- 1Department of Food Science and Nutrition, University of Minnesota, St. Paul, MN, United States
- 2Department of Horticultural Science, University of Minnesota, St. Paul, MN, United States
- 3Department of Soil, Water, and Climate, University of Minnesota, St. Paul, MN, United States
Arugula (Eruca sativa), mizuna (Brassica rapa var. nipponsinca), red giant mustard (Brassica juncea), and spinach (Spinaciaoleracea “Tyee”) are fresh produce crops high in nutritive value that provide shortfall and high interest nutrients addressed in the U.S. Dietary Guidelines. The primary objective of this project was to evaluate fertility treatments unique to these crops that optimize their nutritional capacity. Measurements discussed include: vitamin C, dietary fiber, calcium, iron, potassium, sodium, and nitrate. Plants were grown at the University of Minnesota St. Paul Campus (St. Paul, MN) in a greenhouse from November to April under an 18 h photoperiod and a 24/13°C day/night temperature. Plants were grown using five different fertility treatments, including four organic treatments and one conventional control. The plant treatment combinations were replicated three times and the entire experiment was duplicated. Fertility treatments had a high impact on vitamin C (with over a 3-fold difference in treatments in the first experiment), nitrate (over 10-fold difference among fertility treatments in some species) and potassium concentrations (over 5-fold difference among fertility treatments in some species) in analyzed plant tissue. No consistent differences were found for fiber, calcium, iron and sodium concentrations in tissue analyzed. This is the first study to analyze the impact that different organic treatments can have on multiple nutrients and compounds addressed by the U.S. Dietary Guidelines for high-impact, highly-consumed produce crops.
Introduction
Diets high in fruits and vegetables are widely recommended for their health promoting properties (Slavin and Lloyd, 2012). Fruits and vegetables contain vitamin, minerals, electrolytes, dietary fiber, and phytochemicals especially antioxidants. Although the positive aspects of consumption of fruits and vegetables are promoted, vegetables especially leafy green vegetables are concentrated in nitrates that have been linked to adverse health effects especially in children (Karnpanit et al., 2018). Production practices that increase the nutrient content of vegetables while limiting high amounts of potential negative compounds such as nitrates are of interest.
Organic fruits and vegetables have dominated the organic market in the past three plus decades (Donaldson, 2021). In 2014, organic food sales measured more than twice those of 1994. A 2016 survey found that 68% of Americans had purchased organic food in the previous month. Seventy-one percent of Americans reported that nutrition and ingredients labels were factors in choosing which foods to buy. Additionally, 63% of respondents who paid particular attention to consuming healthy and nutritious foods reported that organic food composes at least some of their intake. On the other hand, in the respondents who did not pay particular attention to consuming healthy and nutritious foods, 22% reported that organic food composes at least some of their food intake (Funk and Kennedy, 2016).
Consumers mainly buy organic due to the alignment of their beliefs with the ideology behind organic production. Accordingly, the above mentioned 2016 survey determined that individuals very concerned about genetically modified foods tended to have chosen organic and GMO-free labeled foods in the previous month. Furthermore, the same 2016 survey reported that 76% of those who had purchased organic in the previous month cited health as motivation. Comparatively, 33% of this same subset claimed environmental reasons, and 22% reported convenience. Finally, 72% of Americans make organic vs. conventional purchase decisions by considering price differences (Funk and Kennedy, 2016).
Almost 75% of conventional grocery stores and close to 20,000 natural food stores sell organic goods (Donaldson, 2021), Generally organic foods are more expensive and more likely to be purchased by higher income consumers (Mie et al., 2017). Lack of access to organic foods for certain populations opposes one of the four principles of organic agriculture: fairness. This principle maintains that organic agriculture should foster equity and justice (IFOAM-Organics International, 2020).
Overall, organic farming principles and practices aim to create a food system that utilizes the biological cycles and resources of the earth in a way that protects biodiversity and preserves the balance of the ecosystem (Hunter et al., 2011). Key fundamentals of traditional organic production encourage long-term fertility of soils, minimize carbon footprints and maintain genetic diversity in current food systems.
In enclosed environment greenhouse systems, key organic practices are implemented, like the use of non-synthetic fertilizers and pesticides, although they are often not complete replacements of traditional organic practices used in open field environments. For example, in open-field organic farming, concern for health of the soil necessitates use of cover crops. Yet in greenhouse production, cover crops are not generally employed.
Differences in organically and conventionally produced foods have been extensively reviewed, based on their nutritive value, sensory qualities and overall safety, but limited information exists on differences in crops grown in greenhouses. Arugula (Eruca sativa), mizuna (Brassica rapavar. nipposinica), and red giant mustard (Brassica juncea) are Mesclun mixture plants that are nutrient-dense and consumed in many regions. Spinach (Spinacia oleracea) is commonly consumed as both a plate vegetable and salad green (Lucier et al., 2004). Consumption of fresh lettuces and spinach, termed microgreens has increased greatly, but little information exists on the nutrient content of these greens, especially when grown in greenhouses (Mir et al., 2017).
Minerals play many critical roles in human physiology and are responsible for a wide range of activities in the body. In plants, iron plays a critical role as a cofactor in chloroplast biosynthesis (Soetan et al., 2010). Calcium is known for its ability to aid in the formation of stable cell walls and membranes, and regulates stimulus of cells (Soetan et al., 2010). Potassium primarily acts as a cofactor for protein synthesis and is a major solute in maintaining water balance and osmosis (Soetan et al., 2010). In the human body, iron, calcium, and potassium are essential nutrients often under consumed.
In plants, vitamin C plays many roles, and the content of Vitamin C in plants varies greatly depending on cultivar, growing conditions, maturity at harvest, and postharvest handling, processing, and storage (Phillips et al., 2018). In the human body, vitamin C plays many roles, including the ability to act as a water-soluble antioxidant, aid in collagen synthesis, increase absorption of iron from the diet and plays other critical roles in the metabolism of folate and some amino acids (Phillips et al., 2018). Dietary fiber is a critical shortfall nutrient in the United States. The typical U.S. individual consumes 17 g/d while the Daily Value is 28 g/day (Slavin, 2013).
Nitrate is a chemical substance naturally found in plants and heavy use of chemical fertilizers, especially nitrogen to increase crop yield increases nitrate levels in plants (Karnpanit et al., 2018). Many vegetables, especially green leafy vegetables contain high amounts of nitrate (Leon and Luzardo, 2020). Nitrate is generally considered safe to consume in moderate amounts and is easily converted to nitrite through reduction. Although nitrate and nitrite are not carcinogenic themselves, they can easily yield carcinogenic compounds. Nitrate reacts with secondary and tertiary amines endogenously, forming N-nitroso compounds (Walker, 2009; Karwowska and Kononiuk, 2020). N-nitroso compounds have been associated with higher risk of developing esophagus, stomach and liver carcinomas. Nitrate levels of vegetables are particularly of concern in childrens' diets (Karnpanit et al., 2018).
Nitrate accumulation is common in Brassica plants as well as other leafy vegetables such as spinach and lettuce and is influenced heavily by nitrogen fertilization practices (Hamdard et al., 2009). Cultivation practices are known to affect nitrate levels in vegetables (Karnpanit et al., 2018). Nitrate contents of most leafy vegetable grown under organic and and GAP (good agricultural practice) cultivation in Thailand were lower than those grown with conventional production (Karnpanit et al., 2018). Other surveys of nitrate concentrations in conventional and organic-labels raw vegetables at retail find that samples of fresh broccoli, cabbage, celery, lettuce and spinach categorized as conventional or organic by label in 5 major cities in diffierent geographic regions of the United States showed no differences in mean nitrate values (Nunez de Gonzalez et al., 2015). Yet in most cases, organic vegetables were numerically lower in nitrate content than their conventional counterparts in their analysis. A study of nitrate contents in regulated and non-regulated leafy vegetables of high consumption in the Canary islands, Spain reported that levels of nitrates in organic vegetables were significantly higher than those of conventional cultivation for chard and watercress (Leon and Luzardo, 2020). No seasonal differences were observed and overall nitrate levels were lower than those reported in other studies. Nitrate levels in organically grown crops were reviewed by Baranski et al. (2014) who concluded that nitrate levels are generally higher in conventionally grown crops because of high mineral nitrogen inputs.
The purpose of this project was to address differences in nutrients of interest in greenhouse-grown arugula, red giant mustard, mizuna and spinach. Specifically we are interested in how nutrient composition of these species is affected by various organic amendments along with a conventional comparison. These four plants were chosen because they have a high nutrient density and they have high amounts of common shortfall nutrients. In addition, they are of interest to growers in cold climates like Minnesota for winter greenhouse production and they are frequently consumed by many populations worldwide. We also determined nitrate content of these leafy green vegetables, both grown conventionally and organically.
