xi) and end (Xf) of fermentation [measured as OD and converted to dry mass (mg L−1)] to the glycerol (S) consumption [difference between the initial (Si) and final (Sf) concentration (Equation 3)].
The product yield from glycerol consumption (YP/S) was calculated using Equation 4, where P is 1,3-PD, butyrate, or acetate (mM), and S is the glycerol concentration (mM).
Productivity (P) was calculated by considering the variation in 1,3-PD concentration (mM) and fermentation time (Equation 5).
Finally, carbon balance (C) was calculated by considering the moles of carbon from consumed glycerol (C-glycerol) diverted to products (C-products). 1,3-PD, butyric acid, acetic acid, and cell, considering the cell formula as C4H7O2N (Biebl, 2001), were counted as products. The percent ratio between the sum of carbon in the products and the carbon in consumed glycerol corresponded to total carbon recovery in the products in the liquid phase (Equation 6).
Analytical Methods
Substrate and product concentrations during the fermentation assays were determined by gas chromatography (GC, SHIMADZU – 2014, Shimadzu, Tokyo, Japan) coupled with a flame ionization detector (FID). Separation was performed on a Stabilwax®-DA capillary column (30 m x 0.25 mm I.D., 0.25-mm film thickness, Shimadzu, Kyoto, Japan). Sample preparation comprised its dilution in ethanol (1:3 v v−1) according to Egoburo et al. (2017). The chromatograph detector temperature was initially set at 185 °C for 3 min, increased to 220°C at 40°C min−1, and maintained at 220°C for 1 min. Then, the injector and the detector temperatures were kept at 290 and 300°C, respectively. The carrier gas was nitrogen at a flow rate of 2.5 mL min−1. The GC-FID injected volume was 2.0 μL in a 1/30 split ratio. The interval between the analyses was 3 min. Ethanol (GC grade, Sigma Saint Louis, USA) was used for both sample preparation and column cleaning. Retention times were given by an analytical curve for all the analytes, and peak areas were calculated with the GC-Solutions software.
Statistical Methods
All the fermentative assays were carried out as independent triplicates. To compare the results of the fermentation tests, two-factor analysis of variance (ANOVA) analysis and Tukey's test at 5% significance were performed with the software Statistica 7.0. The two factors considered were initial glycerol concentration and the microorganism.
Results and Discussion
Effect of Glycerol Concentration on Growth
During batch fermentations, an increase in the initial substrate concentration might inhibit the substrate and decrease the product yield. On the other hand, low substrate concentration might diminish the biochemical reaction rates.
When we cultured C. beijerinckii Br21 (Figures 1A,B) and C. pasteurianum DSMZ 525 (Figures 1C,D) in 80, 390, or 1,100 mM glycerol, we verified that the glycerol concentration affected C. beijerinckii growth to a larger extent. Glycerol at 390 mM elicited the highest variation in C. beijerinckii Br21 cell concentration (Figure 1A). At the lowest (80 mM) and highest (1,100 mM) glycerol concentrations, strain Br21 grew to a lesser extent (Figure 1A), increasing to only 200.83 and 230.21 mg L−1 of dry mass, respectively. Furthermore, in 1,100 mM glycerol, the growth lag phase increased, ~ 15 h, compared to <2 h in 80 and 390 mM glycerol (Figure 1A). As for C. beijerinckii TISTR 1,390 in glycerol ranging from 20 to 60 g L−1 (217 and 651 mM, respectively), Sanguanchaipaiwong and Leksawasdi (2017) obtained the highest cell concentration in 20 g L−1 glycerol, but only after cultivation for 189 h. Wischral (2015) obtained the highest C. beijerinckii DSM 791 cell concentration in 8 g L−1 (87 mM) glycerol. During batch cultivation, our strain grew to a larger extent in 390 mM glycerol (Figure 1A).
