- 1Teagasc Food Research Centre, Dublin, Ireland
- 2Herd Health Unit, School of Veterinary Medicine, University College Dublin, Dublin, Ireland
- 3Microbial Ecology Laboratory, School of Natural Sciences, National University of Ireland, Galway, Ireland
- 4Teagasc Environmental Research Centre, Wexford, Ireland
This study investigated the survival of Salmonella Senftenberg, Escherichia coli O157:H7, Listeria monocytogenes, Enterococcus faecalis and Clostridium sporogenes in sandy and clay loam textured soils when applied in bovine slurry or unpasteurised digestate, using laboratory based inoculation studies. The run-off rate for a test bacterium, Listeria innocua, when applied to grass in slurry and digestate, was also examined using field studies. Bovine slurry and digestate were inoculated with the target bacteria to a final concentration of 106 log10 cfu/g or spores/g, thoroughly mixed into soil samples and incubated at 4°C or 14°C. Samples were removed periodically and the surviving cells enumerated using AOAC or equivalent methods. The loss of viability/culturability phase followed first order kinetics and T90 values ranged from 11.9 to 166.7 d at 4°C and from 6.0 to 156 d at 14°C. With the exception of E. coli O157:H7 and E. faecalis in sandy loam textured soil at 14°C (T90 values were significantly (P < 0.05) higher in slurry) the type of soil texture or application material (slurry or digestate) did not affect survival rates. In the field study, 12 grass covered micro-plots were prepared. L. innocua was applied in digestate and bovine slurry and rainfall was simulated at a target rate of ~11 mm per plot per h−1. Rainfall simulation (30 min) took place after 24, 48 h, 14 d and 30 d. Run-off samples were tested for the L. innocua strain using Brilliance Listeria agar supplemented with streptomycin sulphate (1,000 μg/ml) at 37°C for 48 h, as were soil samples after 30, 58, 86 and 112 d. Significantly (P < 0.05) lower counts were obtained in the run-off from digestate after 1, 2 and 30 d as compared to slurry. It was concluded that the type of organic fertiliser does not affect the bacterial survival rates in sandy and clay soils, with the exception of E. coli O157:H7 and E. faecalis in sandy loam textured soil at 14°C. Furthermore, bacteria may be retained better in the soil-digestate matrices during rainfall although additional research is required to further validate and provide the scientific basis for this observation.
Introduction
Anaerobic digestion (AD) is an efficient and environmentally sustainable method for processing large amounts of farm, food and wastewater treatment materials (Nolan et al., 2018). Increasing the application of AD is a key objective of the European Green Deal, the blueprint for turning the EU into the first climate neutral continent by 2050 (European Commission, 2020). Co-digestion of combined wastes produces biogas (methane) and digestate, a nutrient rich organic material frequently used as a fertiliser (Alkanok et al., 2014). In addition to contributing to international renewable energy targets, land spreading digestate recycles nutrients from biowaste back into food production, a critical activity in sustainable farming (Johansson et al., 2005). Historically, bovine slurry, which makes up ~80% of the 1.6 billion tonne of animal waste produced in Europe every year (Foged et al., 2011), has been used as the main organic fertiliser on farmland. However, as the use of AD has increased, so too has the application of digestate.
Both slurry and digestate enhance soil health while providing organic matter and essential plant micronutrients (Nikoli and Matsi, 2011; Larkin, 2015; Bhattacharya et al., 2016; Slepetiene et al., 2020). Slurry is often contaminated with a range of bacterial, viral and parasitic pathogens including Salmonella spp., Escherichia coli O157:H7, Listeria monocytogenes, Streptococcus pyogenes, enteropathogenic E. coli (EPEC), Clostridium spp., Campylobacter spp. Mycobacterium spp., Cryptosporidium parvum, Giardia sp., swine Hepatitis E, and norovirus (Alam and Zurek, 2006; Ferens and Hovde, 2011; Russell et al., 2020). Digestate may also contain pathogens from contaminated feedstock materials (Russell et al., 2020), that survive the AD process (Olsen and Larsen, 1987; Sahlström et al., 2004; Bagge et al., 2005; Goberna et al., 2009). Post-reactor contamination from the environment, insect and animal vectors, may also occur during storage (Sidhu et al., 2001; Bagge et al., 2005; Zaleski et al., 2005; Pepper et al., 2006). The absence of unpleasant odours, reduced methane emissions, decreased biochemical oxygen demand and a more balanced nutrient mix and bioavailability make digestate more suitable for land spreading than slurry. However, digestate has the added risks of introducing pathogens such as E. coli O157, Salmonella, Listeria, Campylobacter, Cryptosporidium, Ascaris, Mycobacterium avium subspecies paratuberculosis, and Giardia' not already present on the farm receiving the digestate and/or disseminating pathogens from a single source to multiple farms (Nolan et al., 2018).
Current European Regulations (EC) 1774/2002 and 1069/2009 permit the application of animal wastes and digestate as organic fertilisers and soil improvers and set limits for the pathogen loads permitted, eg. digestate must have faecal indicator bacteria (total coliforms, E. coli, and Enterococcus spp.) <1,000 cfu g−1 to be permitted for landspreading, while highlighting the requirement for further research on the risks associated with pathogens in slurry and digestate when applied to agricultural land. Crops contaminated with enteric pathogens from organic fertilisers, spread via water and soil have been a source of human illness (Warriner et al., 2009; Castro-Ibáñez et al., 2015). Indeed, in recent years fresh produce has surpassed meat as the leading source of foodborne illness outbreaks (Herman et al., 2015). Waste materials, temporarily mobilised during rainfall events may spread to adjacent fields or rivers, further disseminating pathogens like Salmonella spp. and E. coli O157 (Ongeng et al., 2015; Peyton et al., 2016; Clagnan et al., 2019). However, the opportunity to cross-contaminate crops is dependent on the survival properties of the bacteria and the environmental conditions. It is generally accepted that soil is a hostile environment where enteric bacteria die-off as a result of exposure to stresses such as suboptimal temperatures, low water availability, metal toxicity (especially copper and zinc), nutrient deprivation, exposure to metabolites from soil microorganisms and UV combined with predatory protozoa (Artz and Killham, 2002; Coohill and Sagripanti, 2009; Ongeng et al., 2015). Thus, soils are a dynamic environment with multiple and interacting factors influencing survival, which may also be affected by the application material (slurry or digestate) (Ongeng et al., 2015).
Previous research has investigated the survival of bacteria in soil amended with animal slurry including Salmonella in soils amended with pig manure (Holley et al., 2006) and bovine slurry (Nicholson et al., 2005) as well as E. coli O157:H7 and Enterococci in bovine faeces spread on grassland (Bolton et al., 1999; Byappanahalli and Fujioka, 2004). However, there is a dearth of similar studies for digestate. Moreover, to the best of our knowledge, studies that compare bacterial survival in soils amended with slurry vs. digestate are limited. Survival studies are therefore required to determine the survival of key pathogens in slurry and digestate amended soils. Laboratory studies, where variables other than the application material can be controlled, are particularly useful for this but may not be representative of the dynamic nature of the natural environment especially the impact of rainfall on the physical removal of bacteria (Ongeng et al., 2015), whereas field-based trials are more representative (Wang et al., 2018). Moreover, such studies often used a rainfall simulator to examine bacterial run-off from soil under field conditions (Brennan et al., 2012; Healy et al., 2017).
The primary objective of this study was to investigate bacterial pathogen survival in amended soil when either slurry or digestate was applied to the two most common soil types in Ireland using laboratory trials. A second study was also undertaken using rainfall simulation in a field trial that investigated the run-off rate of Listeria innocua when applied in slurry and digestate.
Materials and Methods
Laboratory-Scale Soil Survival Study
Soil Preparation
A sandy loam (52% sand, 30% silt, 18% clay) and a clay loam (44% sand, 36% silt, 20% clay) soil (Brennan et al., 2012) were extracted from a permanent grassland site in Teagasc Johnstown Castle, Co. Wexford, Ireland. Pebbles and small stones were removed by hand prior to portioning into samples. In total 40 boxes (GenBox Jar, 2.5L; Biomeriéux, Basingstoke, UK) were labelled, 20 as “sandy loam” and 20 as “clay loam”, and 450 g of the appropriate soil sample was weighed into each. These boxes were sealed to prevent moisture loss and wrapped in aluminium foil to exclude light.
Inoculum Preparation
Marked strains (streptomycin resistance 1,000 μg/ml) of Salmonella Senftenberg and Listeria. monocytogenes, and reference strains of E. coli O157 (EDL 933), Enterococcus faecalis (NCTC 12697) and Clostridium sporogenes (DSM 767), used to represent proteolytic C. botulinum were obtained from the Teagasc culture collection held in the Teagasc Food Research Centre (Ashotwn, Dublin, Ireland). To prepare inocula for all target bacteria, except C. sporogenes, a cryoprotective bead from frozen (−80°C) storage was streaked on Tryptone Soya Agar (TSA; Oxoid, Fannin Ltd., Ireland) and incubated at 37°C for 24 h. A single colony from the resultant culture was aseptically mixed into 10 ml of Tryptone Soya Broth (TSB; Oxoid, Fannin Ltd., Ireland) and incubated overnight at 37°C. The culture obtained was centrifuged at 8,014 × g (Centrifuge 5801 R, Eppendorf, Cambridge, UK) for 10 min, washed and resuspended in phosphate buffered saline (PBS; Oxoid, Fannin Ltd., Ireland) three times, before resuspension in PBS and serially diluted to obtain cell concentrations of ~107 cfu/ml.
