- Department of Food Engineering and Technology (DETA), Institute of Biosciences, Humanities and Exact Sciences (IBILCE), UNESP - São Paulo State University, São José do Rio Preto, Brazil
Microencapsulation can improve protection for compounds that degrade easily, such as β-carotene that is present in large amounts in buriti oil (Mauritia flexuosa). Encapsulating matrices are mainly composed of proteins and polysaccharides, which are often combined to improve their performance as a protective barrier. Beans, such as dark red kidney beans (Phaseolus vulgaris) and mung beans (Vigna radiata), are excellent protein sources that contain significant amounts of the essential amino acids. Bean flours are low in fat and naturally provide a blend of high-quality protein and carbohydrates that may stabilize lipophilic compounds for subsequent spray-drying. Whole bean flours, rather than refined individual biopolymers, may represent more sustainable alternative wall materials for microencapsulate bioactive compounds. This work aimed to evaluate the use of flours produced from red kidney beans and mung beans, which have been submitted to different physical pretreatments, as wall materials for microencapsulation of buriti oil by spray-drying. Different bean treatments were evaluated: untreated (control), soaked in water for 24 h, and soaked in water for 24 h followed by boiling for 30 min. The flours' proximate composition was not affected by the treatments (p < 0.05), showing similar values of carbohydrate (63.8–67.9%), protein (19.2–24.6%), and lipid (1.2–1.9%) contents. Both bean species had the water absorption capacity (WAC) increased by boiling, while the oil absorption capacity (OAC) was not altered by the treatments. Flours produced with raw or soaked beans showed emulsion activity (EA) and emulsion stability (ES) greater than 70%. Raw bean flours also showed better foaming properties, which may be indicative of higher levels of antinutritional factors. The soaked bean flours showed the best results for both type of beans, especially with regard to emulsifying properties, and were selected as wall materials for buriti oil microencapsulation. Different ratios of flour and maltodextrin were used to produce oil-in-water emulsions that were then spray-dried. Buriti oil microcapsules showed good physicochemical properties, with moisture around 3%, aw <0.3, and hygroscopicity around 5%. The carotenoid encapsulation efficiency ranged from 68.2 to 77.9%. Bean flours showed to function as a sustainable and nutrient-rich alternative wall material for microencapsulation.
Introduction
Microencapsulation is widely applied for protection of bioactive compounds through formation of microcapsules or microparticles, in which the value compounds are enclosed or immobilized by one or more encapsulating (wall) material, generally biopolymers. The encapsulation process provides physical protection against external conditions, such as moisture and oxygen, increasing the stability of the encapsulated (core) compounds. Spray-drying is one of the most used techniques for encapsulating food ingredients, being an economical method that allows continuous operation. It consists of atomizing a suspension or emulsion in a drying chamber with hot air circulation. The air temperature is high enough to allow the material to dry quickly. Drying time is generally very short, which limits thermal degradation of heat-sensitive compounds (Flores et al., 2014).
Encapsulating matrices are mainly composed of proteins and polysaccharides, which are often combined to improve their performance as a protective barrier. The use of different polysaccharides has been reported, such as maltodextrin, pectin, and gum Arabic (Rascón et al., 2011; Carneiro et al., 2013; Böger et al., 2018; Ribeiro et al., 2020). Among the different proteins, encapsulation using whey and soy proteins have been reported (Robert et al., 2010; Tang and Li, 2013; Flores et al., 2014; Nesterenko et al., 2014; Zhang et al., 2014; Pereira et al., 2019). Due to changes in eating habits and the increase in vegetarian/vegan diets, new sources of plant proteins have been investigated. Pulses are of particular interest because they contain high amounts of protein (18–32%), including essential amino acids and bioactive peptides (Boye et al., 2010). Pulse proteins combined with polysaccharides have shown promising results for encapsulation purposes (Costa et al., 2015; Tamm et al., 2016; Moser et al., 2020). For example, microcapsules of flaxseed oil, using chickpea and lentil protein isolates in conjunction with maltodextrin as wall material, exhibited an efficient protective effect against oxidation during storage (Karaca et al., 2013).
In general, the proteins used as wall material are in the form of isolates and concentrates. Beans, such as red kidney beans (Phaseolus vulgaris) and mung beans (Vigna radiata), are considered as excellent sources of plant proteins, with significant amounts of the essential amino acids. Bean flours provide a natural blend of high-quality protein and complex carbohydrates (e.g., starch, fibers) that can be used as wall materials for microencapsulation.
The effects of thermal treatments on the functional properties of flours from particular types of pulses have been investigated (Giami, 1993; Ma et al., 2011; Ferawati et al., 2019; Medhe et al., 2019; Lin and Fernández-Fraguas, 2020; Stone et al., 2021). Heat-treatment (autoclave) was reported to improve the water holding capacity of cowpea flour (Giami, 1993). Thermal treatment (boiling) of common bean flour increased its water holding capacity and emulsion stability (Lin and Fernández-Fraguas, 2020). Roasting and boiling resulted in better emulsifying activities of chickpea and yellow pea flours (Ma et al., 2011). Emulsifying properties are most relevant when it comes to microencapsulation of lipophilic compounds, which requires stabilization of the dispersed lipophilic compounds for subsequent spray drying.
Buriti (Mauritia flexuosa) is a palm tree native to Brazil. Its fruit has an oval shape, a scaly red pericarp and an oily pulp (Silva et al., 2009; Cruz et al., 2020). Buriti oil has a bright and reddish color and is rich in carotenoids, especially β-carotene, which represents ~90% of the total carotenes (Silva et al., 2009; Zanatta et al., 2010). Buriti oil contains a high content of unsaturated fatty acids, which makes it susceptible to oxidation. The encapsulation of buriti oil is an alternative for the prevention of oxidative processes that may occur (Freitas et al., 2017).
The objective of this work was to evaluate the functional properties of whole meal flours obtained from red kidney beans and mung beans, which were submitted to different physical treatments (soaking and boiling), and their use as wall materials for microencapsulation of buriti oil by spray drying.
Materials and Methods
Materials
Mung bean (Vigna radiata) and dark red kidney bean (Phaseolus vulgaris) seeds were purchased from a local market. Buriti oil (Mauritia flexuosa) was supplied by Amazon Oil™ (Ananindeua, Brazil) and maltodextrin DE 10 was supplied by Get do Brasil (São João da Boa Vista, Brazil).
Methods
Physical Pretreatments of Beans
Beans were previously selected for eliminating damaged seeds and underwent different pretreatments: soaking (S) and soaking + boiling (B). The control group (C) consisted of raw beans (untreated). Soaking (S) was carried out by immersing the seeds in distilled water at a ratio of 1:5 (seeds: water, w/v) for 24 h; the water was then discarded, the seeds were dried in an air-circulation oven (60°C/12 h), and ground in an impact mill to produce a flour that passed through a 400-μm sieve. The soaked + boiled seeds (B) were soaked as previously described, and then boiled in distilled water (1:3, w/v) for 30 min. After that, the seeds were dried and ground as in the S treatment. The control seeds (C) were only ground to produce the flour. The flours were stored in zip-lock bags and kept in a desiccator until use.
