- 1International Crops Research Institute for the Semi-Arid Tropics, Patancheru, India
- 2Swedish University of Agricultural Sciences (SLU), Uppsala, Sweden
- 3ICAR-Central Agroforestry Research Institute, Jhansi, India
This article presents the evidence on how the traditional rainwater management system (haveli system) has contributed toward rehabilitating degraded landscapes and changing them into a productive form in Bundelkhand region of Central India. The haveli system was the lifeline of the region for water security for the last 300 years. Farmers (~1–5%) situated at the upstream of the landscape were harvesting surface runoff in their fields during monsoon by constructing earthen embankments along with provision to drain out water after receding of the monsoon. Farmers traditionally cultivated only during the post-monsoon period, using residual soil moisture along with supplemental irrigation from shallow dug wells. However, this system became defunct due to apathy and poor maintenance. The traditional design of the havelis were also often malfunctioning due to new rainfall patterns and storm events. Farmers are facing new need for haveli rejuvenation and the traditional design and knowledge calls for new innovations, particularly from research and external expertise. In this context, ICRISAT and consortium partners have introduced an innovative approach for haveli rejuvenation by constructing masonry core wall along with outlet at a suitable location. Totally 40 haveli structures were constructed between 2010 and 2021 across seven districts of Bundelkhand region. One of the pilot sites (i.e., Parasai-Sindh) was intensively monitored in order to capture the landscape hydrology, change in land use, cropping intensity and crop productivity, between 2011 and 2017. Out of 750 mm rainfall received during July and September, generated surface runoff is about 135 mm (18% of rainfall) on average. However, rainfall below 450 mm (dry years) rarely generates surplus water as most of the rainfall received in such years are absorbed within the vadose zone, whereas, wet years with over 900 mm rainfall, generate runoff of about 250–300 mm (~30–35%). Rejuvenation of the haveli system created an opportunity to harvest surface runoff within farmers' fields which helped to improve groundwater levels in shallow dug wells (additionally by 2–5 m hydraulic head) which remained available during the following years. This has increased cropping intensity—by converting about 20% of permanent fallow lands into productive agriculture lands—and ensured irrigation availability especially during the critical crop growth stage. This enhanced land and water use efficiency of the system and increased household net income by two to three folds as compared to the baseline status. This article further establishes the link between landscape rejuvenation through haveli system, groundwater resource availability, production system and household income in the fragile ecosystem of Central India. The results are helpful for various stakeholders so that they can take informed decisions on sustainable natural resource management.
Introduction
Drylands cover ~40% of the global land area and support two billion people and 90% of them live in developing countries (UNEMG, 2011). Drylands suffer from water scarcity and land degradation which are major causes for poor agriculture and livestock productivity (Robinson et al., 2015; Wani et al., 2016; Schlaepfer et al., 2017; Singh et al., 2018; Singh C. et al., 2019). Land degradation in drylands costs an estimated 4–8% of gross domestic product in developing countries each year, and these ecologies also coincide with poverty, malnutrition, and poor economic development (Cowie et al., 2018; Barrio et al., 2021; Prǎvǎlie, 2021). Agriculture and allied sectors are the major source of livelihood for more than 70% of the population residing in these areas (Anantha et al., 2021a). Climate change and unsustainable resource use has driven the degradation further, thus reducing the provisioning ability of the system as well as weakening regulatory and supporting ecosystem services (Gordon et al., 2010; Rockström et al., 2014; DeClerck et al., 2016; Queiroz et al., 2021).
Drylands of the semi-arid tropics are characterized by limited rainy days (~20–60 days) with large variability in terms of its amount and distribution (Rao et al., 2013; Mukherjee, 2021). These regions are also marked by high mean temperatures and often a prolonged dry season leading to non-productive evaporation losses (Rao et al., 2013). In addition, due to poor moisture retention ability (exposed soil surface with deforestation or overgrazing and low organic carbon), rainfall received in these landscapes frequently generate flash floods, but insubstantially contribute to groundwater recharge which is the important source of freshwater for domestic and agricultural use especially in non-monsoonal periods (De Wit and Stankiewicz, 2006; Singh et al., 2014, 2021; Garg et al., 2020b). Given poor groundwater availability, agriculture suffers from increased risk of crop failure and poor productivity (Garg et al., 2012). Crop yield in this ecosystem oscillates between 0.5 t/ha and 1.5 ton/ha which is also referred to as “one-ton agriculture.” Due to limited livelihood opportunities deriving exclusively from agriculture, rural communities are often compelled to migrate to nearby urban centers in search of food and other resources (Villholth, 2013; Singh et al., 2014; Padmaja et al., 2020).
As freshwater availability is the key factor for sustainable development, farmers use their traditional wisdom to develop a range of innovative methods to harvest rainwater in a decentralized manner across the world (Reddy et al., 2018). These interventions range from field scale water harvesting structures, such as farm ponds of 300–1,000 cubic meter storage capacity to the community scale reservoirs with 0.01–1.0 million cubic meter storage capacity, which are helpful in meeting their freshwater needs for domestic, livestock and agricultural use. Water harvested from these structures are either used directly or facilitate recharging of groundwater sources depending on various topographical and biophysical factors (e.g., catchment area, soil type, geological formation, etc.). Water harvesting structures were an integral part of dryland farming and a lifeline for most of the dryland ecosystems and contributed significantly in terms of flood control, drought mitigation and in building system level resilience (Golani and Ozha, 2006; Saha et al., 2007; Singh et al., 2014, 2021; Garg et al., 2020a,b, 2021, 2022b).
In India, agriculture is the main source of livelihood for about 60% of the population. About 68% of the total cultivable area comes under the category of drylands (Vijayan, 2016). A range of rainwater harvesting systems were designed, innovated and practiced across the country since ancient times. In these methods, generated surface runoff is harvested through construction of small-scale water structures. Surface water tanks in southern India; haveli system in central India; khadins and johads in western India, and ruza in eastern India are some of the examples of traditional practices of decentralized water harvesting systems (Grewal et al., 1989; Verma and Sarma, 1990; Agarwal and Narain, 2003; Pandey et al., 2003; Golani and Ozha, 2006; Saha et al., 2007; Sahu et al., 2015; Garg et al., 2020b, 2021). These systems have contributed significantly not only for generating various provisioning services but also in supporting the landscape to maintain biodiversity and continued to be productive. The haveli system used to secure surface and groundwater resources as well as maintained the green cover and a range of flora and fauna. Further, deposition of silt at haveli bed over a period helped to maintain productivity level. Due to apathy and lack of maintenance, a number of these structures became obsolete in the last 3–4 decades and focus shifted toward groundwater sources with innovation in pumping technologies and subsidized power supply (Garg et al., 2020b). In the 1960s nearly one million borewells were pumping up 25 Billion Cubic Meters (BCM) which has increased to 20 million borehole wells with total withdrawal of 250 BCM in recent years (Shah, 2009; Gleeson et al., 2016). India is the largest user of groundwater in the world, which is over a quarter of the global total. Out of 6,881 assessment units for groundwater use status in India, 972 units are under semi-critical category, 313 units critical, and 1,186 are over-exploited, which indicates that withdrawal in these blocks is higher than its replenishment (Government of India, 2019). Therefore, groundwater levels have reached below 200–300 m resulting in frequent well failure, loss of capital investment, higher energy consumption and cost of cultivation (Anantha, 2013; Garg et al., 2020a; Sidhu et al., 2020; Singh et al., 2021).
With this background, this article presents a case study from Central India on rehabilitation of the traditional rainwater management system called Haveli cultivation which has emerged as a promising solution for addressing the water scarcity issue in this region. The overarching goal of the article is to understand the impact of traditional rainwater management system on: (i) groundwater recharge; (ii) opportunities for crop intensification; (iii) agricultural productivity; and (iv) farmers' income.
Materials and Methods
Bundelkhand Region of Central India
Bundelkhand region of Central India belongs to Ken and Betwa catchment of Yamuna sub-basin of Ganga river basin (Figure 1). The region occupies a geographical area of 6.9 Million hectares, shared between Uttar Pradesh and Madhya Pradesh states with a total population of 18 million. Agriculture and allied sectors are the major source of livelihood for more than 80% of the rural population in the region. However, agricultural and livestock productivity in the region is much lower than the national average and more than 90% of the farming families live below the poverty threshold of US$ 1.25/day/person (Anantha et al., 2022b). The region is the hotspot of water scarcity, land degradation, poverty and malnutrition (Singh, 2020).