Dried C. sporogenes cultures obtained from DSMZ were rehydrated, following the instructions provided, in an anaerobic environment. C. sporogenes spores were prepared by first adding 100 μl of rehydrated culture to 20 tubes of cooked meat medium (CMM; Oxoid, Fannin Ltd., Ireland) broth (20 ml) and incubating in an anaerobic workstation (Don Whitley, United Kingdom) for 12–18 h at 37°C. Clostridium sporulation agar was prepared as described by Casadei et al. (2000) and placed in a Whitley A35 anaerobic chamber (Don Whitley Scientific, West Yorkshire, UK) overnight using the ANO2 gas mixture (10% H2, 10% CO2, 80% N2; Air Products Ireland, Dublin, Ireland) to exclude all oxygen. Aliquots (300 μl) of the overnight CMM broth were then spread onto 300 plates of Clostridium Sporulation Agar (CSA) (Casadei et al., 2000) (inside the anaerobic chamber) before transfer to anaerobic boxes (GenBOX jars; BioMérieux UK Ltd., Basingstoke UK) containing Anaerogen sachets (Oxoid, Fannin Ltd., Ireland) and incubated at 37°C for 12 days. Spores were harvested by adding ~5 ml ice-cold sterile distilled water onto the surface of the CSA plates and agitating the surface of the agar with a sterile spreader to release spores from the agar. The suspension was then transferred to the next agar plate and the process repeated. The suspensions were then pooled in 50 ml tubes, centrifuged at 7,500 x g at 4°C for 10 min and washed with iced water, reducing the amount of liquid over the course of repeated cycles until a spore suspension of ~107 spores/ml (estimated by phase contrast microscopic examination) was obtained. The spore suspension was kept on ice throughout the entire harvesting process. This concentration was then confirmed by plating out on Columbia Blood Agar (CBA; Oxoid, Fannin Ltd., Ireland) with 5% defibrinated horse blood (Cruinn diagnostics, Ireland) in anaerobic conditions as described above. The spore preparations (1 ml aliquots) were stored at −80°C. Prior to inoculation, spore preparations were thawed at room temperature and heat treated at 80°C for 10 min to ensure the destruction of any vegetative cells.
Soil Inoculation and Storage
Fresh bovine slurry was obtained from a beef farm in County Meath, Ireland, while digestate was obtained from a commercial AD plant in the east of Ireland that operates a mesophilic, industrial scale continuous system, using mainly food waste and slurry as feedstock materials. Twenty slurry samples (45 g) and twenty digestate samples (45 g) were prepared. For each bacterium of interest, four digestate and four slurry samples were inoculated in 5 ml PBS to give a concentration of 106 log10 cfu/g or spores/g. These samples were then thoroughly mixed manually using a sterile metal spoon into the previously prepared soil samples (450 g) to obtain four of each of the following combinations for each target bacterium: digestate plus sandy loam, digestate plus clay loam, slurry plus sandy loam, and slurry plus clay loam. The boxes were sealed and 2 of each combination incubated at 4 or 14°C (mean winter and summer temperatures respectively in Ireland) and sampled at times (t) = 0, 12, 26, 40, 54, 68, 82, 96, 110 and 124 d. Immediately prior to sampling the contents were thoroughly mixed again and 10 g removed.
pH and Water Activity
The pH and water activity (aw) of the soil samples was recorded at each sampling time-point. The pH was tested using a Eutech pH 150 probe (Thermo Scientific, USA) which was calibrated using pH 4, 7 and 10 standards prior to use. The water activity was measured using an Aqualab Pre water activity metre (Labcell). The metre was calibrated prior to use using a saturated solution of sodium chloride (KCl, aw = 0.984 ± 0.003 at 20°C).
Microbiological Analysis
Direct Counts
A 10 g soil sample was added to 90 ml of maximum recovery diluent (MRD, Oxoid, Fannin Ltd, Ireland) and stomached (Colworth Stomacher 400) for 60 s. A serial dilution was prepared in MRD and the target bacteria were enumerated in duplicate using selective agar as follows: (1) Xylose Lysine Desoxycholate agar (XLD: Oxoid, Fannin Ltd., Ireland) supplemented with streptomycin sulphate (1,000 μg/ml; Sigma Aldrich Ireland Ltd., Wicklow, Ireland) incubated at 37°C for 24 h for S. Senftenberg; (2) Sorbital MacConkey Agar supplemented with cefixime-tellurite (CT-SMAC: Oxoid, Fannin Ltd., Ireland) at 37°C for 24 h for E. coli O157:H7; (3) Brilliance Listeria Agar (OCLA; Oxoid, Fannin Ltd., Ireland) with streptomycin sulphate (1,000 μg/ml) incubated at 37°C for 48 h for L. monocytogenes; (4) Slanetz and Bartley Agar (Oxoid, Fannin Ltd., Ireland) incubated at 37°C for 24 h, followed by 42°C for a further 24 h to enumerate E. faecalis; and (5) Reinforced Clostridial Agar (RCA; Oxoid, Fannin Ltd., Ireland) incubated anaerobically, using Anaerogen sachets in anaerobic jars at 37°C for 48 h for C. sporogenes. As RCA is not sufficiently selective, presumptive C. sporogenes colonies were confirmed using real-time PCR, as described by Morandi et al. (2015).
Enrichment
Enrichment procedures were used to detect target bacteria when the residual concentration was below the limit of detection using direct plating methods. Enrichment cultures of S. Senftenberg were prepared by adding 10 g of the soil sample to 90 ml buffered peptone water (BPW) and incubating at 37°C for 24 h before plating on Modified Semi-Solid Rappaport Vassiliadis medium (MSRV; Oxoid, Fanning Ltd., Ireland) with novobiocin supplement (20 mg/l, Oxoid, Fannin Ltd., Ireland) and incubating at 42°C for 24 h. Presumptive Salmonella colonies which exhibited haloed growth were streaked onto Xylose Lysine Deoxycholate agar (XLD, Oxoid, Fannin Ltd, Ireland) and incubated at 37°C for 24 h (Hutchinson et al., 2004).
For E. coli O157, a 10 g soil sample was added to 90 ml of modified Tryptone Soya Broth (mTSB; Oxoid, Fanning Ltd., Ireland) with cefixime (50 μg/l) and vancomycin (6 mg/l), stomached for 60 s and incubated at 37°C for 24 h. Immunomagnetic separation (IMS, Dynal®BeadRetriever, Thermo Fisher Scientific, Ireland) was undertaken using Dynabeads™ anti-E. coli O157 (Thermo Fisher Scientific, Ireland) and recovered cells were plated on CT-SMAC, incubated at 37°C for 24 h. Suspect colonies were streaked onto both Eosin Methyl Blue agar (EMB, Oxoid, Fannin Ltd, Ireland) and plate count agar (PCA, Oxoid, Fannin Ltd, Ireland), then incubated at 37°C for 24 h. EMB plates were inspected for a green metallic sheen, and corresponding colonies on PCA plates were used to carry out agglutination testing using the Sifin Anti-coli O157 sera test (Cruinn Diagnostics Ltd, Ireland) (International Standards Organisation, 2017).
Low concentrations of L. monocytogenes were detected by adding 10 g of the soil sample to 90 ml of half strength Fraser Broth (Oxoid, Fannin Ltd., Ireland) incubated overnight at 30°C, after which 0.1 ml of the resultant culture was added to 10 ml of full-strength Fraser broth (Oxoid, Fannin Ltd., Ireland) and incubated at 37°C for 48 h. Duplicate 100 μl aliquots were plated on Listeria selective Oxford Agar (Oxoid, Fannin Ltd., Ireland) with streptomycin sulphate (1,000 μg/ml) and characteristic colonies were considered to be L. monocytogenes.
For E. faecalis, 10 g of soil sample was combined with 90 ml of BBL enterococcosel broth (Becton Dickinson, Limerick, Ireland) and incubated at 37°C for 24 h before plating on Slanetz and Bartley Agar (Oxoid, Fannin Ltd., Ireland), incubated at 37°C for 24 h and 42°C for a further 24 h. Pink/red colonies were considered to be E. faecalis.
Low concentrations of C. sporogenes were detected by adding exactly 10 g of soil sample to 90 ml of Reinforced Clostridial Medium (RCM; Oxoid, Fannin Ltd., Ireland) and incubating anaerobically as previously described at 37°C for 48 h. The enrichment culture was then plated on RCA in duplicate, and colonies confirmed using the qPCR method as described above.
Micro-Plot Survival Study
Plot Preparation
An outdoor study site of approximately 0.13 acres (526 m2) was selected at the Teagasc campus in Ashtown, Dublin (latitude 53.381590, longitude −6.336162) in the east of Ireland. The area has a temperate maritime climate, with an average annual temperature of 10.4°C and mean annual precipitation of 807.6 mm (Met Éireann, 2019). The site was un-grazed and has been covered in a grass sward for many years with regular cutting but no slurry or other fertiliser treatment. The topography was slightly undulating with an average slope of ~10° along the length and 4° across the width. Listeria innocua was selected as the model organism as it is non-pathogenic, absent from the soil samples tested at the site and capable of surviving in soil. Exactly 12 micro-plots were prepared and randomly assigned to three groups (four plots each); (1) control (uninoculated); (2) L. innocua applied in digestate; and (3) L. innocua applied in bovine slurry.