The flours produced from untreated, soaked, or soaked + boiled mung beans were repectively coded as MC, MS, and MB; the flours produced from untreated, soaked, or soaked + boiled red kidney beans were repectively coded as KC, KS, and KB.
Proximate Composition of Flours
The proximate composition of the bean flours was analyzed according to the Association of Official Analytical Chemists (AOAC, 1990). Proteins were determined by the Kjeldahl method, using 6.25 as the conversion factor. Lipids were determined in Soxhlet equipment with petroleum ether percolation. Moisture content was determined by gravimetry after drying in a vacuum oven at 70°C. Ash was determined by gravimetry after incinerating the samples at 550°C. Carbohydrate content was calculated as the residual weight after subtracting the amounts of protein, lipids, water, and ash found experimentally.
Scanning Electron Microscopy (SEM)
The microstructure of bean flours was analyzed by scanning electron microscopy (SEM). Samples were prepared by attaching small amounts of flour to SEM stubs and subsequent gold coating under vacuum. Images were captured using a JEOL JSM-7500F microscope (JEOL Ltd., Japan) at 400× magnification.
Functional Properties of Flours
Water Absorption Capacity and Oil Absorption Capacity
The water absorption capacity (WAC) and oil absorption capacity (OAC) of the flours were determined according to Argel et al. (2020), with modifications. About 1 gram of flour was added into falcon tubes and 10 mL of distilled water or soybean oil was added with subsequent vortexing of the tubes for 1 min. The tubes were centrifuged (3,000 × g, 20 min) and the supernatant was discarded. The tube contents were reweighed and the water or oil absorption capacity was calculated and expressed as g of water or oil g−1 of solids.
Color
The flour color was assessed in a Konica Minolta equipment (CR-S, Konica Minolta, Japan) by measuring the CIELab parameters: L (luminosity), a* (+a* = red, -a* = green) and b* (+b * = yellow, –b* = blue). Chroma (C) and Hue angle (h) were calculated from Equations (1) and (2):
Emulsifying Activity and Emulsion Stability
The emulsifying properties of the flours were analyzed according to Argel et al. (2020). To determine the emulsifying activity (EA), 1.5 g of flour was dispersed in 30 mL of distilled water and homogenized at 20,000 rpm (T-25 Ultra-Turrax®, IKA, Germany) for 2 min. Then, 30 mL of soybean oil was added with further homogenization at 20,000 rpm for 2 min. The produced emulsion was centrifuged at 750 × g for 5 min, the height of the upper phase was measured in the tube and expressed as a percentage in relation to the total height. The emulsion stability (ES) was evaluated by immersing the emulsion in a water bath at 80°C for 30 min, with subsequent centrifugation and measurement of the upper phase height, following the same procedure described for EA.
Foaming Capacity and Foam Stability
The foaming properties of the flours were determined according to Vinayashree and Vasu (2021), with modifications. The foaming capacity (FC) was evaluated by adding 1.5 g of flour in 50 mL of water, followed by stirring at 20,000 rpm (T-25 Ultra-Turrax®, IKA, Germany) for 2 min. The obtained foam was added to a graduated cylinder and the volume of the formed foam was measured. The foaming capacity was calculated by Equation (3), whereas the foam stability (FS) was evaluated by measuring the foam volume reduction after 120 min of its preparation (Equation 4).
Least Gelation Concentration
The least gelation concentration (LGC) was evaluated for flour suspensions at different concentrations (2–20%, w/v). The suspensions were prepared in distilled water and kept in a water bath at boiling temperature for 30 min. Then, the suspensions were refrigerated for 24 h and the tubes were inverted. LGC was determined as the lowest concentration necessary for hindering flow after the tube inversion (Sahni et al., 2020).
Microencapsulation by Spray Drying of Buriti Oil
Based on the results obtained for the functional properties, the flours treated by soaking (MS and KS treatments) were selected for microencapsulation of buriti oil. Four emulsion formulations were defined to evaluate two protein concentrations (1 or 2 g of protein in the flour/100 g emulsion), based on the proximate composition of MS and KS flours. The emulsion formulations are shown in Table 1.
The flour was dispersed in water and the pH was adjusted to 10 (using NaOH 0.1 M) to increase protein solubility. The buriti oil was added and the emulsion was homogenized (T-25 Ultra-Turrax®, IKA, Germany) at 20,000 rpm for 10 min, followed by addition of maltodextrin DE 10 and further homogenization at 20,000 rpm for 10 min. Maltodextrin DE 10 was added as a carrier agent, since spray drying was not feasible in the absence of this adjuvant. After homogenization, the emulsions were sonicated (Sonic Ruptor 4000, Omni International, USA) for 3 min (240 W/20 kHz) to help reduce droplet size.
The emulsions were fed into the spray-dryer (B-290, Buchi, Switzerland) equipped with an atomizing nozzle of 0.7 mm diameter. The operating conditions were based on previous work (Pereira et al., 2019), with inlet air temperature of 140°C, emulsion feed rate of 2 mL/min, drying air suction rate of 35 m3/h and atomization airflow rate of 819 L/h. After drying, the microcapsules were stored in polyethylene packages coated with aluminum foil and kept in a desiccator until analysis.
Characterization of Buriti Oil Microcapsules
Drying Yield
The drying yield was expressed as a percentage from the ratio between the mass of microcapsules obtained after drying and the solids present in the emulsion fed into the spray-dryer (including core and wall materials) (Equation 5).
Moisture, Water Activity and Hygroscopicity
Moisture of the microcapsules was determined by gravimetry, after drying in a vacuum oven at 70°C for 48 h (AOAC, 1990). Water activity (aw) was determined at room temperature using an electronic hygrometer (AW Sprint, Novasina, Axair Ltd., Switzerland). The hygroscopicity of the microcapsules was determined according to Cai and Corke (2000). Approximately 1 g of microcapsules were stored in a desiccator containing saturated NaCl solution (relative humidity = 75.3%) for 7 days. After storage, the samples were weighed to determine the mass of water absorbed by mass of dry material. All measurements were conducted in triplicate.
Morphology and Color Parameters
The morphology of the microcapsules was evaluated by SEM analysis [as previously described in section Scanning Electron Microscopy (SEM)] at 2000× magnification. The CIELAB color parameters were determined using the Konica Minolta equipment (CR-S, Konica Minolta, Japan), as presented in section Color.
