- 1Unidad de Investigación en Nutrición, Salud, Alimentos Funcionales y Nutraceúticos, Universidad San Ignacio de Loyola (UNUSAN-USIL), Lima, Peru
- 2Facultad de Ciencias de la Salud, Universidad San Ignacio de Loyola, Lima, Peru
- 3Instituto de la Grasa, CSIC, Campus Universidad Pablo de Olavide, Sevilla, Spain
- 4Instituto de Ciencias de los Alimentos y Nutrición, Universidad San Ignacio de Loyola (ICAN-USIL), Lima, Peru
The chemical composition and CIELAB color parameters of oil from three aguaje morphotypes (Mauritia flexuosa L.f.) extracted by supercritical carbon dioxide was investigated. By chromatography (HPLC and GC), spectrometry (UV/vis), and digital image colorimetry (digital camera), carotenoids, tocopherols, tocotrienols, fatty acids, total polyphenols, and CIELAB color space were analyzed. These findings showed that the oil obtained from morphotype 3 was superior in several analytes (carotenoids, polyphenols, oleic acid, β-sitosterol, campesterol, and stigmasterol), while morphotype 2 and morphotype 1 showed very close profiles. The most similar chemical components in the oils of the three morphotypes were stigmasterol (16.00 to 17.81%), β-sitosterol (66.39 to 68.94%), palmitic acid (15.56 to 20.69%), and oleic acid (73.29 to 79.54%). The chromatic parameters (L*, a*, b*, and ) were quite different except for the hue angle (hab) (66.55 to 69.71 U), which showed some similarity. Aguaje oil is an interesting resource that stands out for its high content of carotenoids. All three morphotypes may be suitable for potential commercial applications.
Introduction
Aguaje or buriti (Mauritia flexuosa L.f.) is a palm tree native to the Peruvian Amazon (Koolen et al., 2018), a resource with great economic potential for the Amazon rainforest people. In Peru, M. flexuosa grows mainly in the swamp forest known as “aguajales” (Endress et al., 2013). This native species is also found in several Amazonian countries, such as Brazil, Colombia, Ecuador, Bolivia, Venezuela, Suriname, Guyana, and French Guiana (Virapongse et al., 2017). Although in all these countries M. flexuosa is found in the wild, in Peru, as a way of conservation, monoculture and agroforestry systems have been established (CIMA, 2012a) to meet the growing market demand for the aguaje fruit. Different parts of the plant, such as the leaves, flowers, fruits, stems, seeds, and roots are used by man for the manufacture of toys (dice and spinning tops), handicrafts (bags, baskets, hats, brooms, and hammock), and food products (soft drink “aguajina,” jam, aguaje pulp dough, porridge, ice cream, popsicles, and oil) (CIMA, 2012b; Mesa Castellanos et al., 2017; USIL, 2018). Other products fortified with M. flexuosa include cookies made with oil to improve the vitamin A and lipid profiles (Aquino et al., 2016), and milk-based beverage enriched with pulp lyophilized powder to increase the carotenoid content and improve the chromatic profile (Best et al., 2020). A recent study on the effect of buriti oil intake in the diet of lambs improved the tenderness, flavor, and “goat” aroma intensity in meat (de Sousa et al., 2022). Furthermore, it increases intramuscular fat and fatty acids in subcutaneous fat (Parente et al., 2020).
Several morphotypes have been described for the aguaje; these characteristics are associated with the mesocarp color. The yellowish-reddish color of the mesocarp is due to the concentration of carotenoid pigments. According to the mesocarp color, these are the following morphotypes: “amarillo” or “posheco” (when the pulp is yellowish), “color” (when only the superficial part is reddish), and “shambo” (whole pulp is reddish) (Delgado et al., 2007; Vásquez-Ocmín et al., 2010; CIMA, 2012b; Best et al., 2020). The use of aguaje fruit byproducts and pulp has been documented in recent years. Oil and defatted bran are obtained from pulp, whereas flour is acquired from exocarp (shell) and endocarp. The aguaje byproduct flours are rich in dietary fiber and phytochemical antioxidants (Resende et al., 2019). Different chemical components have been found in the aguaje pulp, including minerals, vitamins, phenolic compounds, and saccharides (Candido et al., 2015; de Souza et al., 2020), while aguaje oil is rich in fatty acids, sterols, carotenoids, and tocols (Serra et al., 2019; Mesquita et al., 2020).
The aguaje oil is rich in several constituents and has shown interesting biological properties, such as the antimicrobial and antioxidant activities (Santos et al., 2018). It has recently been reported that nanostructured lipid carriers composed of interesterified aguaje oil improve the bioavailability and stability of bioactive compounds, without showing any cytotoxic effects on Caco-2 and HepG2 cell lines (Reis et al., 2020). Furthermore, aguaje oil, especially its carotenoids (i.e., 13-cis-β-carotene, 9-cis-β-carotene, and α-carotene) have shown interaction energies against 2GTB peptidase. Therefore, these phytochemicals can become potential candidates with enzymatic inhibitory action to combat coronavirus disease (Costa et al., 2020). In another study, this oil showed no toxicity to mononuclear phagocyte system to human blood and increased the rate of cellular phagocytosis against enteropathogenic Escherichia coli (Cruz et al., 2020).
Different extractive techniques that include conventional (hydraulic pressing, expeller pressing, malaxing process, and solvent extraction) and eco-friendly technologies (supercritical fluid extraction, pressurized liquid extraction, microwave-assisted extraction, ultrasonic-assisted extraction, and pulsed electric fields extraction) have been used for the extraction of vegetable oils (Aydar, 2018; Ramos-Escudero et al., 2019; Veneziani et al., 2019; Zhang et al., 2019; Fomo et al., 2020). Supercritical fluid has been used in several Amazon palm trees for oil extraction (Orbignya phalerata, Oenocarpus distichus Mart., and Maximiliana maripa). The efficiency of supercritical fluid CO2 extraction depends on the variation of solubility with temperature (40 to 80°C) and pressure (20 to 35 MPa) (Cunha et al., 2019; de Oliveira et al., 2019; Barbi et al., 2020). This technique has been used to evaluate the extraction performance, the quality of lipids (fatty acids, sterols, tocols, terpenoids, and volatile compounds), plant pigments (non-polar compounds), and bioactive compounds (polar compounds), the latter using co-solvents, such as methanol, ethanol, and water (Radzali et al., 2020).
The aim of this study was to characterize the oil from three aguaje morphotypes extracted by supercritical fluid with CO2. The oils obtained through this green technology was evaluated for its chemical composition, lipid quality, and chromatic parameters.
Materials and Methods
Chemicals
All reagents were of analytical, chromatographic, and spectroscopic grade. Toluene, ethanol, 36% hydrochloric acid, methanol, ethyl acetate, acetone, tetrahydrofuran, pyrogallol, ammonium acetate, 2,2-diphenyl-1-picrylhydrazyl, and Folin–Ciocalteu's phenol reagent were purchased from Merck KGaA (Darmstadt, Germany). The standards α-cholestanol, all-trans-β-carotene, tocol standards, and gallic acid were supplied by Merck KGaA (Darmstadt, Germany). All other chemicals used were of the highest commercially available degree.
Raw Materials
The fruits of three morphotypes of aguaje that were used in this study were acquired from the “Veinte de Enero” Community (Iquitos, Loreto, Peru) in January 2019. The chromatic characteristics described for the pulp lyophilized powder of morphotypes of Mauritia flexuosa L.f. were L* (80.94, 80.86, and 76.96 U), a* (3.70, −0.68, and 9.35 U), and b* (47.70, 69.04, and 76.14 U) (Best et al., 2020). The pulp lyophilized powder was milled using an E310 commercial blender (Vitamix®, OH, USA) (Figure 1). Subsequently, the powder passed through an 800-μm mesh sieve. The material was vacuum packaged, kept at freezing temperature, and protected from light until extraction.
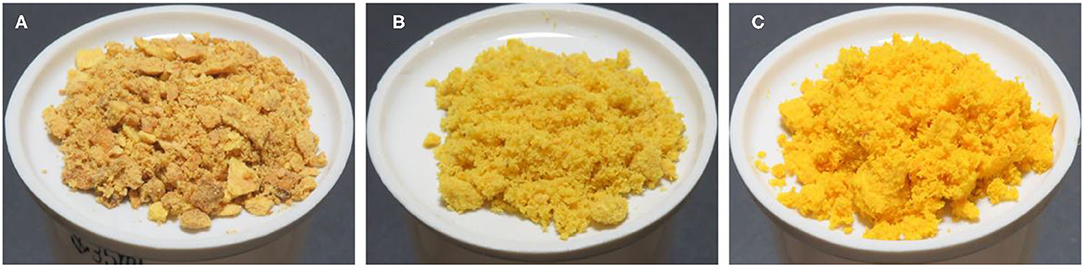
Figure 1. Pulp lyophilized powder of three aguaje morphotypes: (A) morphotype 1, (B) morphotype 2, and (C) morphotype 3.
Supercritical CO2 Oil Extraction
Supercritical fluid extraction was carried out with a Multi-solvent Extraction System (Top Industrie, Vaux-le-Pénil, France) equipped with CO2 and co-solvent pumps. Oil was obtained from aguaje pulp lyophilized powder via supercritical CO2 extraction under the conditions of 315.15 K, 20-MPa pressure, and CO2 mass flow was 42 g/min. Experiments were carried out using the extraction procedures described by Corzzini et al. (2017). The different morphotypes presented an oil content that varied between 46 and 55% (solvent extraction) (Best et al., 2020), the moisture in the samples was less than 6%, and the size of the particles ranged between 250 and 800 μm. The oil was collected every 15 min in 15-ml conical-bottom glass tubes with screw caps; when the oil was depleted in the matrix, each tube was filled with nitrogen in the headspace and protected from light with an aluminum foil to prevent oxidation. Sample oil was kept under refrigeration until the time of analysis.