These micro-plots were prepared as described by Brennan et al. (2012) and Nolan et al. (2020), with the longest dimension in the direction of the slope and laid out using the micro-plot aluminium frame (0.4 x 0.9 m) as shown in Figure 1. The frame was hammered into the soil and each plot was a minimum of one frame width apart. The edges of the plot were sealed with clay (to prevent infiltration from outside of the plot area) and a 0.6 m polypropylene plastic run-off collection channel was fitted. After installation, plots were initially left uncovered to allow natural rainfall to wash away any soil disturbed during the process. From the application date, rain out shelters were used to cover plots and exclude natural rainfall (Nolan et al., 2020).
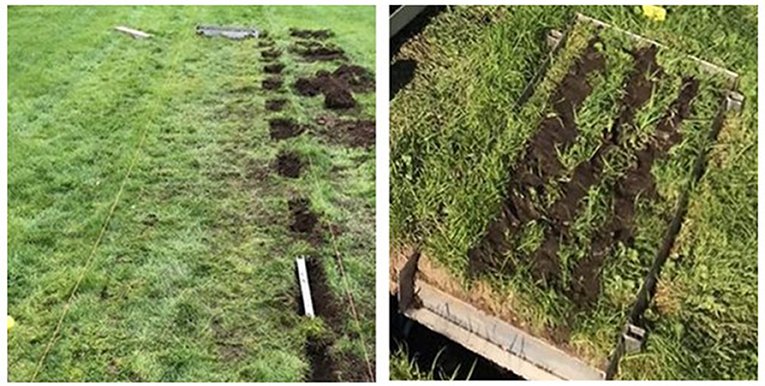
Figure 1. The layout of the micro-plots (left) and a micro-plot with the aluminium frame and collection channel installed (right).
Inoculum Preparation and Application
Three environmental L. innocua strains (isolated in fields grazed by cattle) were chosen from the Teagasc culture collection (designated M220, M344 and T1095) and streptomycin sulphate resistant variants of these strains (1,000 μg/ml) (Sigma Aldrich Ireland Ltd., Wicklow, Ireland) were prepared using the method of Blackburn and Davies (1994). Marked strains were stored on cryoprotective beads at −80°C. To prepare an inoculum, a cryoprotective bead was streaked on TSA and incubated at 37°C for 24 h, after which a single colony was removed using a sterile loop, mixed into 10 ml of tryptone soya broth (TSB; Oxoid, Fannin Ltd., Ireland) and incubated overnight at 37°C. The cultures obtained were centrifuged and washed three times with PBS (Oxoid, Fannin Ltd., Ireland), before resuspension in PBS. The 3 strains were then mixed to create a single L. innocua cocktail and serially diluted to give a final concentration of ~5 log10 cfu/g when inoculated into the slurry or digestate, which was performed as previously described.
Treatment of Soils With Inoculated Slurry or Digestate
To ensure the field trials mimicked reality and that the results were comparable, the amount of slurry and digestate applied to the micro plots was governed by the phosphorous (P) content of these materials and the P index of the soil. All the micro-plots were classified into index 2 soil for grassland crops (soil P range of 3.1 to 5.0 mg/L) so the slurry and digestate were applied at a rate of 40 Kg per hectare (Wall and Plunkett, 2020). Thus 3,838 g of slurry and 1,644 g of digestate were spread on each micro-plot. These were applied in rows using a watering can to mimic application via dribble bar on a slurry spreader (Figure 2).
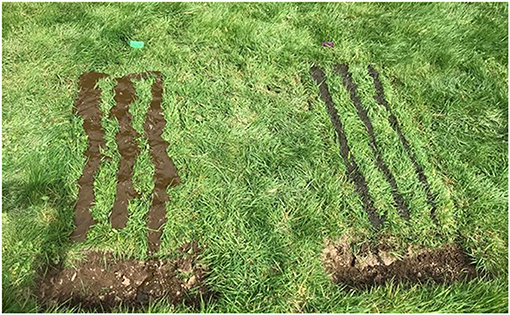
Figure 2. Inoculated slurry (left) and digestate (right) were applied to the microplots in linear rows.
Rainfall was simulated using the Amsterdam drip type simulator (Bowyer-Bower and Burt, 1989) at a target rate of ~11 mm per plot per h−1, as used in other similar studies (Peyton et al., 2016). The rainfall simulator formed droplets of median diameter of 2.3 mm, spaced 30 mm apart in a 1,000 × 500 × 8 mm perspex plate over a 0.5 m2 simulator area. To maximise control and to prevent runoff losses due to natural rainfall events, individual micro-plots were covered between treatments by large sheets of plastic. The first rainfall simulation (30 min) took place 24 h after treatment application thus representing a worst-case scenario that is contrary to current regulations, which stipulate that spreading of organic manure should not be carried out within 48 h of forecasted heavy rain [Nitrates Directive (91/676/EEC)]. The second was performed after 48 h (a scenario compliant with current regulations) the third after 14 d and the final rainfall simulation after 30 d. The first 50 ml, the last 50 ml and the runoff in between were collected separately and tested for the L. innocua strains using brilliance listeria agar (formerly Oxoid Chromogenic Listeria Agar (OCLA), (Oxoid, Fannin Ltd., Ireland) supplemented with streptomycin sulphate (1,000 μl/g; Sigma Aldrich Ireland Ltd., Wicklow, Ireland) and incubated at 37°C for 48 h. Soil samples were taken using a soil plugging tool on the day of the final rainfall simulation (30 d) and also after 58, 86 and 112 d and tested for L. innocua (as above).
Data Analysis
The laboratory-based soil survival study was performed in duplicate and repeated on 3 separate occasions. Bacterial counts were converted into log10 cfu/g and the T90-values (the time required to achieve a 90% (1 log) reduction in the population) were determined by linear regression using Graphpad Prism 7 software (San Diego, CA, USA), considering each replicate Y-value as an individual point. Differences between slopes were examined using one-way ANOVA and Tukeys multiple comparison tests (GraphPad Prism 7.02). Statistical significance was set at the 5% level (P < 0.05). For the field study, L. innocua counts were converted to log10 cfu/ml and differences in the mean counts in each sample type (first 50 ml, last 50 ml and overall) were analysed using one-way ANOVA (Graphpad Prism 7.02).
Results
In the laboratory survival studies the pH of the sandy soil amended with slurry ranged from 5.9 to 7.0. The corresponding figures for sandy soil plus digestate, clay soil plus slurry and clay soil plus digestate were 5.3 to 6.9, 5.2 to 6.7 and 4.9 to 6.3 regardless of bacterial inoculum or storage temperature (Table 1). The aw in all soil-amendment combinations ranged from 0.95 to 1.0, regardless of storage temperature (also Table 1).
The decimal reduction curves are shown in Figures 3–7 and the T90 values are provided in Table 2. Growth was not detected and the decline phase followed first order kinetics for all of the target bacteria regardless of soil type, storage temperature or amendment type. Although there was no shoulders observed, tailing was obtained at 4°C (slurry and digestate) with S. Senftenberg (sandy soil), E. coli O157:H7 (both soil types) and E. faecalis (sandy soil). The T90 values obtained for S. Senftenberg stored at 4°C were 21.3, 18.5, 17.9 and 16.7 d in sandy-slurry, sandy-digestate, clay-slurry and clay-digestate, respectively. The corresponding T90 values for E. coli O157:H7 were 25.0, 23.9, 24.4 and 21.7 d, respectively, for L. monocytogenes were 11.9, 12.4, 12.8 and 12.1 d, and for E. faecalis were 33.3, 30.3, 50.0 and 41.7 d. The reduction in C. sporogenes was marginal (1 log10 cfu/g or less over 124 d) and this is reflected in the T90 values, which were considerably higher at 166.7, 111.1, 111.1 and 100.0 d. For a given bacterium, the T90 values were not significantly (P > 0.05) different regardless of the soil type or amendment (slurry or digestate) except for E. coli O157 and E. faecalis, both in sandy soil where the T90 values obtained in slurry-amended soil were significantly higher than the corresponding values in digestate-treated soil.
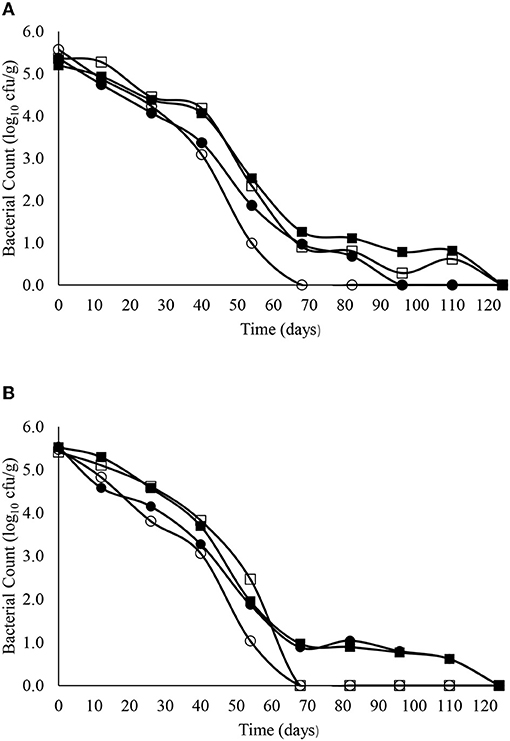
Figure 3. The reduction in S. Senftenberg over time in sandy (A) and clay (B) soils including with slurry at 4°C (■), digestate at 4°C (□), slurry at 14°C (•) and digestate at 14°C (o).