Materials and Methods
Plants
Arugula (Eruca sativa), mizuna (Brassica rapa var. nipponsinca), red giant mustard (Brassica juncea), and spinach (Spinacia oleracea “Tyee”) were grown on five different fertilizer/media combinations. All seed for this study was obtained from Johnny's Selected Seeds (Winslow, ME). Arugula, mizuna, and red giant mustard seeds were sown into standard 1,020 trays (28 × 54 × 6 cm) at a density of 11 mL per tray. Spinach was sown into 50 cell deep plug trays with two seeds per cell, plants were thinned to one plant per cell after germination. All four species were grown on all five fertilizer/media combinations and replicated three times. The entire experiment was conducted twice, once starting in November 2014 and once starting in February 2015. Plants were watered by hand as needed. The experiment was set up using a randomized complete block design.
Fertility Treatments
Media and fertility treatments (Table 1) were mixed prior to planting. Fertility was scaled to meet field nitrogen requirements for crops on low organic matter soils according to University of Minnesota Extension materials (Rosen and Eliason, 2005). Treatments were fertilized by converting the recommended kg N/ha to kg N/m3 using a depth of 15 cm, then calculating the weight of solid fertilizer or volume of liquid fertilizer to apply to the volume of media in each container using the guaranteed analysis of each fertilizer product. Both the custom mix and all-in-one potting mix exceeded nitrogen recommendations and so these treatments were not further amended. Full nitrogen recommendation was the equivalent of 112 kg/ha for leafy greens. Bulk densities of the compared media ranged from 0.086 to 0.307 g/cm3 for the treatment groups (Table 1). Chemical analysis of media nutrients was conducted for complete comparison of soil nutrients (Table 2). Greens were grown to baby leaf lettuce size, and so they did not require the second application.
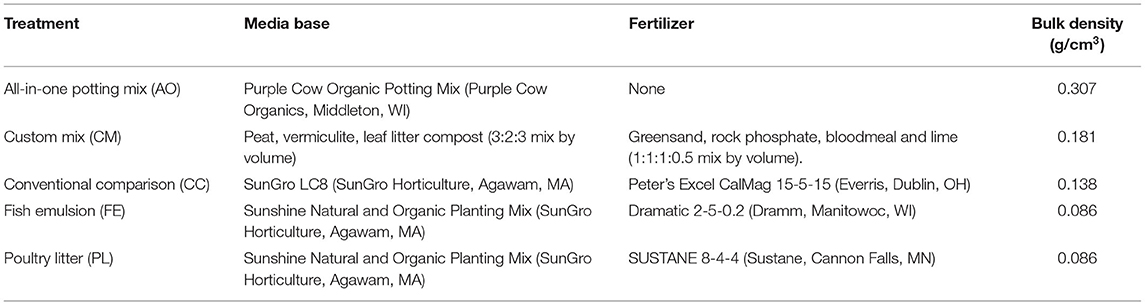
Table 1. Fertility treatment combinations of media, fertility sources, and bulk densities of compared media.
Plant Harvest
All plants were grown on benches in a greenhouse maintained with a 24°C day temperature and 13°C night temperature. Benches were blocked by treatment. Greens were harvested when the majority of leaves were 10 cm long by cutting with scissors. Greens were harvested between 1 and 3 times depending on plant vigor. All plant tissue and soil data were taken at time of first harvest. Harvest occurred between 4 and 9 weeks after planting. Tissue samples for vitamin C and dietary fiber analysis were frozen immediately at −80°C. Tissue samples for mineral analysis were dried to completion at 60°C, and crosschecked to control for moisture removal completion.
Comparison plants were grown at three greenhouses in Minnesota that participated as volunteers in this study. Growers were provided with packets of detailed instructions for the experiment and provided with all required materials. Regular visits were scheduled with research staff to ensure compliance and conduct analysis.
Media and Tissue Analysis
Exchangeable calcium was extracted from the media by mixing 10 mL or 1 molar, pH 7, ammonium acetate with 10 g of air-dried sample, and then placed in a shaker for 5 min. The filtered extract was analyzed with an inductively coupled plasma atomic emission spectrometer (ICP-AES). Extractable iron was determined by treating a 10 g sample of air-dried media with 20 mL of DTPA (Diethylenetriamine-pentaacetic acid) extracting solution. Samples were placed in a shaker for 2 h, and then filtered and analyzed with an ICP-AES for iron concentration of media. Available potassium was extracted from the media by mixing 10 mL of 1 molar ammonium acetate, pH7, with 1 g of air-dried media and then placed in a shaker for 5 min. Available potassium was then measured by analyzing the filtered extract on an ICP-AES set on emission mode at 776 nm. Nitrate-nitrogen was determined by adding 60 mL of KCl extracting solution to a 2 g sample of air-dried media, and then placed in a shaker for 15 min. The nitrate level in the filtered extract was measured on a Lachat QuckChem 8,500 Flow Injection Analyzer by the cadmium reduction method. Bulk densities of media were calculated according to Grafton et al. with minor revisions (Grafton et al., 2015). Plant tissue minerals (calcium, iron, potassium and sodium) were analyzed by weighing 500 mg of air-dried tissue into a 20 mL high form silica crucible and dry ashed at 485°C for 12 h (covered). Ash was then mixed with 5 mL of 20% HCl at room temperature for 30 min, followed by an addition of 5 mL of deionized water, then allowed to settle for 3 h prior to ICP-AES analysis. Plant tissue nitrate-nitrogen was extracted by shaking 300 mg of dried sample with 30 mL 0.1 m CaSO4 solution for 30 min, followed by the addition of 0.85 cc of prewashed charcoal, followed by shaking for an additional 5 min. Samples were filtered through Whatman (No. 42) filter paper and nitrate concentrations in the filtrate were determined colorimetrically by the cadmium reduction method.
Vitamin C and Fiber Quantitation
Vitamin C was quantified using the AOAC 967.22 method from 50 g samples of frozen (−80°C) plant tissue. Total dietary fiber was quantified using the AOAC 991.43 method from 100 g samples of frozen (−80°C) plant tissue.
Statistical Analysis
All statistical analysis was conducted using SPSS (SPSS Chicago, IL). Analysis of variance (ANOVA) with Tukey HSD was used for all tests measuring differences among means. Log transformations were applied where necessary based on regression fit. Statistical significance was achieved for p-values < 0.05.
Comparison of Plants Grown in Experiment 1 and Experiment 2
ANOVA indicated that there were significant differences between experiment 1 and experiment 2 for fertility treatment interactions (p < 0.001), so biomass, nitrate and nutrient levels were analyzed separately for each experiment.
Results
Harvested Biomass
Harvested biomass for arugula varied among the five treatments in experiment 1 (Figure 1). Custom Mix (CM) had the highest harvested biomass (598.5 g/m2), which was significantly more than the Fish Emulsion (FE) treatment (330.7 g/m2; p = 0.039). The CM, All-in-One Potting Mix (AO), Conventional Comparison (CC), and Poultry Litter (PL) treatments were all similar to one another. In experiment 2, the CC and CM treatments resulted in significantly greater harvested biomass than the AO, FE, and PL treatments (p < 0.01).
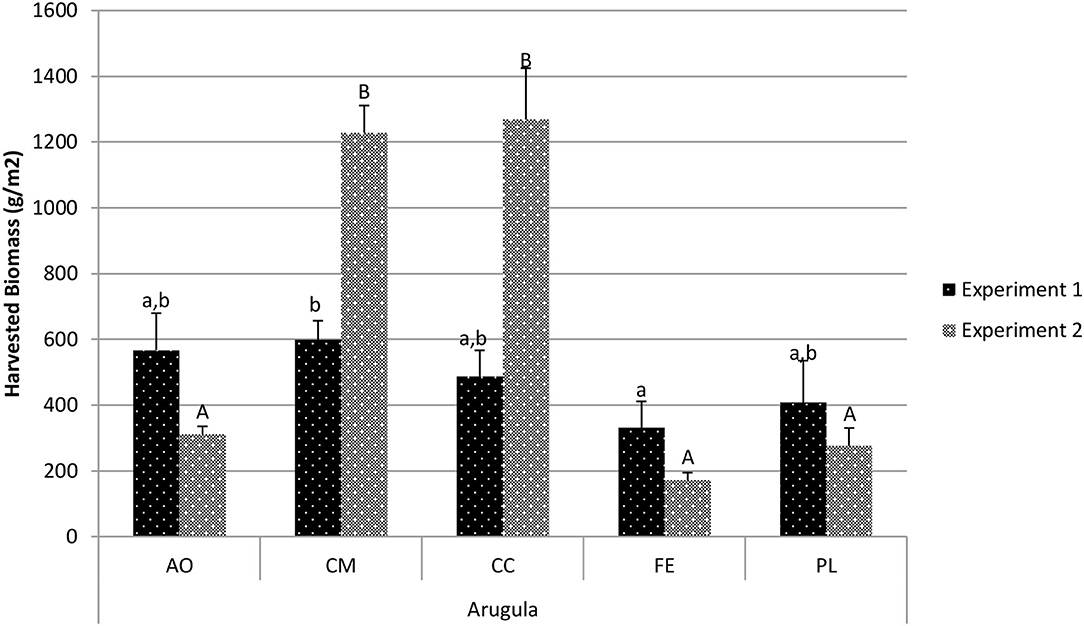
Figure 1. Comparison of arugula harvested biomass between treatments in experiment 1 and experiment 2. Data shown are mean ± standard deviation for two different replicate experiments. Significant differences among mean values are indicated with different letters above bars (p < 0.05) with lower-case letters for experiment 1 and capital letters for experiment 2. Results analyzed using ANOVA Tukey HSD for significance testing.