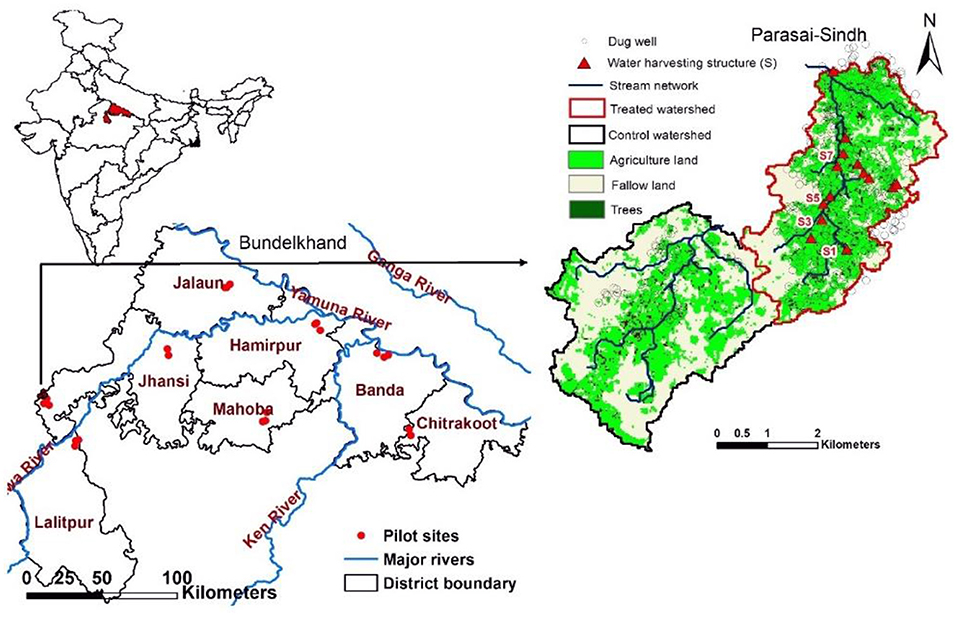
Figure 1. Location map (on left) shows study area indicating major rivers, seven districts of Bundelkhand region along with pilot villages; enlarged map (on right) shows Parasai-Sindh watershed of Jhansi district, which was intensively monitored along with the control watershed.
The agriculture in the region is largely dependent on rainfed farming along with limited access to supplemental irrigation. Shallow dug wells are the major source of groundwater; however, there are some blocks where borehole wells are also used (e.g., Jalaun and Banda), but those are largely situated in the delta region of the Ken and Betwa catchment area (Figure 1). This shallow dug well (5–15 m depth) system recharges during the monsoon and that water is being utilized during dry spells in monsoon and post-monsoonal period for domestic and agricultural uses (Thomas et al., 2015). The geology of the region is largely dominated by hard rock (granite) and a top layer of up to 20–50 m is the only weathered zone (unconfined aquifers) which facilitate the storage of groundwater (Singh P. K. et al., 2019). In such a situation, shallow dug wells are largely functional and scope for drilling deep borehole wells are limited (Thomas et al., 2015). Due to poor specific yield (i.e., 1–2%), groundwater depletes soon after the rains recede which poses a high risk of crop failure if supplemental irrigation is not made available at the critical growth stage. Farmers cultivate low-water requiring crops such as millets, sorghum, pulses (blackgram, green gram, pigeonpea) and oilseed crops (groundnut and sesame) during the monsoon season (called kharif season), whereas mustard, chickpea, lentils, barley and wheat are cultivated during the post-monsoon period (called rabi season). Crop selection is largely based on rainfall, water availability in wells and soil types.
Haveli cultivation was one of the traditional rainwater harvesting systems in Bundelkhand region which evolved over 300–500 years ago (Sahu et al., 2015). Farmers used to construct 100–300 m length of earthen embankment (4–10 m wide and 1–3 m height) across the land slope depending on catchment (Meter et al., 2014, 2016; Sahu et al., 2015; Garg et al., 2020b; Singh et al., 2021). This system was equipped with spillway facility for releasing excess runoff during monsoon period along with an option to drain out all the water through a conduit. Traditionally, communities used to maintain these structures by repairing earthen embankment, water outlets and schedule water release (Meter et al., 2016). Normally, the catchment of a haveli system ranges from 10 to 200 ha. Runoff generated from the catchment is harvested in these structures during the monsoon which facilitates the recharge of shallow dug wells and borehole wells across the village. Once the monsoon recedes (generally by end of October), the impounded water is also drained out and the haveli bed is prepared for cultivating rabi crops using residual soil moisture (Sahu et al., 2015; Garg et al., 2020a). Drained water from the haveli is also used in pre-sowing irrigation by downstream farmers. The haveli system acts as a reservoir (shallow water bodies with 1–3 m depth) during the monsoon season and gets converted into agricultural fields during the post-monsoon season (Figure 2). Therefore, farmers do not lose their land by practicing haveli cultivation. Due to decomposed organic matter and excess humus, the productivity of crops fed by the haveli in the rabi season is 15–25% higher as compared to nearby normal fields (Sahu et al., 2015). In addition, the irrigation requirement is reduced by 50% which thus reduces the cost of cultivation significantly and fetches an enhanced net income by more than 60–70%. These factors motivate farmers to practice haveli cultivation despite their fields getting submerged during the monsoon period. However, haveli cultivation that once used to meet the freshwater demand in the region and supported various ecosystem services, gradually became defunct due to the following reasons: (i) Most of the earthen embankments caved in, particularly during heavy intensity rainfall events largely due to burrowing and insufficient structure strength; (ii) Insufficient outlets provided for safe disposal of excess runoff; (iii) disintegration of water user groups and rural institutions (Reddy et al., 2018; Garg et al., 2020b).
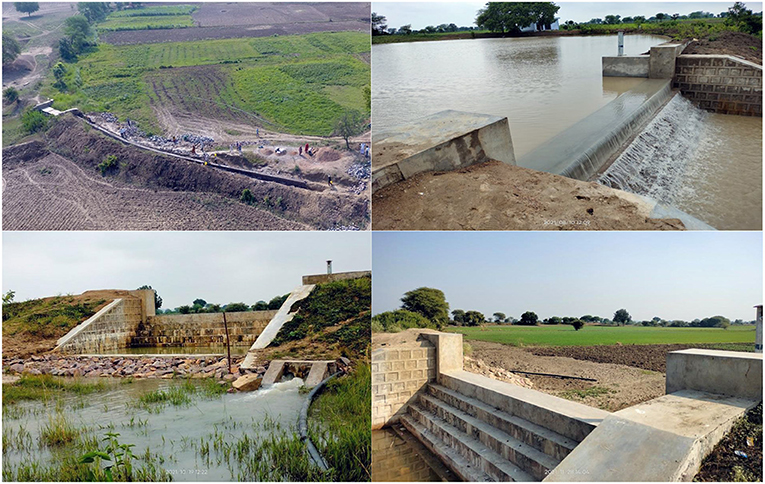
Figure 2. Construction of masonry core wall and spillway (top left); Harvested rainwater in haveli structure during monsoon period (top right); draining out water from haveli before post-monsoon crop (bottom left); wheat crop cultivated on haveli bed during post-monsoon season (bottom right).
Pilot Sites and Interventions Undertaken
To address the above mentioned challenges, the International Crops Research Institute for the Semi-Arid Tropics (ICRISAT) and its consortium partners have developed model sites of learning in seven districts of Uttar Pradesh's Bundelkhand region in a phased manner with support from the Government of Uttar Pradesh (Figure 1). These pilots are largely located at uppermost toposequence of the landscape in respective districts, especially in those areas that were suffering due to poor groundwater recharge and about 20% of the cultivable area is permanently fallow. In addition, about 30–50% of cultivable land was also left fallow in the rabi season due to water scarcity.
In this initiative, the landscape was divided into several clusters—ranging from 300 to 500 ha of geographical area—for implementing a range of climate resilient technologies. An array of landscape-based and field scale interventions were undertaken by following the ridge to valley approach. The major focus was put on rejuvenation of the traditional rainwater management system, called haveli cultivation. In this approach, upland fields were divided into 0.20–0.25 ha plots by earthen field bunds along with construction of masonry field drainage structures such that land degradation could be minimized, while surface runoff generated from 10 to 200 ha catchment is harvested by construction of haveli structures. Totally 40 haveli structures were constructed or rejuvenated between 2011 and 2021 with total storage capacity of 3 million cubic meter. To bring stability to the haveli structure, an innovative core wall concept was introduced. In place of earthen embankments, a masonry core wall with appropriate height (along with required foundation) was constructed so that targeted haveli fields could be brought into submergence at its full capacity (Figure 2). After construction of the core wall, it was covered with soil to attain adequate cross section to get the required structural strength and to avoid thermal hysteresis for longer life. In addition, a masonry outlet was constructed at a suitable place (at elevated points) by following appropriate engineering designs.
Data Collection and Monitoring
To understand the impact of haveli rejuvenation along with rainwater harvesting structures, paired watersheds (treated vs. control) located at Jhansi district were intensively monitored. Below is a detailed description of the instrumentation.
Hydrological Monitoring
Rainfall and Surface Runoff
Automatic-cum-manual rainfall gauges were installed in project watersheds (treated and control) for monitoring rainfall on a daily time scale. To analyze the impact of various rainwater harvesting (RWH) structures, particularly the haveli system, hydrological monitoring system was established in selected structures (Figure 1: S1, S3, S5, S7). Outlet (i.e., spillway structure) of the haveli structure (i.e., S1) was constructed as a rectangular weir and a stilling well was installed at upstream of the outlet (Garg et al., 2020b). DIVER (i.e., pressure transducers; Model DI801 TD, having capacity of recording 10 m pressure head) was placed in the stilling well to record the hydraulic head at an interval of every 15 min. The depth of water layer passing over the weir across different time periods was recorded (spillway discharge) using Equation (1).
In addition, the amount of water received (inflow) at check dam was also estimated using DIVER recorded data. A stage-volume relationship was established from topographical survey which indicates the amount of water available at any given time in the haveli structure. Inflow is estimated using Equation (2).
Moreover, to understand the climate change aspects in the study area (all seven districts), daily rainfall data from 23 rain gauge stations were retrieved from the India Meteorological Department (IMD), Pune, India, from 1950 to 2017. The data were analyzed to understand the change pattern in rainfall (amount and distribution) across different districts. The data were also divided into two periods, i.e., 1950–1983 and 1984–2017, and compared in terms of number of rainy days with different intensities, and total amount received.
