- 1Department of Horticulture, College of Agriculture and Environmental Sciences, University of Gondar, Gondar, Ethiopia
- 2Department of Rural Development and Agricultural Extension, College of Agriculture and Environmental Sciences, University of Gondar, Gondar, Ethiopia
The purpose of this research was to determine the optimum dehydration temperature and time length for a better physicochemical quality of tomato powders. The treatments consisted of 70, 80 and 90°C dehydration temperatures and 9, 11, and 13 h time durations arranged in a completely randomized design (CRD) with triplicates. The inlet and outlet air temperatures of the hot air oven dryer were about 24 and 60°C respectively with an air velocity of about 2.9 m/s. Fresh tomatoes were used as a control. It has been observed that the major quality parameters of tomato powders were significantly increased with increasing dehydration temperature and time up to 80°C and 11 h respectively. When the dehydration temperature and time length went beyond 80°C and 11 h respectively, the quality of tomato powders declined. The maximum TSS content (7.433 °Brix), titratable acidity (0.4967), rehydration ratio (3.437), lycopene content (0.4512 mg/100 g) and lower pH value (4.433) were obtained at 80°C temperature and 11 h dehydration time. Moisture content was reduced to 11% at the same dehydration temperature and time length without considerable physiochemical quality loss. Inversely, vitamin C was reduced as dehydration temperature and time increased. Generally, the results of this study showed that dehydration of tomatoes with 80°C and 11 h temperature and time length respectively, provides better physicochemical quality of tomato powders. Future research is suggested on consumers' acceptance of dehydrated tomato powders.
Introduction
Tomato (Solanum lycopersicum L.) belongs to the family Solanaceae is one of the most popular vegetable crops worldwide with a great nutritional contribution to the human diet (Dewanto et al., 2002; Ali et al., 2021). Tomato contains carotenoids (lycopene and β-carotenoids) which are primarily known for their health benefits (Sirivastava and Kulshreshtha, 2013). It is an excellent source of vitamin C, biotin, vitamin K, vitamin A (in the form of beta-carotene), vitamin B1, vitamin E, foliate and niacin (Ali et al., 2021). Tomato is consumed in fresh or processed forms such as paste, sauce, juiced and dehydrated slices or powder (Abdullahi et al., 2016). Tomato is also the most processed vegetable crop worldwide (Kozlova et al., 2020). However, its nutritional value, texture, appearance, color, flavor and taste are all subject to change as a result of the dehydration process (Taylor et al., 2008; Zhang et al., 2017).
Tomatoes are highly perishable fruit vegetables from which the postharvest losses reach 25 to 50%. Various dehydration methods have been developed to preserve tomatoes for extended use. Out of these methods, hot-air dehydration, microwave dehydration, solar-tunnel dehydration and freeze-dehydration are the widely employed for tomato dehydration (Singh and Heldman, 2014; Zhang et al., 2017). Hot-air dryers have been mostly preferred for low operating cost, lingering microorganisms hence better food safety and shelf life as well as a less environmental influence (Mujumdar and Law, 2010; Rayaguru and Routray, 2011; Zhang et al., 2017).
Authors (Subramanian, 2016; Karla and Flávio, 2019) reported that dehydrated tomatoes have inferior nutritional quality compared to fresh fruits. In contrast, many others (Khazaei et al., 2008; Ma et al., 2012; Mohammed and Malami, 2013; Tilahun et al., 2021) argue that dehydration of tomatoes increases the concentration of sugars (sources of energy) and carotenoids (lycopene and β-carotenoids) hence, dehydration enhances the quality of tomato. Additionally, dehydration can prevent or linger the growth of most microorganisms by reducing water activity and lowering pH (Amit et al., 2017). On the other hand, temperature extremes along with an extended dehydrating period may lead to a significant loss of vitamins, flavor and aroma (Mujumdar and Law, 2010; Alfeo et al., 2021). Several authors also reported different optimum dehydrating temperatures and time lengths for tomatoes; (Idah et al., 2010) 90°C for 1 h; (Correia et al., 2015) 60°C for 35–44 h; (Ashebir et al., 2009) 75°C for 3 h and (Yusufe et al., 2017) 80°C for 7 h. The quality of a dehydrated tomato depends on the variety of the crop, total soluble solid content (°Brix) of the fresh product, dehydrating air humidity, the size of the tomato segments, the air temperature and velocity and the efficiency of the dehydration system (Ghavidel and Davoodi, 2010). Consequently, all the aforementioned combinations of dehydrating temperatures and time length are seldom effective for improved hybrid tomato varieties having higher fruit moisture content, thick and firm pericarp.
Global tomato production is rapidly increasing because of enhancing agricultural technologies such as improved varieties, fertilization, pest management, irrigation systems, protected cultivation, and hydroponics global tomato production (Costa and Huevelink, 2007; Sora, 2018). Nonetheless, large quantities of tomatoes are not reaching consumers due to post-harvest losses (FAO and World Bank, 2010). The postharvest loss is severe in developing countries where processing technologies are limited. Up to 50% of the produced tomatoes are lost during harvesting, handling, transporting, storage, and distribution, as well as at the wholesalers and retailers level (Kasso and Bekele, 2016; Minten et al., 2019). At peak harvest season, the selling price of tomatoes declines below the cost of production while in the off-seasons, the price hikes beyond the reach of average consumers. This critical problem causes market fluctuation, monetary loss and inadequate food intake (Gabriel, 2021). Postharvest problems also affect food safety and consumers' acceptability (Saran et al., 2017). Determining the optimum dehydrating temperature and time length will assure a year-round supply of dehydrated tomato powder with an average price thus producers, wholesalers, retailers and consumers will be benefited. This research was therefore initiated to investigate the optimum dehydrating temperature and time for better physicochemical quality retention of tomato powder.
Materials and Methods
The dehydration process was conducted at Horticulture department laboratory of College of Agriculture and Environmental Sciences, University of Gondar, Ethiopia and physicochemical quality of the dehydrated tomatoes were analyzed in Institute of Technology, Faculty of Chemical and Food Engineering, Bahir Dar University, Ethiopia.
