- 1Risk and Sustainability Division, Research, Testing and Development, Eskom Holdings SOC, Rosherville, South Africa
- 2Global Change Institute, University of the Witwatersrand, Johannesburg, South Africa
- 3Department of Geography, Environmental Management and Energy Studies, University of Johannesburg, Johannesburg, South Africa
Water and energy interdependency, in the context of climate change, threatens security of these resources. Anecdotal evidence suggests that risks associated with the “Water-Energy-Climate Change” (WECC) nexus will affect all subsectors of the economy. Yet the WECC nexus has not received commensurate attention in research and policy. Some countries have begun identifying avenues to understand and forestall WECC impacts, but less so in developing countries. This has compromised the adaptive capacity and resilience of developing countries' economies to WECC impacts. This paper analyses existing literature, historical and contemporary secondary data on climate change, water and energy interdependency in South Africa. It outlines the interlinkages and implications of these three components on each other, particularly within the discourse of sustainable water resource management. The paper recommends water-centric approaches to improve evidence-based institutional and policy frameworks to address the myriad challenges of this nexus in a holistic and integrated manner.
Introduction
The constant supply of water and energy resources has become an absolute imperative for sustaining modern life. Intensified need for these resources in socio-economic development programs has resulted in an exponential increase in their demand at global, regional, national and local levels (Hussey and Pittock, 2012; Sparks et al., 2014; Carpenter, 2015). The supply of water and energy has, however, became quite complex, given the influence of factors such as rapid population growth, increased food production and consumption, and economic development, all of which combine to result in high demand for these resources in a context characterized by the emergence of climate change and associated environmental stresses. The supply of these resources, together with the need to address climate change, has emerged as one of the central issues for discussion among sustainable development researchers and policy makers. These debates have become topical as suggestions emerge on how the water, energy, and climate change elements relate to each other and how they influences socio-economic issues, resulting in the concept of the Water-Energy-Climate Change (WECC) nexus (Cammerman, 2009; Head and Cammerman, 2010). Anecdotal evidence suggests that the existence of WECC impedes the attainment of environmental and socio-economic goals for communities across the world, hence the need for management of the challenges arising from this nexus. There is, therefore, an urgent need for the development of resilient and adaptive strategies to mitigate the impact of WECC on Sustainable Development Goals (SDGs), particularly the SDGs, 6, 7, and 13.
As demand increases for measures to ensure sustainable water and energy supply while simultaneously combating climate change, contemporary evidence suggests that the understanding of how WECC components are interlinked is limited. This knowledge gap is reflected mainly in the current segmented policy development and planning approaches for addressing water, energy, and climate change challenges–weaknesses that are aggravated by a lack of integration and limited application of analytical tools to manage these WECC components (Bizikova et al., 2013; Rasul and Sharma, 2015; Pahl-Wostl et al., 2018; Gobin et al., 2019). These planning and policy segmentations are common in developing and middle-income countries such as those in Sub-Saharan Africa (SSA). This has resulted in minimal progress toward implementing the principles of sustainable ecological and socio-economic development in the region. While there is a need for coordinated and holistic planning for management of WECC risks and opportunities, approaches should be based on sound empirical evidence and a clear understanding of the interfaces between water, energy, and climate change.
In South Africa, the country's National Developmental Plans (NDP) envisage the reliable supply of water and energy resources under combatted climate impact. Mathetsa et al. (2019) argued that South Africa is prone to the realities of the WECC nexus and its associated repercussions. For instance, the country's energy production system is centralized on water reliant fossil-fuel burning technology, which contributes significantly to climate change through the release of Greenhouse Gases (GHGs). In the same vein, climate change, together with the current reliance on water-intensive energy generation processes to address electricity demands will exert additional pressure on constrained water resources in the country. The current water and energy supply constraints, together with the growing need to address climate changes, impose critical challenges on the attainment of national development plans in South Africa (World Bank, 2017). This raises a need for the country to strengthen analysis and understanding of WECC locally to ensure the development of effective strategies for addressing the risks related to this nexus. As is the case in most developing and some developed countries, South Africa's fragmented approach to planning and policy development on energy, water and climate change is one of the key barriers to the effective integration of these sectors (Prasad et al., 2012; Mabhaudhi et al., 2016; Nhamo et al., 2018; Mathetsa et al., 2019). This is despite internal reforms implemented within each of these sectors. It is against the background of these challenges and inadequacies that the need for a comprehensive understanding of WECC and the promotion of a sectorally integrated and effective management approach be emphasized.
