- Smith Laboratory, Department of Plant Science, McGill University, Montreal, QC, Canada
Seed germination and early plant growth are key stages in plant development that are, susceptible to salinity stress. Plant growth promoting microorganisms (PGPMs) produce substances, in their growth media, that could enhance plant growth under more optimal conditions, and or mitigate abiotic stresses, such as salinity. This study was carried out to elucidate the ability of a NaCl tolerant Bacillus amyloliquefaciens strain's cell-free supernatant to enhance germination and radicle length of corn and soybean, under optimal and NaCl stressed growth conditions. Three NaCl levels (0, 50, and 75 mM) and four cell-free supernatant concentrations (1.0, 0.2, 0.13, and 0.1% v/v) were used to formulate treatments that were used in the study. There were observed variations in the effect of treatments on mean radicle length and mean percentage germination of corn and soybean. Overall, the study showed that Bacillus amyloliquefaciens (BA) EB2003 cell-free supernatant could enhance mean percentage germination and or mean radicle length of corn and soybean. At optimal conditions (0 mM NaCl), 0.2% BA, 0.13% BA, and 0.1% BA concentrations resulted in 36.4, 39.70, and 39.91%, increase in mean radicle length of soybean, respectively. No significant observations were observed in mean radicle length of corn, and mean percentage germination of both corn and soybean. At 50 mM NaCl, 1.0% BA resulted in 48.65% increase in mean percentage germination of soybean, at 24 h. There was no observed effect of the cell-free supernatant on mean radicle length and mean percentage germination, at 72 and 48 h, in soybean. In corn however, at 50 mM NaCl, treatment with 0.2% BA and 0.13% BA enhanced mean radicle length by 23.73 and 37.5%, respectively. The resulting radicle lengths (43.675 and 49.7125 cm) were not significantly different from that of the 0 mM control. There was no observed significant effect of the cell-free supernatant on mean germination percentage of corn, at 50 mM NaCl. At 75 mM NaCl, none of the treatments enhanced mean radicle length or mean percentage germination to levels significantly higher than the 75 mM NaCl. Treatment with 1.0% BA, however, enhanced mean percentage germination to a level not significantly different from that of the 0 mM control, at 72 h. Likewise, in corn, none of the treatments enhanced radicle length to lengths significantly higher than the 75 mM control, although treatment with 1.0% BA, 0.13% BA, and 0.1% BA elongated radicles to lengths not significantly different from the 0 mM NaCl control. Treatment with 0.2% BA, 0.13% BA, and 0.1% BA resulted in mean percentage germination significantly higher than the 75 mM NaCl by 25.3% (in all 3), not significantly different from that of the 0 mM NaCl. In conclusion, concentration of cell-free supernatant and NaCl level influence the effect of Bacillus amyloliquefaciens strain EB2003A cell-free supernatant on mean percentage germination and mean radicle length of corn and soybean.
Introduction
Germination and seedling establishment are significant stages of plant growth and development, which if compromised, can have significant effects on overall crop growth, including, quality and quantity of yield. Fast and uniform germination is a desirable characteristic of seed, for commercial purposes. Salinity stress can cause physiological and biochemical changes in germinating seeds, such as inhibition of water uptake during imbibition, which may subsequently affect the germination process, establishment of seedlings and general plant growth (Ibrahim, 2016; El Moukhtari et al., 2020). Na+, Cl−, Mg2+, and ions are the most dominant in saline soils, due their high solubility, and hence, ease of deposition by water, of minerals such as NaCl, MgSO4, CaSO4, MgCl2, KCl, Na2CO3, Na2SO4 and [Na2Mg(SO4)2] (Tanji, 2002), NaCl the dominant in most saline environments (Forni et al., 2017). Salinity may cause osmotic, ionic and oxidative stresses in the seed, which may delay or cause complete failure of a seed to germinate (Rouhi et al., 2011; Ansari and Sharif Zadeh, 2012; Ilangumaran et al., 2021). Osmotic stress may result in reduced activity or denaturation of plant cytosolic and organelle proteins (Forni et al., 2017), decrease of cytosolic and vacuolar volumes which may negatively impact plant growth, due to reduced photosynthesis and increased production of reactive oxygen species (ROS), which may be detrimental to plant cell components and physiology (Forni et al., 2017). Ionic stress, due to accumulation of ions such as Na+, interferes with plant nutrient availability, which may also affect plant growth and yield (Forni et al., 2017). Oxidative stress occurs when there is increase in the production of ROS such as, singlet oxygen, superoxide ions and peroxides, above what is scavenged by plant cells, causing toxicity and subsequent damage to vital plant cells and their components such as proteins, membrane lipids and nucleic acids (Ahmad et al., 2010, 2019; Kohli et al., 2019). Corn and soybean are important crops worldwide and are all considered moderately sensitive to salinity (Mozafariyan et al., 2016; Bai et al., 2018; Diouf et al., 2018), although variation occurs inter- and intra-species.
Irrigation and use of chemicals, has led to increased secondary salinity, which is detrimental to seed germination and subsequent plant growth (Li et al., 2015; Mozafariyan et al., 2016; Bai et al., 2018). A soil is classified as saline when the electrical conductivity of a saturated soil paste extract (ECe) is ≥4 dS m−1, which is equivalent to 40 mM NaCl (US Salinity Laboratory Staff, 1954; Shrivastava and Kumar, 2015; Forni et al., 2017). Unfortunately, globally, due to agricultural practices and climate change, which has resulted in changing rainfall patterns, evapotranspiration and landscape hydrology (Bui, 2013), soil salinization is expected to expand at a rate of 10% annually (Shrivastava and Kumar, 2015), hence an estimated 50% of arable land is projected to be salinity affected by 2050.
Use of PGPM technology, is a potentially sustainable and ecofriendly mechanism of mitigating salinity stress effects on crops (Ilangumaran et al., 2021; Naamala and Smith, 2021a,b). Use of pure microbial derived active compounds is especially a section of PGPM technology, that has currently gained popularity due to ease of patent and certification process compared to live cells (García-García et al., 2020; Naamala and Smith, 2020).
Microorganisms produce metabolites and proteins in their growth environment (exometabolome and exoproteome), for various reasons, such as adaptation mechanism to stressful conditions, signaling purposes, etc. (Zhao et al., 2020; Kazerooni et al., 2021; Subramanian et al., 2021). Improvement in technology has allowed for isolation and independent use of pure active compounds, as plant growth stimulants (García-García et al., 2020; Naamala and Smith, 2021b; Subramanian et al., 2021). A series of steps are usually followed before a compound is discovered. The first step is to test microbial-cell free supernatant for bioactivity, on a crop species of choice. If the cell-free supernatant exhibits bioactivity, the biologically active compound(s) can then be isolated using techniques such as high pressure liquid chromatography (HPLC) and identified using techniques such as mass spectrometry (Gray, 2005; Subramanian et al., 2021). Through such steps, the microbe-to-plant signal compounds thuricn17 and lipochitooligosaccharide (LCO) were isolated in the Smith laboratory and have been reported to successfully enhance plant growth, under stressed and optimal conditions (Souleimanov et al., 2002; Schwinghamer et al., 2015; Subramanian et al., 2016). Research on microbe-derived compounds has gained popularity among researchers, with hopes that compounds could complement, supplement or at least, address some of the inconsistencies associated with the use of PGPM cells, especially under field conditions (Naamala and Smith, 2021a; Subramanian et al., 2021).
This study, therefore, focused on the bioactivity on corn and soybean, of a cell-free supernatant obtained from the salt tolerant Bacillus amyloliquefaciens EB2003A strain, under NaCl stressed and optimal conditions. This particular strain, a property of EVL Inc., was isolated in their laboratories and is a component of the EVL Enhancer solution, a unique plant growth biostimulant developed by the same company (Macouzet, 2016). The aim of the study was to understand whether, when exposed to salinity stress, Bacillus amyloliquefaciens EB2003A produces plant growth promoting substances and excretes these into its growth media.
Materials and Methods
Obtaining Microbial Cell-Free Supernatant and Formulation of Treatments
Bacillus amyloliquefaciens strain EB2003A and its growth media (M13) were provided by EVL Inc. (http://www.evl-global.com). To obtain the cell-free supernatant, the Bacillus amyloliquefaciens EB2003A cells were cultured in sterilized M13 medium, supplemented with 200 mM NaCl, and incubated for 48 h, at 30°C and 120 rpm. At 48 h, the bacteria culture was centrifuged for, for 10 min, at 10,000 rpm and 4°C, to pellet the bacterial cells and separate them from the cell-free supernatant (Gray, 2005; Gray et al., 2006; Subramanian et al., 2021). The cell-free supernatant was further filtered using 0.22 μm nylon filters to remove any microbial cells remaining after centrifugation.
Three NaCl (0, 50 and 75 mM NaCl) and four cell free supernatant concentrations [1.00, 0.20, 0.13, and 0.10% (v/v)] were dissolved in distilled water to formulate treatments (30 mL each) that were used in the study. Table 1 shows the quantity of water, microbial cell-free supernatant and NaCl, mixed to formulate 30 mL of each of the different treatments used in the study. For each microbial cell-free supernatant concentration, a similar concentration of M13 bacterial growth medium (not inoculated with bacteria), was used as a positive control. The different treatments and controls per experiment are presented in Table 2.
