- 1Genetic Resources Center, International Institute of Tropical Agriculture, Ibadan, Nigeria
- 2Department of Crop Protection and Environmental Biology, University of Ibadan, Ibadan, Nigeria
In Sub-Saharan Africa (SSA), both crop production and the hidden hunger index (HHI, a combination of zinc, iron, and vitamin A deficiency), continue to be worse than the rest of the world. Currently, 31 out of 36 countries of SSA show the highest HHI. At the same time, several studies show climate change as a major constraint to agriculture productivity and a significant threat to SSA food security without significant action regarding adaptation. The food security of SSA is dependent on a few major crops, with many of them providing largely only an energy source in the diet. To address this, crop diversification and climate-resilient crops that have adaptation to climate change can be used and one route toward this is promoting the cultivation of African orphan (neglected or underutilized) crops. These crops, particularly legumes, have the potential to improve food and nutrition security in SSA due to their cultural linkage with the regional food habits of the communities, nutritionally rich food, untapped genetic diversity, and adaptation to harsh climate conditions and poor marginal soils. Despite the wide distribution of orphan legumes across the landscape of SSA, these important crop species are characterized by low yield and decreasing utilization due in part to a lack of improved varieties and a lack of adequate research attention. Genomic-assisted breeding (GAB) can contribute to developing improved varieties that yield more, have improved resilience, and high nutritional value. The availability of large and diverse collections of germplasm is an essential resource for crop improvement. In the Genetic Resources Center of the International Institute of Tropical Agriculture, the collections of orphan legumes, particularly the Bambara groundnut, African yambean, and Kersting's groundnut, have been characterized and evaluated for their key traits, and new collections are being undertaken to fill gaps and to widen the genetic diversity available to underpin breeding that can be further utilized with GAB tools to develop faster and cost-effective climate-resilient cultivars with a high nutrition value for SSA farmers. However, a greater investment of resources is required for applying modern breeding to orphan legume crops if their full potential is to be realized.
Introduction
At present, Sub-Saharan Africa (SSA) is facing multiple challenges including hunger due to low agriculture productivity, a malnourished population, high population growth rate, and climate change (Eriksson et al., 2016; Rippke et al., 2016; IFPRI, 2017; Hendre et al., 2019; Giller, 2020). The food security of Africa is largely dependent on ten staple crops: cereals (maize, pearl millet, rice, and sorghum), tubers (cassava, yam, banana/plantain, and sweet potato), and legumes (cowpea and beans) (Eriksson et al., 2018). However, their average yield is low compared to the average world production and far lower than North America and Europe (Eriksson et al., 2018). There are several factors, such as low soil fertility, rainfed agriculture, insect and pest attacks, and biotic and abiotic stresses, that can significantly reduce the crop yield. In SSA, the commonly used dose of nitrogen, phosphorous, and potassium (NPK) fertilizers by farmers is an average of 8 kg/ha compared to the recommended 30–120 kg/ha. In the past decades, the increased agricultural production of SSA was largely achieved by the cultivation of a larger area rather than an increase in productivity (Sanchez, 2002). However, a remarkable increase in the agriculture productivity of Ethiopia was noticed in statistical data due to the utilization of high-yielding improved cultivars and efficient implementation of the Agriculture Growth Plan of the Ethiopian government (Abate et al., 2015; van Dijk et al., 2020). By 2050, the population of SSA is predicted to increase 2.5-fold and achieving a zero-hunger target under SDG2 (Sustainable Development Goal 2) in SSA is a most essential and difficult challenge. The objective of SDG2 in SSA is reducing hunger, improving nutrition, and ensuring food security through promoting sustainable agriculture. The occurrence of undernourishment in Africa has increased from 17.6 to 19.1% of the population from the year 2014 to 2019, which is more than twice the world average and the highest globally (FAO, 2020). At the same time, numerous climate studies and simulation reports have confirmed that climate change is a major constraint, significantly negatively impacting on agriculture productivity livelihoods and food security (IPCC, 2012; Ramirez-Villegas et al., 2017; Girvetz et al., 2019). The CIMP5 project with the GCM model predicted a rise in the environmental temperature of Africa of 1.7°C, 2.7°C, and 4.5°C by the years 2030, 2050, and 2080, respectively (Girvetz et al., 2019). A meta-database of 16 studies was used for a study on future crop yield prediction, in which the results indicate large changes in yield, ranging from −50 to +90%, with a median yield loss near −11% for the thirteen major staple crops of African countries including West Africa (Roudier et al., 2011). The crop production of SSA is predicted to be among the most significantly reduced, with average production losses between 20 and 40% by 2050 (Ramirez-Villegas and Thornton, 2015). At the same time, biodiversity is declining (Ringler et al., 2010). There is a need to improve nutrition in many rural and urban communities and to develop food systems that are more resilient to climate change. Underutilized or orphan crops have an important role in this (Chivenge et al., 2015; Tadele, 2018). Orphan legume crops have typically been cultivated in a particular region and gone through natural and farmer selection over many years (Dawson et al., 2007). They serve as a means of income generation for the local subsistence farmers in the rural areas (Dawson et al., 2007). Orphan legumes are often rich in micronutrients that are capable of ameliorating nutrient deficiencies.
Modern breeding methods including genomics-assisted breeding (GAB) are playing an increasing role in improving the yield and nutrition content in staple crops that cannot be easily and rapidly achieved by conventional breeding (Cerrudo et al., 2018; Mbanjo et al., 2021; Prasanna et al., 2021). Genomic approaches have been utilized in crops for exploring the diversity of collections (Hiremath et al., 2012; Tamiru et al., 2020); precise parental crossing; dense and comprehensive linkage genetic maps (Hiremath et al., 2012; Paliwal et al., 2012; Saxena et al., 2012); quantitative trait loci (QTL) discovery for biotic, abiotic, and nutritional traits (Varshney et al., 2009; Sarvamangala et al., 2011; Paliwal et al., 2012; Jaganathan et al., 2015; Tamiru et al., 2020); and development of improved lines through the introgression of QTL into elite breeding lines (Saghai-Maroof et al., 2008; Varshney et al., 2015). In maize, the genetic gain for heat and drought increased from 4.4 to 19.4% using GAB tools (Cerrudo et al., 2018). The genetic gain of 19.4% under drought is very significant and indicates that GAB tools could play an important role in developing climate-resilient improved maize lines. The marker-assisted selection (MAS) for root yields, dry matter content and pro-vitamin A, cassava green mite, and cassava mosaic virus disease (CMD) resistance have been applied in the cassava breeding program at the IITA to develop improved varieties using GAB tools (do Carmo et al., 2015; Mbanjo et al., 2021). GRC conserves 6,747 accessions of orphan legumes, including African yambean (AYB), Kersting's groundnut (KG), and Bambara groundnut (BG), which are important indigenous African crops. This diverse collection of orphan legumes has a significant potential to provide genetic resources to initiate crop breeding for essential agronomical traits, including climate-adaptive traits and nutritional traits. However, despite their impressive climate resilience and nutritional profiles, the utilization of conserved collections in crop improvement has been limited due to a lack of knowledge on diversity information, both phenotypic and molecular. Presently, several genomic research activities, including genetic diversity, linkage mapping, and marker–trait association, are in progress using genotyping by sequencing at the GRC in BG, AYB, and KG. This review summarizes the economic potential, utilization, and constraints to yield production in AYB, BG, and KG crops. We also focus on the available germplasm collection and its gap analysis and genomics progress toward the improvement of these African orphan legumes.
African Yambean
AYB [Sphenostylis stenocarpa (Hochst ex. A. Rich.) Harms] (Figure 1a) is an African underutilized legume with a significant potential to enhance food security. It produces edible seeds that are rich in protein and underground swollen roots (NRC., 2007). Legumes are the major sources of protein in many developing countries of the world (Allen and Allen, 1981). The genus Sphenostylis is very small and has species with a growth habit, which can be prostrate, climbing, or erect (Potter, 1992). The AYB is the most cultivated and economically important species out of the seven in the genus Sphenostylis (Potter, 1992; Potter and Doyle, 1992). It is one of the most important tuberous legumes among indigenous African food cultures.
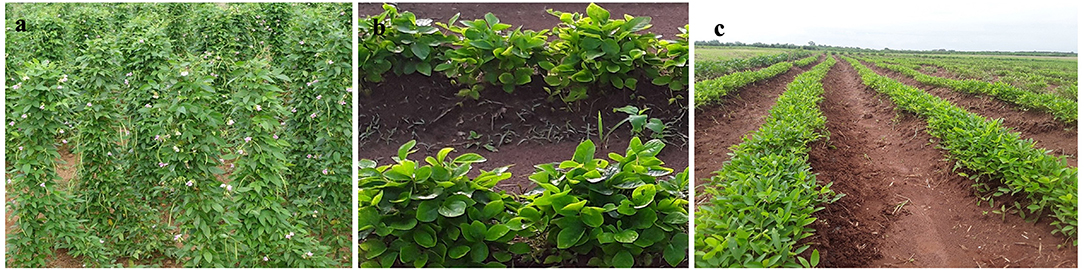
Figure 1. Field images of African orphan legumes (a) African yambean, (b) Kersting's groundnut, (c) Bambara groundnut.
Origin and Domestication
AYB is mostly cultivated in West and Central Africa (Bhat and Karim, 2009; Toyosi et al., 2020) especially in Nigeria, Ghana, Cote d'Ivoire, Cameroon, and Togo. Its domestication cannot be traced to a single locality as the occurrence of the wild relatives has been reported in different parts of Africa (Idowu 2014; Potter and Doyle, 1992). The different possibilities of the origin have been proposed (Dalziel, 1937; Harlan, 1971; Kay, 1973; Okigbo, 1973; Potter and Doyle, 1992). One of the hypotheses proposed a single domestication event in Central Africa (Potter, 1992), West Africa (Murdock, 1959; Purseglove, 1976), or Ethiopia (Dalziel, 1937), after which the domesticated races were dispersed by humans till date. Okigbo (1973) proposed two events of domestication and suggested that the domestication of the cultivated seed races might have occurred in West Africa, while the domestication of tuber-cultivated races may have occurred in Central Africa. Based on the chloroplast DNA, isozyme, and morphological variation, Potter and Doyle (1992) suggested two hypotheses: (a) it was domesticated independently in West and Central Africa, but its selection involved a single restricted gene pool; (b) a single domestication event happened in one of the two regions, and later, human dispersal occurred to the second regions prior to the dispersal within either region. Similar hypotheses were reported by Harlan (1971), which suggested that the AYB is not assignable to a single center of origin, and its center of origin should be classified based on its ecological zones in which it was domesticated (West and Central Africa).
Germplasm Collections
The majority of the holders of the AYB germplasm are aged female subsistence farmers. Therefore, preventing the genetic erosion of AYB requires a concerted effort on the exploration, collection, and conservation of the germplasm; the GRC, in collaboration with national gene banks and other national research institutes, has consistently been carrying out a germplasm exploration of AYB. The center currently conserves a diverse collection of over 450 accessions of the AYB landraces collected from Ghana, Nigeria, and the Democratic Republic of the Congo and over 50% of the accessions collected from Nigeria. The phenotypic characterization of this diverse collection is in progress according to the AYB crop descriptor at GRC, IITA (Figure 2).
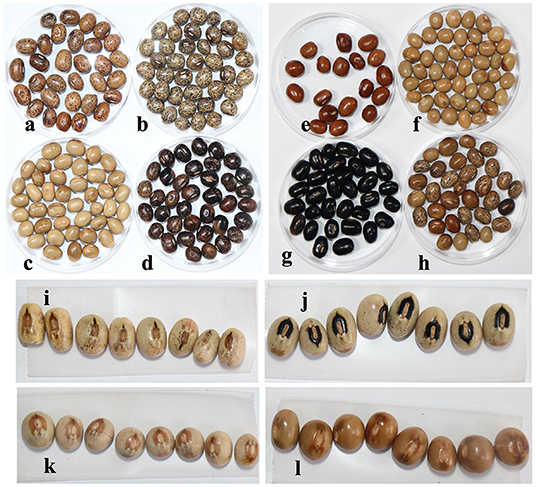
Figure 2. Genetic varaition of seed triats in African yambean landraces for seed basel color (a) TSs-24 brown basal color, (b) TSs-27 Cream basal, (c) TSs-30 not variegated, (d) TSs-67 black basal; type of seed shape (e) TSs-39 Oval, (f) TSs-1 Rhomboid, (g) TSs-66 Oblong, (h) TSs-62 Round/globular; and seed eye color (i) TSs-81A clean eye color of white seed, (j) TSs-47 black eye color of white seed, (k) TSs-11 brown eye color of white seed, (l) TSs-1 non-white seed.
Economic Potential and Utilization
AYB is an efficient nitrogen-fixing legume (Assefa and Kleiner, 1997; Oganale, 2009). It is a dual food crop that produces seed and underground tubers that are like potatoes (Adewale and Dumet, 2010). The nutrient density of AYB gives it potential as a crop to mitigate the challenge of malnutrition faced in SSA. It has a crude protein content of 21–29% in its seed (Edem et al., 1990; Eromosele et al., 2008; Abioye et al., 2015; Duodu and Apea-Bah, 2017; Anya and Ozung, 2019; Adegboyega et al., 2020). The protein content of AYB compares favorably with other legume crops including common bean, chickpea, BG, and pigeon pea (Toyosi et al., 2020). Ajibola et al. (2016) found that albumin and globulin are the most common proteins that are present in AYB seeds. Its seeds are also rich in carbohydrates (Oshodi et al., 1997; Klu et al., 2000; Ndidi et al., 2014; Ajibola and Olapade, 2016), dietary fiber (Ndidi et al., 2014; Baiyeri et al., 2018; Anya and Ozung, 2019), and high levels of essential amino acid present in the protein, especially lysine and methionine levels (Okigbo, 1973; NRC., 2007; Ade-Omowaye et al., 2015; Chinonyerem et al., 2017). The AYB is also a source of essential minerals, namely, calcium, iron, magnesium, and zinc, in which the content is either higher or comparable to soybean and common bean (Adamu et al., 2015). Adeyeye and Agesin (2007) reported that protein, carbohydrate, and mineral concentrations were found in the hull of AYB samples, suggesting higher concentrations of minerals, protein, and carbohydrates in AYB seeds with its hull than in the dehulled AYB seeds.
Aside from its nutritional richness, AYB is also a source of bioactive and phytochemical compounds (Ade-Omowaye et al., 2015; Uchegbu, 2015; Soetan et al., 2018), which are secondary metabolites that may provide health benefits by decreasing the risk for several lifestyle diseases including degenerative disease linked with an antioxidant imbalance in the human body and cardiovascular disease (Oboh, 2006; Oboh et al., 2009; Ade-Omowaye et al., 2015). Phenolic acids and flavonoids are the two most essential bioactive compounds that were reported in the AYB (Oboh, 2006; Oboh et al., 2009; Ade-Omowaye et al., 2015; Soetan et al., 2018).
The crop also has possible medicinal importance (Potter, 1992), as the paste prepared from its seeds is used as a cure for stomachaches and traditionally for the treatment of acute drunkenness (Asuzu, 1986). High levels of antinutritional factors (ANFs) and long cooking time (Fasoyiro et al., 2006) as well as low seed yield (Saka et al., 2004), agronomic demand for stakes, long maturation period, and photoperiodic sensitivity (Okpara and Omaliko, 1995) have greatly limited the utilization of the AYB.