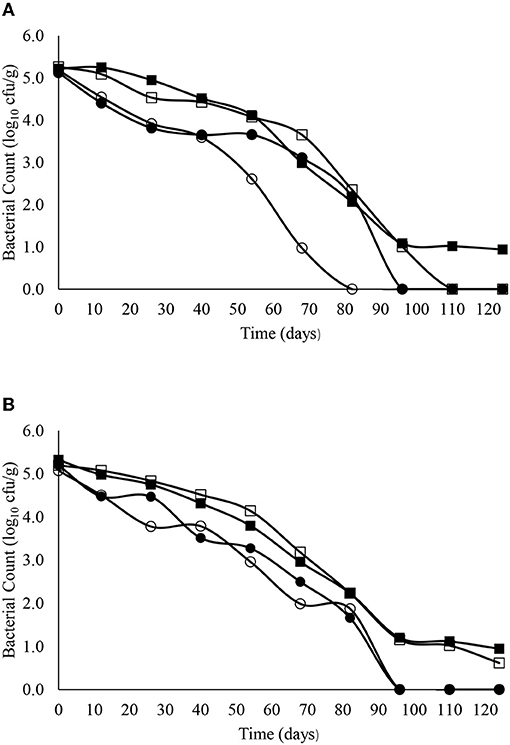
Figure 4. The reduction in E. coli O157:H7 over time in sandy (A) and clay (B) soils including with slurry at 4°C (■), digestate at 4°C (□), slurry at 14°C (•) and digestate at 14°C (o).
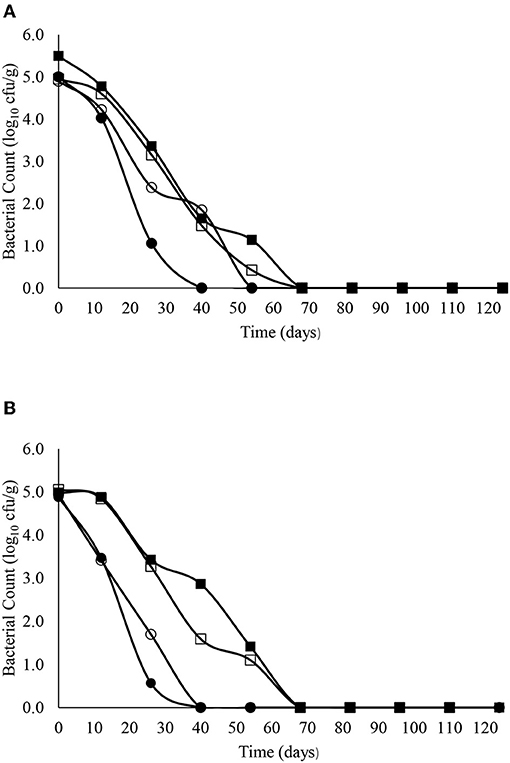
Figure 5. The reduction in L. monocytogenes over time in sandy (A) and clay (B) soils including with slurry at 4°C (■), digestate at 4°C (□), slurry at 14°C (•) and digestate at 14°C (o).
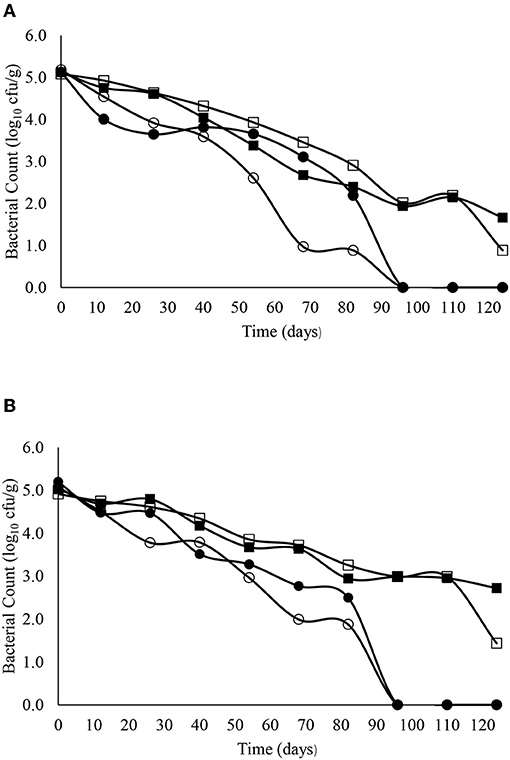
Figure 6. The reduction in E. faecalis over time in sandy (A) and clay (B) soils including with slurry at 4°C (■), digestate at 4°C (□), slurry at 14°C (•) and digestate at 14°C (o).
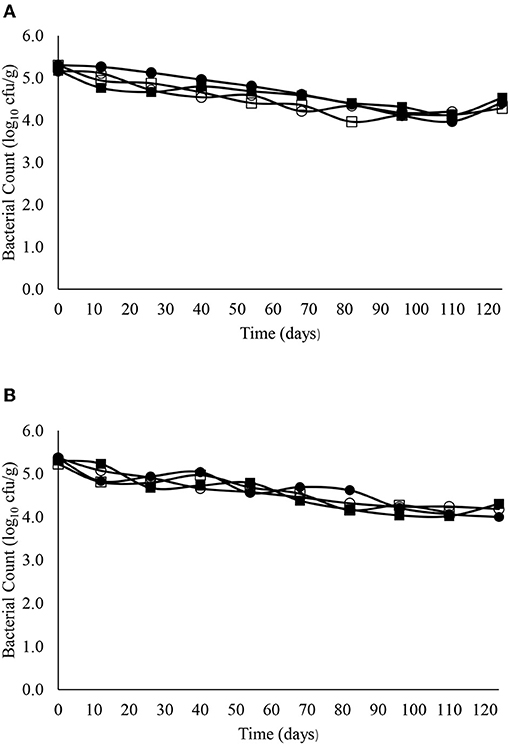
Figure 7. The reduction in C. sporogenes over time in sandy (A) and clay (B) soils including with slurry at 4°C (■), digestate at 4°C (□), slurry at 14°C (•) and digestate at 14°C (o).
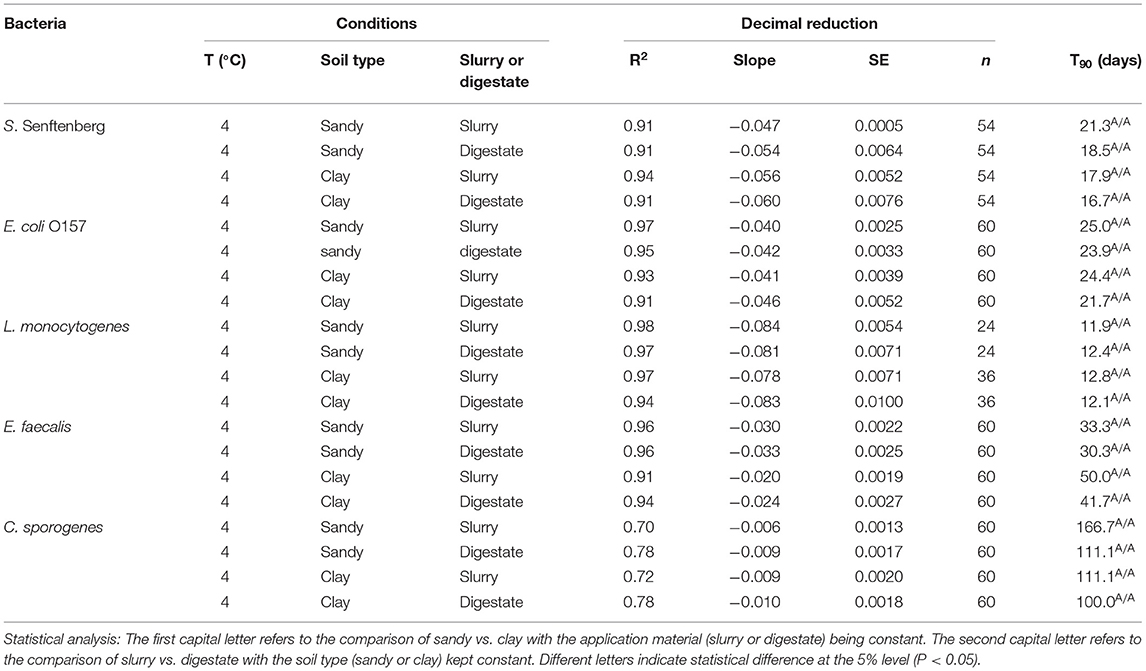
Table 2. T90 values for the different bacteria applied in slurry and digestate to sandy loam and clay loam soils and stored at 4°C in the laboratory trials.
At 14°C the T90 values for S. Senftenberg, E. coli O157:H7, L. monocytogenes, E. faecalis and C. sporogenes ranged from 12.4 to 20.8 d, 17.6 to 36.6 d, 6.0 to 12.2 d, 18.1 to 36.6 d and 106.5 to 156.0 d, respectively (Table 3). For most pathogens soil type or application material had no effect with the exception of E. coli O157 and E. faecalis in sandy soils where significantly (P < 0.05) higher T90 values were obtained in slurry compared to digestate.