Oil Retention and Encapsulation Efficiency
The buriti oil retention (RTO) and encapsulation efficiency (EEO) were calculated from the initial oil content present in the emulsions (IO), the oil present on the microcapsule surface (SO) and the total oil present in the microcapsules (TO), based on the methodology described by Ribeiro et al. (2020). The surface oil (SO) was quantified by adding 50 mg of microcapsules to 5 mL of chloroform in a tube and manually stirring for 1 min. The organic phase was filtered on filter paper, transferred to a Petri dish and oven-dried at 60°C until constant weight. The total oil (TO) was determined by weighing 100 mg of microcapsules and mixing 20 mL of buffer solution (pH 7.4), stirring in a vortex for 30 s and keeping the mixture to rest overnight. Then, 25 mL of chloroform was added and vortexed again for 30 s and sonicated for 20 min. The aqueous and organic phases were separated by centrifugation at 1,800 rpm for 10 min and the organic phase was transferred to a Petri dish and oven dried at 60°C until constant weight. The encapsulation efficiency (EEO) and retention (RTO) of buriti oil were calculated, respectively, by Equations (6) and (7):
Encapsulation Efficiency of Carotenoids
The encapsulation efficiency of carotenoids (EEC) was calculated by quantifying the carotenoids present on the microcapsule surface (SC), and the total carotenoids present in the microcapsules (TC), as described by Ribeiro et al. (2020). The SC and TC were determined starting by the same procedure described in section Oil Retention and Encapsulation Efficiency for extracting the oil from the microcapsules; after that the mixtures of oil and solvent were added to 5- and 25-mL volumetric flasks, respectively, having their contents completed with chloroform. The absorbance was measured at 465 nm and the carotenoid contents, expressed as β-carotene, were determined by Equation (8):
In which C is the carotenoid concentration (μg β-carotene/g sample), Abs is the absorbance reading, V is the dilution volume (mL) and m is the sample mass (g). The carotenoid encapsulation efficiency (EEC) was calculated by Equation (9):
Statistical Analysis
The analyzes were carried out in triplicate. The data were submitted to Analysis of Variance (ANOVA) and the Tukey Test in order to detect significant differences in the results. All tests were applied at a significance level of 5% using OriginPro 2016 software.
Results and Discussion
Proximate Composition, Microstructure and Color of Flours
The composition of mung and kidney bean flours are shown in Table 2. The proximate composition of the two species was quite similar in terms of proteins, carbohydrates and lipids. The physical pre-treatments (soaking and boiling) had no effect on the composition of macronutrients present in the flours (p < 0.05).
The lowest moisture content observed for soaked and soaked + boiled flours (5.9–8.0%) is due to the drying of the seeds after the pre-treatment, which reduced the moisture levels to values lower than those of untreated seeds (~13%). The high levels of carbohydrates (63.8–67.9%) and proteins (19.2–24.6%), and the low levels of lipids (1.2–1.9%) observed for beans are similar to other pulses such as green pea, yellow pea, and navy bean (Millar et al., 2019; Kenar et al., 2020). Ash content ranged from 2.8 to 3.7% for bean flours, and these high ash contents (>1%) are also characteristic of pulse flours. Although a greater amount of ash brings nutritional benefits, it can also lead to lower quality flours for the production of bakery products, due to the dilution effect of functional proteins (Millar et al., 2019).
Soaking and boiling pretreatments had an impact on the flour microstructure of the two bean species (Figure 1). The SEM images show that the flours from untreated seeds presented the starch granules with an oval shape and smooth surfaces (Figures 1A,B), which appear to be larger in the red kidney bean flour. The soaked bean flours also showed whole starch granules that maintained their oval shape, but appeared to be in smaller numbers and with a more rough surface, which may have resulted from the partial hydration of the granules (Figures 1C,D). After the beans were boiled, the flours presented larger and lumpy structures, in which most of the starch granules that still remained intact appear wrapped in the protein matrix, possibly combined with leached starch fractions (Figures 1E,F), mainly in the mung bean flour. The heat treatment contributed to reduce the compartmentalization between the starch granules and the protein matrix. Acevedo et al. (2017) observed the same aspect for the microstructure of pigeon pea flour subjected to soaking and boiling pretreatments.
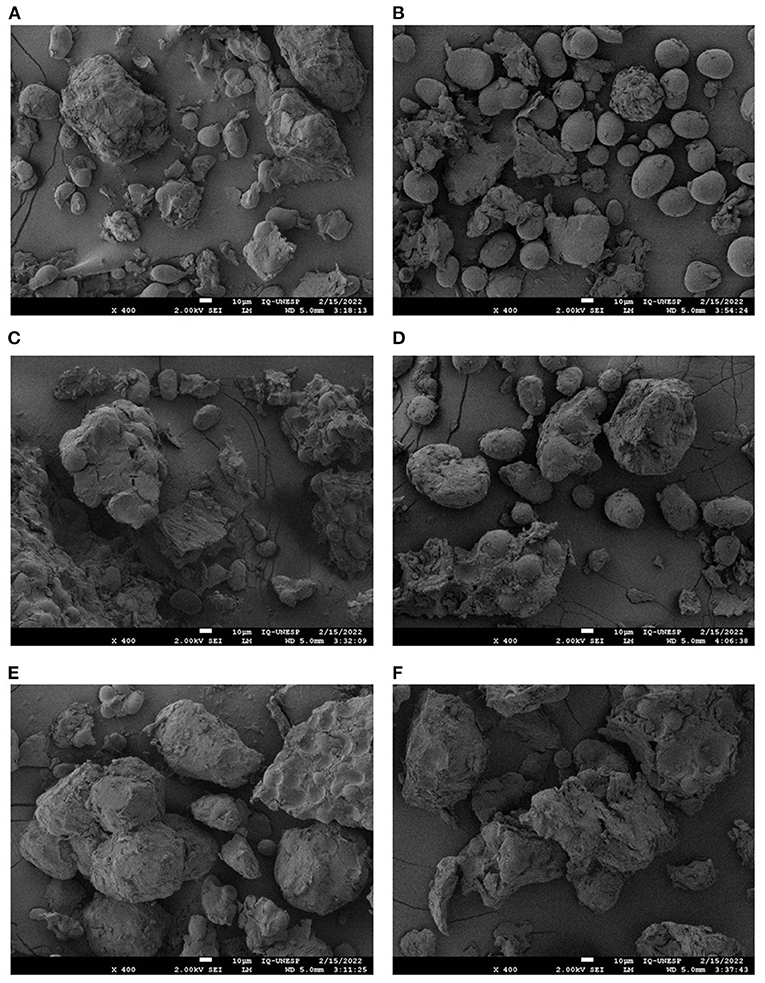
Figure 1. SEM micrographs (400× -magnification) of bean flours produced from mung bean (A,C,E) and red kidney bean (B,D,F) untreated (control) (A,B); soaked (C,D); soaked + boiled (E,F).