Constraints to Yield Production
AYB has a lot of potential in terms of its nutritional contents and resilience capabilities, but the AYB production has been limited by many constraints, ranging from the lack of improved varieties due to little or no research attention on the crop to high ANFs, long cooking time (Fasoyiro et al., 2006), low seed yield (Saka et al., 2004), laborious staking requirement, photoperiodic sensitivity, non-synchronized pod maturity, and long maturation period (Okpara and Omaliko, 1995). These factors have been the major setbacks for the commercialization of the AYB, particularly in the SSA where the climatic condition for AYB production is mostly favorable. Adequate research attention is therefore necessary to overcome these challenges.
Kersting's Groundnut
KG [Macrotyloma geocarpum (Harms) Maréchal & Baudet] is also known as the geocarpa groundnut or ground bean (Dako and Vodouhè, 2006; Figures 1b, 3). KG [M. geocarpum (Harms) Maréchal and Baudet] is a leguminous crop cultivated on a small scale in the western part of Africa (Pasquet and Vigna, 2001). It is an annual herbaceous legume that is grown in arid and semi-arid regions. It is the third subterranean legume after the groundnut and BG (Adu-Gyamfi et al., 2011). It is an indigenous, high-protein, and herbaceous legume that is grown in arid and semi-arid areas of West Africa (Aremu et al., 2006). Studies have shown that it is mainly grown for its seeds (Buah et al., 2006).
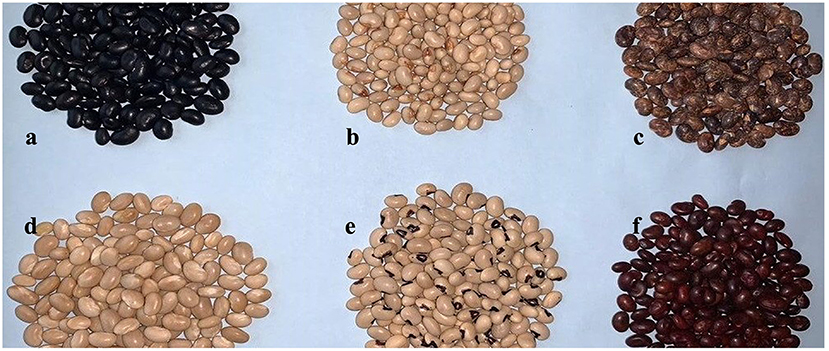
Figure 3. Kersting's groundnut seed coat colors (a) Black, (b) White mottled with grayed orange eye, (c) Brown mottled with grayed orange eye, (d) White, (e) White mottled with black eye, (f) Brown. Source: Coulibaly et al. (2020).
Origin and Domestication
The center of origin of KG is not precisely known, but it is believed to be in northern Togo or central Benin (Dako and Vodouhè, 2006). KG is cultivated in Mali, Nigeria, Burkina Faso, Ghana, Togo, and Benin with low morphological diversity, manifested only in the seed color (Pasquet and Vigna, 2001). Outside West Africa, it is cultivated in Tanzania, Mauritius, and Fiji (Aremu et al., 2006; Dako and Vodouhè, 2006). Harms Kersting, a German colonial civil servant, first named it Kerstingiella geocarpa Harms from the domesticated material collected in Togo during the years 1905 and 1907 (Harms, 1908). Two years later, Chevalier described it as Voandzeia poiaaoni A. Chev. from the materials collected in Benin, but, a few months later, Chevalier recognized that his plant was identical to the one described by Kersting. KG was found to have cogeneric characters with Macrotyloma and, therefore, given a new name, M. geocarpum by Marechal and Baudet (Amujoyegbe et al., 2007). The studies carried out by Baudoin and Mergeai (2001) showed that KG is a typical West African plant whose wild ancestry was discovered in Cameroon and Central African Republic on the banks of the Ubangi river.
Germplasm Collection
Many African indigenous legumes are almost forgotten and no longer widely cultivated; hence, there is an urgent need to capture the diversity to avoid genetic erosion. KG is one of the important but neglected indigenous legumes that are almost going into extinction. GRC conserves only 28 accessions of KG from Nigeria and Ghana. Out of 28 accessions, 22 accessions were recently collected from farmers in the 3 western states of Nigeria where the crop is being majorly grown; these limited number of accessions call for the urgent need for exploration of its germplasm to pave way for the genetic improvement of the crop. In January 2019, a GRC team in collaboration with the National Center for Genetic Resources and Biotechnology embarked on a mini exploration of KG (Macrotyloma geocarpum) in the areas historically known for the cultivation of the crop in the Ondo and Benue state of Nigeria (Figure 4A). Two local government areas (LGAs) of the Ondo and Benue states were selected for germplasm exploration based on the predominance of KG production in those areas. The KG collection was carried out in eight LGAs of the state (Akoko Northwest, Akoko Northeast, Akoko Southwest, Akoko Southeast, Owo, Ose, Akure South, and Akure North) which covers about 2,465.6 km2 and is situated at an altitude between 270 and 2,750 m above sea level. The Benue state lies within the Lower River Benue Trough in the middle belt region of Nigeria. Five villages from each local government were selected based on their involvement in KG farming. A total of twenty-two accessions of KG consisting of the different cultivars that are predominately being grown in these areas were collected directly from farmers for morphological and molecular characterization.
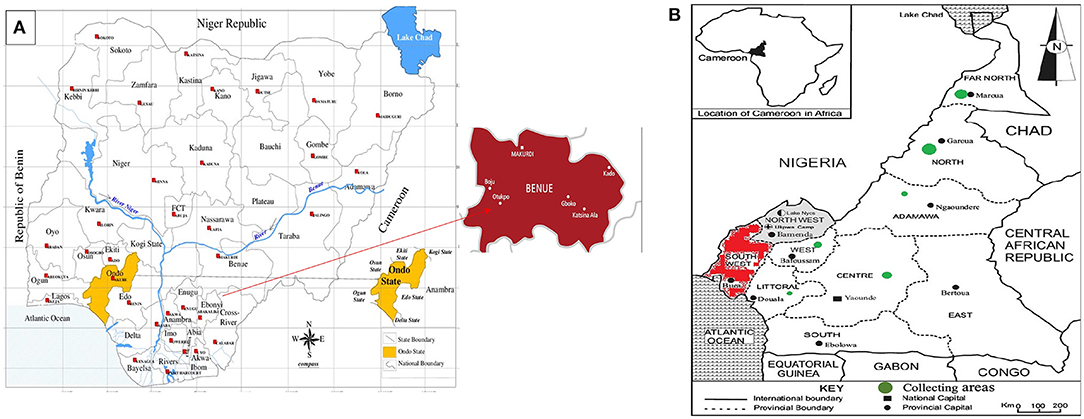
Figure 4. Germplasm collection collected in year 2019 for African orphan legumes; (A) Map of geographic locations in Nigeria showing Ondo and Benue state where Kersting's groundnut germplasm were collected, (B) map of geographic locations in Cameroon where Bambara groundnut germplasm collected (The map is adapted from Henry and Roger, 2012). The collecting areas of Bambara groundnut are shown in green circles in the map.
Economic Potential and Utilization
KG is well-adapted to drought regions and seeds with high nutritional values. It is used as a multipurpose plant in which its seeds are utilized for food, animal feed, and traditional medicine, while its leaves and vines are also used for animal feed (Agoyi et al., 2019). KG is currently being cultivated on a relatively small scale by local farmers in the West African savanna zones of Cameroon and Chad, The global production statistics for KG are not available but there have been reports of its production in Fiji, Mauritius, and Tanzania (Amuti, 1988). Its seeds are orthodox, which can be stored in a dry condition (15–20% relative humidity) as well as in −20°C (Ayenan and Ezin, 2016). The seeds are highly nutritious and contain protein (21.3%), crude fiber (6.2%), many essential amino acids, particularly arginine, histidine, methionine, and lysine, vitamins, ash (3.2%), and carbohydrates (61.53–73.3%) (Oyetayo and Ajayi, 2005; Ajayi and Oyetayo, 2009; Aremu et al., 2011). Arginine is the most common amino acid in its seeds and well-studied for arginine's role in the growth of children (Pittari et al., 1992; Ajayi and Oyetayo, 2009; Van-Vught et al., 2013). Its seeds also provide many important minerals, namely, Ca, Fe, Na, P, Mg, and K (Aremu et al., 2006). They have low fat content, which offers an optional food for those who wish to lose weight (Ajayi and Oyetayo, 2009). Its fresh green leaves are used either in vegetables or added in soup (Mergeai, 1993). Many people boiled its seeds with water to drink as a remedy of diarrhea and also mixed its dry seed powder with hot water to use as an emetic in case of poisoning (Amuti, 1988; Ayenan and Ezin, 2016). As an orphan crop, KG is known as hardy and climate resilient, which adapts in drought regions where it can be sustained in low rainfall (500–600 mm) (Mergeai, 1993; Agoyi et al., 2019) and poor sandy and acidic soils (Agoyi et al., 2019). It can be a potential crop option in a vulnerable climate scenario, particularly for intra-season drought in West African regions (Masih et al., 2014; Ologou, 2015).
Constraints to Yield Production
Several reasons for the decrease of KG were reported by the farmers interviewed during the survey conducted by the GRC in 2019, among which are high costs of inputs (93.6% of responses), susceptibility to high soil moisture (68.1%), and high labor requirement (85.1%) (Table 1). It is usually planted between June and August, and most farmers do not intercrop it. This makes the cost of production very high, which is one of the major reasons why many farmers tend to abandon the cultivation of the crop.
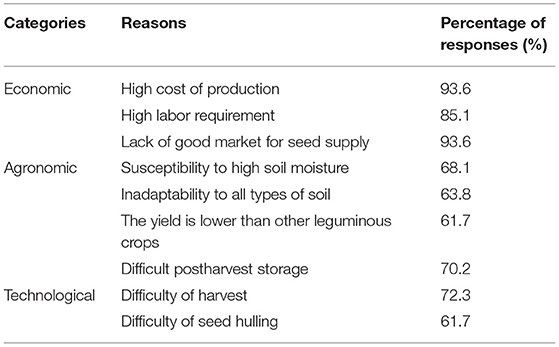
Table 1. Reasons for decrease in Kersting's groundnut production based on IITA genebank survey in year 2019 in Ondo and Benue States of Nigeria.
Farmers use various traditional conservation tools (buckets, sacks, jar, calabash, metal drums, etc.) to preserve the seeds. KG seeds are very susceptible to insect infestation (Eric et al., 2019), particularly weevils; therefore, different preventative methods are employed by farmers to minimize insect infestation, among which are using cotton insecticide (27.6% respondents), wood ash (14.9% respondents), and the plant extract from the neem tree (Azadirachta indica) (6.4% respondents). The storage period ranges from 5 to 12 months, depending on the type of preservation method used. The survey revealed that most of the farmers that are still cultivating KG in Nigeria are women above 50 years old; this corroborated the reports of Amujoyegbe et al. (2010) and Bampuori (2007). Most of the farmers cultivate the crop mainly for subsistence purposes due to the high cost of production and post-harvest handling (Assogba et al., 2016). There is an urgent need to capture the available genetic resources, particularly in West Africa, where there are few KG farmers to prevent genetic erosion and the possible extinction of this important legume.
Bambara Groundnut
BG [Vigna subterranea. (L.) Verdc.] belongs to the Leguminosae family, it is a popular crop in many African countries (Ahmad et al., 2013; Figure 1c). It is similar to the groundnut (Arachis hypogea) in terms of the nutritional value and vegetal and culinary attributes (Boateng et al., 2013). It is known to be capable of tolerating adverse weather conditions as it can tolerate a range of environmental conditions and durations of stress (Feldman et al., 2019). BG has a chromosome number 2n = 2x = 22 (Heller et al., 1997). Two botanical varieties of BG exist, namely, V. subterranea var. spontanea, which includes the wild types found in Cameroon, Sudan, and some parts of Nigeria and V. subterranea var. subterranea, which is widely cultivated in SSA (Basu et al., 2007).
Origin and Domestication
BG [V. subterranea (L.) Verdc] is believed to have originated from Central Africa prior to the introduction of groundnut (Arachis hypogea) from South America (Goli, 1997). Hillocks et al. (2012) reported Nigeria to be the center of origin of BG. BG has two botanical varieties: V. subterranea var. spontanea, which is comprised of the wild types in Sudan, Cameroon, and parts of Nigeria, and V. subterranea var. subterranean. BG is one of the underutilized legumes with a lot of potential for resilience and possible climate-smart crop for the future. BG is mainly cultivated for its edible seeds (Olukolu et al., 2012).
Germplasm Collection
GRC conserves over 1,700 accessions of BG from 28 African countries, which makes it the most diverse collection of BG crops globally. Most of the BG collection (68%) belonged to Nigeria (310 accessions), followed by Zambia (278 accessions), Zimbabwe (235 accessions), Cameroon (205 accessions), and Togo (134 accessions), respectively. Although IITA has the largest accessions in conservation as reported by Olukolu et al. (2012), a gap analysis based on passport information has been carried out. This analysis identified Cameroon as the target gaps and hotspot areas of high diversity for BG. In Cameroon, the hotspot regions of diversity were mapped out and then a collection of sixty-one BG samples was made in the year 2019. All these samples were collected from the West, Littoral, Central, Adamawa, North, and the Far-North regions of Cameroon (Table 2, Figure 4B). This collection will help to enhance the genetic diversity of the existing BG collection and provide an additional genetic diversity from Cameroon for future breeding research. The field characterization of vast BG collection is in progress for different phenotypic traits according to the BG crop descriptor at the GRC, IITA (Figure 5).
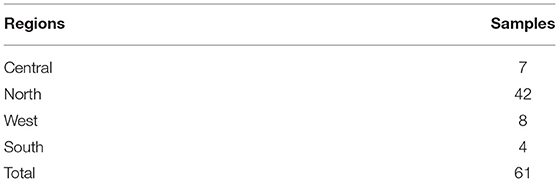
Table 2. Bambara groundnut samples collected in four different regions of Cameroon in the year 2019.
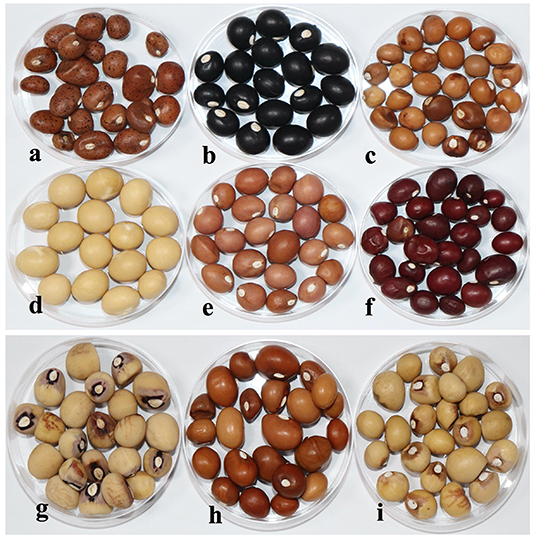
Figure 5. Genetic varaition of seed triats in Bambara groundnut landraces for seed color (a) TVSu-1749 others type, (b) TVSu-1918 black, (c) TVSu-1574 tan brown, (d) TVSu-1981 cream, (e) TVSu-1399 light brownish red, (f) TVSu-2032 dark red and seed shape type (g) TVSu-1412 round type, (h) TVSu-2014 other type, (i) TVSu-1397 oval type.