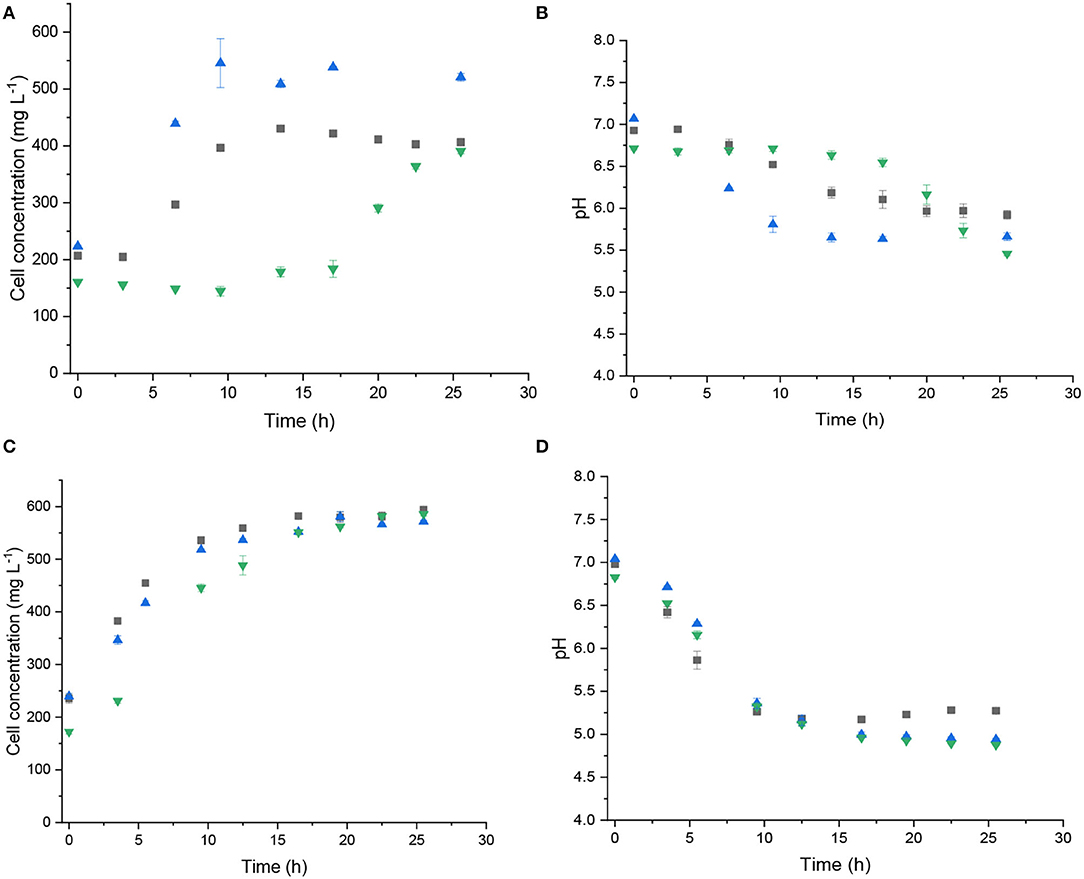
Figure 1. Cell concentration and pH during glycerol batch fermentations with C. beijerinckii Br21 (A,B) or C. pasteurianum DSMZ 525 (C,D). Glycerol at 80 mM (■), 390 mM (), and 1,100 mM (
).
Clostridium growth is associated with organic acid generation, mainly acetic and butyric acids, which decreases the pH of the cultivation medium (Zeng and Biebl, 2002). Irrespective of glycerol concentration, C. beijerinckii Br21 had similar pH variations, around 1.4 units, which lowered the pH to 5.68 ± 0.20 (Figure 1B).
For C. pasteurianum DSMZ 525, cell growth and the pH profile were similar in all glycerol concentrations, 80, 390, or 1,100 mM (Figures 1C,D). The highest glycerol concentration (1,100 mM) did not inhibit C. pasteurianum DSMZ 525 growth. However, under the same conditions, 1,100 mM glycerol inhibited C. beijerinckii Br21 growth: the lag phase increased to ~15 h. The lag phase also resembled the lag phase in other glycerol concentrations. Biebl (2001) reported inhibited growth for strain DSMZ 525 at glycerol concentrations higher than 60 g L−1 (~650 mM). However, this author kept the fermentation pH at 6.0, whereas here we did not control the pH. The initial pH was 7.0 and decreased to 5.03 (± 0.25) during fermentation at all the glycerol concentrations.
Sarchami et al. (2016) evaluated 1,3-PD production by C. pasteurianum DSMZ 525 in 330 mM glycerol at different pH: 4.7, 5.0, 5.3, 5.6, or 5.9. The lower the pH, the lesser the extent of cell growth and fermentation, which guided metabolism to butanol instead of 1,3-PD formation. In contrast, fermentation at higher pH allowed higher cell growth and favored 1,3-PD generation. The pH range, between 7.0 and 5.2, of our fermentations (Figure 1D) helped C. pasteurianum DSMZ 525 grow and probably did not inhibit 1,3-PD production.
To alleviate substrate inhibition, cell immobilization, fed-batch operation, and continuous operation have been successfully employed to convert glycerol to 1,3-PD. Continuous 1,3-PD production in the presence of C. beijerinckii NRRL B-593 and 40 g L−1 crude glycerol increased glycerol consumption to 74 and 100% in a system with suspended and immobilized cells, respectively (Gungormusler et al., 2011). Compared to a batch approach, fed-batch enhanced 1,3-PD production from glycerol by C. beijerinckii A1 from 18.9 to 26 g L−1 (Wischral et al., 2016).
