- 1Africa Center of Excellence for Climate-Smart Agriculture and Biodiversity Conservation, Haramaya University, Dire Dawa, Ethiopia
- 2College of Agriculture, Department of Soil and Geological, Sokoine University of Agriculture, Morogoro, Tanzania
- 3School of Natural Resources Management and Environmental Sciences, Haramaya University, Dire Dawa, Ethiopia
- 4College of Agriculture and Environmental Sciences, Bahir Dar University, Bahir Dar, Ethiopia
Aim: This study investigated the impacts of system of rice intensification (SRI) and conventional management practice (CP) on rice growth, grain yield, and nitrogen use efficiency by nitrogen application.
Methods: Field experiments were conducted in wet and dry seasons; each season, the experiment was set in a split-plot randomized complete block design in triplicate with crop management practices in main plots and nitrogen levels in subplots.
Results: The average grain yield by SRI was 7.1 and 6.7 t ha−1, while by CP it was 6.1 and 4.4 t ha−1in the wet and dry seasons, respectively. The grain yield of the SRI practice was significantly (p < 0.05) greater than that of the conventional practice (CP) at all levels of nitrogen application. The average yield under the treatment interaction of SRI and nitrogen levels were increased by 13.1% in the wet season and 35.8 % in the dry season. Roots of SRI plants had significantly (p < 0.05) greater fresh weight, length, and volume as indicated by increased root dry weight per hill. SRI improved crop growth, effective tillers, filled grains per panicle, grain filling rate, panicle weight, spikelet per panicle, straw yield, and 1,000-grain weight. Nitrogen application rate had a significant effect (p < 0.05) on agronomic nitrogen use efficiency (ANUE). As the N application rate was increased beyond 90 kg N ha−1, the ANUE and partial factor productivity (PFP) under both SRI and CP were significantly decreased in both seasons.
Conclusion: Overall, the SRI production system with 60 kg N ha−1 improved rice growth, yield, and nitrogen use efficiency compared to the CP.
Introduction
Rice (Oryza sativa L.) is one of the most important grain crops in the world, and more than three billion people worldwide consume rice as a staple food (Zhao et al., 2021). Tanzania is the largest (947,303 km2) country in East Africa and accounts for 9% (2.6 million tons) of African rice production (30.8 million tons) (FAOSTAT, 2014; Materu et al., 2018). Rice is the second most popular staple food crop after maize in Tanzania and the second most important crop commercially (Gowele et al., 2020). Although rice ranks as the second most consumed cereal in Tanzania, its productivity is lower than it should be and could be at 0.5–2 t ha−1 for upland ecologies and 4.5–6 t ha−1 for irrigated ecologies. This is well below the potential yields of 5 and 10–11 t ha−1, respectively, for these different agroecosystems (IRRI, 2013). Low productivity, low nitrogen use efficiency, and poor soil fertility as well environmental degradation are factors contributing to low rice production (Thakur et al., 2013).
Among nutrients, nitrogen (N) is universally deficient in rice cropping systems worldwide (Jiang et al., 2004; Thakur et al., 2013). The recovery of applied N, and the proportion taken up by crop plants, is usually <50% in traditionally flooded paddy rice, due to rapid nitrogen losses through various pathways like denitrification, ammonia volatilization, leaching and surface runoff, and has low N use efficiency (Chen et al., 2017; Hameed et al., 2019). Most of the world's rice crop is produced by subsistence farmers who grow rice with conventional methods. Conventional methods consume large amounts of water resources and increase losses of nitrogen because of leaching, runoff, and agricultural drainage (Yang et al., 2017; Gowele et al., 2020; Zhao et al., 2021).
An alternative way of dealing with these problems is adoption of an agroecological crop management approach (Gleissman, 2014) that utilizes biological and genetic potentials that presently exist in crop plants and soil systems. Such agroecological crop management is the system of rice intensification (SRI). The SRI involves six principles as described by Kahimba et al. (2013), and Thakur et al. (2016): (i) transplanting a single seedling, (ii) transplanting younger seedlings in the 2–3 leaf stage (8–12 days old), (iii) wide plant spacing of 25 × 25 cm or wider, planted in lines, (iv) minimum water applications during vegetative growth period keeping soils moist but well-drained and aerated, (v) frequent weeding with a simple mechanical hand weeder, and (vi) application of organic matters with preference to chemical fertilizers. These principles have beneficial effects according to Thakur et al. (2016): (a) transplanting young seedlings is advantageous for early crop establishment, in part because this avoids or minimizes “transplanting shock,” thereby enhancing the growing plants' tillering and rooting; (b) single seedlings per hill have been found to be superior to transplanting three seedlings per hill (as recommended for conventional approaches), improving tillering, root development, RuBisCO contents, and cytokinin levels. (c) wide-spacing reduces interplant competition for nutrients, water, light, and air, which significantly enhances individual hill performance under SRI management, and this permits prolific tillering and associated root development, along with increased grain development that can more compensate for reduced plant populations on an area basis; (d) alternate wetting and drying (AWD) is considered an effective water-saving technology in rice production, with studies showing that moderate AWD not only saves water but also can increase grain yield; (e) organic manure application under AWD has been seen to increase significantly the uptake of N, P, and K, causing significant increase in filled grains per panicle, 1,000-grain weight, and grain yield, and the beneficial effect of an integrated (organic and mineral) fertilizer strategy has also been significant for grain yields under waterlogged conditions; (f) weeding is essential for SRI, because under an AWD moisture regime, weed growth readily becomes problematic. Mechanical weed control has the advantage of aerating the soil while incorporating weeds into the soil, which enhances both root growth and health and soil populations of beneficial soil microbes. Uphoff and Randriamiharisoa (2002) recommended that these respective individual practices, because of the interactions involved, i.e., between soil moisture/irrigation regime and plant spacing/density, are most effective when used in combination with other SRI practices.
It has shown in more than 60 countries that SRI can raise rice yields significantly by up to 20–100% or more with reduced costs of production (SRI-Rice, 2020), thereby contributing to attaining global food security. Also, it can increase water productivity, reduce greenhouse gas emission by 20–30% (Gathorne-Hardy et al., 2016; Thakur and Uphoff, 2017), and achieve water saving of 25–50% and up to 90% reduction in required seed (Gowele et al., 2020). This improved performance of SRI practices has been attributed to many factors, including more nutrient uptake and proper management of water and fertilizers (Gowele et al., 2020).
Along with SRI performance, soil fertility status, nutrient supply capacity of soil, and nitrogen use efficiency need due attention. Depletion of soil fertility and nitrogen deficiency are major problems in rice cropping systems, particularly in Tanzania (Massawe, 2016). This has been reported by Jumanne (2016), who conducted a study on 9 farms in a Mkindo farmer-managed irrigation scheme and found that 100% of soils had low nitrogen. The same was also reported by Amuri et al. (2013) that some selected paddy growing soils of Tanzania including that in Mkindo farmer-managed irrigation scheme have low total nitrogen, low yields, and low nitrogen use efficiency. Nitrogen use efficiency (NUE) is an established metric used to benchmark N management (Congreves et al., 2021). Also, it is used for environmental and economic objectives of minimizing nutrient losses and negative impact on surrounding water, air, and ecosystems, as well as reducing costs associated with excessive fertilizer inputs (Galloway et al., 2014; Congreves et al., 2021). Improvement of nitrogen use efficiency (NUE) is a challenging task because of its several losses by ammonia volatilization, surface runoff, leaching, and nitrification-denitrification (Islam et al., 2018).
Studies have indicated increased rice yields due to the interaction of nitrogen and water. In China, Zhao et al. (2009) reported an increase in nitrogen uptake, ANUE, and partial factor productivity of applied nitrogen. Combining SRI and appropriate nitrogen levels holds promise in increasing rice productivity and NUE. Furthermore, it is not well-known how SRI modifies crop-soil- water-nutrient use efficiencies and if it requires different N-fertilizer management compared with CP in rice cultivation systems in Tanzania context. We hypothesized that applying N will better interact with the SRI package and could improve rice growth, grain yield, and N fertilizer use efficiency. Our aim was to identify an optimal interaction that could improve yield and N use efficiencies, and reduce fertilizer cost with positive environmental implications.
Materials and Methods
Study Site and Soil
Two consecutive field experiments using the same plots were conducted in 2019 and 2020. The wet season experiment covered the period February 19, 2019 to July 5, 2019, and the dry season began on September 5, 2019 and ended on January 21, 2020.
The field experiments were conducted at the Mkindo farmer-managed irrigation scheme located in Mkindo village in Hembeti Ward, Mvomero District, Morogoro Region, Eastern Tanzania (Figure 1). The district is located between latitude 6°16' and 6°18' south, and longitude 37°32' and 37°36' east, and its altitude ranges between 345 and 365 m amsl. The experimental site is located at latitude 6°15'13” south and longitude 37°32'19”.
The Mkindo farmer-managed irrigation scheme is about 85 km from Morogoro municipality (Gowele et al., 2020). The scheme was constructed in the period between 1980 and 1983. The scheme started producing rice in 1985 with only 17 ha under cultivation. Rice is the only crop produced in the scheme, which serves as a food and income generator. Currently the scheme has an arable area of 740 ha with 300 ha under rice cultivation, and an expansion in the near future is expected for about 620 ha downstream of the current cultivated area.
The climate is tropical with two distinct seasons, dry and wet seasons. The average monthly maximum temperature at the experimental site ranges between 35.1 and 28.5°C for February and June, while the average monthly minimum temperature ranges between 20.4 and 15.8°C for January, March, and July, as shown in Table 1. The mean relative humidity is 67.5%. The area has a bimodal rainfall regime with short rains extending from October to December (OND) and long rains from March to May (MAM). The long rains (masika) receive more rains which ranges between 112.6 and 250.3 mm with a total rainfall of 571.1 mm compared to the short rains (vuli) which receives fewer rains which varies between 52.6 and 116 mm with a total rainfall of 254.5 mm, respectively. The average annual rainfall ranges between 716.5 and 1,503.5 mm (Kahimba et al., 2013; Reuben et al., 2016; Gowele et al., 2020).
The Mkindo rice irrigation scheme has a well-organized irrigation infrastructure (headwork on Mkindo Perennial River of the Wami River Basin, partly lined main canal and unlined secondary and tertiary canals, and drainage canals (de Bruin et al., 2019).
The soils of Mkindo fall under the World Reference Base (WRB) Soil subunit Ferralic Cambisol with low natural fertility. The soils have diffuse horizon boundaries, a clay assemblage dominated by low activity clays (mainly kaolinite) and high content of sesquioxides (ARI-Mlingano, 2006).