Assessment of existing literature and empirical data is crucial for understanding the interfaces between the WECC components. For instance, empirical approaches that allow for the collection, analysis and presentation of data on these components over a chosen period enable an improved understanding of the WECC interdependencies thus allowing the utilization of such physical data is critical for providing vital knowledge for decision-making on issues related to different nexus configurations (Bless et al., 2013; Liu et al., 2017; Aboelgna et al., 2018). Such knowledge can only be attained where there are systems that enable the collection, availability, accessibility, and usability of data. However, there is a lack of such systems across the globe particularly in the less-economically developed regions of the world. In SSA, for example, Yillia (2019) argued that broadened understanding and progress toward nexus integration are hindered by a lack of information or data, particularly at the grassroots level where most activities are undertaken. Similarly, in South Africa, the unavailability of data and information is one of the causes of the current policy fragmentation and emergent nexus risks (Gobin et al., 2019). This observation suggests the urgent need for South Africa to utilize physical data analysis as one of the key tools for understanding the interactions between water and energy resources, and climate change. Understanding these concurrent interactions will likely promote an integrated approach toward addressing the risks and opportunities emanating from the WECC nexus.
A water-centric approach is one of the most pertinent amongst the different existing methods for analyzing the complexities of the WECC nexus (Cammerman, 2009; Bizikova et al., 2014; McCartney and Brunner, 2020). While this perspective should not promote water governance above other sectoral approaches, the importance of water across different systems suggests that sustainable management of these resources is critical for addressing nexus challenges. Against this background, Head and Cammerman (2010) argue that holistic, coordinated and informative management of the WECC nexus can adopt water-related approaches such as the Integrated Water Resources Management (IWRM) Framework. This framework holistically addresses complex systems that connect water and related resources such as energy and food (Global Water Partnership, 2000; Fatch, 2009; Fulazzaky, 2014). In South Africa, the rationale for applying water-centric approaches such as IWRM in managing WECC is motivated by the dire status of water resources in the country, a situation that is predicted to worsen with serious impacts on related resources and other sectors such as energy and agriculture, respectively. Moreover, existing literature shows how water limitations experienced in the several countries necessitate the adoption of the IWRM approach for understanding and managing WECC, particularly within the context of water being a finite resource (Cammerman, 2009). Based on the preceding arguments, this paper uses existing literature and secondary data obtained from different stakeholders to validate/quantify the relationship that water has with energy and climate change sectors in South Africa. The paper articulates the significance and relevance of a water-centric approach to Assessing and understanding WECC issues. The paper concludes with recommendations on actions that could enhance the promotion of integrated WECC management in South Africa.
The IWRM as a Framework to Analyse the WECC Nexus in South Africa
Studies in the recent past explored the significant role that the nexus has on achieving the sustainable development goals (SDGs). A number of these studies have, mainly focused on the inextricable linkage between key resources of water, energy, and food, resulting in the configurations of Water-Energy (WE) and Water-Energy-Food (WEF) (Prasad et al., 2012; Gulati et al., 2013; Carter and Gulati, 2014; Cullis et al., 2018). Several studies define the nexus as a tool that promotes synergies and trade-off of water, energy and food systems, thus guiding coordinated and integrated approaches for formulating development policy and for the purposes of ensuring resource efficiency and optimization (Albrecht et al., 2008; Mathetsa et al., 2019; Pahl-Wostl et al., 2020). Given the rising need to equally address the water, energy and food constrains, development of contemporary, integrated and analytical measures becomes imperative to ensure security of these resources. The assessment of the interlinkages of the nexus and the development of such approaches to understand and measure their interactions, could probably be executed by way of employing a sectoral approach and creating an inventory of resource relationships. Several scholarly literature suggests approaches that are within a water-centric system and which can potentially be used to analyse the resource efficiencies embedded within the nexus discourse (Cammerman, 2009; Mathetsa et al., 2019; McCartney and Brunner, 2020). From a point of view therefore, we can argue that the nexus approach can potentially offer a framework through which to comprehensively understand and manage the social and economic implications it has in water-stressed countries such as South Africa. It is against these observations that in this paper we assess the nexus in the context of IWRM Framework, an approach highly regarded as suitable for promoting sustainable water resource management. Selection of the framework is triggered by the fact that South Africa is the 30th driest country in the world and has had devastating impacts from water scarcity (Carpenter, 2015; Mathetsa et al., 2019).
Although several scholars define IWRM differently, its primary goal remains the promotion of sustainable water resources management. Global Water Partnership (2000), for example, define the IWRM as “a process which promotes the coordinated development and management of water, land and related resources, in order to maximize the resultant economic and social welfare in an equitable manner without compromising the sustainability of vital ecosystems”. This definition is embedded in the need for coordinated and effective management of all aspects of surface and groundwater and their hydrological boundaries, as well as “related resources,” depending on or influencing water availability. In this case, water-dependence sectors such as food production, energy generation, domestic utilization and climatic variables change highlight key interactions between human-induced processes and water (Funke et al., 2007; Bindra et al., 2014). It is argued that while IWRM should not be viewed as a replacement for policy, its principles facilitate the development of strategies, tools or management guidelines, thus initiating sustainable water resource management. The successes of this framework are based on its ability to coordinate the management of water resources and associated activities in a holistic and risk-based manner (Global Water Partnership, 2000; Leendertse et al., 2008; Fulazzaky, 2014). This is despite its documented shortcomings such as lack of innovative approaches (Biswas, 2008).