Economic Potential and Utilization
BG is an indigenous orphan crop that is the most widely cultivated as a legume food source after the cowpea in many countries of the SSA because it provides balanced food with high nutritional composition and has a climate-resilient nature (Mayes et al., 2019; Paliwal et al., 2020, 2021). It is well-known as hardy and climate-resilient crop that can survive in poor soils and drought regions, particularly in the semi-arid zones with low rainfall (<800 mm) of the SSA where other crops fail to survive (Gunjal et al., 2009; Mubaiwa et al., 2018; Feldman et al., 2019; Mayes et al., 2019). Its major climate adaptive trait is drought resistance which make it more popular in local populations of SSA (Adzawla et al., 2016a,b; Olayide et al., 2018). Nigeria is the highest producer of BG (100,000 MT), followed by Burkina Faso (44,000 MT), Niger (30,000 MT), Mali (25,000 MT), and Cameroon (24,000 MT) (Hillocks et al., 2012; Aviara et al., 2013). In Africa, the average BG yield was reported as 0.85 t ha1, while its yield production ranged between 0.5 and 3.0 t ha1 (Azam-Ali et al., 2001; Mayes et al., 2019). Under drought, BG can produce a reasonable seed yield from 1.3 to 2.1 t ha1 (Mwale et al., 2007); that yield is higher than chickpea and drought-tolerant groundnut varieties (Leport et al., 1999; Collino et al., 2000). Its biological nitrogen fixation (BNF) activity helps it to survive in poor soils with marginal input (Paliwal et al., 2020). BG is rich in protein, carbohydrates, dietary fiber, and minerals, which make it a cheap complete balanced food (Mubaiwa et al., 2018; Paliwal et al., 2020). Several reports on nutritional composition have shown that its seeds per 100 g of dry weight may provide 15–20% protein, 49–63.5% carbohydrates, 5.2–6.4% dietary fiber, 0.70% ash, and 4.5–7.4% fat (Onimawo et al., 1998; Murevanhema and Jideani, 2013). BG milk is more preferred than other legume's milk because of its color, flavor (Goli, 1997), and especially for its nutritional content with high protein (15–16%) compared to 4% protein of soymilk (Murevanhema and Jideani, 2013). Its seeds are also rich in mineral content, namely, Ca, Fe, Zn, and K (Karikari et al., 1997). Interestingly, Muimba-Kankolongo (2018) indicated that red-seed cultivars have almost double Fe content compared to the cream seed cultivars and these red-seed cultivars could be used for cultivation in the iron-deficiency regions of SSA. The characterization of the phytochemical properties of BG has been initiated, and it is reported that its antioxidant potency is equal to the other legumes such as chickpea, lentils, and common beans (Nyau et al., 2015). Many other secondary metabolites such as flavonoids, tannins, and alkaloids have been identified in BG and a study also shows that the alkaloids possess analgesic properties (Olaleye et al., 2013). The antimicrobial and antioxidant properties of BG could be utilized in the treatment of many ailments (Udeh et al., 2020). There are still more efforts needed toward examining the antimicrobial and nutraceutical values of BG (Udeh et al., 2020) against the pathogens of economic importance.
Constraints to Yield Production
BG has constraints that reduce not only the yield but also the acceptability due to the long cooking time and ANFs. A successful hybridization of the available landraces can make a difference in addressing various bottlenecks in BG, especially the yield and adaptation to diverse environments with respect to climate change. The constraints such as the long cooking time discourage consumers as it takes more fuel to cook BG, especially the dried types. Moreover, the beany taste after cooking, when prepared as a meal, is another constraint surrounding its acceptability; this is due to the ANFs present in BG. It will be quite interesting to assess the ANF level in diverse BG genotypes conserved in the gene bank for its improvement. An assessment of the available germplasm can show variation in BG ANFs and the discovery of genotypes with low ANF and short cooking time, which might improve nutrition and acceptability. A BG meal preparation varies from one country to another. In Nigeria, it is mostly consumed either as a snack by boiling the fresh pods with salt as refreshment or prepared as a meal called okpa. This meal is very common in the middle belt and eastern part of the country. Okpa is prepared by soaking the dried BG seeds in water overnight, dehulling them, and adding some ingredients like cray fish and pepper and grinding the mixture to become paste, red oil, or vegetable oil. Other condiments are added, and the mixture is thoroughly mixed and divided into small containers or wrapped in leaves and steamed by cooking in a pot for about 20–30 min.
Another constraint is uneven germination, which can cause yield reduction. BG is mostly cultivated in a dry environment, and the of soil moisture stress, especially from flower production and at other reproductive stages, is another major production constraint (Smart, 1985; Begemann, 1990; Linnemann and Azam-Ali, 1993; Sesay et al., 1999; Hampson et al., 2000), although the effect varies from landraces (Massawe et al., 2005). There is a need for a concerted research effort to address these constraints using selected parental and promising lines that have adapted different agro-climatic zones. Till date, no improved varieties of BG are available, neither are there any research centers for the improvement of this crop. To improve the yield and to address the bottlenecks in BG, the artificial hybridization of this crop is crucial (Massawe et al., 2005). The outcome of past works using morphological and molecular characterization and high-throughput molecular markers to evaluate BG has brought about the discovery of some BG landraces with useful traits for further breeding work. The University of Nottingham, United Kingdom and Crops For the Future Research Center, Malaysia have characterized and evaluated some landraces using high-throughput molecular markers and a shelter house to study various BG genotypes with diverse origin. The origin of these selected landraces spans through Africa and Southeast Asia (IITA Nigeria, Namibia, Botswana, Ghana, and Indonesia). This work has resulted in the identification of some landraces that can be used as a parent stock to address BG improvement for biotic and abiotic stresses through hybridization (Massawe et al., 2005; Sesay et al., 2010; Mayes et al., 2013; Gao et al., 2020).
Indigenous African Orphan Legume Crop Breeding
Currently, the farm-level selection of these orphan crops has been carried out where farmers evaluate and multiply the seeds of existing landraces and store them for next-cycle crop production (Massawe et al., 2005). Because of the absence of the targeted breeding of these crops, there are few improved cultivars developed. One of the significant challenges of orphan crop breeding is their low yield production compared with other major crops (Ademola et al., 2020; Sidibe et al., 2020; Temegne et al., 2020). The low crop production may be due to poor seed germination and/or no fertilizer. In BG, the available genetic resources show significant variation for important breeding traits like the flower number, days to maturity, photoperiod sensitivity, leaf number, pod development, pod number, pod weight, seed color, cooking time, and yield (Massawe et al., 2005). These traits could play a critical role in developing farmer-preferred high-yielding improved lines in BG. The days to maturity should be taken into account to develop early- and late-maturity cultivars to avoid overstaying the pod in the soil after the pod maturity. Several reports indicate that BG is less affected by insects and pests than other legumes (Tanimu and Aliyu, 1990; Purseglove, 1992). BG is also infested by several fungal and viral diseases (Brink et al., 2006; Hema et al., 2014; Fourie et al., 2017). Kosini and Nukenine (2017) reported that Bruchids (Callosobruchus maculatus) are significant pests attacking the BG seeds during crop storage. In AYB, the flowering time, crop maturity, photoperiod sensitivity, pod shattering, cooking time, antinutritional factors, seed color, pod yield, pod number, and seed yields are observed as important traits during the field characterization at GRC-IITA. These traits could be considered for developing improved high-yielding cultivars under AYB crop breeding programs. In these orphan crops, yield loss from biotic stresses is less than abiotic stresses, particularly heat and drought, which are the major yield reduction factor causing unstable yield. The breeding research on nutritional traits and antinutritional characteristics is limited in these orphan legume crops. Under climate change, the breeding should focus on high-yielding, climate-resilient traits, the cooking time, nutrition, and ANF traits in these orphan African legume crops.
Genomic Approaches Toward Genetic Improvement of Indigenous African Orphan Legumes
The breeding of these crops has been very limited and based mainly on landrace selection (Zeven, 1998; Mayes et al., 2019). The breeding and genomic research of indigenous African orphan legumes lag far behind other major legume crops, including cowpea, soybean, common bean, pigeon pea, and chickpea. Very limited molecular studies have been carried out. Because of the advances in next-generation sequencing (NGS), the genetic improvement of crops, including major legume crops, has accelerated in recent years through the integrated utilization of conventional and genomic-assisted breeding tools. Recently, high-throughput sequencing genotyping is highly demanded and globally applied on crop molecular breeding for the genotyping of large volumes of DNA samples faster, cost-effectively, and with better genome-wide coverage (Elshire et al., 2011; Poland and Rife, 2012). The application of these cutting-edge genomics tools has been used in crop breeding research for the speedy genetic gain of climate resilience traits and nutritional traits (Tables 3, 4).
Advances in NGS technology have enhanced our knowledge of the genomes of over 236 plant species (Chen et al., 2018), including indigenous African orphan legume crops. The African Orphan Crops Consortium (AOCC; http://www.africanorphancrops.org) targeted 101 African orphan crops, to sequence, assemble, and annotate the genomes that contribute to traditional African food supplies (Hendre et al., 2019). Recently, both the nuclear (550 Mbp) and mitochondrial (152,015 bp) genomes of BG have been sequenced using high-density Illumina short-read data (Chang et al., 2018a,b; Liao et al., 2019). The whole-genome sequencing of AYB is in progress using a hybrid sequencing approach (Illumina short-read data and Oxford Nanopore) under the Alliance for Accelerated Crop Improvement in Africa (https://acaciaafrica.org/bioinformatics-community-practice/full-genome-sequencing-and-annotation-of-the-african-yam-bean/) at the Bioscience eastern and central Africa-International Livestock Research Institute (BecA-ILRI) Hub, Nairobi, Kenya. The complete genome sequencing of KG is also in the list of sequencing crops of 101 species and is yet to be begun by the AOCC. The genome sequencing results will help single-nucleotide polymorphism (SNP) analysis, leading to a better understanding of the genetic diversity, syntenic relationship with other legume crops, development of linkage map, precise QTL/gene discovery, and enhanced molecular breeding.
Exploring the knowledge of genetic diversity enables the selection of parental lines for population development. Different generations of molecular markers [AFLP (amplified fragment length polymorphism), isozyme, RAPD (random amplified polymorphic DNA), ISSR (inter simple sequence repeat), SSR (simple sequence repeat), DArT (diversity arrays technology), and SNP] have been used to explore the genetic diversity and population structure analysis (Table 3). Initially, isozyme markers were utilized in BG to explore the genetic diversity between domesticated and wild accessions and reported higher diversity in the wild (Ht = 0.087) than cultivated (Ht = 0.052) accessions, while the intrapopulation diversity was vice versa (Pasquet et al., 1999). The genetic diversity analysis of 363 accessions using RAPD and ISSR markers showed a close genetic relationship between Cameroon, Nigerian, and other West African accessions (Rungnoi et al., 2012). They reported two population structures where most of the accessions belonged to the first group and the remaining eleven accessions in the second subpopulation structure. Many other genetic diversity studies based on the SSR and SNP markers also reported similar results and indicate two major populations, namely, the West and Central African and South and East African populations (Somta et al., 2011; Molosiwa et al., 2015), while Redjeki et al. (2020) reported three populations in which the third subpopulation was from an Indonesian collection.
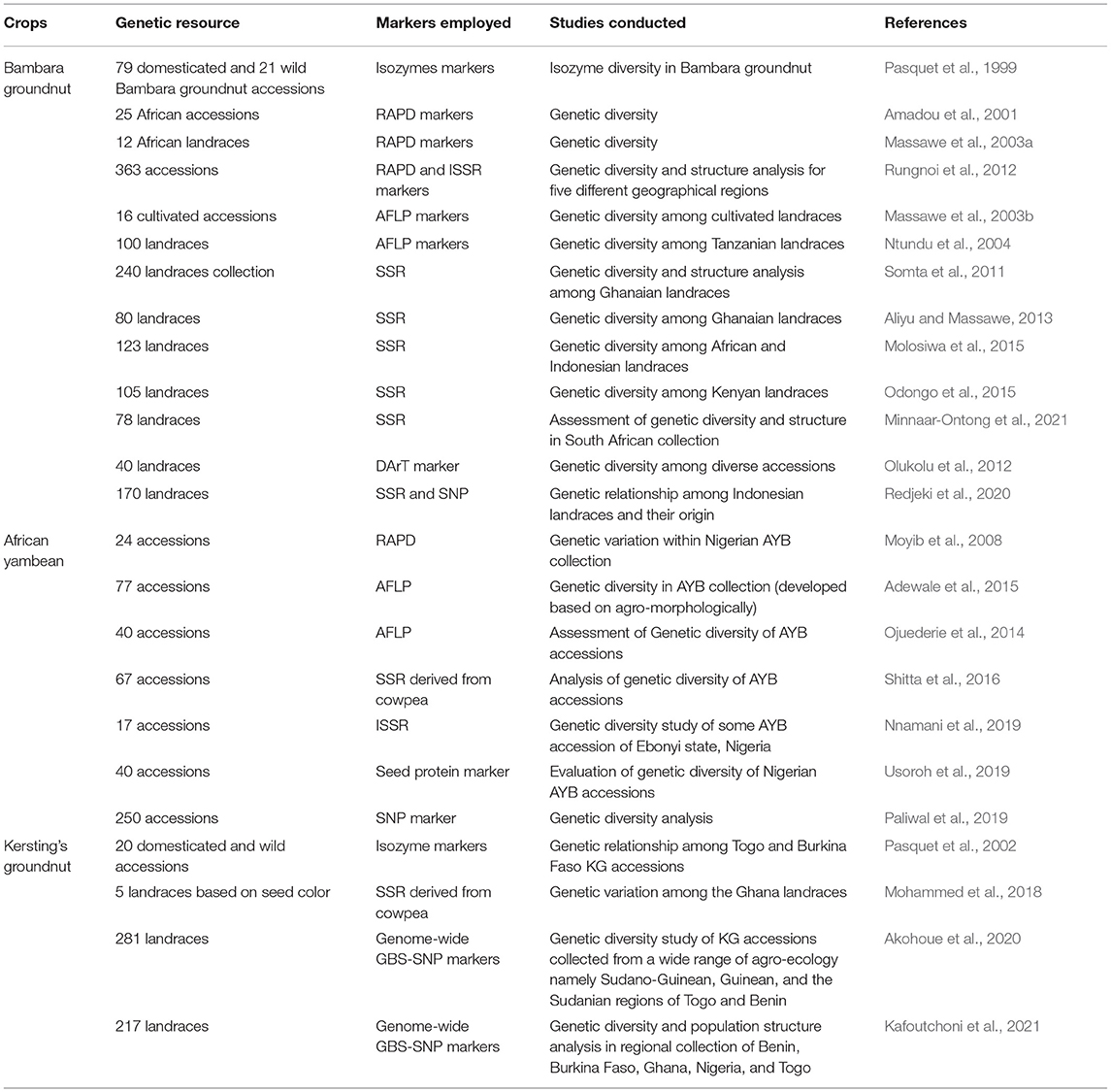
Table 3. Plant genetic resources characterized using DNA markers in indigenous African Orphan legumes.
In AYB, several types of DNA markers, namely, RAPD (Moyib et al., 2008), AFLP (Ojuederie et al., 2014; Adewale et al., 2015), SSR (Shitta et al., 2016), and ISSR (Nnamani et al., 2019), have been utilized and showed that the wide genetic diversity in the genetic variation of different populations was mostly clustered in 3–4 clusters for genetic diversity (Adewale et al., 2015; Shitta et al., 2016; Nnamani et al., 2019) and population structure analysis (Ojuederie et al., 2014; Nnamani et al., 2019).