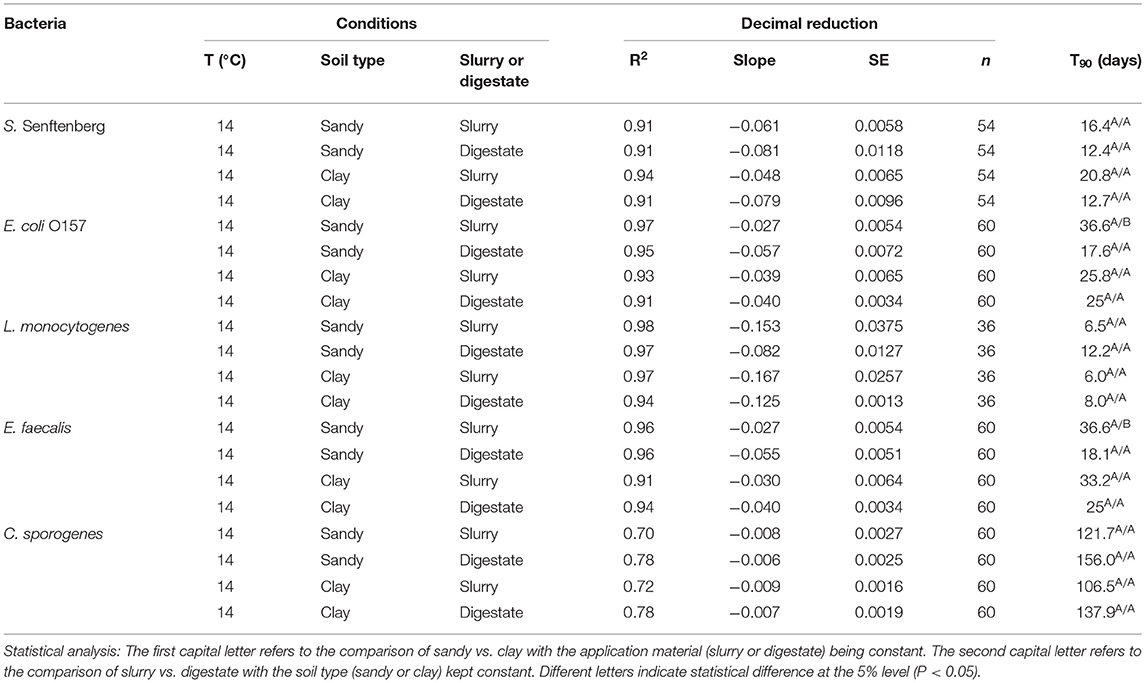
Table 3. T90 values for the different bacteria applied in slurry and digestate to sandy loam and clay loam soils and stored at 14°C in the laboratory trials.
The uninoculated slurry, digestate and the soil in the field plots all tested negative for L. innocua (10 samples each) prior to commencement of the field trial. The initial concentrations of L. innocua in the inoculated slurry and digestate was 5 log10 cfu/g (data not shown). In slurry and digestate this concentration decreased to 3.1 log10 cfu/g and 4.5 log10 cfu/g after 2 days, respectively. In both materials the L. innocua population remained at this level until at least day 14. Thereafter it was not possible to test the slurry or digestate for L. innocua as these materials had become absorbed and mixed into the soil. Soil samples were therefore obtained after day 30 and tested. Indeed, it was advised not to take soil samples before this stage as removal of soil plugs from the plot could influence the way in which the rainfall penetrated the soil and hence the concentrations of L. innocua in the run-off. L. innocua was not detected in the soil samples taken throughout this experiment at 30, 58, 86 or 112 days (data not shown).
Rainfall was simulated at a rate of ~11 mm per plot h−1 for 30 min, the runoff collected and the bacterial concentration in the first 50 ml, last 50 ml and overall runoff measured. On the first day the L. innocua counts from slurry amended soil were 2.4, 2.0 and 2.0 log10 cfu/ml, respectively and the corresponding counts when applied in digestate were 1.9, 1.9 and 1.6 log10 cfu/ml (Table 4). On day 2 the equivalent counts were 2.4, 2.5 and 2.2 log10 cfu/ml for slurry and 2.0, 2.0 and 1.7 log10 cfu/ml for digestate. On day 14 a similar pattern was observed (2.0, 2.2 and 2.0 log10 cfu/ml for slurry vs. 1.7, 1.7 and 1.3 log10 cfu/ml for digestate) and again on day 30 (2.4, 2.4 and 1.7 log10 cfu/ml for slurry and 1.6, 1.7 and below the level of detection for digestate). Overall, significantly (P < 0.05) lower L. innocua counts were obtained when applied in digestate on day 1 (first 50 ml and entire sample), day 2 and day 30 (all samples).
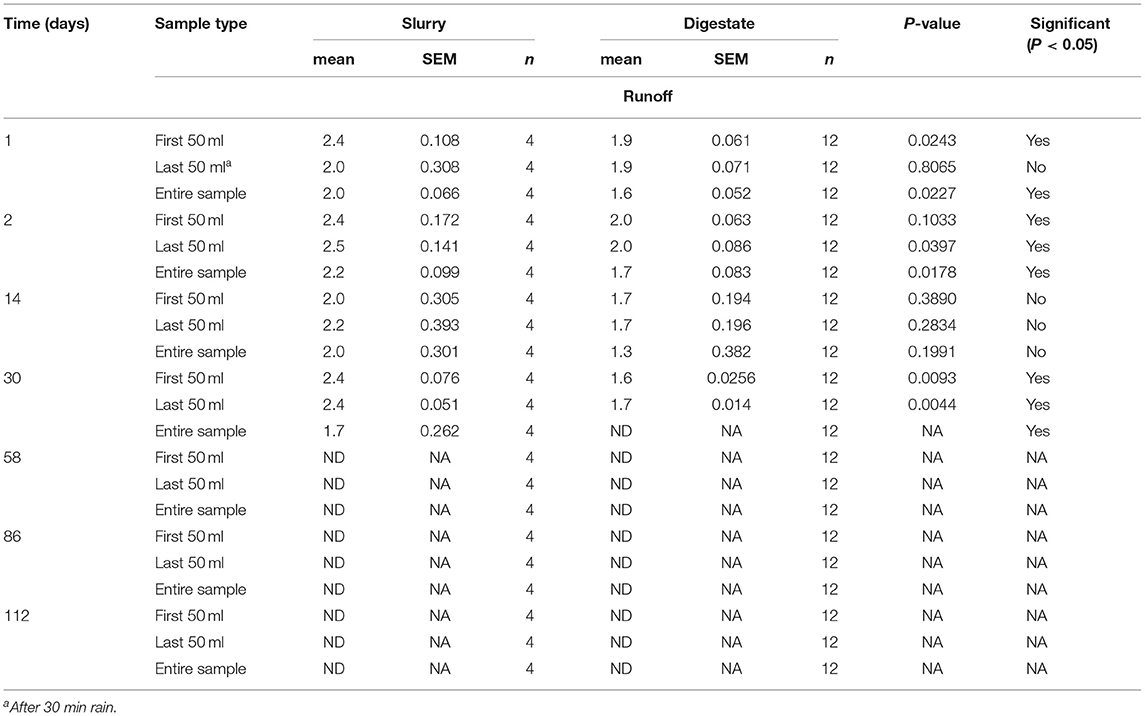
Table 4. The concentrations of L. innocua in leachate during rainfall simulation from inoculated slurry and digestate.
Discussion
The T90 values obtained for S. Senftenberg stored at 4°C ranged from 16.7 to 21.3 d and at 14°C from 12.4 to 20.8 d, regardless of soil type or amendment. Holley et al. (2006) mixed a 6-strain cocktail of Salmonella serovars (Agona, Hadar, Heidelberg, Montevideo, Oranienburg, and Typhimurium) into pig manure and added to a Reinfeld loamy sand and a Marquette clay soil before incubation at different temperature sequences. These represented the winter to summer (−18, 4, 10, 25°C), spring to summer (4, 10, 25, 30°C), or summer to winter (25, 10, 4, −18°C) seasonal periods with each temperature step lasting 45 d. In all cases the T90 values were ≤ 30 d which was consistent with our findings. Nicholson et al. (2005) inoculated bovine slurry with Salmonella Typhimurium before spreading on agricultural land in summer (temperatures were ≤ 20°C). These bacteria generally survived for up to 1 month when applied to both sandy and clay soils. Our findings therefore support the hypothesis that Salmonella spp. die-off within approximately 4 weeks when applied to land, regardless of soil type, amendment (manure, slurry or digestate) or temperature.