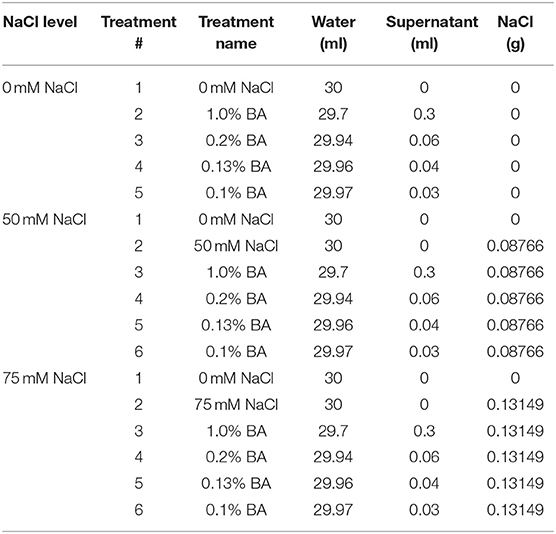
Table 1. Quantity of water, microbial cell-free supernatant and NaCl, mixed together to obtain 30 mL of each treatment.
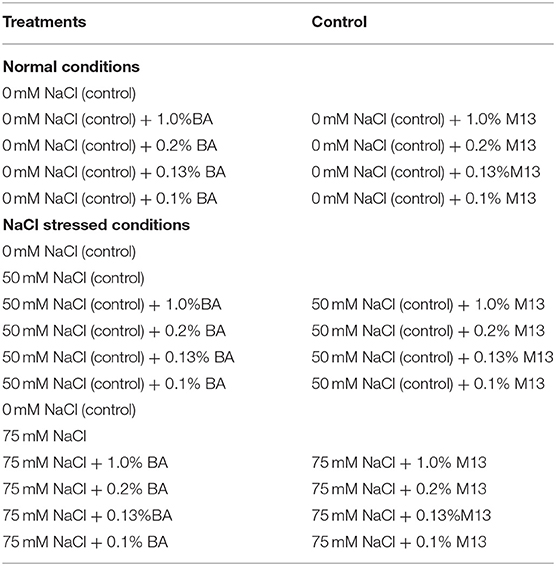
Table 2. The treatments and controls used in the study. BA refers to Bacillus amyloliquefaciens cell-free supernatant, M13 refers to M13 bacterial growth medium.
Percentage Germination and Total Radicle Length
Soybean (cultivar P0962X) and corn (Hybrid 25M75) were used to study the effect of the cell-free supernatant on germination and radicle length, under optimal (0 mM NaCl) and NaCl stressed conditions (50 and 75 mM NaCl). The ability of microbial derived compounds to enhance plant growth varies between and within crop species, hence, more than one crop was studied, to increase the possibility of observing bioactivity. A different experiment was carried for each crop species and even then, every NaCl level was studied separately. In summary, 3 separate experiments, corresponding to each NaCl level, were conducted per plant species and data for each experiment were analyzed separately. Ten seeds per species were surface sterilized using 2% sodium hypochlorite, for 2 min, rinsed with 5 changes of sterilized distilled water and placed on petri-plates (Cat.no. 431760, sterile 100 × 15 mm polystyrene Petri dish, Fisher Scientific Co., Whitby, ON, Canada), lined with filter article (09-795D, QualitativeP8, porosity coarse, Fisher Scientific Co., Pittsburg, PA, USA). Petri plates with seeds were then randomly applied to the treatments as shown in Table 1, with four replicates per treatment, in a completely randomized design (CRD). The Petri plates were then sealed with parafilm and incubated for 7 days in the dark, at a temperature of 25°C. Total number of germinated seeds per plate was recorded at 24 h intervals, for 72 h, as a percentage of the total number of seeds in the plate. i.e., (× /10) * 100. After 7 days, radicle length was measured, in centimeters (cm). For each replicate, radicle length for all the germinated seeds, was summed, to obtain total length of germinated seeds per plate. Each experiment was repeated twice. Percentage germination data for each time interval (24, 48, and 72 h) were analyzed separately.
Data Analysis
Data from the two sets of experiments were pooled and analyzed using PROC GLM (SAS 9.4). Type III tests were used to determine effects of treatments on seed germination while differences between the treatments were assessed using a student t-test with the least square means (LSMEANS) statement, with Tukey's adjustment for multiple comparisons. Differences were considered significant at p ≤ 0.05.
Results
0 mM NaCl (Optimal Conditions)
Soybean
There were observed variations in the effect of treatments on mean percentage germination and mean radicle length of soybean. Treatment with the different concentrations of microbial cell-free supernatant resulted in increase in observed mean radicle length of soybean seedlings. Concentrations 0.2% BA, 0.13% BA, and 0.1% BA resulted in 36.4, 39.7, and 39.91% increases in mean radicle length, respectively, significantly higher than the negative control. Although not significantly different, the concentration 1.0% BA, resulted in mean radicle length higher that of the negative control by 24.74%. The highest mean radicle length (109.08 cm) was observed in seedlings treated with 0.1% BA, while the lowest (67.35 cm) was observed in seedlings treated with 1.0% M13. Positive controls 0.13 and 0.1% M13 also resulted in radicle lengths significantly longer than the negative control. Treatment 0.2% BA (103.04 cm) was significantly higher than the negative control and its corresponding positive control, 0.2% M13 as shown in Figure 1. Table 3 shows the effect of treatment on mean radicle length and mean percentage germination of soybean exposed to 0, 50 and 75 mM NaCl, at 72 h.
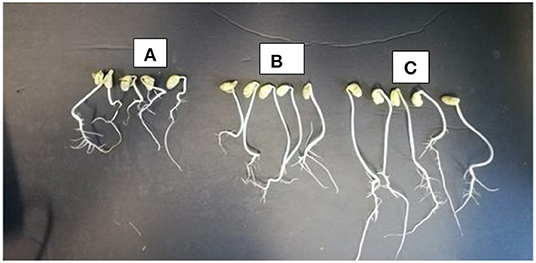
Figure 1. Radicle length of soybean seedlings treated with 0 mM NaCl (A), 0 mM NaCl + 0.20% M13 (B), and 0 mM NaCl + 0.2% BA (C).
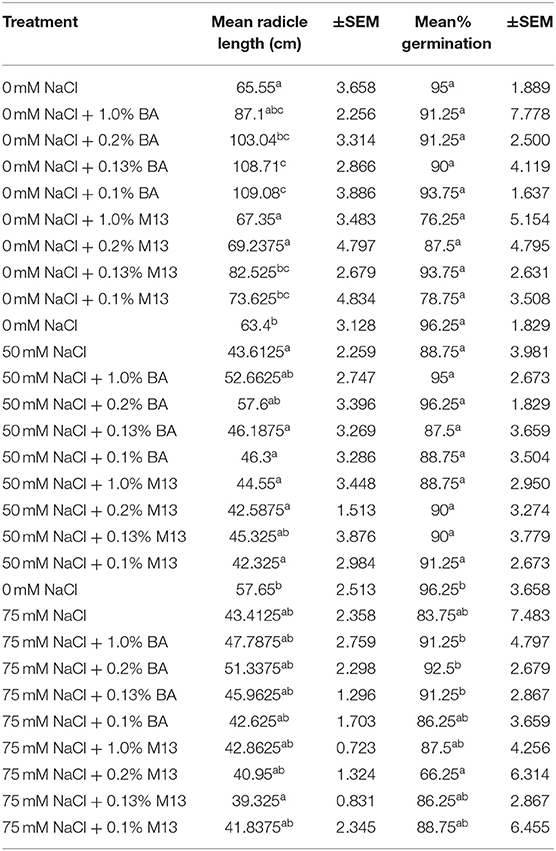
Table 3. Effect of treatment on mean radicle length and mean percentage germination of soybean exposed to 0, 50, and 75 mM NaCl, for 72 h.
Treatments had no significant effect on mean percentage germination, at 24, 48, and 72 h. The mean percentage germination of the positive control was highest but not significantly different from that of the other treatments. However, it is worth noting that, at 72 h, the mean germination percentage of soybean treated with BA cell free supernatant was more uniform (91.25, 91.25, 90, and 93.75%,) compared to the positive controls (76.25, 87.5, 93.75, and 78.75%,). A similar observation was also made at both 48 and 24 h. Soybean treated with BA cell free supernatant was higher and more uniform (ranging between 85 and 92.5%), compared to that soybean treated with M13 media, which ranged between 73.75 and 87.5%, at 48 h. The highest percentage was observed in soybean treated with 0.1% BA while the lowest was observed in soybean treated with 1.0% M13. At 24 h, the highest mean percentage germination (58.75%) was observed in soybean treated with 1.0% BA while the lowest (37.5%) was observed in soybean treated with 0.1% M13. Overall, result suggest that, at 0 mM NaCl, Bacillus amyloliquefaciens cell-free supernatant significantly enhances mean radicle length and may enhance uniformity, and higher mean percentage germination, in soybean. Tables 4, 5 show the effect of treatment on mean percentage germination of soybean exposed to 0, 50 and 75 mM NaCl, for 48 and 24 h, respectively.