Groundwater Recharge
To understand the dynamics of groundwater recharge, water levels in dug wells were monitored on a monthly time scale. Water level indicators were used to measure the water table (depth from the soil surface) in dug wells manually. Totally 538 wells were monitored in treated (Parasai-Sindh) and nearby control watersheds between 2012 and 2017, on a monthly time scale. The water table fluctuation method is a well-accepted method for estimating groundwater recharge in hard rock regions (Sharda et al., 2006; Dewandel et al., 2010; Glendenning and Vervoort, 2010; Pavelic et al., 2012; Garg and Wani, 2013; Tilahun et al., 2020). Groundwater recharge was estimated using difference in water table technique as described in Equation (3):
Land Use and NDVI Mapping
Normalized Difference Vegetation Index (NDVI) was used as an indicator of crop intensification especially in the rabi season. NDVI was mapped for the month of February before (2011) and after (2014 and 2015) watershed interventions. In addition, cropped area under different crops was also captured through primary survey for all farmers in the watershed.
Crop Yield, Production and Income
Crop yields were estimated through crop cutting studies (Tek et al., 2016). In all 300 crop cutting studies were undertaken over the 4 years (2012–2016) on different kharif and rabi crops. Further, cost of cultivation before and after project interventions were estimated through farmers' interviews for different kharif and rabi crops, and net income was estimated using Equation (4).
Where, NIa= Net income (US$/household/year); Yi is crop yield (t ha−1) for plot i; Ai is area of the plot i (ha); Mi is market price; Ci is cost of cultivation of plot i (US$/ha); n = number of plots farmers owning.
In addition, income from livestock was considered, but only from milk production.
Results
Rainfall Characterization
Bundelkhand region receives about 85% of its annual rainfall from July to September. Long-term data (between 1950 and 2017) of 23 rainfall stations covering all seven districts of Uttar Pradesh's Bundelkhand region showed declining rainfall status. Figure 3 shows spatial pattern in rainfall variability between 1950–1983 and 1984–2017. It revealed that districts such as Jalaun, Hamirpur, Mahoba are affected more in terms of a reduction in rainfall amount in recent decades (1984–2017) as compared to previous decades (1950–1983). The average annual rainfall of the study region was 867 mm in 1950–1983, which declined to 683 mm in 1984–2017. Such a declining trend is due to reduction in number of rainfall events—from 47 in 1950–1983 to 39 in 1984–2017—in different categories (medium: 10–30 mm, high: 30–50 mm and very high: >50 mm).
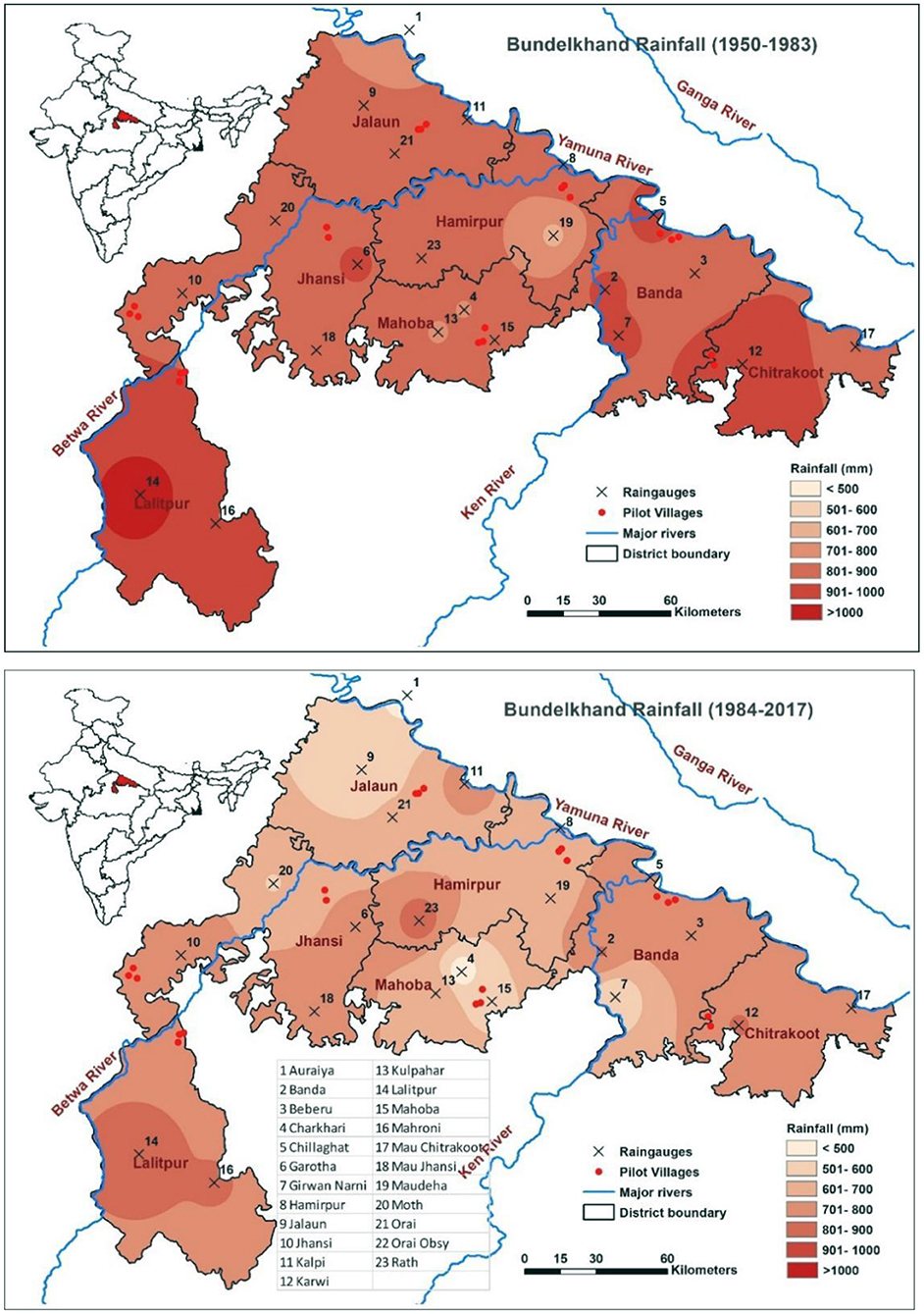
Figure 3. Spatial and temporal variability of annual rainfall in Uttar Pradesh's Bundelkhand region between 1950 and 2017.
Figure 4 further compares rainfall status across 23 gauging stations along with its variability (through box-plot) at the respective time period. Data clearly indicates that rainfall from most of the stations has declined. There were very few incidences when the rainfall was <400 mm during 1950–1983, whereas there was more likelihood of rainfall even <400 mm in more than 30% stations during 1950–1983. In addition, average annual rainfall of these monitoring stations were in the range of 700 and 1,100 mm during 1950–1983, which has reduced to 500 and 900 mm range in 1984–2017.
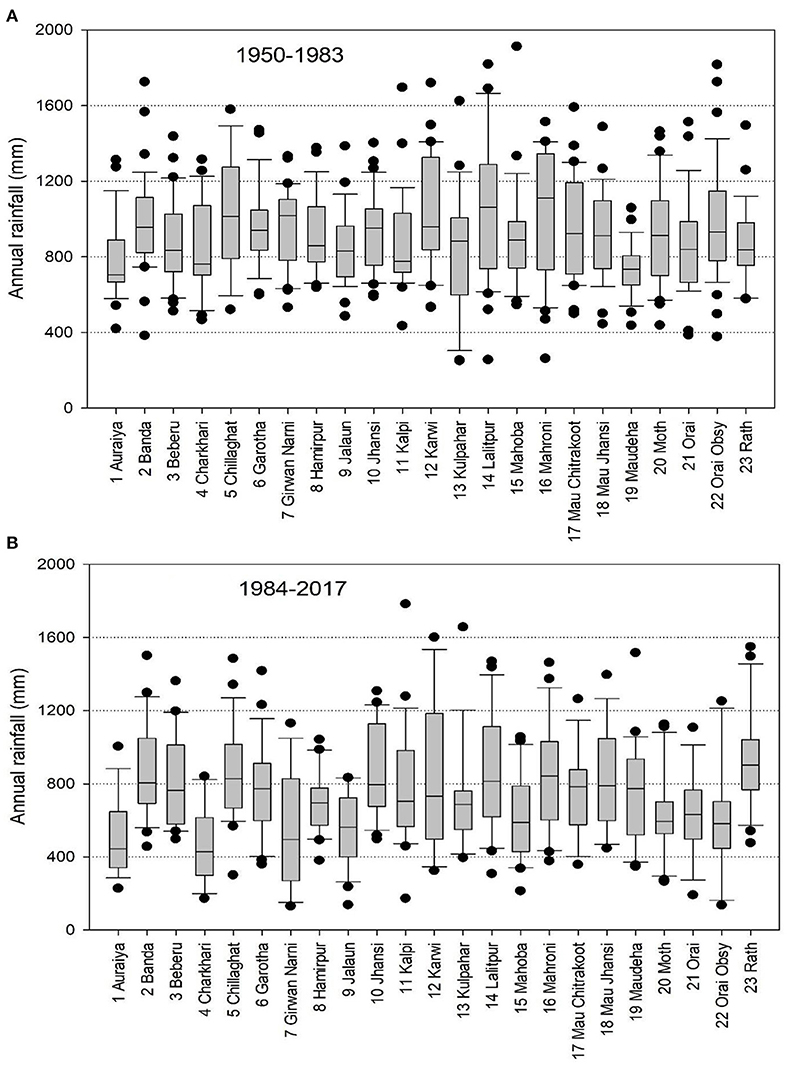
Figure 4. Comparison of annual average rainfall of 23 stations in Bundelkhand region of Uttar Pradesh between (A) 1950–1983 and (B) 1984–2017.