In KG, a collection of 281 accessions was grouped into four clusters (neighbor-joining tree method) based on white, red, black, and white with black eye seeded accessions, and it was grouped into two subpopulations with high differentiation across ecological regions that indicate the effect of geographic origins on the genetic diversity (Akohoue et al., 2020). Interestingly, another study with 217 accessions was grouped in eight clusters using the discriminant analysis of principal components and NJ tree method, based on the Edwards distance (Kafoutchoni et al., 2021). Cultivated landraces could be used as founder lines for intercrossing with diverse landraces to build a broader genetic base for future breeding use.
The domesticated/adapted landraces of African orphan legumes are widely cultivated across Africa in the absence of improved varieties. Genomic tools, namely, linkage mapping, QTL discovery using both biparental mapping and a genome-wide association study (GWAS), MAS, and genomic selection (GS), have been utilized in the recent past to speed up major legume crop (soybean, cowpea, chickpea, and common bean) breeding programs to develop high-yielding cultivars. The use of these genomic tools in African orphan legumes has been initiated and is in the early stage of progress. An integrated genetic linkage map has been reported for BG using two mapping populations (IITA686 × Ankpa4; F2 263 lines and Tiga Nicuru × DipC; F3, 71 lines) that generated 11 linkage groups with DArT Silico (presence/absence) and DarTseq SNP makers (Ho et al., 2017). The total distance covered by both linkage maps ranged from 1,376.7 (F3) to 1,395.2 cM (F2), which was higher than the earlier-reported 608.3 cM distance of 21 linkage groups by Ahmad et al. (2016). These mapping populations have been used for QTL discovery and identified several QTLs for morphological, growth-habit, and yield-related traits (Table 4; Ahmad et al., 2016; Ho et al., 2017). The SNP position of internode length QTL showed a syntactic relationship with other legume genomes, namely, cowpea, mung bean, and adzuki bean (Ho et al., 2017), a possibility of discovery for potential conserved candidate genes in close-related species genomes for the underlying traits of interest. There is no GWAS study available in BG, but it is in progress for different yield-related traits, drought, and nutritional traits at GRC. Recently, in the AYB, a GWAS study reported on the marker–trait association for protein, starch, and oil traits (Table 4; Oluwole et al., 2020). They identified 31 linked QTLs for protein, 11 QTLs for starch, and 8 QTLs for oil traits. So far, there is no linkage and the QTL mapping report is available in the AYB. The development of mapping populations for yield traits and abiotic stresses has been initiated, and its advancement (F2 generation) is in progress at the GRC. Similarly, there is no study reported for linkage and QTL mapping in KG, while recently, a marker–trait association research was reported by Akohoue et al. (2020) for morphological traits (days to 50% flowering and days to maturity) and yield-related traits including 100 seed weight and grain yield. GS in African orphan legumes is only reported for KG where GS revealed a moderate-to-high prediction accuracy using the stratified sampling technique (Akohoue et al., 2020). They found a strong correlation between the observed and predicted breeding value of 100 seed weight (0.62), days to 50% flowering (0.79), and days to maturity (0.72).
Molecular studies (Tables 3, 4) in African orphan legumes are in the very early stage and need more efforts to develop different types of mapping population (F2, recombinant inbred lines, backcross, double haploid mapping population, and near-isogeneic lines) including MAGIC (multi-parent advanced generation inter-cross) population and its QTL mapping, GWAS, and GS, which will provide the opportunity to identify more precise and stable QTL/genes (Huang et al., 2015; Varshney et al., 2018) for yield and climate-adapted traits that could be further used in MAS to speed up the development of improved climate-resilient lines. GWAS and GS approaches could be more helpful for the crop genomics research of BG because developing different types of mapping population is not easy as compared to AYB and KG due to the reproductive biology of the BG crop. Several reports indicate that the attempts made at crossing by hand pollination among parental lines were not successful in a natural field condition (Goli, 1997; Suwanprasert et al., 2006). In BG, QTL mapping could be useful in the mapping populations that are developed under the artificial crossing method, optimized in the United Kingdom (University of Nottingham) and Thailand under the BAMLINK initiative project funded by the European Union for BG research (https://cordis.europa.eu/project/id/15459/reporting). More efforts are required for the whole-genome sequencing and resequencing of many diverse lines to develop the pan-genome reference, which will increase the accuracy of SNP discovery and identify structural variation, comparative genomics with other close-relative legume species, and breeding efforts (Tao et al., 2019; Yang et al., 2019) to increase the commercial value. A recent advanced biotechnological tool known as gene-editing technology could play a vital role in indigenous African orphan legume crop breeding. The gene-editing technology has utilized different types of sequence-specific nucleases such as zinc finger nucleases, transcription activation-like effector nucleases, and CRISPER-Cas (clustered regularly interspaced short palindromic repeats) in gene-editing research. The CRISPER-Cas gene-editing tool is widely used in crop research for editing the genes for complex traits like yield, biotic and abiotic stresses, and undesirable traits in different crops (Makhotenko et al., 2019; Wang et al., 2019; Bouzroud et al., 2020; Zeng et al., 2020; Zheng et al., 2020; Li et al., 2021; Liu et al., 2021) including the legumes soybean (Zhang et al., 2020), alfalfa (Meng et al., 2015), and cowpea (Ji et al., 2019). In groundcherry, three ortholog genes (SP, SP5G, and CLV1) that control the plant architecture and flower production and fruit development were introduced from tomato to improve major productive traits in groundcherry (Lemmon et al., 2018). This technology could play a crucial role to edit the gene control flower morphology in BG to make the hand-made hybridization crossing technique easy and successful. Therefore, it could also be utilized to improve yield, climate-resilient traits, and antinutritional traits in the future crop breeding of indigenous African orphan legumes.
Biological Nitrogen Fixation (BNF)
In SSA, low productivity is a common issue facing agriculture, and low yield is often linked with poor soil fertility. Most of the farmers are smallholders who cannot afford a sufficient use of fertilizers, including minerals (Yanggen et al., 1998). Most fertilizers and minerals are imported (Mugabe, 1994; Chianu et al., 2012). BNF in indigenous orphan legumes could be a key source of nitrogen for farmers to sustaining yield in marginal soil. Legume crops often develop root nodules with the compatible rhizobia population in soil, which change atmospheric nitrogen to ammonia for plant use. In the field, the reported BNF values range from cowpea (17–198 kg N ha−1) (Belane and Dakora, 2010), followed by groundnut (22–124 kg N ha−1) (Rebafka et al., 1993; Katayama et al., 1995; Ncube et al., 2007), soybean (3–112 kg N ha−1) (Sanginga et al., 1997; Chikowo et al., 2004; Mapope and Dakora, 2016), and common bean (8–31 kg N ha−1) (Ojiem et al., 2007). Pigeon pea also reported a very high BNF contribution (37–164 kg N ha−1), which is generally associated with a longer crop cycle (Adu-Gyamfi et al., 2007; Egbe et al., 2007). In SSA, the BNF values of BG have been reported of 4–200 kg N ha−1 (Mohale et al., 2014) and 32–82 kg N ha−1 under the Malaysian acidic soil condition (Musa et al., 2016). In an intercropping system, BG can fix 11.27–39.47 kg N ha−1 in the BG/maize and BG/cowpea intercrop system (Egbe et al., 2013), respectively.
In AYB, Adegboyega (2019) studied the nitrogen fixation values of 25 accessions by using the carbon isotope method and found BNF ranged from 12.76 to 22.47 kg N ha−1. Kermah et al. (2017) also reported similar results where legumes BNF ranged from 11–31 kg N ha−1 in two different geographical regions, namely, southern Guinea savanna and northern Guinea savanna of Ghana. In KG, the nitrogen fixation ranged between 12 and 50 kg N ha−1 (Mohammed et al., 2018). These reports indicate that the amount of BNF added in soil by the AYB and KG is lower than other legumes such as BG, cowpea, groundnut, and soybean (Sanginga et al., 1997; Chikowo et al., 2004; Belane and Dakora, 2010; Mohale et al., 2014). The total BNF fixed over time differs mainly due to several factors like the legume species, cropping system, variety, location/site, climate, and crop management practices. The identification of locally well-adapted rhizobia strains is also important because they could be utilized as inoculants to competitively increase BNF and crop yield in these indigenous African orphan legumes. The knowledge of taxonomy and phylogenetic relationship among rhizobia and its population is an essential step to move forward for improving the productivity of these legumes and soil health using BNF (Chidebe et al., 2018). Therefore, there is an urgent need for more studies to enhance the understanding of the phylogenetic and functional diversity of rhizobia populations that modulates these indigenous orphan African legumes.
Conservation and Distribution of Orphan Legumes
The conservation and availability of genetic resources for distribution are an important pillar for the sustainable utilization of crop diversity for global food security and a prerequisite for the improvement of any crop species. GRC-IITA is one of the CGIAR gene banks at Ibadan, Nigeria that conserves over 6,747 accessions of orphan legumes of SSA, including BG, AYB, KG, and other minor legumes. Digital object identifiers (DOIs) have been generated, for all these accessions and are available online in Genesys, which is an open-access data portal (https://www.genesys-pgr.org/). DOI is a unique alphanumeric string which gives a permanent digital reference to each accession in gene bank. DOIs are also linked with their existing identifiers so curators do not need to modify their current process. DOIs provide a promising method to standardize the identification and tracking of accessions within or across the gene banks, publications, breeding programs, and research institutes. For sustainable conservation, these collections are also backed up for safety in other CGIAR gene banks outside Africa and in the Svalbard Global Seed Vault in Norway. GRC has distributed 9,598 accessions of these orphan legumes to different parts of the world for various purposes including national and international research centers in the last 20 years (Figure 6). Out of 9,598 distributed accessions, 6,528 accessions were distributed only for BG (3,599 accessions), AYB (2,878 accessions), and KG (51 accessions) in last 10 years. The distribution pattern of orphan legumes in 10 years indicate that a higher proportion of BG (average 360 accession/year), followed by the AYB (average 288 accessions/year), was distributed to 11 different African countries and European (Germany, Spain, and the United Kingdom), and Asian (India and Indonesia) regions.
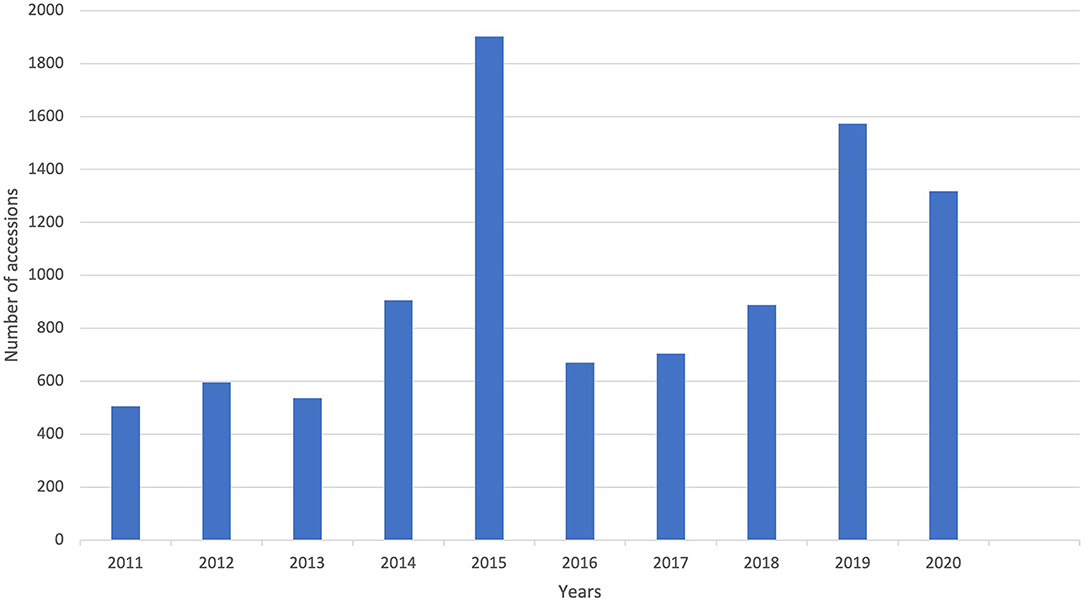
Figure 6. The number of distributed germplasm samples of orphan legumes in IITA genebank in last 10 years.
Conclusion
In this review, the potential of indigenous African orphan legumes for food and nutrition security to SSA and the need to intensify modern breeding research and fill the existing gaps in the germplasm collections and conservation were highlighted. The available genetic resources of these legumes can be utilized to ameliorate food shortage and nutrition deficiencies among the rural population; it could also serve the purpose of income generation for women and rural farmers. The available conserved collection of indigenous African orphan legumes at GRC is still insufficient, particularly AYB and KG collections, as compared to the genetic resources available for major legume crops worldwide. The availability of a large and diverse collection of genetic resources is essential for the improvement of any crop species. Most of the farmers growing these indigenous African legumes are aged women in rural areas in many parts of SSA. Genetic erosion is a clear threat, and there is a need for a strategic approach to capture the existing genetic diversity of these legumes by expanding the existing ex situ collection for both the wild and landraces of these legumes, particularly the KG and AYB, by filling the gaps in the collection in both the national and international gene banks. Adequate documentation of the traditional knowledge about the cultivation and uses of the legumes is also necessary as it is currently in the hands of the aged farmers in the rural areas where the crops are predominantly being cultivated.
The research on most of these African indigenous legumes is still far behind compared to other legumes such as cowpea, groundnut, and soybean; therefore, an investment from national and international funding agencies for the research of these orphan legumes will be necessary to develop their full potential with modern crop breeding. The available genetic resource could be utilized to identify the accession for important agronomical traits, including nutrition, biotic, and abiotic stress traits using high-throughput phenotyping and genotyping and could further be used as parental material in different breeding programs using modern breeding tools. In BG, the artificial crossing method has been developed to develop successful breeding progenies, but the development of the easy crossing method is a prerequisite to initiate large-scale breeding programs in these crops. The development of improved short duration and low anti-nutrition factors and synchronized pod maturity lines should be prioritized in AYB crop breeding programs. The government and private sector can help enhance the market demand of these crops by producing more value-added food products of these legumes for the African population using food technology.
These legumes are a combination of high-nutrition and high-calorie food, making them nutritional food security crops of poor livelihoods in SSA; reducing the ANF and long cooking time could be a significant advantage to promote their use in the food plate of the African population. Several reports indicate the significant negative effect of climate change on crop production in SSA. It can reduce up to 20–40% of crop production by 2050 in humid and West African countries. To mitigate the climate change effect, indigenous African legumes could be promoted as an alternative or substitute crops to diversify the African cropping system as well as food diets. The application of GAB tools such as high-throughput molecular characterization and modern genomic tools could play a major role to unleash the potential of these legumes and speed up the breeding programs of African indigenous orphan legumes to develop an improved cultivar for nutrition and high yielding under harsh climate. Enhancing food security in SSA countries requires improved climate-resilient and high-nutrition-content varieties of these orphan legumes to help with the SSA region's sustainable food and nutrition security. These leguminous crops will also improve soil fertility and play an essential role in integrated soil fertility management. Most of the African indigenous legumes possess a great potential for climate resilience, which could pave the way for food and nutrition security in the face of climate change, but they are still being underexploited in terms of the solution to hunger and poverty they could offer. There is an urgent need to explore more of this potential.
Author Contributions
MA and RP reviewed and edited the manuscript. All authors contributed to the writing of the original draft of the manuscript, read, and approved the final manuscript.
Funding
The work of GRC was funded by Global Crop Diversity Trust and the CGIAR. The Funders had no association in the present study design, data collection, as well as publication decision.
Conflict of Interest
The authors declare that the research was conducted in the absence of any commercial or financial relationships that could be construed as a potential conflict of interest.