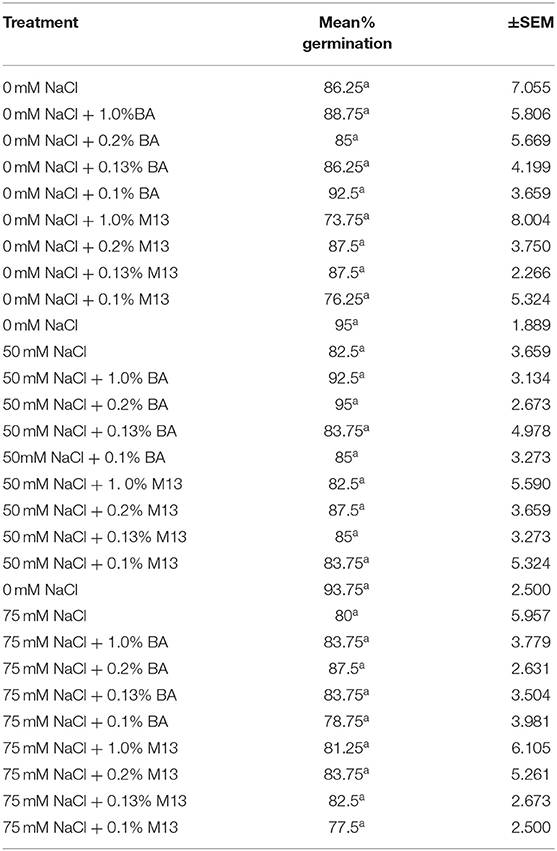
Table 4. Effect of treatment on mean percentage germination of soybean exposed to 0, 50, and 75 mM NaCl, for 48 h.
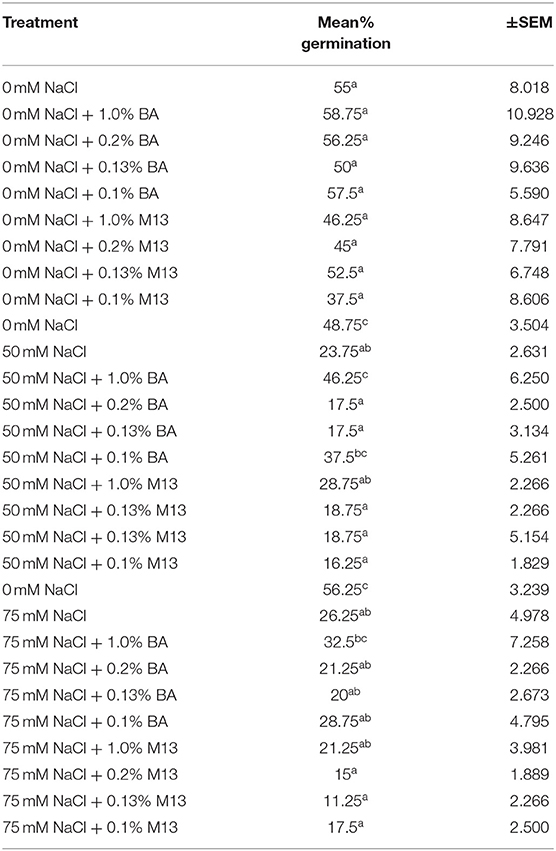
Table 5. Effect of treatment on mean percentage germination of soybean exposed to 0, 50, and 75 mM NaCl, for 24 h.
Corn
There was an observed significant difference in the effect of the treatments on mean radicle length of corn. None of the treatments raised mean radicle length to levels significantly higher than the negative control. However, treatment with 0.1% BA resulted in radicles longer than the control by 5.36%. Interestingly, treatment with 1.0% M13 lowered mean radicle length by 15.4875%, significantly lower than the negative control. Radicle length of corn treated with 1.0% BA was significantly lower than that of corn treated with 0.1% BA by 25.95%, suggesting that the lower the concentration of the cell free supernatant, the more effective it is at enhancing radicle length in corn. Note that the radicle length of corn treated with 0.13% (58.2875 cm) is higher than that of corn treated with 1.0% BA and lower than that of corn treated with 0.1% BA. Overall, there was no significant effect of microbial cell-free supernatant on mean radicle length of corn. Table 6 shows the effect of treatment on mean radicle length and mean percentage germination of corn exposed to 0, 50 and 75 mM NaCl, for 72 h.
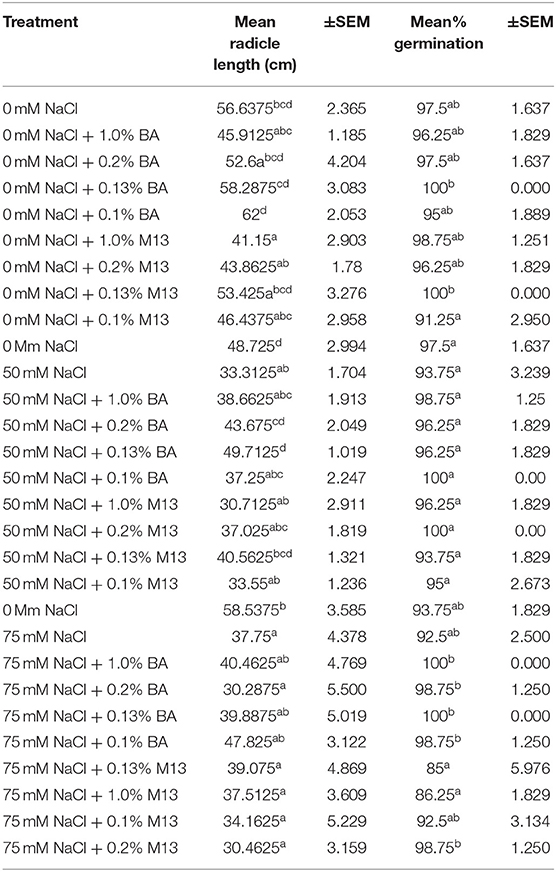
Table 6. Effect of treatment on mean radicle length and mean percentage germination of corn exposed to 0, 50, and 75 mM NaCl, for 72 h.
The effect of treatments on mean percentage germination varied with time. At 72 h, there was no observed significant difference in the effect of treatments on mean percentage germination of corn. The highest mean percentage germination (100%) was observed in corn treated with 0.13% BA and 0.13% M13, while the lowest was observed in corn treated with 0.1% M13. At 48 h, the effect of treatments on mean percentage germination was not significantly different. The highest mean percentage germination was 96.25%, observed in corn treated with 0.13% M13 while the lowest was 86.25%, observed in corn treated with 0.2% M13. Table 7 shows the effect of treatment on mean percentage germination of corn exposed to 0, 50 and 75 mM NaCl, for 48 h.
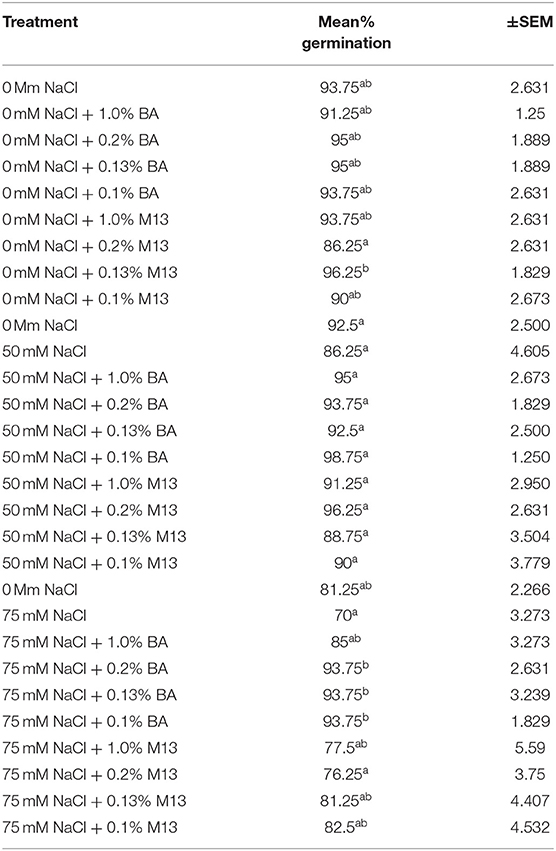
Table 7. Effect of treatment on mean percentage germination of corn exposed to 0, 50, and 75 mM NaCl, for 48 h.
At 24 h, significant differences were observed in the effect of treatments on mean percentage germination. Corn treated with 1.0% BA, 0.2% BA, and 0.13% BA enhanced mean percentage germination by 36.842, 46.66, and 48.94%, respectively. The resulting mean percentage germinations were significantly higher than the control. Likewise, corn treated with 0.2% M3, 0.13% M13, and 0.1% M13 exhibited mean percentage germination significantly higher than the control by 47.83, 47.83, and 36.842%, respectively. The highest mean percentage germination was 58.75%, observed in corn treated with 0.13% BA while the lowest was 33.75%, observed in corn treated with 1.0% M13. Table 8 shows effect of treatments on mean percentage germination of corn exposed to 0 and 50 mM NaCl, for 24 h. Figure 2 below show the effect of Bacillus amyloliquefaciens cell-free supernatant on the mean percentage germination of corn and soybean at 24 h, under optimal conditions while Figure 3 shows the effect of Bacillus amyloliquefaciens cell free supernatant on mean radicle length (cm) of corn and soybean under optimal conditions. Figure 2 below shows the effect BA cell-free supernatant on mean percentage germination of corn and soybean, at 24 h. Figure 3 shows the effect of BA cell-free supernatant on the mean radicle length (cm) of corn and soybean under optimal conditions.