Effect of Glycerol Concentration on Fermentation Products Profile
Initial substrate concentration can elicit different microorganism metabolisms, as revealed by the product profiles. Glycerol consumption and 1,3-PD, acetate, and butyrate formation by C. beijerinckii Br21 and C. pasteurianum DSMZ 525 (Figures 2A–F) were different, particularly the glycerol uptake.
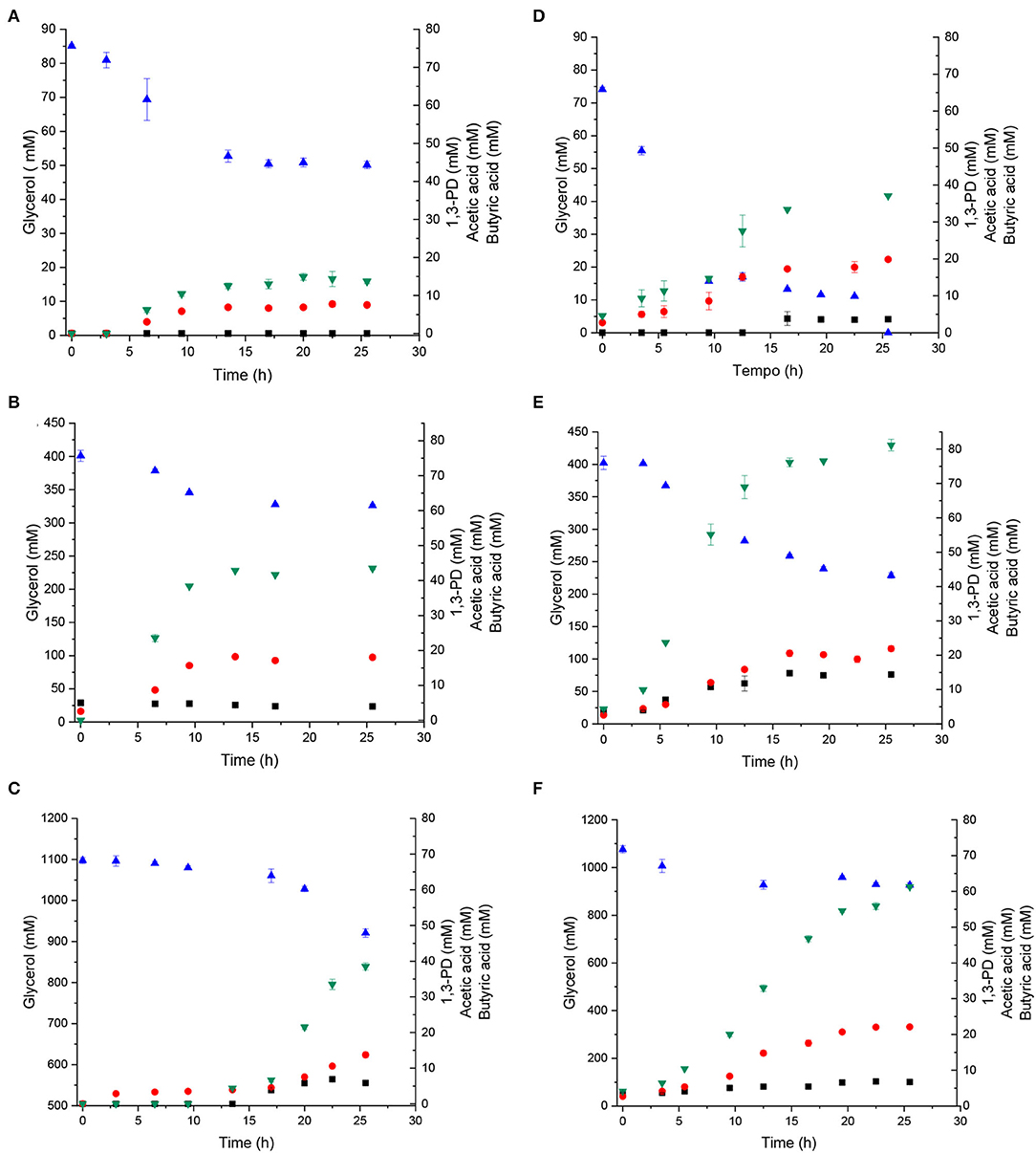
Figure 2. Glycerol and product concentrations during glycerol fermentation by C. beijerinckii Br21 (A–C) and C. pasteurianum DSMZ 525 (D–F). Glycerol at 80 mM (A,D), 390 mM (B,E), and 1,100 mM (C,F). Glycerol (), 1,3-PD (
), acetate (■), butyrate (
).