Impact of Rainwater Harvesting Interventions
Water Resources Availability
Surface Runoff
Table 1 shows major water balance components of treated and control watershed in Jhansi district. This Table describes the water balance of 2011–2017; 2011–12 is considered to be the pre-watershed period; whereas 2013 onwards can be considered as treated condition. The haveli structure constructed in 2012 had 70,000 m3 storage capacity which was 70% of total water harvesting target. Rainfall data indicates that during the project period, 2013 was one of the wettest years which received 1,276 mm rainfall in total, 2016–17 was the normal year with 768 mm rainfall, and the remaining years (2014–15, 2015–16, and 2017–18) were dry years. This indicates that out of 5 years one was wet, one normal, and three were dry years. To understand surface hydrology of the landscape runoff monitor at S1 (Haveli) and S5 (check dam) in treated watershed see Table 1 and Figure 1. The catchment area of S1 and S5 was 80 and 567 ha, respectively, indicating the upstream and downstream effects of treated landscape. It is to be noted that runoff from control watershed could not be measured as the instrument (DIVER) was lost. Runoff measured at S1 was 356 mm in 2013-14, that is 29% of total rainfall received, while runoff received during the normal year was found to be 210 mm (27% of rainfall), and runoff during the dry years ranged between 0 and 102 mm which is 0–16% of total rainfall received. On the other hand, runoff measured at downstream site (i.e., S5) varied significantly except in wet years. Runoff at S5 during 2013–14 was 28% (close to S1, i.e., 351 mm). However, runoff during 2016–17 and 2017–18 was recorded as 124 mm (16% of rainfall) and 15 mm (2% of rainfall), respectively. On average, rainfall received during the study period (2013–2017) was 720 mm, of which 136 mm (19% of rainfall) runoff was recorded at S1, and 99 mm (14% of rainfall) at S5.
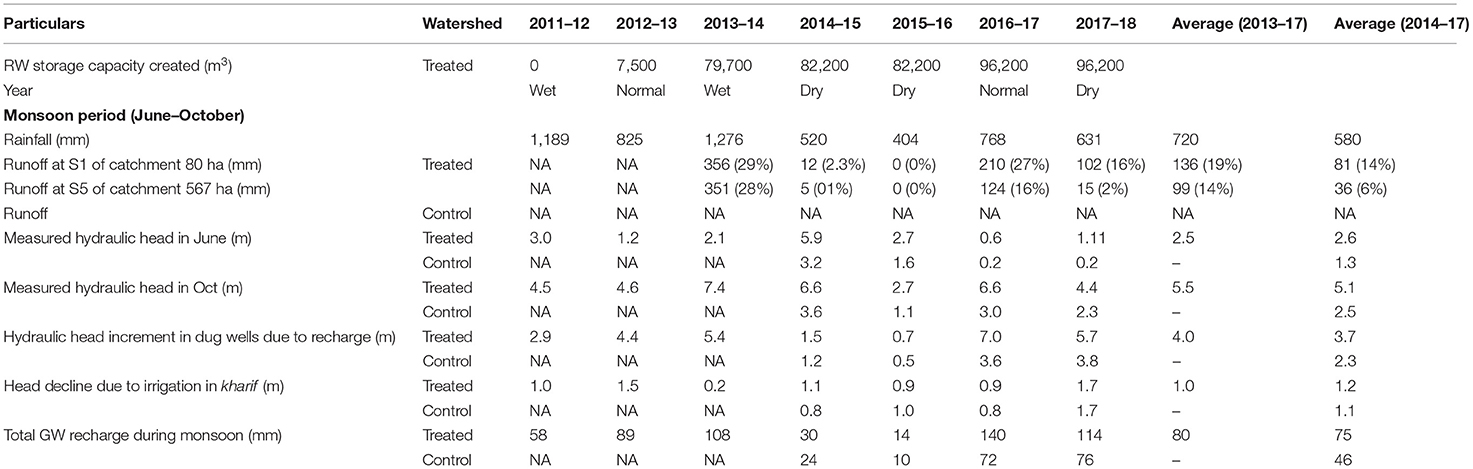
Table 1. Major water balance components of Parasai-Sindh (treated) and nearby control watersheds in Jhansi district.
Reservoir (Haveli) Hydrology
Figure 5 shows the reservoir (haveli) hydrology of the treated watershed. Figure 5A shows cumulative rainfall vs. cumulative inflow measured at S1. There is a positive relationship between rainfall and inflow received. Inflow received at S1 largely depends on the intensity of the rainfall. For example, in 2013 there was not much runoff received—only up to 200 mm cumulative rainfall—as the rainfall intensity was relatively low during the month of June. But in 2017, a few incidences of high intensity events generated runoff, even going up to 50 mm of cumulative rainfall. Figure 5B also describes the generated outflow (spillway amount) from the haveli structure. As the water harvesting capacity of haveli structure was 70,000 m3 (equivalent to 87.5 mm runoff volume up to its catchment level of 80 ha), the generated inflow was initially harvested in the beginning of the monsoon period, so no spillway amount was recorded in June. Most of the spillway amount was recorded only in July or August months.
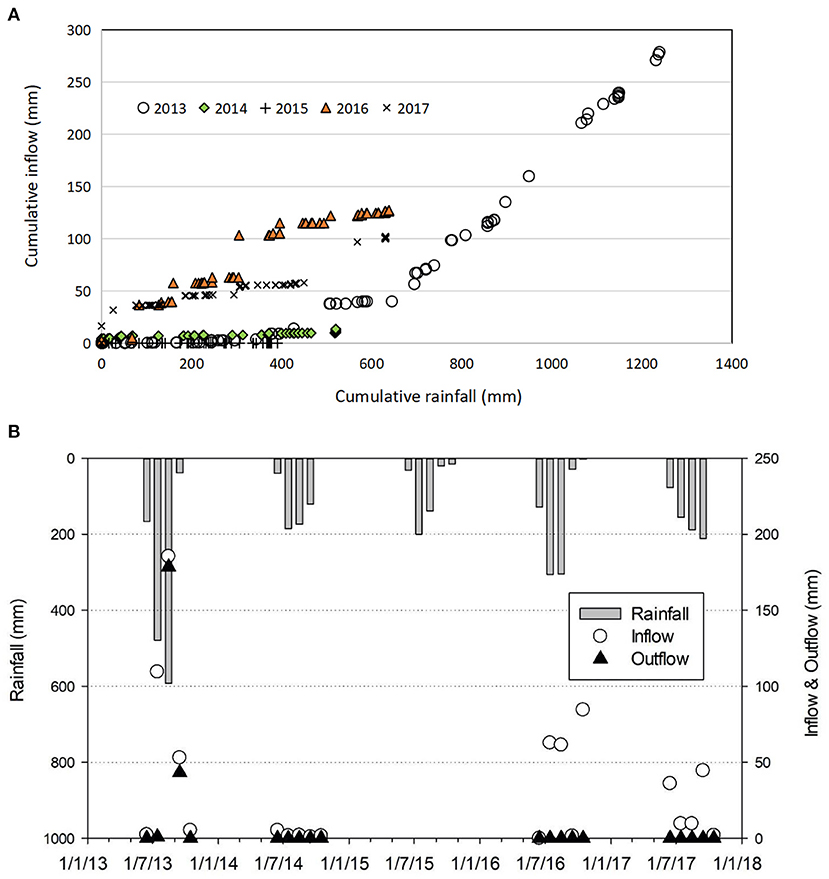
Figure 5. (A) Cumulative rainfall vs. inflow at Haveli structure; (B) Inflow and outflow in different years.
Reservoir water balance (inflow, outflow and storage) of the haveli (S1) along with rainfall is further presented at daily intervals for the wet year (2013), and normal year (2016) in Figures 6A,B. In order to maintain uniformity among measuring units, all the reservoir water balance components (i.e., inflow, outflow, and reservoir storage) are shown in volumetric term (cubic meter). In 2013, about 4,000–20,000 m3 surface runoff was generated by 3–4 high rainfall events (including 79.4 mm on 20 July) that was harvested in the haveli structure. This helped accumulate an amount of 24,000 m3 water on a single day, i.e., 20th July 2013. Inflow received between 20th and 30th July was <1,700 m3 due to low intensity rainfall events. During this period, the volume of harvested water also declined due to percolation. About 10,000 m3 of rainwater percolated within 11 days (i.e., 20th−30th July). The watershed witnessed high intensity rainfall events on 31st July and 01st August (2 day cumulative rainfall of 105 mm). Apart from this, 12 small to medium rainfall events occurred between 01 and 15 August and generated a significant surface runoff (inflow) leading to filling of the haveli to its full capacity. Inflow and outflow volumes were found almost same after 15th August as the haveli was at its full capacity and all the generated runoff got spilled over downstream.
On the other hand, in 2016, inflow generated by different rainfall events was not sufficient to fill the haveli to its full capacity. A total of five events—of about 50 mm rainfall events (daily basis)—were received between July and August 2016 with an almost uniform temporal distribution (7–10 days interval). Generated runoff was completely captured in the haveli as shown in Figure 6B and no spillover (outflow) was recorded from this structure in 2016.