The harvested biomass for mizuna (Figure 2) in experiment 1 varied among treatments, and also varied among treatments in experiment 2. In experiment 1, the CM, CC, FE, and PL treatments all resulted in similar harvested weights, and biomass in the AO treatment was significantly greater than that in the other four treatments (1,719.6 g/m2; p < 0.001). In experiment 2, the biomass with the CM treatment yielded a higher harvest biomass than the other four treatments (2,087 g/m2; p < 0.01).
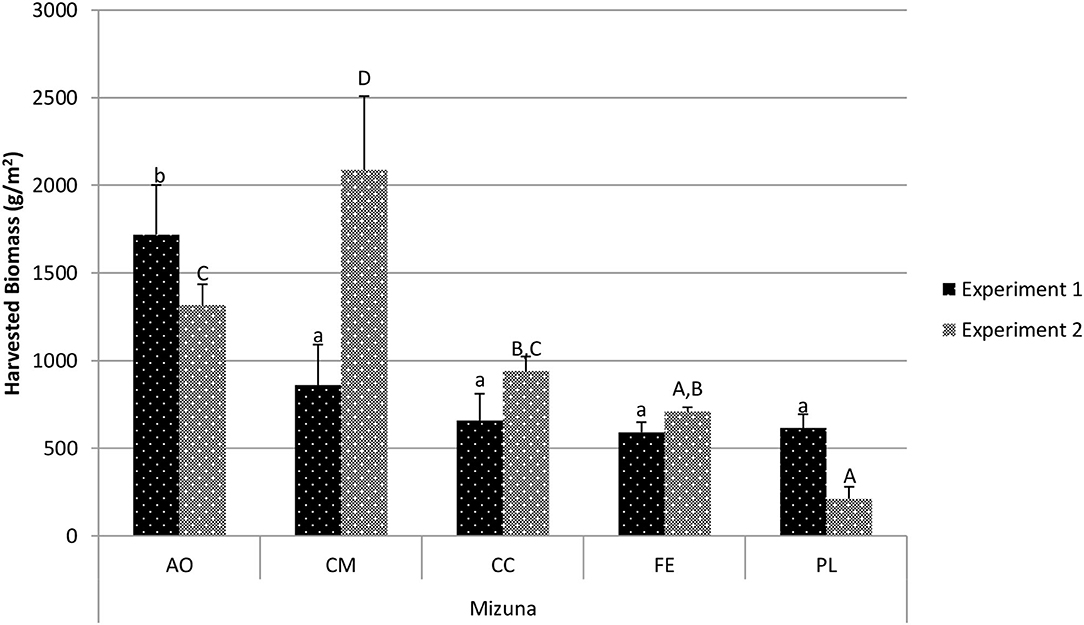
Figure 2. Comparison of mizuna harvested biomass between treatments in experiment 1 and experiment 2. Data shown are mean ± standard deviation for two different replicate experiments. Significant differences among mean values are indicated with different letters above bars (p < 0.05) with lower-case letters for experiment 1 and capital letters for experiment 2. Results analyzed using ANOVA Tukey HSD for significance testing.
The harvested biomass for red giant mustard (Figure 3) was highest in experiment 1 for the AO treatment (1,314.3 g/m2) and was significantly higher than all other treatments in experiment 1 (p < 0.002). Yield in the CC, FE, and PL treatments were all statistically similar to one another, while the CM treatment resulted in significantly lower yields than these three treatments (303.6 g/m2; p < 0.01). In experiment 2 the yield with the CM treatment had the highest harvested biomass (1,747.3 g/m2) and was statistically similar to the AO treatment (1,435.2 g/m2; p = 0.22) and the CC treatment (1,343.2 g/m2; p = 0.081). The PL treatment resulted in the lowest harvested biomass (205.0 g/m2) and was significantly lower than all other treatments (p < 0.001).
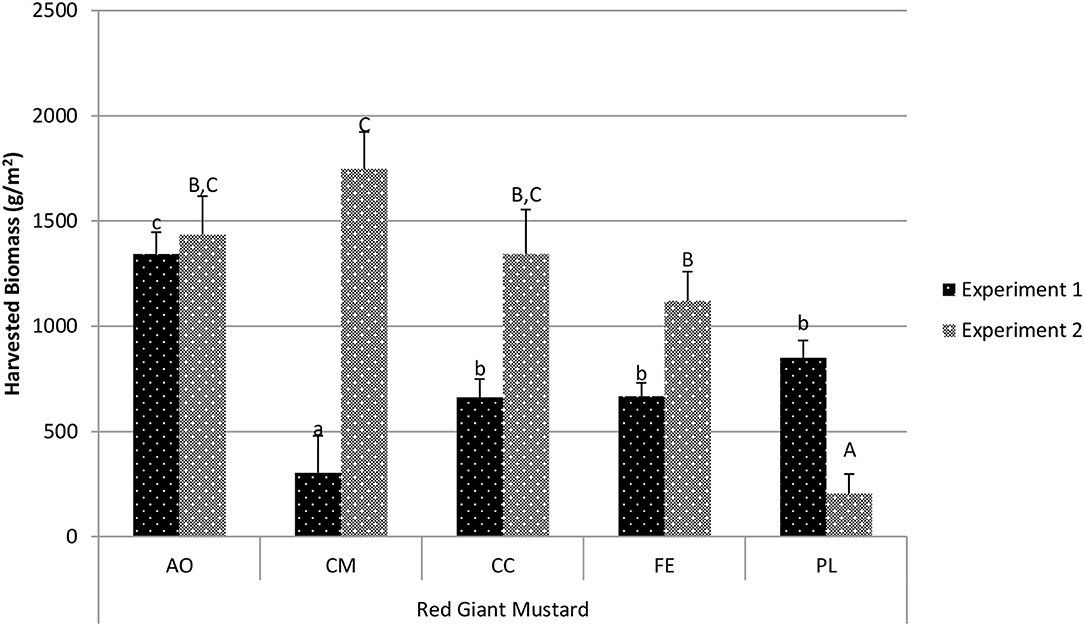
Figure 3. Comparison of red giant mustard harvested biomass between treatments in experiment 1 and experiment 2. Data shown are mean ± standard deviation for two different replicate experiments. Significant differences among mean values are indicated with different letters above bars (p < 0.05) with lower-case letters for experiment 1 and capital letters for experiment 2. Results analyzed using ANOVA Tukey HSD for significance testing.
Harvested spinach biomass for experiment 1 (Figure 4) differed among treatments. The AO treatment (1,161.5 g/m2) was similar to the CC treatment (834.6 g/m2; p = 0.392), but significantly higher than the CM, FE, and PL treatments (p < 0.05). The CM treatment had the lowest overall harvested biomass (418.6 g/m2), but was statistically similar to the CC, FE and PL treatments. In experiment 2, the AO resulted in the highest overall harvested biomass (1,494.1 g/m2) and was statistically similar to the CM treatment (1,195.8 g/m2; p = 0.376) and the CC treatment (996.4 g/m2; p = 0.058).
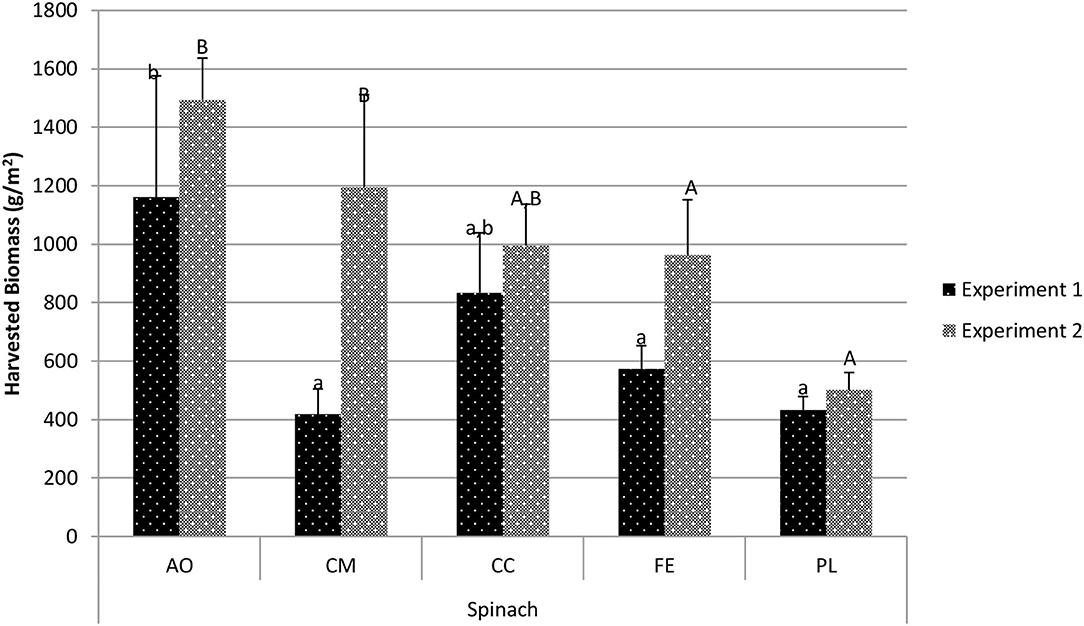
Figure 4. Comparison of spinach harvested biomass between treatments in experiment 1 and experiment 2. Data shown are mean ± standard deviation for two different replicate experiments. Significant differences among mean values are indicated with different letters above bars (p < 0.05) with lower-case letters for experiment 1 and capital letters for experiment 2. Results analyzed using ANOVA Tukey HSD for significance testing.