C. beijerinckii Br21 consumed less glycerol than C. pasteurianum DSMZ 525: 41.1, 22.3, and 16.3% and 93, 44.5, and 14% in 80, 390, and 1,100 mM glycerol, respectively. Low pH could affect glycerol consumption by C. beijerinckii Br21, as observed for xylose fermentation by the same strain (Fonseca et al., 2020). Low pH increases the concentration of non-dissociated organic acids, which are stronger inhibitors than the corresponding ionized species. Total strain Br21 inhibition was observed at 10 mM of total non-dissociated acid (Fonseca et al., 2020). Therefore, the non-dissociated butyric or acetic acid concentration at ~5 mM during 390 mM glycerol fermentation could contribute to inhibiting Clostridium metabolism. Furthermore, strain Br21 seemed to be more affected by pH drop than C. pasteurianum.
Besides pH, other factors might have caused low glycerol consumption by C. beijerinckii Br21. Especially at 1,100 mM glycerol and after fermentation for 20 h, the pH remained about 6.0 (Figure 1). In this condition, the non-dissociated butyric acid concentration was about 1.5 mM of the total non-dissociated acid, which is considered not inhibitory. Probably, extended fermentation could be conducted to increase glycerol consumption and 1,3-PD concentration.
Both C. beijerinckii Br21 and C. pasteurianum DSMZ 525 in 390 mM glycerol provided the highest 1,3-PD titer, 43.47 and 76.75 mM, respectively (Table 1). Thus, intermediate glycerol concentration favored 1,3-PD production by both the microorganisms. Indeed, glycerol concentrations ranging from 270 to 540 mM and from 87 to 330 mM have been described to allow 1,3-PD production by C. pasteurianum (Biebl, 2001; Sarchami et al., 2016; Gallardo et al., 2017) and C. beijerinckii (Wischral, 2015; Wischral et al., 2016) strains, respectively. These concentrations are in the same order of magnitude as the ones we obtained with C. beijerinckii Br 21, a non-solventogenic strain, reported for 1,3-PD production for the first time herein.
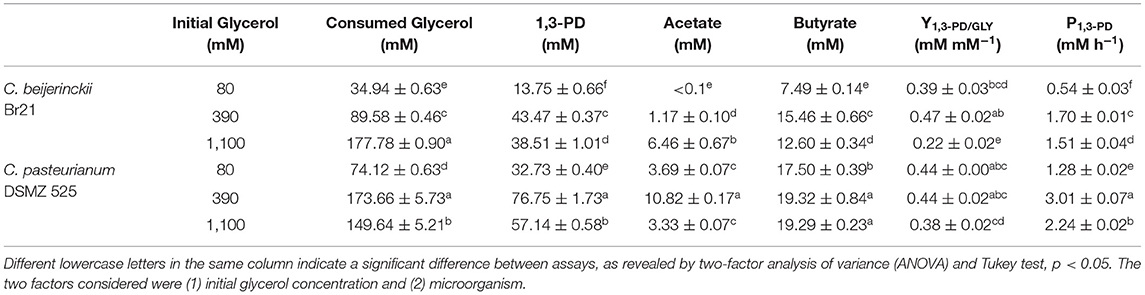
Table 1. Glycerol consumption, product concentrations, yields, and fermentation productivities by C. beijerinckii Br21 and C. pasteurianum DMSZ 525 in different initial glycerol concentrations.
Glycerol conversion to 1,3-PD gives acetic acid and butyric acid as by-products (Equation 1) (Tong and Cameron, 1992). The major by-product from glycerol fermentation by strain Br21 was butyric acid, and its formation was associated with 1,3-PD (Equation 1). The same glycerol concentration (390 mM) that afforded the highest 1,3-PD yield also gave the highest butyric acid concentration (15.46 mM) (Figure 2B). In the case of glycerol fermentation by C. beijerinckii in 1,100 mM glycerol, we detected 1,3-PD and organic acids only 15 h after the fermentation started, corresponding to the microorganism growth profile. In 1,100 mM glycerol, fermentation was slower and 1,3-PD productivity decreased (Table 1).
Acetic acid was detected during fermentation by C. beijerinckii Br21 only in 390 and 1,100 mM glycerol at 1.17 and 6.46 mM, respectively. However, these concentrations diminished along with fermentation. The fact that strain Br21 can consume acetate and form butyrate has already been observed in our previous works (Fonseca et al., 2021).
Substrate consumption does not necessarily indicate 1,3-PD production. Fermentations by C. beijerinckii Br21 in 80 and 1,100 mM glycerol produced 13.75 and 38.51 mM 1,3-PD, or a yield (Y1, 3−PD/G) of 0.39 and 0.22 mol mol−1, respectively (Table 1). On the other hand, 390 mM glycerol fermentation by C. beijerinckii Br21 resulted in 43.47 mM 1,3-PD, leading to a yield of 0.47 mol mol−1. In 390 mM glycerol, C. beijerinckii converted glycerol to 1,3-PD as efficiently as C. pasteurianum: 0.47 (±0.02) and 0.44 (±0.02) mol of 1,3-PD per mol of glycerol, respectively.