Fatty Acid and Health-Promoting Index
Fatty acids were determined according to the method described by Ichihara and Fukubayashi (2010). Each oil sample (1 mg) was dissolved in 200 μl of toluene. This lipid solution was derivatized with a mixture of methanol (1.5 ml) and 8% HCl (300 μl). Then, the mixture was incubated at 45°C for 16 h in a water bath. After cooling to room temperature, 1 ml of hexane was added for the extraction of the fatty acid methyl esters. Fatty acid analysis was conducted using a gas chromatography system (Varian CP-3800, Walnut Creek, CA, USA). Separations were made on a FAMEWAX® WCOT fused silica capillary column (30 m × 0.25 mm i.d.: 0.25 μm film thickness, Restek Co.). The injector and detector temperatures were 250 and 260°C, respectively. The oven was kept at 120°C for 1 min, programmed to 160°C at a rate of 30°C/min, where it was maintained for 1 min, and then raised again to 240°C, at a rate of 4°C/min and then held constant for 7 min. Carrier gas flow rate (helium) was 1.0 ml/min, the injection volume was 1 μl (splitless mode). The result of the fatty acid content was expressed as a percentage of total fatty acids in the aguaje oil. The health-promoting index (HPI) was calculated as follows:
in which ΣUFA = the sum of unsaturated fatty acids, C12:0 = lauric acid, C14:0 = myristic acid, and C16:0 = palmitic acid.
Tocopherols and Tocotrienols Analysis
Tocol analysis was carried out according to the IUPAC standard method 2,432. A 10-mg quantity of oil was diluted with 1 ml of HPLC-grade hexane and directly injected into a liquid chromatograph with a Shimadzu RF-10AXL fluorescence detector. The separations were performed using a Si-column (250 × 4.0 mm i.d.; 4-μm particle size). The mobile phase used consisted of a mixture of hexane:isopropyl alcohol (99:1, v/v) at a flow rate of 1.0 ml/min, with an injection volume of 2 μl. The excitation and emission wavelengths are described in Chasquibol et al. (2016). Tocol standards were used for identification and quantification; the results were expressed in mg/kg oil.
Sterol Analysis
The sterol fraction was analyzed according to the methodology proposed by the Commission Regulation 2568/91 (EU) (2019) N° 2568/91. The oil sample (5 g) was placed in a 250-ml round-bottomed glass flask. The hot saponification was started by the incorporation of 50 ml of potassium hydroxide ethanolic solution (2 N) and lasted for 1 h under reflux. The extraction of the unsaponifiable matter was carried out in a 500-ml glass separating funnel with 80 ml of anhydrous diethyl ether (three times). Supernatant was washed using distilled water until complete neutralization. The diethyl ether phase was then dried with anhydrous sodium sulfate, and the residue was evaporated. Then 10 ml of acetone was added and evaporated again. The unsaponifiable matter was resuspended in 1.5 ml of ethyl acetate and the sterol fraction was separated by thin layer chromatography using a hexane:diethyl ether (65:35, v/v) mixture, and then revealed with a 2,7-dichlorofluorescein solution, recovered, filtered, evaporated, and derivatized (350 μl of a mixture of HMDS:TMCS:Pyridine, 3:1:9, v/v/v). GC analyses were carried out using an Agilent 6890N gas chromatograph (Agilent, Santa Clara, CA, USA) equipped with an SPB®-5 capillary column (30 m × 0.25 mm i.d.: 0.2-μm film thickness, Merck KGaA) and a flame ionization detector (FID). The oven program for the sterol analysis was isothermal at 265°C, with a 1:50 split ratio. Analysis time was 30 min, and flow rate was 1 ml/min, using hydrogen as the carrier gas. Identification and quantification of individual sterols was carried out as described in previous works (Fernandes et al., 2017).
Carotenoid Analysis
The saponification of the oil sample was carried out according to the description of Cortés-Herrera et al. (2019) with some modifications. A 50-mg quantity of oil was weighed and diluted with 1.2 ml of ethanol. Then 350 μl of potassium hydroxide (30 %) and 100 μl of pyrogallol (75 mg/ml) were added. Afterward, the mixture was made to saponify at 80°C for 2 h in a water bath. The unsaponifiable lipids were extracted with 5 ml of a mixture of hexane-ethyl acetate (8:2, v/v) and 1 ml of distilled water. The organic phase was recovered and evaporated by drying with a stream of nitrogen and then resuspended with 3 ml of acetone. This last phase was filtered through 0.45 μm PTFE membrane filter before HPLC analysis.
The analysis was performed with HPLC Hitachi LaChrom Elite® System equipped with a DAD. The software used for the acquisition of the chromatographic separations was EZChrom Elite 3.1.7. The analyte separations were conducted using a LiChroCART C18 column (250 × 4.6 mm i.d., 5-μm film thickness, Merck KGaA). The temperature of the column was maintained at 25°C. The mobile phase was a mixture of methanol HPLC (A), methanol-0.5 N ammonium acetate buffer (80:20, v/v) (B), and tetrahydrofuran (C), using the following gradient over a total run time of 35 min: at 0 min 100% B, at 5 min 98% A and 2% C, at 17.2 min 80% A and 20% C, at 25 min 80% A and 20% C, at 26 min 98% A and 2% C, at 28 min 100% B, and at 35 min 100% B, with a flow rate of 1 ml/min and an injection volume of 20 μl. Chromatograms were recorded at 450 nm. Carotenoid quantification was performed using calibration curves for β-carotene and all-trans-β-carotene; the results were expressed in mg/kg of oil.
Total Polyphenols
The total phenolic content of oil samples was determined using the Folin–Ciocalteu assay (Singleton et al., 1999). A 50-mg oil sample of was diluted with 450 μl of toluene. Of the dilution, 100 μl was mixed with 750 μl of Folin–Ciocalteu's phenol reagent (0.2 N), then the mixture was stirred for 5 min using an LP vortex mixer (Thermo Scientific, Waltham, MA, USA) at maximum speed. The polar fraction was recovered after centrifugation at 2,500 rpm over 5 min. Sodium carbonate (7.5%, 750 μl) was added to the translucent fraction and then allowed to react for 1 h at 37°C in a water bath. The developed blue color was measured at 725 nm using an Orion AquaMate 8100 UV-visible spectrophotometer (Thermo Scientific, Waltham, MA, USA). The results were expressed as milligram gallic acid equivalent per kg oil (mg GAE/kg).
Color Measurements
Color measurement of oil samples were carried out according to the methodology described by Milanez and Pontes (2014). Oil sample (1 ml) was previously centrifuged at 10,000 × g for 5 min in a 5418R micro-centrifuge (Eppendorf AG, Hamburg, Germany). Then the supernatant was put in a Quartz Suprasil pathlength 10 × 4-mm semi-micro cuvette (Hellma® Analytics, Müllheim, Germany). Image acquisition was obtained using a digital camera (Canon, Power Shot SX60 HS, full HD 65X optical zoom, Tokyo, Japan) with the Camera Connect App to transfer images shot to an iphone X smartphone (Apple Inc., USA) connected via wifi®. The illumination in the box was performed by using an OSRAM 17-W high power led lamp and a color temperature of 6,500 K. Red, green, and blue (RGB) channels were obtained using a digital image processing program (ImageJ 1.51j software, National Institutes of Health by Wayne Rasband, USA). Color squares images were generated by converting RGB values to lightness (L*), redness (a*), and yellowness (b*) values using the online software ColorHexa.com interface. The color attributes for (chroma) and hab (hue angle) were calculated as follows:
Statistical Analysis
All measurements were performed as follows: for chromatographic assays (n = 2), for UV/vis spectrophotometric assays (n = 3), and for digital image colorimetric assays (n = 10). Data are expressed as mean ± standard deviation. The analysis of variance (ANOVA) with Tukey's multiple comparison test was used to compare different aguaje morphotypes using the STATISTICA version 8.0 software (StatSoft, Inc., Tulsa, OK, USA) with significance set at p < 0.05. Clustered heatmaps and the degree of association among the different variables studied were verified using a Pearson's correlation heatmap in Metaboanalyst 5.0 (https://www.metaboanalyst.ca).
Results and Discussion
Fatty Acid Composition
The yield of aguaje lyophilized oil of pulp obtained through supercritical fluid-CO2 extraction varied between 44 to 46% (Table 1), while Lage et al. (2018) reported an oil content of around 56% in aguaje pulp (or buriti) using solvent extraction. Oil recovery percentages using supercritical fluid extraction is subject to various factors such as temperature, pressure, sample, and CO2 flow rate. Anjaneyulu et al. (2017) showed remarkably similar oil yields between solvent extraction (57.2%) and supercritical fluid extraction (57.0 %). However, the solvent-extracted oil presented higher levels of free fatty acid, peroxide value, and a high phosphorous content, compared with the supercritical CO2-extracted oil. Several authors have reported that supercritical fluid-extracted oils yield superior-quality oils (Naz et al., 2019).
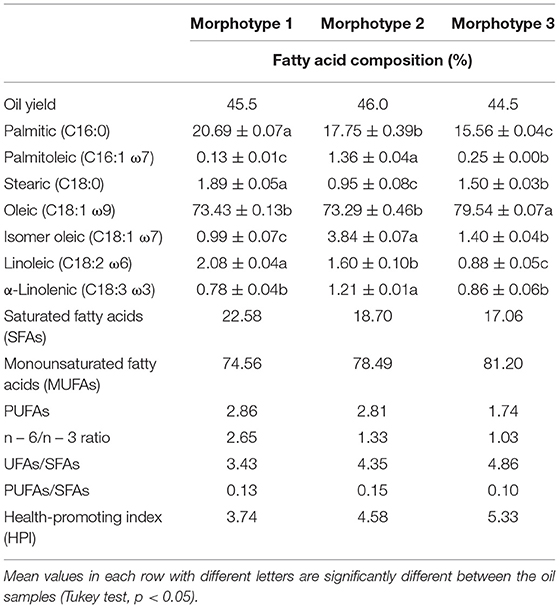
Table 1. Fatty acid composition of oil from three aguaje morphotypes obtained by supercritical fluid-CO2 extraction.