Experimental Materials, Standards, and Chemicals
The tomato fruits, Gelilea hybrid variety grown on farmers' open-field were used for the dehydration process. Galilea is a hybrid tomato variety released by Hazera Genetics Ltd., Israel (MANR, 2016) produced by Hazera Seeds Ltd, South Africa (Pty) and commercially supplied in Ethiopia by Greenlife Trading PLC. The variety is high-quality Roma-type fruit with a high yield and wide range of resistances, good for bush and stacking production, adapted for various climates, and suitable for processing (https://hazeraafrica.com/?s=galilea, October 2021). Thus, it is preferred by most of the producers and processing industries. Chemical and standards such as polyphenol standards (gallic acid, tannic acid, and catechin) with > 97.5% purity, FolinCiocâlteu reagent, aluminum chloride, oxalic acid, ascorbic acid, sodium nitrite, 2, 2-diphenyl-1-picrylhydrazyl (DPPH) and 2, 6-dichlorophenol indophenol dye were also used.
Treatments and Experimental Design
Treatments and experimental design dehydration temperature (70, 80, and 90°C) and time length (9, 11, and 13 h) were the experimental factors. 60°C temperature and 7 h time length were initially included, yet rejected after inefficient results. Fresh tomato fruits were used as a control. All treatment combinations were triplicated forming 30 total runs aligned in a completely randomized design (CRD).
Sample Preparation and Dehydration Process
Sample preparation and dehydration process fully matured tomato fruits, “Gelilea” variety were collected from farmers' fields, Mecha district. The variety “Galilea” is produced by Hazera Seeds Ltd, South Africa (Pty) and commercially supplied in Ethiopia by Greenlife Trading PLC. Before the dehydration process begins, the tomato fruits were sorted to remove the damaged ones, washed in clean water and weighed. The tomatoes were then subjected to hot water blanching for 3–5 mins and be allowed to cool afterwards (Figure 1). The blanched tomatoes were measured by calipers and cut into 8 mm thick slices using a sharp stainless steel knife (Jayathunge et al., 2012). To assure uniform dehydration, each of the tomato slices was spread out on aluminum foil and placed in a single layer in a hot air oven. Then, the predetermined temperatures of 70, 80, and 90°C were adjusted until the required period 9, 11, and 13 h. The inlet and outlet air temperatures were about 24 and 60°C, respectively with an air velocity of about 2.9 m/s air velocity (Nurkhoeriyati et al., 2021). Finally, dehydrated tomato slices were cooled for about an hour inside desiccators to prevent reabsorption of moisture and packed in a plastic bag. Then, the physicochemical quality of the dehydrated tomatoes was analyzed in the laboratory of Bahir Dar University, Institute of Technology, Faculty of Chemical and Food Engineering.
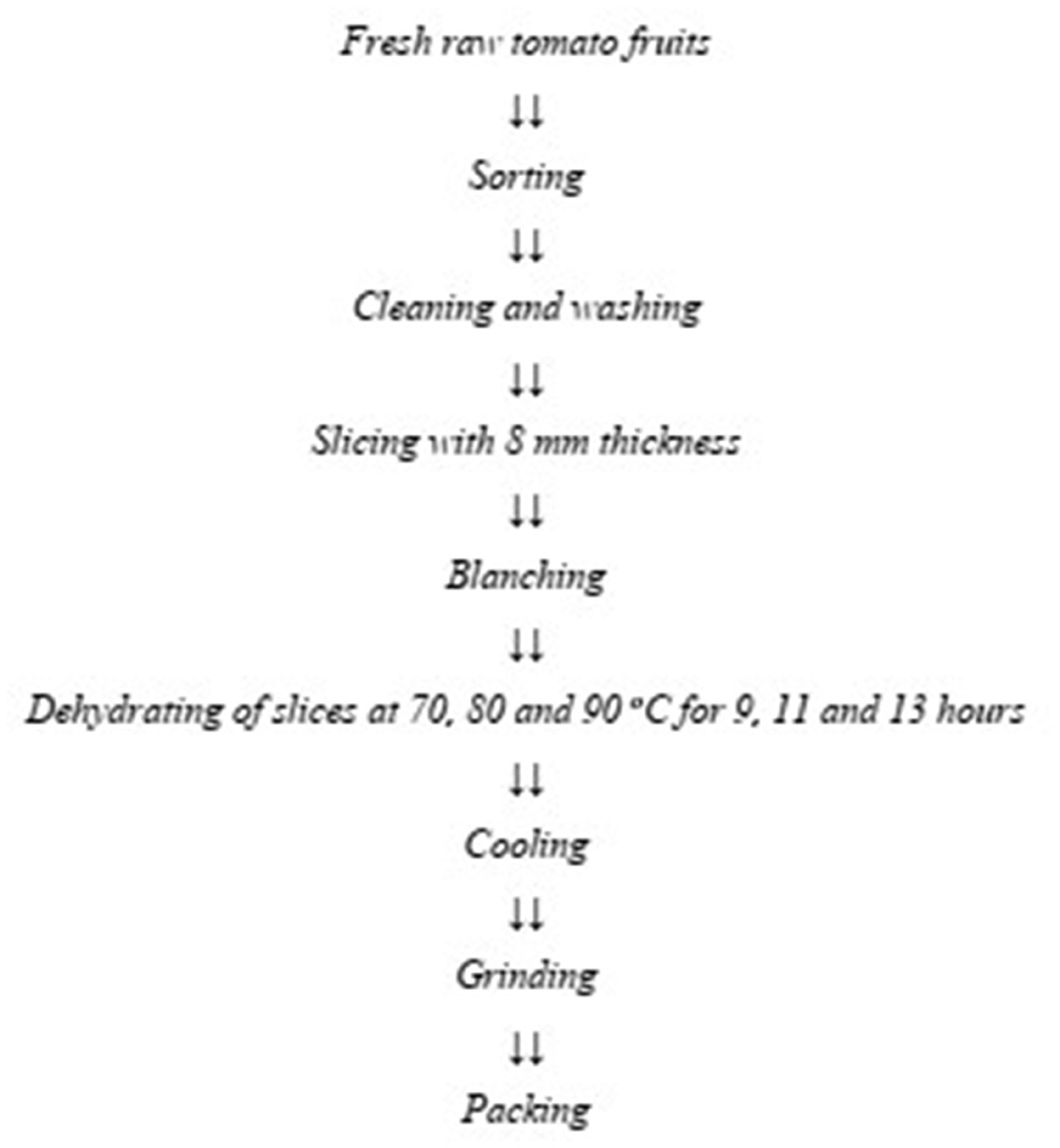
Figure 1. Flowchart for tomato dehydration process adopted and modified from Surendar et al. (2018).