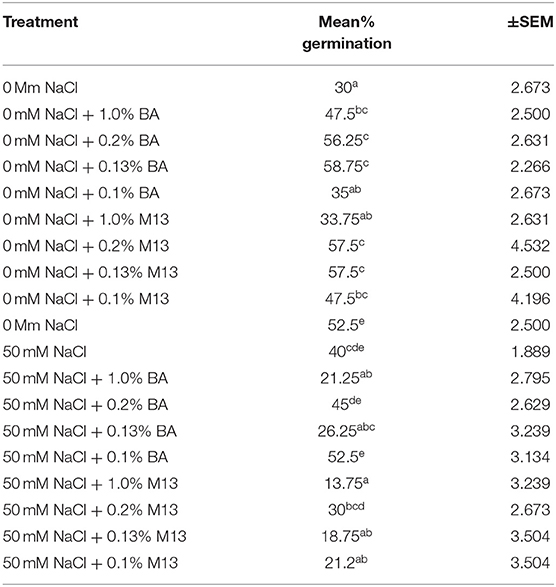
Table 8. Effect of treatment on mean percentage germination of corn exposed to 0 and 50 mM NaCl, for 24 h.
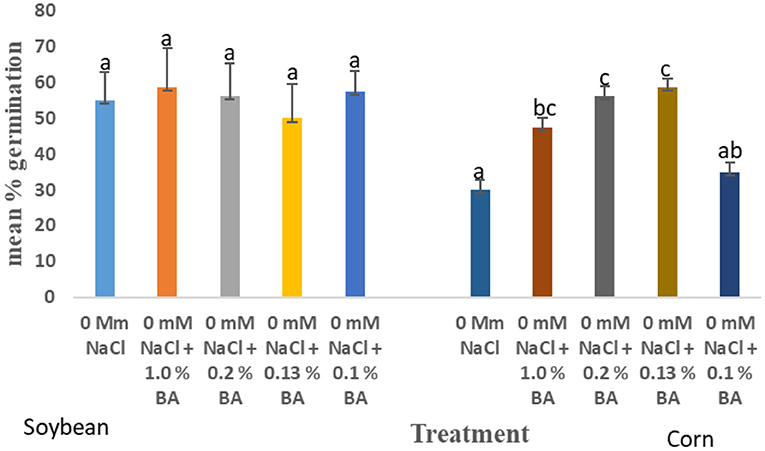
Figure 2. Effect of treatment on the mean percentage germination of corn and soybean, at 24 h, under optimal conditions. Treatments with similar letters are not significantly different. Treatments with different letters are significantly different.
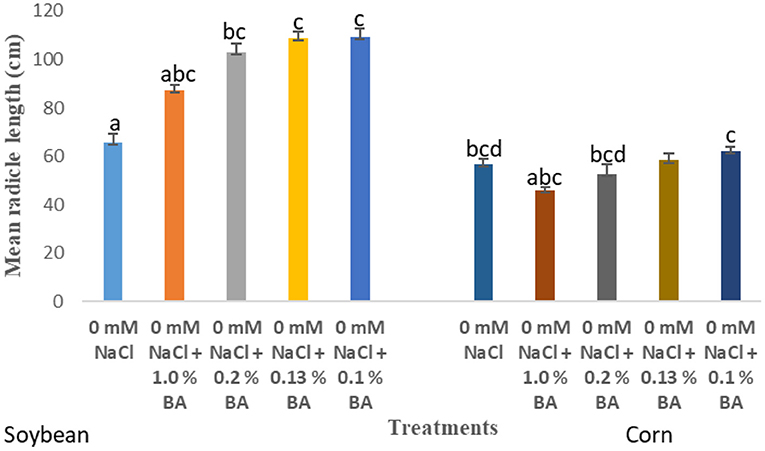
Figure 3. Effect of treatment on the mean radicle length (cm) of corn and soybean under optimal conditions. Treatments with similar letters are not significantly different. Treatments with different letters are significantly different.
50 mM NaCl
Corn
There were observed significant differences in the effect of treatments on mean radicle length of corn. Treatment with 50 mM NaCl significantly lowered mean radicle length by 31.63%, compared to mean radicle length of corn at 0 mM NaCl. However, treatment with concentrations of 0.13% BA and 0.2% BA significantly enhanced mean radicle lengths by 32.99 and 23.73%, to lengths not significantly different from that of the 0 mM control. This implies that, the two BA concentrations may have mitigated the limitations of 50 mM NaCl on corn radicle growth, hence, enhancing radicle length growth. Other treatments also enhanced radicle length but, to lengths not significantly different from that on the 50 mM control, although, the effect of 0.13% M13 was also not significantly different from that of the 0 mM control (Figure 8).
The effect of treatments on mean percentage germination varied across time intervals. At 72 h, there was no observed significant difference in the effect of treatments on mean percentage germination. In fact, the effect of all treatments on mean percentage germination was not significantly different from that of the 0 mM NaCl control. The highest mean percentage germination (100%) was observed in corn treated with 0.1% BA and 0.2% M13, while the lowest was observed in corn treated with 0.13% M13 and 50 mM NaCl. Results suggest that germination of corn was tolerant to 50 mM NaCl. At 48 h, still no significant difference was observed in the effect of treatments on mean percentage germination, although treatment with 0.1% BA resulted in a rise in mean percentage germination, from 86.56% observed at 50 mM NaCl, to 98.75%, the highest observed at 48 h. In fact, the mean percentage germination of all treatments as higher than that of the 50 mM NaCl control. This suggests a possibility that the treatments may have had an effect, but it was not significant because of the already high percentages of the control. At 24 h, there were observed significant differences in the effect of treatments on mean percentage germination. Like at 72 and 48 h, there was no significant difference in the mean percentage 0 mM NaCl and 50 mM NaCl, although the latter resulted in a 23.81% decrease in mean percentage germination was. Treatment with 0.13% M13, 0.1% M13, and 1.0% BA resulted in 18.75, 21.2, and 21.25% mean percentage germination, respectively, significantly lower than that of the 50 mM control.
Soybean
50 mM NaCl lowered mean radicle length of soybean by 19.79%, in comparison to mean radicle length of soybean grown under optimal conditions. At 50 mM NaCl, there was no observed significant difference in the effect of treatments on the mean radicle length of soybean. The highest mean radicle length, 57.67 cm, was observed in soybean treated with 0.2% BA, while the least, 42.35 cm, was observed in soybean treated with 0.1% M13. Although the effect of all treatments was not significantly different from the negative control (50 mM NaCl), microbial cell-free supernatant concentrations of 1.0% BA (52.67 cm) and 0.2% BA, resulted in mean radicle length that was not significantly different from that of soybean grown under optimal conditions (63.4 cm).
The effect of treatments on mean percentage germination varied across time. At 72 h, there was no observed significant difference in the effect of treatments on mean percentage germination. There was an observed 7.5% decline in the mean percentage germination at 50 mM NaCl, compared to 0 mM NaCl. The mean percentage germination of all treatments was not significantly different from that of soybean grown under normal conditions. The highest mean percentage germination (96.25%) was observed in soybean treated with 0 mM NaCl and 0.2% BA while the lowest (87.5%) was observed in soybean treated with 0.13% M13. At 48 h, there was no significant difference in the effect of treatments on mean percentage germination. At 50 mM NaCl, the observed mean germination percentage was 12.5% lower than the mean percentage germination at 0 mM NaCl. The observed mean percentage germination of soybean treated by all the treatments was not significantly different from that of the 0 mM control. The highest mean germination was observed in soybean treated with 0.2% BA (95%) while the lowest was observed in soybean treated with 1.0% M13 (82.5%). At 24 h, there was an observed difference in the effect of treatments on mean percentage germination. 50 mM NaCl decreased mean percentage germination of soybean by 51.28%, compared to mean germination percentage at 0 mM NaCl. Treatment with 1.0% BA increased mean percentage germination by 48.64%, significantly higher than that of 50 mM NaCl and significantly different from that of 0 mM NaCl. Observed mean percentage germination of soybean treated with 0.1% BA, was also noticeably high (37.5%), not significantly different from that observed at 0 mM NaCl. The least mean germination percentage was observed in soybean treated with 0.1% M13. Figure 4 below shows the effect of BA cell-free supernatant on mean percentage germination of corn and soybean exposed to 50 mM NaCl for 24 h. Figure 5 shows the effect BA cell-free supernatant on mean radicle length of corn and soybean exposed to 50 mM NaCl.