The T90 values for E. coli O157:H7 ranged from 21.7 to 25.0 d at 4°C and from 17.6 to 36.6 d at 14°C. In contrast, the Nicholson et al. (2005) study mentioned above, reported an approximate 3.5 log10 cfu/g reduction in E. coli O157:H7 in 4 days when applied in bovine slurry to sandy soils but a residual population of ~0.3 log10cfu/g persisted until day 25. After a short initial growth (increase from 3.5 to 4.0 log10 cfu/g) in slurry spread on clay soils, the E. coli O157:H7 decrease followed first order kinetics and was not detected after 35 days. Previous research by our group investigated the survival of a non-toxigenic strain of E. coli O157:H7 in bovine faeces spread on grassland. A 4.0–5.0 log10 cfu/g reduction was observed within 50 d but the organism was still detectable in the surrounding soil for up to 99 d (Bolton et al., 1999). More recently our group also examined the survival of 6 bovine non-O157 Shiga toxin-producing E. coli (STEC) in sandy and clay soils at 10°C for up to 201 days and obtained T90 values that ranged from 50·3 to 75·6 days in sandy soils and from 31.6 to 48.3 days in clay soils (Bolton et al., 2011). Thus, there is no consistency in the T90 values reported for E. coli O157:H7 or STEC survival in soil possibly due to differences in soil composition, temperature, pH, moisture, exposure to UV light from the sun, protozoan predation and experimental design (Hutchinson et al., 2004; Franz et al., 2005; Jacobsen and Bech, 2012).
The corresponding T90 values for L. monocytogenes, E. faecalis and C. sporogenes ranged from 11.9 to 12.8 d, 30.3 to 50.0 d and 100 to 166.7 d (regardless of soil type or amendment), respectively at 4°C and from 6.0 to 12.2 d, 18.1 to 36.6 d and 106.5 to 156.0 d, respectively, at 14°C. Nicholson et al. (2005) reported a decrease from 2.5 log10 cfu/g to “not detected” in sandy soil after 8 days but, as with E. coli O157:H7, the decrease on clay soil was almost linear, with the same decrease requiring 32 days. Although E. faecalis and Clostridium spp. are common in bovine faeces and digestate (Russell et al., 2020), studies on their survival in amended soils are limited. Byappanahalli and Fujioka (2004) reported that Enterococci are well-adapted to survive in soil, while Sinton et al. (2007) reported a decimal reduction of Enterococci after 56 days in bovine faeces on pasture. Our data suggests Clostridium spp. persist for extended periods in soil. This was not unexpected as soil is the natural habitat for Clostridium spp. such as Clostridium botulinum and inoculation studies in soil have demonstrated persistence for several months (Gessler and Böhnel, 2006). Moreover, Girardin et al. (2005) reported that C. sporogenes inoculated into soil in field trials declined by <0.7 log after 16 months.
With the exception E. coli O157:H7 and E. faecalis, in sandy soil (where the T90 values obtained were significantly higher for slurry as compared to digestate-amended soil), the T90 values were not significantly (P > 0.05) different regardless of the soil type or application material (slurry or digestate). Thus, our findings are more or less consistent with those of Saunders et al. (2012), who reported no significant difference in the die-off rate of E. coli and faecal coliforms in slurry when compared to digestate obtained from the anaerobic digestion of dairy waste). Given the temperature, pH and aw were similar, the significantly reduced survival of E. coli O157:H7 and E. faecalis in digestate amended soil may be the result of lower organic matter and/or nutrient levels (García-Orenes et al., 2010; Nolan et al., 2020). Moreover, the concentration of volatile acids and microbial diversity may also play a role (Klein et al., 2011; Orzi et al., 2015). However, further research would be required to confirm this hypothesis and to determine whether the effects are direct or indirectly via changes in the indigenous soil microbial community (Vinten et al., 2002).
The storage temperatures used in our study (4°C and 14°C) did not appear to exert a consistent effect on bacterial survival. Of the twenty inoculated soil combinations, the T90 values were higher at 4°C on 12 occasions, similar twice and lower for the remainder (6). Phan-Thien et al. (2020) investigated the effects of soil type and temperature on the survival of a cocktail of 5 Salmonella enterica serotypes (Enteritidis, Infantis, Montevideo, Typhimurium and Zanzibar) in poultry manure amended sandy and clay soils at 5, 21 and 37°C for 6 weeks. Higher reductions (3 to 4 log10cfu/g) were obtained at the higher temperature while a 1 to 2 log reduction was observed at 5°C. Another similar study reported that Salmonella Typhimurium survived better in topsoil at 5°C as compared to 15 or 25°C (García-Orenes et al., 2010). Moynihan et al. (2013) also observed the maintenance of a cocktail of 2 E. coli O157:H7 strains at 4°C in Bearsted soil (a typical brown earth, pH 6.07) and Evesham soil (a typical calcareous pelosol, described, pH 6.6). However, field studies suggest that E. coli levels decline in soil when the temperature decreases below 5°C, although the L. innocua persists, independent of temperature (Reed-Jones et al., 2016).
A key finding of our field trial was the retention of L. innocua in digestate resulting in significantly (P < 0.05) lower L. innocua counts in the run-off on day 1 (first 50 ml and entire sample), day 2 and day 30 (all samples). During rainfall, the water either infiltrates into the soil and may be bound by the matrix within gel-like structures or runs off with the latter being more important in terms of the risk of contamination of adjacent water and/or crops (Gentry et al., 2017). This is particularly important when heavy rainfall causes flooding that carries pathogens to adjacent fields and water bodies (Steele and Odumeru, 2004). Water flow in soil systems is complex and can be influenced by soil (including organic matter such as slurry or digestate) texture, structure, hydraulic properties, slope and cover (Jacobsen and Bech, 2012). In addition to the properties of the soil, cellular characteristics such as size, electric charge and hydrophobicity can influence bacterial concentrations found in run-off (Jacobsen and Bech, 2012).
In general, adding organic matter to soil improves the soil structure and fertility (Frøseth et al., 2014). However, the effect is dependent on the type of organic matter with some organic waste materials increasing porosity and water permeability while other have the opposite effect (De Gryze et al., 2006; Benito et al., 2016). Thus, although several studies have demonstrated the dissemination of faecal bacteria from contaminated material on the soil surface to both surface and ground water (Vinten et al., 2002; Gentry et al., 2017), the results may not be comparable to those obtained with L. innocua in this study. Pastorelli et al. (2021) observed an improvement in soil aggregate stability when digestate was added but the proportion of transmission pores and fissures decreased thereby decreasing soil permeability. Digestates also contain higher concentrations of hydrophobic components than cattle slurry (Šimon et al., 2016). Thus, we hypothesise that rainwater falling on freshly applied digestate (as compared to slurry) will have lower penetration and reduced ability to remove bacteria in the resultant run-off. Furthermore, over time the permeability of the digestate amended soil may be less than slurry-treated pastures, reducing leeching of any surviving bacteria into ground water, but thorough investigation is required.
Conclusion
It was concluded that with exceptions [E. coli O157:H7 and E. faecalis in sandy soil (slurry vs. digestate)], the type of organic fertiliser (slurry vs. unpasteurised digestate) does not affect survival rates of the bacteria tested in soil. However, a higher proportion of bacteria may be retained in the soil-digestate matrix during periods of rainfall, thereby reducing the risk of ground and surface water contamination.
Data Availability Statement
The raw data supporting the conclusions of this article will be made available by the authors, without undue reservation.
Author Contributions
DB was responsible for conceiving and the design of the study and obtained the funding. LR acquired and analysed and interpreted the data. DB drafted the manuscript while LR, PW, AZ, SG, BM, TW, SN, VO'F, FA, KR, and OF reviewed the manuscript. All authors contributed to the article and approved the submitted version.
Funding
This study was funded by the Food Institute Research Measure administered by the Department of Agriculture, Food and Marine (Project 14/SF/487). LR was supported by the Teagasc Walsh Scholarship Programme (Number 2014239).
Conflict of Interest
The authors declare that the research was conducted in the absence of any commercial or financial relationships that could be construed as a potential conflict of interest.
Publisher's Note
All claims expressed in this article are solely those of the authors and do not necessarily represent those of their affiliated organizations, or those of the publisher, the editors and the reviewers. Any product that may be evaluated in this article, or claim that may be made by its manufacturer, is not guaranteed or endorsed by the publisher.
Acknowledgments
The authors acknowledge the help provided by Dr. Des Walsh, Ms. Siobhán McSharry, Mr. Bernardino Moreira, Dr. Kenneth Dygico, and Dr. Leonard Koolman while undertaking the field studies.