Publisher's Note
All claims expressed in this article are solely those of the authors and do not necessarily represent those of their affiliated organizations, or those of the publisher, the editors and the reviewers. Any product that may be evaluated in this article, or claim that may be made by its manufacturer, is not guaranteed or endorsed by the publisher.
References
Abate, T., Shiferaw, B., Menkir, A., Wegary, D., Kebede, Y., Tesfaye, K., et al. (2015). Factors that transformed maize productivity in Ethiopia. Food Sec. 7, 965–981. doi: 10.1007/s12571-015-0488-z
Abioye, V., Olanipekun, B., and Omotosho, O. (2015). Effect of varieties on the proximate, nutritional and anti-nutritional composition of nine variants of African yam bean seeds (Sphenostylis stenocarpa). Donnish J. Food Sci. Technol. 1, 17–21. Available online at: http://donnishjournals.org/djfst/abstract/2015/august/abioye-et-al.php
Adamu, G., Ezeokoli, O., Dawodu, A., Adebayo-Oyetoro, A., and Ofodile, L. (2015). Macronutrients and micronutrients profile of some underutilized beans in South Western Nigeria. Int. J. Biochem. Res. Rev. 7, 80–89. doi: 10.9734/IJBCRR/2015/17219
Adegboyega, T. T. (2019). Genetic diversity and nitrogen fixation in underutilized tropical legumes. [Ph.D. thesis], North-West University, Potchefstroom (South Africa). Available online at: https://hdl.handle.net/10568/109972
Adegboyega, T. T., Abberton, M. T., AbdelAziz, H. A. G., Dianda, M., Maziya-Dixon, B., Olaniyi, A. O., et al. (2020). Evaluation of nutritional and antinutritional properties of African yam bean [Sphenostylis stenocarpa (Hochst ex. A. Rich. Harms.] seeds. J. Food Qual. 2020, 6569420. doi: 10.1155/2020/6569420
Ademola, I. A., Christopher, O. I., Ukoabasi, O. E., Olaniyi, O., Daniel, P., and Abberton, M. T. (2020). Morphological Characterisation and variability analysis of African yam bean (Sphenostylis stenocarpaHochst. ex. A. Rich) Harms. Int. J. Plant Res. 10, 45–52. doi: 10.5923/j.plant.20201003.01
Ade-Omowaye, B. I. O., Tucker, G. A., and Smetanska, I. (2015). Nutritional potential of nine underexploited legumes in Southwest Nigeria. Int. Food Res. J. 22, 798–806. Available online at: http://www.ifrj.upm.edu.my/22%20(02)%202015/(48).pdf
Adewale, B., Vroh-Bi, I., Dumet, D., Nnadi, S., Kehinde, O., Ojo, D., et al. (2015). Genetic diversity in African yam bean accessions based on AFLP markers: Towards a platform for germplasm improve- ment and utilization. Plant Genet. Resourc. 13, 111–118. doi: 10.1017/S1479262114000707
Adewale, B. D., and Dumet, D. J. (2010). African yam bean: a crop with food security potentials for Africa. Afr. Technol. Deve. Forum 6, 66–71. Available online at: https://academicjournals.org/journal/AJFS/article-references/358356F65971
Adeyeye, E. I., and Agesin, O. O. (2007). Dehulling the African yam bean (Sphenostylis stenocarpa Hochst. Ex A. Rich) seeds: any nutritional importance? Bangl. J. Sci. Indust. Res. 42, 163–174. doi: 10.3329/bjsir.v42i2.469
Adu-Gyamfi, J. J., Myaka, F. A., Sakala, W. D., Odgaard, R., Vesterager, J. M., and Hogh-Jensen, H. (2007). Biological nitrogen fixation and nitrogen and phosphorus budgets in farmer-managed intercrops of maize–pigeon pea in semi-arid southern and eastern Africa. Plant Soil 295, 127–136. doi: 10.1007/s11104-007-9270-0
Adu-Gyamfi, R., Fearon, J., Bayorbor, T. B., Dzomeku, I. K., and Avornyo, V. K. (2011). The status of Kersting's groundnut [Macrotyloma geocarpum (Harms) Marechal and Baudet]: an underexploited legume in Northern Ghana. Outlook Agric. 40, 259–262. doi: 10.5367/oa.2011.0050
Adzawla, W., Donkoh, S., Al Nyarko, G., O'Reilly, P. J., Olayide, O., Mayes, S., et al. (2016a). Adoption of Bambara groundnut production and its effects on farmers' welfare in North Ghana. African J. Agric. Res. 11, 583–594. doi: 10.5897/AJAR2015.10568
Adzawla, W., Donkoh, S. A., Nyarko, G., O'Reilly, P., and Mayes, S. (2016b). Use patterns and perceptions about the attributes of Bambara groundnut [Vigna subterranea (L.) Verdc.] in Northern Ghana. Ghana J. Sci. Technol. Dev. 4, 56–71. doi: 10.47881/88.967x
Agoyi, E. E., N'danikou, S., Kafoutchoni, M., Ayena, M., Sodedji, F. A., Agbahoungba, S., et al. (2019). Kersting's groundnut [Macrotyloma geocarpum (Harms) Maréchal and Baudet] crop attracts more field pests and diseases than reported before. Agric. Res. Technol. 21, 226–230. doi: 10.19080/artoaj.2019.21.556180
Ahmad, N. S., Redjeki, E. S., Ho, W. K., Aliyu, S., Mayes, K., Massawe, F., et al. (2016). Construction of a genetic linkage map and QTL analysis in Bambara grouudnut [Vigna subterranean (L.) Verdc.]. Genome 59, 459–472. doi: 10.1139/gen-2015-0153
Ahmad, S., Ahsan-ul-Haq, Yousaf, M., Kamran, Z., Ata-ur-Rehman, Sohail, M. U., et al. (2013). Effect of feeding whole linseed as a source of polyunsaturated fatty acids on performance and egg characteristics of laying hens kept at high ambient temperature. Braz. J. Poult. Sci. 15, 21–25. doi: 10.1590/S1516-635X2013000100004
Ajayi, O. B., and Oyetayo, F. L. (2009). Potentials of Kerstingiella geocarpa as a health food. J. Med. Food 12, 184–187. doi: 10.1089/jmf.2008.0100
Ajibola, C. F., Malomo, S. A., Fagbemi, T. N., and Aluko, R. E. (2016). Polypeptide composition and functional properties of African yam bean seed (Sphenostylis stenocarpa) albumin, globulin and protein concentrate. Food Hydrocoll. 56, 189–200. doi: 10.1016/j.foodhyd.2015.12.013
Ajibola, G. O., and Olapade, A. A. (2016). Physical, proximate, and anti-nutritional composition of African yam bean (Sphenostylis stenocarpa) seeds varieties. J. Food Res. 5, 67. doi: 10.5539/jfr.v5n2p67
Akohoue, F., Achigan-Dako, E. G., Sneller, C., van-Deynze, A., and Sibiya, J. (2020). Genetic diversity, SNP-trait associations and genomic selection accuracy in a west African collection of Kersting's groundnut [Macrotyloma geocarpum (Harms) Mare chal & Baudet]. PLoS ONE 15, e0234769. doi: 10.1371/journal.pone.0234769
Aliyu, S., and Massawe, F. J. (2013). Microsatellites based marker molecular analysis of Ghanaian Bambara groundnut [Vigna subterranea (L.) Verdc.] landraces alongside morphological characterization. Genet. Resour. Crop Evol. 60, 777–787. doi: 10.1007/s10722-012-9874-y
Allen, O. N., and Allen, E. K. (1981). The Leguminosae, a Source Book of Characteristics, Uses, and Modulation. London: Macmillan Publishers Ltd., 5–29.
Amadou, H. I., Bebeli, P. J., and Kaltsikes, P. J. (2001). Genetic diversity in bambara groundnut (Vigna subterranea L.) germplasm revealed by RAPD markers. Genome 44, 995–999. doi: 10.1139/gen-44-6-995
Amujoyegbe, B., Opabode, J., and Olayinka, A. (2010). Effect of organic and inorganic fertilizer on yield and chlorophyll content of maize (Zea mays L.) and sorghum (Sorghum bicolour (L.) Moench). Afr. J. Biotechnol. 6, 1869–1873. doi: 10.5897/AJB2007.000-2278
Amujoyegbe, B. J., Obisesan, I. O., Ajayi, A. O., and Aderanti, F. A. (2007). Disappearance of Kersting's groundnut [Macrotyloma geocarpum (Harms) Marechal and Baudet] in south-western Nigeria: an indicator of genetic erosion. Plant Genet. Resour. Newslett. 152, 45–50. Available online at: https://www.bioversityinternational.org/fileadmin/PGR/article-issue_152-art_5-lang_en.html
Amuti, K. (1988). Geocarpa groundnut (Kerstingiella geocarpa). Econ. Bot. 34, 358–361. doi: 10.1007/BF02858310
Anya, M. I., and Ozung, P. O. (2019). Proximate, mineral and anti-nutritional compositions of raw and processed African Yambean (Sphenostylis stenocarpa) seeds in Cross River State, Nigeria. Global J. Agric. Sci. 18, 19. doi: 10.4314/gjass.v18i1.3
Aremu, M. O., Olaofe, O., and Akintayo, T. E. (2006). A comparative study on the chemical and amino acid composition of some Nigerian under-utilized legume flours. Pak. J. Nutr. 5, 34–38. doi: 10.3923/pjn.2006.34.38
Aremu, M. O., Osinfade, B. G., Basu, S. K., and Ablaku, B. E. (2011). Development and nutritional quality evaluation of Kersting's groundnut-ogi for African weaning diet. Am. J. Food Technol. 6, 1021–1033. doi: 10.3923/ajft.2011.1021.1033
Assefa, F., and Kleiner, D. (1997). Nodulation of African yam bean (Sphenostylis stenocarpa) by Bradyrhizobium sp. isolated from Erythrina brucei. Biol. Fertil. Soils 25, 209–210. doi: 10.1007/s003740050305
Assogba, P., Ewedje, E. E. B. K., Dansi, A., Loko, Y. L., Adjatin, A., Dansi, M., et al. (2016). Indigenous knowledge and agro-morphological evaluation of the minor crop Kersting's groundnut (Macrotyloma geocarpum (Harms) Maréchal et Baudet) cultivars of Benin. Genet. Resour. Crop. Evol. 63, 513–529. doi: 10.1007/s10722-015-0268-9
Asuzu, I. U. (1986). Pharmacological evaluation of the folklore use of Sphenostylis stenocarpa. J. Ethnopharm 16, 263–267. doi: 10.1016/0378-8741(86)90092-9
Aviara, N. A., Lawal, A. A., Atiku, A. A., and Haque, M. A. (2013). BG processing, storage and utilization in northeastern Nigeria. Cont. J. Eng. Sci. 8, 28–36. doi: 10.5707/cjengsci.2013.8.1.28.36
Ayenan, M. A. T., and Ezin, V. A. (2016). Potential of Kersting's groundnut [Macrotyloma geocarpum (Harms) Maréchal & Baudet] and prospects for its promotion. Agric. Food Secur. 5, 10. doi: 10.1186/s40066-016-0058-4
Azam-Ali, S. N., Sesay, A., Karikari, S. K., Massawe, F., Aguilar-Manjarrez, J., Bannayan, M., et al. (2001). Assessing the potential of an underutilized crop—a case study using Bambara groundnut. Exp. Agric. 37, 433–472. doi: 10.1017/S0014479701000412
Baiyeri, S., Uguru, M., Ogbonna, P., Samuel-Baiyeri, C. C., Okechukwu, R., Kumaga, F., et al. (2018). Evaluation if the nutritional composition of the seeds of some selected African yam bean (Sphenostylis stenocarpa). Agro Sci. J. Trop. Agric. Food Environ. Ext. 17, 37–44. doi: 10.4314/as.v17i2.5
Bampuori, A. H. (2007). Effect of traditional farming practices on the yield of indigenous kersting's groundnut (macrotyloma geocarpum harms) crop in the upper west region of Ghana. J. Develop. Sustain. Agri. 2, 28–144. doi: 10.11178/jdsa.2.128
Basu, S., Mayes, S., Davey, M., Roberts, J. A., Azam-Ali, S. N., Mithen, R., and Pasquet, R. S. (2007). Inheritance of “domestication” traits in bambara groundnut (Vigna subterranea (L.) Verdc.). Euphytica. 157, 59–68. doi: 10.1007/s10681-007-9396-4
Baudoin, J. P., and Mergeai, G. (2001). “Kersting's groundnut macrotyloma geocarpum (harms) marechal and baudet,” in Crop Production in Tropical Africa, ed H. R. Roman (Brussels), 352–354.
Begemann, F. (1990). “Future research priorities of bambara groundnut improvement in Zambia,” in Proceedings of the First National Workshop on Bambara Groundnut Improvement in Zambia.
Belane, A. K., and Dakora, F. D. (2010). Symbiotic N2 fixation in 30 field-grown cowpea (Vigna unguiculata L. Walp.) genotypes in the Upper West Region of Ghana measured using 15N natural abundance. Biol. Fertil. Soils 46, 1911–1998. doi: 10.1007/s00374-009-0415-6
Bhat, R., and Karim, A. A. (2009). Exploring the nutritional potential of wild and underutilized legumes. Compr. Rev. Food Sci. Food Saf. 8, 305–331. doi: 10.1111/j.1541-4337.2009.00084.x
Boateng, M. A., Addo, J. K., Okyere, H., Berchie, J. N., and Tetteh, A. (2013). Physicochemical and functional properties of proteinates of two bambara groundnut (Vigna subterranea (L) Verdc.) landraces. Afr. J. Food Sci. Technol. 4, 64–70. Available online at: http://csirspace.csirgh.com/bitstream/handle/123456789/1204/physicochemical-and-functional-properties-ofproteinates-of-two-bambara-groundnut-vignasubterranean-landraces.pdf?sequence=1&isAllowed=y
Bouzroud, S., Gasparini, K., Hu, G., Barbosa, M. A. M., Rosa, B. L., Fahr, M., et al. (2020). Down regulation and loss of auxin response factor 4 function using CRISPR/Cas9 alters plant growth, stomatal function and improves tomato tolerance to salinity and osmotic stress. Genes 11, 272. doi: 10.3390/genes11030272
Brink, M., Ramolemana, G. M., and Sibuga, K. P. (2006). “Vigna subterranea (L.) Verdc,” in Plant Resources of Tropical Africa/Ressources vegetales de l'Afrique tropicale, eds M. Brink, and G. Belay (Wageningen: PROTA).
Buah, S., Huudu, A., Tanzubil, P., Ahiabor, B., Yakubu, S., and Abujuan, M. A. (2006). “Kersting's groundnut cultivation manual,” in Savannah Biodiversity Conservation Project Newsletter, ed A. J. Musah. p. 6–7.