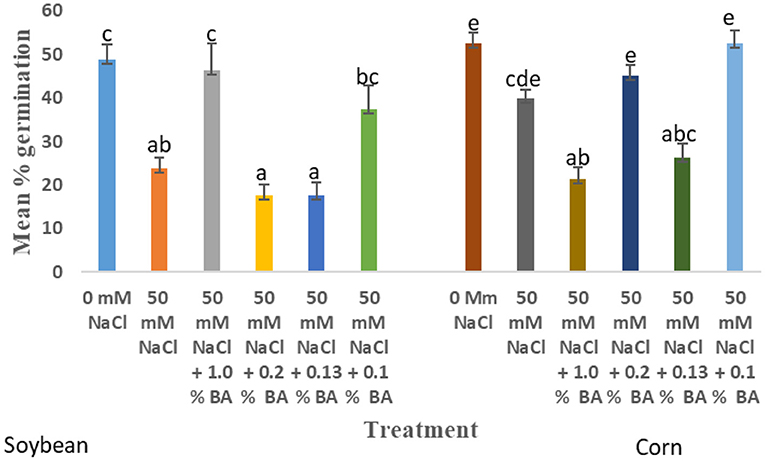
Figure 4. Effect of BA cell-free supernatant on mean percentage germination of corn and soybean exposed to 50 mM NaCl for 24 h. Treatments with similar letters are not significantly different. Treatments with different letters are significantly different.
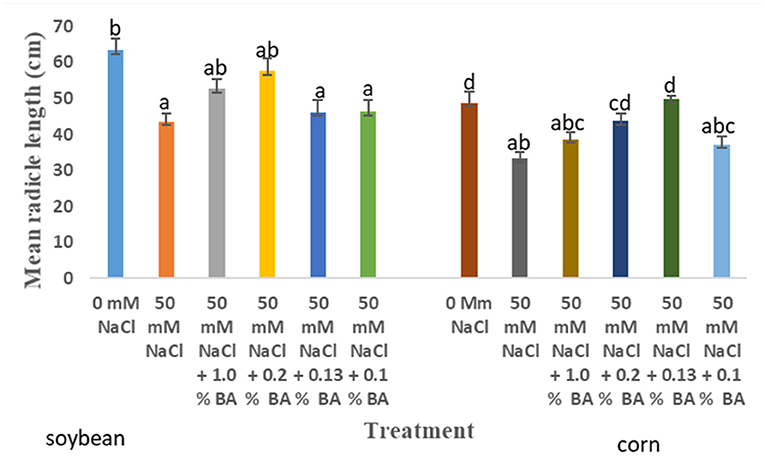
Figure 5. Effect of BA cell-free supernatant on mean radicle length of corn and soybean exposed to 50 mM NaCl. Treatments with similar letters are not significantly different. Treatments with different letters are significantly different.
75 mM NaCl
Corn
There was an observed significant difference in the effect of treatments on mean radicle length of corn. 75 mM NaCl significantly lowered mean radicle length by 35.51%, compared to the control. Radicle length of corn treated with both BA and M13 cell-free supernatant was not significantly different from that of the 75 mM NaCl. However, 1.0% BA, 0.13% BA, and 0.1% BA increased mean radicle length to levels not significantly from the 0 mM NaCl control. The highest mean radicle length was observed in the 0 mM control while the lowest was observed in corn treated with 0.2% BA (30.2875 cm).
There was a significant difference in the effect of treatments on mean percentage germination. At 72 h, there was no significant effect of 75 mM NaCl on mean percentage germination of corn. Mean radicle length of corn treated with 1.0% BA (100%), 0.2% BA (98.75%), 0.13% BA (100%), and 0.1% BA (98.75%) were significantly higher than that of corn treated with 1.0% M13 (85%) and 0.2% M13 (86.25%). At 48 h, 75 mM NaCl lowered mean percentage germination by 13.46%. Overall, BA cell-free supernatant enhanced mean percentage germination. Mean percentage germination of corn treated with 0.2% BA, 0.13% BA, and 0.1% BA was significantly higher than that of 75 mM NaCl by 25.33%. The three also exhibited the highest observed mean percentage germination. The lowest was observed in corn treated with 0.13% M13 (81.25%). At 24 h, there was no observed germination at 75 mM NaCl, even to corn treated with BA and M13 cell-free supernatant.
Soybean
There was an observed difference in the effect of treatments on mean radicle length of soybean. 75 mM NaCl lowered mean radicle length by 24.7% although the difference was not significant. Mean radicle length of soybean treated with 0.13% M13 was significantly lower than the 0 mM NaCl control. Other treatments were not significantly different from 0 mM and 75 Mm NaCl.
The effect on treatments on mean percentage germination varied across time. At 72 h, there was an observed significant difference in the effect of the treatments on mean percentage germination. It was lowered by 12. Ninety-eight percentage, at 75 mM NaCl, compared to mean percentage germination under optimal conditions, although the difference was not significant. Mean percentage germination of soybean treated with 0.2% M13 was significantly lower than that of the 0 mM control. The two exhibited the lowest and highest mean percentage germination, respectively. At 48 h, mean percentage germination was reduced by 14.67% at 75 mM NaCl, compared to the mean percentage germination of soybean grown under optimal conditions (93.75%), which was also the highest. There was no significant difference in the effect of the treatments on mean percentage germination. The lowest mean percentage germination (95.75%) was observed in soybean treated with 0.1% M13. At 24 h, mean percentage germination was significantly 75 mM NaCl, by 53.3% compared to the mean percentage germination of soybean under optimal conditions. There was no significant difference between mean percentage germination of soybean treated with microbial derived compounds and the 75 mM NaCl control. However, the mean percentage germination of soybean treated with 1.0% BA was also not significantly different from that observed under optimal conditions. Mean percentage germination of soybean treated with M13 except 1.0% M13, was significantly lower than the 75 mM control. The lowest mean percentage germination was observed in soybean treated with 0.13% M13. Figure 6 below shows the effect of BA cell-free supernatant on corn and soybean exposed to 75 mM NaCl for 72 h. Figure 7 shows the effect of BA cell-free supernatant on mean radicle length of corn and soybean exposed to 75 mM NaCl.
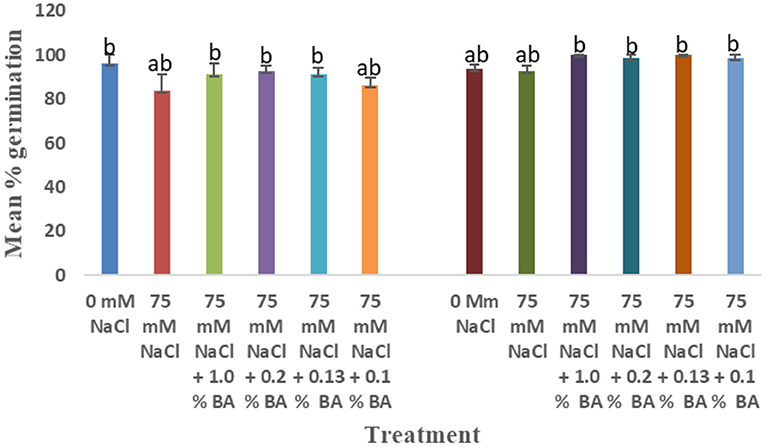
Figure 6. Effect of BA cell-free supernatant on mean percentage germination of corn and soybean exposed to 75 mM NaCl for 72 h. Treatments with similar letters are not significantly different. Treatments with different letters are significantly different.
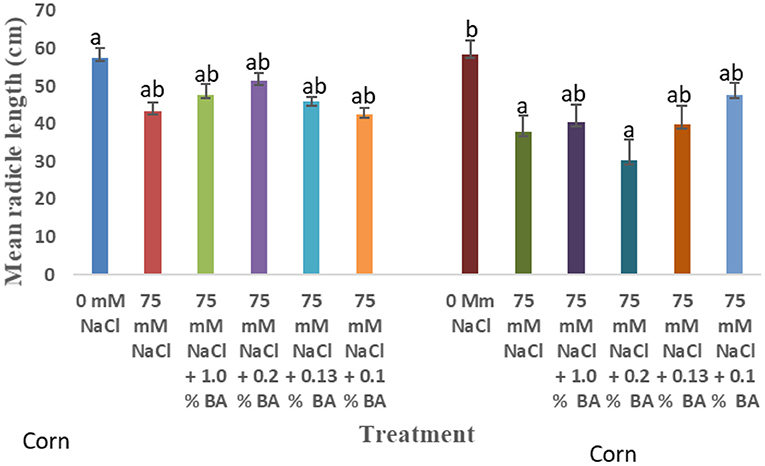
Figure 7. Effect of BA cell-free supernatant on mean radicle length of corn and soybean exposed to 75 mM NaCl. Treatments with similar letters are not significantly different. Treatments with different letters are significantly different.
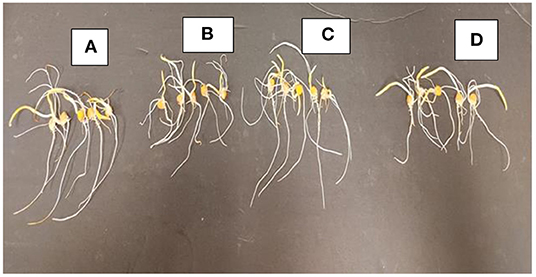
Figure 8. Radicle length of corn with 0 mM NaCl (A), 50 mM NaCl (B), 50 mM NaCl + 0.13% BA (C), and 50 mM NaCl + 0.13% M13 (D).