Minerals
In experiment 1, the mineral concentration in the harvested plants varied greatly among treatments and species (Table 3). For red giant mustard, there was no treatment difference for iron, potassium and sodium concentrations. For calcium, the CC resulted in the highest concentration (191.3 mg/100 g fw), which was statistically higher than the CM treatment (138.2 mg/100 g fw; p = 0.031), while the FE, AO and PL treatments were all similar. For the mizuna plants the potassium and sodium concentrations were most affected by treatment. For potassium, the CM treatment (860.3 mg/100 g fw) was significantly higher than the CC (393.1 mg/100 g fw), FE (120.16 mg/100 g fw), and PL (164.39 mg/100 g fw) treatments (p < 0.001). Sodium concentrations were also greatly affected by the treatments in the mizuna plants with nearly a 10-fold difference between the CM treatment (14.25 mg/100 g fw) and the FE treatment (142.66 mg/100 g fw; p < 0.001). Statistical relations between the collected soil and tissue mineral data in experiment 1 showed a significant correlation between potassium concentrations in the collected mizuna plants and soil at time of harvest (p = 0.028), and a positive correlation for mizuna and red giant mustard (soil mineral data only collected for experiment 1).
Vitamin C
Mizuna vitamin C was analyzed for all five fertility treatments in experiment 1 and for four fertility treatments for experiment 2. In experiment 1, the PL treatment resulted in the highest average tissue vitamin C concentration (16.1 mg/100 g fw), but was statistically similar to all other treatments, except for the CM treatment (p = 0.002; Figure 5). In experiment 2, the CC treatment resulted in the highest vitamin C concentration (48.13 mg/100 g fw) compared to all other treatments (p < 0.001; Figure 5). Tissue vitamin C concentration in plants grown in CM was higher than FE (16.86 mg/100 g fw; p = 0.018) and AO (16.03 mg/100 g fw; p = 0.008). Between experiments, fertility treatments resulted in a wide fluctuation in mizuna vitamin C concentrations, with all treatment averages lower in the first experiment. For the CC treatment, the average tissue vitamin C for experiment 1 was 11.94 mg/100 g fw whereas in experiment 2 it was 48.13 mg/100 g fw.
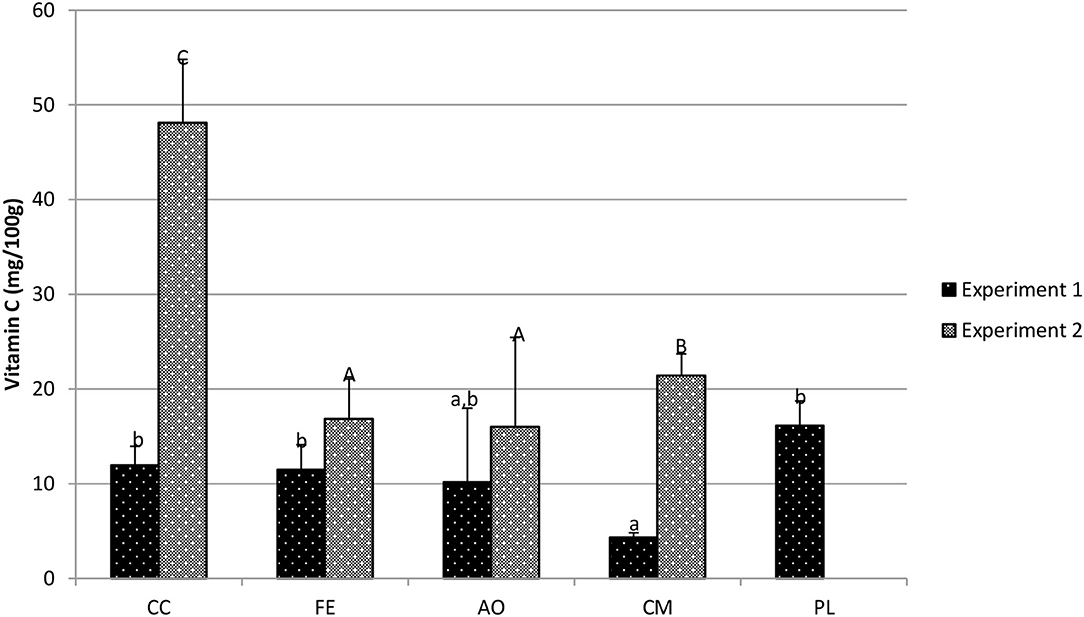
Figure 5. Mizuna vitamin c concentration (mg/100 g fresh weight) in harvested biomass—experiment 1 and experiment 2. Data shown are mean ± standard deviation for two different replicate experiments. Significant differences among mean values are indicated with different letters above bars (p < 0.05) with lower-case letters for experiment 1 and capital letters for experiment 2. Results analyzed using ANOVA Tukey HSD for significance testing.
In experiment 1, the red giant mustard PL treatment resulted in the highest mean vitamin C concentration (21.26 mg/100 g fw), but was statistically similar to the CC treatment (15.7 mg/100 g fw; p = 0.116) (Figure 6). The PL treatment resulted in significantly higher vitamin C concentrations than the FE treatment (14.03 mg/100 g fw; p = 0.049), AO treatment (12.48 mg/100 g fw; p = 0.022) and CM treatment (8.45 mg/100 g fw; p = 0.022). In experiment 2 (Figure 6), the CC treatment (32.4 mg/100 g fw) resulted in similar vitamin C concentrations to the FE treatment (27.53 mg/100 g fw; p = 0.369), the AO treatment (43.23 mg/100 g fw; p = 0.067) and CM treatment (23.36 mg/100 g fw; p = 0.115).
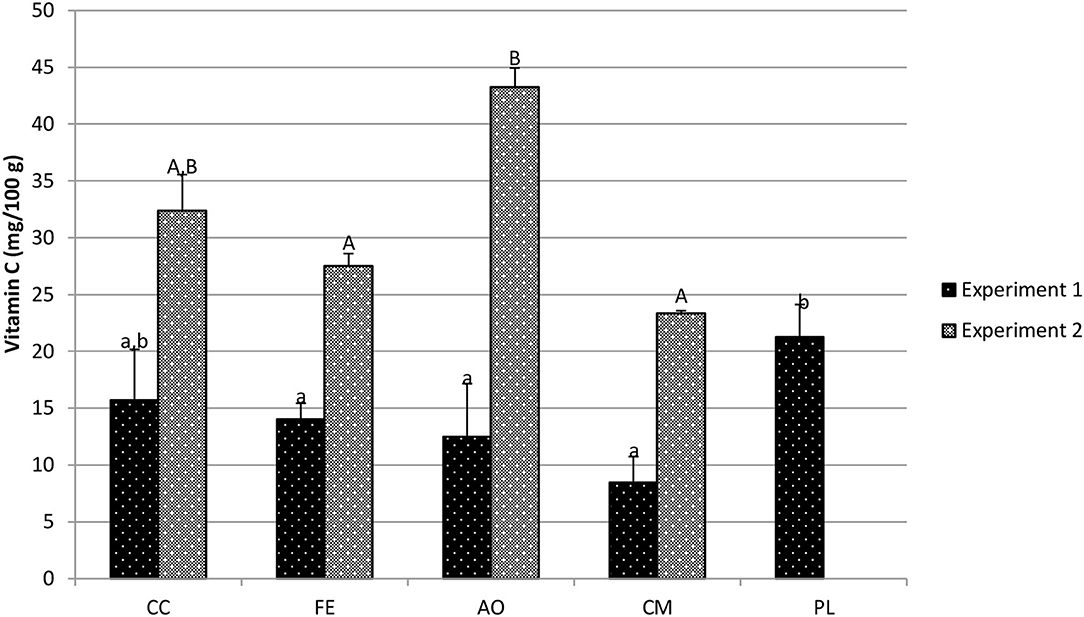
Figure 6. Red giant mustard vitamin c concentration (mg/100 g fresh weight) in harvested biomass—experiment 1 and experiment 2. Data shown are mean ± standard deviation for two different replicate experiments. Significant differences among mean values are indicated with different letters above bars (p < 0.05) with lower-case letters for experiment 1 and capital letters for experiment 2. Results analyzed using ANOVA Tukey HSD for significance testing.