Regarding the color parameters, the values for L* decreased when the flours were boiled (p < 0.05) (Table 3). The a* and b* parameters varied in a similar way between the flours, with a predominance of the positive b* coordinate with a tendency to yellow, as evidenced by the hue angle. Kenar et al. (2020) observed similar results, with the boiling pretreatment affecting the color of navy bean flours, which was attributed to the loss of phenolic compounds that give color to the seeds, as well as to the redistribution of components in the seed matrix.
Functional Properties of Flours
The functional properties of the flours are related to the interaction of their constituents with other food components, mainly water and lipids. In the case of bean flours, the protein composition and structure (amino acid sequence, arrangements, distribution of charges, etc.), as well as the carbohydrate fraction, play an important tole. Structural changes that proteins may undergo during processing affect their functional properties (Bessada et al., 2019). In this section, the functional properties of mung and kidney bean flours produced from untreated seeds (MC and KC), after soaking (MS and KS), or after soaking and boiling (MB and KB) are discussed.
Water and Oil Absorption Capacity
The water absorption capacity (WAC) is defined by the maximum amount of water that can be absorbed by 1 g of protein or flour, whereas the oil absorption capacity (OAC) corresponds to the amount of oil that can be absorbed by 1 g of protein or flour (Bessada et al., 2019). Both bean species had the WAC increased by boiling (p < 0.05). The MC and MS flours had values of 2.3 and 2.4 g g−1, respectively, while the MB flour had the highest value (3 g g−1). The KC and KS flours had values of 2.1 and 2.0, respectively, while the KB flour had a higher value (2.6 g g−1). Ferawati et al. (2019) also observed an increase in WAC caused by boiling in yellow pea, gray pea, faba bean and white bean flours. The flour WAC is probably due to the presence of hydrophilic compounds, such as polysaccharides. Bean flours are rich in starch and fibers, which easily interact with water, contributing to WAC value (Kaur et al., 2007; Acevedo et al., 2017). Pretreatments involving heat application contribute to starch gelatinization, thus increasing water retention. In addition, heat treatment may unfold the proteins and expose polar amino acids, which have a greater affinity for water (Bhinder et al., 2020).
Regarding OAC, no difference was observed between treatments for both beans (p > 0.05). The MC, MS, and MB flours presented OAC values of 0.8 g g−1, while the KC, KS and KB flours presented values of 1.0, 0.9 and 0.8 g g−1, respectively. These values are lower than those found by Wani et al. (2013) and Gupta et al. (2018) for the same bean species. These differences may be related to seed maturation, cultivation conditions, storage and transport. OAC of pulse flour is related to the starch and protein contents of seeds and the presence and availability of non-polar side chain amino acids (Bhinder et al., 2020).
Emulsifying Properties
The emulsifying properties of flours can be mainly attributed to their protein components, which act as emulsifiers in the formation of a film around the oil particles dispersed in an aqueous medium, thus avoiding coalescence (Argel et al., 2020). Emulsifying activity (EA) indicates the ability of the protein to allow emulsion formation, while emulsion stability (ES) is a measure of the protein film strength at the oil/water interface over a certain period of time (Bhinder et al., 2020). The EA and ES results for mung and kidney bean flours are shown in Figures 2A,B. Boiling treatment (MB and KB) reduced EA and ES of pulse flours, while the other flours (MC, MS, KC and KS) presented values >70% for both parameters (p > 0.05). These values are higher than those found by Argel et al. (2020) for peas, chickpeas and common beans.
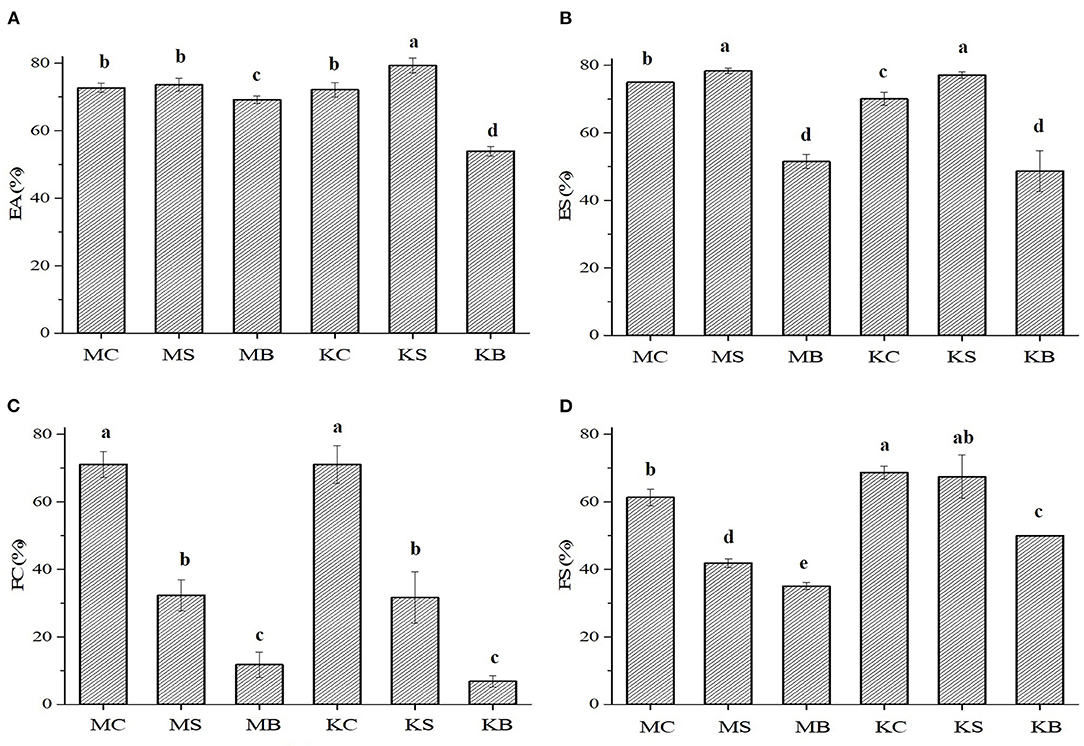
Figure 2. Emulsifying capacity (EA) (A), emulsion stability (ES) (B), foaming capacity (FC) (C) and foam stability (FS) (D) of flours produced from untreated mung bean (control) (MC); soaked mung bean (MS); soaked + boiled mung bean (MB); untreated red kidney bean (control) (KC); soaked red kidney bean (KS); soaked + boiled red kidney bean (KB). Significant differences among samples are indicated by different letters (p < 0.05).
Ferawati et al. (2019) also observed a reduction in EA and ES caused by the boiling treatment in white bean flour. According to Pallares et al. (2021), this reduction may occur due to the thermal denaturation of bean proteins during boiling, leading to the occurrence of intra- and intermolecular interactions that cause protein aggregation. This process can result in loss of protein solubility, making them unable to adsorb at the oil/water interface.