Discussion
Seed germination and root establishment are essential stages of plant growth and development, which when compromised, may have a significant effect on overall quantity and quality of crop yield (Rifna et al., 2019). A high and uniform germination percentage is a sought after trait by seed companies and farmers. There are several seed and soil related factors that could affect seed germination and root establishment, even under supposedly ideal conditions (Rifna et al., 2019). Delay in seed germination may increase susceptibility of seedlings to soil borne pathogens, decrease plant vigor, delay plant maturity and lower crop yield (Schwinghamer et al., 2015). The radicle grows into the primary root/tap which anchors the seedling in the soil. A poorly developed radicle may negatively influence plant establishment and water uptake. Therefore, a technology to enhance seed germination and seedling establishment is essential. Use of PGPM and their derived compounds is a environmentally sustainable approach that enhances seed germination and plant growth. In this study, Bacillus amyloliquefaciens EB2003A's cell free supernatant enhanced mean percentage germination and mean radicle length of corn (Zea mays) and soybean (Glycine max L. merill), under optimal and NaCl stressed conditions. There are no previous studies on the effect of BA cell-free supernatant on plant growth. However, previous studies have shown that compounds derived from cell-free supernatant of other PGPM enhance seed germination and plant growth. Subramanian et al. (2016) observed an increase in germination of soybean treated with thuricin 17 and lipochitooligosaccharide (LCO). The two compounds were isolated from cell-free supernatant of Bacillus thuringiensis NEB17 and Bradyrhizobium japonicum 532C, respectively. Souleimanov et al. (2002) also observed an increase in root length of soybean seedlings treated with LCO. Bacillus amyloliquefaciens strains have been reported to mitigate salt effects in different plants through production of volatile organic compounds (Chen et al., 2016; Cappellari and Banchio, 2020; Liu et al., 2020). For instance, Liu et al. (2020) observed a significant increase in the biomass and maintenance of chlorophyll content of Arabidopsis thaliana plants treated with Bacillus amyloliquefaciens FZB42 VOCs, compared to the control. Chen et al. (2016) observed increases in chlorophyll content, production of antioxidant enzymes and K+/Na+ ratio, in 100 mM NaCl stressed corn plants exposed to Bacillus amyloliquefaciens SQR9 VOCs. The same strain also enhanced salt tolerance through production of spermidine (Chen et al., 2017). In rice, tested Bacillus amyloliquefaciens strains enhanced salt tolerance through production of phytohormones such as auxins, abscisic acid and gibberellic acid Shahzad et al., 2016, 2017. All these findings are evidence that, indeed some Bacillus amyloliquefaciens strains can produce substances which mitigate salt stress in a range of crop species. In this study, there were observed variations in the effect of the cell-free supernatant on mean percentage germination and mean radicle length, at different NaCl levels, cell free supernatant concentration and plant species. Previous studies have reported that indeed the effectiveness of a given microbial derived compound may vary across plant species, concentration of compound, soil conditions and different biotic and abiotic stresses (Naamala and Smith, 2021a).
Effect of Cell-Free Supernatant Concentration Level
In this study, BA cell-free supernatant enhanced mean radicle length and mean percentage germination, under optimal and NaCl conditions. There were variations observed in the effect of the different concentrations on both mean percentage germination and mean radicle length of corn and soybean. It not surprising that such observations were made, given the fact the bioactivity of the cell-free supernatant is presumed to be due to compounds exuded by Bacillus amyloliquefaciens, in the growth media. Majority of such microbial derived compounds are signaling molecules such as phytohormones, whose concentration has been for long known to either enhance or inhibit plant growth (Lyu et al., 2020; Antar et al., 2021; Naamala and Smith, 2021a). Some compounds will enhance plant growth at very low concentrations while others will be required in relatively high concentrations for positive significant effects to be observed. The same observation was made in this study, where at a given concentration, mean radicle length and or mean percentage germination were significantly enhanced, significantly lowered or no effect observed. For instance, in soybean, results of mean radicle length at optimal conditions showed an increase in mean radicle length of soybean as concentration of the cell-free supernatant reduced from 1.0% BA to 0.1% BA. Results on mean radicle length in corn, under optimal conditions seem to suggest the same as concentration 1.0% BA exhibited a significantly lower radicle length than that of 0.1% BA. In a different scenario, at 75 mM NaCl, concentration 1.0% BA significantly enhanced mean percentage germination while 0.2% BA, 0.13%, and 0.1% BA did not, at 24 h. However, 0.1% BA the effect of 0.1% BA was not significantly different from that of 1.0% BA. Whether it is the same potential compound inducing these significant effects is a question that cannot be answered at this stage of the study. Overall, our results suggest that concentration plays a significant role in the effectiveness of the cell-free supernatant. The findings are in line with previous studies which also showed that effectiveness of some microbe-derived compounds can be dependent on concentration of the compound (Schwinghamer et al., 2015; Gautam et al., 2016). In their study on canola, Schwinghamer et al., observed a variation in the effect of LCO concentration on germination. A concentration of 10−6 enhanced early canola germination while a concentration of 10−9 delayed germination but enhanced uniformity in germination (Schwinghamer et al., 2015). Souleimanov et al. (2002) studied the effect of different concentrations of LCO on soybean and observed differences in the effect of different concentrations on soybean growth. Concentration of 10−7 to 10−9 enhanced soybean growth while concentrations of 10−11 did not (Souleimanov et al., 2002). A thuricin 17 concentration of 10−9 enhanced soybean germination at 100 mM NaCl, while a concentration of 10−11 did not (Subramanian et al., 2016).
Effect of NaCl Level
In this study, the role NaCl level played on seed germination and radicle development cannot be ignored. NaCl (75 mM NaCl and 50 mM NaCl) lowered mean percentage and mean radicle length of both soybean and corn, in some cases, significantly, in others not. Salinity stress is a major global abiotic stress that affects crops, at all stages of development, including seed germination, radicle length and general plant growth (Subramanian et al., 2016; Ilangumaran et al., 2021). As little as 0.1 M NaCl causes visible reductions in plant height in some corn cultivars, compared to corn grown under optimal conditions (Farooq et al., 2015). For soybean, high salt concentrations affect germination (Kondetti et al., 2012), early growth and the nitrogen fixation process (Zahran, 1997; Zaharan, 1999; Egamberdieva and Lugtenberg, 2014).
Microbial derived compounds have been reported to enhance plant growth under saline conditions (Naamala and Smith, 2021b). In this study, the effect BA cell-free supernatant on mean radicle length and mean percentage germination varied at different NaCl levels, in corn and soybean. In soybean, BA cell-free supernatant was more effective at 0 mM NaCl, and not 50 and 75 mM NaCl. In corn however, significant results were observed at 50 and 75 mM NaCl, but not 0 Mm NaCl. It should also be noted that effectiveness was higher at 50 mM NaCl than at 75 mM NaCl. For instance, in corn, concentrations 0.2% BA and 0.13% resulted in a significantly higher mean radicle length at 50 mM while at 75 mM the effect of the two concentrations was not significantly different from that of the 75 mM control. In fact, at 75 mM NaCl, mean radicle length reduced with the decrease in BA cell free supernatant.
Previous studies have reported that level of stress plays a significant role in the effect of microbial derived compounds on growth of different plants species, in some being effective under stressed conditions, while in others under optimal conditions, yet, in others at both. Subramanian and co observed that thuricin 17 and LCO enhanced germination in soybean NaCl stressed and optimal conditions (Subramanian et al., 2016). Canola subjected to low temperature stress responded to treatment with LCO while that grown under optimal temperature conditions did not (Schwinghamer et al., 2015).
Results also seem to suggest that higher NaCl levels require higher cell-free supernatant concentrations to have a chance at effectiveness. For instance, at 0 mM NaCl, in soybean and corn, lower concentrations seemed to be more effective at enhancing radicle length than, higher concentrations. However, the reverse is true at both 50 and 75 mM NaCl. For instance, at 50 mM NaCl, radicle length of soybean treated with the four BA concentrations was not significantly different from that of 50 mM NaCl, but radicle length of 1.0% BA and 0.2% BA was also not significantly different from that of the 0 mM NaCl control. The mean percentage germination was also higher in soybean treated with the two concentrations. In corn, at 75 mM NaCl, mean radicle length reduced with the decrease in BA cell free supernatant. However, at 50 mM NaCl, 0.2% BA and 0.13% induced higher than the control. Plants have a stress defend system, that is activated when a plant is exposed to stress. Perhaps, at lower concentrations, the plant stress defensive system is able to mitigate a great deal of NaCl stress, requiring only a little boost from the microbial derived cell-free supernatant, to exhibit significant effects on plant growth. However, it should be noted that as stress builds up in and around the plant, some signals involved in the plant defensive system, such as ethylene can also accumulate to levels toxic to the plant. In addition to that high stress levels can damage the plant proteins and nucleic acids, perhaps, to levels that even microbial derived compounds cannot rehabilitate, hence, the effect on plant growth process such as germination and radicle length. Subramanian and co observed that a concentration of 10−7 (Subramanian et al., 2016). LCO enhanced soybean germination at 100 Mm and not at 150, 175, and 200 mM NaCl.