Preparation of Tomato Powder
Preparation of tomato powder the dehydrated tomato slices were collected and ground using a clean household grinder packed and stored in sealed plastic containers before further analysis. Optimum processing conditions to develop dehydrated tomato powder were determined based on product color, moisture content, physicochemical analysis and rehydration ratio of the powder.
Procedures for Preparing Tomato Powder
Moisture Content Determination
An aluminum foil was weighed then the digital sensitive balance was reset to zero. The initial moisture content of the slices was measured by drying in an oven at 105°C for 24 h, moved to the desiccator then allowed to cool and dehydrated samples were weighed and expressed as kg water/kg dry solids (AOAC, 2000) which varied between 12.477 and 13.492 kg water/kg dry solids. The 500 g sliced samples were placed on the aluminum foils (W1). The samples were put in the oven at 70, 80 and 90°C for 9, 11, and 13 h. Then, the samples were moved to the desiccator and allowed to cool. The dehydrated samples were weighed (W2). The percent moisture contents of dehydrated tomato powders were calculated as follows and expressed in percent;
Where,
W1 = weight of the sample before dehydration
W2 = weight of the sample after dehydration.
Physicochemical Quality Analysis
pH and Titratable Acidity (TA)
pH and titratable acidity (TA) were obtained following AOAC (2000) procedures. The pH was measured using the Hanna pH meter at about 27°C temperature. Five grams of samples were dissolved in 50 ml distilled water. After shaking well the samples, the pH was measured with a well-calibrated pH meter. During TA estimation, 5 ml of sample was titrated with 0.1 N NaOH up to pH 8.1. Results were expressed in grams of citric acid per 100 g of dry tomato weight.
Where TA = titratable acidity
*Equivalent weight of acid = 0.0064 (Citric acid).
Determination of Ascorbic Acid (Vitamin C) Content
About 5 ml of a standard solution of ascorbic acid was pipetted into a 100 ml conical flask. 10 ml of oxalic acid was added and the solution titrated against the dye (V1 ml) until a pink color persisted for 15 seconds. The dye that was consumed is equivalent to the amount of ascorbic acid. Also, 0.5 g of the sample was extracted in 4% oxalic acid and made up to 100 ml. The solution was filtered. 10 ml of oxalic acid was added to 5 ml of the filtrate above. The solution was then titrated against the dye solution (2, 6—dichlorophenol indophenol). The volume of dye used was recorded as (V2 ml) (Ibitoye, 2005).
Where W = sample weight.
Total soluble solids (TSS)
Total soluble solids (TSS) was determined according to AOAC (2000) method using an Atago DR-A1digital refractometer (Atago Co. Ld., Japan) at 25°C. The result was expressed as a percent.
Determination of Lycopene Content
Determination of lycopene content lycopene estimation was done by using (AOAC, 2000). The samples 5 to 10 g were extracted with acetone. The extraction was repeated until the filtrate changed colorless. The acetone extract was then moved to a separating funnel filled with 10 to 15 ml petroleum ether. Then, 5% sodium sulfate solution was added. The lower acetone phase was repeatedly extracted with petroleum ether similarly until it become colorless. The upper petroleum ether extract was pooled and its volume made up to 50 ml with petroleum ether and the color was measured in a 1 cm cell at 503 nm in a spectrophotometer (Systronics UV-VIS double beam spectrophotometer 2201) using petroleum ether as blank. Finally, the lycopene was calculated using the following equation:
where A is the absorbance at 503 nm, D is the dilution of extract to 100 ml, and W is the weight of the sample taken.
Color Measurement
Color measurement the color of dehydrated tomatoes was measured by hunter color difference meter (Color Quest XE, Hunter Lab), calibrated with a white tile. “L” represents the lightness index (“0” for black to “100” for white), “a” represents redness and greenness (“+100” for red and “−80” for green) while, “b” represents for yellowness and blueness (“+70” for yellow and “−80” for blue). Three replications of each of the treatment combinations were analyzed.
Rehydration Capacity
Rehydration capacity the determination of rehydration capacities of tomato powders were determined following a procedure set by Lewicki (1998). The initial weight was taken by weighing 2 grams of sample powder. This sample was added into 250 ml beakers and dissolved with 50 ml of distilled. After about 2.0 h, the water was filtered using filter paper by a vacuum pump (D-7800, German) until all the water was drained out at room temperature. The suspended water was removed by laboratory tissue paper. Lastly, the weight of water absorbed by samples was taken as the final weight. Then, rehydration capacities were determined using the following formula.
Statistical Analysis
The data generated from laboratory analysis were subjected to analysis of variance (ANOVA) using the GenStat 18th Edition (64 bit) statistical software and GraphPad 8.0.2. Treatment differences were determined using Fisher's LSD (Least Significant Difference Test) at a 5% level of significance.
Result and Discussion
Results revealed that the physicochemical quality of tomato powders was significantly influenced by dehydration temperature and length of time.
Moisture Content
Dehydration temperature significantly (P < 0.001) reduced moisture contents of dehydrated powders of tomato while dehydration time had no significant difference.
As it can be predicted, the maximum moisture content (92.58%) was obtained from fresh tomatoes (Table 1). The exclusive comparison of dehydrated tomatoes showed that the maximum moisture content (12.18%) was obtained at 70°C while the minimum (9.39%) was at 90°C dehydration temperatures. The decrease in moisture content with increasing temperature is accompanied by an increased percentage of TSS since it is the major component of dry matter. This result is supported by Abe-Inge et al. (2018). Food products with high moisture content are suitable for microbial development causing product spoilage. Therefore, such food products would have a shorter shelf life (Lewicki, 1998). Abdullahi et al. (2016) reported a reduced microbial growth on dehydrated tomatoes with lower moisture content. The lower moisture content of the tomato powder might reduce the probability of microbial development and potentially extends shelf life.