Fiber
For mizuna plants in experiment 1 (Figure 7), the CC treatment (2.94 g/100 g fw) resulted in statistically similar concentrations of total dietary fiber compared to the PL treatment (3.24 g/100 g fw; p = 0.051) and also to the FE treatment (3.06 g/100 g fw; p = 0.367). Plants grown in both the CM treatment (2.04 g/100 g fw) and the AO treatment (1.82 g/100 g fw) had similar total dietary fiber concentrations, p < 0.001 and p < 0.001, respectively. In experiment 2, the CC treatment (4.30 g/100 g fw) resulted in significantly higher total dietary fiber than the CM treatment (2.47 g/100 g fw; p < 0.001) and lower than the AO treatment (3.77 g/100 g fw; p = 0.02) and the FE treatment (4.96 g/100 g fw; p = 0.007).
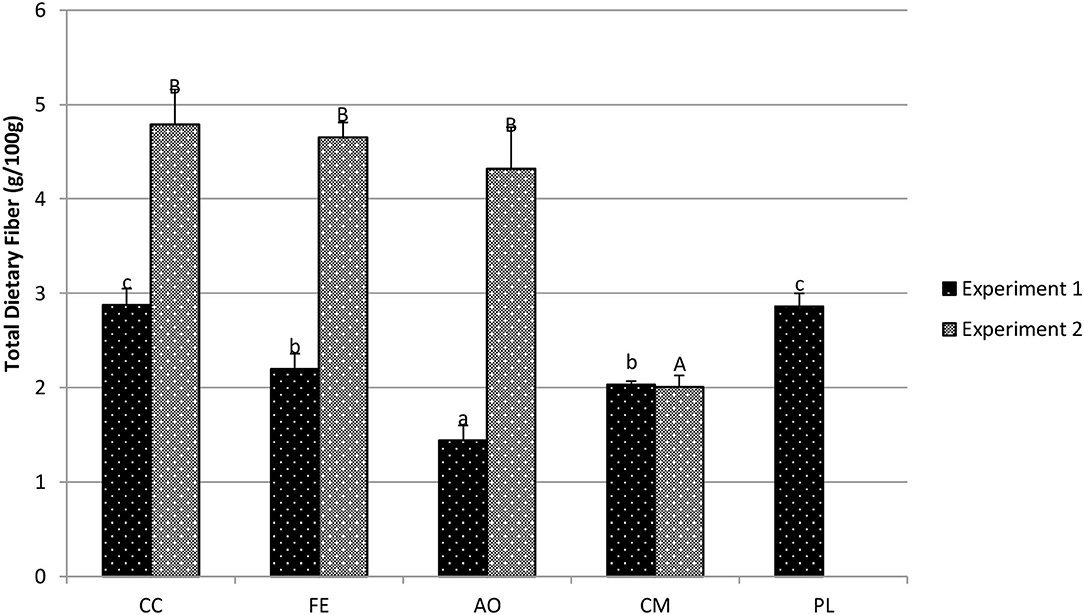
Figure 7. Total dietary fiber in mizuna (g/100 g fresh weight) determined with AOAC 991.43. Data shown are mean ± standard deviation for two different replicate experiments. Significant differences among mean values are indicated with different letters above bars (p < 0.05) with lower-case letters for experiment 1 and capital letters for experiment 2. Results analyzed using ANOVA Tukey HSD for significance testing.
For red giant mustard plants in experiment 1 (Figure 8), the CC treatment (2.88 g/100 g fw) resulted in statistically similar total dietary fiber concentrations compared to the PL treatment (2.86 g/100 g fw; p = 0.868). In contrast, the CM treatment (2.03 g/100 g fw; p < 0.001), the FE treatment (2.20 g/100 g fw; p < 0.001) and AO treatment (1.44 g/100 g fw; p < 0.001) produced plants with significantly less dietary fiber than the CC treatment. In experiment 2, total dietary fiber concentrations in plants grown in the CC treatment (4.79 g/100 g fw) were statistically similar to both the FE treatment (4.65 g/100 g fw; p = 0.588) and the AO treatment (4.32 g/100 g fw; p = 0.091), but were greater than the CM treatment (2.01 g/100 g fw; p < 0.001).
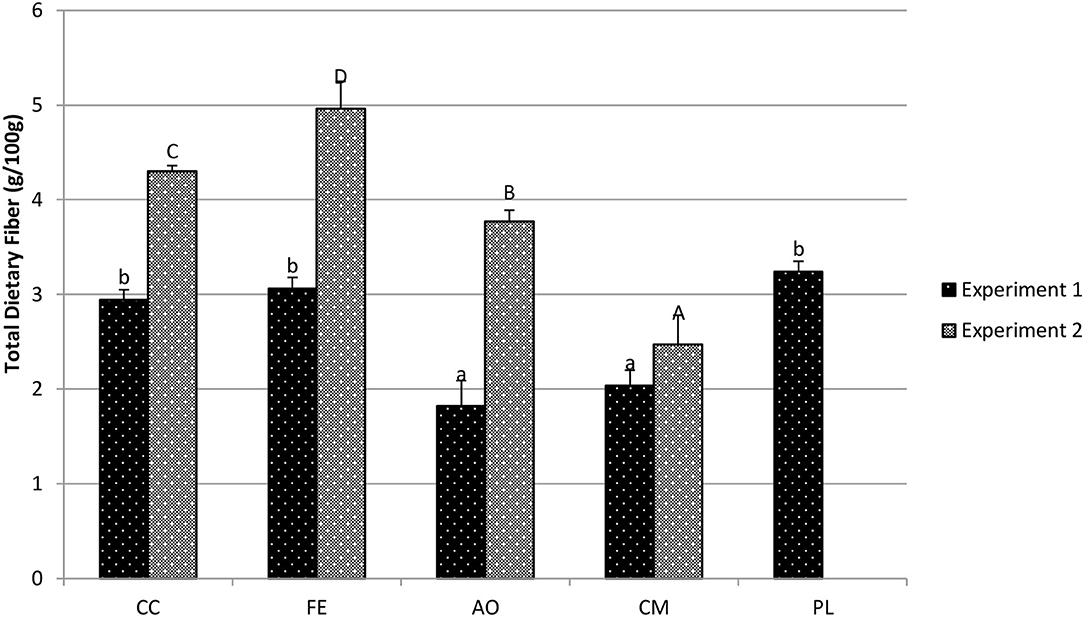
Figure 8. Total dietary fiber in red giant mustard (g/100 g fresh weight) determined with AOAC 991.43. Data shown are mean ± standard deviation for two different replicate experiments. Significant differences among mean values are indicated with different letters above bars (p < 0.05) with lower-case letters for experiment 1 and capital letters for experiment 2. Results analyzed using ANOVA Tukey HSD for significance testing.
Nitrate-N
Compared to the four other treatments, the plants grown in the CM treatment had significantly higher nitrate-N concentrations than all other treatments (p < 0.01; Table 4). For the arugula in experiment 1, tissue nitrate-N concentrations in the CM treatment [1,244 ppm (mg/kg fresh weight)] and the AO treatment (1,255 ppm) were significantly higher than in the other three treatments (p < 0.001). In the mizuna plants, tissue nitrate concentrations in the CM (1,443 ppm) and AO (1,656 ppm) treatments were significantly higher than the other treatments (p < 0.001). In experiment 2, arugula had the same nitrate-N concentration for the CC, FE, and PL treatments, while tissue nitrate-N in the CM treatment (734 ppm) was significantly higher than the rest (p < 0.001). For the spinach, mizuna and red giant mustard plants analyzed in experiment 2, the CM treatment resulted in the highest tissue nitrate-N concentration (p < 0.001). Statistical relations between the soil nitrate-N concentrations (taken at first harvest) and plant tissue nitrate-N concentrations showed a significant correlation (p < 0.05) for arugula, mizuna and red giant mustard plants analyzed from experiment 1 (Table 5).
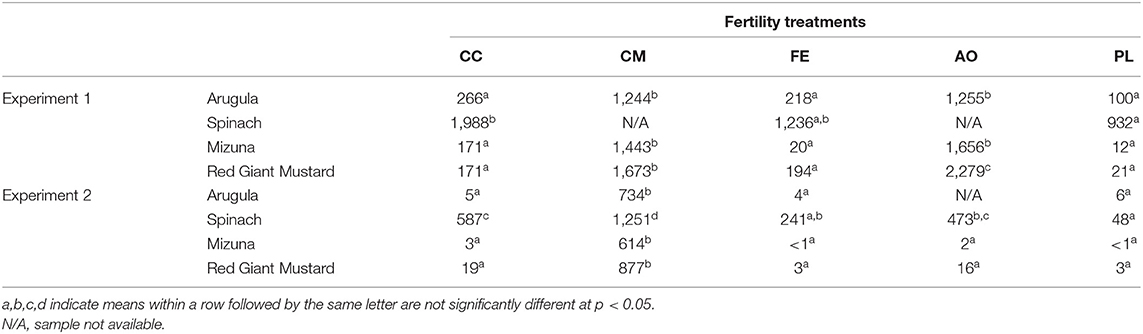
Table 4. Nitrate-N concentrations (NO3-N ppm; mg/kg fresh weight) in arugula, spinach, mizuna and red giant mustard for experiment 1 and experiment 2.