Rice cultivation is the major use of land in the study area, and rice is the only crop produced in the scheme, which serves as a food and income generator. This area is dominated by smallholder farmers who cultivate rice by irrigation. Most of them produce rice with conventional practices. Irrigation water in the rice field is kept at continuous flooding. Some farmers fertilize their rice crop mostly by nitrogen-containing fertilizers. Few apply phosphorus fertilizers (personal communication, April 2018). The Morogoro region is among the largest rice-producing area in the country, producing between 300,000 and 350,000 tons per year (Wilson, 2008) and is one of the key food basket regions.
Soil Sampling and Analysis
Soils were sampled before establishing the experiments and analyzed, where ten spots were sampled at a depth of 0–20 cm. The quartering method was used to get a composite soil sample, which was transferred for analysis to Soil Science Laboratory at Sokoine University of Agriculture, Morogoro, Tanzania. Stones, debris, and other foreign materials were carefully removed from the soil samples. Then, the soil samples were air-dried, ground, and passed through a 2-mm mesh sieve and were analyzed for particle size distribution for textural class with Hydrometer method, soil pH with a pH meter in 1:2.5 soil-water, organic carbon with the Walkley-Black Method, total nitrogen with the micro-Kjedahl digestion method, available phosphorus with the Bray and Kurtz method, exchangeable cations (Ca2+, Mg2+, K+, Na+) with NH4+ acetate filtrates by ammonium acetate saturation, and available micronutrients (Zn, Cu, Mn, and Fe) with the DTPA extraction method. The analysis showed that the soil was acidic with a pH of 5.36 and had other chemical characteristics as shown in Table 2. The soil was also characterized for physical properties as sand clay loamy (22.6% clay, 7.6% silt, and 69.8% sand) with a field capacity of 22.2% volume, wilting point of 14.4% volume; available water 0.08 cm/cm, bulk density of 1.59 g/cm3; saturation 40% volume, hydraulic conductivity at 1.43E-6 mm/h, saturated hydraulic conductivity (Ks) at 13.3 mm/h, and matric potential of 175 kPa.
Experimental Design and Treatment Details
Each season, the experiment was arranged in a split-plot randomized complete block design and in triplicate with two factors (crop management practices in main plots and nitrogen levels in subplots). The main plot was then divided into six subplots with a size of 16 m2 (4 × 4 m). All the plots were surrounded by consolidated bunds, and 2-m buffer strips were left between the main plots and 1 m for the subplots to provide access pathways and, more importantly, to minimize the lateral movement of irrigation water and fertilizers between the plots.
Fertilizer treatments comprised six nitrogen levels; these include absolute control treatment (ABC), which did not receive any kind of fertilizer. The absolute control treatment was intended to evaluate rice response under natural soil fertility. The N fertilizer treatments included a control treatment (N0) without any nitrogen fertilizer application but received P and K fertilizers, and this treatment was required to assess crop response to nitrogen fertilizer application and to calculate fertilizer use efficiency. The other treatments, 60, 90, 120, and 150 kg N ha−1, annotated as 60 N, 90 N, 120 N, and 150 N, contained 50, 75, 100, and 125% of the blanket recommended amount of nitrogen fertilizer. The 120 N treatment represents the current blanket recommendation for rice grown in Mkindo. The source of N fertilizer was urea (CON2H4, total N 46%) and was applied twice, that is 50% 14 days after transplanting and 50% during the panicle initiation stage. Panicle initiation (PI) is the second best time to apply N to a rice crop with pre-permanent water being the most efficient. N applications at PI are relatively efficient because the full crop canopy reduces fertilizer volatilization, and the extensive near surface root system takes up N soon after application.
The sources of full dose of phosphorus and potassium were 60 kg ha−1 triple super phosphate (45% P2O5) and 60 kg ha−1 muriate of potash (60% K2O). Phosphorus and potassium fertilizers were applied at same rate to all the plots and were evenly broadcasted and mixed with soil during transplanting. No amount of compost was applied as a source of nutrients. Compost is recommended in SRI if it is available.
Crop Management and Irrigation
Crop Establishment
A TXD 360 semi-aromatic variety, commonly referred to as SARO, was used. This variety is mid-late season rice (120–130 days cycle) that is grown in rain-fed or irrigated production ecologies with a yield potential of 7.0–8.5 t ha−1. It is medium in stature, resistant to lodging, and has a good tillering ability (more than 20 tillers per hill depending on management). Seedling nurseries for each season were prepared by pudding the soil.
Before sowing in the nurseries, seeds were prepared by separating unfilled grains from filled grains so as to get vigorous plants. Only seeds with good density and formation were used for nursery preparations. To get the best seeds, clean tap water was used and seed priming techniques were adopted. The seeds were submerged in a container of tap clean water and stirred well; any light and inferior seeds that floated in the water were discarded. The best seeds were then soaked in clean water for 48 h (seed priming) and incubated in a warm and dark place for 3 days. The practice of soaking seeds before planting enhances the rate of germination and seedling emergence. Also, incubation keeps the seeds warm and hence increases growth of the embryo and results in uniform germination. Thereafter, the germinated seeds were uniformly broadcasted for nursery establishment.
No manuring and fertilization were applied, but water management practices were followed in order to raise healthy seedlings. Land preparation was started 1 month prior to transplant of the seedlings. The land was thoroughly prepared with a power tiller. Subsequently, the land was sufficiently irrigated and plowed and cross plowed three times followed by laddering to have good tilth. All kinds of stubble and residues of previous crop were removed from the field. After uniform leveling, the experimental plots were laid out according to the requirement of the treatment.
In the SRI plot, for transplanting with regular spacing, a square grid pattern was created on the soil's surface using a wooden marker that demarcated distances of 25–25 cm between perpendicular lines. Ten-day-old seedlings were uprooted from the nurseries along with seed sac, and we transplanted one seedling per hill within 30 min of uprooting in both seasons. Rotary cono-weeder and hand were used in weeding. Based on the results of earlier studies with the same rice variety and same area (Mkindo irrigation scheme conditions) (Kahimba et al., 2013; Reuben et al., 2016), it was decided to adopt the recommended SRI principles.
In the CP, 25-day-old seedlings were transplanted in the puddled field at 20 × 20 cm spacing keeping three seedlings hill−1, and hand weeding was conducted for weed management. Experimental details are shown in Table 3.
Water Irrigation Management
The application of continuous flooding irrigation was based mainly on local farmers' practice in CP plots. For the first 14 days after transplanting, a 3–5-cm water depth was maintained under both irrigation regimes to facilitate seedling recovery. Thereafter, the SRI and CP plots were managed differently. Plots under the CP were continuously flooded with a 3–10-cm water level until 10 days before harvest.
After the first 14 days of transplanting, the SRI plots were kept with a layer of 2 cm of water until 14 days after the panicle initiation stage, and during the rest of the growing cycle, the plots were maintained without standing water for 3–5 days before re-irrigation. Thereafter, the SRI plots were re-irrigated to 2 cm when water depth dropped to 15 cm below the soil, and this took a 2- to 3-day interval as recommended by (Kahimba et al., 2013).
Soil water depths were measured and monitored in each SRI plot using PVC pipes installed in the plots at a 15 cm depth as described by Lampayan et al. (2015) (Figure 2). Water depth was measured at 8:00 am and 14:00 pm each day using a101 p7 flat tape water level meter (Solinst Canada Ltd., Georgetown, Ontario, Canada).
PVC pipes were installed in the SRI plots with perforated holes having a diameter of about 0.5 cm each and spaced about 2 cm away from one another. The pipes were installed near the bund for easy water monitoring. After burying the soil inside the tubes was removed so as bottom level is visible. Water level inside the tubes was checked and was the same as the outside. Each of the main plots was irrigated separately. Irrigation water was provided from an irrigation canal and measured with a plastic ruler inserted into the plot.
Assessment of Growth-Contributing Characters
Plant Height
Five plants from each plot were selected randomly and measured in different stages of crop growth until maturity. For juvenile plants, height was measured from plant base to the tip of the tallest leaf. For mature plants, plant height was measured from plant base to the tip of the tallest panicle with a tape measure.
Number of tillers per hill was counted from five plants in each experimental plot on the same day that plant height data were collected, and was counted individually per plant.
Chlorophyll Content
For each sub plot, five hills were randomly selected for measurements, and 5 flag leaves were selected. The CC of leaves was measured using a tLEAF CHL PLUS meter (FT Green LLC, 1000N.West St. Suite 1200# 638 Wilmington, DE 19801 United States; www.atleaf.com was accessed on May 2, 2019) in the panicle initiation and milk grain stages of the rice plant. Measurements were taken on a clear sunny day between 10:00 and 11:30 a.m. before the midday.
Assessment of Root Growth
For root study, root length, root fresh and dry weights, and root volume were examined. Five (5) hills from each subplot were randomly selected for root assessment in the panicle initiation stage during the wet season. For each root sampling, a soil cube (20 × 20 × 30 cm; length, width, and depth) around each individual hill was dug up using a sampling core as described by Xu et al. (2019). Such a cube contains ~95% of total root biomass (Yang et al., 2008). Roots were washed using flowing tap water and by carefully spraying with water until the attached soil and sand particles were removed. A 0.5-mm-diameter sieve was used to prevent loss of fine roots during washing. The samples were labeled, placed in plastic bags, and transported immediately to Soil Science Laboratory at Sokoine University of Agriculture for assessment. Root volume was measured with the water displacement method by putting all the roots in a measuring cylinder of 100 ml and getting the displaced water volume as described by Ndiiri et al. (2012) and Pascual and Wang (2017).
Root length was determined by direct manual measurements of top roots using a ruler against a millimeter paper (Pascual and Wang, 2017). The fresh and dry weights of the roots were determined using a digital Endel Precision Weighing scale (EJB-NB-6000, Dubai) and were expressed in grams. Root dry biomass per hill was determined after oven-drying with Memmert 854 (Memmert GmbH + Co., KGSchwabach, Bavaria, 91126, Germany) at 70°C for 24 h to constant weight (in g).
Assessment of Yield and Yield Components
At harvest, grain yield and yield components were determined. Yield components include harvest index, straw yield, effective and non-effective tillers, number of panicles per square meter, panicle length, panicle weight, number of panicles per hill, grain number per panicle, grain weight per panicle, and filled and unfilled grains per panicle.
Grain yield was determined in a net plot of 2 × 2 m (64 hills) (100 hills) excluding border rows for the SRI and CP plots. In each net plot, a sub-sample of 5 hills was selected for assessment of yield components, and plants were cut at soil level.