The fatty acids of oil from the three aguaje morphotypes were palmitic (C16:0), palmitoleic (C16:1 ω7), stearic (C18:0), oleic (C18:1 both ω9 and ω7 isomers), linoleic (C18:2 ω6), and α-linolenic (C18:3 ω3) acids (Table 1). This is in accordance with the description by de Souza et al. (2020). When all morphotypes were considered, the main fatty acids showed the following order: oleic acid (73.29 to 79.54%) > palmitic acid (15.56 to 20.69%) > linoleic acid (0.88 to 2.08%) > stearic acid (0.95 to 1.89%) > palmitoleic acid (0.13 to 1.36%). All fatty acids presented significant differences (p < 0.05), with morphotype 1 the one with the highest palmitic, stearic, and linoleic acid contents of the three. Morphotype 2 was mainly represented by palmitoleic, oleic, and linolenic acids, whereas morphotype 3 was a good source of oleic acid, whose content was 79.54%. Besides, monounsaturated fatty acids (MUFAs) represented between 74.56 and 81.20% of the total fatty acid content. These results are similar to the findings of Serra et al. (2019). MUFAs, especially oleic acid, have shown several beneficial effects on human health for the prevention of type 2 diabetes mellitus, reduces the systolic and diastolic blood pressure, reduces triglycerides and low-density lipoprotein, and inhibits the tumor necrosis factor α (TNFα) (Sales-Campos et al., 2013; Granado-Casas and Mauricio, 2019). Precisely, the oleic acid content in this oil is very similar to that of olive (70.1–82.5%), almond (50.4–81.2%), hazelnut (76.3–86.5%), and pecan nut (49.6–62.1%) oils (Ramos-Escudero et al., 2015; Fernandes et al., 2017). Noteworthy, the proportion of saturated fatty acids (SFAs) in this oil ranges from 17.06 to 22.58%. These values are in agreement with the findings of Vásquez-Ocmín et al. (2010), while this oil showed a low content of polyunsaturated fatty acids (1.74 to 2.86%). Consequently, the PUFAs/SFAs ratio was between 0.10 and 0.15, and the n – 6/n – 3 ratio ranged from 1.03 to 2.65. Compared with aguaje oil, chia and sacha inchi oils have higher PUFAs/SFAs ratios (7.46–8.03 and 9.45–13.57, respectively). These indexes are typically used to assess the impact of the diet on cardiac wellbeing, cancer, obesity, and inflammatory diseases (Simopoulos, 2016; Chen and Liu, 2020). On the other hand, the health-promotion index (HPI) in aguaje oil ranged from 3.74 to 5.33. Chen and Liu (2020) reported HPI values for dairy products (butter, cheese, cream, milk, and yogurt) in which they varied from 0.16 to 0.66. Food products with a high HPI value are healthier for human health.
Sterol, Tocopherol, and Tocotrienol Composition
Table 2 shows the sterol composition and total sterol contents of the oils obtained from the lyophilized pulp of the aguaje morphotypes. Among those, there was no significant difference (p > 0.05) regarding the following sterol content: chlerosterol, β-sitosterol, sitostanol, D7-stigmastenol, and D7-avenasterol. Concerning the sterol composition, all aguaje oils of different morphotypes showed the following order: β-sitosterol (66.39 to 68.94%) > stigmasterol (16.00 to 17.81%) > campesterol (5.43 to 5.97%) > cholesterol (4.20 to 6.01%). Other sterols were also present in concentrations below 1%, such as chlerosterol (<0.98%), D5-avenasterol (<0.94%), brassicasterol (<0.83%), sitostanol (<0.69%), D7-campesterol (<0.55%), D7-stigmastenol (<0.19%), and D7-avenasterol (<0.14 %). In morphotypes 2 and 3, D5,24-stigmastadienol was found with a content of 0.30 and 0.16%, respectively, while in morphotype 1, 24-methylene cholesterol was found with a value of 0.89%. Regarding total sterol contents, morphotype 2 had the highest concentration (1,181.00 mg/kg), whereas morphotypes 1 and 3 presented the lowest contents (835.35 and 850.40 mg/kg, respectively). These values are higher than those of other Arecaceae oils, such as palm (656 to 660 mg/kg) (Hassanien, 2013) but comparable with seje oil (Jessenia bataua) (985 to 1,551 mg/kg) (Navas Hernández et al., 2009).
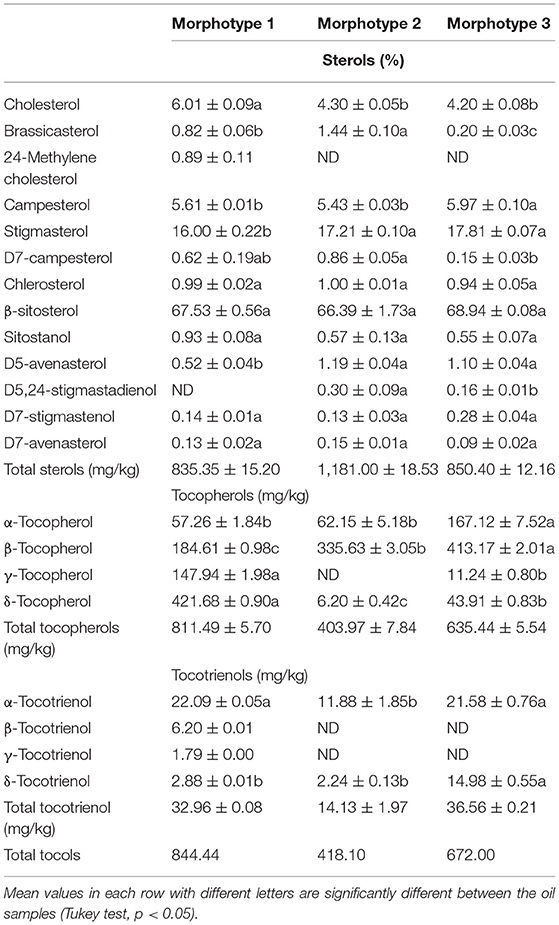
Table 2. Sterol, tocopherol, and tocotrienol contents of oil from three aguaje morphotypes obtained by supercritical fluid-CO2 extraction.
Four tocopherols (α, β, γ, and δ-tocopherols) were detected (Table 2), although γ-tocopherol was not found in morphotype 2. When all morphotypes were considered, the α-tocopherol content varied between 57.26 and 167.12 mg/kg, and β- and δ-tocopherol ranged from 184.61 to 413.17 mg/kg, and from 6.20 to 421.68 mg/kg, respectively. Furthermore, δ-tocopherol was present in morphotypes 1 (147.94 mg/kg) and 3 (11.24 mg/kg). The content of individual tocopherols in aguaje (Mauritia flexuosa L.f.) oil is highly variable in different studies (Santos et al., 2013; Speranza et al., 2016; Freitas et al., 2017; Serra et al., 2019). In our study, β-tocopherol was the main tocopherol in the aguaje oils obtained from morphotypes 2 and 3. These results are very similar to those found by Freitas et al. (2017) and Serra et al. (2019). On the contrary, δ-tocopherol was reported as the main tocopherol in morphotype 1. As seen in Table 2, the aguaje oils of the different morphotypes presented a content of total tocopherols ranging from 403.87 to 811.49 mg/kg. In agreement with the values in the literature, it was found that total tocopherols (1,040.14 to 2,364.10 mg/kg) were higher than those reported in this study (Santos et al., 2013; Speranza et al., 2016; Freitas et al., 2017; Serra et al., 2019). This variation is probably due to the type of oil extraction system, pedoclimatic conditions, morphotypes, and harvest maturity influence (Carvalho et al., 2017; Nayab et al., 2020).
The tocotrienol composition of oil from the three aguaje morphotypes studied in the present work is described in Table 2. Tocotrienols (α, β, γ, and δ) were detected in morphotype 1, while in morphotypes 2 and 3 only α- and δ-tocopherol were identified. α-Tocopherol was the most abundant one. The three aguaje morphotypes differed in tocotrienol contents. α-Tocotrienol ranged from 11.88 to 22.09 mg/kg of oil from three aguaje morphotypes, while δ-tocotrienol ranged from 2.24 to 14.98 mg/kg, comparable with those described by Serra et al. (2019) for buriti fruit oil extracted by cold pressing. The total content of tocotrienols in oil of morphotypes of aguaje ranged from 14.13 to 36.56 mg/kg. In addition, tocols contribute to the quality of vegetable oils as well as their bioactivity and health-promoting properties (Delgado et al., 2020).
Carotenoids and Total Phenolic Contents
Figure 2A showed an HPLC-DAD chromatogram of the carotenoid profile of the aguaje oil of morphotype 2. Carotenoid compounds eluted at 20.73, 25.15, 25.71, 26.08, and 26.57 min were unknown, β-carotene, all-trans-β-carotene, 9-cis-β-carotene, and 13-cis-β-carotene with λmax of 428, 454; 452, 477; 446, 471; and 444, 469 nm (Figures 2B–E). Five carotenoid compounds were identified and quantified as described in detail in Table 3. However, Santos et al. (2015) have identified 10 carotenoid compounds in buriti oil, namely, luteoxanthin, lutein, cis lutein, 5,8 epoxy β-carotene, cis γ-carotene, γ-carotene, cis α-carotene, α-carotene, cis β-carotene, and β-carotene. The content of individual carotenoids showed the following trend in aguaje morphotypes: morphotype 3 > morphotype 2 > morphotype 1. All-trans-β-carotene are mainly contributing to the total carotenoid content. The content of all-trans-β-carotene in the aguaje oils of different morphotypes varied from 846 to 3,962.65 mg/kg. On the contrary, β-carotene has been reported as the main carotenoid with a content of ~295 mg/kg of oil (Santos et al., 2015) compared with the oil from three aguaje morphotypes that showed more or less similar contents (174.43 to 347.11 mg/kg). Other significant carotenoids were 9-cis-β-carotene and 13-cis-β-carotene, whose concentrations ranged from 447.98 to 1,529.14 mg/kg, and 440.18 to 1,777.84 mg/kg, respectively. The total carotenoid levels in the oils from three aguaje morphotypes were higher than those reported by Santos et al. (2015) and Serra et al. (2019). Olive (21.50 mg/kg), sunflower (6.30 mg/kg), and palm (500–800 mg/kg) (Choudhary and Grover, 2019) oils had lower total carotenoid contents than the three aguaje morphotypes.