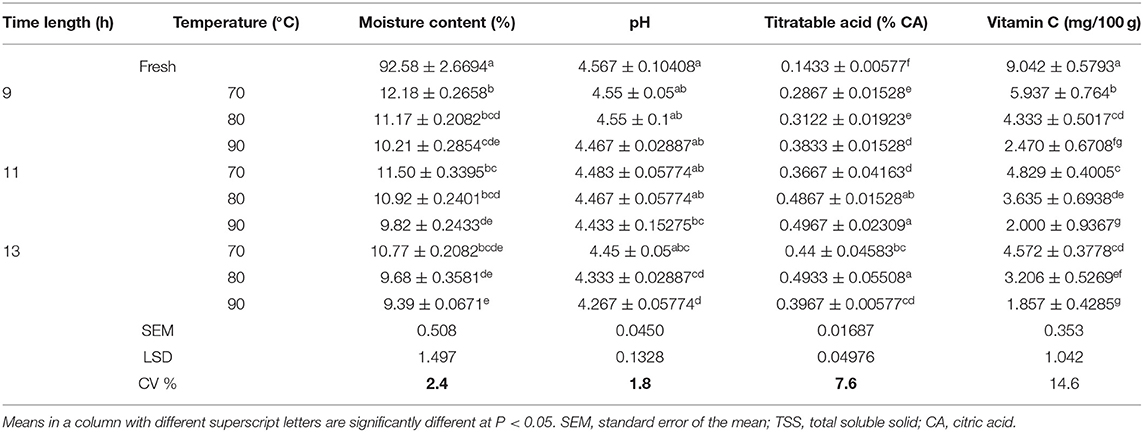
Table 1. Effects of dehydration temperature and time length on moisture, pH, TA and vitamin C contents.
pH and Titratable Acidity (TA)
Both pH and TA were significantly (P < 0.01 and P < 0.001 respectively) influenced by dehydration temperature and time length. The maximum pH value (4.567) was recorded from fresh tomato fruits whereas the minimum (4.333) was obtained at 90°C with 13 h dehydration temperature and time length respectively. Dehydrated tomato powders showed a reduction in pH values compared with fresh fruits. pH values were decreasing along with the increasing dehydration temperature and time (Table 1). On the contrary, Alfeo et al. (2021) reported no significant difference in pH values among dehydration temperatures of 50, 60, and 70°C. However, a decrease in pH value has been reported by Yusufe et al. (2017) from tomato slices dehydrated at 90°C for 8 h.
Titratable acidity (TA) was also increased with increasing temperature and time length. The maximum TA (0.4967%) was obtained at 90°C dehydration for 11 h (Figure 2). This result was statistically similar with the same temperature but 13 h of dehydration time. Previous studies (Khazaei et al., 2008) also reported an increase in TA with increasing dehydration temperature. The increase in TA with dehydration temperature and time might be due that organic acids in dehydrated tomatoes became more concentrated. The minimum TA (0.0433%) was observed from fresh tomatoes followed by the lowest dehydration temperature of 70°C and 9 h dehydration time (0.2867%). When dehydration time is extended from 11 to 13 h at a constant temperature of 90°C, there was about a 20.13% reduction in TA content (Table 2). This might be due to acetic acid degradation by higher temperatures. This result is consistent with several studies (Khazaei et al., 2008; Aderibigbe et al., 2018) that reported degradation of citric acid at higher temperatures with extended dehydration time.
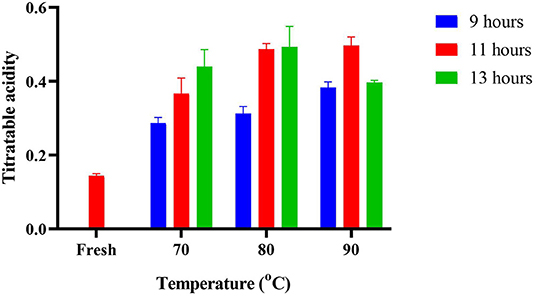
Figure 2. Effect of dehydration temperature and time length on TA of tomato powder (mean + standard deviation).
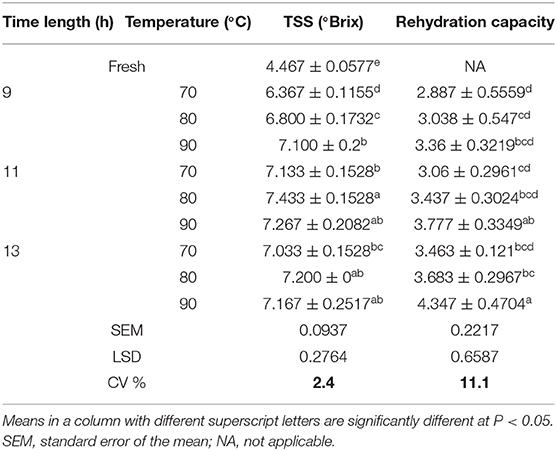
Table 2. Effects of dehydration temperature and time length on TSS and rehydration ratio of dehydrated tomato powder.
pH and titratable acidity (TA) contents are associated with the amount of citric acid. Citric acid is the most important acid present in tomatoes. A high value of citric acid is associated with a satisfactory acidic flavor (Yusufe et al., 2017). It has been reported that tomato fruits with below 4.5 pH values are desirable to cease the proliferation of microorganisms after industrial processing (Qiu et al., 2017). TA and pH are interrelated in terms of acidity but have different impacts on food quality. While TA influences more on the flavor and taste of products, pH provides a degree of strength of acidity (Ma et al., 2012; Aderibigbe et al., 2018). Lower pH provides more inhibition of microbial growth (Cases, 2021). So, acidity contributes to both food taste and food safety.
Vitamin C Content
There was a significant (P < 0.001) change in vitamin C content of dehydrated tomato powders compared with fresh tomato fruits (Figure 3; Table 1). Vitamin C content significantly decreased with increasing temperature while dehydration time had no significant influence (Figure 3). The result is consistent with Dewanto et al. (2002) who testified a decreased vitamin C content with a dehydration temperature at 88°C. Many other authors (Ashebir et al., 2009; Arslan and Özcan, 2011; Basri and Aziz, 2012) also reported Vitamin C reduction with increasing temperature. It might probably be due to the thermos-sensitivity of ascorbic acid. Basri and Aziz (2012) justified that the decrease in vitamin C contents with increasing processing temperature is due to Vitamin C being a very heat-labile component. Thus, vitamin C retention is affected by thermal processing. Dewanto et al. (2002) further clarified that loss of vitamin C occurs primarily by chemical degradation involving oxidation of ascorbic acid into dehydroascorbic acid (DHAA); then, the DHAA is hydrolyzed into 2, 3-diketogulonic acid which finally forms nutritionally inactive products.