As predicted by the theoretical reaction (Equation 1), the formation of a mixture of butyric and acetic acids is common during 1,3-PD production (Biebl et al., 1992). When acetic acid was the only metabolite, the 1,3-PD yield decreased to 0.75 mol mol−1 glycerol, (Equation 2), and when both acetic acid and butyric acid were the by-products, the 1,3-PD yield decreased to 0.60 mol mol−1 glycerol (Equation 1). Total organic acid concentrations in fermentation by C. pasteurianum might have lowered its 1,3-PD yield from glycerol (Table 1). Carbon mass balance from the fermentations also suggested that acetic acid was the main difference between the metabolites obtained in the presence of both strains (Figure 3). As expected, carbon recovery was near 75% in most fermentations, given that we did not detect CO2 release during glycerol oxidation (Figure 3).
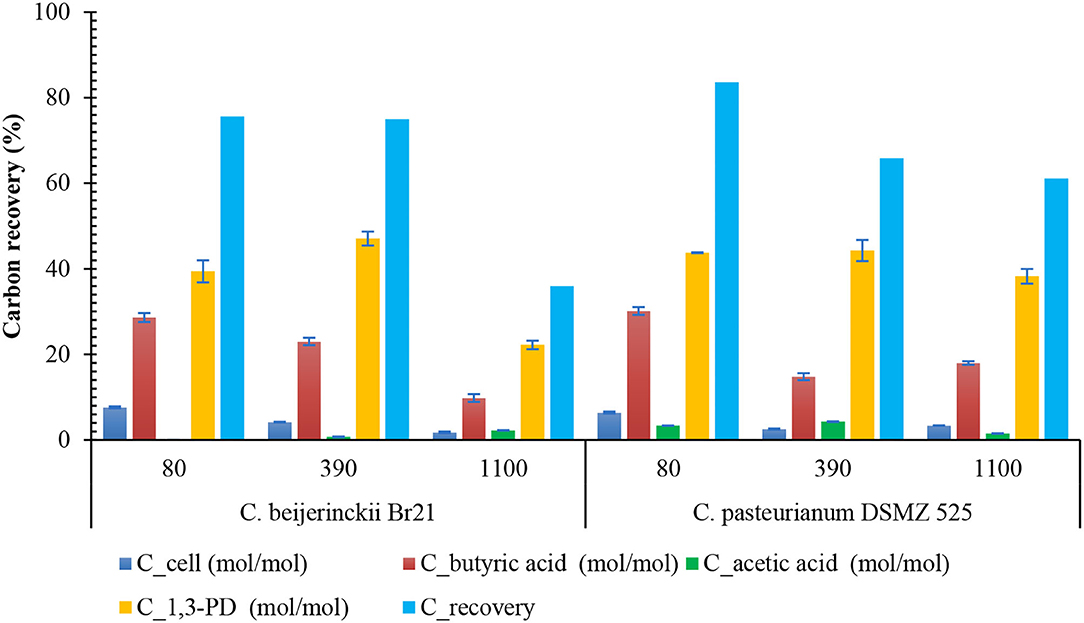
Figure 3. Carbon balance of fermentations with initial glycerol concentrations of 80, 390, or 1,100 mM by C. beijerinckii Br21 and C. pasteurianum DMSZ 525. Cell mass was considered as C4H7O2N (Biebl, 2001).
Regardless of the initial glycerol concentration, the ratios of glycerol conversion to 1,3-PD (YP/S) were similar and around 0.44 mM for C. pasteurianum and glycerol-dependent for C. beijerinckii Br21. At 80 and 390 mM glycerol, YP/S values were the same for both strains. However, C. beijerinckii Br21 was inhibited at 1,100 mM glycerol within 25 h of fermentation (Table 1).
Moreover, C. pasteunianum afforded higher 1,3-PD productivities than C. beijerinckii Br21: 3.01 and 2.24 mM h−1 and 1.70 and 1.51 mM h−1 of 1,3-PD in 390 and 1,100 mM glycerol, respectively (Table 1). In the literature, 1,3-PD productivity achieved with C. pasteurianum varies widely from 2.9 up to 8.8 mM h−1 of 1,3-PD (Moon et al., 2011; Gallardo et al., 2017), in the same range as ours. However, C. beijerinckii productivities covered a smaller range of 0.42–0.71 g L−1 h−1 when pure glycerol was used (Wischral et al., 2016). Here, we attained 0.04 and 0.13 g L−1 h−1 in 80 and 390 mM glycerol, respectively. Low 1,3-PD productivity by strain Br21 might be related to small substrate consumption, which did not exceed 50%.