Foaming Properties
Foaming properties, such as foaming capacity (FC) and foam stability (FS), are important parameters to evaluate the use of pulse flours in aerated food systems such as cakes, ice cream and whipped toppings. FC indicates the ability of proteins to create a film around air bubbles during whipping, while FS assesses the ability of this film to maintain air bubbles over time, resisting coalescence of air bubbles and drainage of liquid (Bhinder et al., 2020).
FC was higher for the control flours (71.1% for MC and KC), significantly decreasing for the flours that underwent soaking (32.4 and 31.7% for MS and KS, respectively) and boiling (11.7 and 6.8% for MB and KB respectively) (Figure 2C) (p < 0.05). In addition to protein denaturation and loss of functionality caused by boiling, contact with water during soaking can result in the leaching of components that are also important for foaming. Saponins, for example, are compounds that can contribute to foam formation due to their amphiphilic nature (Kenar et al., 2020).
Mung bean flour showed a similar result for FS, with MC (61.3%) having higher values compared to MS flour (41.9%) and MB (35.0%) (p < 0.05). For kidney bean flours, there was no difference between KC and KS (68.7 and 67.5%, respectively), but both had FS values higher than KB (50.0%) (Figure 2D) (p < 0.05). Ferawati et al. (2019) also observed lower FS values for yellow pea, gray pea, faba bean, and white bean flours that underwent heat treatment (boiling and roasting).
Gel Formation
Gelation of proteins and starch gelatinization are important properties for application in various food products such as frozen desserts (mousses, puddings, flans, jellies, among others). After denaturation, globular proteins (found in vegetables) are able to form gels by aggregation and interaction of proteins with other components found in food systems (Bessada et al., 2019). Starch granules can absorb water and increase in size, which also contributes to gel formation (Pallares et al., 2021).
Least gelation concentration (LGC) is a functional property that determines the least concentration of a protein extract or flour required to produce stable gels. This property depends on factors such as: growing conditions, seed composition, presence/absence of lipids, competition for water between protein and starch, ionic strength, pH and solvent temperature (Gupta et al., 2018). LGC was 10% for MC and MS samples, 12% for MB, KC and KS samples and 14% for KB sample. Gupta et al. (2018) determined higher values for gelatinization of mung bean and kidney bean flours (16 and 14%, respectively). In both cases, a slightly higher concentration of flour was necessary for the formation of gels from flours produced from seeds that underwent boiling pretreatment. Prinyawiwatkul et al. (1997) reported similar results for cowpea flour and suggested that the requirement of greater amounts of flour from boiled cowpeas to achieve thermal gel formation is linked to protein denaturation and starch gelatinization.
Microencapsulation of Buriti Oil Using Whole Bean Flours as Wall Material
Considering the results obtained for the functional properties (mainly for emulsifying properties), the flours produced after soaking pretreatment (MS and KS flours) were selected to be applied as wall materials on the microencapsulation by spray drying of buriti oil. In general, both flours allowed the production of carotenoid-rich microcapsules with suitable physicochemical properties (Table 4).
Figure 3 shows SEM images of buriti oil microcapsules produced with bean flours. Both flours resulted in microcapsules with a slightly flattened rounded shape characteristic of the spray-drying process. The surfaces of the microcapsules were smooth and without porosities or holes, which is positive for the stability of the encapsulated oil. However, the mung bean flour (Figures 3A,B) resulted in larger microcapsules when compared to those produced by red kidney bean flour (Figures 3B,C), especially for formulations with a higher amount of flour. Moser et al. (2019) observed greater agglomeration in buriti oil microcapsules produced with chickpea protein concentrate, pectin and maltodextrin. This suggests that the polysaccharides present in whole flour (e.g., starch and fibers) may have a positive effect in reducing the agglomeration of spray-dried microcapsules.
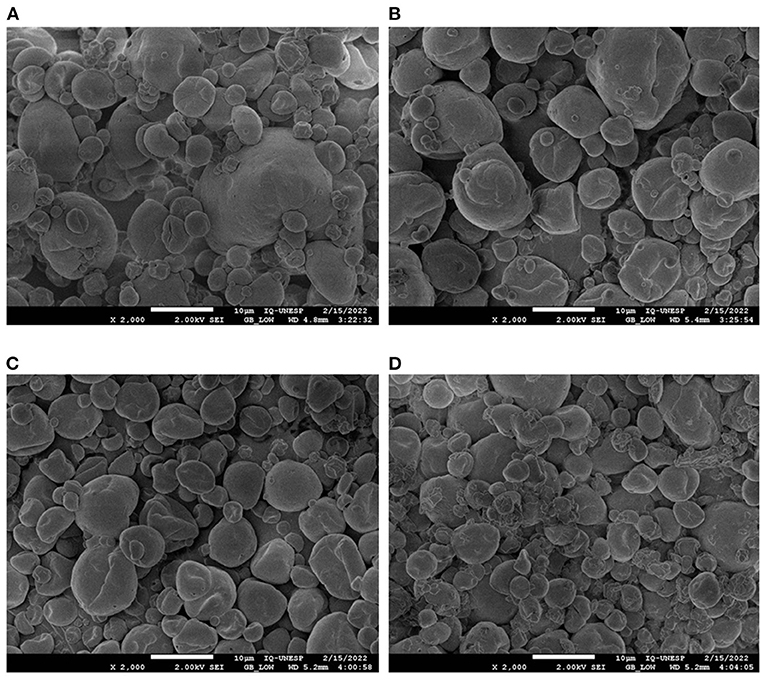
Figure 3. SEM micrographs (2000× -magnification) of buriti oil microcapsules stabilized with mung bean (A,B) and red kidney bean (C,D) flours at a concentration equivalent to 1% (w w−1) (A,C) or 2% (w w−1) (B,D) of protein in the formulation.
The drying yield of microcapsules produced with mung bean flour was higher than with red kidney bean flour (p < 0.05). The proportion of flour in the formulation also had an impact on the drying yield, with less flour proportion (flour mass:total mass of emulsion) showing higher yield (p < 0.05). It is important to highlight that the low yield obtained was due to losses during the process on a laboratory scale equipment, since the small diameter of the drying chamber leads to high losses of product adhered on the dryer walls. These losses are easily avoided in the industry, with large-scale equipment.
Physical properties such as moisture, aw and hygroscopicity of powdered products are related to stability and their functional properties during desired applications. The moisture content of the powders was in the range of 3%, which is below the limit of 5% proposed for the water content contained in the powders dried by spray drying (Ramakrishnan et al., 2018). All formulations presented minimum values for aw, <0.3. There was no significant difference between the values obtained for the hygroscopicity of the microcapsules (p <0.05), which can be considered very low, demonstrating that the particles are little hygroscopic.