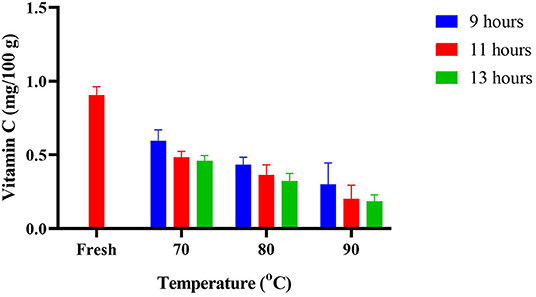
Figure 3. Effect of dehydration temperature and time length on vitamin C content of tomato powder (mean + standard deviation).
Total Soluble Solids (TSS)
Both the sole implementation and interaction of dehydration temperature and duration of time significantly (P < 0.001) influenced the TSS content of dehydrated tomatoes (Table 2). The maximum TSS content (7.433 °Brix) (Figure 4) was recorded when tomatoes are dehydrated at 80°C for 11 h which was statistically at par with 90°C*11 h, 80°C*13 h and 90°C *13 h. Whereas the minimum TSS (4.467°Brix) was observed from fresh tomatoes followed by dehydration at 70°C for 9 h. This result was in line with Alfeo et al. (2021) who observed an increased TSS with increasing temperature (50 to 60 then up to 70°C). Ashebir et al. (2009) also reported increased TSS with increasing temperature from 55, to 65 to 75°C. TSS content is one of the most crucial quality parameters in processed tomatoes. 50–65% of soluble solid contents are sugars, glucose and fructose, and their amount and proportion influence the organoleptic quality of tomatoes (Basri and Aziz, 2012). Thus, increased TSS with temperature and dehydration time might assure that there was a decrease in moisture content. On the other hand, dehydration temperatures beyond 80°C decreased the TSS content even though the change was not significant. Consistently, TSS contents were reportedly decreased after 80°C and extended dehydration time (Khazaei et al., 2008; Yusufe et al., 2017). The higher temperatures along with longer dehydration time lengths may be attributed to degrade sugars (Basri and Aziz, 2012).
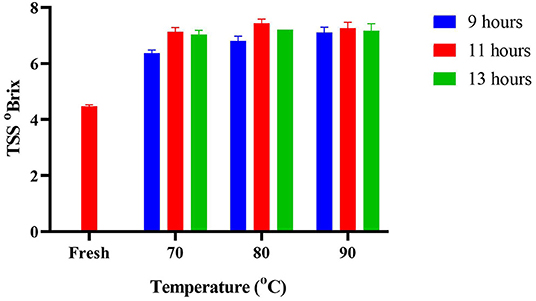
Figure 4. Effect of dehydration temperature and time length on TSS of tomato powder (mean + standard deviation).
Lycopene Content
There was a significant difference (P < 0.001) in the lycopene content of fresh and dehydrated tomatoes as well as within dehydrated tomatoes. Increasing dehydration temperature from 70 to 80°C significantly increased lycopene content (Table 3; Figure 5). On the other hand, dehydration time and its interaction with temperature had no significant difference. It may indicate that lycopene degradation is more sensitive for a higher temperature than time length.
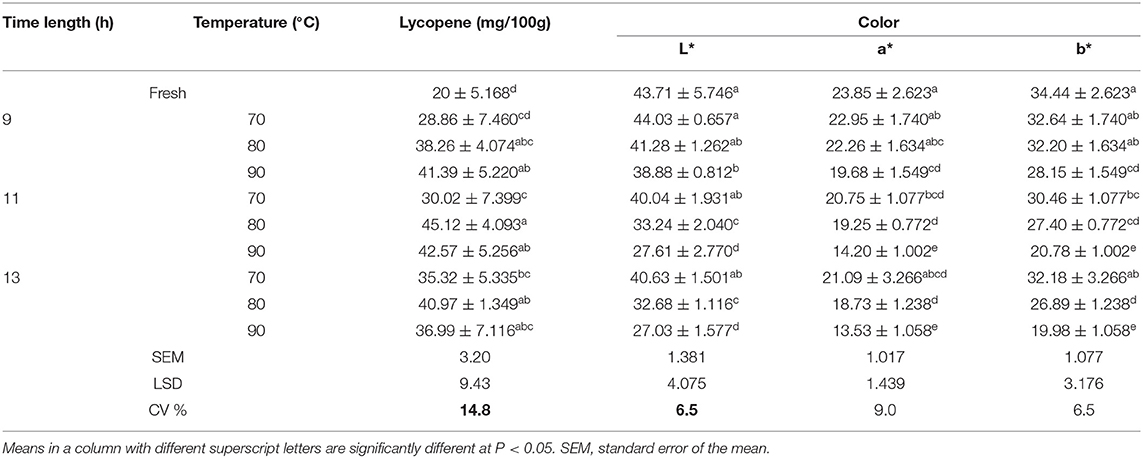
Table 3. Effects of dehydration temperature and time length on lycopene content and color of tomato powder.
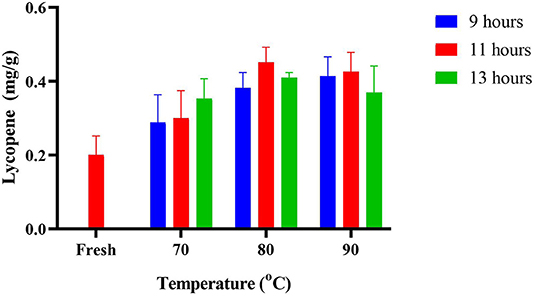
Figure 5. Effect of dehydration temperature and time length on lycopene content of tomato powder (mean + standard deviation).