The grains were threshed from the panicles. The straw (including peduncle and rachis) was oven-dried (Memmert 854 oven; MEMMERT GmbH + Co. KG Schwabach, 91126 Bavaria, Germany) at 60°C for 72 h to reach a constant weight, while grains were sun-dried before determining weight and moisture. Grain moisture was measured with an 8988N grain moisture meter (Xiamen Hyhoo Imp. & Exp. Co., Ltd., Fujian, China) and adjusted to 14% moisture content using Equation (4). The grain and straw yields obtained were dried under the sun and weighed with Endel Precision Weighing Scale (EJB-NB-6000, Dubai) to record yield/plot and finally converted to t/ha using Equation (1). Grain harvest index was calculated based on the ratio of grain yield to total biomass produced as in Equation (2).
The number of productive tillers and non-productive tillers was obtained by counting the number of tillers with panicles bearing at least one filled grain, which are referred to as effective tillers vice versa is true. Panicle weight was determined at a constant weight after oven-drying at 70°C for 72 h. Panicle length was recorded from the basal node of the rachis to the apex of each panicle with a centimeter ruler. The filled spikelets were separated from the unfilled spikelets using an HMC 67 seed blower (Hoffman Manufacturing Inc., Corvallis, OR 97330, United States), and grain filling rate was calculated on a mass basis as the ratio of filled grain weight to t total grain weight per panicle multiplied by 100, as shown in Equation (3).
One thousand (1,000) grains were randomly counted from the harvested grains in each replicate for 1,000-grain weight determination using seed counter Seedburo 801 Count-A-Pak® (801-10/C model, serial Co 655; Chicago, IL, United States):
where Wf is the final weight at 14% moisture, mcf is the final moisture (14%), mci is the initial moisture, and Wi is the initial grain weight.
Assessment of Nitrogen Use Efficiency
Different measures of NUE such as agronomic nitrogen use rate, partial factor productivity of applied N, and nitrogen contribution rate (FCRN) were calculated (Thakur et al., 2013):
where ANUE is for agronomic N use efficiency, PFPN is for partial factor nitrogen productivity, FCRN is for nitrogen contribution rate, Y is for grain yield with nitrogen application, Y0 is for grain yield without nitrogen application, and F is for amount of nitrogen applied,
Statistical Analysis
All data were statistically analyzed using the procedures described by Gomez and Gomez (1984). Analysis of variance (ANOVA) was performed on a split-plot design, with cultivation system as the main factor and N rates as the sub-factor in each season using the Gen start 4th edition for Windows (Rothamsted Research, United States). Significance of the treatment effect was determined by F-test. Mean differences between treatments were compared using the least significant difference (LSD) method at 5% level of probability, and ordering of treatments was conducted after Duncan's range test.
Results
Rice Growth-Contributing Characters
Plant Height and Number of Tillers
Plant height increased with increase in the amount of nitrogen application and slightly decreased in the later stage of harvest (Tables 4, 5). A significant effect of CMP on plant height was observed in the booting and dough stages in the wet season and in the dough and harvest in the dry season when SRI recorded taller plants than the CP (Tables 4, 5). Nitrogen levels had a significant effect on all stages of crop growth present in the dry season, and during the wet season there was no significant effect recorded on the panicle initiation stage. Treatment interaction had a significant effect on all the three recorded growth stages in the dry season. During both seasons, the absolute control (ABC) and N control (0 kg N ha−1) treatments had shorter plants than the other treatments. Under all the treatments, the highest value of plant height was mainly concentrated in the dough stage. The highest plant height was 130 and 101.7 cm under the 150 kg N ha−1 and SRI ×90 kg N ha−1 treatments in the wet and dry seasons, respectively (data not shown here).
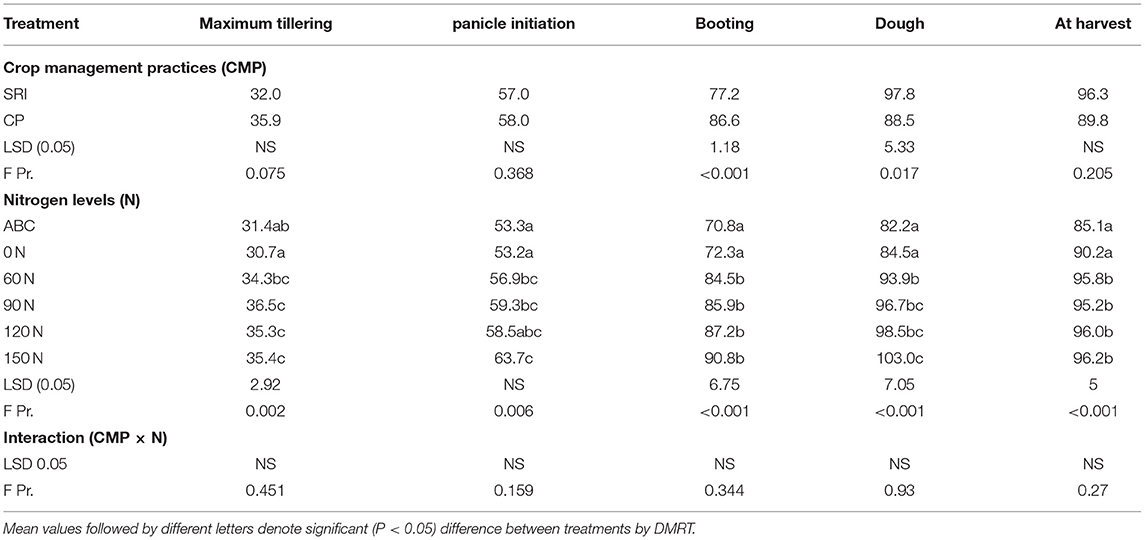
Table 4. Plant height (in cm) during the wet season was affected by crop management practices and nitrogen levels.
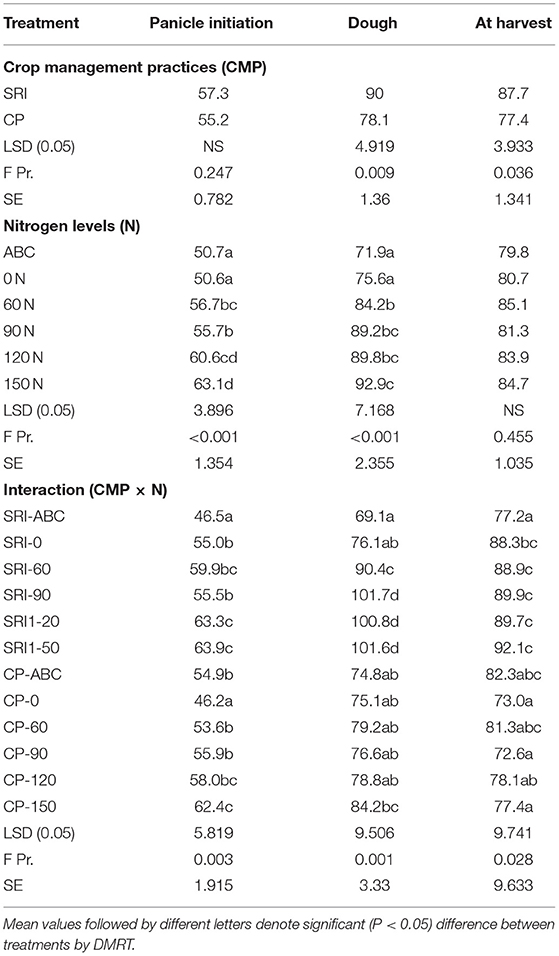
Table 5. Plant height (in cm) during the dry season was affected by crop management practices and nitrogen levels.
With increase in the amount of nitrogen application, the number of tillers increased (Tables 6, 7). The number of tillers indicated a continuous increase in all the stages, but a slight decrease was recorded at harvest. The highest number of tillers (15.2 and 20.3) was recorded under 120 kg N ha−1 and SRI × 90 kg N ha−1 during the wet and dry seasons, respectively. Treatment interaction affected number of tillers during the harvest stage in both seasons. Plants under SRI recorded a high number of tillers than the CP plants.
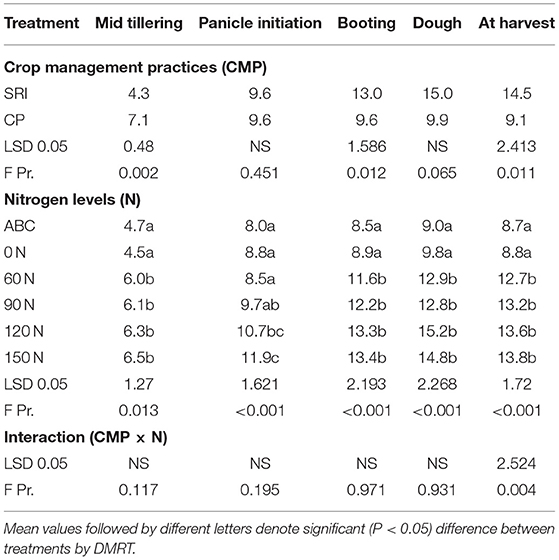
Table 6. Number of tillers during the wet season was affected by crop management practices and nitrogen levels.
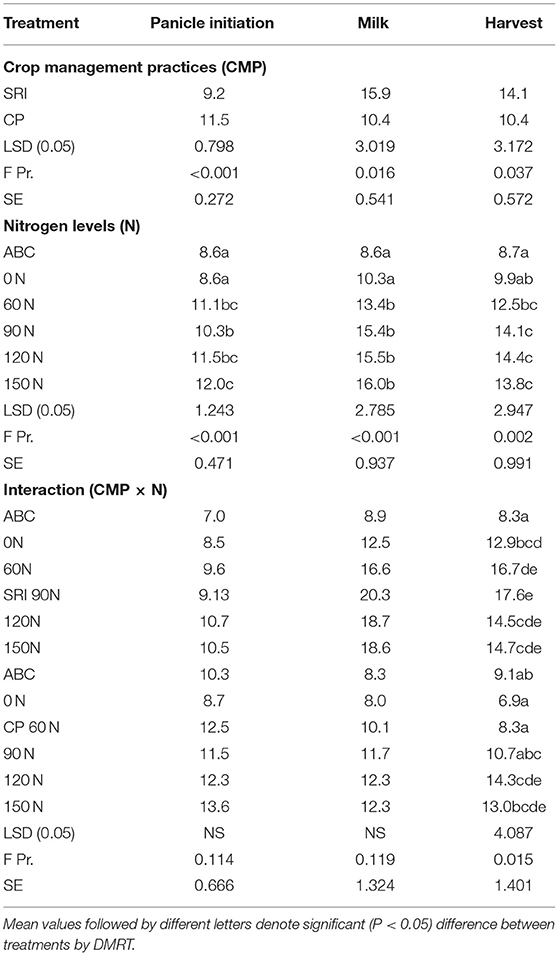
Table 7. Number of tillers during the dry season was affected by crop management practices and nitrogen levels.