Previous reports show that PGPM and PGPM derived compounds enhance plant growth under saline conditions by mitigating the effect of salt on the plant, through employing one of the following mechanisms: upregulation of the plant's defense system through induced systemic resistance or production of antioxidants that degrade ROS (Amna et al., 2019). Production of exopolysaccharides which can act to improve water holding capacity and bind Na+ hence, lowering osmotic and ionic stress in plants (Tewari and Arora, 2014; Shrivastava and Kumar, 2015; Amna et al., 2019). Production of ACC deaminase which breaks down ethylene, there by lowering its concentration in plant tissue to levels less toxic (Hayat et al., 2010; Nautiyal et al., 2013; Amna et al., 2019).
Effect of Plant Species
In his study, there were variations in the effect of treatments on soybean and corn. For instance, at optimal conditions, soybean radicle length was more responsive to treatments while at 50 mM NaCl, corn radicle length was more responsive. The effect of microbial derived compounds on plants growth has been reported to vary between and within plant species. For example, LCO was reported to exhibit varying effects on canola varieties, enhancing growth in cultivar polo and not the other cultivars under study (Schwinghamer et al., 2015). Souleimanov et al. (2002) observed varying effect of LCO on corn and soybean. In soybean, significant effects on root length and root dry weight were observed while in corn, significant increases in shoot dry weight were observed. This study supports findings of these two studies. Corn and soybean have different structural and genetic components which may explain the differences in their response to treatments. Also, the two crops may respond to different microbial signals differently, some being compatible and others not. It is possible that a single potential bioactive compound causes both effects in corn and soybean. It is also possible that the bioactive compound which caused the effect in soybean is different from what caused effects in corn.
Conclusions
The cell-free supernatant obtained from salt tolerant Bacillus amyloliquefaciens EB2003A strain exposed to 200 mM NaCl, enhanced germination and radicle length of corn, under NaCl stressed and optimal conditions. The effect of treatments varied across plant species, concentration of BA cell free-supernatant and NaCl level. Results of the study suggest that that Bacillus amyloliquefaciens EB2003A produces in its growth media, bioactive compound(s), with ability to enhance mean radicle length and mean percentage germination in corn and soybean. The identity and quantity of the potential compound are yet to be known. Findings of this study can be used as a baseline to further study the cell-free supernatant for isolation and identification of potential compounds with ability to enhance plant growth, independently.
Data Availability Statement
The raw data supporting the conclusions of this article will be made available by the authors, without undue reservation.
Author Contributions
JN set up the experiment and wrote the manuscript. LM and MA helped with data collection and experimental set up. SS advised on scientific approach and provided background knowledge. DS provided funding, guided in scientific knowledge, provided the intellectual context, and extensive editorial input. All authors contributed to the article and approved the submitted version.
Funding
This work was funded through a grant from Consortium de recherche et innovations en bioprocédés industriels au Québec, number CRIBIQ 2017-034-C30.
Conflict of Interest
The authors declare that the research was conducted in the absence of any commercial or financial relationships that could be construed as a potential conflict of interest.
Publisher's Note
All claims expressed in this article are solely those of the authors and do not necessarily represent those of their affiliated organizations, or those of the publisher, the editors and the reviewers. Any product that may be evaluated in this article, or claim that may be made by its manufacturer, is not guaranteed or endorsed by the publisher.
Acknowledgments
We acknowledge EVL Inc., for provision of Bacillus amyloliquefaciens strains and their growth media. Special appreciation to Martin Macouzet for always sending microbial media on time, even when it is short notice. We also acknowledge Synagri for guidance and expertise input in this work.
References
Ahmad, P., Jaleel, C. A., Salem, M. A., Nabi, G., and Sharma, S. (2010). Roles of enzymatic and nonenzymatic antioxidants in plants during abiotic stress. Crit. Rev. Biotechnol. 30, 161–175. doi: 10.3109/07388550903524243
Ahmad, P., Tripathi, D. K., Deshmukh, R., Singh, V. P., and Corpas, F. J. (2019). Revisiting the role of ROS and RNS in plants under changing environment. Environ Exp Bot. 161, 1–3. doi: 10.1016/j.envexpbot.2019.02.017
Amna, D. B. U., Sarfraz, S., Xia, Y., Kamran, M. A., Javed, M. T., et al. (2019). Mechanistic elucidation of germination potential and growth of wheat inoculated with exopolysaccharide and ACC- deaminase producing Bacillus strains under induced salinity stress. Ecotoxicol. Environ. Safety 183, 109466. doi: 10.1016/j.ecoenv.2019.109466
Ansari, O., and Sharif Zadeh, F. (2012). Does gibberelic acid (GA), salicylic acid (SA) and ascorbic acid (ASc) improve mountain rye (Secale montanum) seeds germination and seedlings growth under cold stress? Intl. Res. J. Appl. Basic Sci. 3, 1651–1657.
Antar, M., Gopal, P., Msimbira, L. A., Naamala, J., Nazari, N., Overbeek, W., et al. (2021). “Inter-organismal signaling in the rhizosphere,” in Rhizosphere Biology: Interactions Between Microbes and Plants. Rhizosphere Biology, editors V. V. S. R. Gupta, A. K. Sharma (Singapore: Springer).
Bai, Y., Kissoudis, C., Yan, Z., Visser, R. G., and van der Linden, G. (2018). Plant behaviour under combined stress: tomato responses to combined salinity and pathogen stress. Plant J. 93, 781–793. doi: 10.1111/tpj.13800
Bui, E. N. (2013). Soil salinity: a neglected factor in plant ecology and biogeography. J. Arid Environ. 92, 14–25. doi: 10.1016/j.jaridenv.2012.12.014
Cappellari, L. R., and Banchio, E. (2020). Microbial volatile organic compounds produced by Bacillus amyloliquefaciens GB03 ameliorate the effects of salt stress in Mentha piperita principally through acetoin emission. J. Plant Growth Reg. 39, 764–775. doi: 10.1007/s00344-019-10020-3
Chen, L., Liu, Y., Wu, G., Njeri, K. V., Shen, Q., Zhang, N., et al. (2016). Induced maize salt tolerance by rhizosphere inoculation of Bacillus amyloliquefaciens SQR9. Physiol. Plantarum 158, 34–44. doi: 10.1111/ppl.12441
Chen, L., Liu, Y., Wu, G., Zhang, N., Shen, Q., and Zhang, R. (2017). Beneficial Rhizobacterium Bacillus amyloliquefaciens SQR9 induces plant salt tolerance through spermidine production. MPMI 30, 423–432. doi: 10.1094/MPMI-02-17-0027-R
Diouf, I. A., Derivot, L., Bitton, F., Pascual, L., and Causse, M. (2018). Water deficit and salinity stress reveal many specific QTL for plant growth and fruit quality traits in tomato. Front. Plant Sci. 9, 279. doi: 10.3389/fpls.2018.00279
Egamberdieva, D., and Lugtenberg, B. (2014). “Use of plant growth-promoting rhizobacteria to alleviate salinity stress in plants,” in Use of Microbes for the Alleviation of Soil Stresses, editor M. Miransari. Vol. 1 (New York, NY: Springer Science+Business Media), 73–96.
El Moukhtari, A., Cabassa-Hourton, C., Farissi, M., and Savoure, A. (2020). How does proline treatment promote salt stress tolerance during crop plant development? Front. Plant Sci. 11, 1127. doi: 10.3389/fpls.2020.01127
Farooq, M., Hussain, M., Wakeel, A., and Siddique, K. H. M. (2015). Salt stress in maize: effects, resistance mechanisms, and management. A review. Agron. Sustain. Dev. 35, 461. doi: 10.1007/s13593-015-0287-0
Forni, C., Duca, D., and Glick, B. R. (2017). Mechanisms of plant response to salt and drought stress and their alteration by rhizobacteria. Plant Soil 410, 335–356. doi: 10.1007/s11104-016-3007-x
García-García, A. L., García-Machado, F. J., Borges, A. A., Morales-Sierra, S., Boto, A., and Jiménez-Arias, D. (2020). Pure organic active compounds against abiotic stress: a biostimulant overview. Front. Plant Sci. 11, 575829. doi: 10.3389/fpls.2020.575829
Gautam, K., Schwinghamer, T. D., and Smith, D. L. (2016). The response of soybean to nod factors and a bacteriocin. Plant Signal. Behav. 11, 10. doi: 10.1080/15592324.2016.1241934
Gray, E. (2005). Identification of a Novel Bacteriocin, Thuricin 17 Produced by Bacillus thuringiensis NEB17. (PhD thesis),McGill University, Quebec.