The apparent increase in lycopene after dehydration is attributed to the loss of water and concentrated lycopene in the dehydrated product. Basri and Aziz (2012) reported similar findings. Maximum lycopene content (0.4512 mg/100 g) was obtained at 80°C dehydration temperature for 11 h (Table 3) whereas the minimum (0.2 mg/100 g) was recorded from fresh tomato fruits. This finding is in line with Dewanto et al. (2002) who observed an increased lycopene content up to 88°C. However, lycopene content was declined at 90°C despite the reduction was not significant (Figure 5). It may also indicate that extreme temperatures damage the lycopene pigments. This assumption is in agreement with Akanbi and Oludemi (2004) who reported lower lycopene contents in processed tomatoes. Amaya (1997) stated that the red color appears when lycopene is formed in the tomato fruit fibers. The attractive red color of the tomato fruit and its products is due to the presence of lycopene (Basri and Aziz, 2012). Therefore, dehydration methods with higher lycopene contents can be considered best for consumer preference.
Color
Product color is another quality parameter that needs to be maintained during tomato processing. There was no significant difference in the color of fresh fruits and tomato powders dehydrated at lower dehydration temperatures for a shorter time length. However, significantly different L*, a* and b* values of tomato powders were observed at interaction effects of higher temperatures and longer dehydration times, i.e., 80°C *11 h, 80°C *13 h, 90°C *11 h, and 13°C *13 h. When the temperature was increased with time length, the L* (brightness), a* (redness) and b* (yellowness) values were decreased (Table 3). In line with this result, Arslan and Özcan (2011) reported reduced L*, a* and b* values of dehydrated tomatoes compared with fresh fruits. Ashebir et al. (2009) and Arslan and Özcan (2011) also reported decreased L* values of tomato slices with increasing dehydration temperature. The reduction in brightness (L* value) of dehydrated products is likely. The color change can be either due to pigment degradation or browning reaction or both during dehydration (Akanbi and Oludemi, 2004; Mwende et al., 2018). The color change is associated with quality reduction. Hence dehydration of tomatoes with higher temperatures may reduce consumers' acceptability.
Rehydration Ratio
Significant (P < 0.01) changes have been observed in the rehydration ratio of dehydrated tomato powders in response to dehydration temperature and time length. The maximum rehydration ratio (4.347) was recorded at the maximum temperature (90°C) with 13 h time length, followed by the same temperature with 11 h time length (Figure 6; Table 2). Rehydration is the process of moisturizing a dehydrated material with abundant water (Lewicki, 1998). It is also considered as a measure of structural injury to the processed product caused by dehydration (Singh and Heldman, 2014). Whereas rehydration represents the ability of the same product for restoring its fresh quality in rehydration solution (Zhang et al., 2017). Consequently, the increased rehydration ratio with increasing temperature and time duration implies that the physical structure of tomato powder was not harshly damaged by increased temperature and time. So that it was able to promote better reabsorption and reconstitution of the product. Ghavidel and Davoodi (2010) reported similar findings from solar and tunnel dehydration methods. Farooq et al. (2020) observed a bit less rehydration ratio ranging from 1.51–1.82 for hot-air-dehydrated tomato powders. The difference is attributed to differences in a variety having different dry matter content. Dehydrated powders with higher dry matter content have lower moisture content so a higher rehydration ratio (Farooq et al., 2020). Increased attraction of water results in increased flow into the tissue and therefore improved rehydration (Ghavidel and Davoodi, 2010).
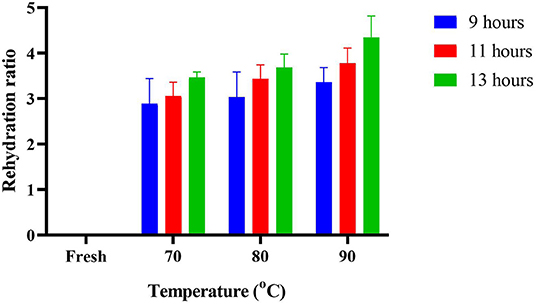
Figure 6. Effect of dehydration temperature and time length on rehydration ratio of tomato powder (mean + standard deviation).
Conclusions
Results revealed that up to 80°C dehydration temperature and 11 h time length significantly increased the major quality parameters of tomato powders. When the dehydration temperature and time length went beyond 80°C and 11 h respectively, the quality of tomato powders declined.
• Maximum TSS (7.433 °Brix), TA (0.4967% CA), lycopene (0.4512 mg/100 g) contents; as low as 4.433 pH and best rehydration ratio (3.437) were obtained at 80°C temperature and 11 h dehydration time.
• It was also possible to reduce the moisture content of tomato powders below 11% with the same temperature and time length while acceptable color L* (brightness/darkness), a* (redness/greenness) and b* (yellowness/blueness) values were maintained.
• However, ascorbic acid (vitamin C) content was significantly reduced by the increased dehydration temperature whereas time length had less influence on vitamin C content.
Generally, the results of this study showed that dehydration of tomatoes with 80°C and 11 h temperature and time length respectively, provides better physicochemical quality of tomato powders. Future research is suggested on consumers' acceptance of dehydrated tomato powders.
Data Availability Statement
The raw data supporting the conclusions of this article will be made available by the authors, without undue reservation.
Author Contributions
AD was the principal investigator of the study, thus he has been involved in data collection, analysis, write-up, organization, and overall coordination. FA, KE, DA, TT, and AA contributed a lot during the research implementation, data collection, and analysis as well as in the entire manuscript preparation. All authors contributed to the article and approved the submitted version.
Funding
This research work was funded by the University of Gondar, Ethiopia.
Conflict of Interest
The authors declare that the research was conducted in the absence of any commercial or financial relationships that could be construed as a potential conflict of interest.
Publisher's Note
All claims expressed in this article are solely those of the authors and do not necessarily represent those of their affiliated organizations, or those of the publisher, the editors and the reviewers. Any product that may be evaluated in this article, or claim that may be made by its manufacturer, is not guaranteed or endorsed by the publisher.
Acknowledgments
The authors would like to thank the University of Gondar for funding this particular study. The authors' deepest gratitude extended to Bahir Dar University, Institute of Technology, Faculty of Chemical and Food Engineering for undertaking the laboratory parts of this experiment. The authors would like to thank the University of Gondar for funding this particular study. The authors' deepest gratitude extended to Bahir Dar University, Institute of Technology, Faculty of Chemical and Food Engineering for undertaking the laboratory parts of this experiment.