Cerrudo, D., Cao, S., Yuan, Y., Martinez, C., Suarez, E. A., Babu, R., et al. (2018). Genomic selection outperforms marker assisted selection for grain yield and physiological traits in a maize doubled haploid population across water treatments. Front. Plant Sci. 9:366. doi: 10.3389/fpls.2018.00366
Chang, Y., Liu, H., Liu, M., Liu, M., Liao, X., Sahu, S. K., et al. (2018a). The draft genome of five agriculturally important African orphan crops. Giga Sci. 8, 1–16. doi: 10.1093/gigascience/giy152
Chang, Y., Liu, H., Liu, M., Liu, M., Liao, X., Sahu, S. K., et al. (2018b). Genomic Data of the Bambara Groundnut (Vigna subterranea). GigaScience Database. doi: 10.5524/101055
Chen, F., Dong, W., Zhang, J., Guo, X., Chen, J., Wang, Z., et al. (2018). The sequenced angiosperm genomes and genome databases. Front. Plant Sci. 9, 418. doi: 10.3389/fpls.2018.00418
Chianu, J. N., Chianu, J. N., and Mairura, F. (2012). Mineral fertilizers in the farming systems of sub-Saharan Africa. A review. Agron. Sustain. Dev. 32, 545–566. doi: 10.1007/s13593-011-0050-0
Chidebe, I. N., Jaiswal, S. K., and Dakora, F. D. (2018). Distribution and phylogeny of microsymbionts associated with cowpea (Vigna unguiculata) nodulation in three agroecological regions of Mozambique. Appl. Environ. Microbiol. 84, e01712–e01717. doi: 10.1128/AEM.01712-17
Chikowo, R., Mapfumo, P., Nyamugafata, P., and Giller, K. E. (2004). Maize productivity and mineral nitrogen dynamics following different soil fertility management practices on a depleted sandy soil in Zimbabwe. Agric. Ecosyst. Environ. 102:119–131. doi: 10.1016/j.agee.2003.08.009
Chinonyerem, A., Obioha, O., and Ebere, U. (2017). Amino acid composition, amino acid scores and predicted protein efficiency ratio of raw and cooked African yam bean (Sphenostylis sternocarpa). J. Appl. Life Sci. Int. 13, 1–10. doi: 10.9734/JALSI/2017/33595
Chivenge, P., Mabhaudhi, T., Modi, A., and Mafongoya, P. (2015). The potential role of neglected and underutilised crop species as future crops under water scarce conditions in Sub-Saharan Africa. Int. J. Environ. Res. Public Health 12, 5685–5711. doi: 10.3390/ijerph120605685
Collino, D. J., Dardanelli, J. L., Sereno, R., and Racca, R. (2000). Physiological responses of argentine peanut varieties to water stress. Water uptake and water use efficiency. Field Crops Res. 68, 133–142. doi: 10.1016/S0378-4290(00)00115-5
Coulibaly, M., Agossou, C. O. A., Akohoué, F., Sawadogo, M., and Achigan-Dako, E. G. (2020). Farmers' preferences for genetic resources of Kersting's groundnut [macrotyloma geocarpum (harms) maréchal and baudet] in the production systems of Burkina Faso and Ghana. Agronomy. 10. doi: 10.3390/agronomy10030371
Dako, A. E. G., and Vodouhè, S. R. (2006). “Macrotyloma geocarpum (Harms) Maréchal & Baudet,” in Record from PROTA4U, eds M. Brink and G. Belay (Wageningen: PROTA), 903–922.
Dalziel, J. M. (1937). “The useful plants of West Tropical Africa,” in The Crown Agents for the Colonies (London), 52–560.
Dawson, I., Guarino, L., and Jaenicke, H. (2007). Underutilised Plant Species: Impacts of Promotion on Biodiversity. Position Paper No. 2. Colombo: International Centre for Underutilised Crops, 23 pp.
do Carmo, C. D., da Silva, M. S., Oliveira, G. A. F., and de Oliveira, E. J. (2015). Molecular-assisted selection for resistance to cassava mosaic disease in Manihot esculenta Crantz. Sci. Agric. 72, 520–527. doi: 10.1590/0103-9016-2014-0348
Duodu, K. G., and Apea-Bah, F. B. (2017). African Legumes: Nutritional and Health-Promoting Attributes. Gluten-free Ancient Grains: Cereals, Pseudocereals, and Legumes: Sustainable, Nutritious, and Health-Promoting Foods for the 21st Century. Amsterdam: Woodhead Publishing, Elsevier, 223–269. doi: 10.1016/B978-0-08-100866-9.00009-1
Edem, D. O., Amugo, C. I., and Eka, O. U. (1990). Chemical composition of yam beans (Sphenostylis stenocarpa). Trop. Sci. 30, 59–63.
Egbe, M. O., Alhassan, G. A., and Ijoyah, M. (2013). Nodulation, nitrogen yield and fixation by Bambara groundnut [Vigna Subterranea (L.) Verdc.] landraces intercropped with cowpea and maize in Southern Guinea savanna of Nigeria. Agri. Sci. 1, 15–28. doi: 10.12735/as.v1i4p15
Egbe, O. M., Idoga, S., and Idoko, J. A. (2007). Preliminary investigation of residual benefits of pigeon pea genotypes intercropped with maize in southern Guinea savanna of Nigeria. J. Sust. Dev. Agric. Environ. 3, 58–75.
Elshire, R. J., Glaubitz, J. C., Sun, Q., Poland, J. A., Kawamoto, K., Buckler, E. S., et al. (2011). A robust, simple genotyping-by-sequencing (GBS) approach for high diversity species. PLoS ONE 6, e19379. doi: 10.1371/journal.pone.0019379
Eric, E., Agoyi, N. S., Medard, K., Mathieu, A., Frejus, A. K. S., Agbahoungba, S., et al. (2019). Kersting's Groundnut [Macrotyloma geocarpum (Harms) Marechal & Baudet] crop attracts more field pests and diseases than reported before. Agri. Res. Tech. J. 21, 556180. doi: 10.19080/ARTOAJ.2019.21.556180
Eriksson, D., Akoroda, M., Azmach, G., Labuschagne, M., Mahungu, N., and Ortiz, R. (2018). Measuring the impact of plant breeding on sub-Saharan African staple crops. Outlook Agric. 47, 163–180. doi: 10.1177/0030727018800723
Eriksson, D., Goldsmith, D., Teitsson, S., Jackson, J., and Van Nooten, F. (2016). Cross-sectional survey in CKD patients across Europe describing the association between quality of life and anaemia. BMC Nephrol. 17, 1–10. doi: 10.1186/s12882-016-0312-9
Eromosele, C. O., Arogundade, L. A., Eromosele, I. C., and Ademuyiwa, O. (2008). Extractability of African yam bean (Sphenostylis stenocarpa) protein in acid, salt and alkaline aqueous media. Food Hydro. 22, 1622–1628. doi: 10.1016/j.foodhyd.2007.11.003
FAO., IFAD., UNICEF., WFP., and WHO. (2020). The State of Food Security and Nutrition in the World. Transforming Food Systems to Deliver Affordable Healthy Diets for All. Rome: FAO.
Fasoyiro, S. B., Ajibade, S. R., Omole, A. J., Adeniyan, O. N., and Farinde, E. O. (2006). Proximate, minerals and antinutritional factors of some underutilized grain legumes in south-western Nigeria. Nutr. Food Sci. 36, 18–23. doi: 10.1108/00346650610642151
Feldman, A., Ho, W. K., Massawe, F., and Mayes, S. (2019). “Bambara groundnut is a climate-resilient crop: how could a drought-tolerant and nutritious legume improve community resilience in the face of climate change?,” in Sustainable Solutions for Food Security (Cham: Springer), 151–167. doi: 10.1007/978-3-319-77878-5_8
Fourie, H., Mc Donald, A. H., Steenkamp, S., and De Waele, D. (2017). “Nematode pests of leguminous and oilseed crops,” in Nematology in South Africa: A View From the 21st Century, eds H. Fourie, V. W. Spaull, R. K. Jones, M. S. Daneel, and D. De Waele (Cham: Springer), 201–230. doi: 10.1007/978-3-319-44210-5_9
Gao, X., Bamba, A. S. A., Kundy, A. C., Mateva, K. I., Chai, H. H., Ho, W. K., et al. (2020). Variation of phenotypic traits in twelve bambara groundnut [Vigna subterranea (L.) Verdc.] genotypes and two F2 bi-parental segregating populations. Agronomy 10, 1451. doi: 10.3390/agronomy10101451
Giller, K. E. (2020). The food security conundrum of sub-Saharan Africa. Glob. Food Sec. 26, 100431. doi: 10.1016/j.gfs.2020.100431
Girvetz, E., Ramirez-Villegas, J., Claessens, L., Lamanna, C., Navarro-Racines, C., Nowak, A., et al. (2019). “Future climate projections in africa: where are we headed?” in The Climate-Smart Agriculture Papers, eds T. S. Rosenstock, A. Nowak, E. Girvetz (Cham: Springer), pp. 15–27. doi: 10.1007/978-3-319-92798-5_2
Goli, A. E. (1997). “Bambara groundnut [Vigna subterranea (L) Verdc.],” in Promoting the Conservation and Use of Underutilized and Neglected Crops, Vol 9, eds J. Heller, F. Begemann, J. Mushonda (Rome: International Plant Genetic Resources Institute), 167.
Gunjal, K., Goodbody, S., Delbaere, J., Kenefick, E., and Rammala, V. (2009). FAO/WFP Crop and Food Security Assessment Mission to Zimbabwe. Special Report 22. Available online at: http://www.fao.org/3/ak352e/ak352e00.htm (accessed May 11, 2021).
Hampson, S. E., Skinner, T. C., Hart, J., Storey, L., Gage, H., Foxcroft, D., et al. (2000). Behavioral interventions for adolescents with type 1 diabetes: How effective are they? Diab. Care. 23, 1416–1422. doi: 10.2337/diacare.23.9.1416
Harlan, J. R. (1971). Agricultural origins: centers and non-centers. Science 174, 468–474. doi: 10.1126/science.174.4008.468
Harms, H. (1908). Über geokarpie bei einer afrikanischen leguminosae. Bericht. Deutsch. Bot. Gesellschaft. 26, 225–231.
Heller, J., Begemann, F., and Mushonga, J. (1997). Bambara groundnut. Vigna subterranea (L.) Verdc. Promoting the conservation and use of underutilised and neglected crops. 9. Proceedings of the Workshop on Conservation and Improvement of Bambara Groundnut (Vigna subterranea (L.) Verdc.), 14–16 November 1995, Harare, Zimbabwe. Institute of Plant Genetics and Crop Plant Research, Gatersleben/Department of Research & Specialist Services, Harare/International Plant Genetic Resources Institute, Rome, Italy.
Hema, M., Sreenivasulu, P., Patil, B. L., Kumar, P. L., and Reddy, D. V. (2014). Tropical food legumes: virus diseases of economic importance and their control. Adv. Virus Res. 90, 431–505. doi: 10.1016/B978-0-12-801246-8.00009-3
Hendre, P. S., Muthemba, S., Kariba, R., Muchugi, A., Fu, Y., Chang, Y., et al. (2019). African orphan crops consortium (AOCC): status of developing genomic resources for African orphan crops. Planta 250, 989–1003. doi: 10.1007/s00425-019-03156-9
Henry, N. B., and Roger, F. (2012). Social risks and challenges in post-disaster resettlement: the case of Lake Nyos, Cameroon. J. Risk Res. 15, 1141–1157. doi: 10.1080/13669877.2012.705315
Hillocks, R. J., Bennett, C., and Mponda, O. M. (2012). Bambara nut: a review of utilisation, market potential and crop improvement. Afr. Crop Sci. J. 20, 1–16. Available online at: https://www.ajol.info/index.php/acsj/article/view/78601
Hiremath, P. J., Kumar, A., Penmetsa, R. V., Farmer, A., Schlueter, J. A., Chamarthi, S. K., et al. (2012). Large-scale development of cost-effective SNP marker assays for diversity assessment and genetic mapping in chickpea and comparative mapping in legumes. Plant Biotechnol. J. 10, 716–732. doi: 10.1111/j.1467-7652.2012.00710.x
Ho, W. K., Chai, H. H., Kendabie, P., Ahmad, N. S., Jani, J., Massawe, F., et al. (2017). Integrating genetic maps in Bambara groundnut [Vigna subterranea (L.) Verdc.] and their syntenic relationships among closely related legumes. BMC Genomics 1, 192. doi: 10.1186/s12864-016-3393-8
Huang, B. E., Verbyla, K. L., Verbyla, A. P., Raghavan, C., Singh, V. K., Gaur, P., et al. (2015). MAGIC populations in crops: current status and future prospects. Theor. Appl. Genet. 128, 999–1017. doi: 10.1007/s00122-015-2506-0
IPCC (2012). 2012 summary for policymakers. Managing the Risks of Extreme Events and Disasters to Advance Climate Change Adaptation, A Special Report of Working Groups I and II of the Intergovernmental Panel on Climate Change, 2012, eds C. B. Field, V. Barros, T. F. Stocker (Cambridge: Cambridge University Press).
Jaganathan, D., Thudi, M., Kale, S., Azam, S., Roorkiwal, M., Gaur, P. M., et al. (2015). Genotyping-by-sequencing based intra-specific genetic map refines a “QTL-hotspot” region for drought tolerance in chickpea. Mol. Genet. Genomics 290, 559–571. doi: 10.1007/s00438-014-0932-3
Ji, J., Zhang, C., Sun, Z., Wang, L., Duanmu, D., and Fan, Q. (2019). Genome editing in cowpea Vigna unguiculata using CRISPR-Cas9. Int. J. Mol. Sci. 20, 2471. doi: 10.3390/ijms20102471
Kafoutchoni, K. M., Agoyi, E. E., Agbahoungba, S., Assogbadjo, A. E., and Agbangla, C. (2021). Genetic diversity and population structure in a regional collection of Kersting's groundnut (Macrotyloma geocarpum (Harms) Maréchal & Baudet). Genet. Resour. Crop Evol. 68, 3285–3300. doi: 10.1007/s10722-021-01187-4
Karikari, S. K., Wigglesworth, D. J., Kwerepe, B. C., Balole, T. V., Sebolai, B., and Munthali, D. C. (1997). “Country Reports:Botswana,” in Bambara groundnut (Vigna subterranea (L.) Verdc.). Promoting the conservation and use of underutilized and neglected crops. 9. Proceedings of the workshop on Conservation and Improvement of Bambara Groundnut (Vigna subterranea (L.) Verdc.), 14–16 November 1995, Harare, Zimbabwe, eds J. Heller, F. Begemann and J. Mushonga (Rome: Institute of Plant Genetics and Crop Plant Research, Gatersleben/Department of Research & Specialist Services, Harare/International Plant Genetic Resources Institute), 11–19.
Katayama, K., Ito, O., Matsunanga, R., Adu-Gyamfi, J. J., Rao, T. R., Anders, M. M., et al. (1995). Nitrogen balance and root behavior in four pigeon pea based intercropping systems. Fert. Res. 42, 315–319. doi: 10.1007/978-94-009-1706-4_29
Kay, D. E. (1973). Root Crops. London: Tropical Products Institute Murdock, G. P. 1959. Africa: Its Peoples and Their Culture History. New York, NY: McGraw-Hill.