C. pasteurianum DSMZ 525 afforded higher 1,3-PD concentrations and hence higher 1,3-PD productivities. However, C. beijerinckii Br21 provided similar glycerol conversion to 1,3-PD (Y1, 3−PD/GLY), especially in 390 mM glycerol. We were not able to prove our assumption that the lack of solventogenesis by strain Br21 saves electrons, which could be directed to the reductive 1,3-PD generation stage. Nevertheless, we did not detect ABE solvents in fermentations with C. beijerinckii Br21 or C. pasteurianum. Such an effect might be related to the substrate. ABE fermentation is more favored with glucose as the substrate, its oxidation gives 24 electrons, while glycerol oxidation provides 14 electrons (Wischral et al., 2016).
Although C. pasteurianum DSMZ 525 is a solventogenic strain, butanol formation demands longer fermentations. According to Malaviya et al. (2012) and Khanna et al. (2013), glycerol fermentation into butanol is better after fermentation for 2 and 7 days of fermentation, respectively. Indeed, Taconi et al. (2009) reported maximum butanol concentration of 7 g L−1 with an initial glycerol concentration of 25 g L−1 only after fermentation for 10 days. Thus, the short fermentation period (25 h) used here may have led to the lack of butanol, even though the solventogenic DSMZ 525 strain was used.
Lack of solventogenesis did not result in a higher 1,3-PD yield by C. beijerinckii, but in fewer and lower concentrations of by-products as compared to C. pasteurianum (Figure 3). Acetic acid concentration, albeit low, was higher in the fermentations with C. pasteurianum (Table 1). On the other hand, only butyric acid was detected as a by-product of glycerol fermentation by C. beijerinckii at 80 mM glycerol. Downstream processes may become more economical because fewer by-products were produced by the strain Br21. Hence, maintaining the glycerol concentration between 80 and 390 mM during glycerol fermentation with C. beijerinckii Br21 would avoid inhibition as well as the formation of by-products.
Estimating the glycerol concentration that converges higher productivities and yields is important and supports the bioreactor operation. Fed-batch bioreactors or continuous reactors could be employed because they allow substrate concentration to be controlled. Thus, C. beijerinckii Br21 performance during fermentation could be further improved by controlling the glycerol supply by operating the bioreactor in a fed-batch or continuous mode.
Conclusion
C. beijerinckii Br21 is more affected by high glycerol concentration and consumes less glycerol than C. pasteurianum DSMZ 525. Glycerol at 390 mM favors 1,3-PD production by C. beijerinckii Br21 and C. pasteurianum. Butyric acid is the main by-product during glycerol fermentation by C. beijerinckii strain, which can help in the downstream process. 1,3-PD productivity could be improved by refining glycerol concentration and controlling pH during fed-batch fermentation, making C. beijerinckii Br21 an even more promising 1,3-PD producer.
Data Availability Statement
The original contributions presented in the study are included in the article/supplementary material, further inquiries can be directed to the corresponding author/s.
Author Contributions
AA and VR conceived the project and wrote the final manuscript. BCM and JB conducted the fermentation assays, substrate, and product analysis. All authors have read and approved the manuscript.
Funding
This work was supported by the Brazilian research funding agencies FAPESP (grants numbers 2021/01134-7, 2020/03168-3, and 2018/12471-1), Coordenação de Aperfeiçoamento de Pessoal de Nîvel Superior Brazil (CAPES) Finance Code 001, and National Council for Scientific and Technological Development Brazil (grant numbers 465571/2014-0 and 303817/2019-4).
Conflict of Interest
The authors declare that the research was conducted in the absence of any commercial or financial relationships that could be construed as a potential conflict of interest.
Publisher's Note
All claims expressed in this article are solely those of the authors and do not necessarily represent those of their affiliated organizations, or those of the publisher, the editors and the reviewers. Any product that may be evaluated in this article, or claim that may be made by its manufacturer, is not guaranteed or endorsed by the publisher.
Acknowledgments
The authors thank Cynthia Maria de Campos Prado Manso for review of the English language.