Abbreviations
TSS, total soluble solid; TA, Titratable acidity; CA, citric acid.
References
Abdullahi, I. I., Abdullahi, N., Abdu, A. M., and Ibrahim, A. S. (2016). Proximate, mineral and vitamin analysis of fresh and canned tomato. Biosci. Biotechnol. Res. Asia 13, 1163–1169. doi: 10.13005/bbra/2147
Abe-Inge, V., Agbenorhevi, J. K., Kpodo, F. M., and Adzinyo, O. A. (2018). Effect of different drying techniques on quality characteristics of african palmyra palm (Borassus aethiopum) fruit flour. Food Res. 2, 331–339. doi: 10.26656/fr.2017.2(4)0.050
Aderibigbe, O. R., Owolade, O. S., Egbekunle, K. O., Popoola, F. O., and Jiboku, O. O. (2018). Quality attributes of tomato powder as affected by different pre-drying treatments. Int. Food Res. J. 25, 1126–1132. Available online at: http://www.ifrj.upm.edu.my/25%20(03)%202018/(33).pdf
Akanbi, C. T., and Oludemi, F. O. (2004). Effect of processing and packaging on the lycopene content of tomato products. Int. J. Food Prop. 7, 139–152. doi: 10.1081/JFP-120024173
Alfeo, V., Planeta, D., Velotto, S., Palmeri, R., and Todaro, A. (2021). Cherry tomato drying: Sun versus convective oven. Horticulturae 7, 1–12. doi: 10.3390/horticulturae7030040
Ali, M. Y., Sina, A. A. I., Khandker, S. S., Neesa, L., Tanvir, E. M., Kabir, A., et al. (2021). Nutritional composition and bioactive compounds in tomatoes and their impact on human health and disease: a review. Foods 10, 45. doi: 10.3390/foods10010045
Amaya, D. B. R. (1997). “Carotenoids and food preparation : The retention of provitamin a carotenoids in prepared, processed, and stored foods,” in Contract. Available online at: https://pdfs.semanticscholar.org/2774/fe0167e3e8cfd5850c2fbb078c6a230c100c.pdf
Amit, S. K., Uddin, M. M., Rahman, R., Islam, S. M. R., and Khan, M. S. (2017). A review on mechanisms and commercial aspects of food preservation and processing. Agric. Food Secur. 6, 1–23. doi: 10.1186/s40066-017-0130-8
Arslan, D., and Özcan, M. M. (2011). Drying of tomato slices: Changes in drying kinetics, mineral contents, antioxidant activity and color parameters. CYTA - J. Food 9, 229–236. doi: 10.1080/19476337.2010.522734
Ashebir, D., Jezik, K., Weingartemann, H., and Gretzmacher, R. (2009). Change in color and other fruit quality characteristics of tomato cultivars after hot-air drying at low final-moisture content. Int. J. Food Sci. Nutr. 60, 308–315. doi: 10.1080/09637480903114128
Basri, N. F., and Aziz, F. A. (2012). Asian Journal of Food and Agro-Industry. Characterisation Stud. cocoa butter β(V) polymorphs from Sabah, Malaysia 5, 71–78.
Cases, M. (2021). The Introduction to Microbiology - 8.3: The effects of pH and temperature on microbial growth. Manchester Community Coll. 1, 317–323.
Correia, A. F. K., Loro, A. C., Zanatta, S., Spoto, M. H. F., and Vieira, T. M. F. S. (2015). Effect of temperature, time, and material thickness on the dehydration process of tomato. Int. J. Food Sci. 2015, 724. doi: 10.1155/2015/970724
Costa, M., and Huevelink, E. (2007). Today's worldwide tomato production. Int. Suppliers Guid. 12, 2006–2008.
Dewanto, V., Xianzhong, W., Adom, K. K., and Liu, R. H. (2002). Thermal processing enhances the nutritional value of tomatoes by increasing total antioxidant activity. J. Agric. Food Chem. 50, 3010–3014. doi: 10.1021/jf0115589
FAO and World Bank (2010). FAO / World Bank workshop on reducing post-harvest losses in grain supply chains in Africa. Lessons Learn. Pract. Guidel. 10, 1–121. Available online at: https://www.fao.org/3/au092e/au092e.pdf
Farooq, S. A, Rather, S., Gull, A., Ahmad Ganai, S., Masoodi, F. A., et al. (2020). Physicochemical and nutraceutical properties of tomato powder as affected by pretreatments, drying methods, and storage period. Int. J. Food Prop. 23, 797–808. doi: 10.1080/10942912.2020.1758716
Gabriel, D. (2021). Practices and constraints of tomato production. African J. food, Agric. Nutr. Dev. 21, 17560–17580. doi: 10.18697/ajfand.97.19905
Ghavidel, R. A., and Davoodi, M. G. (2010). Studies on physiochemical properties of tomato powder as affected by different dehydration methods and pretreatments. World Acad. Sci. Eng. Technol. 70, 596–605. Available online at: https://citeseerx.ist.psu.edu/viewdoc/download?doi=10.1.1.190.4494&rep=rep1&type=pdf
Ibitoye, A. A. (2005). Laboratory Manual of Basic Methods in Analytical Chemistry, 1st Edn. concept + IT and educational consult, Akure
Idah, P. A., Musa, J. J., and Olaleye, S. T. (2010). Effect of temperature and drying time on some nutritional quality parameters of dried tomatoes. AU J. T. 14, 25–32. Available online at: https://www.thaiscience.info/journals/Article/AUJT/10817632.pdf
Jayathunge, K., Kapilarathne, R., Thilakarathne, B., Palipane, K., and Prasanna, P. (2012). Development of a methodology for production of dehydrated tomato powder and study the acceptability of the product. J. Agric. Technol. 8, 765–773. Available online at: http://ijat-aatsea.com/pdf/v8_n2_12_March/31_IJAT%202012_8_2__K.G.L.R.pdf
Karla, A. S. M., and Flávio, L. S. (2019). The tomato paste quality attributes along the industrial processing chain. African J. Food Sci. 13, 215–224. doi: 10.5897/AJFS2019.1825
Kasso, M., and Bekele, A. (2016). Post-harvest loss and quality deterioration of horticultural crops in Dire Dawa Region, Ethiopia.