Implementation of IWRM has been key in water sector reform processes in South Africa. For instance, application of the IWRM framework is integral to post-apartheid administrative tools such as the National Water Act (Act No. 36 of 1998) and the National Water Resource Strategy, which was designed to enhance sustainable and equitable water resources management and use at both national and catchment levels [Department of Water and Sanitation (DWS), 2004, 2014; Pollard and Du Toit, 2008]. The application of this framework in some parts of South Africa has, however, not been effective particularly at ground level. Claasen (2013) attributes this inconsistency to various factors, which include limited experience and lack of information, inadequate capacity and inability to innovate among various stakeholders. These inadequacies are cause for serious concern, as is the ineffective management of water resources at the grassroots level and the potential impact it has on water security and other sectors.
While the application of IWRM is mainly on water resources management, Cammerman (2009) and Fulazzaky (2014) suggest that this framework can be considered as a tool for management of other complex systems, particularly those prompted or generated by human interaction with nature. The WECC nexus provides a good example of a complex system amenable to the application of the IWRM framework as it elaborates the inextricable linkages between water, energy and climate change–elements that are closely linked to human activities. Moreover, such a framework allows for the consideration and incorporation of other relevant disciplines and stakeholders in ensuring sustainability, equity, and efficiency, not only in the water sector but in other sectors as well (Bindra et al., 2014). Centralizing WECC arguments based on water-related information, therefore, enable promotion of integrated management of this nexus.
As stated, the pronounced interdependence of water and energy resources, both of which are finite, presents systematic challenges on how to address socio-economic developmental needs. Of the two, water resource security has been more scrutinized because of its intimate linkage to climate change and the limit in terms of alternatives to water. Within SSA, South Africa is an example of a country with water resource predicament in several ways. First, it is semi-arid, with low rainfall, and deteriorating water quality due to anthropogenic activities and over allocation, suggesting that the country's water resources are undoubtedly finite. Secondly, South Africa's vulnerability to climate change exacerbates its water insecurity. Last, water plays a critical role in various sectors of the country's economy, including in energy and food production, which means the country faces the urgent need for development of effective adaptation and mitigation measures to ensure sustainable use and the protection of limited water resources.
Furthermore, the water resources constraints in South Africa are aggravated by the current administrative tools' inability to address emerging risks and opportunities, thus hindering attainment of national developmental endevours [Department of Water and Sanitation (DWS), 2014]. This is likely to influence governance of multiple systems that incorporate or interact with water resource dynamics thereby affecting the attainment of the country's NDPs. It is therefore important to understand the water user-use relationship. In this study, large freshwater users such as the energy generation and agriculture sectors are considered significant to management of water resources. Therefore, the concept of water as a finite resource is among the key themes used for analysis of the risks associated with WECC. This perspective is aligned to the proposition by Funke et al. (2007) that South Africa should adopt an inclusive approach to the management of water resources, which considers all hydrological cycles and water's interactions with other resources and the ecosystem as a whole. This study, therefore, emphasizes the need to acknowledge the IWRM principle that calls for the full recognition of the finite and indispensable nature of freshwater resources in the coordinated governance of the nexus.
Methodology
The study applied different approaches to attain its objectives. Firstly, a rapid appraisal of the literature on the nexus subject was conducted. Both Scopus and Google Scholar were used to search for the words “water”, “energy”, “food”, “climate change”, “nexus” and IWRM in the abstract, title and keywords. The search resulted in approximately 250 peer-reviewed articles. In addition, tools and methods for the nexus application developed by non-governmental or inter-governmental research and policy institutions (e.g., FAO, WRC, IPCC, DWS, and DEA) were used to supplement the literature reviewed. Articles and reports meeting the following criteria were used: (1) they employ the nexus concept in terms of natural resource sustainability; (2) they include all four sectors of water, energy, food and climate change, and (3). they outline methodologies or analytical tools to assess the nexus studies, and (4). The IWRM successes and failures are defined. After a rapid appraisal of the 250 articles, 36 articles were classified as “methodological”, 82 were “conceptual”, while the remaining 132 were excluded from the study as they did not met the above defined criterion. The selected articles and reports were further analyzed using a meta-analysis technique and they form the basis of the current paper.