Groundwater Availability
Table 1 indicates the difference in measured average hydraulic head before and after monsoon in respective years. June month is considered as pre-monsoon period and October is post-monsoon period. Difference in hydraulic head between October and June is considered as net recharge in respective watersheds (i.e., treated and control). In general, the difference in water table technique also helps in estimating net amount of water applied as irrigation during kharif in different years in respective watersheds, as presented in Table 1. The groundwater table measured in the control watershed was initiated from 2014 onwards. Therefore, comparison between treated and control watershed was only possible for 4 years. Groundwater recharge on average was estimated to be 80 mm during the monsoon period. There was year-to-year variation in groundwater recharge as per rainfall variability. Groundwater recharge in 2013 in the treated watershed was found to be 108 mm (after) as compared to 58 mm (before) in 2011–12 which was also one of the wet years. This indicates that introduction of haveli system has improved the groundwater recharge additionally by 50 mm across the watershed. In 2014–15, the groundwater recharge was almost the same as the insignificant runoff that was harvested in these structures. In 2016–17, which was the normal year (rainfall 768 mm), groundwater recharge in treated watershed was 140 mm compared to 72 mm in control watershed. On average, groundwater recharge in the treated watershed during 2014–17 was found to be 75 mm as compared to 46 mm in the control watershed.
Figure 7 depicts the functioning status of dug wells in both treated and control watersheds. To understand the temporal variability, all functioning wells were categorized into five groups based on available hydraulic head: dry (no water); poor (<1 m); moderate (1–3 m); good (3–5 m); and excellent (>5 m). Figures 7A,B showed the water availability (pressure head) status of functioning wells across the months between 2014 and 2016, in treated and control watersheds.
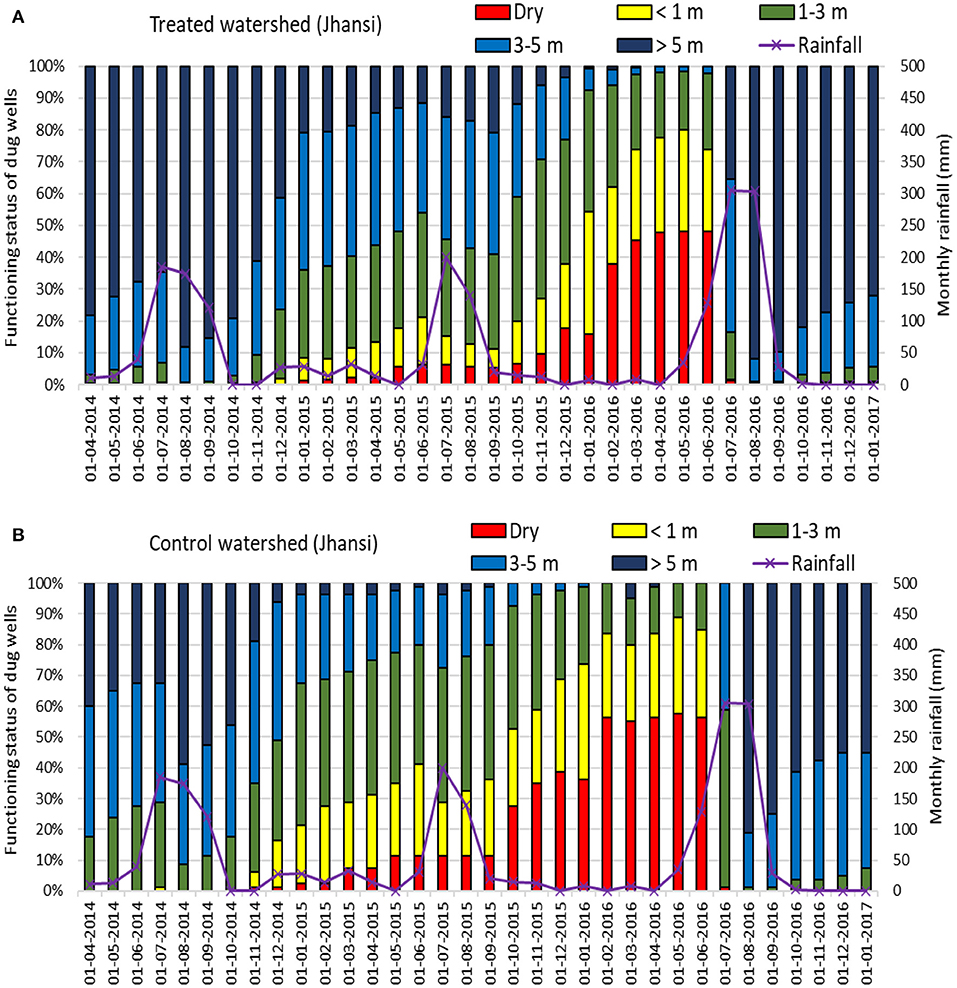
Figure 7. Impact of RWH intervention on functioning status of dug wells in (A) treated and (B) control watersheds at Jhansi.
During 2014, 60–80% of dug wells in the treated watershed had excellent yields (>5 m head) as compared to 30–50% in the control watershed, between April and December months. Results showed that about 10–20% of dug wells were yielding excellently (>5 m head), 35–40% were good (3–5 m head); 20–25% had moderate yield (1–3 m); and <20% showed poor yield in the treated watershed during June–September 2015. On the other hand, only 2–5% of total wells were in the excellent category (>5 m), <20% were good (3–5 m head); 40% moderate; and 30–50% wells were in poor yielding/non-functional category in the control watershed during June to September 2015. By February 2016, more than 40% of the wells were yielding with moderate to good hydraulic head in the treated watershed as compared to only 17% in the control watershed. At any point of time, 20% wells in treated watersheds were yielding at least with moderate status as compared to 10% in control watershed. During the beginning of the July 2016 monsoon, 35% of wells were rejuvenated back to excellent, 40% to good, and 33% to moderate in the treated watershed. However, in July 2016 about 40% and 60% of wells were showing good and moderate yielding status in the control watershed.
Results showed that about 5% (treated watershed) and 10% (control watershed) of the dug wells were dry even during monsoon season in 2015 (June–October) due to deficit rainfall. As indicated earlier, 2015 was one of the driest years with a total rainfall of 404 mm, and 2016 was close to normal with a rainfall of 768 mm. A remarkable difference was found after the end of the monsoon as 30% wells dried in the control watershed as compared to 6% in the treated watershed by November 2015. By the end of February 2016, 17% dug wells were dry in the treated watershed as compared to 37% in the control. Major change was further recorded by end of March 2016 as about 40% and 57% of wells were dry in treated and control watersheds, respectively. Shallow perched groundwater is largely pumped out between November and March as farmers provide supplemental irrigation to rabi crops. No noticeable change was observed in the status of dug wells between April and May 2016, as there were not many agricultural activities except fodder cultivation in <5% of fields. Subsequent to the receipt of good rains in July 2016, all the dug wells in the treated and control watersheds got rejuvenated.
Crop Intensification and Crop Yield
NDVI of treated watershed during February months for 2010–11 (before interventions) and 2013–14 and 2014–15 (after interventions) is shown in Figure 8. Rainfall received during 2010–11 was 1,170 mm. However, about 30% of land at upland was left fallow during rabi season before project interventions despite 2010–11 being a wet year in the uplands of the landscape because water was scarce in the post-monsoon season and farmers were reluctant to cultivate. The only farming was found in fields close to the peripheral stream network, but after the project interventions, almost 95% of the area was brought under cultivation in 2013–14—as indicated by the NDVI map. February is peak vegetative crop growth stage of wheat crop in this area. Further, it must be noted that 2014–15, which was one of the dry years, also had a relatively better crop acreage as compared to 2010–11. This has been possible due to enhanced groundwater availability which was supported from previous year. Despite having negligible groundwater recharge in 2014–15, the landscape was able to support the cultivation of a second season crop with previously available groundwater reserves.
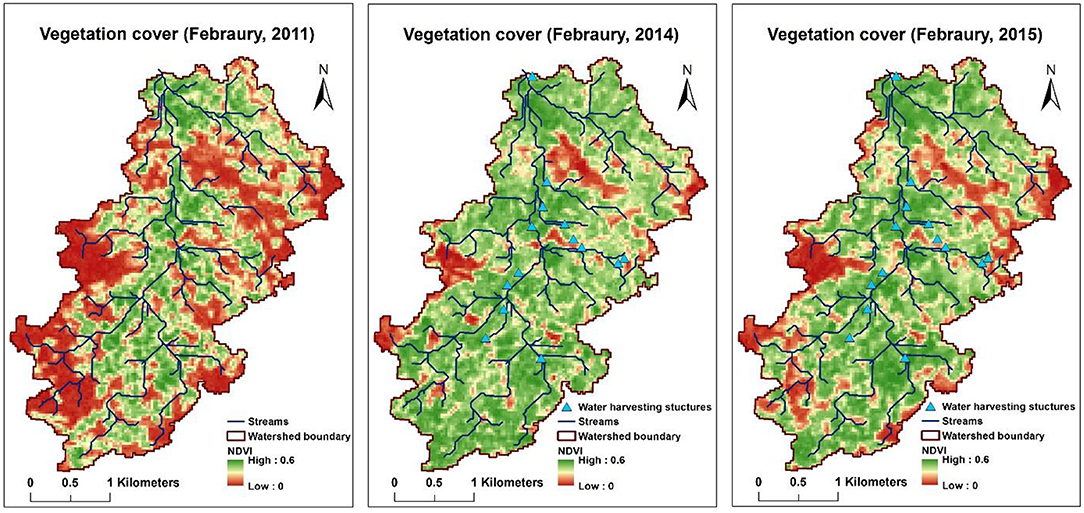
Figure 8. NDVI mapping from remote sensing during February represents rabi crop area at Parasai-Sindh watershed before (2011) and after (2014 and 2015) the watershed interventions. Rainfall = 2010–11: 1,190 mm; 2013–14: 1,270 mm; 2014–15: 520 mm.