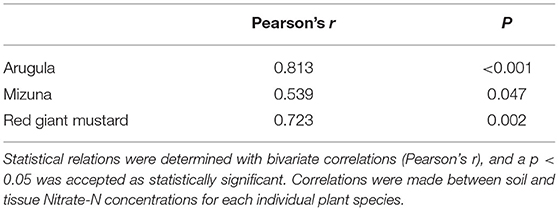
Table 5. Soil nitrate-N concentrations (NO3-N ppm; mg/kg dry weight) correlated to plant tissue nitrate-N concentrations (NO3-N ppm; mg/kg fresh weight) in arugula, mizuna and red giant mustard in experiment 1.
Comparison With Plants Grown in Commercial Greenhouses
Red giant mustard and mizuna plants were collected from three commercial greenhouses in Minnesota for shortfall nutrients and mineral comparison throughout experiment 1 and experiment 2 (Table 6). The red giant mustard vitamin C concentrations in experiment 2 nearly all fell within range of produce grown in commercial greenhouses, while for experiment 1 vitamin C was slightly lower than in the commercial greenhouses. Calcium levels in the commercial produce were higher than both experiment 1 and 2 for red giant mustard. For mizuna, experiment 1 had slightly lower vitamin C concentrations than the comparison produce, while experiment 2 had similar concentrations. Similar to red giant mustard, the mizuna plants also had much lower calcium concentrations in experiments 1 and 2 (128–251 mg/100 g) compared to the comparison mizuna (229–422 mg/100 g). The average concentration in the commercial comparison red giant mustard was 1,599 ppm, which was less than the CM and AO treatments in experiment 1, but greater than all other red giant mustard plants analyzed. The commercial comparison mizuna had an average concentration of 1,175 ppm, which was only less than the CM and AO treatments in experiment 1, and greater than all other mizuna plants analyzed, which was the same trend for both species of plants analyzed.
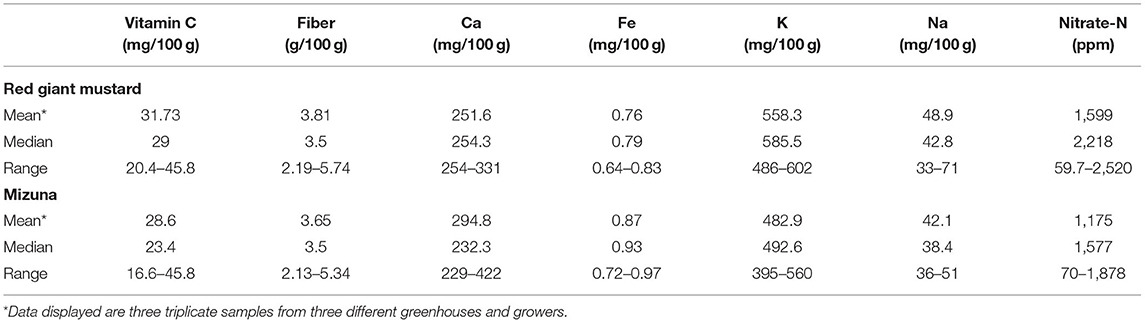
Table 6. Conventionally grown produce collected throughout both experiments from three Minnesota growers and greenhouses.
Discussion
Minerals
For the arugula, red giant mustard, mizuna and spinach plants evaluated in these experiments, significant differences in iron, potassium, calcium, sodium, vitamin C, and fiber between the fertility treatments were dependent on the experiment. Changes in any of these shortfall nutrients or nutrients of high interest depended on the season in which the plants were grown. Calcium concentrations were most likely higher in the CC treatment plants because the CC treatment had the second highest calcium concentration of all the media analyzed.
Under the FDA requirements for labeling, the minimum requirement of calcium in a product needed to make a “good source” nutrient content claim is 130 mg per 100 g serving (10% of 1,300 mg required by DV), nearly all of the plants under all the fertility treatments would qualify from both experiments. For potassium, a “good source” claim would require a minimum of 470 mg per 100 g serving. This claim could only be made for the CM and AO treatments (based on averages from both experiments) in the red giant mustard, mizuna and arugula plants analyzed.
The use of different fertility treatments affects potassium which is relevant as potassium is a nutrient of concern and now required on the Nutrition Facts panel. The FE treatment produced the highest average sodium concentrations in all plants analyzed in experiments 1 and 2 (with the exception of arugula in experiment 2). Dietary recommendations support lowering sodium intake, but the amount of sodium in the greens is universally low.
Few other studies have been published on the mineral content of vegetables grown by organic vs. conventional growing methods. Trace minerals were measured in the five most-consumed vegetables in the US, potato, lettuce, tomato, carrot, and onion for conventional vs. organic production (Hadayat et al., 2018). The vegetables were collected at grocery stores and not produced in a greenhouse or research plot. They reported little differences in content of trace minerals between conventional and organic vegetables. Most of the interest in mineral content of organic crops is based on lack of synthetic pesticides and mineral fertilizers in organic production.
Vitamins
Vitamin C is a highly unstable water-soluble vitamin known for its antioxidant properties, both in humans and plants. Key effects on vitamin C concentration include the growing conditions, plant stage at harvest, storage temperature and wide range of postharvest conditions (Phillips et al., 2018). Traditionally, accumulation of vitamin C is increased whenever plants are exposed to high oxidative stress, including full sunlight, low nitrogen availability in the soil and drought conditions (Kaack et al., 2001). For fresh produce, vitamin C losses can be enhanced when postharvest storage is extended, or at higher temperature, low relative humidity and freezing. High nitrogen fertilizers have also been associated with decreased vitamin C concentrations in many fruits and vegetables (Lee and Kader, 2000).
For all treatments in both experiments, for both mizuna and red giant mustard plants, plants harvested in experiment 2 had significantly higher vitamin C concentrations than in experiment 1 (p < 0.001). Plants in experiment 2 were grown in early spring, whereas plants grown in experiment 1 were grown during the winter months. Consequently, fluctuations in vitamin C concentrations between experiments were most likely due to changes in sunlight exposure. Among all the combined samples analyzed, the CC treatment had the highest vitamin C concentrations (p = 0.02) compared with the other treatments, and equal or higher calcium concentrations in those analyzed plants, although only in experiment 1.
Many pre-harvest factors (genotypic, climatic and environmental) influence vitamin C; thus, variation between experiments and growing seasons is common (Phillips et al., 2018).
Fiber
Dietary fiber is a mixture of complex organic substances that are non-digestible in the upper gastrointestinal tract, present as both soluble and insoluble compounds. Studies have analyzed dietary fiber differences between conventionally and organically grown plums, with little differences observed (Lombardi-Boccia et al., 2004). To our knowledge, dietary fiber has not been analyzed in nutrient dense crops that address shortfall nutrients, comparing either organic or conventional nutrient sources.
Under the 2016 FDA Nutrition and Supplements Facts Label Revisions, the new DV for dietary fiber is increasing to 28 g/d. Based on this new rule, 2.8 g fiber/serving will be required to make a “good source” nutrient content claim. Based on the combined averages from both experiments, only the mizuna and red giant mustard with the CC and FE treatments would qualify for this claim. Based on a single experiment, the mizuna and red giant mustard with the PL treatment would also qualify.
Nitrate-N
Nitrate-N concentrations varied greatly among treatments, species and experiments. Additionally, the significant correlation (p < 0.05) between plant tissue nitrate-N concentrations in arugula, mizuna and red giant mustard from experiment 1, and soil nitrate-N concentrations, showed that high nitrate-N in soil leads to high nitrate-N in harvested plant tissue.
Reviews of differences between organic and conventionally grown produce is that variables shared alike by organic and conventional produce during production, harvest, and postharvest handling and storage are not applied (Lester and Saftner, 2011). Therefore, many reviews find no evidence of a difference in nutrient quality between organically and conventionally produced foodstuffs (Dangour et al., 2009). More recent reviews and meta-analyses report that organic crops, on average, have higher concentrations of antioxidants and lower concentrations of pesticide residues than the non-organic comparators across regions and production seasons (Baranski et al., 2014). The authors note that a main challenge to interpreting comparisons of organic and inorganic food production systems is the high heterogeneity arising from combinations of crops, crop types, countries, and agronomic background conditions. In these analyses, heterogeneity is extremely high for most of the composition parameters for which significant differences are detected.
It has been estimated that 80% of the nitrate in people's diets comes from vegetable consumption (Karwowska and Kononiuk, 2020) as plants require bioavailable nitrogen to carry out photosynthesis for plant growth and development. Vegetables accumulate nitrates when the uptake of the nitrate ion exceeds its reduction and subsequent assimilation (Hakeem et al., 2017). This accumulation depends on numerous factors such as the composition of the soil where the plant is grown, the type of crop production, the type of fertilizer used, the season of the yar the crop is grown, and environmental conditions in which the crop is grown. Nitrate content also differs in plant species and varieties with generally leafy greens being concentrated in nitrates as they are good nitrate accumulators due to their rapid growth and that nitrates tend to accumulate in leaves and the leaves are what is consumed by consumers.