Secondly, the study adopted a quantitative approach to assess the water, energy and climate change relationships within the context of South Africa. Several scholars have suggested that the discussions and interpretations on the WECC configurations are more strengthened and informative when the nexus is assessed within the discourses of both physical and social measurements (Liu et al., 2017; Aboelgna et al., 2018). This approach was chosen based on views in the literature that assert that the assessment of nexus configurations can be streamlined by using statistical methods to quantify the interlinkages (Cammerman, 2009; Bless et al., 2013; de Strasser et al., 2016; Aboelgna et al., 2018). This approach was used to particularly to quantify the qualitative data in order to comprehensively understand the interactive role that water, a finite resource in South Africa, has with both the energy and climate change systems.
The study analyzed secondary data from sectors that play a key role in the management of climate change, and energy and water resources in South Africa. Purposive sampling was used to select sources for data collection from these key sectors and relevant departments. This sampling method involves the deliberate identification and selection of role players who are knowledgeable in the subject and context under study as key informants to provide data from these key sectors/departments (Etikan et al., 2016).
The power utility Eskom, the South African Weather Services (SAWS), Water Service Providers (Municipality and Rand Water), the Departments of Water and Sanitation (DWS) and Environmental Affairs (DEA) were selected as sources from which sector-specific data would be sourced. Data was mainly collected from the power generation regions of Waterberg and Highveld, as illustrated in Figures 1, 2. Data collection was enhanced by a snowball technique where referrals were used to ensure data is obtained from relevant personnel within the selected sectors. This resulted in interviews with key data management personnel who also supplied secondary data in excel format. As illustrated in Table 1, secondary data collected from Eskom and SAWS was of primary significance in generating information used for the study, while data from the other sources was mainly used for appraisal purposes. The sourced data was predominantly generated from the Waterberg and Highveld regions, which are both major locations of coal-fired energy generation technology in South Africa.
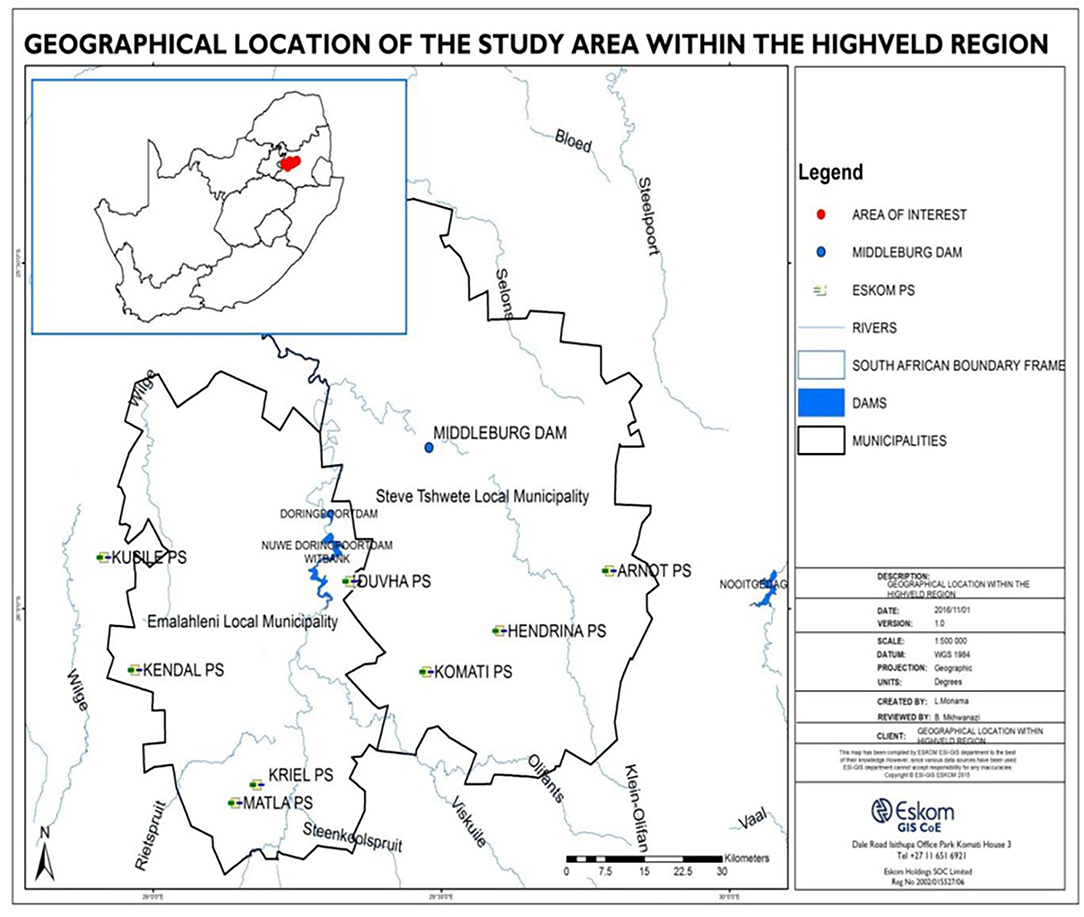
Figure 1. Geographical location of the Power Stations at Highveld region, South Africa. Data Source: Eskom (2019).