Kermah, M., Franke, A., Adjei-Nsiah, S., Ahiabor, B., Abaidoo, R. C., and Giller, K. (2017). N2-fixation and N contribution by grain legumes under different soil fertility status and cropping systems in the Guinea savanna of northern Ghana. Agric. Ecosyst. Environ. 261, 201–210. doi: 10.1016/j.agee.2017.08.028
Klu, G. Y., Bansa, D., Kumaga, F. K., Aboagye, L. M., Benett-Lartey, S. O., and Gamedoagbao, D. K. (2000). The African yam bean (Sphenostylis stenocarpa): a neglected crop in Ghana. West Afr. J. Appl. Ecol. 1, 53–60. doi: 10.4314/wajae.v1i1.40570
Kosini, D., and Nukenine, E. N. (2017). Bioactivity of novel botanical insecticide from Gnidia kaussiana (Thymeleaceae) against Callosobruchus maculatus (Coleoptera: Chrysomelidae) in stored Vigna subterranea (Fabaceae) grains. J. Insect Sci. 17, 31–37. doi: 10.1093/jisesa/iex004
Lemmon, Z. H., Reem, N. T., Dalrymple, J., Soyk, S., Swartwood, K. E., Rodriguez-Leal, D., et al. (2018). Rapid improvement of domestication traits in an orphan crop by genome editing. Nat. Plants 4, 766–770. doi: 10.1038/s41477-018-0259-x
Leport, L., Turner, N. C., French, R. J., Barr, M. D., Duda, R., Davies, S. L., et al. (1999). Physiological responses of chickpea genotypes to terminal drought in a Mediterranean- type environment. Eur. J. Agron. 11, 279–291. doi: 10.1016/S1161-0301(99)00039-8
Li, M., Chen, R., Jiang, Q., Sun, X., Zhang, H., and Hu, Z. (2021). GmNAC06, a NAC domain transcription factor enhances salt stress tolerance in soybean. Plant Mol. Biol. 105, 333–345. doi: 10.1007/s11103-020-01091-y
Liao, X., Yang, L., and Huan, L. (2019). The complete plastid genomes of two Fabaceae orphan crops from Africa. Mitochond. DNA Part B 4, 93–94. doi: 10.1080/23802359.2018.1536481
Linnemann, A. R., and Azam-Ali, S. (1993). “Bambara groundnut (Vigna subterranea),” in Pulses and vegetables, ed J. T. William (Chapman and Hall), 13–57.
Liu, L., Gallagher, J., Arevalo, E. D., Chen, R., Skopelitis, T., Wu, Q., et al. (2021). Enhancing grain-yield-related traits by CRISPR–Cas9 promoter editing of maize CLE genes. Nat. Plants 7, 287–294. doi: 10.1038/s41477-021-00858-5
Makhotenko, A., Khromov, A., Snigir, E., Makarova, S., Makarov, V., Suprunova, T., et al. (2019). “Functional analysis of coilin in virus resistance and stress tolerance of potato Solanum tuberosum using CRISPR-Cas9 editing,” in Doklady Biochemistry and Biophysics, ed A. G. Gabibov (Cham: Springer), 88–91. doi: 10.1134/S1607672919010241
Mapope, N., and Dakora, F. D. (2016). N2 fixation, carbon accumulation, and plant water relations in soybean (Glycine max L. Merrill) varieties sampled from farmers' fields in South Africa, measured using15N and 13C natural abundance. Agric. Ecosyst. Environ. 221, 174–186. doi: 10.1016/j.agee.2016.01.023
Masih, I., Maskey, S., Mussa, F. E. F., and Trambauer, P. (2014). A review of droughts on the African continent: a geospatial and long-term perspective. Hydrol Earth Syst Sci. 18, 3635–3649. doi: 10.5194/hess-18-3635-2014
Massawe, F., Dickinson, M., Roberts, J., and Azam-Ali, S. (2003b). Genetic diversity in bambara groundnut [Vigna subterranea (L.) Verdc] landraces revealed by AFLP markers. Genome 45, 1175–1180. doi: 10.1139/g02-093
Massawe, F., Roberts, J., Azam-Ali, S., and Davey, M. R. (2003a). Genetic diversity in bambara groundnut [Vigna subterranea (L.) Verdc] landraces assessed by random amplified polymorphic DNA (RAPD) markers. Genet. Resour. Crop Evol. 50, 737–741. doi: 10.1023/A:1025041301787
Massawe, F. J., Mwale, S. S., Azam-Ali, S. N., and Roberts, J. A. (2005). Breeding in bambara groundnut (Vigna subterranea (L.) Verdc.): Strategic considerations. Afr. J. Biotechnol. 4, 463–471. doi: 10.5897/AJB2005.000-3084
Mayes, S., Basu, S. M., Molosiwa, O., Redjeki, E. S., Ahmad, N. S., Khan, F., et al. (2013). Molecular analysis of Bambara groundnut, an underutilised African legume crop as part of the BAMLINK project—what lessons can we learn. Acta Hortic. 979, 451–458. doi: 10.17660/ActaHortic.2013.979.48
Mayes, S., Ho, W. K., Chai, H. H., Gao, X., Kundy, A. C., Mateva, K. I., et al. (2019). Bambara groundnut: an exemplar underutilised legume for resilience under climate change. Planta. 250, 803–820. doi: 10.1007/s00425-019-03191-6
Mbanjo, E. G. N., Rabbi, I. Y., Ferguson, M. E., Kayondo, S. I., Eng, N. H., Tripathi, L., et al. (2021). Technological Innovations for improving cassava production in Sub-Saharan Africa. Front. Genet. 11, 623736. doi: 10.3389/fgene.2020.623736
Meng, L., Li, H., Zhang, L., and Wang, J. (2015). QTL IciMapping: integrated software for genetic linkage map construction and quantitative trait locus mapping in biparental populations. Crop J. 3, 269–283. doi: 10.1016/j.cj.2015.01.001
Mergeai, G. (1993). Influence des facteurs sociologiques sur la conservation des ressources phytogenetiques. Le cas de la lentille de terre [Macrotyloma geocarpum (Harms) Marechal et Baudet] au Togo. Bull. Rech Agron Gembloux 28, 487–500.
Minnaar-Ontong, A., Gerrano, A. S., and Labuschagne, M. T. (2021). Assessment of genetic diversity and structure of bambara groundnut [Vigna subterranea (L.) verdc.] landraces in South Africa. Sci. Rep. 11, 7408. doi: 10.1038/s41598-021-86977-7
Mohale, K. C., Belane, A. K., and Dakora, F. D. (2014). Symbiotic N nutrition, C assimilation, and plant water use efficiency in Bambara groundnut [Vigna subterranea L. Verdc] grown in farmers' fields in South Africa, measured using 15 N and 13 C natural abundance. Biol. Fertil. Soils 50, 307–319. doi: 10.1007/s00374-013-0841-3
Mohammed, M., Sanjay, K. J., Elias, N. K., Sowley, B. D., and Felix, D. D. (2018). Symbiotic N2 fixation and grain yield of endangered Kersting's groundnut landraces in response to soil and plant associated bradyrhizobium inoculation to promote ecological resource-use efficiency. Front. Microbiol. 9, 2105. doi: 10.3389/fmicb.2018.02105
Molosiwa, O. O., Aliyu, S., Stadler, F., Mayes, K., Massawe, F., Kilian, A., et al. (2015). SSR marker development, genetic diversity and population structure analysis of bambara groundnut [Vigna subterranea (L.) Verdc.] landraces. Genet. Resour. Crop Evol. 62, 1225–1243. doi: 10.1007/s10722-015-0226-6
Moyib, O. K., Gbadegesin, M. A., Aina, O. O., and Odunola, A. O. (2008). Genetic variation within a collection of Nigerian accessions of African yam bean (Sphenostylis stenocarpa) revealed by RAPD primers. Afr. J. Biotechnol. 7, 1839–1846. doi: 10.5897/AJB08.117
Mubaiwa, J., Fogliano, V., Chidewe, C., Bakker, E. J., and Linnemann, A. R. (2018). Utilization of bambara groundnut [Vigna subterranea (L.) Verdc.] for sustainable food and nutrition security in semi-arid regions of Zimbabwe. PLoS ONE 13, e0204817. doi: 10.1371/journal.pone.0204817
Mugabe, J. (1994). Research on biofertilizers: Kenya, Zimbabwe and Tanzania. Biotechnol. Dev. Mon. 18, 9–10.
Muimba-Kankolongo, A. (2018). “Chapter 10, leguminous crops,” in Food Crop Production by Smallholder Farmers in Southern Africa, ed. A. Muimba-Kankolongo (Academic Press), 173–203. doi: 10.1016/B978-0-12-814383-4.00010-4
Murevanhema, Y. Y., and Jideani, V. A. (2013). Potential of bambara groundnut [Vigna subterranea (L.) Verdc] milk as a probiotic beverage-areview. Crit. Rev. Food Sci. Nutr. 53, 954–967. doi: 10.1080/10408398.2011.574803
Musa, M., Massawe, F., Sean, M., Ibraheem, A., and Singh, A. (2016). Nitrogen fixation and N-balance studies on bambara groundnut [Vigna subterranea L. Verdc] landraces grown on tropical acidic soils of Malaysia. Commun. Soil Sci. Plant Anal. 47, 533–542. doi: 10.1080/00103624.2016.1141928
Mwale, S. S., Azam-Ali, S. N., and Massawe, F. J. (2007). Growth and development of BG (Vigna subterranea) in response to soil moisture. 2. Resource capture and conversion. Eur. J. Agron. 26, 354–362. doi: 10.1016/j.eja.2006.12.008
Ncube, B., Twomlow, S. T., van Wijk, M. T., Dimes, J. P., and Giller, K. E. (2007). Productivity and residual benefits of grain legumes to sorghum under semi-arid conditions in southwestern Zimbabwe. Plant Soil 299, 1–15. doi: 10.1007/s11104-007-9330-5
Ndidi, U. S., Ndidi, C. U., Olagunju, A., Muhammad, A., Billy, F. G., and Okpe, O. (2014). Proximate, antinutrients and mineral composition of raw and processed (boiled and roasted) Sphenostylis stenocarpa seeds from Southern Kaduna, Northwest Nigeria. ISRN Nutr. 2014, 280837. doi: 10.1155/2014/280837
Nnamani, C. V., Afiukwa, C. A., Oselebe, H. O., Igwe, D. O., Uhuo, C. A., Idika, K. O., et al. (2019). Genetic diversity of some African yam bean accessions in ebonyi state assessed using intersimple sequence repeat (ISSR) markers. J. Underutil. Legumes 1, 20–33.
NRC. (2007). Lost Crops of Africa: Vegetables and Yam Bean Development, Security and Cooperation Policy and Global; Affairs (DSC). Washington, DC: NRC.
Ntundu, W. H., Bach, I. C., Christiansen, J. L., and Andersen, S. B. (2004). Analysis of genetic diversity in bambara groundnut [Vigna subterranea (L.) Verdc.] landraces using amplified fragment length polymorphism (AFLP) markers. Afr. J. Biotechnol. 3, 220–225.
Nyau, V., Prakash, S., Rodrigues, J., and Farrant, J. (2015). Antioxidant activities of bambara groundnuts as assessed by FRAP and DPPH assays. Am. J. Food Nutr. 3, 7–11. doi: 10.12691/ajfn-3-1-2
Oboh, G. (2006). Antioxidant properties of some commonly consumed and underutilized tropical legumes. Eur. Food Res. Technol. 224, 61–65. doi: 10.1007/s00217-006-0289-x
Oboh, G., Ademiluyi, A. O., and Akindahunsi, A. A. (2009). Changes in polyphenols distribution and antioxidant activity during fermentation of some underutilized legumes. Food Sci. Technol. Int. 15, 41–46. doi: 10.1177/1082013208101022
Odongo, F. O., Oyoo, M. E., Wasike, V., Owuoche, J. O., Karanja, L., and Korir, P. (2015). Genetic diversity of Bambara groundnut [Vigna subterranea (L.) verdc.] landraces in Kenya using microsatellite markers. Afr. J. Biotechnol. 14, 283–291. doi: 10.5897/AJB2014.14082
Oganale, D. (2009). “Nodulation and nitrogen fixation of African yam beam (Sphenostylis stenocarpa),” in Proceedings of African Crop Science Society Conference (Cape Town: Science and Technology Supporting Food Security in Africa.
Ojiem, J. O., Vanlauwe, B., de Ridder, N., and Giller, K. E. (2007). Niche-based assessment of contributions of legumes to the nitrogen economy of Western Kenya smallholder farms. Plant Soil 292, 119–135. doi: 10.1007/s11104-007-9207-7
Ojuederie, O. B., Morufat, O. B., Iyiola, F., David, O. I., and Mercy, O. O. (2014). Assessment of the genetic diversity of African yam bean [Sphenostylis stenocarpa Hochst ex. A Rich. Hams] accessions using amplified fragment length polymorphism (AFLP) markers. Afr. J. Biotechnol. 18, 1850–1858. doi: 10.5897/AJB2014.13734
Okigbo, B. N. (1973). “Introducing the yam bean (Sphenostylis stenocarpa Hochst ex. A. Rich. Harms),” in Proceedings of the First IITA Grain Legume Improvement Workshop (Ibadan), 224–238.
Okpara, D. A., and Omaliko, C. P. E. (1995). Effects of staking, nitrogen and phosphorus fertilizer rates on yield and yield components of African yam bean (Sphenostylis stenocarpa). Ghana J. Agric. Sci. 28, 23–28. doi: 10.4314/gjas.v28i1.2004
Olaleye, A., Adeyeye, E., and Adesina, A. (2013). Chemical composition of bambara groundnut (V. subterranea L. Verdc) seed parts. Bangladesh J. Sci. Indust. Res. 48, 167–178. doi: 10.3329/bjsir.v48i3.17325
Olayide, O. E., Donkoh, S. A., Ansah, G. K., Adzawla, W., O'Reilly, P. J., Mayes, S., et al. (2018). “Assessing the socioeconomic factors influencing bambara groundnut as an indigenous climate resilient crop in Nigeria,” in Handbook of Climate Change Resilience, ed W. L. Filho WL (Basel: Springer Nature). doi: 10.1007/978-3-319-71025-9_158-1
Ologou, S. (2015). Analyse des Systemes de Production de la Lentille de Terre (Macrotyloma geocarpum. Harms.) dans la Commune de Djidja. Abomey-Calavi: Universite d'Abomey-Calavi.
Olukolu, B. A., Mayes, S., Stadler, F., Ng, N. Q., Fawole, I., Dominique, D., et al. (2012). Genetic diversity in bambara groundnut [Vigna sub-terranea (L) Verdc] as revealed by phenotypic descriptors and DArTmarker analysis. Genet. Resour. Crop Evol. 59, 347–358. doi: 10.1007/s10722-011-9686-5
Oluwole, O. O., Olomitutu, O. E., Paliwal, R., Oyatomi, O. A., Abberton, M. T., and Obembe, O. O. (2020). Preliminary assessment of the association between DArT-SEQ SNP and some nutritional traits in African yam bean. Trop. J. Nat. Prod. Res. 4, 877–879. doi: 10.26538/tjnpr/v4i11.5
Onimawo, I., Momoh, A., and Usman, A. (1998). Proximate composition and functional properties of four cultivars of bambara groundnut (Voandezeia subterranea). Plant Foods Hum. Nutr. 53, 153–158. doi: 10.1023/A:1008027030095
Oshodi, A. A., Ipinmoroti, K. O., and Adeyeye, E. I. (1997). Functional properties of some varieties of African yam bean (Sphenostylis stenocarpa) flour. Int. J. Food Sci. Nutr. 48, 243–250. doi: 10.3109/09637489709028568
Oyetayo, F. L., and Ajayi, B. O. (2005). Chemical profile and zinc bioavailability on “Hausa groundnut” (Kerstingiella geocarpa). Biosci. Biotechnol. Res. Asia 3, 47–50. Available online at: http://www.biotech-asia.org/vol3no1/chemical-profile-and-zinc-bioavailability-studies-on-hausa-groundnut-kerstingiella-geocarpa/
Paliwal, R., Abberton, M., Faloye, B., and Olaniyi, O. (2020). Developing the role of legumes in West Africa under climate change. Curr. Opin. Plant Biol. 56, 242–258. doi: 10.1016/j.pbi.2020.05.002
Paliwal, R., Oyatomi, O., Faloye, B., and Abberton, M. (2019). “Unlocking the genetic diversity of underutilized IITA legumes,” in Society for Underutilized Legumes, 3rd Annual Conference (Ibadan: IAR&T).