Chlorophyll Content
Chlorophyll content was significantly affected (p < 0.05) by CMPs during the panicle initiation stage, and SRI recorded high CC by 9% compared to the CP (Table 8). The increase in CC from panicle initiation to milk stage was observed in both CMPs. Nitrogen levels had a significant effect on CC in both growth stages, and CC increased with increase in nitrogen levels. Treatment interaction had a significant effect on the panicle initiation stage; however, higher content (50.4) was recorded upon the application of 150 kg N ha−1 during the milk stage.
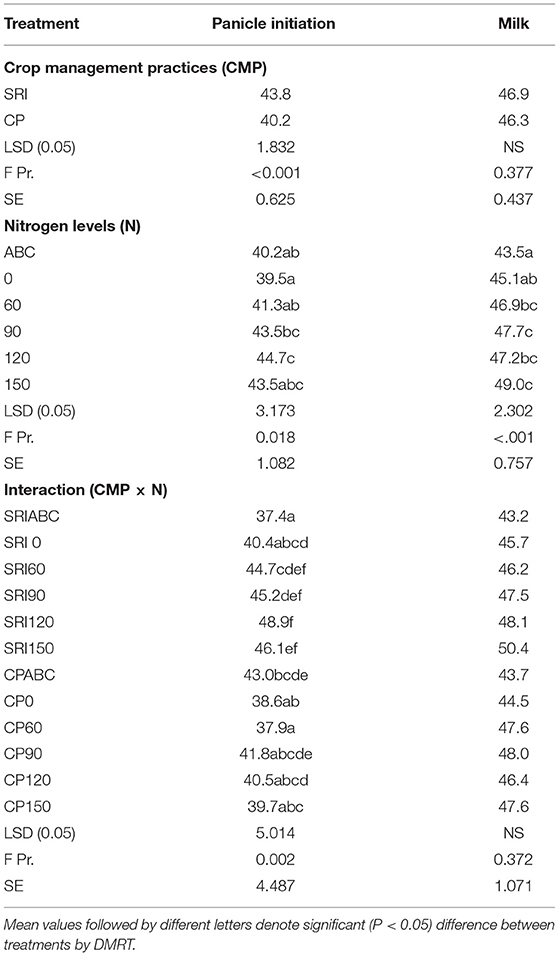
Table 8. Effects of crop management practices and nitrogen levels on leaf chlorophyll content (atLEAF values).
Root Growth Characteristics
The SRI practice affected the root characteristics significantly (p < 0.05) (Table 9). Roots collected from similar soil volumes, growth stage, and season under both practices showed that with SRI practices, hills had significantly fresh weight, length, volume as indicated by increased root dry weight per hill. Root dry weight per hill was 55% higher for SRI than for CP, even though SRI had only one plant hill−1 whereas CP had three plants. Nitrogen levels affected root dry weight significantly, and higher dry weight (13 g) was recorded with 150 kg N. Interaction treatments under SRI × 150 kg N ha reported higher dry weight hill−1 (15.2 g) though the weight was not significant from other treatments.
Yield and Yield Components
The grain yield of SRI was significantly (p < 0.05) greater than that of the conventional practice (CP) and at all levels of nitrogen application (Table 10). Under crop management practices, the average highest rice grain yield was found in the SRI treatments (6.7 and 6.4 t ha−1), and this is 10.7 and 34% higher than that of the CP (6.4 and 4.2 t ha−1) during the wet and dry seasons, respectively. N fertilizer applications always increased the grain yields compared with the zero-N and absolute control in both seasons. Grain yield increased with increase in nitrogen levels; however, there was no yield increase beyond 120 kg N ha−1 in the wet season and 90 kg N ha−1 in the dry season.
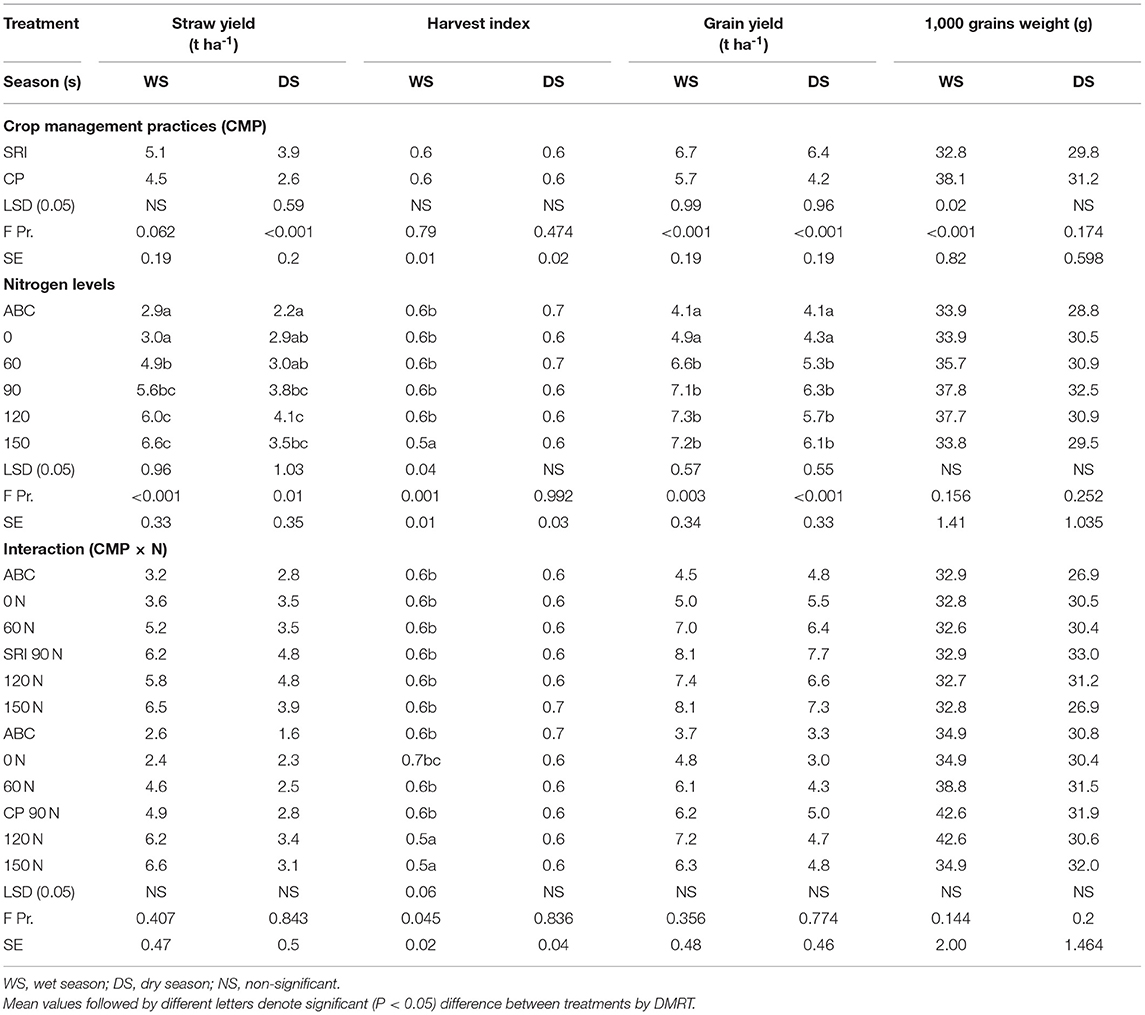
Table 10. Effects of crop management practices and N fertilizer levels on yield components in rice crop.
In the whole range of N application levels, the average yield under SRI management increased by 16.2% during the wet season and 55.6% during the dry season. Among the N treatments, the maximum yield under SRI was 8.1 t ha−1 with the application of 120 and 150 kg N ha−1 in the wet season, and 7.7 tha−1 with 90 kg N ha−1 in the dry season. The maximum yield under the CP was 7.2 t ha−1 with 120 kg N ha−1 in the wet season and 5.0 tha−1 with 90 kg N ha−1 in the dry season. The grain yield achieved under the CP with application of 90–120 kg N ha−1 mostly in the dry season was equivalent to the yield achieved with half or two-thirds as much N fertilizer under the SRI method, i.e., 60 kg N ha−1.
Yield Components
The CMPs significantly affected straw yield during the dry season, and SRI recorded increased yield by 33.3% over CP (Table 10). Straw yield increased with increase in nitrogen levels in both seasons. Highest yield in the wet season (6.6 and 6.5 t ha−1) was recorded in the 150 Kg N ha−1, SRI × 150 kg N ha−1, and CP × 150 Kg N ha−1 treatments. Harvest index (HI) was significantly affected by N levels during the wet season, whereas the lowest HI of 0.5 was recorded in the application of 150 kg N ha−1. No effect was observed on treatment interaction. Thousand grain weight was significant under the CP in the wet season. No significant effect was observed on nitrogen levels and treatment interaction. The CMPs affected panicle weight and spikelet panicle−1 significantly in both seasons, and SRI had higher panicle weight and spikelet panicle−1 (Table 11).