Table 4 shows that the color parameters of the microcapsules were influenced by the color of buriti oil. The capsules showed a yellow color (Figure 4), as observed by the h angle, which was located in the yellow region (h ~ 80) and the predominance of the positive b* coordinate for yellow. Chroma values around 50 indicated that the samples had high color saturation. Regarding the L* parameter, the formulations M1 and K1 with a smaller amount of flour were lighter than the microcapsules formulated with the higher flour content, i.e., the M2 and K2 samples (p < 0.05). The values obtained for the color parameters are in agreement with those found by other authors who produced buriti oil microcapsules using different wall materials, such as chickpea protein concentrate and pectin (Moser et al., 2019), soy protein isolate and pectin (Ribeiro et al., 2020), or gelatin and alginate (Lemos et al., 2017).
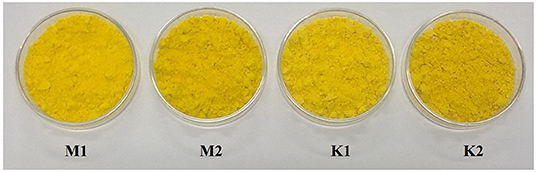
Figure 4. Physical aspect of buriti oil microcapsules stabilized with: mung bean flour equivalent to 1% (w w−1) of protein (M1); mung bean flour equivalent to 2% (w w−1) of protein (M2); red kidney bean flour equivalent to 1% (w w−1) of protein (K1); red kidney bean flour equivalent to 2% (w w−1) of protein (K2) in the formulation.
High encapsulation efficiency and oil retention is desirable for the stability of microcapsules during storage. While encapsulation efficiency indicates the amount of oil or carotenoids that are truly encapsulated, i.e., entrapped inside the matrix and covered by the wall material, oil retention is a measure of the total oil contained in the microcapsules, including the oil that may be only adsorbed at the particle surface, and thus exposed to oxygen and light (non-encapsulated oil).
The encapsulation efficiency of buriti oil (EEO) in microcapsules ranged from 65.2 to 72.6%, while the retention of buriti oil (RTO) ranged from 76.9 to 85.9%. Regarding the encapsulation efficiency of carotenoids (EEC), the results ranged from 68.2 to 77.9%. No difference was observed between the EEO, RTO, and EEC values for the different formulations evaluated (p > 0.05) (Table 4). Small losses of carotenoids may have occurred during spray drying due to the exposition to drying air, in spite of the low exposure times typical of this process.
Ribeiro et al. (2020) observed oil encapsulation efficiency ranging from 52.4 to 65.7% for buriti oil microencapsulated using soybean isolate, high methoxy pectin and maltodextrin as wall materials. The authors also determined a total oil retention in the microcapsules, obtaining values that ranged from 51.5 to 56.4%. Moser et al. (2019) reported carotenoid encapsulation efficiency ranging from 81.9 to 91.6% for buriti oil microcapsules stabilized by chickpea protein, high methoxy pectin and maltodextrin. The authors highlighted that an interaction of pectin with the protein allowed greater encapsulation efficiency, compared to formulations stabilized only by proteins. In addition to the effect of emulsion stability on the retention and encapsulation efficiency values, these parameters may also be affected by the wall material composition. The fast formation of a glassy crust during drying of droplets that occurs in formulations containing higher maltodextrin content, as well as in formulations with higher wall material concentrations, favors higher encapsulation efficiency (Adhikari et al., 2003; Tonon et al., 2011).
In the present work, it was possible to obtain buriti oil microcapsules stabilized with whole bean flours with EEO, RTO and EEC values similar or even higher than those found by other authors using protein isolates or a combination of proteins and carbohydrates. Both bean species showed similar results in the microencapsulation of buriti oil. However, SEM images suggest a different microstructure for both types of microcapsules, mainly in relation to their size. This aspect can cause differences during their long-term storage, as well as the release of encapsulated compounds from the matrices. In this pilot study we were able to produce microcapsules stabilized with whole bean flour that showed efficient encapsulation of buriti oil and its carotenoid content.
Conclusions
Bean flours submitted to soaking and boiling pretreatments showed no changes in the composition of their macronutrients when compared to untreated beans. Flours produced with soaked seeds showed good emulsifying properties, whereas the boiled seeds resulted in flours with greater water absorption capacity, but a decrease in emulsifying, foaming and gel-forming properties. Whole mung and red kidney bean flours showed potential for application in buriti oil microencapsulation processes, demonstrated by the encapsulation efficiency and total oil retention values. The use of bean flours, which are rich in proteins, fibers, and starch is a promising alternative for more sustainable microencapsulation processes, without the need to previously fractionate or purify the biopolymers used as wall materials. Future work is needed to clarify other important aspects of using whole bean flours for microencapsulation, such as: (i) impact of antinutritional factors on microcapsules; (ii) in-depth understanding of the interpolymeric interactions within the whole flour matrix; (iii) changes in carbohydrates/starch present in the flours and their impact on the stabilization of microcapsules.
Data Availability Statement
The raw data supporting the conclusions of this article will be made available by the authors, without undue reservation.
Author Contributions
AL-P, MK, CF, and VN contributed to conception and design of the study. AL-P, MK, and CF carried out the experimental work, organized the database, and performed the statistical analysis. AP and CF wrote the first draft of the manuscript. MK and VN wrote sections of the manuscript. All authors contributed to manuscript revision, read, and approved the submitted version.
Conflict of Interest
The authors declare that the research was conducted in the absence of any commercial or financial relationships that could be construed as a potential conflict of interest.
Publisher's Note
All claims expressed in this article are solely those of the authors and do not necessarily represent those of their affiliated organizations, or those of the publisher, the editors and the reviewers. Any product that may be evaluated in this article, or claim that may be made by its manufacturer, is not guaranteed or endorsed by the publisher.
Acknowledgments
This work was possible thanks to the scholarship granted from the Brazilian Federal Agency for Support and Evaluation of Graduate Education (CAPES), in the scope of the Program CAPES-PrInt, process number 88887.310463/2018-00, International Cooperation Project number 88887.310309/2018-00, and Mobility number 88887.572473/2020-00. This study was financed in part by the Coordenação de Aperfeiçoamento de Pessoal de Nível Superior – Brasil (Capes) – Finance Code 001.
References
Acevedo, B. A., Thompson, C. M. B., González Foutel, N. S., Chaves, M. G., and Avanza, M. V. (2017). Effect of different treatments on the microstructure and functional and pasting properties of pigeon pea (Cajanus cajan L.), dolichos bean (Dolichos lablab L.) and jack bean (Canavalia ensiformis) flours from the north-east Argentina. Int. J. Food Sci. Technol. 52, 222–230. doi: 10.1111/ijfs.13271
Adhikari, B., Howes, T., Bhandari, B. R., and Troung, V. (2003). Surface stickiness of drops of carbohydrate and organic acid solutions during convective drying: experiments and modeling. Drying Technol. 21, 839–873. doi: 10.1081/DRT-120021689
AOAC (1990). Official Methods of Analysis of the Association of Official Analytical Chemists, 15th Edn. Arlington, VA: Association of Official Analytical Chemists.