Paliwal, R., Röder, M. S., Kumar, U., Srivastava, J. P., and Joshi, A. K. (2012). QTL mapping of terminal heat tolerance in hexaploid wheat (T. aestivum L.). Theor. Appl. Genet. 125, 561–575. doi: 10.1007/s00122-012-1853-3
Paliwal, R., Taofeek, T. A., Abberton, M., Faloye, B., and Olaniyi, O. (2021). Potential of genomics for the improvement of underutilized legumes in sub-Saharan Africa. Legume Sci. 3, 1–16. doi: 10.1002/leg3.69
Pasquet, R., and Vigna, S. (2001). Royal Botanic Gardens, eds B. Mackinder, P. R. Polhill, and B. Verdcourt (London: Flora Zambesiaca), 121–156.
Pasquet, R. S., Mergeai, G., and Baudoin, J. (2002). Genetic diversity of the African geocarpic legume kersting's groundnut, Macrotyloma geocarpum (Tribe Phaseoleae: Fabaceae). Biochem. Syst. Ecol. 30, 943–952. doi: 10.1016/S0305-1978(02)00040-6
Pasquet, R. S., Schwedes, S., and Gepts, P. (1999). Isozyme diversity in bambara groundnut. Crop Sci. 39, 1228–1236. doi: 10.2135/cropsci1999.0011183X003900040045x
Pittari, A. M., Becherucci, P., La Cauza, F., and Seminara, S. (1992). Therapy with arginine chlorohydrate in children with short constitutional stature. Min. Pediatr. 45, 61–65.
Poland, J. A., and Rife, T. W. (2012). Genotyping-by-sequencing for plant breeding and genetics. Plant Genome 5, 92–102. doi: 10.3835/plantgenome2012.05.0005
Potter, D. (1992). Economic botany of Sphenostylis (Leguminosae). Econ. Bot. 46, 262–275. doi: 10.1007/BF02866625
Potter, D., and Doyle, J. J. (1992). Origin of African yam bean (Sphenostylis stenocarpa, Leguminosae): evidence from morphology, isozymes, chloroplast DNA and Linguistics. Econ. Bot. 46, 276–292. doi: 10.1007/BF02866626
Prasanna, B. M., Cairns, J. E., Zaidi, P. H., Beyene, Y., Makumbi, D., Gowda, M., et al. (2021). Beat the stress: breeding for climate resilience in maize for the tropical rainfed environments. Theor. Appl. Genet. 1, 3. doi: 10.1007/s00122-021-03773-7
Purseglove, J. W. (1976). “The origins and migrations of crops in Tropical Africa,” in Origins of African Plant Domestication, ed. Harlan, 291–309.
Ramirez-Villegas, J., Koehler, A. K., and Challinor, A. J. (2017). Assessing uncertainty and complexity in regional-scale crop model simulations. Eur. J. Agronom. 88, 84–95. doi: 10.1016/j.eja.2015.11.021
Ramirez-Villegas, J., and Thornton, P. K. (2015). “Climate change impacts on African crop production,” in CCAFS Working Paper no. 119. CGIARresearch program on Climate Change, Agriculture and FoodSecurity (CCAFS). Copenhagen. Available online at: www.ccafs.cgiar.org
Rebafka, F. P., Ndunguru, B. J., and Marschner, H. (1993). Crop residue application increases nitrogen fixation and dry matter production in groundnut (Arachis hypogaea L.) grown on an acid sandy soil in Niger, West Africa. Plant Soil 150, 213–222. doi: 10.1007/BF00013018
Redjeki, E. S., Ho, W. K., Shah, N., Molosiwa, O. O., Ardiarini, N. R., and Kuswanto, M. S. (2020). Understanding the genetic relationships between Indonesian bambara groundnut landraces and investigating their origins. Genome 63, 319–327. doi: 10.1139/gen-2019-0137
Ringler, C., Zhu, T., Cai, X., Koo, J., and Wang, D. (2010). Climate change impacts on food security in sub-saharan africa insights from comprehensive climate change scenarios. Food Policy 28, 466. Available online at: https://www.semanticscholar.org/paper/Climate-change-impacts-on-food-security-in-Africa%3A-Ringler-Zhu/312dcf69d132f8511e4d1ceeb7e505445e41d8bd
Rippke, U., Ramirez-Villegas, J., Jarvis, A., Vermeulen, S. J., Parker, L., Mer, F., et al. (2016). Timescales of transformational climate change adaptation in sub-Saharan African agriculture. Nat. Clim. Chang. 6, 605–609. doi: 10.1038/nclimate2947
Roudier, P., Sultan, B., Quirion, P., and Berg, A. (2011). The impact of future climate change on West African crop yields: what does therecent literature say? Glob. Environ. Change 21, 1073–1083 doi: 10.1016/j.gloenvcha.2011.04.007
Rungnoi, O., Suwanprasert, J., Somta, P., and Srinives, P. (2012). Molecular genetic diversity of bambara groundnut (Vigna subterranea L. Verdc.) revealed by RAPD and ISSR marker analysis. Sabrao J. Breed. Genet. 44, 87–101.
Saghai-Maroof, M. A., Jeong, S. C., Gunduz, I., Tucker, D. M., Buss, G. R., and Tolm, S. A. (2008). Pyramiding of soybean mosaic virus resistance genes by marker assisted selection. Crop Sci. 48, 517–526. doi: 10.2135/cropsci2007.08.0479
Saka, J. O., Ajibade, S. R., Adeniyan, O. N., Olowoyo, R. B., and Ogunbodede, B. A. (2004). Survey of underutilized grain Legume production systems in the Southwest agricultural zone of Nigeria. J. Agric. Food Inform. 6, 93–107. doi: 10.1300/J108v06n02_08
Sanchez, P. A. (2002). Soil fertility and hunger in Africa. Science 295, 2019–2020. doi: 10.1126/science.1065256
Sanginga, N., Dashiell, K., and Okugan, J. A. (1997). Nitrogen fixation and N contribution by promiscuous nodulating soybeans in southern guinea savanna of Nigeria. Plant Soil 195, 257–266. doi: 10.1023/A:1004207530131
Sarvamangala, C., Gowda, C. L. L., and Varshney, R. K. (2011). Identification of quantitative trait loci for protein content, oil content and oil quality for groundnut (Arachis hypogaea L.). Field Crops Res. 122, 49–59. doi: 10.1016/j.fcr.2011.02.010
Saxena, R. K., Penmetsa, R. V., Upadhyaya, H. D., Kumar, A., Carrasquilla-Garcia, N., Schlueter, J. A., et al. (2012). Large-scale development of cost-effective single-nucleotide polymorphism marker assays for genetic mapping in pigeonpea and comparative mapping in legumes. DNA Res. 19, 449–461. doi: 10.1093/dnares/dss025
Sesay, A., Kunene, I. S., and Earnshaw, D. M. (1999). Farmers' knowledge and cultivation of bambara groundnut (Vigna subterranea (L.) Verdc.) in Swaziland. Uniswa Res. Agric. Sci. Tech. 3, 27–37.
Sesay, A., Mpuisang, T., Morake, T. S., Al-Shareef, I., Chepete, H. J., and Moseki, B. (2010). Preliminary assessment of bambara groundnut (Vigna subterranea L.) landraces for temperature and water stress tolerance under field conditions in Botswana. South African J. Plant Soil 27, 312–321. doi: 10.1080/02571862.2010.10640000
Shitta, N. S., Abberton, M. T., Adesoye, A. I., and Adewale, D. B. (2016). Analysis of genetic diversity of African yam bean using SSR markers derived from cowpea. Plant Genet. Resourc. 14, 50–56. doi: 10.1017/S1479262115000064
Sidibe, A., Meldrum, G., Coulibaly, H., Padulosi, S., Traore, I., Diawara, G., et al. (2020). Revitalizing cultivation and strengthening the seed systems of fonio and Bambara groundnut in mali through a community biodiversity management approach. Plant Genet. Resourc. 18, 31–48. doi: 10.1017/S1479262120000076
Soetan, K. O., Olaiya, C. O., and Karigidi, K. O. (2018). Comparative in vitro antioxidant activities of six accessions of African yam beans (Sphenostylis stenocarpa L.). Ann. Food Sci. Technol. 19. Available online at: https://journals.indexcopernicus.com/api/file/viewByFileId/618209.pdf
Somta, P., Chankaew, S., Rungnoi, O., and Srinives, P. (2011). Genetic diversity of the bambara groundnut [Vigna subterranea (L.) Verdc.] as assessed by SSR markers. Genome 54, 898–910. doi: 10.1139/g11-056
Suwanprasert, J., Toojinda, T., Srinives, P., and Chanprame, S. (2006). Hybridization technique for Bambara groundnut. Breed. Sci. 2, 125–129. doi: 10.1270/jsbbs.56.125
Tadele, Z. (2018). African orphan crops under abiotic stresses: challenges and opportunities. Science 2018, 1–19. doi: 10.1155/2018/1451894
Tamiru, A., Paliwal, R., Manthi, S. J., Odeny, D. A., Midega, C. A. O., Khan, Z. R., et al. (2020). Genome wide association analysis of a stemborer egg induced “call-for-help” defence trait in maize. Sci. Rep. 10, 11205. doi: 10.1038/s41598-020-68075-2
Tanimu, B. S. A., and Aliyu, L. (1990). “Genotypic variability in Bambara groundnut cultivars at Samaru,” in Proceedings of the 17th Annual Conference of the Genetic Society of Nigeria (Nigeria: Genetics Society of Nigeria), 54–56.
Tao, Y. F., Zhao, X., Mace, E., Henry, R., and Jordan, D. (2019). Exploring and exploiting pan-genomics for crop improvement. Mol. Plant 12, 156–169. doi: 10.1016/j.molp.2018.12.016
Temegne, N. C., Dooh, J. P. N., Nbendah, P., Ntsomboh-Ntsefong, G., Taffouo, V. D., and Youmbi, E. (2020). Cultivation and utilization of Bambara groundnut [Vigna subterranea (L.) verdc.], a neglected plant in Cameroon. Asian Plant Res. J. 4, 9–21. doi: 10.9734/aprj/2020/v4i230081
Toyosi, T. G., Anthony, O. O., and Samson, A. O. (2020). The prospects of African yam bean: past and future importance. Heliyon 6, 11. doi: 10.1016/j.heliyon.2020.e05458
Uchegbu, N. N. (2015). Antioxidant activity of germinated african yam bean (sphenostylis stenocarpa) in alloxan diabetic rats. world academy of science, engineering and technology. Int. J. Biol. Biomol. Agri. Food. Biotechnol. Eng. 9, 239–243.
Udeh, E. L., Nyila, M. A., and Kanu, S. A. (2020). Nutraceutical and antimicrobial potentials of bambara groundnut (Vigna subterranean): a review. Heliyon 6, e05205. doi: 10.1016/j.heliyon.2020.e05205
Usoroh, J., Eneobong, E., Abraham, S., and Umoyen, A. (2019). Evaluation of the genetic diversity of African yam bean [Sphenostylis stenocarpa (Hoechst. ex. A Rich.) Harms]. Using Seed Protein Marker. Arch. Curr. Res. Int. 17, 1–10. doi: 10.9734/acri/2019/v17i430117
van Dijk, M., Morley, T., van Loon, M., Reidsma, P., Tesfaye, K., and van Ittersum, M. K. (2020). Reducing the maize yield gap in Ethiopia: decomposition and policy simulation. Agric. Syst. 183, 102828. doi: 10.1016/j.agsy.2020.102828
Van-Vught, A. J., Dagnelie, P. C., Arts, I. C., Froberg, K., Andersen, L. B., El-Naaman, B., et al. (2013). Heitman BL. Dietary arginine and linear growth: the Copenhagen School Child Intervention Study. Br. J. Nutr. 109, 1031–1039. doi: 10.1017/S0007114512002942
Varshney, R. K., Hiremath, P. J., Lekha, P., Kashiwagi, J., Balaji, J., Deokar, A. A., et al. (2009). A comprehensive resource of drought- and salinity- responsive ESTs for gene discovery and marker development in chickpea (Cicer arietinum L.). BMC Genomics 10:523. doi: 10.1186/1471-2164-10-523
Varshney, R. K., Kudapa, H., Pazhamala, L., Chitikineni, A., Thudi, M., Bohra, A., et al. (2015). Translational genomics in agriculture: some examples in grain legumes. Crit. Rev. Plant Sci. 34, 169–194. doi: 10.1080/07352689.2014.897909
Varshney, R. K., Singh, V. K., Kumar, A., Powell, W., and Sorrells, M. E. (2018). Can genomics deliver climate-change ready crops? Curr. Opin. Plant Biol. 45, 1–7. doi: 10.1016/j.pbi.2018.03.007
Wang, T., Zhang, H., and Zhu, H. (2019). CRISPR technology is revolutionizing the improvement of tomato and other fruit crops. Hortic. Res. 6, 77. doi: 10.1038/s41438-019-0159-x
Yang, N., Liu, J., Gao, Q., Gui, S., Chen, L., Yang, L., et al. (2019). Genome assembly of a tropical maize inbred line provides insights into structural variation and crop improvement. Nat. Genet. 51, 1052–1059. doi: 10.1038/s41588-019-0427-6
Yanggen, D., Kelly, V., Reardon, T., and Naseem, A. (1998). Incentives for fertilizer use in sub-Saharan Africa: A review of empirical evidence on fertilizer response and profitability, MSU International Development Working Paper No. 70. East Lansing, MI: Department of Agricultural Economics, Michigan State University
Zeng, Y., Wen, J., Zhao, W., Wang, Q., and Huang, W. (2020). Rational improvement of Rice yield and cold tolerance by editing the three genes OsPIN5b, GS3, and OsMYB30 with the CRISPR–Cas9 System. Front. Plant Sci. 10, 1663. doi: 10.3389/fpls.2019.01663
Zeven, A. C. (1998). Landraces: a review of definitions and classifications. Euphytica 104, 127–113. doi: 10.1023/A:1018683119237
Zhang, P., Du, H., Wang, J., Pu, Y., Yang, C., Yan, R., et al. (2020). Multiplex CRISPR/Cas9-mediated metabolic engineering increases soya bean isoflavone content and resistance to soya bean mosaic virus. Plant Biotechnol. J. 18, 1384–1395. doi: 10.1111/pbi.13302
Keywords: orphan crops, climate change, genetic resources, genomic-assisted breeding, Sub-Saharan Africa, Bambara groundnut, African yambean, Kersting's groundnut
Citation: Abberton M, Paliwal R, Faloye B, Marimagne T, Moriam A and Oyatomi O (2022) Indigenous African Orphan Legumes: Potential for Food and Nutrition Security in SSA. Front. Sustain. Food Syst. 6:708124. doi: 10.3389/fsufs.2022.708124
Received: 11 May 2021; Accepted: 18 February 2022;
Published: 25 April 2022.
Edited by:
E. O. Deedi Sogbohossou, Wageningen University and Research, NetherlandsReviewed by:
Deepranjan Sarkar, Banaras Hindu University, IndiaDinesh Joshi, Indian Council of Agricultural Research (ICAR), India
Copyright © 2022 Abberton, Paliwal, Faloye, Marimagne, Moriam and Oyatomi. This is an open-access article distributed under the terms of the Creative Commons Attribution License (CC BY). The use, distribution or reproduction in other forums is permitted, provided the original author(s) and the copyright owner(s) are credited and that the original publication in this journal is cited, in accordance with accepted academic practice. No use, distribution or reproduction is permitted which does not comply with these terms.
*Correspondence: Michael Abberton, bS5hYmJlcnRvbiYjeDAwMDQwO2NnaWFyLm9yZw==