Khazaei, J., Chegini, G. R., and Bakhshiani, M. (2008). A novel alternative method for modeling the effects of air temperature and slice thickness on quality and drying kinetics of tomato slices: Superposition technique. Dry. Technol. 26, 759–775. doi: 10.1080/07373930802046427
Kozlova, I., Esaulova, L., and Garkusha, S. (2020). Mechanical harvesting and processing of tomato varieties. IOP Conf. Ser. Mater. Sci. Eng. 1001. doi: 10.1088/1757-899X/1001/1/012126
Lewicki, P. P. (1998). Effect of pre-drying treatment, drying and rehydration on plant tissue properties: a review. Int. J. Food Prop. 1, 1–22. doi: 10.1080/10942919809524561
Ma, H., Wang, Z., Amissah, A., and Owusu, J. (2012). Effect of drying methods on physicochemical properties of pretreated tomato (lycopersicon esculentum mill.) slices. Hrvat. časopis za prehrambenu Tehnol. Biotehnol. i Nutr. 7, 106–111.
MANR (2016). Plant variety release. protection and seed quality control directorate. Crop Var. Regist. 16, 330.
Minten, B., Tamru, S., and Reardon, T. (2019). Post-harvest losses in rural-urban value chains : Evidence from Ethiopia. doi: 10.2499/p15738coll2.133411
Mohammed, M. I., and Malami, D. I. (2013). Effect of heat treatment on the lycopene content of tomato puree. 4, 18–21.
Mujumdar, A. S., and Law, C. L. (2010). Drying Technology: Trends and applications in postharvest processing. Food Bioprocess Technol. 3, 843–852. doi: 10.1007/s11947-010-0353-1
Mwende, R., Owino, W., and Imathiu, S. (2018). Effects of pretreatment during drying on the antioxidant properties and color of selected tomato varieties. Food Sci. Nutr. 6, 503–511. doi: 10.1002/fsn3.581
Nurkhoeriyati, T., Kulig, B., Sturm, B., and Hensel, O. (2021). The effect of pre-drying treatment and drying conditions on quality and energy consumption of hot air-dried celeriac slices: Optimisation. Foods 10, 1758. doi: 10.3390/foods10081758
Qiu, J., Vuist, J.-E., Boom, R., and Schutyser, M. (2017). Change in Taste of Tomato During Thermal Processing and Drying: Quantitative Analyses On Ascorbic Acid and Pyroglutamic Acid.
Rayaguru, K., and Routray, W. (2011). Microwave drying kinetics and quality characteristics of aromatic Pandanus amaryllifolius leaves. Int. Food Res. J. 18, 1035–1042. Available online at: http://www.ifrj.upm.edu.my/18%20(03)%202011/(26)IFRJ-2010-282.pdf
Saran, S., Jayanth, T. A. S., Anand, S., Pandey, V., and Sumathi, N. (2017). Tomato processing industry management. Int. J. Latest Technol. Eng. Manag. Appl. Sci. VI, 124–128.
Sirivastava, S., and Kulshreshtha, K. (2013). Nutritional content and significance of tomato powder. Ann. Arid Zone 52, 121–124.
Sora, S. A. (2018). Review on productivity of released tomato (Solanum lycopersicum Mill.) Varieties in Different Parts of Ethiopia. J. Hortic. Sci. For. 1, 5. Available online at: http://article.scholarena.com/Review-on-Productivity-of-Released-Tomato-Solanum-Lycopersicum-Mill-Varieties-in-Different-Parts-of-Ethiopia.pdf
Subramanian, R. (2016). India processing tomato segment current status, trends and opportunities for engagement. India Green Innov. Cent. Agri-Food Sect. 16, 28. Available online at: https://avrdc.org/download/publications/technical-reports/reports/GIZ_India-Processed-Tomato-Study_16Sept2016.pdf
Surendar, J., Shere, D. M, Gadhe, K. S., and Sawate, A. R. (2018). Drying characteristics on physicochemical characteristics of tomato powder. Int. J. Chem. Stud. 6, 237–239.
Taylor, P., Cui, Z., Li, C., Song, C., and Song, Y. (2008). Combined Microwave-vacuum and Freeze Drying of Carrot and Apple Chips.
Tilahun, L., Hofacker, W., Esper, A., and Hensel, O. (2021). Physicochemical quality of twin layer solar tunnel dried tomato slices. Heliyon 7, e07127. doi: 10.1016/j.heliyon.2021.e07127
Yusufe, M., Mohammed, A., and Satheesh, N. (2017). Effect of duration and drying temperature on characteristics of dried tomato (Lycopersicon esculentum L.) Cochoro variety. Acta Univ. Cibiniensis. Ser. E Food Technol. 21, 41–50. doi: 10.1515/aucft-2017-0005
Keywords: dehydration temperature, lycopene, physicochemical quality, time length, tomato powder
Citation: Degwale A, Asrat F, Eniyew K, Asres D, Tesfa T and Ayalew A (2022) Influence of Dehydration Temperature and Time on Physicochemical Properties of Tomato (Solanum lycopersicum L.) Powder. Front. Sustain. Food Syst. 6:839385. doi: 10.3389/fsufs.2022.839385
Received: 19 December 2021; Accepted: 24 January 2022;
Published: 25 March 2022.
Edited by:
Pankaj B. Pathare, Sultan Qaboos University, OmanReviewed by:
Farah Taip, Putra Malaysia University, MalaysiaHany El-Mesery, Jiangsu University, China
Copyright © 2022 Degwale, Asrat, Eniyew, Asres, Tesfa and Ayalew. This is an open-access article distributed under the terms of the Creative Commons Attribution License (CC BY). The use, distribution or reproduction in other forums is permitted, provided the original author(s) and the copyright owner(s) are credited and that the original publication in this journal is cited, in accordance with accepted academic practice. No use, distribution or reproduction is permitted which does not comply with these terms.
*Correspondence: Alemu Degwale, alemudegwalle@gmail.com; orcid.org/0000-0002-3494-1285