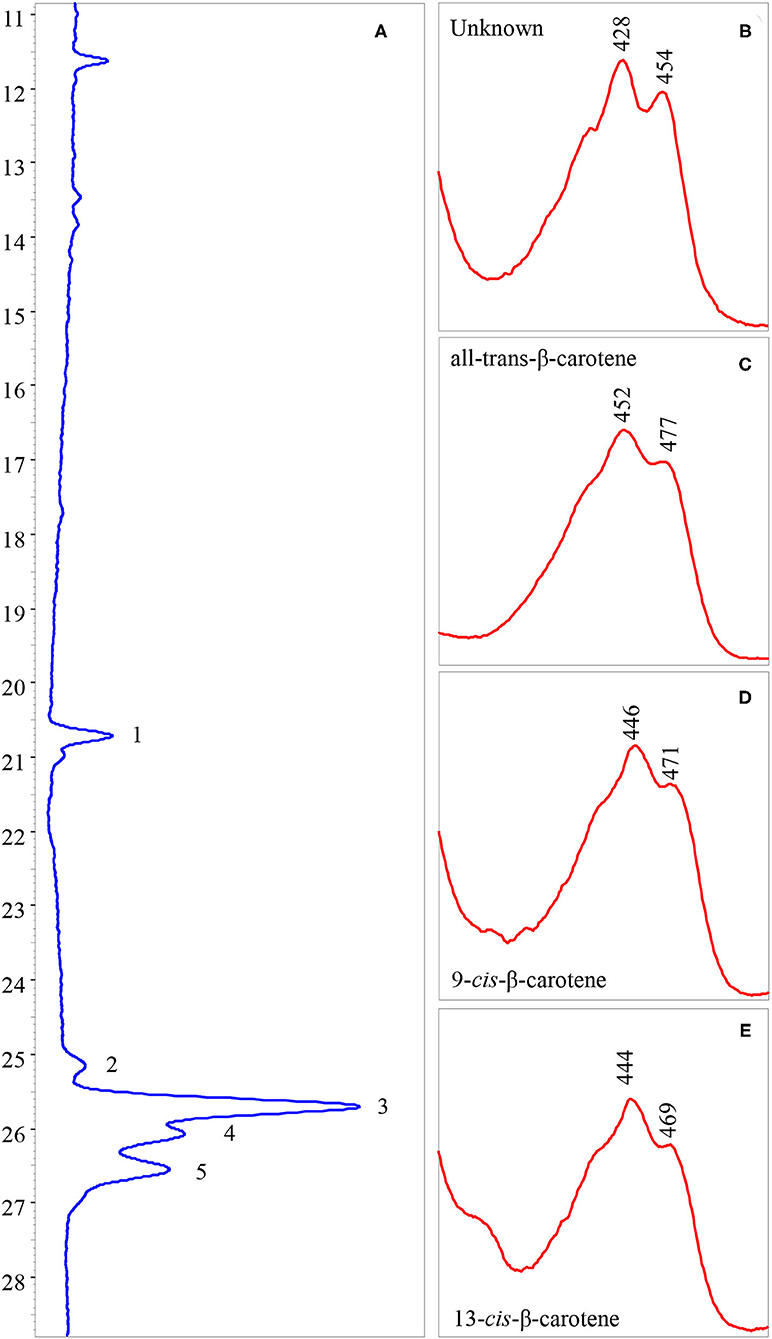
Figure 2. HPLC-DAD chromatogram of the carotenoid profile of the aguaje oil of morphotype 2 (A). UV-visible spectra for the individual carotenoids (B–E).
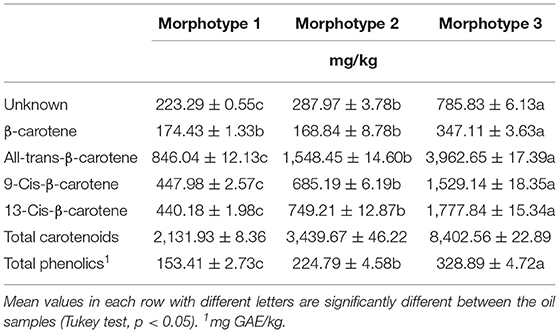
Table 3. Carotenoids and total polyphenol content of oil from three aguaje morphotypes obtained by supercritical fluid-CO2 extraction.
The total phenolic contents of aguaje oil samples are shown in Table 3. Statistical differences were found between the samples (p < 0.05). The oil obtained from morphotype 3 showed higher content of total polyphenols followed by morphotypes 2 and 1. According to our results, the aguaje oil obtained from these morphotypes ranged from 153.41 to 328.89 mg/kg and was much higher than the value (107.0 mg GAE/kg) reported previously by Speranza et al. (2016). The polyphenol content of aguaje oil is higher than those of other vegetable oils, such as palm (31.20 to 70.18 mg/kg), linseed (61.76 to 85.24 mg/kg), and sacha inchi (17.75 to 62.0 mg/kg) (Kaur et al., 2017; Abdullah et al., 2018; Ramos-Escudero et al., 2021) and is comparable with that of olive oil (86.90 to 367.60 mg/kg) (Ramos-Escudero et al., 2015). Vegetable oils possess a wide range of polyphenolic compounds including simple phenols, phenolic acids, tannins, phenylethanoids, esters of hydroxycinnamic acids, coumarins and chromans, stilbenes, flavonoids, lignans, and secoiridoids (Ramos-Escudero et al., 2021; Zeb, 2021). These antioxidant compounds are present in edible oils providing several activities (antioxidant, anti-inflammatory, anticancer, cytoprotective, and hypocholesterolemic). On the other hand, aguaje oil can become an excellent resource for the enrichment of vegetable oils and consequently used to improve the oxidative stability and shelf life due to its content of polyphenols and carotenoids.
Aguaje Oil Color
The results of the chromatic parameters are summarized in Table 4. The L* values of the oils from three aguaje morphotypes analyzed varied from 44.38 to 62.25 U, with morphotype 3 the one that showed the lowest L* values (42.62 to 46.40) compared with morphotype 2 (53.86 to 57.19) and morphotype 1 (59.17 to 64.87). Analysis of variance showed significant differences (p < 0.05) among morphotypes. The L* value of aguaje oil was higher than that of crude palm oil (30.57 to 31.05 U), while higher values were observed in virgin olive oil (78.76 U), virgin sunflower oil (83.21 U), virgin sacha inchi oil (91 to near 100 U), and refined sunflower oil (83.52 U) (Ramos-Escudero et al., 2019; Corbu et al., 2020). The high L* values may be observed in light-colored oil; this value may decrease due to the vegetable pigments in the edible oils. The chroma () values varied from 45.00 to 74.32 U. Moreover, it is interesting to point out that the values were close to the color component (b*) (47.04 to 67.81 U). This has also been observed in palm oil ( = 24.77 to 25.14 U; and b* = 20.05 to 21.13 U). However, in sacha inchi oil and olive oil, these values are virtually identical. These differences are probably due to the higher content of carotenoids in aguaje oil compared with other vegetable oils (refined palm oil, refined palm olein, refined palm stearin, olive oil, and sacha inchi oil) (Almeida et al., 2019; Ramos-Escudero et al., 2019). Regarding the a* chromatic coordinate, it was observed that the aguaje oil samples showed the following order: morphotype 1 (18.10 to 26.94 U), morphotype 2 (27.15 to 34.40 U), and morphotype 3 (42.61 to 51.35 U). Taking into consideration the three morphotypes, the coordinate a* showed an average of 33.60 U. Some vegetable oils that show positive values are crude palm oil, corn oil, and crude soybean oil, while oils with negative values include sunflower, olive, canola, grapeseed, sacha inchi, and refined oils (Almeida et al., 2019; Ramos-Escudero et al., 2019). Giacomelli et al. (2006) have reported that more positive a* values are related to yellowish, orange, and reddish colors. These colors are related to the content of carotenoids since in aguaje oil, the absorption of light occurs between 400 and 500 nm. The oil obtained from morphotype 3 shows higher absorbance than morphotype 2 and morphotype 1 (Figure 3). The hab readings obtained in the oil from three aguaje morphotypes varied from 62.54° to 72.96°. These results are higher when compared with crude palm oil, which ranged from 54.03° to 57.19° (Almeida et al., 2019). The observed hab values in the aguaje oils correspond to a visual orange tone, while the values that fluctuate between 20 to 40 correspond to a visual red color.
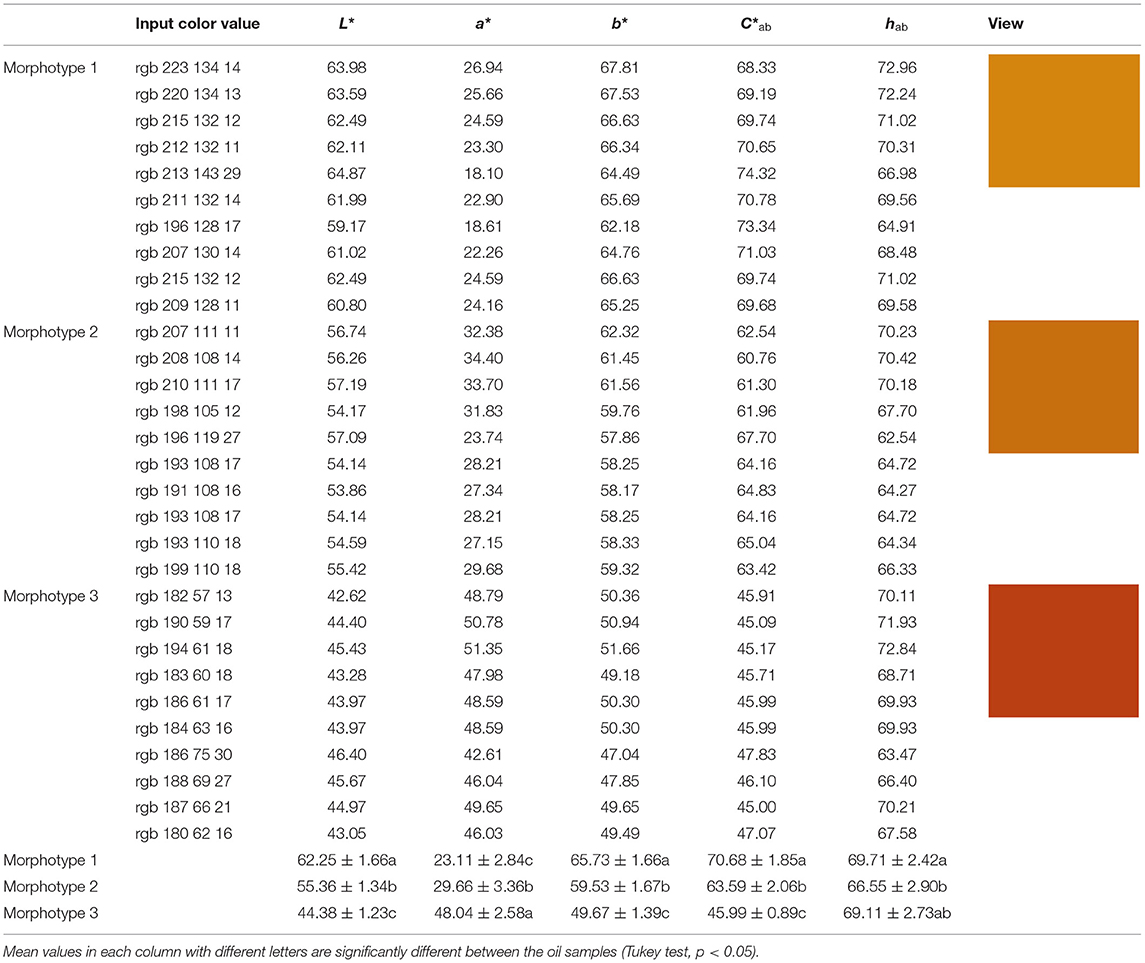
Table 4. Chromatic properties of oil from three aguaje morphotypes obtained by supercritical fluid-CO2 extraction.