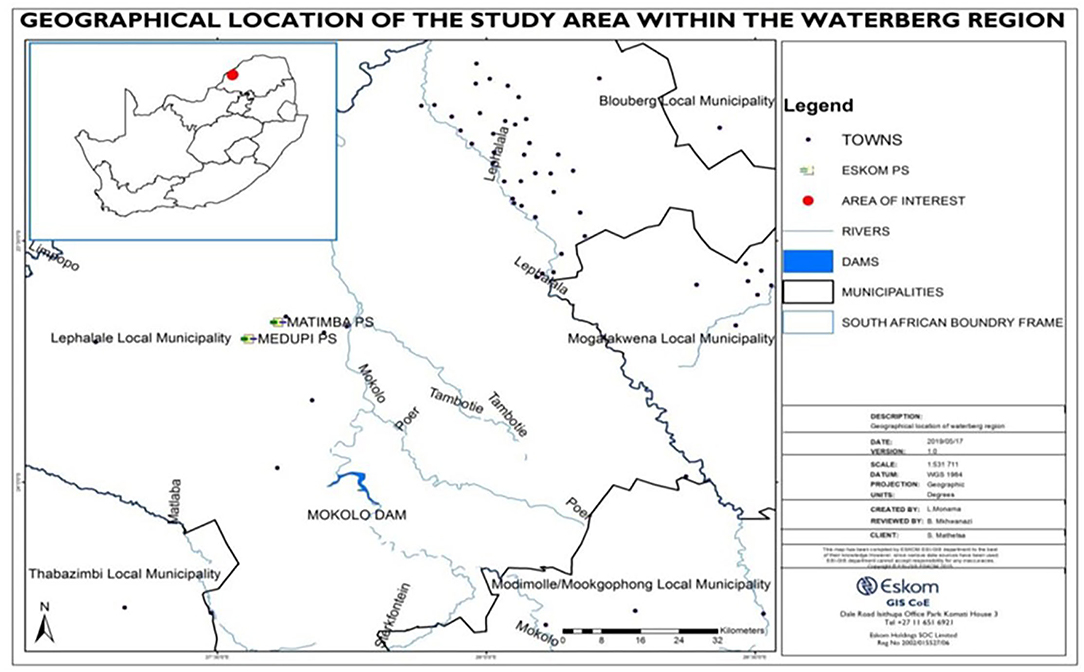
Figure 2. Geographical location of the Power Stations at Waterberg Region, South Africa. Data Source: Eskom (2019).
Data from 2008 to 2018 was analyzed using descriptive statistical analysis. Application of the method resulted in interpretative graphs derived from the mean values attained from the data, thus highlighting the key points at which water conversely interacted with energy and climate change. The analysis enabled the understanding and subsequent discussion of WECC nexus complexities and their interdependencies.
Results and Discussion
The collected data allowed for the assessment of the water-energy; energy-climate change; and climate change-water nexus interfaces. The deliberations adopted the water-centric approach to enable understanding of the complex WECC nexus in South Africa.
Converse Water-Energy Interchange
Coal combustion, which requires water as a key input, accounts for approximately 70% of energy generation globally (Carpenter, 2015). It is established that nearly 75% of freshwater withdrawals are used solely for electricity generation processes, particularly coal-combustion technology (Cammerman, 2009; Carpenter, 2015). In South Africa, approximately 85% of energy is generated from coal-combustion, thus exerting enormous pressure on the supply of water resources. Figure 3 shows the severity and magnitude of water usage at the power generation stations in the Highveld and Waterberg regions of South Africa. These power plants contribute approximately 32,000 megawatts (MW) of the country's total electricity generation installed capacity of 45,000 MW. Figure 3 also draws a comparison of the amount of water, in liters (l), used to produce Kilowatt per hour Send Out (KWhSO) electricity from each region between the years 2008 to 2018.