Argel, N. S., Ranalli, N., Califano, A. N., and Andrés, S. C. (2020). Influence of partial pork meat replacement by pulse flour on physicochemical and sensory characteristics of low-fat burgers. J. Sci. Food Agric. 100, 3932–3941. doi: 10.1002/jsfa.10436
Bessada, S. M. F., Barreira, J. C. M., and Oliveira, M. B. P. P. (2019). Pulses and food security: dietary protein, digestibility, bioactive and functional properties. Trends Food Sci. Technol. 93, 53–68. doi: 10.1016/j.tifs.2019.08.022
Bhinder, S., Kaur, A., Singh, B., Yadav, M. P., and Singh, N. (2020). Proximate composition, amino acid profile, pasting and process characteristics of flour from different Tartary buckwheat varieties. Food Res. Int. 130, 108946. doi: 10.1016/j.foodres.2019.108946
Böger, B. R., Georgetti, S. R., and Kurozawa, L. E. (2018). Microencapsulation of grape seed oil by spray drying. Food Sci. Technol. 38, 263–270. doi: 10.1590/fst.04417
Boye, J., Zare, F., and Pletch, A. (2010). Pulse proteins: Processing, characterization, functional properties and applications in food and feed. Food Res. Int. 43, 414–431. doi: 10.1016/j.foodres.2009.09.003
Cai, Y. Z., and Corke, H. (2000). Production and properties of spray-dried Amaranthus betacyanin pigments. J. Food Sci. 65, 1248–1252. doi: 10.1111/j.1365-2621.2000.tb10273.x
Carneiro, H. C. F., Tonon, R. V., Grosso, C. R. F., and Hubinger, M. D. (2013). Encapsulation efficiency and oxidative stability of flaxseed oil microencapsulated by spray drying using different combinations of wall materials. J. Food Eng. 115, 443–451. doi: 10.1016/j.jfoodeng.2012.03.033
Costa, A. M. M., Nunes, J. C., Lima, B. N. B., Pedrosa, C., Calado, V., Torres, A. G., et al. (2015). Effective stabilization of CLA by microencapsulation in pea protein. Food Chem. 168, 157–166. doi: 10.1016/j.foodchem.2014.07.016
Cruz, M. B., Oliveira, W., da, S., Araújo, R. L., Honório França, A. C., and Pertuzatti, P. B. (2020). Buriti (Mauritia flexuosa L.) pulp oil as an immunomodulator against enteropathogenic Escherichia coli. Ind. Crops Prod. 149, 112330. doi: 10.1016/j.indcrop.2020.112330
Ferawati, F., Hefni, M., and Witthöft, C. (2019). Flours from Swedish pulses: Effects of treatment on functional properties and nutrient content. Food Sci. Nutr. 7, 4116–4126. doi: 10.1002/fsn3.1280
Flores, F. P., Singh, R. K., Kerr, W. L., Pegg, R. B., and Kong, F. (2014). Total phenolics content and antioxidant capacities of microencapsulated blueberry anthocyanins during in vitro digestion. Food Chem. 153, 272–278. doi: 10.1016/j.foodchem.2013.12.063
Freitas, M. L. F., Chist,é, R. C., Polachini, T. G., Sardella, L. A. C. Z., Aranha, C. P., Ribeiro, A. P. B., et al. (2017). Quality characteristics and thermal behavior of buriti (Mauritia flexuosa L.) oil. Grasas y Aceites 68, e220. doi: 10.3989/gya.0557171
Giami, S. Y.. (1993). Effect of processing on the proximate composition and functional properties of cowpea (Vigna unguiculata) flour. Food Chem. 47, 153–158. doi: 10.1016/0308-8146(93)90237-A
Gupta, S., Chhabra, G. S., Liu, C., Bakshi, J. S., and Sathe, S. K. (2018). Functional properties of select dry bean seeds and flours. J. Food Sci. 83, 2052–2061. doi: 10.1111/1750-3841.14213
Karaca, A. C., Nickerson, M., and Low, N. H. (2013). Microcapsule production employing chickpea or lentil protein isolates and maltodextrin: physicochemical properties and oxidative protection of encapsulated flaxseed oil. Food Chem. 139, 448–457. doi: 10.1016/j.foodchem.2013.01.040
Kaur, M., Sandhu, K. S., and Singh, N. (2007). Comparative study of the functional, thermal and pasting properties of flours from different field pea (Pisum sativum L.) and pigeon pea (Cajanus cajan L.) cultivars. Food Chem. 104, 259–267. doi: 10.1016/j.foodchem.2006.11.037
Kenar, J. A., Felker, F. C., Singh, M., Byars, J. A., Berhow, M. A., Bowman, M. J., et al. (2020). Comparison of composition and physical properties of soluble and insoluble navy bean flour components after jet-cooking, soaking, and cooking. LWT 130, 109765. doi: 10.1016/j.lwt.2020.109765
Lemos, Y. P., Mariano Marfil, P. H., and Nicoletti, V. R. (2017). Particle size characteristics of buriti oil microcapsules produced by gelatin-sodium alginate complex coacervation: effect of stirring speed. Int. J. Food Properties 20, 1438–1447. doi: 10.1080/10942912.2017.1349139
Lin, T., and Fernández-Fraguas, C. (2020). Effect of thermal and high-pressure processing on the thermo-rheological and functional properties of common bean (Phaseolus vulgaris L.) flours. LWT 127, 109325. doi: 10.1016/j.lwt.2020.109325
Ma, Z., Boye, J. I., Simpson, B. K., Prasher, S. O., Monpetit, D., and Malcolmson, L. (2011). Thermal processing effects on the functional properties and microstructure of lentil, chickpea, and pea flours. Food Res. Int. 44, 2534–2544. doi: 10.1016/j.foodres.2010.12.017
Medhe, S., Jain, S., and Anal, A. K. (2019). Effects of sprouting and cooking processes on physicochemical and functional properties of moth bean (Vigna aconitifolia) seed and flour. J. Food Sci. Technol. 56, 2115–2125. doi: 10.1007/s13197-019-03692-y
Millar, K. A., Gallagher, E., Burke, R., McCarthy, S., and Barry-Ryan, C. (2019). Proximate composition and anti-nutritional factors of fava-bean (Vicia faba), green-pea and yellow-pea (Pisum sativum) flour. J. Food Composition Anal. 82, 103233. doi: 10.1016/j.jfca.2019.103233
Moser, P., Ferreira, S., and Nicoletti, V. R. (2019). Buriti oil microencapsulation in chickpea protein-pectin matrix as affected by spray drying parameters. Food Bioproducts Process. 117, 183–193. doi: 10.1016/j.fbp.2019.07.009
Moser, P., Nicoletti, V. R., Drusch, S., and Brückner-Gühmann, M. (2020). Functional properties of chickpea protein-pectin interfacial complex in buriti oil emulsions and spray dried microcapsules. Food Hydrocoll. 107, 105929. doi: 10.1016/j.foodhyd.2020.105929