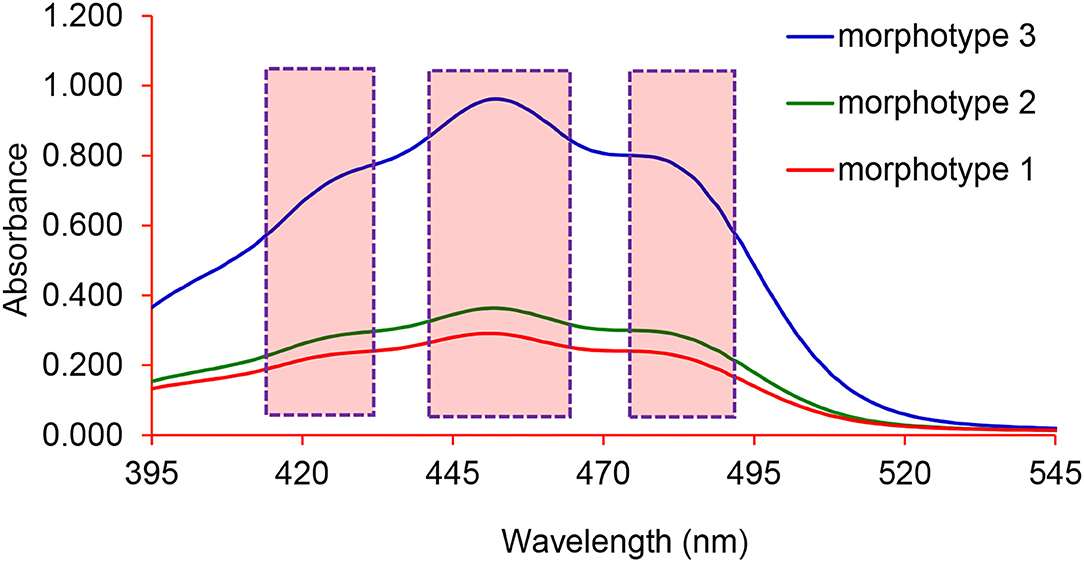
Figure 3. UV/vis spectra of oil from three aguaje morphotypes (Spectra were taken in glass cuvettes, 2-mm pathlength).
Correlations
As Figure 4 shows, the value of L* shows a high correlation (r > 0.7) with b* and , while little if any correlation is observed with a* and hab. In addition, the values of a* and hab showed a low correlation (r = −0.5 to 0.5) for all variables. These results agree with Abdelaali et al. (2018) that reported low correlations (a* values) with phytoene, lycopene, β-cryptoxanthin, zeaxanthin, antheraxanthin, (all-E) -violaxanthin, (9Z) -violaxanthin, β-carotene, and lutein (r = −0.287 to 0.156). In this study, the L*, b*, and values had a negative correlation with β-carotene, all-trans-β-carotene, 9-cis-β-carotene, and 13-cis-β-carotene in the aguaje oil samples. In addition, L*, b*, and were significantly positively related to the D7-avenasterol, linoleic, and palmitic (r > 0.8). Interestingly, the carotenoids, δ-tocotrienol, α-tocopherol, β-tocopherol, D7-stigmastenol, stigmasterol, campesterol, total phenolics, and oleic acid showed a high correlation (r > 0.7).
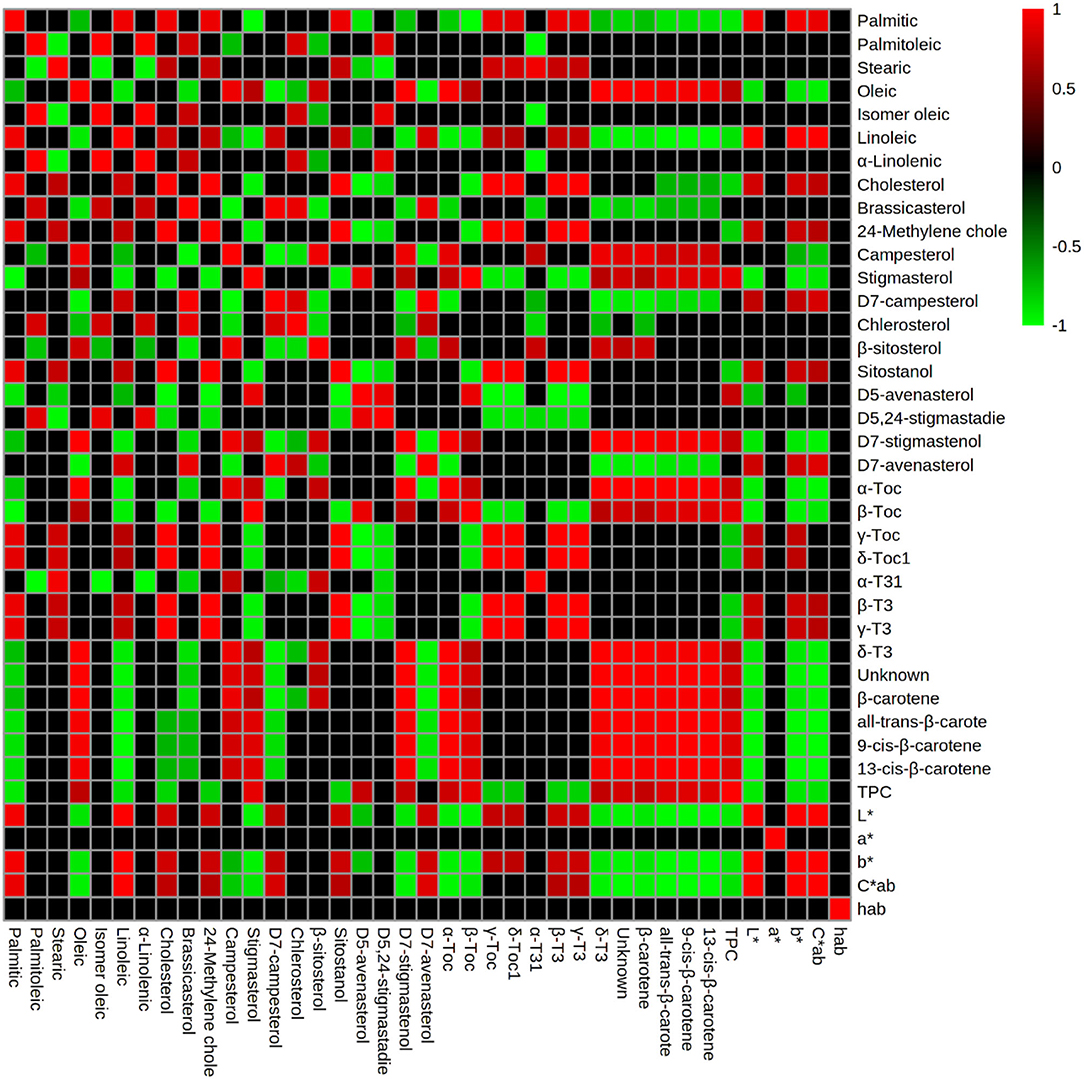
Figure 4. Heatmap plot of the correlation of the chemical compounds and chromatic parameters of oil from three aguaje morphotypes. The correlation coefficients are color coded from deep green (−1) to deep red (1).
The applied HCA on the datasets (Figure 5) showed two clusters: cluster 1 consisted of β-carotene, all-trans-β-carotene, 9-cis-β-carotene, 13-cis-β-carotene, total phenolics (TPC), δ-tocotrienol, α-tocopherol, β-tocopherol, oleic acid, stigmasterol, D5-avenasterol, D5,24-stigmastadiene, campesterol, beta-sitosterol, and D7-stigmastenol. Cluster 2 consisted of palmitoleic, isomer oleic, α-linolenic, stearic, palmitic, linoleic, chlerosterol, D7-avenasterol, brassicasterol, D7-campesterol, sitostanol, cholesterol, 24-methylene cholesterol, α-tocotrienol, β-tocotrienol, γ-tocotrienol, γ-tocopherol, and δ-tocopherol. These results show that the analytes with the highest abundance for each chemical compound are grouped in cluster 1, while those with the lowest relative abundance are listed in cluster 2. Besides, morphotype 1 and morphotype 2 are closely clustered, compared with morphotype 3, which is markedly separated.