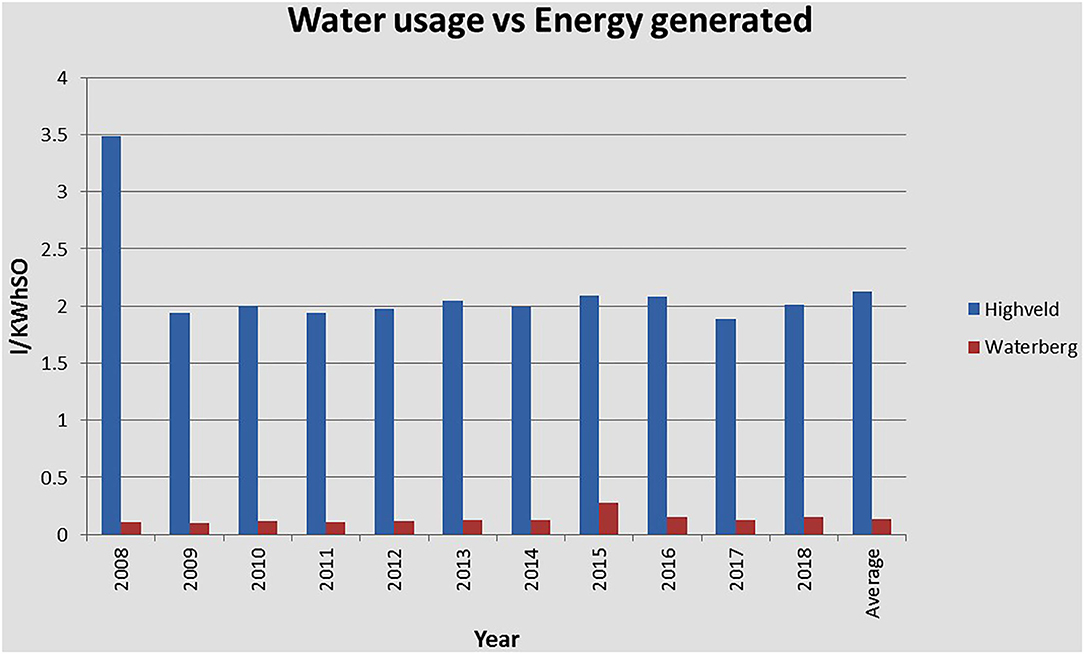
Figure 3. Water used per Kilowatt per hour Sent Out for the Highveld and Waterberg stations. Source: Data from Eskom (2019).
It is evident from Figure 3 that the power plants in the Highveld region consume more water than those in the Waterberg. In 2008, for example, the Highveld power plants to generate 1 KWhSO compared to 0.1 l /KWhSO in the Waterberg stations used 3.5 l. On average, Highveld power plants utilized 2 l/KWhSO more than those in the Waterberg did over the 11-year period for which records were analyzed. This is influenced by significant variances at the Highveld power plants between 2008 and 2010, and at the Waterberg stations between 2014 to 2016. For example, Eskom (2019) highlights that addition and reduction of generating units between 2008 and 2011 was a contributing factor in the observed variances in water consumption. While Figure 1 highlights the significant role of water use in electricity generation, the quantity of water required is influenced by several factors. First, is the capacity of power plants. For example, the power plants in the Highveld have an installed capacity of approximately 24,000 MW, compared to the 8,000 MW capacity in the Waterberg. The power plants in the Highveld therefore use more water in their generation processes. The second factor influencing water consumption is the technology applied in each power plant. All power plants in the Waterberg employ a dry-cooled process in contrast to six of the eight in the Highveld, with the remaining two being dry-cooled. Both technologies, however, do rely on water. The dry-cooling power generation process consumes approximately 15% less water than wet-cooled power generation (Pather, 2004; Cullis et al., 2018). This high demand for water in electricity generation has pushed the country toward the building of dry-cooled power plants post the 1980s [Department of Water and Sanitation (DWS), 2014].
The water needs for electricity generation resulted in categorization of the energy sector as a “strategic water user” with a high assurance of 2% freshwater allocation. However, the sector remains vulnerable due to the current and projected freshwater shortages in the country, which threatens energy security. The vulnerability of the energy sector to freshwater shortages has resulted in supply to the power plants in the Highveld region being maintained through water imports from pristine catchments such as Usuthu and Inkomati. Furthermore, the country has adopted strategies and initiatives such as the Mokolo-Crocodile Augmentation Process and the Lesotho Highlands Water Project to avert shortages in water constrained regions such as the Waterberg. These strategies have thus elevated the current and projected freshwater withdrawals for energy and other sectors such as agriculture and domestic consumption.
The contribution of the energy sector to the water value chain is equally important. Approximately 3% of the energy produced globally is consumed by water related processes, and in South Africa, this consumption is the third largest in the world (South African Cities Network, 2014; Gobin et al., 2019). Mainly the rising population, industrialization, urbanization, and pollution drive the increasing demand for energy in the water and wastewater treatment industry in the country. Moreover, the long distance between water sources and intended users increases the energy needed for pumping and treatment (Cullis et al., 2018). Despite the importance of this subject globally, information related to energy usage in the water value chain is hard to come by and difficult to maintain due to a variety of factors. These include the fact that there is a wide variety of water users, while water usage also varies in several sectors, which include domestic, industrial and other sectors (Liu et al., 2017). In a few cases, however, countries such as the United States and Australia have effectively quantified the energy needed for their water supply value chain (abstraction, treatment and distribution) to inform their planning processes (Cammerman, 2009; Carpenter, 2015).