References
Alam, M. J., and Zurek, L. (2006). Seasonal prevalence of Escherichia coli O157:H7 in beef cattle feces. J. Food Prot. 69, 3018–3020. doi: 10.4315/0362-028X-69.12.3018
Alkanok, G., Demirel, B., and Onay, T. T. (2014). Determination of biogas generation potential as a renewable energy source from supermarket wastes. Was. Man. 34, 13–140. doi: 10.1016/j.wasman.2013.09.015
Artz, R. E., and Killham, K. (2002). Survival of Escherichia coli O157:H7 in private drinking water wells: influences of protozoan grazing and elevated copper concentrations. FEMS. Microbiol. Lett. 216, 117–122. doi: 10.1111/j.1574-6968.2002.tb11424.x
Bagge, E., Sahlström, L., and Albihn, A. (2005). The effect of hygienic treatment on the microbial flora of biowaste in biogas plants. Water. Res. 39, 4879–4886. doi: 10.1016/j.watres.2005.03.016
Benito, P., Bortolotti, V., Fornasari, G., and Vannini, M. (2016). Evaluation of effect of soil organic matter on pores by 1H time-domain magnetic resonance relaxometry and adsorption-desorption of N2. Eur. J. Soil Sci. 67, 314–323. doi: 10.1111/ejss.12336
Bhattacharya, S. S., Kim, K. H., Das, S., Uchimiya, M., Jeon, B. H., Kwon, E., et al. (2016). A review on the role of organic inputs in maintaining the soil carbon pool of the terrestrial ecosystem. J. Environ. Manag. 167, 214–227. doi: 10.1016/j.jenvman.2015.09.042
Blackburn, C. W., and Davies, A. R. (1994). Development of antibiotic-resistant strains for the enumeration of foodborne pathogenic bacteria in stored foods. Int. J. Food Microbiol. 24, 125–136. doi: 10.1016/0168-1605(94)90112-0
Bolton, D. J., Byrne, C. M., Sheridan, J. J., McDowell, D. A., and Blair, I. S. (1999). The survival characteristics of a non-toxigenic strain of Escherichia coli O157:H7. J. Appl. Microbiol. 86, 407–411. doi: 10.1046/j.1365-2672.1999.00677.x
Bolton, D. J., Monaghan, A., Byrne, B., Fanning, S., Sweeney, T., and McDowell, D. A. (2011). Incidence and survival of non-O157 verocytotoxigenic Escherichia coli in soil. J. Appl. Microbiol. 111, 484–490. doi: 10.1111/j.1365-2672.2011.05057.x
Bowyer-Bower, T. A. S., and Burt, T. P. (1989). Rainfall simulators for investigating soil response to rainfall. Soil Technol. 2, 1–16. doi: 10.1016/S0933-3630(89)80002-9
Brennan, R. B., Healy, M. G., Grant, J., Ibrahim, T. G., and Fenton, O. (2012). Incidental phosphorus and nitrogen loss from grassland plots receiving chemically amended dairy cattle slurry. Sci. Tot. Environ. 441, 132–140. doi: 10.1016/j.scitotenv.2012.09.078
Byappanahalli, M., and Fujioka, R. (2004). Indigenous soil bacteria and low moisture may limit but allow faecal bacteria to multiply and become a minor population in tropical soils. Water Sci Technol. 50, 27–32. doi: 10.2166/wst.2004.0009
Casadei, M. A., Ingram, R., Skinner, R. J., and Gaze, J. E. (2000). Heat resistance of Paenibacillus polymyxa in relation to pH and acidulants. J. Appl. Microbiol. 89, 801–806. doi: 10.1111/j.1365-2672.2000.01181.x
Castro-Ibáñez, I., Gil, M. I., Tudela, J. A., and Allende, A. (2015). Microbial safety considerations of flooding in primary production of leafy greens: a case study. Food Res. Int. 68, 62–69. doi: 10.1016/j.foodres.2014.05.065
Clagnan, E., Thornton, S. F., Rolfe, S. A., Wells, N. S., Knöller, K., Murphy, J., et al. (2019). An integrated assessment of nitrogen source, transformation and fate within an intensive dairy system to inform management change. PLoS ONE 14, e219479. doi: 10.1371/journal.pone.0219479
Coohill, T. P., and Sagripanti, J. L. (2009). Bacterial inactivation by solar ultraviolet radiation compared with sensitivity to 254 nm radiation. Photochem. Photobiol. 85, 1043–1052. doi: 10.1111/j.1751-1097.2009.00586.x
De Gryze, S., Jassogne, L., Six, J., Bossuyt, H., Wevers, M., and Merckx, R. (2006). Pore structure changes during decomposition of fresh residue: X-ray tomography analyses. Geoderma 134, 82–96. doi: 10.1016/j.geoderma.2005.09.002
European Commission (2020). A Farm to Fork Strategy for a Fair, Healthy and Environmentally-Friendly Food System. Available at https://ec.europa.eu/food/horizontal-topics/farm-fork-strategy_en (accessed October 10, 2021).
Ferens, W. A., and Hovde, C. J. (2011). Escherichia coli O157:H7: animal reservoir and sources of human infection. Foodborne Pathog. Dis. 8, 465–487. doi: 10.1089/fpd.2010.0673
Foged, H. L., Flotats, X., Bonmati, A., Palatsi, B. J., Magri, A., and Schelde, K. M. (2011). Inventory of Manure Processing Activities in Europe. Technical Report No. I Concerning “Manure Processing Activities in Europe” to the European Commission, Directorate-General Environment. Available online at: https://op.europa.eu/en/publication-detail/-/publication/d629448f-d26a-4829-a220-136aad51d1d9 (accessed October 10, 2021).
Franz, E., van Diepeningen, A. D., de Vos, O. J., and van Bruggen, A. H. C. (2005). Effects of cattle feeding regimen and soil management type on the fate of Escherichia coli O157:H7 and Salmonella enterica serovar Typhimurium in manure, manure-amended soil, and lettuce. Appl. Environ. Microbiol. 71, 6165–6174. doi: 10.1128/AEM.71.10.6165-6174.2005
Frøseth, R. B., Bakken, A. K., Bleken, M. A., Riley, H., Pommeresche, R., Thorup-Kristensen, K., et al. (2014). Effects of green manure herbage management and its digestate from biogas production on barley yield; N recovery; soil structure and earthworm populations. Eur. J. Agron. 52, 90–102. doi: 10.1016/j.eja.2013.10.006
García-Orenes, F., Guerrero, C., Roldán, A., Mataix-Solera, J., Cerd,à, A., Campoy, M., et al. (2010). Soil microbial biomass and activity under different agricultural management systems in a semiarid Mediterranean agroecosystem. Soil Till. Res. 109, 110–115. doi: 10.1016/j.still.2010.05.005
Gentry, R. R., Froehlich, H. E., Grimm, D., Kareiva, P., Parke, M., Rust, M., et al. (2017). Mapping the global potential for marine aquaculture. Nat. Ecol. Evol. 1, 1317–1324. doi: 10.1038/s41559-017-0257-9
Gessler, F., and Böhnel, H. (2006). Persistence and mobility of a Clostridium botulinum spore population introduced to soil with spiked compost. FEMS Microbiol. Ecol. 58, 384–393. doi: 10.1111/j.1574-6941.2006.00183.x
Girardin, H., Morris, C. E., Albagnac, C., Dreux, N., Glaux, C., and Nguyen-The, C. (2005). Behaviour of the pathogen surrogates Listeria innocua and Clostridium sporogenes during production of parsley in fields fertilized with contaminated amendments. FEMS Microbiol. Ecol. 54, 287–295. doi: 10.1016/j.femsec.2005.04.003
Goberna, M., Insam, H., and Franke-Whittle, I. H. (2009). Effect of biowaste sludge maturation on the diversity of thermophilic bacteria and archaea in an anaerobic reactor. Appl. Environ. Microbiol. 75, 2566–2572. doi: 10.1128/AEM.02260-08
Healy, M. G., Fenton, O., Cormican, M., Peyton, D. P., Ordsmith, N., Kimber, K., et al. (2017). Antimicrobial compounds (triclosan and triclocarban) in sewage sludges and their presence in runoff following land application. Ecotoxicol. Environ. Saf. 142, 448–453. doi: 10.1016/j.ecoenv.2017.04.046
Herman, K. M., Hall, A. J., and Gould, L. H. (2015). Outbreaks attributed to fresh leafy vegetables, United States, 1973-2012. Epidemiol. Infect. 143, 3011–3021. doi: 10.1017/S0950268815000047
Holley, R. A., Arrus, K. M., Ominski, K. H., Tenuta, M., and Blank, G. (2006). Salmonella survival in manure-treated soils during simulated seasonal temperature exposure. J. Environ. Qual. 35, 1170–1180. doi: 10.2134/jeq2005.0449
Hutchinson, M. L., Walters, L. D., Moore, A., Crookes, K. M., and Avery, S. M. (2004). Effect of length of time before incorporation on survival of pathogenic bacteria present in livestock wastes applied to agricultural soil. Appl. Environ. Microbiol. 70, 5111–5118. doi: 10.1128/AEM.70.9.5111-5118.2004
International Standards Organisation (2017). ISO 6579-1:2017 Microbiology of the Food Chain - Horizontal Method for the Detection, Enumeration and Serotyping of Salmonella. Available online at: https://www.iso.org/standard/56712.html (accessed June 1, 2021).
Jacobsen, C. S., and Bech, T. B. (2012). Soil survival of Salmonella and transfer to freshwater and fresh produce. Food Res. Inter. 45, 557–566. doi: 10.1016/j.foodres.2011.07.026
Johansson, M., Emmoth, E., Salomonsson, A. C., and Albihn, A. (2005). Potential risks when spreading anaerobic digestion residues on grass silage crops - survival of bacteria, moulds and viruses. Grass Forage Sci. 60, 175e185.doi: 10.1111/j.1365-2494.2005.00466.x
Klein, M., Brown, L., Ashbolt, N. J., Stuetz, R. M., and Roser, D. J. (2011). Inactivation of indicators and pathogens in cattle feedlot manures and compost as determined by molecular and culture assays. FEMS Microbiol. Ecol. 77, 200–210. doi: 10.1111/j.1574-6941.2011.01098.x
Larkin, R. P. (2015). Soil health paradigms and implications for disease management. Annu. Rev. Phytopathol. 53, 199–221. doi: 10.1146/annurev-phyto-080614-120357
Met Éireann (2019). Monthly Values for the Phoenix Park. Available online at: https://www.met.ie/climate/available-data/monthly-data (accessed November 20, 2021).