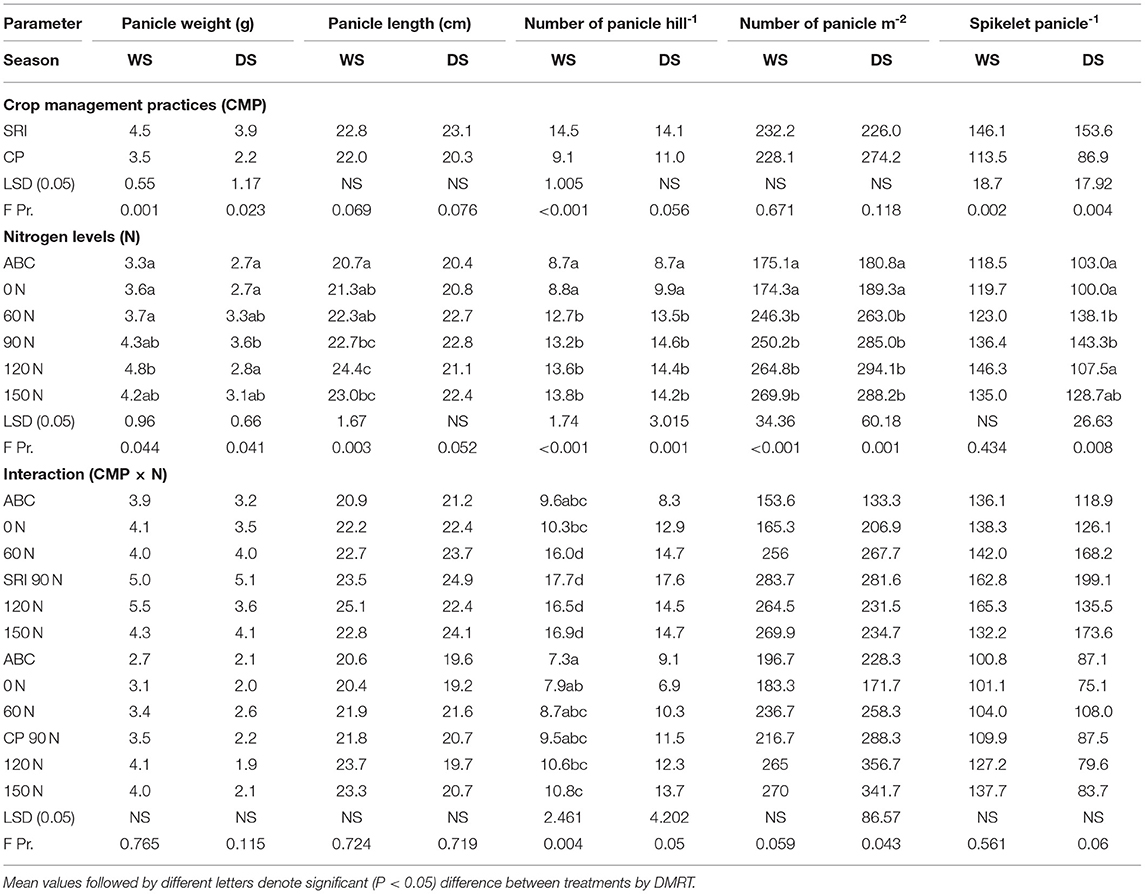
Table 11. Effects of crop management practices and fertilizer N levels on yield components in rice crop.
Number of panicle hill−1 was significant during wet season and SRI recorded 37 and 22% higher over CP in wet and dry seasons. Nitrogen levels and treatment interaction also had a significant effect on number of panicles. Nitrogen levels and CMPs had a significant effect on panicle weight in both seasons; however, no significant effect was observed on treatment interaction. SRI reported higher panicle weight percentage (22.2 and 43.6%) in the wet and dry seasons.
Panicle weight increased with increase in nitrogen levels; however, there was no increase observed beyond 120 kg N−1 in the wet season and 90 kg N ha−1 in the wet and dry seasons. Panicle length was significantly (p < 0.05) affected by nitrogen levels, and the length increased with increase in nitrogen levels as in the wet season.
Number of panicle per hill was significantly (p < 0.05) affected by the CMPs in the wet season, and SRI recorded higher (14.5) number of panicle per hill than the CP (9.1). Nitrogen levels and treatment interaction had significant effects on number of panicles per hill.
Number of panicle per square meter was significantly (p < 0.05) influenced by nitrogen levels, and an increase was observed with increase in nitrogen levels. Spikelet per panicle was significantly influenced by the CMPs, and SRI had higher number of spikelets per panicles (146.1, 153.6) than the CP (113.5, 86.9) in the wet and dry seasons. Nitrogen levels also had a significant influence on number of spikelets per panicles during the dry season, and higher number of spikelet's (143.3) was recorded in 90N. Effective tillers were significantly affected by crop management, nitrogen levels, and their interaction (p < 0.05) in both seasons, and SRI recorded higher effective tillers than the CP (Table 12). Filled grain panicle−1 was significantly affected by the CMPs (p < 0.05) in both seasons.
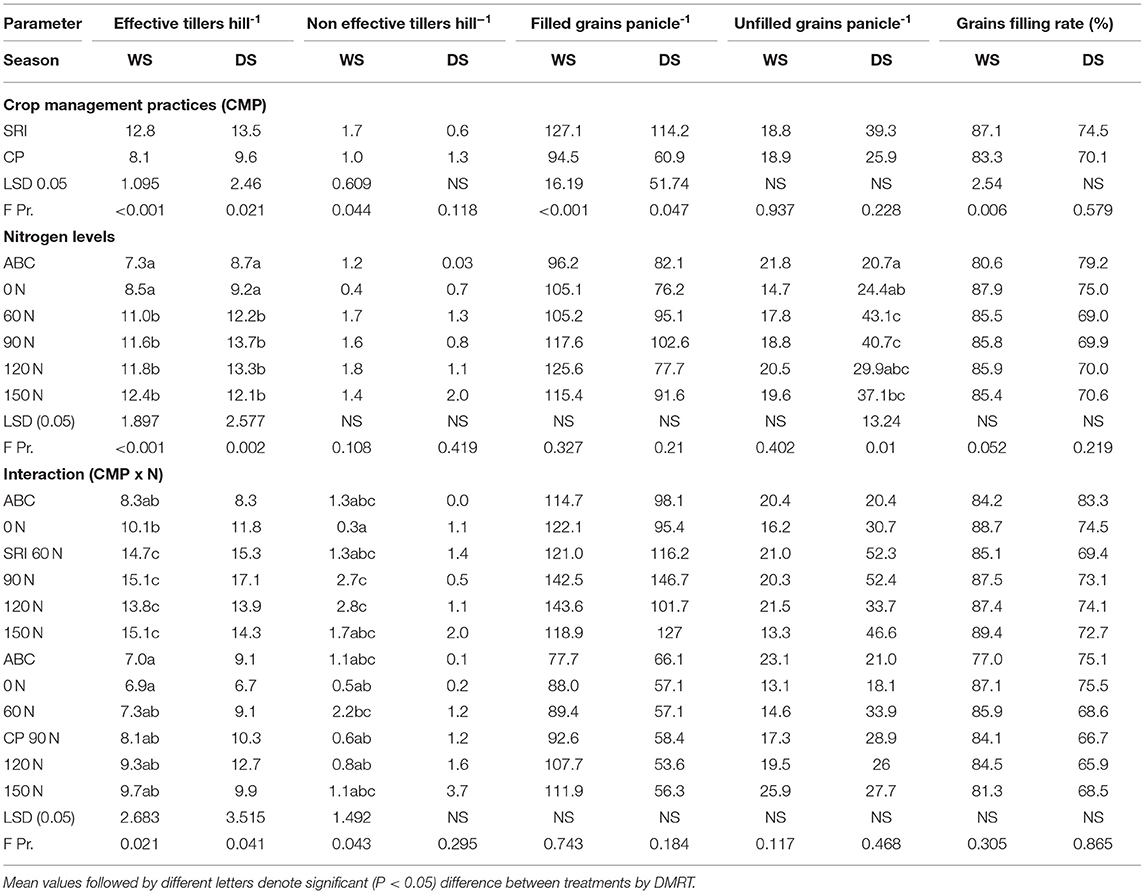
Table 12. Effects of crop management practices and fertilizer N levels on yield components in rice crop.
Grains filling rate was significantly affected by the CMPs in the wet season, and SRI increased it by 4.6, 5.9% compared to the CP in the wet and dry seasons. There was also a significant effect on nitrogen levels in the dry season. Belder et al. (2004) and Zheng et al. (2020) found that percentage of filled grains was not significantly affected by the cultivation methods.
Nitrogen Use Efficiency
Nitrogen application rate had a significant effect (p < 0.05) on agronomic N use efficiency (ANUE) (Table 13). As N rate increased beyond 90 kg N ha−1, the ANUE and PFP under both SRI and CP significantly decreased in both seasons. Zhao et al. (2009) and Djaman et al. (2018) reported a similar trend. Agronomic N use efficiency (ANUE) ranged from 19.7 to 33.7 kg grain kg−1 N in the wet season and from 7.08 to 25.83 kg grain kg−1 N in the dry season under SRI. Under the CP, the ANUE ranged from 10 to 21.7 kg grain kg−1 N in the wet season and from 12 to 21.85 kg grain kg−1 N in the dry season. Highest ANUE was found with the application rate of 90 kg N ha−1 under both SRI and CP in both seasons. The ANUE of the SRI plants increased by 51.2–121.7% in the wet season and 20.7–18.2% in the dry season compared to the CP plants (Table 13).
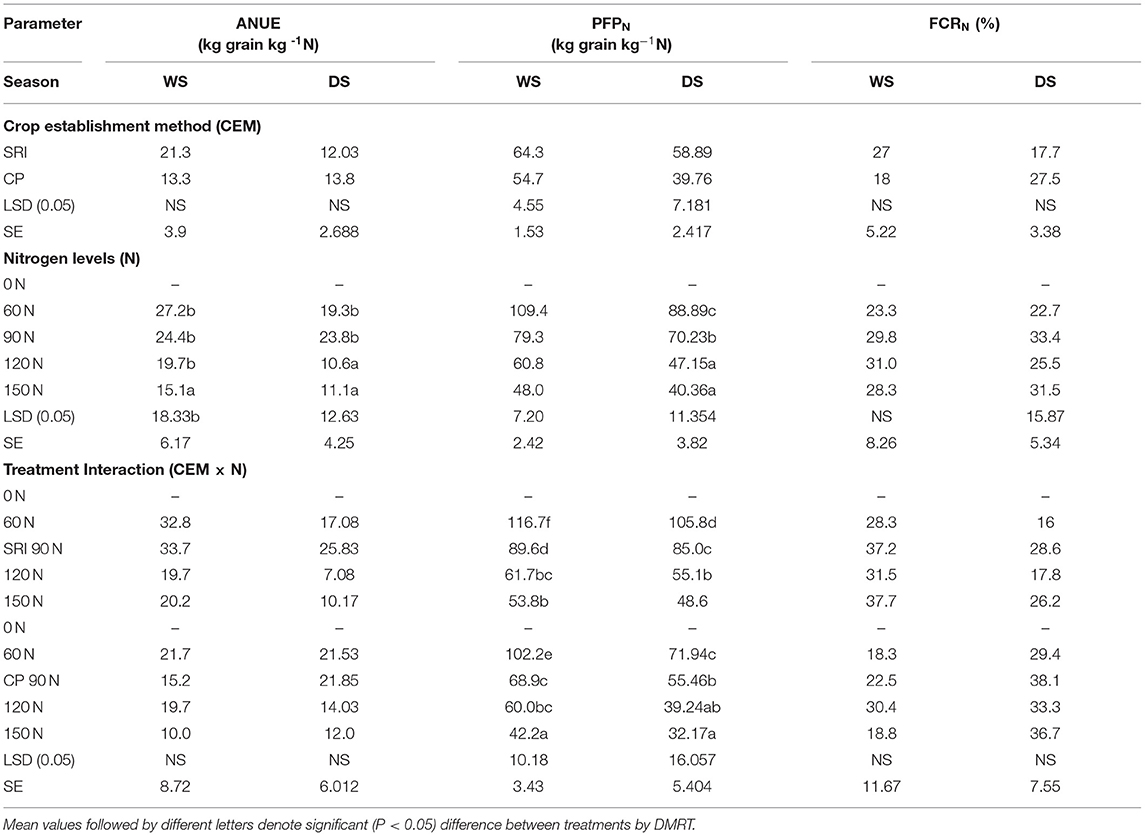
Table 13. Effect of crop management practices and nitrogen fertilization on nitrogen use efficiency.