References
Analysis of Biofuels' Current Outlook (2020). Ministry of Mines and Energy. Available online at: https://www.epe.gov.br/sites-en/publicacoes-dados-abertos/publicacoes/Paginas/Analysis-Of-Biofuels-Current-Outlook-2020.aspx
Biebl, H. (2001). Fermentation of glycerol by Clostridium pasteurianum batch and continuous culture studies. J. Ind. Microbiol. Biotechnol. 27, 18–26. doi: 10.1038/sj.jim.7000155
Biebl, H., Marten, S., Hippe, H., and Deckwer, W. D. (1992). Glycerol conversion to 1,3-propanediol by newly isolated clostridia. Appl. Microbiol. Biotechnol. 36, 592–597. doi: 10.1007/BF00183234
Checa, M., Nogales-Delgado, S., Montes, V., and Encinar, J. M. (2020). Recent advances in glycerol catalytic valorization: a review. Catalysts 10, 1279. doi: 10.3390/catal10111279
Chen, W. M., Tseng, Z. J., Lee, K. S., and Chang, J. S. (2005). Fermentative hydrogen production with Clostridium butyricum CGS5 isolated from anaerobic sewage sludge. Int. J. Hydrog. Energy 30, 1063–1070. doi: 10.1016/j.ijhydene.2004.09.008
Chen, Z., and Liu, D. (2016). Toward glycerol biorefinery: metabolic engineering for the production of biofuels and chemicals from glycerol. Biotechnol. Biofuels 9, 205. doi: 10.1186/s13068-016-0625-8
Egoburo, D. E., Peña, R. D., Kolender, A., and Pettinari, M. J. (2017). Optimization and validation of a GC–FID method for quantitative determination of 1,3-propanediol in bacterial culture aqueous supernatants containing glycerol. Chromatographia. 80, 1121. doi: 10.1007/s10337-017-3310-6
Fonseca, B. C., Bortolucci, J., Silva, T. M., Passos, V. F., Gouvêa, P. F., Dinamarco, T. M., et al. (2020). Butyric acid as sole product from xylose fermentation by a non-solventogenic Clostridium beijerinckii strain under controlled pH and nutritional conditions. Bioresour. Technol. Rep. 10, 100426. doi: 10.1016/j.biteb.2020.100426
Fonseca, B. C., Guazzaroni, M. E., and Reginatto, V. (2016). Fermentative production of H2 from different concentrations of galactose by the new isolate Clostridium beijerinckii Br21. Int. J. Hydrog. Energy 41, 21109–21120. doi: 10.1016/j.ijhydene.2016.09.110
Fonseca, B. C., Reginatto, V., López-Linares, J. C., Lucas, S. M, García-Cubero, M. T., et al. (2021). Ideal conditions of microwave-assisted acid pretreatment of sugarcane straw allow fermentative butyric acid production without detoxification step. Bioresour. Technol. 329, 124929. doi: 10.1016/j.biortech.2021.124929
Fonseca, B. C., Riaño-Pachón, D. M., Guazzaroni, M. E., and Reginatto, V. (2019). Genome sequence of the H2-producing Clostridium beijerinckii strain Br21 isolated from a sugarcane vinasse treatment plant. Genet. Mol. Biol. 42, 139–144. doi: 10.1590/1678-4685-gmb-2017-0315
Gallardo, R., Alves, M., and Rodrigues, L. R. (2017). Influence of nutritional and operational parameters on the production of butanol or 1,3-propanediol from glycerol by a mutant Clostridium pasteurianum. New Biotechnol. 34, 59–67. doi: 10.1016/j.nbt.2016.03.002
Gungormusler, M., Gonen, C., and Azbar, N. (2011). Continuous production of 1,3-propanediol using raw glycerol with immobilized Clostridium beijerinckii NRRL B-593 in comparison to suspended culture. Bioprocess Biosyst. Eng. 34, 727–733. doi: 10.1007/s00449-011-0522-2
Khanna, S., Goyal, A., and Moholkar, V. S. (2013). Effect of fermentation parameters on bio-alcohols production from glycerol using immobilized Clostridium pasteurianum: an optimization study. Prep. Biochem. Biotechnol. 43, 828–847. doi: 10.1080/10826068.2013.805625
Malaviya, A., Jang, Y. S., and Lee, S. Y. (2012). Continuous butanol production with reduced byproducts formation from glycerol by a hyper producing mutant of Clostridium pasteurianum. Appl. Microbiol. Biotechnol. 93, 1485–1494. doi: 10.1007/s00253-011-3629-0
Martins, F. F., Liberato, V. S. S., Ribeiro, C. M. S., Coelho, M. A. Z., and Ferreira, T. F. (2020). Low-cost medium for 1,3-propanediol production from crude glycerol by Clostridium butyricum. Biofuels, Bioprod. Biorefin. 14, 1125–1134. doi: 10.1002/bbb.2133
Moon, C., Lee, C. H., Sang, B. I., and Um, Y. (2011). Optimization of medium compositions favoring butanol and 1,3-propanediol production from glycerol by Clostridium pasteurianum. Bioresour. Technol. 102, 10561–10568. doi: 10.1016/j.biortech.2011.08.094