Gray, E. J., Di Falco, M., Souleimanov, A., and Smith, D. L. (2006). Proteomic analysis of the bacteriocin thuricin 17 produced by Bacillus thuringiensis NEB17. FEMS Microbiol. Lett. 255, 27–32. doi: 10.1111/j.1574-6968.2005.00054.x
Hayat, R., Ali, S., Amara, U., Khalid, R., and Ahmed, I. (2010). Soil beneficial bacteria and their role in plant growth promotion: a review. Ann. Microbiol. 60, 579–598. doi: 10.1007/s13213-010-0117-1
Ibrahim, E.A. (2016). Seed priming to alleviate salinity stress in germinating seeds. J. Plant Physiol. 192, 38–46. doi: 10.1016/j.jplph.2015.12.011
Ilangumaran, G., Schwinghamer, T. D., and Smith, D. L. (2021). Rhizobacteria from root nodules of an indigenous legume enhance salinity stress tolerance in soybean. Front. Sustain. Food Syst. 4, 617978. doi: 10.3389/fsufs.2020.617978
Kazerooni, E. A., Maharachchikumbura, S. S. N., Adhikari, A., Al-Sadi, A. M., Kang, S. M., Kim, L. R, and Lee, I. J. (2021). Rhizospheric bacillus amyloliquefaciens protects Capsicum annuum cv. geumsugangsan from multiple abiotic stresses via multifarious plant growth-promoting attributes. Front. Plant Sci. 12, 669693. doi: 10.3389/fpls.2021.669693
Kohli, S. K, Khanna, K., Bhardwaj, R., AbdAllah, E. F., Ahmad, P., and Corpas, F. J. (2019). Assessment of subcellular ROS and NO metabolism in higher plants: multifunctional signaling molecules. Antioxidants 8, 641. doi: 10.3390/antiox8120641
Kondetti, P., Jawali, N., Apte, S. K., and Shitole, M. G. (2012). Salt tolerance in Indian soybean (Glycine max (L.) Merill) varieties at germination and early seedling growth. Ann. Biol. Res. 3, 1489–1498.
Li, H., Zhu, Y., Hu, Y., Han, W., and Gong, H. (2015). Beneficial effects of silicon in alleviating salinity stress of tomato seedlings grown under sand culture. Acta. Physiol. Plant. 37, 71. doi: 10.1007/s11738-015-1818-7
Liu, S., Tian, Y., Jia, M., Lu, X., Yue, L., Zhao, X., et al. (2020). Induction of Salt Tolerance in Arabidopsis thaliana by volatiles from Bacillus amyloliquefaciens FZB42 via the jasmonic acid signaling pathway. Front. Microbiol. 11, 562934. doi: 10.3389/fmicb.2020.562934
Lyu, D., Backer, R., Subramanian, S., and Smith, D. L. (2020). Phytomicrobiome coordination signals hold potential for climate change-resilient agriculture. Front. Plant Sci. 11, 634. doi: 10.3389/fpls.2020.00634
Macouzet, M. (2016). Critical aspects in the conception and production of microbial plant biostimulants. Probiotic Intelligentsia 5, 29–38. doi: 10.1111/j.1365-3040.2009.02041.x
Mozafariyan, M., Kamelmanesh, M. M., and Hawrylak-Nowak, B. (2016). Ameliorative effect of selenium on tomato plants grown under salinity stress. Arch. Agron. Soil Sci. 62, 1368–1380. doi: 10.1080/03650340.2016.1149816
Naamala, J., and Smith, D. (2020). Relevance of plant growth promoting microorganisms and their derived compounds, in the face of climate change. Agronomy 10, 1179. doi: 10.3390/agronomy10081179
Naamala, J., and Smith, D. L (2021a). Microbial derived compounds, a step toward enhancing microbial inoculants technology for sustainable agriculture. Front. Microbiol. 12, 634807. doi: 10.3389/fmicb.2021.634807
Naamala, J., and Smith, D. L. (2021b). Microbial derived compounds are a promising approach to mitigating salinity stress in agricultural crops. Front. Microbiol. 12, 765320. doi: 10.3389/fmicb.2021.765320
Nautiyal, C. S., Srivastava, S., Chauhan, P. S., Seem, K., Mishra, A., and Sopory, S. K. (2013). Plant growth-promoting bacteria Bacillus amyloliquefaciens NBRISN13 modulates gene expression profile of leaf and rhizosphere community in rice during salt stress. Plant Physiol. Biochem. 66, 1–9. doi: 10.1016/j.plaphy.2013.01.020
Rifna, E. J., Ramanan, K. R., and Mahendran, R. (2019). Emerging technology applications for improving seed germination. Trends Food Sci. Technol. 86, 95–108. doi: 10.1016/j.tifs.2019.02.029
Rouhi, H. R., Aboutalebian, M. A., and Sharif-zadeh, F. (2011). Effects of hydro and osmopriming on drought stress tolerance during germination in four grass species. Int. J. Agric. Sci. 1, 107–114.
Schwinghamer, T., Souleimanov, A., Dutilleul, P., and Smith, D. (2015). The plant growth regulator lipo-chitooligosaccharide (LCO) enhances the germination of canola (Brassica napus [L.]). J. Plant Growth Regul. 34, 183–195. doi: 10.1007/s00344-014-9456-7
Shahzad, R., Waqas, M., Khan, A. L., Asaf, S., Khan, M. A., Kang, S. M., et al. (2016). Seed-borne endophytic Bacillus amyloliquefaciens RWL-1 produces gibberellins and regulates endogenous phytohormones of Oryza sativa. Plant Physiol. Biochem. 106, 236e243. doi: 10.1016/j.plaphy.2016.05.006
Shahzada, R., Khan, A. L., Bilal, S., Waqas, M., Kang, S., and Lee, I. J. (2017). Inoculation of abscisic acid-producing endophytic bacteria enhances salinity stress tolerance in Oryza sativa. Environ. Exp. Bot. 136, 68–77. doi: 10.1016/j.envexpbot.2017.01.010
Shrivastava, P., and Kumar, R. (2015). Soil salinity: A serious environmental issue and plant growth promoting bacteria as one of the tools for its alleviation. Saudi J. Biol. Sci. 22, 123–131. doi: 10.1016/j.sjbs.2014.12.001
Souleimanov, A., Prithiviraj, B., and Smith, D. L. (2002). The major Nod factor of Bradyrhizobium japonicum promotes early growth of soybean and corn. J. Exp. Bot. 53, 1929–1934. doi: 10.1093/jxb/erf034
Subramanian, S., Ricci, E., Souleimanov, A., and Smith, D. L. (2016). A proteomic approach to lipochitooligosaccharide and thuricin 17 effects on soybean germination unstressed and salt stress. PLoS ONE 11, e0160660. doi: 10.1371/journal.pone.0160660
Subramanian, S., Souleimanov, A., and Smith, D. L. (2021). Thuricin17 production and proteome differences in Bacillus thuringiensis NEB17 cell-free supernatant under NaCl stress. Front. Sustain. Food Syst. 5, 630628. doi: 10.3389/fsufs.2021.630628
Tanji, K. K. (2002). “Salinity in the soil environment,” in Salinity: Environment - Plants – Molecules, editors A. Läuchli and U. Lüttge (Dordrecht: Springer).
Tewari, S., and Arora, N. K. (2014). Multifunctional exopolysaccharides from Pseudomonas aeruginosa PF23 involved in plant growth stimulation, biocontrol and stress amelioration in sunflower under saline conditions. Curr. Microbiol. 69, 484–494. doi: 10.1007/s00284-014-0612-x
US Salinity Laboratory Staff (1954). Diagnosis and Improvement of Saline and Alkali Soils. USDA Handbook No.60. Washington, DC: U.S. Government Printing Office.
Zaharan, H. H. (1999). Rhizobium-legume symbiosis and nitrogen fixation under severe conditions and in an arid climate. Microbiol. Mol. Biol. Rev. 63, 968–989. doi: 10.1128/MMBR.63.4.968-989.1999
Zahran, H. H. (1997). Diversity, adaptation, and activity of the bacterial flora in saline environments. Biol. Fertility Soils 25, 211–223. doi: 10.1007/s003740050306
Keywords: cell-free, supernatant, radicle, length, germination, soybean, corn, tomato
Citation: Naamala J, Msimbira LA, Antar M, Subramanian S and Smith DL (2022) Cell-Free Supernatant Obtained From a Salt Tolerant Bacillus amyloliquefaciens Strain Enhances Germination and Radicle Length Under NaCl Stressed and Optimal Conditions. Front. Sustain. Food Syst. 6:788939. doi: 10.3389/fsufs.2022.788939
Received: 03 October 2021; Accepted: 24 January 2022;
Published: 17 February 2022.
Edited by:
Pushp Sheel Shukla, Dalhousie University, CanadaReviewed by:
Yaosheng Wang, Chinese Academy of Agricultural Sciences (CAAS), ChinaParvaiz Ahmad, Government Degree College, Pulwama, India
Copyright © 2022 Naamala, Msimbira, Antar, Subramanian and Smith. This is an open-access article distributed under the terms of the Creative Commons Attribution License (CC BY). The use, distribution or reproduction in other forums is permitted, provided the original author(s) and the copyright owner(s) are credited and that the original publication in this journal is cited, in accordance with accepted academic practice. No use, distribution or reproduction is permitted which does not comply with these terms.
*Correspondence: Donald L. Smith, donald.smith@mcgill.ca