In South Africa, the present study has identified data collection and accessibility challenges in relation to the energy-water interface. For instance, data on energy utilized for water and wastewater treatment is rarely quantified or made available by key institutions such as water service providers and municipalities. This creates a scenario where institutions develop policies and strategies that are misaligned and serious deficiencies in governance processes on water and energy management. This finding calls for key role players in the energy and water supply value chain to urgently address the data collection and accessibility gaps.
Energy-Climate Change Relationship
The energy sector's contribution to climate change is a global challenge. This is particularly so I relation to GHG emissions from coal combustion [Intergovernmental Panel on Climate Change (IPCC), 2014; Carpenter, 2015]. Figures 4, 5 show the relative amount of carbon dioxide (CO2) and nitrous oxide (N20) observed at coal-fired power plants, with minimal contribution from Open Cycle Gas Turbines (OCGT). In South Africa, for example, coal combustion is currently the most reliable source of energy, and yet it contributes significantly toward GHG emissions (Seymore et al., 2014; Cullis et al., 2018). The difference in the emissions from these two electricity-generating technologies is due to the higher output of GHGs from coal combustion as compared to OCGT and the predominance of coal combustion for electricity production. Eskom (2019) states that OCTG usage is minimal and it comes online only when supply from coal-fired power plants is severely constrained.
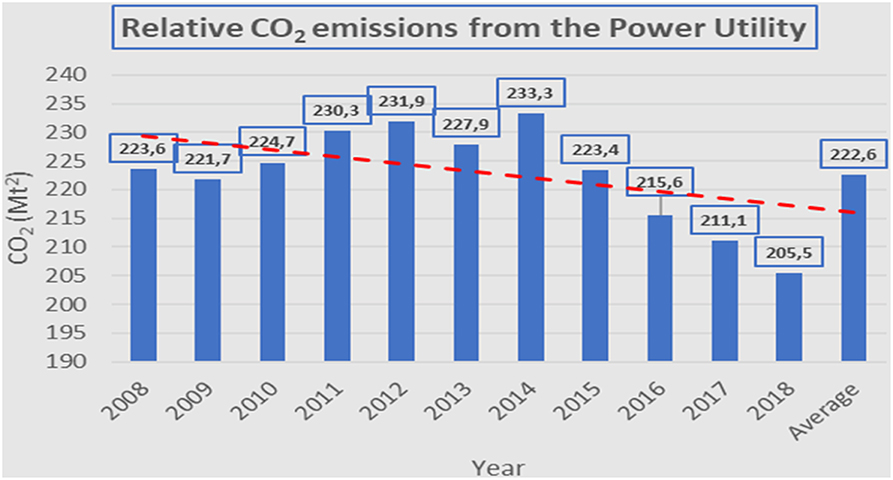
Figure 4. Relative CO2 produced during fuel-burning electricity generation. Source: Data from Eskom (2019).
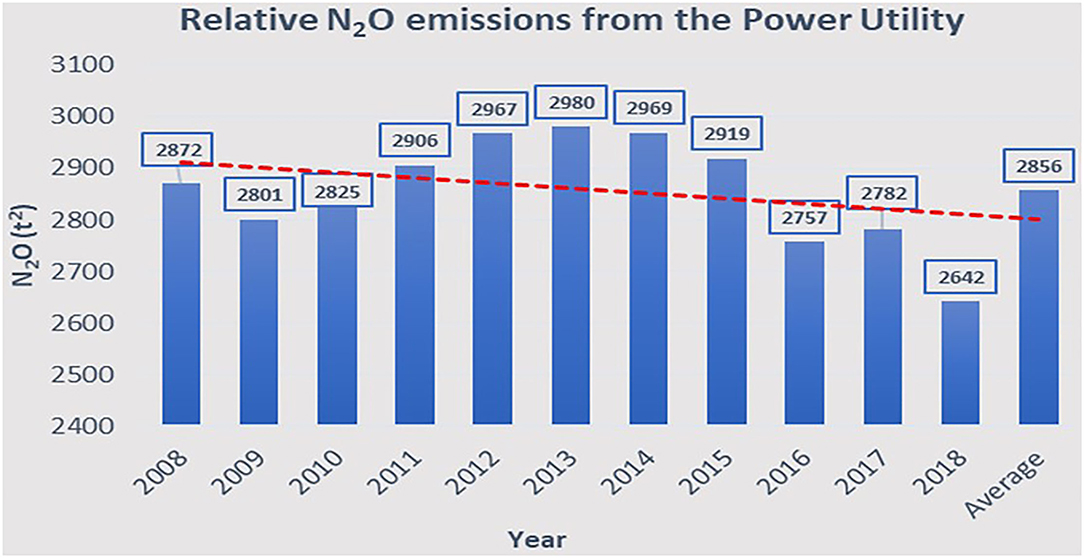
Figure 5. Relative N2O produced during fuel-burning electricity generation process. Source: Data from Eskom (2019).