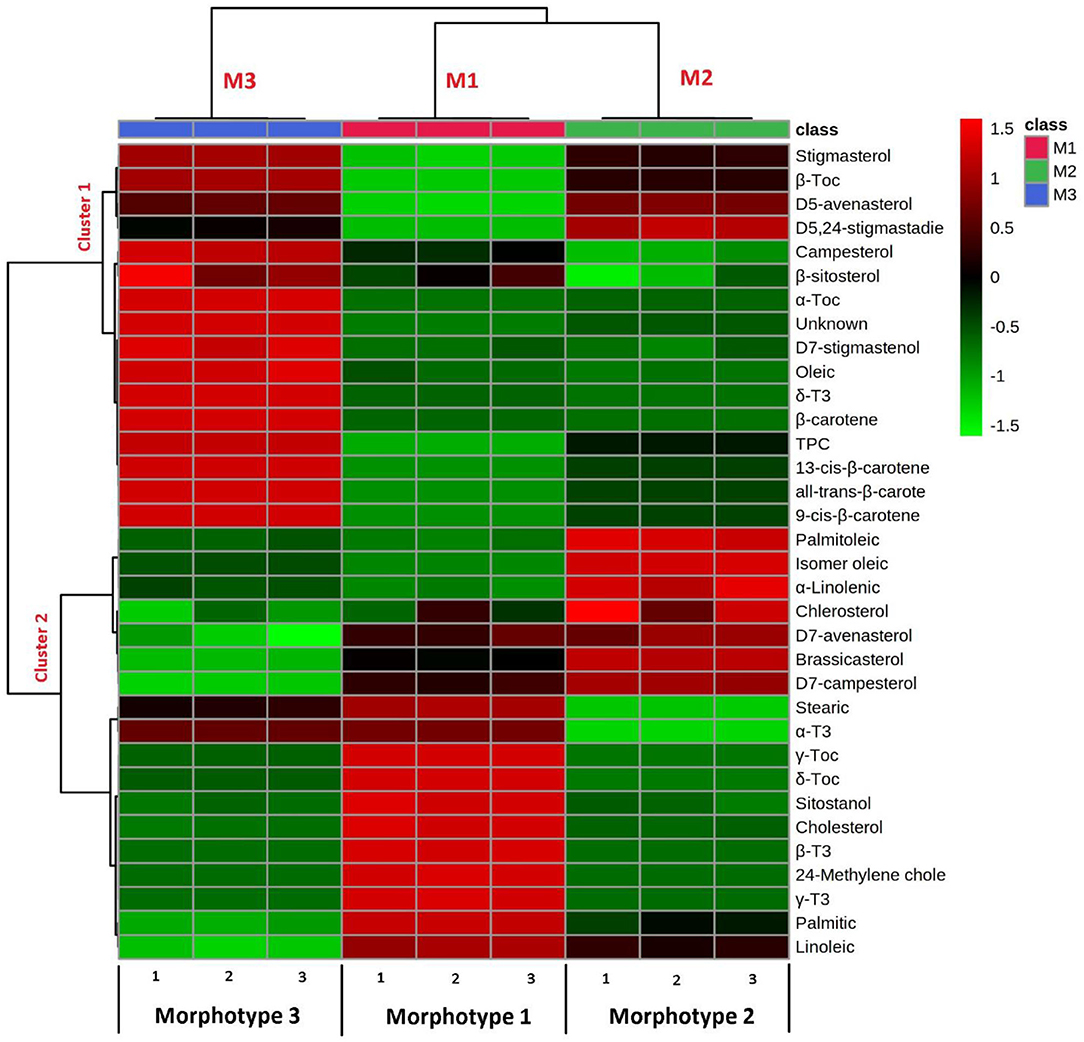
Figure 5. Hierarchical clustering and heatmap visualization of chemical compounds of oil from three aguaje morphotypes. Heatmap has a color scale from −1.5 to 1.5. The bottom side is represented by the green color (low content), while the upper side is represented by the red color (high content).
Ternary phase diagram for morphotypes 1, 2, and 3 are presented in Figure 6. In general, morphotype 1 oil exhibited a higher mean value for 24-methylene cholesterol, γ-tocopherol, δ-tocopherol, β-tocotrienol, and γ-tocotrienol. In addition, a higher concentration of palmitoleic, isomer oleic, brassicasterol, D7-campesterol, and D5,24-stigmastadienol were observed in morphotype 2 oil. Furthermore, it was observed that the morphotype 3 oil presented a higher content of α-tocopherol, δ-tocotrienol, all-trans-β-carotene, 9-cis-β-carotene, and 13-cis-β-carotene.
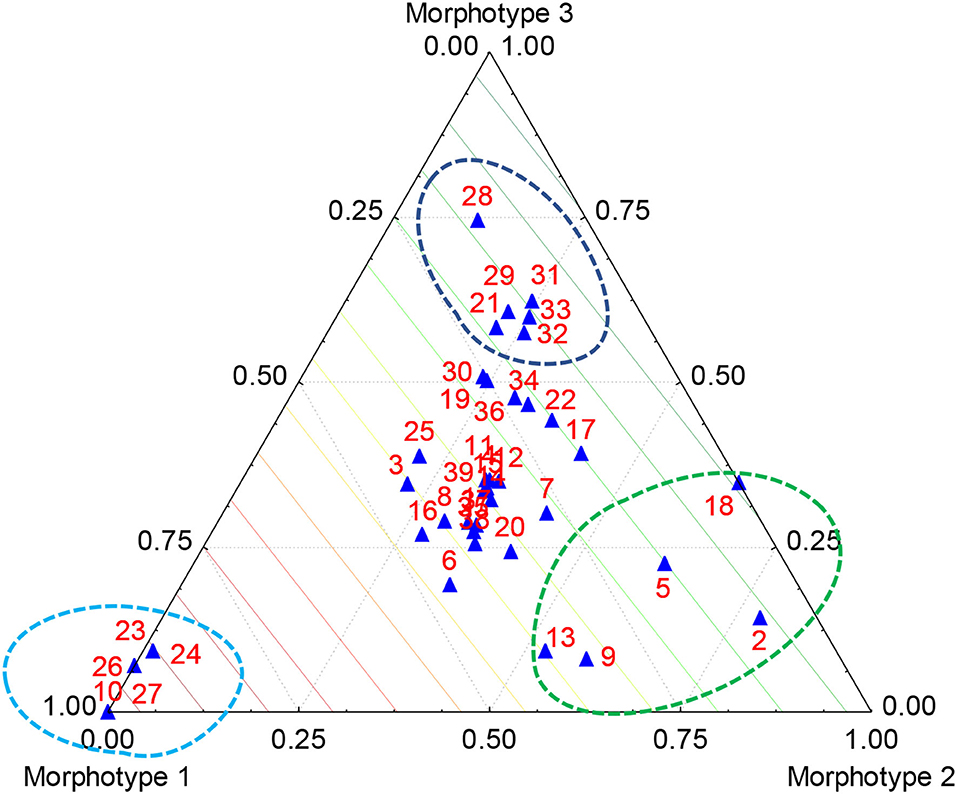
Figure 6. Ternary classification of the relative content of the chemical components that best characterize the oil from three aguaje morphotypes. Morphotype 1: 24-methylene cholesterol (10), γ-tocopherol (23), δ-tocopherol (24), β-tocotrienol (26), and γ-tocotrienol (27). Morphotype 2: palmitoleic (2), isomer oleic (5), brassicasterol (9), D7-campesterol (13), and D5,24-stigmastadienol (18). Morphotype 3: α-tocopherol (21), δ-tocotrienol (28), all-trans-β-carotene (31), 9-cis-β-carotene (32), 13-cis-β-carotene (33).
Conclusions
This study provides an analysis of the chemical composition and chromatic properties of oil from three aguaje morphotypes (Mauritia flexuosa L.f.) extracted by supercritical carbon dioxide. The results show important differences between morphotypes in relation to their chemical composition and CIELAB color parameters. The most relevant chemical components in aguaje oils (different morphotypes) were palmitoleic and w7 oleic acids, brassicasterol, 24-methylene cholesterol, campesterol, stigmasterol, D7-campesterol, D5,24-stigmastadienol, α-tocopherol, γ-tocopherol, δ-tocopherol, β-tocotrienol, γ-tocotrienol, δ-tocotrienol, all-trans-β-carotene, 9-cis-β-carotene, and 13-cis-β-carotene. The oil obtained from morphotype 3 presented higher content of carotenoids, polyphenols, oleic acid, β-sitosterol, campesterol, and stigmasterol, while morphotype 2 and morphotype 1 showed close similarity. The oils from three aguaje morphotypes provide an excellent source of bioactive components, especially carotenoids that can be used in the cosmetic, pharmaceutical, and food industries. In addition, it can be used as a replacement to improve the shelf stability of highly polyunsaturated oils due to its high concentration of oleic acid and considerable natural antioxidant content.
Data Availability Statement
The raw data supporting the conclusions of this article will be made available by the authors, without undue reservation.
Author Contributions
FR-E: conceptualization, methodology, investigation, formal analysis, writing-original draft, and project administration. RG-C: conceptualization, methodology, investigation, and writing-review and editing. AM: visualization, writing-reviewing and editing, supervision, and resources. LF-C: investigation, writing-reviewing and editing, and funding acquisition. MP-C: conceptualization, methodology, writing-reviewing and editing, supervision, and resources. All authors contributed to the article and approved the submitted version.
Funding
This project was funded by a special project—CACAOAGUAJE 2018-2020 (Universidad San Ignacio de Loyola, USIL).
Conflict of Interest
The authors declare that the research was conducted in the absence of any commercial or financial relationships that could be construed as a potential conflict of interest.
Publisher's Note
All claims expressed in this article are solely those of the authors and do not necessarily represent those of their affiliated organizations, or those of the publisher, the editors and the reviewers. Any product that may be evaluated in this article, or claim that may be made by its manufacturer, is not guaranteed or endorsed by the publisher.
Acknowledgments
We would like to acknowledge the Office of Management and Development (R&D&I) Projects of Universidad San Ignacio de Loyola for their collaboration in the acquisition of the morphotypes of Mauritia flexuosa L.f.