Table 2 indicates cultivated area under different crops (monsoon and post-monsoon) before and after project interventions in Parasai-Sindh watershed. Out of a total cultivable area of 1,106 ha, 63% was under groundnut, 24% under pulses, and 12% of the area was left fallow in monsoon before project interventions. After project interventions, farmers elected to increase area under groundnut, which increased to 82%, whereas the area under pulses reduced to 14%, and about 4% was left fallow in the monsoon season. On the other hand, during post-monsoon season, before project interventions, 51% was under wheat, 11% under mustard, and 10% under other crops such as chickpea, lentils, and 28% was left fallow. After project interventions, a remarkable change took place with 87% of total cultivated land converted into wheat, and 10% with other crops, and only 2% area was left fallow. The area under fodder and vegetable crops increased from 5 to 50 ha during the summer period with increased water availability.
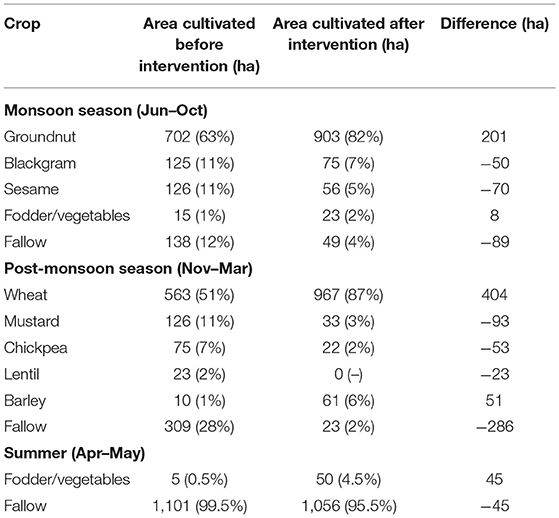
Table 2. Cultivated area under different crops before and after project interventions in Parasai-Sindh watershed (figures in parenthesis indicate percent of total cultivable land).
Figure 9 compares the yield of major crops before and after project interventions. Yield for monsoon season crops (sesame, blackgram, groundnut) increased marginally, but significant difference was found in crop yield obtained in post-monsoon season crops. Wheat yield which was 1,700 kg/ha has increased to 2,750 kg/ha. Similarly, barley yield also increased from 1,800 to 2,600 kg/ha after project interventions.
Household Income
Table 3 indicates the change in average household income before and after project interventions. Out of 417 households, total net income from agriculture before the watershed interventions was estimated as USD 0.26 million, which increased to USD 0.73 million (i.e., a 180% increase). Annual income increased from livestock was estimated as USD 0.21 million (increased from USD 0.19 million to USD 0.40 million). However, this article does not focus on livestock component. This income is mainly generated from increased buffalo population from 950 (before) to 1,300 (after) and increased milk yield from 6 L/day/animal to 8.5 L/day/animal (with lactation period of 180 days). Overall, average household income increased from USD 1,075/year (before) to USD 2,725/year (after), thus making a significant improvement in their livelihood within the short period of project interventions.
Discussion
Recharging Shallow Aquifer System-Built Resilience for Smallholders
Water balance analysis indicates that dry years generally do not contribute in terms of groundwater recharge as generated runoff is limited. However, the opportunity to harvest runoff is only available during normal and wet years. In the absence of decentralized rainwater harvesting interventions, recharge from natural landscape is not sufficient even in wet years, which therefore results in water scarcity for domestic and agriculture use. In the current study, it was realized that once groundwater got recharged during the wet years, it would remain available in subsequent years and help to build resilience. The decentralized rainwater harvesting system is beneficial for the shallow dug well system as these wells quickly get recharged with increased retention ability of the landscape. The generated runoff is harvested in upstream structures which facilitate percolation to underlying aquifers, thus making fresh water readily available for groundwater withdrawal. In such ecologies, the concept of rejuvenating the traditional haveli system is one of the good adaptation measures for addressing water scarcity problems of the region. Most of the havelis are located near human habitats, therefore, rejuvenation of these havelis hold opportunities to address the fresh water needs of domestic and livestock sectors (Dev et al., 2022). These structures, in general, fill 2–3 times of their storage capacity depending on rainfall intensity, location of structure on toposequence and catchment area. Thousands of such structures are available across the region which require adequate attention for their rejuvenation, as it holds huge potential to address the water scarcity issue. Under the changing climate scenario, decentralized management of rainwater is crucial as frequent dry spells followed by wet years are expected. These interventions are helpful both in dry and wet years. Wet years provide opportunity to harvest runoff within the landscape and reduce the floods in downstream areas. Furthermore, harvested water from wet years in the form of groundwater could be utilized in subsequent years. Unlike surface water irrigation system, once groundwater is recharged it is available for a relatively longer period, and enhances the baseflow availability which is indeed important for rejuvenating the riverine ecosystem (Singh et al., 2014). Decentralized rainwater harvesting not only strengthens the provisioning services but also regulates and supports ecosystem services for long-term sustainability (Singh et al., 2014; Carmenta et al., 2020; Garg et al., 2020b, 2022a; Reed et al., 2020; Anantha et al., 2021b,c, 2022a; Wable et al., 2021).
Sustainable Crop Intensification Opportunities
Degraded ecologies, such as the Bundelkhand region, has a cropping intensity that is relatively low (80–110%) despite receiving moderate rainfall ranging from 600 to 1,000 mm. About 20% of cultivable areas in uplands are left permanently fallow and other cultivable lands are under seasonal fallow due to water scarcity. The results clearly indicated that haveli rejuvenation along with decentralized rainwater harvesting interventions have helped to turn these unproductive landscapes into productive landscapes within a short period. In the absence of supplemental irrigation, cultivation in uplands always involved risk of crop failure. Therefore, adoption of different field scale technologies, such as improved crop cultivars, fertilizers, and mechanization is also relatively low (Anantha et al., 2021c). Anantha et al. (2021c) indicated that until and unless moisture availability is ensured, the adoption of improved technologies will always be low. Therefore, landscape-based resource conservation technologies need to be adopted for achieving sustainable crop intensification.
There was a significant amount of residual soil moisture that was lost as non-productive evaporation prior to these project interventions. With availability of supplemental irrigation, these landscapes are now supporting two crops per year, bringing about enhanced land and water use efficiency (Garg et al., 2022b). The water balance of the landscape indicates that out of 135 mm generated runoff, only 35–40 mm (i.e., 25%) is harvested at upstream locations which has brought a significant difference in terms of crop intensification, productivity and income. Scaling up of such activities may affect downstream water availability. However, this is creating a significant difference in the well-being of upland farmers. Further, detailed analysis of water balance indicates that such tradeoff is not always negative. Harvested water at upland during wet years does not negatively affect downstream water availability as generated runoff is several times higher than the harvested amount. But generated runoff in dry years is too low to meet the requirements of both upstream and downstream ecosystems. The difference could be only visible during a normal year. However, at the same time it controls land degradation, improves agriculture productivity, and livelihoods of upland farmers.
Conclusions
This article addresses the challenges of water scarcity and poor crop intensification through decentralized rainwater harvesting measures—especially the haveli system of traditional rainwater harvesting—in one of the degraded ecologies of Central India. Rejuvenation of the haveli cultivation system was demonstrated in all seven districts of Bundelkhand region, out of which one paired watershed was selected to monitor the landscape hydrology between 2011 and 2017. Key monitoring indicators such as surface runoff, groundwater recharge, change in cropping intensity and crop productivity were measured. The findings of the study are given below.
• Water balance of the landscape—between 2013 and 2017—indicates that on average 135 mm of runoff was generated from 720 mm rainfall under natural landscape condition. Haveli system and other structures harvested 35 mm runoff on average, and facilitated enhanced groundwater recharge. However, year-to-year variability is recorded in runoff generation. The haveli system of rainwater harvesting garnered runoff 2–3 times of their storage capacity and enhanced groundwater availability.
• With decentralized rainwater harvesting measures, defunct dug wells have gotten rejuvenated and started functioning for a longer time duration. Between 2013 and 2017 the estimated annual average groundwater recharge for treated watershed was 75 mm as compared to 46 mm in control watershed.
• With improved groundwater availability 20% permanent fallow was brought into productive cultivation, and crop yield increased from 10 to 70% over the baseline period. Significant increase in crop yield was found in rabi season as it addressed the water scarcity problem of the landscape. This has translated into net income gain from USD 1,075/household/year (before) to USD 2,725/household/year (after).
• There is huge opportunity for addressing water scarcity, poor agriculture productivity and income insecurity of rural households through the rejuvenation of the traditional rainwater harvesting structures in Central India. These interventions were found to be not only strengthening various provisioning ecosystem services but also regulating upstream groundwater availability, flood control and enhancing base flow. Moreover, only a meager 25% of generated runoff was harvested from upstream and the rest was available for downstream ecosystem.
This study submits science-backed evidence indicating the potential of dryland regions to address food and water scarcity, poor resource use efficiency, and marginal livelihood options through integrated natural resource management interventions, particularly by developing a hybrid model blending traditional wisdom with new knowledge. These learnings are helpful in addressing the challenges of similar agroecological regions of Asia and Africa and can help address the United Nations Sustainable Development Goals of 2030.
Data Availability Statement
The raw data supporting the conclusions of this article will be made available by the authors, without undue reservation.