The results of this study are in agreement with other studies. Zhao et al. (2009), Thakur et al. (2013), and Zhang et al. (2020) reported decrease in PFP and AUNE with increase in nitrogen dose. Djaman et al. (2018) reported a range of ANUE of 20.6–159.1 kg grain kg−1 N, and Zhao et al. (2009) found that rice NUE was affected by SRI integrated with AWD, and that NUE varied from 2 to 17.9 kg grain kg−1 N and 7.1 to 13.1 kg grain kg−1 N in SRI and traditional flooding, respectively. Thakur et al. (2013) reported that the value of ANUE ranged from 31.3 to 44.3 kg grain kg−1N under SRI, and from 23.3 to 31.6 kg grain kg−1N under transplanted flooded rice, and that ANUE with SRI was 34–40% higher than in transplanted flooded rice plants. Cassman and Pingali (1996) reported a farmer field NUE range of 15–20 in the Philippines. Low NUE (9.1 and 6.4 kg grain kg−1 N) has been reported in farmers' fields in China by Peng et al. (2002) as compared to global average of 20 kg grain·kg−1N.
Partial factor productivity (PFP) was significant (p < 0.05) affected by CMPs, nitrogen levels, and their interaction. SRI had PFP ranges of 53.8–116.7 and 48.6–105.8 kg grain kg−1N and CP 42.2–102.2 and 32.17–71.94 kg grain kg−1N during the wet and dry seasons.
Highest PFP was found with the application rate of 60 kg N ha−1 under both SRI and CP as well as N levels in both seasons. However, there was decrease in PFP with increase in N levels beyond 60 kg N ha−1 in both nitrogen treatments and the interaction. From applied nitrogen PFP was 17.7% in wet and 48.2% in dry season and was higher with SRI management when compared to CP. Under SRI, 80.5, 73.6 kg grain was produced with the application of 1 kg N, whereas under CP, only 68.3, 49.7 kg grain resulted per kg N applied in the wet and dry seasons.
As for nitrogen contribution rate, it was in the range of 28–37.7 and 16–28.6% under SRI in the wet and dry seasons, respectively. Under the CP, the range was 18.3–30.4 and 29.4–38.1% in the wet and dry seasons, respectively. Nitrogen levels affected FCRN significantly during both seasons. The maximum was 38.1%, and the lowest, 16%, was recorded under CP150N and SRI 60N. Alternate wetting and drying under SRI is believed to improve oxygen supply to rice roots, thereby decreasing aerenchyma formation, thus causing a stronger, healthier root system with potential advantages for higher nutrient uptake (Hazra and Chandra, 2016). Also, the drying and re-watering cycle in AWD affects biochemical and physical processes, namely, nitrification, denitrification, mineralization, percolation, and leaching, in soil by changing soil water and air equilibrium, which in turn affects the availability of nitrogen nutrition.
Discussion
Nitrogen is involved in cell division and elongation of various internodes, especially basal internodes of rice stems, which lead to increase in plat height (Mazumder et al., 2019; Zhang et al., 2020; Mboyerwa et al., 2021). Transplanting young seedlings under SRI reduces transplant shock because of lesser leaf area during the initial growth stages, which stimulate increased cell division causing more stem elongation resulting in increased plant height as reported by Vijayakumar et al. (2006). Also, the wider spacing in SRI plants enables the plants to get a sufficient space to grow, and increase in light transmission in the canopy leads to increased plant height. This was reported by Shrirame et al. (2000) who found the number of functional leaves, leaf area, and total number of tillers hill−1 to be higher at wider spacing, which increased the photosynthetic rate leading to taller plants. Uphoff (2001) found that water-saving irrigation combined with mechanical weeding enhanced plant height by better aeration, and that incorporation of weeds as a green manure increased the organic carbon content of the soil. Incorporation of weeds with a mechanical weeder increased root activity, which stimulated new cell division in roots by pruning of some upper roots that encouraged deeper root growth, thereby increasing shoot:root ratio.
Increase in number of tillers could be associated with wide spacing (less competition), aeration due to wetting and drying cycles, and root volume that has repercussion in nutrient use and yield increase. An increase in the number of tillers and plant height of SRI plants has been reported in studies conducted in Kahimba et al. (2013); Katambara et al. (2013); Reuben et al. (2016), and Mkindo by Kangile et al. (2018). The lower number of tillers in CP plants could be the case of narrow environment and high plant density per hill with high competition for nutrients and energy among plants.
The high CC in SRI plants was attributed to the higher root-oxidizing activity of more widely spaced rice plants that improves nitrogen uptake and forms part of chlorophyll. CC has been recorded higher in plants grown under wider spacing (30 × 30 cm) than those grown under narrow spacing (20 × 20 cm) (Mishra and Salokhe, 2010). Thakur et al. (2010) reported that canopies in SRI plants had highest leaf area index (LAI) and light interception; these contributed to the maintenance of higher chlorophyll levels, enhanced fluorescence and photosynthesis rates of leaves, and supported more favorable yield attributes and grain yield in individual hills. The study conducted by Hidayati and Anas (2016) reported an improvement in vegetative and generative growth of rice plants under the SRI method due to increase in photosynthesis rate, high chlorophyll content, and increase in nutrient uptake and grain yield.
System of rice intensification management practices significantly affected the root fresh and dry weights, root volume, and root length. Naher et al. (2009) and Thakur et al. (2013) reported that root enhancement also facilitates other physiological processes. Some of these are increases in concentrations of cytokinin in roots and shoots and root oxidation activities, leaf photosynthetic rates, and in the activities of key enzymes involved in sucrose-to-starch conversion in grains. SRI plants tend to form a profuse root system with little or late senescence that enhances the opportunity for beneficial interaction of soil microbes. Also, this enables plant roots to reach the lower horizons and continue taking up nutrients until the end of the cycle; Chen et al. (2021) reported increased K+ concentration in shoots and grains of SRI plants compared to plants grown under continuous flooding practice. It has been reported that at flowering, 78% of roots growing under anaerobic soil conditions had degenerated, and that some rice roots growing under aerobic soil conditions were affected (Hazra and Chandra, 2016). A significant effect of SRI-N root characteristics has been reported by other researchers. Thakur et al. (2013) found an increase of up to 66% of dry weight per hill compared to transplanted flooded rice in the flowering stage. Zhang et al. (2009), and Thakur et al. (2011), reported enhanced root development in alternate wetting and moderate drying soil water regimes, as followed in SRI practice. Ndiiri et al. (2012) reported doubling of root dry weight under SRI compared to continuous flooding.
The grain yield in this study was affected by both the crop establishment methods and the nitrogen fertilization levels applied. The average grain yield in SRI was within the expected yield potential range for the TXD 306 variety (7–8 t ha−1), while the average yield with the CP was below. The increment in grain yield in the SRI treatment was largely attributed to increases in the number of spikelets per panicle and filled grain percentage. The yield of SRI plants could also be linked with root characteristics, i.e., higher volume and root weight. The SRI plants recorded higher root volume and higher length and weight; this increased nitrogen use by plants. Our results are in agreement with previous studies of other researchers, i.e., Sandhu et al. (2017) reported a strong association of root traits such as nodal root number and root dry weight with grain yield. Ashraf et al. (1999) reported that old seedling results in lower rice yields because they suffer from stem and root injury during pulling. Previous studies in the study area with the same variety reported various rice yields. Kahimba et al. (2013) reported a 2.96–4.76 t ha−1 yield, increased by 24.3%, in SRI compared to conventional practices, and Reuben et al. (2016) reported that grain yield ranged from 8.1 to 8.5 t ha−1 under SRI with the same variety. Kangile et al. (2018) recorded the yield of four varieties, namely, TXD 88, TXD 307, TXD 306, and SUPA, as 9.1,8.7,8.5, and 6.2 t ha−1, respectively, and found that the yield of all the varieties were doubled. Thakur et al. (2021) reported increased rice yield by SRI of up to 25–50% or more, and Mati et al. (2021) reported increased rice yields of between 20 and 100% in Kenya. Yang et al. (2007) and Zhang et al. (2009) reported an increase in rice yield of SRI plants by ~10% relative to continuous flooding. Thakur et al. (2014) found that overall, grain yield by SRI was 49% higher than with CP, with the yield enhanced by every N application dose. Qin et al. (2010), Ye et al. (2013), and Islam et al. (2020) observed an increase in rice yields under SRI practices. Sato and Uphoff (2007) summarized the results of 12,133 comparison trials covering 9,429 ha and found that average yield increase was 78% (3.3 t/ha) in SRI compared to conventional practice.
In case of NUE, the results of this study are in agreement with Thakur et al. (2013) who found that NUE and partial factor productivity from applied N were significantly higher in SRI plants than in transplanted flooded rice plants.
Espiritu and Javier (2013) reported a PFP range of 65.7–414.0 kg grain kgN−1 N reported a variation in PFPN between sites and the average PFPN was 52.5 kg grain kg−1N in Changsha, 39.4 kg grain kg−1N in Huaiji, Binyang, and Haikou, and 66.4 kg grain kg−1N in Xingyi. Zhu et al. (2016) reported a rice PFPN that ranged from 26.9 to 69.1 kg grain kg−1N in Hubei province (China), and Yang (1999) reported a range of 29.0–83.1 kg grain kg−1N, with the highest PFPN achieved under moderate AWD treatment.
Conclusion
Rice grown under the system of rice intensification (SRI) management practice used nitrogen fertilizer more efficiently because of profuse root development and improved physiological performance resulting in enhanced grain yield compared to the conventional practice.
This indicates that there is a systematic interaction with lower plant density (single seedling per hill) in combination with alternate wetting and drying (water saving irrigation) and/or N fertilization.
The SRI improved rice root development characteristics, effective tillers, filled grains per panicle, grains filling rate, panicle weight, spikelet per panicle, straw and grain yields, and 1,000-grain weight.