Morandi, S., Cremonesi, P., Silvetti, T., Castiglioni, B., and Brasca, M. (2015). Development of a triplex real-time PCR assay for the simultaneous detection of Clostridium beijerinckii, Clostridium sporogenes and Clostridium tyrobutyricum in milk. Anaerobe 34, 44–49. doi: 10.1016/j.anaerobe.2015.04.005
Moynihan, E. L., Richards, K. G., Ritz, K., Tyrrel, S. F., and Brennan, F. P. (2013). Impact of soil type, biology and temperature on the survival of non-toxigenic Escherichia coli O157. Biol. Environ. 113B, 41–46. doi: 10.1353/bae.2013.0029
Nicholson, F. A., Groves, S. J., and Chambers, B. J. (2005). Pathogen survival during livestock manure storage and following land application. Bioresour Technol. 96, 135–43. doi: 10.1016/j.biortech.2004.02.030
Nikoli, T., and Matsi, T. (2011). Influence of liquid cattle manure on micronutrients content and uptake by corn and their availability in a calcareous soil. Agron. J. 103, 113–118. doi: 10.2134/agronj2010.0273
Nolan, S., Thorn, C. E., Ashekuzzaman, S. M., Kavanagh, I., Nag, R., Bolton, D., et al. (2020). Land-spreading with co-digested cattle slurry, with or without pasteurisation, as a mitigation strategy against pathogen, nutrient and metal contamination associated with untreated slurry. Sci. Total Environ. 744, 140841. doi: 10.1016/j.scitotenv.2020.140841
Nolan, S., Waters, N. R., Brennan, F., Auer, A., Fenton, O., Richards, K., et al. (2018). Toward assessing farm-based anaerobic digestate public health risks: comparative investigation with slurry, effect of pasteurization treatments, and use of miniature bioreactors as proxies for pathogen spiking trials. Front. Sustain. Food Syst. 2, 41. doi: 10.3389/fsufs.2018.00041
Olsen, J. E., and Larsen, H. E. (1987). Bacterial decimation times in anaerobic digestions of animal slurries. Biol. Wastes 21, 153e68. doi: 10.1016/0269-7483(87)90121-2
Ongeng, D., Geeraerd, A. H., Springael, D., Ryckeboer, J., Muyanja, C., and Mauriello, G. (2015). Fate of Escherichia coli O157:H7 and Salmonella enterica in the manure-amended soil-plant ecosystem of fresh vegetable crops: a review. Crit. Rev. Microbiol. 41, 273–294. doi: 10.3109/1040841X.2013.829415
Orzi, V., Scaglia, B., Lonati, S., Riva, C., Boccasile, G., Alborali, G. L., et al. (2015). The role of biological processes in reducing both odor impact and pathogen content during mesophilic anaerobic digestion. Sci. Total Environ. 526, 116–126. doi: 10.1016/j.scitotenv.2015.04.038
Pastorelli, R., Valboa, G., Lagomarsino, A., Fabiani, A., Simoncini, S., Zaghi, M., et al. (2021). Recycling biogas digestate from energy crops: effects on soil properties and crop productivity. Appl. Sci. 11, 750. doi: 10.3390/app11020750
Pepper, I. L., Brooks, J. P., and Gerba, C. P. (2006). Pathogens in biosolids. Adv. Agron. 90, 1–41. doi: 10.1016/S0065-2113(06)90001-7
Peyton, D. P., Healy, M. G., Fleming, G. T. A., Grant, J., Wall, D., Morrison, L., Cormican, M., and Fenton, O. (2016). Nutrient, metal and microbial loss in surface runoff following treated sludge and dairy cattle slurry application to an Irish grassland soil. Sci. Total Environ. 541, 218–29. doi: 10.1016/j.scitotenv.2015.09.053
Phan-Thien, K., Metaferia, M. H., Bell, T. L., Bradbury, M. I., Sassi, H. P., et al. (2020). Effect of soil type and temperature on survival of Salmonella enterica in poultry manure-amended soils. Lett. Appl. Microbiol. 71, 210–217. doi: 10.1111/lam.13302
Reed-Jones, N., Marine, Sash, A., Everts, K., and Micallef, S. (2016). Effects of cover crop species and season on population dynamics of Escherichia coli and Listeria innocua in soil. Appl. Environ. Microbiol. 82, 767–1777. doi: 10.1128/AEM.03712-15
Russell, L., Whyte, P., Zintl, A., Gordon, S., Markey, B., de Waal, T., et al. (2020). A small study of bacterial contamination of anaerobic digestion materials and survival in different feed stocks. Bioengineering 7, 16. doi: 10.3390/bioengineering7030116
Sahlström, L., Aspan, A., Bagge, E., Tham, M. L., and Albihn, A. (2004). Bacterial pathogen incidences in sludge from Swedish sewage treatment plants. Water Res. 38, 1989–1994. doi: 10.1016/j.watres.2004.01.031
Saunders, O. E., Fortuna, A. M., Harrison, J. H., Whitefield, E., Cogger, C. G., Kennedy, A. C., et al. (2012). Comparison of raw dairy manure slurry and anaerobically digested slurry as N sources for grass forage production. Int. J. Agron. 2012, 101074. doi: 10.1155/2012/101074
Sidhu, J., Gibbs, R. A., Ho, G. E., and Unkovich, I. (2001). Role of indigenous microorganisms in suppression of Salmonella regrowth in composted biosolids. Water Resour. 35, 913–920. doi: 10.1016/S0043-1354(00)00352-3
Šimon, T., Kunzová, E., and Friedlová, M. (2016). The effect of digestate, cattle slurry and mineral fertilization on the winter wheat yield and soil quality parameters. Plant Soil Environ. 61, 522–527. doi: 10.17221/530/2015-PSE
Sinton, L. W., Braithwaite, R. R., Hall, C. H., and Mackenzie, M. L. (2007). Survival of indicator and pathogenic bacteria in bovine feces on pasture. Appl. Environ. Microbiol. 73, 7917–725. doi: 10.1128/AEM.01620-07
Slepetiene, A., Volungevicius, J., Jurgutis, L., Liaudanskiene, I., Amaleviciute-Volunge, K., Slepetys, J., et al. (2020). The potential of digestate as a biofertilizer in eroded soils of Lithuania. Waste Manag. 102, 441–451. doi: 10.1016/j.wasman.2019.11.008
Steele, M., and Odumeru, J. (2004). Irrigation water as source of foodborne pathogens on fruit and vegetables. J. Food Prot. 67, 2839–2849. doi: 10.4315/0362-028X-67.12.2839
Vinten, A., Whitmore, A. P., Bloem, J., Howard, R., and Wright, F. (2002). Factors affecting N immobilisation/mineralisation kinetics for cellulose, glucose- and straw-amended sandy soils. Biol. Fert. Soils. 36, 190–199. doi: 10.1007/s00374-002-0524-y
Wall, D., and Plunkett, M. (2020). Major & Micro Nutrient Advice for Productive Agricultural Crops. Available at https://www.teagasc.ie/media/website/publications/2020/Major–Micro-Nutrient-Advice-for-Productive-Agricultural-Crops-2020.pdf (accessed September 12, 2021).
Wang, C., Geng, Y., Cheng, L., and Mao, Y. (2018). Speciation, mass loadings, and fate of phosphorus in the sewage sludge of China. Environ. Sci. Poll. Res. 25, 35531–35537. doi: 10.1007/s11356-018-3520-y
Warriner, K., Huber, A., Namvar, A., Fan, W., Dunfield, K., and Steve, L. T. (2009). Recent advances in the microbial safety of fresh fruits and vegetables. Adv Food Nutr Res 57, 155–208. doi: 10.1016/S1043-4526(09)57004-0
Keywords: bacterial pathogens, survival, soil, slurry, digestate, runoff
Citation: Russell L, Whyte P, Zintl A, Gordon SV, Markey B, de Waal T, Nolan S, O'Flaherty V, Abram F, Richards K, Fenton O and Bolton D (2022) The Survival of Salmonella Senftenberg, Escherichia coli O157:H7, Listeria monocytogenes, Enterococcus faecalis and Clostridium sporogenes in Sandy and Clay Loam Textured Soils When Applied in Bovine Slurry or Unpasteurised Digestate and the Run-Off Rate for a Test Bacterium, Listeria innocua, When Applied to Grass in Slurry and Digestate. Front. Sustain. Food Syst. 6:806920. doi: 10.3389/fsufs.2022.806920
Received: 01 November 2021; Accepted: 26 January 2022;
Published: 21 March 2022.
Edited by:
Helene Nalini Chinivasagam, Queensland Government, AustraliaReviewed by:
Keith Warriner, University of Guelph, CanadaAgnes Kilonzo-Nthenge, Tennessee State University, United States
Copyright © 2022 Russell, Whyte, Zintl, Gordon, Markey, de Waal, Nolan, O'Flaherty, Abram, Richards, Fenton and Bolton. This is an open-access article distributed under the terms of the Creative Commons Attribution License (CC BY). The use, distribution or reproduction in other forums is permitted, provided the original author(s) and the copyright owner(s) are credited and that the original publication in this journal is cited, in accordance with accepted academic practice. No use, distribution or reproduction is permitted which does not comply with these terms.
*Correspondence: Declan Bolton, declan.bolton@teagasc.ie
†These authors have contributed equally to this work and share first authorship