Author Contributions
RS: project administration, supervision, investigation, and data curation. VA: software and methodology. KA: writing—original draft, methodology, and formal analysis. KG: conceptualization, writing—original draft, formal analysis, and methodology. JB, AW, ID, and SD: review and editing. All authors contributed to the article and approved the submitted version.
Conflict of Interest
The authors declare that the research was conducted in the absence of any commercial or financial relationships that could be construed as a potential conflict of interest.
Publisher's Note
All claims expressed in this article are solely those of the authors and do not necessarily represent those of their affiliated organizations, or those of the publisher, the editors and the reviewers. Any product that may be evaluated in this article, or claim that may be made by its manufacturer, is not guaranteed or endorsed by the publisher.
Acknowledgments
The authors would like to thank the Government of Uttar Pradesh for allocating development funds under Rashtriya Krishi Vikas Yojana (RKVY) for undertaking natural resource management interventions in seven districts of Bundelkhand region, Uttar Pradesh. The authors also gratefully acknowledge the financial assistance from CocaCola India Foundation to develop the Parasai-Sindh watershed in Jhansi district. Thanks also to the CGIAR Research Program on Water, Land and Ecosystems for providing support in establishing a hydrological monitoring system.
References
Agarwal, A., and Narain, S. (2003). Dying Wisdom: Rise, Fall and Potential of India's Traditional Water Harvesting Systems; State of India's Environment - A Citizens' Report No. 4. New Delhi: Centre for Science and Environment.
Anantha, K. H.. (2013). Economic implications of groundwater exploitation in hard rock areas of southern peninsular India. Environ. Dev. Sustain. 15, 587–606. doi: 10.1007/s10668-012-9394-0
Anantha, K. H., Garg, K. K., Barron, J., Dixit, S., Venkataradha, A., Singh, R., et al. (2021c). Impact of best management practices on sustainable crop production and climate resilience in smallholder farming systems of South Asia. Agric. Syst. 194, 103276. doi: 10.1016/j.agsy.2021.103276
Anantha, K. H., Garg, K. K., Moses, S. D., Patil, M. D., Sawargaonkar, G. L., Kamdi, P. J., et al. (2021b). Impact of natural resource management interventions on water resources and environmental services in different agroecological regions of India. Groundw. Sustain. Dev. 13, 100574. doi: 10.1016/j.gsd.2021.100574
Anantha, K. H., Garg, K. K., Petrie, C. P., and Dixit, S. (2021a). Seeking sustainable pathways for fostering agricultural transformation in peninsular India. Environ. Res. Lett. 16, 044032. doi: 10.1088/1748-9326/abed7b
Anantha, K. H., Garg, K. K., Singh, R., Venkataradha, A., Dev, I., Petrie, C. A., et al. (2022a). Landscape resource management for sustainable crop intensification. Environ. Res. Lett. 17, 014006. doi: 10.1088/1748-9326/ac413a
Anantha, K. H., Garg, K. K., Singh, S., Dev, I., Venkataradha, A., Srivastava, A. K., et al. (2022b). Exploring the Linkages Between Resource Endowments and Rural Livelihood System in Bundelkhand Region, Central India. Land Use Policy.
Barrio, G. D., Sanjuán, M. E., Martínez-Valderrama, J., Ruiz, A., and Puigdefábregas, J. (2021). Land degradation means a loss of management options. J. Arid Environ. 189, 104502. doi: 10.1016/j.jaridenv.2021.104502
Carmenta, R., Coomes, D. A., DeClerck, F. A. J., Hart, A. K., Harvey, C. A., Milder, J., et al. (2020). Characterizing and evaluating integrated landscape initiatives. One Earth 2, 174–187. doi: 10.1016/j.oneear.2020.01.009
Cowie, A. L., Orr, B. J., Castillo Sanchez, V. M., Chasek, P., Crossman, N. D., Erlewein, A., et al. (2018). Land in balance: the scientific conceptual framework for Land Degradation Neutrality. Environ. Sci. Pol. 79, 25–35. doi: 10.1016/j.envsci.2017.10.011
De Wit, M., and Stankiewicz, J. (2006). Changes in surface water supply across Africa with predicted climate change. Science 311, 1917–1921. doi: 10.1126/science.1119929
DeClerck, F. A. J., Jones, S. K., Attwood, S., Bossio, D., Girvetz, E., Chaplin-Kramer, B., et al. (2016). Agricultural ecosystems and their services: the vanguard of sustainability? Curr. Opin. Environ. Sustain. 23, 92–99. doi: 10.1016/j.cosust.2016.11.016
Dev, I., Singh, R., Garg, K. K., Ram, A., Singh, D., Kumar, N., et al. (2022). Transforming livestock productivity through watershed interventions: a case study of Parasai-Sindh watershed in Bundelkhand region of Central India. Agric. Syst. 196, 103346. doi: 10.1016/j.agsy.2021.103346
Dewandel, B., Perrin, J., Ahmed, S., Aulong, S., Hrkal, Z., Lachassagne, P., et al. (2010). Development of a tool for managing groundwater resources in semi-arid hard rock regions: application to a rural watershed in South India. Hydrol. Proc. 24, 2784–2797. doi: 10.1002/hyp.7696
Garg, K. K., Anantha, K. H., Dixit, S., Nune, R., Venkataradha, A., Wable, P., et al. (2022a). Impact of raised beds on surface runoff and soil loss in Alfisols and Vertisols. CATENA 211, 105972. doi: 10.1016/j.catena.2021.105972
Garg, K. K., Anantha, K. H., Nune, R., Akuraju, V. R., Singh, P., Gumma, M. K., et al. (2020a). Impact of land use changes and management practices on groundwater resources in Kolar district, Southern India. J. Hydrol. Reg. Stud. 31, 100732. doi: 10.1016/j.ejrh.2020.100732
Garg, K. K., Anantha, K. H., Venkataradha, A., Dixit, S., Singh, R., and Ragab, R. (2021). Impact of rainwater harvesting on hydrological processes in a fragile watershed of South Asia. Groundwater. 59, 839–855. doi: 10.1111/gwat.13099
Garg, K. K., Karlberg, L., Barron, J., Wani, S. P., and Rockstrom, J. (2012). Assessing impacts of agricultural water intervention in the Kothapally watershed, Southern India. Hydrol. Proc. 26, 387–404. doi: 10.1002/hyp.8138
Garg, K. K., Singh, R., Anantha, K. H., Singh, A. K., Akuraju, V. R., Barron, J., et al. (2020b). Building climate resilience in degraded agricultural landscapes through water management: a case study of Bundelkhand region, Central India. J. Hydrol. 591, 125592. doi: 10.1016/j.jhydrol.2020.125592
Garg, K. K., Venkataradha, A., Anantha, K. H., Singh, R., Whitbread, A. M., and Dixit, S. (2022b). Identifying potential zones for rainwater harvesting interventions for sustainable intensification in the semi-arid tropics. Sci. Rep. Nat. 12, 3882. doi: 10.1038/s41598-022-07847-4
Garg, K. K., and Wani, S. P. (2013). Opportunities to build groundwater resilience in the semi-arid tropics. Ground Water 51, 679–691. doi: 10.1111/gwat.1007
Gleeson, T., Befus, K. M., Jasechko, S., Luijendijk, E., and Cardendas, M. B. (2016). The global volume and distribution of modern groundwater. Nat. Geosci. 9, 161–167. doi: 10.1038/ngeo2590
Glendenning, C. J., and Vervoort, R. W. (2010). Hydrological impacts of rainwater harvesting (RWH) in a case study catchment: the Arvari river, Rajasthan, India. Part 1: field-scale impacts. Agric. Water Manage. 98, 331–342. doi: 10.1016/j.agwat.2010.09.003
Golani, F. M., and Ozha, D. D. (2006). Rehabilitation of traditional water harvesting systems of Rajasthan and their significance in present context. J. Inst. Public Health Eng. 3, 28–30.
Gordon, L. J., Finlayson, C. M., and Falkenmark, M. (2010). Managing water in agriculture for food production and other ecosystem services. Agric. Water Manage. 97, 512–519. doi: 10.1016/j.agwat.2009.03.017
Government of India (2019). National Compilation on Dynamic Ground Water Resources of India, 2017. Faridabad: Ministry of Jal Shakti, Department of Water Resources, RD & GR Central Ground Water Board.