Very few differences were noted in nitrate concentrations of conventional and “organic” labeled vegetables taken from 5 U.S. metropolitan cities in a survey study sampling of retail outlets (Nunez de Gonzalez et al., 2015). However, they did report differences in nitrate content for some conventional and organic vegetables in different cities with the organic vegetables being lower in nitrate content. There are major research challenges comparing differences in nutrients and nitrates resulting from farming practices as studies have used different experimental designs and been carried out in different regions and growing seasons. An advantage of our research is that we studied vegetables known to be high in nutrients and nitrates, yet a disadvantage is that our studies were done in greenhouses, which allows us to control some variables, yet we did not control growing season, likely a critical variable in nitrate accumulation.
For nearly half of the produce analyzed in this study, an average consumer (68 kg) would exceed their recommended daily nitrate intake with only two servings (100 g/serving) of produce. The European Food Safety Authority (EFSA) and The World Health Association (WHO) recommend that daily nitrate intake be below 3.7 mg dietary nitrate/kg of body weight, as expressed by the Acceptable Daily Intake (ADI). When converted from nitrate to NO3-N as used in this study (multiplying by 0.226) this would be equal to a recommendation 50 mg, or less, of calculated NO3-N for a 60 kg individual). Vegetables are the number one source of nitrates in the diet for many populations, so monitoring intake is crucial to minimize the potentially harmful effects of high nitrate diets (EFSA, 2008; Hord et al., 2009). Careful considerations need to be made when applying nitrogen to both conventional and organic plants, as excessive nitrogen yields excessive amounts of nitrate accumulation in plant tissues. Both organic and conventional produce can have excessive amounts of nitrates, depending on growing and environmental conditions.
Conclusion
Organic vs. conventional growing practices in greenhouse grown leafy greens impacted amounts of Vitamin C and potassium levels in analyzed plant tissue. No consistent differences were found for fiber, calcium, iron and sodium concentrations in the tissue analyzed. Nitrate levels were generally higher in conventionally grown greens, although other factors including growing season were found to influence nitrate levels. Consumer demand for organic production of greens supports that organically grown leafy greens provide important short fall nutrients and a likely lower in nitrate than conventionally grown greens from greenhouses.
Limitations
Comparing nutrient differences in organic vs. conventionally grown crops is incredibly difficult. One limitation of such studies is that food grown in organic systems may consist of more dry matter than conventionally-grown food. If this is in fact the case, using dry weight would not accurately compare nutrient levels between the different production systems. Fresh weight may be a more accurate measure.
A second limitation relates to the study publication source. Guéguen and Pascal note that independent reviews have not been finding significant differences between organically grown and conventionally grown foods, whereas reviews conducted by organic agricultural organizations skew toward the positives of organic farming (Guéguen and Pascal, 2013). Organic agricultural production systems have benefits beyond nutrient levels or lower nitrate levels, yet it is difficult to systematically study these advantages in traditional research designs.
A third limitation is study design. This could encompass the general design of the study, incomplete reporting of study details, lack of statistical interpretation, or lack of control of growing conditions in retail-bought foods (Guéguen and Pascal, 2013). These issues make it difficult to interpret study outcomes in useful ways. Because our research was conducted in greenhouses, we had more control of growing conditions. Yet the most practical research for consumers is the nutrient and nitrate levels of the foods they purchase at the retail market. These studies are confounded as the organic and conventional foods they purchase come from different fields in different countries and the vegetables may have been in the market for days or weeks before being purchased and consumer.
Future Research
Different agricultural management systems may have an impact on the sustainability of food systems and affect human health, food security, and environmental sustainability (Schulz and Slavin, 2021). For different crops, the impact of organic production on nutrient values will vary greatly. Thus, it is not surprising that there is little clear nutritional advantage of consumption of organic foods when reviews of the literature are published.
Our study was an attempt to combine research teams in horticulture, soil science, food science, and nutrition to answer a practical question, are there differences in nutrient and nitrate content when we compare organic vs. conventional growing systems for leafy greens. The producers of leafy greens in deep winter greenhouses in Minnesota were the stakeholders that wanted practical advice on how to grow organic leafy greens in greenhouses and agreed to contribute their produce to determine if results from commercial operations would vary from a research greenhouse. The data on biomass of leafy green were based on the practical need of growers to be sure that changing growing conditions would not lower yield in their product.
Costs for nutrient analysis limit research in this field and we chose our nutrients to measure as ones that are under consumed by the US population and that have health benefits. Our results suggest that factors such as sunlight, other growing conditions, time of the year, are more important on content of Vitamin C than organic vs. conventional growing conditions.
Unlike essential nutrients like vitamins, minerals, and dietary fiber that are known to be beneficial for human health, other compounds like nitrate are associated with health risks. The largest amount of nitrates is accumulated in plants growing in a nitrate-rich environment and the most important sources of dietary intake of nitrate are vegetables and fruits (Karwowska and Kononiuk, 2020). Application of fertilizers, nitrate reductase activity, growth rate and growth conditions, including intensity of light, level of rainfall, significantly affect the nitrate content of vegetables. Leafy green vegetables have higher levels of nitrates compared to seeds or tubers. Lettuce and spinach have the highest nitrate content and the content of nitrates in vegetables is strongly influenced by seasonality and the cultivation system (Lucarini et al., 2012). They reported that lettuce biodynamically grown accumulated 1.3–2 times less nitrate than the respective organically grown plants. Our nitrate results likely show differences in time of season more than organic vs. conventional growing conditions. Other research supports that organic production of leafy green vegetables may lower the nitrate content of the vegetables compared to conventional production, if other variables such as seasonality and production systems are controlled.
Another systemic factor is the difference in nutrient and compound concentrations between a full, open-field organic system following all organic principles and practices, and a limited greenhouse system operating under fewer organic principles and practices. The key question is: how, and to what degree, do nutrient and compound concentrations differ between these two types of systems?
Future research must consider that reasons for adoption of organic growing conditions extend far beyond nutrition. Support for local food production and other societal variables must be considered when analyzing advantages to organic food production. And of course, the economics of organic food production are an overlay that should be considered in future research. If producers are guaranteed a higher price for their organic foods, then it makes sense to invest in organic growing systems on their farm. A fair economic system would ensure the producer a higher price for their product. And this would translate into higher prices for organic foods in the marketplace, which bring about issues of social justice and food security. Research in food systems requires teamwork across agriculture, the government, basic and social scientists across disciplines and of course economists.
In summary, this novel study investigated the effects of organic soil amendments upon nutrients and nitrate, in leafy greens. These soil amendments significantly affected vitamin C, nitrate, and potassium concentrations, although results support that other factors, including seasonality and sunlight were important factors determining the level of these nutrients in organic vs. conventional leafy greens. The treatments did not significantly affect fiber, calcium, iron, or sodium. The various treatment responses support the complexity of determining nutrient content of foods grown by organic and conventional growing practices and the reasons for the limited research data that organic food production is always a win for nutrient content.
Data Availability Statement
The raw data supporting the conclusions of this article will be made available by the authors, without undue reservation.
Author Contributions
JE was the Principal Investigator for the grant. JS and CR were the co-investigators. JG and MR supervised graduate students in plant sciences who worked on the project. JC was a graduate student in Food Science while LP was a graduate student in Horticulture. The study was designed by the PIs and all investigators were involved in conducting the study, sample analysis, and data entry. JC wrote the first draft of this publication and ES revised the manuscript as part of her MS in Nutrition at the University of Minnesota. All authors have reviewed, revised, and approved the manuscript.
Funding
Authors would like to thank the College of Food, Agriculture and Natural Resource Sciences MnDRIVE Global Food Ventures Program for funding for this project. JC was funded by the University of Minnesota Graduate School while working on the project.
Conflict of Interest
The authors declare that the research was conducted in the absence of any commercial or financial relationships that could be construed as a potential conflict of interest.
Publisher's Note
All claims expressed in this article are solely those of the authors and do not necessarily represent those of their affiliated organizations, or those of the publisher, the editors and the reviewers. Any product that may be evaluated in this article, or claim that may be made by its manufacturer, is not guaranteed or endorsed by the publisher.
Acknowledgments
Authors would like to thank MnDRIVE for support of research, the University of Minnesota Doctoral Dissertation Fellowship Program (JC), Esther Gesick for her greenhouse and study support, Julian Esparza for aid in sample collection and analysis and the greenhouses and growers who volunteered time, and samples to help with this project.
References
Baranski, M, Srednicka-Tober, D., Volakakis, N., Seal, C., Sanderson, R., Stewart, G.B., Benbrook, C., et al. (2014). Higher antioxidant and lower cadmium concentrations and lower incidence of pesticide residues in organically grown crops: a systematic literature review and meta-analysis. Br. J Nutr. 112, 794–811. doi: 10.1017/S0007114514001366
Dangour, A. D., Dodhia, S. K., Hayter, A., Allen, E., Lock, K., and Uauy, R. (2009). Nutritional quality or organic foods: a systematic review. Am. J. Clin. Nutr. 90, 680–685. doi: 10.3945/ajcn.2009.28041
Donaldson, D. (2021). Organic Market Summary and Trends. Available online at: https://www.ers.usda.gov/topics/natural-resources-environment/organic-agriculture/organic-market-summary-and-trends/ (accessed July 27, 2021).