References
Abdelaali, S. B., Rodrigo, M. J., Saddoud, O., Zacarías, L., Hajlaoui, M. R., and Mars, M. (2018). Carotenoids and colour diversity of traditional and emerging Tunisian orange cultivars (Citrus sinensis (L.) Osbeck). Sci. Hortic. 227, 296–304. doi: 10.1016/j.scienta.2017.09.023
Abdullah, F., Ismail, R., Ghazali, R., and Idris, Z. (2018). Total phenolic contents and antioxidant activity of palm oils and palm kernel oils at various refining processes. J. Oil Palm Res. 30, 682–692. doi: 10.21894/jopr.2018.0058
Almeida, D. T., Viana, T. V., Costa, M. M., Silva, C. D. S., and Feitosa, S. (2019). Effects of different storage conditions on the oxidative stability of crude and refined palm oil, olein and stearin (Elaeis guineensis). Food Sci. Technol. 39, 211–217. doi: 10.1590/fst.43317
Anjaneyulu, B., Satyannarayana, S., Kanjilal, S., Siddaiah, V., and Prasanna Rani, K. N. (2017). A comparative study of solvent and supercritical CO2 extraction of Simarouba gluaca seed oil. Grasas Aceites. 68, e205. doi: 10.3989/gya.0214171
Aquino, J. S., Vasconcelos, M. H. A., Pessoa, D. C. N. P., Soares, J. K. B., Prado, J. P. S., Mascarenhas, R. J., et al. (2016). Intake of cookies made with buriti oil (Mauritia flexuosa) improves vitamin A status and lipid profiles in young rats. Food Funct. 7, 4442–4450. doi: 10.1039/C6FO00770H
Aydar, A. Y (2018). Emerging extraction technologies in olive oil production. In: Technological Innovation in the Olive Oil Production Chain, ed. I. Muzzalupo (London, UK: IntechOpen), Chapter 2.
Barbi, R. C. T., de Souza, A. R. C., de Melo, A. M., Teixeira, G. L., Corazza, M. L., and Ribani, R. H. (2020). Fatty acid profile and lipid quality of Maximiliana maripa oil obtained by supercritical CO2 and pressurized ethanol. J. Supercrit. Fluids. 165, 104979. doi: 10.1016/j.supflu.2020.104979
Best, I., Casimiro-Gonzales, S., Portugal, A., Olivera-Montenegro, L., Aguilar, L., Muñoz, A. M., et al. (2020). Phytochemical screening and DPPH radical scavenging activity of three morphotypes of Mauritia flexuosa L.f. from Peru, and thermal stability of a milk-based beverage enriched with carotenoids from these fruits. Heliyon. 6, e05209. doi: 10.1016/j.heliyon.2020.e05209
Candido, T. L. N., Silva, M. R., and Agostini-Costa, T. S. (2015). Bioactive compounds and antioxidant capacity of buriti (Mauritia flexuosa L.f.) from the Cerrado and Amazon biomes. Food Chem. 177, 313–319. doi: 10.1016/j.foodchem.2015.01.041
Carvalho, A. V., da Silveira, T. F. F., Mattietto, R. A., de Oliveira, M. S. P., and Godoy, H. T. (2017). Chemical composition and antioxidant capacity of açaí (Euterpe oleracea) genotypes and commercial pulps. J. Sci. Food Agric. 97, 1467–1474. doi: 10.1002/jsfa.7886
Chasquibol, N. A., Gómez-Coca, R. B., Yácono, J. C., Guinda, A., Moreda, W., del Aguila, C., et al. (2016). Markers of quality and genuineness of commercial extra virgin sacha inchi oils. Grasas Aceites. 67, e169. doi: 10.3989/gya.0457161
Chen, J., and Liu, H. (2020). Nutritional indices for assessing fatty acids: a mini-review. Int. J. Mol. Sci. 21:5695. doi: 10.3390/ijms21165695
Choudhary, M., and Grover, K. (2019). Palm (Elaeis guineensis Jacq.) oil. In: Fruit Oils: Chemistry and Functionality, ed. Ramadan, M. (Basel, Switzerland: Springer, Cham). p. 789–802. doi: 10.1007/978-3-030-12473-1_42
CIMA (2012a). Manejo sostenible del aguaje en la zona de amortiguamiento del parque nacional Cordillera Azul. Centro de Conservación, Investigación y Manejo de Áreas Naturales, Lima, Perú.
CIMA (2012b). Revalorización e importancia del aguaje en la zona de amortiguamiento del parque nacional Cordillera Azul. Centro de Conservación, Investigación y Manejo de Áreas Naturales, Lima, Perú.
Commission Regulation 2568/91 (EU) (2019). Regulation on the characteristics of olive oil and olive-residue oil and on the relevant methods of analysis. Official Journal of the European Union, L250. p. 1–14.
Corbu, A. R., Rotaru, A., and Nour, V. (2020). Edible vegetable oils enriched with carotenoids extracted from byproducts of sea buckthorn (Hippophae rhamnoides ssp. sinensis): the investigation of some characteristic properties, oxidative stability and the effect on thermal behaviour. J. Therm. Anal. Calorim. 142, 735–747. doi: 10.1007/s10973-019-08875-5
Cortés-Herrera, C., Chacón, A., Artavia, G., and Granados-Chinchilla, F. (2019). Simultaneous LC/MS analysis of carotenoids and fat-soluble vitamins in Costa Rican avocados (Persea americana Mill.). Molecules 24, 4517. doi: 10.3390/molecules24244517
Corzzini, S. C. S., Barros, H. D. F. Q., Grimaldi, R., and Cabral, F. A. (2017). Extraction of edible avocado oil using supercritical CO2 and a CO2/ethanol mixture as solvents. J. Food Eng. 194, 40–45. doi: 10.1016/j.jfoodeng.2016.09.004
Costa, A.N., de Sá, É.R., Bezerra, R.D., Souza, J.L., and Lima, F.D.C. (2020). Constituents of buriti oil (Mauritia flexuosa L.) like inhibitors of the SARS-Coronavirus main peptidase: an investigation by docking and molecular dynamics. J. Biomol. Struct. Dyn. 39, 4610–7. doi: 10.1080/07391102.2020.1778538
Cruz, M. B., Oliveira, W. S., Araújo, R. L., Franca, A. C. H., and Pertuzatti, P. B. (2020). Buriti (Mauritia flexuosa L.) pulp oil as an immunomodulator against enteropathogenic Escherichia coli. Ind. Crops Prod. 149, 112330. doi: 10.1016/j.indcrop.2020.112330
Cunha, V. M. B., da Silva, M. P., de Sousa, S. H. B., Bezerra, P. N., Menezes, E. G. O., da Silva, N. J. N., et al. (2019). Bacaba-de-leque (Oenocarpus distichus Mart.) oil extraction using supercritical CO2 and bioactive compounds determination in the residual pulp. J. Supercrit. Fluids. 144, 81–90. doi: 10.1016/j.supflu.2018.10.010
de Oliveira, N. A., Mazzali, M. R., Fukumasu, H., Gonçalves, C. B., and de Oliveira, A. L. (2019). Composition and physical properties of babassu seed (Orbignya phalerata) oil obtained by supercritical CO2 extraction. J. Supercrit. Fluids. 150, 21–29. doi: 10.1016/j.supflu.2019.04.009
de Sousa, S. V., Diogenes, L. V., Oliveira, R. L., Souza, M. N. S., Mazza, P. H. S., da Silva Júnior, J. M., et al. (2022). Effect of dietary buriti oil on the quality, fatty acid profile and sensorial attributes of lamb meat. Meat Sci. 186, 108734. doi: 10.1016/j.meatsci.2022.108734
de Souza, F. G., de Araújo, F. F., Farias, D. P., Zanotto, A. W., Neri-Numa, I. A., and Pastore, G. M. (2020). Brazilian fruits of Arecaceae family: An overview of some representatives with promising food, therapeutic and industrial applications. Food Res. Int. 138, 109690. doi: 10.1016/j.foodres.2020.109690
Delgado, A., Al-Hamimi, S., Ramadan, M. F., De Wit, M., Durazzo, A., Nyam, K. L., et al. (2020). Contribution of tocols to food sensorial properties, stability, and overall quality. J. Food Qual. 2020, 8885865. doi: 10.1155/2020/8885865
Delgado, C., Couturier, G., and Mejia, K. (2007). Mauritia flexuosa (Arecaceae: Calamoideae), an Amazonian palm with cultivation purposes in Peru. Fruits 62, 157–169. doi: 10.1051/fruits:2007011
Endress, B. A., Horm, C. M., and Gilmore, M. P. (2013). Mauritia flexuosa palm swamps: Composition, structure and implications for conservation and management. For. Ecol. Manag. 302, 346–353. doi: 10.1016/j.foreco.2013.03.051
Fernandes, G. D., Gómez-Coca, R.- B., Pérez-Camino, M. C., Moreda, W., and Barrera-Arellano, D. (2017). Chemical characterization of major and minor compounds of nut oils: Almond, hazelnut, and pecan nut. J. Chem. 2017, 2609549. doi: 10.1155/2017/2609549
Fomo, G., Madzimbamuto, T. M., and Ojumu, T. V. (2020). Applications of nonconventional green extraction technologies in process industries: challenges, limitations and perspectives. Sustainability. 12, 5244. doi: 10.3390/su12135244
Freitas, M. L. F., Chisté, R. C., Polachini, T. C., Sardella, L. A. C. Z., Aranha, C. P, M., Ribeiro, A. P. B., et al. (2017). Quality characteristics and thermal behavior of buriti (Mauritia flexuosa L.) oil. Grasas Aceites. 68, e220. doi: 10.3989/gya.0557171
Giacomelli, L. M., Mattea, M., and Ceballos, C. D. (2006). Analysis and characterization of edible oils by chemometric methods. J. Am. Oil Chem. Soc. 83, 303–308. doi: 10.1007/s11746-006-1204-0
Granado-Casas, M., and Mauricio, D. (2019). Oleic acid in the diet and what it does: implications for diabetes and its complications. In: Bioactive Food as Dietary Interventions for Diabetes, ed. Watson, R. R. and Preedy, V. R. (London, UK: Academic Press) 211–229. doi: 10.1016/B978-0-12-813822-9.00014-X
Hassanien, M. F. R (2013). Plant sterols and tocols profile of vegetable oils consumed in Egypt. Int. J. Food Prop. 16, 574–585. doi: 10.1080/10942912.2011.557758
Ichihara, K., and Fukubayashi, Y. (2010). Preparation of fatty acid methyl esters for gas-liquid chromatography. J. Lipid Res. 51, 635–640. doi: 10.1194/jlr.D001065
Kaur, R., Kaur, M., and Gill, B. S. (2017). Phenolic acid composition of flaxseed cultivars by ultra-performance liquid chromatography (UPLC) and their antioxidant activities: effect of sand roasting and microwave heating. J. Food Process. Preserv. 41,e13181. doi: 10.1111/jfpp.13181
Koolen, H. F., da Silva, F. M. A., da Silva, V. S. V., Paz, H. P. W., and Bataglion, G. A. (2018). Buriti fruit - Mauritia flexuosa. In: Exotic Fruits Reference Guide, ed. Rodrigues, S., Silva, E. O., and de Brito, E. S. (London, UK: Elsevier Inc.). p. 61–67. doi: 10.1016/B978-0-12-803138-4.00004-6
Lage, N. N., Lopes, J. M. M., Pereira, R. R., Guerra, J. F. C., Pereira, M. F. A., Silva, M., et al. (2018). Antioxidant potential of Buriti (Mauritia flexuosa) pulp flour in diabetic rats. Arch. Latinoam. Nutr. 68, 59–70.