Grewal, S. S., Mittal, S. P., Agnihotri, Y., and Dubey, L. N. (1989). Rainwater harvesting for the management of agricultural droughts in the foothills of northern India. Agric. Water Manage. 16, 309–322. doi: 10.1016/0378-3774(89)90028-0
Meter, K. J. V., Basu, N. B., Tate, E., and Wyckoff, J. (2014). Monsoon harvests: the living legacies of rainwater harvesting systems in South India. Environ. Sci. Technol. 48, 4217–4225. doi: 10.1021/es4040182
Meter, K. J. V., Steiff, M., McLaughlin, D. L., and Basu, N. B. (2016). The socioecohydrology of rainwater harvesting in India: understanding water storage and release dynamics across spatial scales. Hydrol. Earth Syst. Sci. 20, 2629–2647. doi: 10.5194/hess-20-2629-2016
Mukherjee, S.. (2021). Nonlinear recurrence quantification of the monsoon-season heavy rainy-days over northwest Himalaya for the baseline and future periods. Sci. Total Environ. 789, 147754. doi: 10.1016/j.scitotenv.2021.147754
Padmaja, R., Kavitha, K., Pramanik, S., Duche, V. D., Singh, Y. U., Whitbread, A. M., et al. (2020). Gender transformative impacts from watershed interventions: insights from a mixed-methods study in the Bundelkhand region of India. Trans. ASABE. 63, 153–163. doi: 10.13031/trans.13568
Pandey, D. N., Gupta, A. K., and Anderson, D. M. (2003). Rainwater harvesting as an adaptation to climate change. Curr. Sci. 85, 46–59. Available online at: https://hdl.handle.net/10535/2400
Pavelic, P., Patankar, U., Acharya, S., Jella, K., and Gumma, M. K. (2012). Role of groundwater in buffering irrigation production against climate variability at the basin scale in South-West India. Agric. Water Manage. 103, 78–87. doi: 10.1016/j.agwat.2011.10.019
Prǎvǎlie, R.. (2021). Exploring the multiple land degradation pathways across the planet. Earth Sci. Rev. 220, 103689. doi: 10.1016/j.earscirev.2021.103689
Queiroz, C., Norström, A. V., Downing, A., Harmáčková, Z. V., De Coning, C., Adams, V., et al. (2021). Investment in resilient food systems in the most vulnerable and fragile regions is critical. Nat Food. 2, 546–551. doi: 10.1038/s43016-021-00345-2
Rao, A. V. R. K., Wani, S. P., Singh, K. K., Ahmed, M. I., Srinivas, K., Bairagi, S. D., et al. (2013). Increased arid and semi-arid areas in India with associated shifts during 1971-2004. J. Agrometeorol. 15, 11–16. Available online at: http://oar.icrisat.org/id/eprint/6953
Reddy, V. R., Reddy, M. S., and Palanisami, K. (2018). Tank rehabilitation in India: review of experiences and strategies. Agric. Water Manage. 209, 32–43. doi: 10.1016/j.agwat.2018.07.013
Reed, J., Ickowitz, A., Chervier, C., Djoudi, H., Moombe, K., Ros-Tonen, M., et al. (2020). Integrated landscape approaches in the tropics: a brief stock-take. Land Use Policy 99, 104822. doi: 10.1016/j.landusepol.2020.104822
Robinson, L. W., Ericksen, P. J., Chesterman, S., and Worden, J.S. (2015). Sustainable intensification in drylands: what resilience and vulnerability can tell us. Agric. Syst. 135, 133–140. doi: 10.1016/j.agsy.2015.01.005
Rockström, J., Falkenmark, M., Allan, T., Folke, C., Gordon, L., Jägerskog, A., et al. (2014). The unfolding water drama in the Anthropocene: towards a resilience based perspective on water for global sustainability. Ecohydrology. 7, 1249–1261. doi: 10.1002/eco.1562
Saha, R., Ghosh, P. K., Mishra, V. K., and Bujarba-ruah, K. M. (2007). Low-cost micro-rainwater harvesting technology (Jalkund) for new livelihood of rural hill farmers. Curr. Sci. 92, 1258–1265.
Sahu, R. K., Rawat, A. K., and Rao, D. L. N. (2015). Traditional rainwater management system (‘Haveli') in vertisols of central India improves carbon sequestration and biological soil fertility. Agric. Ecosyst. Environ. 200, 94–101. doi: 10.1016/j.agee.2014.11.005
Schlaepfer, D. R., Bradford, J. B., Lauenroth, W. K., Munson, S. M., Seth, M., Tietjen, B., et al. (2017). Climate change reduces extent of temperate drylands and intensifies drought in deep soils. Nat. Commun. 8, 14196. doi: 10.1038/ncomms14196
Shah, T.. (2009). Climate change and groundwater: India's opportunities for mitigation and adaptation. Environ. Res. Lett. 4, 035005. doi: 10.1088/1748-9326/4/3/035005
Sharda, V. N., Kurothe, R. S., Sena, D. R., Pande, V. C., and Tiwari, S. P. (2006). Estimation of groundwater recharge from water storage structures in a semi-arid climate of India. J. Hydrol. 329, 224–243. doi: 10.1016/j.jhydrol.2006.02.015
Sidhu, B. S., Kandlikar, M., and Kutty, R. N. (2020). Power tariffs for groundwater irrigation in India: a comparative analysis of the environmental, equity, and economic trade-offs. World Dev. 128, 104836. doi: 10.1016/j.worlddev.2019.104836
Singh, C., Rahman, A., Srinivas, A., and Bazaz, A. (2018). Risks and responses in rural India: implications for local climate change adaptation action. Climate Risk Manage. 21, 52–68. doi: 10.1016/j.crm.2018.06.001
Singh, C., Solomon, D., Bendapudi, R. K., Kuchimanchi, B., Iyer, S., and Bazaz, A. (2019). What shapes vulnerability and risk management in semi-arid India? Moving towards an agenda of sustainable adaptation. Environ. Dev. 30, 35–50. doi: 10.1016/j.envdev.2019.04.007
Singh, P. K., Verma, S. K., Moreno, J. A., Singh, V. K., Malviya, V. P., Oliveira, E. P., et al. (2019). Geochemistry and Sm Nd isotope systematics of mafic-ultramafic rocks from the Babina and Mauranipur greenstone belts, Bundelkhand Craton, India: implications for tectonic setting and Paleoarchean mantle evolution. Lithos 330, 90–107. doi: 10.1016/j.lithos.2019.02.010
Singh, R., Garg, K. K., Anantha, K. H., Venkataradha, A., Dev, I., Dixit, S., et al. (2021). Building resilient agricultural system through groundwater management interventions in degraded landscapes of Bundelkhand region, Central India. J. Hydrol. Regional Stud. 37, 100929. doi: 10.1016/j.ejrh.2021.100929
Singh, R., Garg, K. K., Wani, S. P., Tewari, R. K., and Dhyani, S. K. (2014). Impact of water management interventions on hydrology and ecosystem services in Garhkundar - Dabar watershed of Bundelkhand region, Central India. J. Hydrol. 509, 132–149. doi: 10.1016/j.jhydrol.2013.11.030
Singh, S.. (2020). Farmers' perception of climate change and adaptation decisions: a micro-level evidence from Bundelkhand Region, India. Ecol. Indic. 116, 106475. doi: 10.1016/j.ecolind.2020.106475
Tek, B. S., Jat, M. L., Jat, R. K., Kapoor, P., and Clare, S. (2016). “Yield estimation of food and non-food crops in smallholder production systems,” in Methods for Measuring Greenhouse Gas Balances and Evaluating Mitigation Options in Smallholder Agriculture, eds T. S. Rosenstock, M. C. Rufino, K. ButterbachBahl, E. Wollenberg, and M. Richards (Cham: Springer), 163–174. doi: 10.1007/978-3-319-29794-1_8
Thomas, T., Jaiswal, R. K., Nayak, P. C., and Ghosh, N. C. (2015). Comprehensive evaluation of the changing drought characteristics in Bundelkhand region of Central India. Meteorol. Atmos. Phys. 127, 163–182. doi: 10.1007/s00703-014-0361-1
Tilahun, S. A., Yilak, D. L., Schmitter, P., Langan, S., Barron, J., Parlange, J. Y., et al. (2020). Establishing irrigation potential of a hillside aquifer in the African highlands. Hydrol. Process. 34, 1741–1753. doi: 10.1002/hyp.13659
UNEMG (2011). Global Drylands: A UN System-Wide Response. Geneva: United Nations Environment Management Group.
Verma, H. N., and Sarma, P. B. S. (1990). Design of storage tanks for water harvesting in rainfed areas. Agric. Water Manage. 18, 195–207. doi: 10.1016/0378-3774(90)90043-X
Vijayan, R.. (2016). Dryland agriculture in India – problems and solutions. Asian J. Environ. Sci. 11, 171–177. doi: 10.15740/HAS/AJES/11.2/171-177
Villholth, K. G.. (2013). Groundwater irrigation for smallholders in Sub-Saharan Africa – a synthesis of current knowledge to guide sustainable outcomes. Water Int. 38, 369–391. doi: 10.1080/02508060.2013.821644
Wable, P. S., Garg, K. K., Nune, R., Venkataradha, A., Srinivasan, V., Ragab, R., et al. (2021). Impact of agricultural water management interventions on upstream–downstream trade-offs in the upper Cauvery catchment, southern India: a modelling study. Irrigat. Drainage 1–23. doi: 10.1002/ird.2662
Keywords: small farm holders, sustainable intensification, water balance components, shallow groundwater aquifer, traditional rainwater harvesting system
Citation: Singh R, Akuraju V, Anantha KH, Garg KK, Barron J, Whitbread AM, Dev I and Dixit S (2022) Traditional Rainwater Management (Haveli cultivation) for Building System Level Resilience in a Fragile Ecosystem of Bundelkhand Region, Central India. Front. Sustain. Food Syst. 6:826722. doi: 10.3389/fsufs.2022.826722
Received: 01 December 2021; Accepted: 07 March 2022;
Published: 01 April 2022.
Edited by:
Olcay Unver, Arizona State University, United StatesReviewed by:
Sisira S. Withanachchi, University of Kassel, GermanyLindsey M. W. Yasarer, Agricultural Research Service (USDA), United States
Copyright © 2022 Singh, Akuraju, Anantha, Garg, Barron, Whitbread, Dev and Dixit. This is an open-access article distributed under the terms of the Creative Commons Attribution License (CC BY). The use, distribution or reproduction in other forums is permitted, provided the original author(s) and the copyright owner(s) are credited and that the original publication in this journal is cited, in accordance with accepted academic practice. No use, distribution or reproduction is permitted which does not comply with these terms.
*Correspondence: Kaushal K. Garg, k.garg@cgiar.org