EFSA (2008). Panel on contaminants in the food chain nitrate in vegetables—scientific opinion of the panel on contaminants in the food chain. EFSA J. 689, 1–79. doi: 10.2903/j.efsa.2008.689
Funk, C., and Kennedy, B. (2016). Americans' Views About and Consumption of Organic Foods. Available online at: https://www.pewresearch.org/science/2016/12/01/americans-views-about-andconsumption-of-organic-foods/ (accessed July 17, 2021).
Grafton, K.B., Joern, A., Mallarino, D., Mengel, J., Dahl, D., Kaiser, D., et al. (2015). Recommended Chemical Soil Test Procedures for the North Central Region (Publication No. 221). Available online at: https://extension.missouri.edu/media/wysiwyg/Extensiondata/Pub/pdf/specialb/sb1001.pdf (accessed June 30, 2017).
Guéguen, L., and Pascal, G. (2013). “Organic foods,” in Encyclopedia of Human Nutrition Vol 3, 3rd Edn. editor. B. Caballero (Amsterdam: Academic Press), 413–416.
Hadayat, N., De Oliveira, L.M., Da Silva, E., Han, L., Hussain, M., Liu, X., et al. (2018). Assessment of trace metals in five most-consumed vegetables in the US: conventional vs. organic. Envirn. Poll. 243, 292–300. doi: 10.1016/j.envpol.2018.08.065
Hakeem, K. R., Sabir, M., Ozturk, M., Akhtar, M. S., and Ibrahim, F.H. (2017). Nitrate and nitrogen oxides: sources, health effects and their remediation. Rev. Environ. Contam. Toxicol. 242, 183–217. doi: 10.1007/398_2016_11
Hamdard, J., Nagar, H., Gairola, S., Umar, S., and Suryapaini, S. (2009). Nitrate accumulation, growth and leaf quality of Spinach beet (Beta vulgaris Linn) as affected by NPK fertilization with special reference to potassium. Indian J. Sci. Technol. 2, 35–40. doi: 10.17485/ijst/2009/v2i2.2
Hord, N. G., Tang, Y., and Bryan, N. S. (2009). Food sources of nitrates and nitrites: the physiologic context for potential health benefits. Am. J. Clin. Nutr. 90, 1–10. doi: 10.3945/ajcn.2008.27131
Hunter, D., Foster, M., McArthur, J. O., Ojha, R., Petocy, P., and Samman, S. (2011). Evaluation of the micronutrient composition of plant foods produced by organic and conventional agricultural methods. Cr. Rev Food Sci. Nutr. 51, 571–582. doi: 10.1080/10408391003721701
IFOAM-Organics International. (2020). The Principle of Fairness. Available online at: https://www.ifoam.bio/why-organic/principles-organic-agriculture/principle-fairness (accessed July 4, 2021).
Kaack, K., Nielsen, M., Christensen, L. P., and Thorup-Kristensen, K. (2001). Nutritionally important chemical constituents and yield of carrot (Daucus carota L.) roots grown organically using ten levels of green manure. Acta Agric. Scand. Sect. B-Plant Soil Sci. 51, 125–136.
Karnpanit, W., Benjapong, W., Srianujata, S., Rojroongwasinkul, N., Tanaviyutpakdee, P., Sakolkittinapakul, J., et al. (2018). Cultivation practice on nitrate, lead and cadmium contents of vegetables and potential health risks in children. Int. J. Veg Sci. 25, 512–528. doi: 10.1080/19315260.2018.1541952
Karwowska, M., and Kononiuk, A. (2020). Nitrates/nitrites in food – risk for nitrosative stress and benefits. Antioxidants 9, 241. doi: 10.3390/antiox9030241
Lee, S. K., and Kader, A. A. (2000). Preharvest and postharvest factors influencing vitamin C content of horticultural crops. Postharvest Biol. Technol. 20, 207–220.
Leon, V. M., and Luzardo, O. P. (2020). Evaluation of nitrate contents in regulated and non-regulated leafy vegetables of high consumption in the Canarry Islands, Spain: risk assessment. Food Chem. Toxicol. 146, 111812. doi: 10.1016/j.fct.2020.111812
Lester, G. E., and Saftner, R. A. (2011). Organically versus conventionally grown produce: common production inputs, nutritional quality, and nitrogen delivery between the ttwo systems. J. Agric. Food Chem. 59, 10401–10406. doi: 10.1021/jf202385x
Lombardi-Boccia, G., Lucarini, M., Lanzi, S., Aguzzi, A., and Cappelloni, M. (2004). Nutrients and antioxidant molecules in yellow plums (Prunus domestica L.) from conventional and organic productions: a comparative study. J. Agric. Food Chem. 52, 90–94.
Lucarini, M., D'Evoli, L., Tufi, S., Garielli, P., Paoletti, S., Di Ferdinando, S., et al. (2012). Influence of growing system on nitrate accumulation in two varieties of lettuce and red radicchio of treviso. J. Sci. Food Agric. 92, 2796–2799. doi: 10.1002/jsfa.5526
Lucier, G., Allshouse, J., and Lin, B. (2004). Factors Affecting Spinach Consumption in the United States. Available online at: https://www.ers.usda.gov/publications/pub-details/?pubid=39496 (accessed June 30, 2017).
Mie, A., Andersen, H.R., Gunnarsson, S., Kahl, J., Kesse-Guyot, E., Rambialkowska, E., et al. (2017). Human health implications of organic food and organic agriculture: a comprehensive review. Enciron. Health 16, 111. doi: 10.1186/s12940-017-0315-4
Mir, S. A., Shah, M. A., and Mir, M. M. (2017). Microgreens: production, shelf life, and bioactive components. Crit. Rev. Food Sci. Nutr. 57, 2730–2736. doi: 10.1080/10408398.2016.1144557
Nunez de Gonzalez, M. T., Osburn, W. N., Hardin, M. D., Longnecker, M., Garg, H. K., Bryan, N. S., and Keeton, J. T. (2015). A survey of nitrate and nitrite concentrations in conventional and organic-labeled raw vegetables at retail. J. Food Sci. 80, C942–9. doi: 10.1111/1750-3841.12858
Phillips, K. M., Tarrago-Trani, M. T., McGinty, R. C., Rasor, A. S., Haytowitz, D. B., and Pehrsson, P. R. (2018). Seasonal variability of the vitamin C content of fresh fruits and vegetables in a local retail market. J. Sci. Food Agric. 98, 4191–4204. doi: 10.1002/jsfa.8941
Rosen, C. J., and Eliason, R. (2005). Nutrient Management for Commercial Fruit and Vegetable Crops in Minnesota. Available online at: https://conservancy.umn.edu/bitstream/handle/11299/197955/nutrient-management-commercial-fruit-vegetable-2005.pdf?sequence=1andisAllowed=y (accessed November 20, 2021).
Schulz, R., and Slavin, J. (2021). Perspective: defining carbohydrate quality for human health and environmental sustainability. Adv. Nutr. 12, 1106–1121. doi: 10.1093/advances/nmab050
Slavin, J. (2013). Fiber and prebiotics: mechanisms and health benefits. Nutrients. 5, 1417–1435. doi: 10.3390/nu5041417
Slavin, J., and Lloyd, B. (2012). Health benefits of fruits and vegetables. Adv. Nutr. 3, 506–516. doi: 10.3945/an.112.002154
Soetan, K. O., Olaiya, C. O., and Oyewole, O. E. (2010). The importance of mineral elements for humans, domestic animals and plants: a review. African J. Food Sci. 4, 200–222. doi: 10.5897/AJFS.9000287
Keywords: organic, nitrate, vitamin C, dietary fiber, minerals, leafy greens
Citation: Swanson EO, Carlson JL, Perkus LA, Grossman J, Rogers MA, Erwin JE, Slavin JL and Rosen CJ (2022) Nutrient and Nitrate Composition of Greenhouse-Grown Leafy Greens: A Trial Comparison Between Conventional and Organic Fertility Treatments. Front. Sustain. Food Syst. 6:811995. doi: 10.3389/fsufs.2022.811995
Received: 09 November 2021; Accepted: 16 February 2022;
Published: 30 March 2022.
Edited by:
Alexandru Rusu, Biozoon Food Innovations GmbH, GermanyReviewed by:
Mareli Telaumbanua, Lampung University, IndonesiaMaria Ewa Rembialkowska, Warsaw University of Life Sciences, Poland
Copyright © 2022 Swanson, Carlson, Perkus, Grossman, Rogers, Erwin, Slavin and Rosen. This is an open-access article distributed under the terms of the Creative Commons Attribution License (CC BY). The use, distribution or reproduction in other forums is permitted, provided the original author(s) and the copyright owner(s) are credited and that the original publication in this journal is cited, in accordance with accepted academic practice. No use, distribution or reproduction is permitted which does not comply with these terms.
*Correspondence: Joanne L. Slavin, anNsYXZpbkB1bW4uZWR1