Nesterenko, A., Alric, I., Violleau, F., Silvestre, F., and Durrieu, V. (2014). The effect of vegetable protein modifications on the microencapsulation process. Food Hydrocoll. 41, 95–102. doi: 10.1016/j.foodhyd.2014.03.017
Pallares, A. P., Gwala, S., Pälchen, K., Duijsens, D., Hendrickx, M., and Grauwet, T. (2021). Pulse seeds as promising and sustainable source of ingredients with naturally bioencapsulated nutrients: literature review and outlook. Comprehens. Rev. Food Sci. Food Safety 20, 1524–1553. doi: 10.1111/1541-4337.12692
Pereira, A. R. L., Cattelan, M. G., and Nicoletti, V. R. (2019). Microencapsulation of pink pepper essential oil: Properties of spray-dried pectin/SPI double-layer versus SPI single-layer stabilized emulsions. Colloids Surfaces A 581, 123806. doi: 10.1016/j.colsurfa.2019.123806
Prinyawiwatkul, W., McWatters, K. H., Beuchat, L. R., and Phillips, R. D. (1997). Functional characteristics of cowpea (Vigna unguiculata) flour and starch as affected by soaking, boiling, and fungal fermentation before milling. Food Chem. 58, 361–372. doi: 10.1016/S0308-8146(96)00259-2
Ramakrishnan, Y., Adzahan, N. M., Yusof, Y. A., and Muhammad, K. (2018). Effect of wall materials on the spray drying efficiency, powder properties and stability of bioactive compounds in tamarillo juice microencapsulation. Powder Technol. 328, 406–414. doi: 10.1016/j.powtec.2017.12.018
Rascón, M. P., Beristain, C. I., García, H. S., and Salgado, M. A. (2011). Carotenoid retention and storage stability of spray-dried encapsulated paprika oleoresin using gum Arabic and Soy protein isolate as wall materials. LWT Food Sci. Technol. 44, 549–557. doi: 10.1016/j.lwt.2010.08.021
Ribeiro, M. L. F. F., Roos, Y. H., Ribeiro, A. P. B., and Nicoletti, V. R. (2020). Effects of maltodextrin content in double-layer emulsion for production and storage of spray-dried carotenoid-rich microcapsules. Food Bioproducts Process. 124, 208–221. doi: 10.1016/j.fbp.2020.09.004
Robert, P., Gorena, T., Romero, N., Sepulveda, E., Chavez, J., and Saenz, C. (2010). Encapsulation of polyphenols and anthocyanins from pomegranate (Punica granatum) by spray drying. Int. J. Food Sci. Technol. 45, 1386–1394. doi: 10.1111/j.1365-2621.2010.02270.x
Sahni, P., Sharma, S., and Surasani, V. K. R. (2020). Influence of processing and pH on amino acid profile, morphology, electrophoretic pattern, bioactive potential and functional characteristics of alfalfa protein isolates. Food Chem. 333, 127503. doi: 10.1016/j.foodchem.2020.127503
Silva, S. M., Sampaio, K. A., Taham, T., Rocco, S. A., Ceriani, R., and Meirelles, A. J. A. (2009). Characterization of oil extracted from buriti fruit (Mauritia flexuosa) grown in the Brazilian Amazon Region. J. Am. Oil Chemists' Soc. 86, 611–616. doi: 10.1007/s11746-009-1400-9
Stone, A. K., Parolia, S., House, J. D., Wang, N., and Nickerson, M. T. (2021). Effect of roasting pulse seeds at different tempering moisture on the flour functional properties and nutritional quality. Food Res. Int. 147, 110489. doi: 10.1016/j.foodres.2021.110489
Tamm, F., Herbst, S., Brodkorb, A., and Drusch, S. (2016). Functional properties of pea protein hydrolysates in emulsions and spray-dried microcapsules. Food Hydrocoll. 58, 204–214. doi: 10.1016/j.foodhyd.2016.02.032
Tang, C. H., and Li, X. R. (2013). Microencapsulation properties of soy protein isolate and storage stability of the correspondingly spray-dried emulsions. Food Res. Int. 52, 419–428. doi: 10.1016/j.foodres.2012.09.010
Tonon, R. V., Grosso, C. R. F., and Hubinger, M. D. (2011). Influence of emulsion composition and inlet air temperature on the microencapsulation of flaxseed oil by spray drying. Food Res. Int. 44, 282–289. doi: 10.1016/j.foodres.2010.10.018
Vinayashree, S., and Vasu, P. (2021). Biochemical, nutritional and functional properties of protein isolate and fractions from pumpkin (Cucurbita moschata var. Kashi Harit) seeds. Food Chem. 340, 128177. doi: 10.1016/j.foodchem.2020.128177
Wani, I. A., Sogi, D. S., Wani, A. A., and Gill, B. S. (2013). Physico-chemical and functional properties of flours from Indian kidney bean (Phaseolus vulgaris L.) cultivars. LWT Food Sci. Technol. 53, 278–284. doi: 10.1016/j.lwt.2013.02.006
Zanatta, C. F., Mitjans, M., Urgatondo, V., Rocha-Filho, P. A., and Vinardell, M. P. (2010). Photoprotective potential of emulsions formulated with Buriti oil (Mauritia flexuosa) against UV irradiation on keratinocytes and fibroblasts cell lines. Food Chem. Toxicol. 48, 70–75. doi: 10.1016/j.fct.2009.09.017
Keywords: pulses, soaking, cooking, phaseolus vulgaris, vigna radiata, mauritia flexuosa, emulsion, spray-drying
Citation: Locali-Pereira AR, Kubo MTK, Fuzetti CG and Nicoletti VR (2022) Functional Properties of Physically Pretreated Kidney Bean and Mung Bean Flours and Their Performance in Microencapsulation of a Carotenoid-Rich Oil. Front. Sustain. Food Syst. 6:845566. doi: 10.3389/fsufs.2022.845566
Received: 30 December 2021; Accepted: 01 March 2022;
Published: 25 March 2022.
Edited by:
Lingyan Kong, University of Alabama, United StatesReviewed by:
Khalid Muzaffar, Islamic University of Science and Technology, IndiaYanqi Zhang, University of Alabama, United States
Tiantian Lin, Cornell University, United States
Copyright © 2022 Locali-Pereira, Kubo, Fuzetti and Nicoletti. This is an open-access article distributed under the terms of the Creative Commons Attribution License (CC BY). The use, distribution or reproduction in other forums is permitted, provided the original author(s) and the copyright owner(s) are credited and that the original publication in this journal is cited, in accordance with accepted academic practice. No use, distribution or reproduction is permitted which does not comply with these terms.
*Correspondence: Vânia Regina Nicoletti, vania.nicoletti@unesp.br
†These authors have contributed equally to this work and share first authorship