Mesa Castellanos, L., Toro Buitrago, A., and Isaza Aranguren, C. (2017). Manejo de Mauritia flexuosa L.f. para la producción de artesanías en la altillanura colombiana. Colombia Forestal. 20, 85–101. doi: 10.14483/udistrital.jour.colomb.for.2017.1.a07
Mesquita, J. A., Oliveira, T. T. S., dos Santos, J. G. S., Gaspar, M. R. G. R. C., Vieira, V. A., Rodrigues, E. C., et al. (2020). Fatty acid profile and physicochemical characterization of buriti oil during storage. Cienc. Rural. 50, e20190997. doi: 10.1590/0103-8478cr20190997
Milanez, K. D. T. M., and Pontes, M. J. C. (2014). Classification of edible vegetable oil using digital image and pattern recognition techniques. Microchem. J. 113, 10–16. doi: 10.1016/j.microc.2013.10.011
Navas Hernández, P. B., Fregapane, G., and Salvador, M. D. (2009). Bioactive compounds, volatiles and antioxidant activity of virgin seje oils (Jessenia bataua) from the Amazonas. J. Food Lipids 16, 629–644. doi: 10.1111/j.1745-4522.2009.01171.x
Nayab, S., Razzaq, K., Ullah, S., Rajwana, I. A., Amin, M., Faried, H. N., et al. (2020). Genotypes and harvest maturity influence the nutritional fruit quality of mulberry. Sci. Hortic. 266, 109311. doi: 10.1016/j.scienta.2020.109311
Naz, S., Shabbir, M. A., Khan, M. R., and Shahid, M. (2019). Comparison of flaxseed oil characteristics of three Pakistani varieties obtained by supercritical CO2 and two conventional extraction methods. Int. Food Res. J. 26, 1599–1607.
Parente, M. O. M., Rocha, K. S., Bessa, R. J. B., Parente, H. N., Zanine, A. M., Machado, N. A. F., et al. (2020). Effects of the dietary inclusion of babassu oil or buriti oil on lamb performance, meat quality and fatty acid composition. Meat Sci. 160, 107971. doi: 10.1016/j.meatsci.2019.107971
Radzali, S. A., Markon, M., and Saleh, N. M. (2020). Co-solvent selection for supercritical fluid extraction (SFE) of phenolic compounds from Labisia pumila. Molecules. 25, 5859. doi: 10.3390/molecules25245859
Ramos-Escudero, F., González-Miret, M. L., Viñas-Ospino, A., and Ramos Escudero, M. (2019). Quality, stability, carotenoids and chromatic parameters of commercial Sacha inchi oil originating from Peruvian cultivars. J. Food Sci. Technol. 56, 4901–4910. doi: 10.1007/s13197-019-03960-x
Ramos-Escudero, F., Morales, M. T., and Asuero, A. G. (2015). Characterization of bioactive compounds from monovarietal virgin olive oils: relationship between phenolic compounds-antioxidant capacities. Int. J. Food Prop. 18, 348–358. doi: 10.1080/10942912.2013.809542
Ramos-Escudero, F., Morales, M. T., Ramos Escudero, M., Muñoz, A. M., Cancino Chavez, K., and Asuero, A. G. (2021). Assessment of phenolic and volatile compounds of commercial Sacha inchi oils and sensory evaluation. Food Res. Int. 140:110022. doi: 10.1016/j.foodres.2020.110022
Reis, L. V. C., Leão, K. M. M., Ribeiro, A. P. B., de Jesus, M. B., Macedo, G. A., and Macedo, J. A. (2020). Evaluation of cytotoxicity of nanolipid carriers with structured buriti oil in the Caco-2 and HepG2 cell lines. Bioprocess Biosyst. Eng. 43, 1105–1118. doi: 10.1007/s00449-020-02308-6
Resende, L. M., Franca, A. S., and Oliveira, L. S. (2019). Buriti (Mauritia flexuosa L. f.) fruit by-products flours: evaluation as source of dietary fibers and natural antioxidants. Food Chem. 270, 53–60. doi: 10.1016/j.foodchem.2018.07.079
Sales-Campos, H., de Souza, P. R., Peghini, B. C., da Silva, J. S., and Cardoso, C. R. (2013). An overview of the modulatory effects of oleic acid in health and disease. Mini Rev. Med. Chem. 13, 201–210. doi: 10.2174/1389557511313020003
Santos, J. S., Escher, G. B., Pereira, J. M. S., Marinho, M. T., do Prado-Silva, L., Sant'Ana, A. S., et al. (2018). 1H NMR combined with chemometrics tools for rapid characterization of edible oils and their biological properties. Ind. Crops Prod. 116, 191–200. doi: 10.1016/j.indcrop.2018.02.063
Santos, M. F. G., Alves, R. E., and Roca, M. (2015). Carotenoid composition in oils obtained from palm fruits from the Brazilian Amazon. Grasas Aceites. 66, e086. doi: 10.3989/gya.1062142
Santos, M. F. G., Alves, R. E., and Ruíz-Méndez, M. V. (2013). Minor components in oils obtained from Amazonian palm fruits. Grasas Aceites. 64, 531–536. doi: 10.3989/gya.048913
Serra, J. L., Rodrigues, A. M. C., de Freitas, R. A., Meirelles, A. J. A., Darnet, S. H., and da Silva, L. H. M. (2019). Alternative sources of oils and fats from Amazonian plants: fatty acids, methyl tocols, total carotenoids and chemical composition. Food Res. Int. 116, 12–19. doi: 10.1016/j.foodres.2018.12.028
Simopoulos, A. P (2016). An increase in the omega-6/omega-3 fatty acid ratio increases the risk for obesity. Nutrients. 8, 128. doi: 10.3390/nu8030128
Singleton, V. L., Orthofer, R., and Lamuela-Raventós, R. M. (1999). Analysis of total phenols and other oxidation substrates and antioxidants by means of Folin-Ciocalteu reagent. Meth. Enzymol. 299, 152–178. doi: 10.1016/S0076-6879(99)99017-1
Speranza, P., de Oliveira Falcão, A., Alves Macedo, J., da Silva, L. H. M., Rodrigues, A. M. C., and Alves Macedo, G. (2016). Amazonian buriti oil: chemical characterization and antioxidant potential. Grasas Aceites. 67, e135. doi: 10.3989/gya.0622152
USIL (2018). Aguaje: Fruto de la vida. Universidad San Ignacio de Loyola. Lima, Perú: Fondo Editorial USIL.
Vásquez-Ocmín, P. G., Alvarado, L. F., Solís, V. S., Torres, R. P., and Mancini-Filho, J. (2010). Chemical characterization and oxidative stability of the oils from three morphotypes of Mauritia flexuosa L.f, from the Peruvian Amazon. Grasas Aceites. 61, 390–397. doi: 10.3989/gya.010110
Veneziani, G., Esposto, S., Taticchi, A., Selvaggini, R., Sordini, B., Lorefice, A., et al. (2019). Extra-virgin olive oil extracted using pulsed electric field technology: cultivar impact on oil yield and quality. Front. Nutr. 6, 134. doi: 10.3389/fnut.2019.00134
Virapongse, A., Endress, B. A., Gilmore, M. P., Horm, C., and Romulo, C. (2017). Ecology, livelihoods, and management of the Mauritia flexuosa palm in South America. Glob. Ecol. Conserv. 10, 70–92. doi: 10.1016/j.gecco.2016.12.005
Zeb, A (2021). A comprehensive review on different classes of polyphenolic compounds present in edible oils. Food Res. Int. 143, 110312. doi: 10.1016/j.foodres.2021.110312
Keywords: aguaje oil, supercritical CO2, fatty acids, sterols, tocols, carotenoids, CIELAB color space
Citation: Ramos-Escudero F, Gómez-Coca RB, Muñoz AM, Fuente-Carmelino LDL and Pérez-Camino MdC (2022) Oil From Three Aguaje Morphotypes (Mauritia flexuosa L.f.) Extracted by Supercritical Fluid With CO2: Chemical Composition and Chromatic Properties. Front. Sustain. Food Syst. 6:843772. doi: 10.3389/fsufs.2022.843772
Received: 26 December 2021; Accepted: 26 January 2022;
Published: 15 March 2022.
Edited by:
Cristobal N. Aguilar, Universidad Autónoma de Coahuila, MexicoReviewed by:
Mohamed Fawzy Ramadan Hassanien, Umm Al-Qura University, Saudi ArabiaJorge Wong-Paz, Technological Institute of Ciudad Valles, Mexico
Copyright © 2022 Ramos-Escudero, Gómez-Coca, Muñoz, Fuente-Carmelino and Pérez-Camino. This is an open-access article distributed under the terms of the Creative Commons Attribution License (CC BY). The use, distribution or reproduction in other forums is permitted, provided the original author(s) and the copyright owner(s) are credited and that the original publication in this journal is cited, in accordance with accepted academic practice. No use, distribution or reproduction is permitted which does not comply with these terms.
*Correspondence: Fernando Ramos-Escudero, ZHJhbW9zJiN4MDAwNDA7dXNpbC5lZHUucGU=; Raquel B. Gómez-Coca, cmFxdWVsLmNvY2EmI3gwMDA0MDtpZy5jc2ljLmVz