Mutton, M. J. R., Ferrari, F. C. S., Freita, L. A., Freita, C. M., Andrietta, M. G. S., and Andrietta, S. R. (2019). Interaction between the production of ethanol and glycerol in fed-batch bioreactors. Braz. J. Microbiol. 50, 389–394. doi: 10.1007/s42770-019-00051-z
OECD/FAO (2020). OECD-FAO Agricultural Outlook 2020-2029. Paris; Rome: OECD Publishing; FAO. doi: 10.1787/1112c23b-en
Sanguanchaipaiwong, V., and Leksawasdi, N. (2017). Using glycerol as a sole carbon source for clostridium beijerinckii fermentation. Energ. Procedia. 138, 1105–1109. doi: 10.1016/j.egypro.2017.10.127
Sarchami, T., Johnson, E., and Rehmann, L. (2016). Optimization of fermentation condition favoring butanol production from glycerol by Clostridium pasteurianum DSM 525. Bio. Tech. 208, 73-80. doi: 10.1016/j.biortech.2016.02.062
Sarma, S. J., Brar, S. K., Sydney, E. B., Bihan, Y. L., Buelna, G., and Soccol, C. R. (2012). Microbial hydrogen production by bioconversion of crude glycerol: a review. Int. J. Hydrog. Energy. 37, 6473–6490. doi: 10.1016/j.ijhydene.2012.01.050
Taconi, K. A., Venkataramanan, K. P., and Johnson, D. T. (2009). Growth and solvent production by Clostridium pasteurianum TM ATCC 6013 utilizing biodiesel-derived crude glycerol as the sole carbon source. Environ. Prog. Sustain. Energy 28, 110. doi: 10.1002/ep.10350
Tong, I. T., and Cameron, D. C. (1992). Enhancement of 1,3-Propanediol production by cofermentation in Escherichia coli expressing Klebsiella pneumoniae dha regulon genes. Appl. Biochem. Biotechnol. 34, 149–159. doi: 10.1007/BF02920542
Uprety, B. K., Venkatesagowda, B., and Rakshit, S. K. (2017). Current prospects on production of microbial lipid and other value-added products using crude glycerol obtained from biodiesel industries. Bioenerg. Res. 10, 1117–1137. doi: 10.1007/s12155-017-9857-0
Wischral, C. (2015). 1,3-Propanediol: Statistical optimization of medium to improve production by Clostridium beijerinckii DSM 791. J. Adv. Biotechnol. 5, 614–623. doi: 10.24297/jbt.v5i2.1560
Wischral, D., Zhang, J., Cheng, C., Lin, M., Souza, L. M. G., Pessoa, F. L. P., et al. (2016). Production of 1,3-propanediol by Clostridium beijerinckii DSM 791 from crude glycerol and corn steep liquor: process optimization and metabolic engineering. Bioresour. Technol. 212, 100–110. doi: 10.1016/j.biortech.2016.04.020
Zabed, H. M., Zhang, Y., Guo, Q., Yun, J., Yang, M., Zhang, G., et al. (2019). Co-biosynthesis of 3-hydroxypropionic acid and 1,3-propanediol by a newly isolated Lactobacillus reuteri strain during whole cell biotransformation of glycerol. J. Clean. Prod. 226, 432–442. doi: 10.1016/j.jclepro.2019.04.071
Zeng, A.-P., and Biebl, H. (2002). Bulk chemicals from biotechnology: the case of 1,3-propanediol production and the new trends. Adv. Biochem. Eng. Biotechnol. 74, 239–259. doi: 10.1007/3-540-45736-4_11
Zhang, C., Sharma, S., Wang, W., and Zeng, A. P. (2021). A novel downstream process for highly pure 1,3-propanediol from an efficient fed-batch fermentation of raw glycerol by Clostridium pasteurianum. Eng. Life. Sci. 21:351–363. doi: 10.1002/elsc.202100012
Keywords: biorefinery, fermentation, glycerol, Clostridium beijerinckii, butyrate, propanediol
Citation: Mermejo BC, Bortolucci J, de Andrade AR and Reginatto V (2022) The Non-solventogenic Clostridium beijerinckii Br21 Produces 1,3-Propanediol From Glycerol With Butyrate as the Main By-Product. Front. Sustain. Food Syst. 6:848022. doi: 10.3389/fsufs.2022.848022
Received: 03 January 2022; Accepted: 03 June 2022;
Published: 07 July 2022.
Edited by:
Maria Pilar Bernal, Center for Edaphology and Applied Biology of Segura (CSIC), SpainReviewed by:
Jan Kolek, University of Chemistry and Technology Prague, CzechiaMatias B. Vanotti, Agricultural Research Service (USDA), United States
Copyright © 2022 Mermejo, Bortolucci, de Andrade and Reginatto. This is an open-access article distributed under the terms of the Creative Commons Attribution License (CC BY). The use, distribution or reproduction in other forums is permitted, provided the original author(s) and the copyright owner(s) are credited and that the original publication in this journal is cited, in accordance with accepted academic practice. No use, distribution or reproduction is permitted which does not comply with these terms.
*Correspondence: Valeria Reginatto, valeriars@ffclrp.usp.br