The SRI affected grain yield significantly, because grain yield was increased by 10.7 and 34% compared to that of the CP during the wet and dry seasons, respectively.
Grain yield was increased with increase in nitrogen levels; however, there was no yield increase beyond 120 kg N ha−1 in the wet season and 90 kg N ha−1 in the dry season with both practices.
Higher PFPN and FCRN was achieved at a relatively low N fertilizer rate (60 kg N ha−1), and higher ANUE was achieved at 90 kg N ha−1 with the SRI.
The results of this study indicated that under SRI management, grain yield and NUE could be increased significantly by applying 60 kg N ha−1 while at the same time greatly economizing on the use of N fertilizer in conventional practice.
Data Availability Statement
The original contributions presented in the study are included in the article/supplementary material, further inquiries can be directed to the corresponding author/s.
Author Contributions
PAM set and managed the experiments, collected the data, and analyzed and wrote the first draft of manuscript. KK, PM, and AA reviewed and edited the manuscript. All authors have read and agreed to the published version of the manuscript.
Funding
This study was sponsored by the Word Bank through the project entitled Climate Smart Agriculture and Biodiversity Conservation Led by the Africa Center of Excellence for Climate Smart Agriculture and Biodiversity Conservation at the Haramaya University, Ethiopia.
Conflict of Interest
The authors declare that the research was conducted in the absence of any commercial or financial relationships that could be construed as a potential conflict of interest.
Publisher's Note
All claims expressed in this article are solely those of the authors and do not necessarily represent those of their affiliated organizations, or those of the publisher, the editors and the reviewers. Any product that may be evaluated in this article, or claim that may be made by its manufacturer, is not guaranteed or endorsed by the publisher.
References
Amuri, N., Semoka, J., Ikerra, S., Kulaya, I., and Msuya, B. (2013). “Enhancing use of phosphorus fertilizers for maize and rice production in small scale farming in eastern and northern zones, Tanzania,” in A Paper Presented at the 27th Soil Science Society of East Africa-6th African Soil Science Society Conference, 21st to 25th October 2013, Nakuru, Kenya, Department of Soil Science and Department of Agricultural Education and Extension, Sokoine University of Agriculture, 1–12.
ARI-Mlingano (2006). Soils of Tanzania and their Potential for Agriculture Development. Mlingano Agricultural Research Institute, Department of Research and Training, Ministry of Agriculture, Food Security and Co-Operatives, Tanga, Tanzania. Available online at: http://unfccc.int/national_reports/items/1408.php (accessed August 8, 2018).
Ashraf, M., Khalid, A., and Ali, K. (1999). Effect of seedling age and density on growth and yield of rice in saline soil. Pakistan J. Biol. Sci. doi: 10.3923/pjbs.1999.860.862
Belder, P., Bouman, B. A. M., Cabangon, R., Guoan, L., Quilang, E. J. P., Yuanhua, L., et al. (2004). Effect of water-saving irrigation on rice yield and water use in typical lowland conditions in Asia. Agric. Water Manage. 65, 193–210. doi: 10.1016/j.agwat.2003.09.002
Cassman, K. G., and Pingali, P. L. (1996). “Extrapolating trends from long-term experiments to farmers' fields: the case of irrigated rice systems in Asia,” in Agricultural Sustainability in Economic, Environmental and Statistical Terms, eds V. Barnet, R. Payne, R. Steiner (London: Wiley), 63–68.
Chen, C., Travis, A. J., Hossain, M., Islam, M. R., Price, A. H., and Norton, G. J. (2021). Genome-wide association mapping of sodium and potassium concentration in rice grains and shoots under alternate wetting and drying and continuously flooded irrigation. Theor. Appl. Genet. 134, 1–20. doi: 10.1007/s00122-021-03828-9
Chen, T., Wilson, L. T., Liang, Q., Xia, G., Chen, W., and Chi, D. (2017). Influences of irrigation, nitrogen and zeolite management on the physicochemical properties of rice. Arch. Agron. Soil Sci. 63, 1210–1226. doi: 10.1080/03650340.2016.1276286
Congreves, K. A., Otchere, O., Ferland, D., Farzadfar, S., Williams, S., and Arcand, M. M. (2021). Nitrogen use efficiency definitions of today and tomorrow. Front. Plant Sci. 12, 637108. doi: 10.3389/fpls.2021.637108
de Bruin, A., Cinderby, S., Mbilinyi, B., Mahoo, H., and Barron, J. (2019). Agricultural water management scenarios in the Mkindo watershed Wami basin, Tanzania. Gates Open Res. 3, 439.
Djaman, K., Mel, V. C., Diop, L., Sow, A., El-Namaky, R., Manneh, B., et al. (2018). Effects of alternate wetting and drying irrigation regime and nitrogen fertilizer on yield and nitrogen use efficiency of irrigated rice in the Sahel. Water 10, 711. doi: 10.3390/w10060711
Espiritu, A. E., and Javier, E. F. (2013). Nitrogen use efficiency of different organic fertilizers applied in paddy rice. Philippine J. Crop Sci. 38, 81–82.
FAOSTAT (2014). Statistical Databases. Rome: Food and Agriculture Organization of the United Nations.
Galloway, J. N., Winiwarter, W., Leip, A., Leach, A. M., Bleeker, A., and Erisman, J. W. (2014). Nitrogen footprints: past, present and future. Environ. Res. Lett. 9, 115003. doi: 10.1088/1748-9326/9/11/115003
Gathorne-Hardy, A., Reddy, D. N., Venkatanarayana, M., and Harriss-White, B. (2016). System of rice intensification provides environmental and economic gains but at the expense of social sustainability—A multidisciplinary analysis in India. Agric. Syst. 143, 159–168. doi: 10.1016/j.agsy.2015.12.012
Gleissman, S. R. (2014). Agroecology: The Ecology of Sustainable Food Systems, 3rd ed. Boca Raton: CRC Press.
Gomez, K. A., and Gomez, A. A. (1984). Statistical Procedures for Agricultural Research. New York, NY: John Wiley & Sons.
Gowele, G. E., Mahoo, H. F., and Kahimba, F. C. (2020). Comparison of silicon status in rice grown under the system of rice intensification and flooding regime in Mkindo irrigation scheme, Morogoro, Tanzania. Tanzania J. Agric. Sci. 19, 216–226.
Hameed, F., Xu, J., Rahim, S. F., Wei, Q., Liao, Q., and Ahmed, S. (2019). Rice growth and nitrogen uptake simulation by using ORYZA (v3) model considering variability in parameters. Pakistan J. Agric. Sci. 56, 245–259. doi: 10.21162/PAKJAS/19.8155
Hazra, K. K., and Chandra, S. (2016). Effect of extended water stress on growth, tiller mortality and nutrient recovery under system of rice intensification. Proc. Natl. Acad. Sci. India Section B Biol. Sci. 86, 105–113. doi: 10.1007/s40011-014-0415-7
Hidayati, N., and Anas, I. (2016). Photosynthesis and transpiration rates of rice cultivated under the system of rice intensification and the effects on growth and yield. HAYATI J. Biosci. 23, 67–72. doi: 10.1016/j.hjb.2016.06.002
Islam, S. M., Gaihre, Y. K., Biswas, J. C., Jahan, M. S., Singh, U., Adhikary, S. K., et al. (2018). Different nitrogen rates and methods of application for dry season rice cultivation with alternate wetting and drying irrigation: fate of nitrogen and grain yield. Agric. Water Manage. 196, 144–153. doi: 10.1016/j.agwat.2017.11.002
Islam, S. M., Gaihre, Y. K., Islam, M. R., Akter, M., Al Mahmud, A., Singh, U., et al. (2020). Effects of water management on greenhouse gas emissions from farmers' rice fields in Bangladesh. Sci. Total Environ. 734, 139382. doi: 10.1016/j.scitotenv.2020.139382
Jiang, L., Dai, T., Jiang, D., Cao, W., Gan, X., and Wei, S. (2004). Characterizing physiological N-use efficiency as influenced by nitrogen management in three rice cultivars. Field Crops Res. 88, 239–250. doi: 10.1016/j.fcr.2004.01.023
Jumanne, E. (2016). Effects of Flooding and System of Rice Intensification on Nitrogen Use Efficiency in Rice Production at Mkindo, Morogoro, Tanzania. Dissertation for award of MSc Degree at Sokoine University of Agriculture, Tanzania, 65 pp. Available online at: http://suaire.suanet.ac.tz/handle/123456789/2342 (accessed September, 2020).
Kahimba, F. C., Kombe, E. E., and Mahoo, H. F. (2013). The potential of system of rice intensification (SRI) to increase rice water productivity: a case of Mkindo irrigation scheme in Morogoro region, Tanzania. Tanzania J. Agric. Sci. 12, 2.
Kangile, R. J., Ng'elenge, H. S., and Busindeli, I. M. (2018). Socio-economic and field performance evaluation of different rice varieties under system of rice intensification in Morogoro. Tanzania Agricult. Res. Technol. J. 17, 556021. doi: 10.19080/ARTOAJ.2018.17.556021
Katambara, Z., Kahimba, F. C., Mahoo, H. F., Mbungu, W. B., Mhenga, F., Reuben, P., et al. (2013). Adopting the system of rice intensification (SRI) in Tanzania: a review. Agric. Sci. 4, 369–375. doi: 10.4236/as.2013.48053
Lampayan, R. M., Rejesus, R. M., Singleton, G. R., and Bouman, B. A. (2015). Adoption and economics of alternate wetting and drying water management for irrigated lowland rice. Field Crops Res. 170, 95–108. doi: 10.1016/j.fcr.2014.10.013
Massawe, H. I. (2016). Effect of Water Management Systems With Different Nutrient Combinations on Performance of Rice on Soils of Mvumi, Kilosa district, Tanzania Dissertation Foraward of MSc Degree at Sokoine University of Agriculture, Tanzania, 82 p. Available online at: http://41.73.194.142/handle/123456789/1526 (accessed September, 2020).
Materu, S. T., Shukla, S., Sishodia, R. P., Tarimo, A., and Tumbo, S. D. (2018). Water use and rice productivity for irrigation management alternatives in Tanzania. Water 10, 1018. doi: 10.3390/w10081018
Mati, B. M., Nyangau, W. W., Ndiiri, J. A., and Wanjogu, R. (2021). Enhancing production while saving water through the system of rice intensification (sri) in Kenya?s irrigation schemes. J. Agri. Sci. Technol. 20, 24–40.
Mazumder, N. I., Sultana, T., Paul, P. C., and Al Noor, M. M. (2019). Influence of NPK fertilizer and spacing on growth parameters of onion (Allium cepa L. Var. BARI piaz-1). Res. Agric. Livestock Fish. 6, 19–25. doi: 10.3329/ralf.v6i1.41382
Mboyerwa, P. A., Kibret, K., Mtakwa, P. W., and Aschalew, A. (2021). Evaluation of growth, yield, and water productivity of paddy rice with water-saving irrigation and optimization of nitrogen fertilization. Agronomy 11, 1629. doi: 10.3390/agronomy11081629
Mishra, A., and Salokhe, V. M. (2010). Flooding stress: the effects of planting pattern and water regime on root morphology, physiology and grain yield of rice. J. Agron. Crop Sci. 196, 368–378. doi: 10.1111/j.1439-037X.2010.00421.x
Naher, U. A., Radziah, O., Halimi, M. S., Shamsuddin, Z. H., and Mohd Razi, I. (2009). Influence of root exudate carbon compounds of three rice genotypes on rhizosphere and endophytic diazotrophs. Pertanika J. Trop. Agric. Sci. 32, 209–223.
Ndiiri, J. A., Mati, B. M., Home, P. G., Odongo, B., and Uphoff, N. (2012). Comparison of water savings of paddy rice under system of rice intensification (SRI) growing rice in Mwea, Kenya. Int. J. Curr. Res. Rev. 4, 63–73.
Pascual, V. J., and Wang, Y. M. (2017). Impact of water management on rice varieties, yield, and water productivity under the system of rice intensification in Southern Taiwan. Water 9, 3. doi: 10.3390/w9010003
Peng, S., Huang, J., Zhong, X., Yang, J., Wang, G., Zou, Y., et al. (2002). Research strategy in improving fertilizer nitrogen use efficiency of irrigated rice in China. Scientia Agricultura Sinica. 35, 1095–1103 (in Chinese).
Qin, Y., Liu, S., Guo, Y., Liu, Q., and Zou, J. (2010). Methane and nitrous oxide emissions from organic and conventional rice cropping systems in Southeast China. Biol. Fertil. Soil. 46, 825-834. doi: 10.1007/s00374-010-0493-5
Reuben, P., Katambara, Z., Kahimba, F. C., Mahoo, H. F., Mbungu, W. B., Mhenga, F., et al. (2016). Influence of transplanting age on paddy yield under the system of rice intensification. Agric. Sci. 7, 154–163. doi: 10.4236/as.2016.73015
Sandhu, N., Subedi, S. R., Yadaw, R. B., Chaudhary, B., Prasai, H., Iftekharuddaula, K., et al. (2017). Root traits enhancing rice grain yield under alternate wetting and drying condition. Front. Plant Sci. 8, 1879. doi: 10.3389/fpls.2017.01879
Sato, S., and Uphoff, N. (2007). Raising factor productivity in irrigated rice production: opportunities with the system of rice intensification. CAB Rev. Perspect. Agric. Vet. Sci. Nutr. Nat. Resources 54, 2. doi: 10.1079/PAVSNNR20072054
Shrirame, M. D., Rajgire, H. J., and Rajgire, A. H. (2000). Effect of spacing and seedling number per hill on growth attributes and yield of rice hybrids under lowland condition. J. Soils Crops 10, 109–113.
SRI-Rice (2020). SRI-Rice Website. Available online at: http://sri.cals.cornell.edu/countries/index.html (accessed July 5, 2020).
Thakur, A. K., Mandal, K. G., Mohanty, R. K., and Uphoff, N. (2021). How agroecological rice intensification can assist in reaching the sustainable development goals. Int. J. Agric. Sustain. 20, 216–230. doi: 10.1080/14735903.2021.1925462
Thakur, A. K., Mohanty, R. K., Patil, D. U., and Kumar, A. (2014). Impact of water management on yield and water productivity with system of rice intensification (SRI) and conventional transplanting system in rice. Paddy Water Environ. 12, 413–424. doi: 10.1007/s10333-013-0397-8
Thakur, A. K., Rath, S., and Mandal, K. G. (2013). Differential responses of system of rice intensification (SRI) and conventional flooded-rice management methods to applications of nitrogen fertilizer. Plant Soil 370, 59–71. doi: 10.1007/s11104-013-1612-5
Thakur, A. K., Rath, S., Patil, D. U., and Kumar, A. (2011). Effects on rice plant morphology and physiology of water and associated management practices of the system of rice intensification and their implications for crop performance. Paddy Water Environ. 9, 13–24. doi: 10.1007/s10333-010-0236-0
Thakur, A. K., Rath, S., Roychowdhury, S., and Uphoff, N. (2010). Comparative performance of rice with system of rice intensification (SRI) and conventional management using different plant spacings. J. Agron. Crop Sci. 196, 146–159. doi: 10.1111/j.1439-037X.2009.00406.x
Thakur, A. K., and Uphoff, N. (2017). How the system of rice intensification can contribute to climate-smart agriculture. Agron. J. 109, 1163–1182. doi: 10.2134/agronj2016.03.0162
Thakur, A. K., Uphoff, N. T., and Stoop, W. A. (2016). Scientific underpinnings of the system of rice intensification (SRI): what is known so far? Adv. Agron. 135, 147–179. doi: 10.1016/bs.agron.2015.09.004
Uphoff, N. (2001). “Scientific issues raised by the system of rice intensification: a less water rice cultivation system,” in Water Saving Rice Production Systems. Proc. International Workshop on Water Saving Rice Production Systems, eds H. Hengsdijk and P. Bindraban (Nanjing University, China), 69–82.
Uphoff, N., and Randriamiharisoa, R. (2002). Reducing Water Use in Irrigated Rice Production With the Madagascar System of Rice Intensification (SRI). Manila: IRRI. p. 71–87.
Vijayakumar, M. S. R. B., Ramesh, S., Prabhakaran, N. K., Subbian, P., and Chandrasekaran, B. (2006). Influence of system of rice intensification (SRI) practices on growth characters, days to flowering, growth analysis and labour productivity of rice. Asian J. Plant Sci. doi: 10.3923/ajps.2006.984.989
Xu, Y., Gu, D., Li, K., Zhang, W., Zhang, H., Wang, Z., et al. (2019). Response of grain quality to alternate wetting and moderate soil drying irrigation in rice. Crop Sci. 59, 1261–1272. doi: 10.2135/cropsci2018.11.0700
Yang, J., Liu, K., Wang, Z., Du, Y., and Zhang, J. (2007). Water-saving and high-yielding irrigation for lowland rice by controlling limiting values of soil water potential. J. Integr. Plant Biol. 49, 1445–1454. doi: 10.1111/j.1672-9072.2007.00555.x
Yang, L., Wang, Y., Kobayashi, K., Zhu, J., Huang, J., Yang, H., et al. (2008). Seasonal changes in the effects of free-air CO2 enrichment (FACE) on growth, morphology and physiology of rice root at three levels of nitrogen fertilization. Glob. Chang. Biol. 14, 1844–1853. doi: 10.1111/j.1365-2486.2008.01624.x
Yang, R., Tong, J., Hu, B. X., Li, J., and Wei, W. (2017). Simulating water and nitrogen loss from an irrigated paddy field under continuously flooded condition with Hydrus-1D model. Environ. Sci. Pollut. Res. 24, 15089–15106. doi: 10.1007/s11356-017-9142-y
Yang, X. (1999). Characteristics of nitrogen nutrition in hybrid rice. Int. Rice Res. Notes 24, 5–8.
Ye, Y., Liang, X., Chen, Y., Liu, J., Gu, J., Guo, R., et al. (2013). Alternate wetting and drying irrigation and controlled-release nitrogen fertilizer in late-season rice. Effects on dry matter accumulation, yield, water and nitrogen use. Field Crops Res. 144, 212–224. doi: 10.1016/j.fcr.2012.12.003
Zhang, H., Xue, Y., Wang, Z., Yang, J., and Zhang, J. (2009). An alternate wetting and moderate soil drying regime improves root and shoot growth in rice. Crop Sci. 49, 2246–2260. doi: 10.2135/cropsci2009.02.0099
Zhang, J., Tong, T., Potcho, P. M., Huang, S., Ma, L., and Tang, X. (2020). Nitrogen effects on yield, quality and physiological characteristics of giant rice. Agronomy 10, 1816. doi: 10.3390/agronomy10111816
Zhao, C., Chen, M., Li, X., Dai, Q., Xu, K., Guo, B., et al. (2021). Effects of soil types and irrigation modes on rice root morphophysiological traits and grain quality. Agronomy 11,120. doi: 10.3390/agronomy11010120
Zhao, L., Wu, L., Li, Y., Lu, X., Zhu, D., and Uphoff, N. (2009). Influence of the system of rice intensification on rice yield and nitrogen and water use efficiency with different N application rates. Exp. Agric. 45, 275–286. doi: 10.1017/S0014479709007583
Zheng, C., Zhang, Z., Hao, S., Chen, W., Pan, Y., and Wang, Z. (2020). Agronomic growth performance of super rice under water-saving irrigation methods with different water-controlled thresholds in different growth stages. Agronomy 10, 239. doi: 10.3390/agronomy10020239
Keywords: crop improvement, nitrogen, rice intensification, agro ecology, rice, nitrogen fertilization level
Citation: Mboyerwa PA, Kibret K, Mtakwa P and Aschalew A (2022) Rice Yield and Nitrogen Use Efficiency With System of Rice Intensification and Conventional Management Practices in Mkindo Irrigation Scheme, Tanzania. Front. Sustain. Food Syst. 6:802267. doi: 10.3389/fsufs.2022.802267
Received: 26 October 2021; Accepted: 15 June 2022;
Published: 22 July 2022.
Edited by:
Luuk Fleskens, Wageningen University and Research, NetherlandsReviewed by:
S. Rakesh, National Academy of Agricultural Research Management (ICAR), IndiaSayed Mohammad Mohsin, Sher-e-Bangla Agricultural University, Bangladesh
Fabio L. C. Mingotte, Universidade Estadual Paulista - UNESP, Brazil
Copyright © 2022 Mboyerwa, Kibret, Mtakwa and Aschalew. This is an open-access article distributed under the terms of the Creative Commons Attribution License (CC BY). The use, distribution or reproduction in other forums is permitted, provided the original author(s) and the copyright owner(s) are credited and that the original publication in this journal is cited, in accordance with accepted academic practice. No use, distribution or reproduction is permitted which does not comply with these terms.
*Correspondence: Primitiva Andrea Mboyerwa, primitivaandrea@yahoo.com; primitivaandrea@sua.ac.tz