Department of Environmental Affairs (DEA) (2015) indicates that CO2 emissions in South Africa are largely due to contributions from the energy sector. An average of 222.6 Mt2 CO2 was emitted annually between 2008 and 2018, with the highest concentrations of 233.3 Mt2 recorded in 2014. However, there was a steady decrease in the CO2 emitted annually from 2014 to 2018. This corresponds with the decline of 0.04% CO2 emissions recorded from the energy sector since 2012 [Department of Environmental Affairs (DEA), 2015]. Similarly, the energy sector emits high concentrations of N2O. For example, it is illustrated in Figure 5 that the average annual N2O concentrations from coal combustion electricity generation was 2,865 t2 between 2008 and 2018, with the highest relative figure of 2,980 t2 recorded in 2013.
These findings are clear evidence of the contribution of the country's energy sector to GHG emissions. The decline in the release of CO2 and N2O, however, is an indication of the efforts being made to manage these emissions, and this could be attributed to the improved policy context and awareness of the impact of climate change on natural and socioeconomic resources, particularly on finite water resources.
Water-Climate Change Relationship
The impact of climate change on water resource availability is widely recognized (Hejazi et al., 2014; Carpenter, 2015; Gobin et al., 2019; Mathetsa et al., 2019). Key drivers of the hydrological cycle include climate change-related factors, such as high temperature and moisture conditions, which, in turn, influence water availability. In South Africa, climate change-related extreme hydrological events such as prolonged droughts, floods and intensified rainfall already occur quite regularly (Nkhonjera, 2017; Mathetsa et al., 2019). Figure 6 shows the relationship between temperature and rainfall, thus water availability, in the Highveld and Waterberg regions. It is evident that the Waterberg experiences higher and more variable temperatures than the Highveld. For example, the Waterberg recorded highly fluctuating temperature profiles for two reporting periods of 2009 to 2011 and 2014 to 2017 while the Highveld remained mainly constant throughout the period 2008 to 2018. The temperature data supports an observation by Eskom (2012) that the Waterberg is among the regions expected to experience significant warming resulting from climate change impacts.
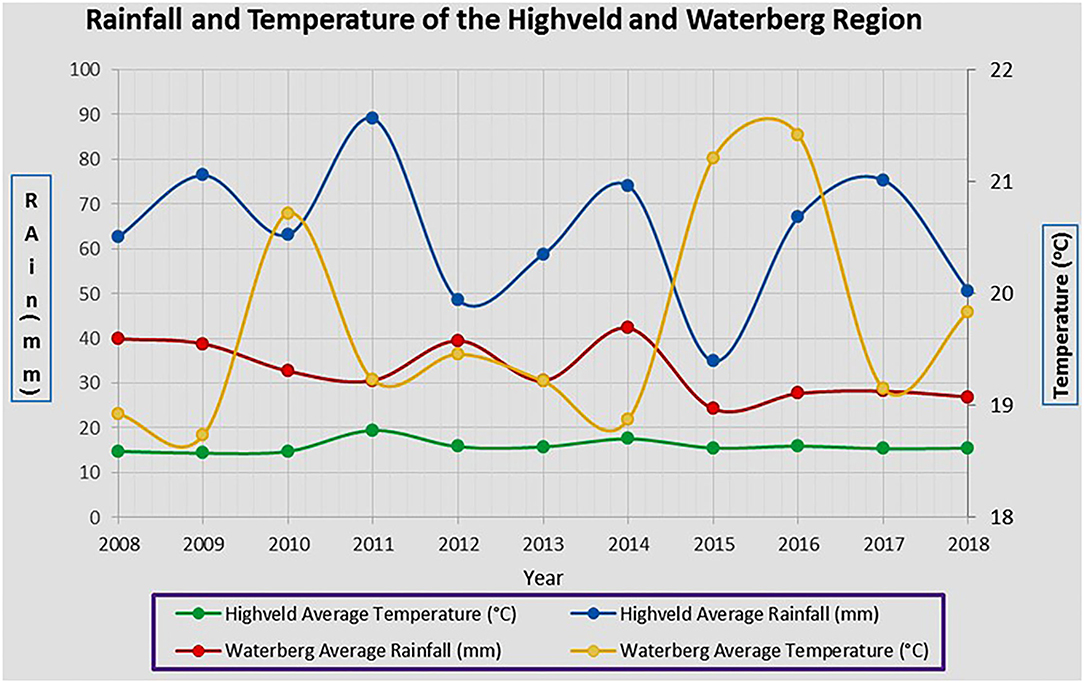
Figure 6. Comparison of the temperature and rainfall interactions. Source: Data from South African Weather Services (SAWS) (2019).
Furthermore, Figure 6 shows how the varying rainfall patterns in each region are influenced by respective temperature profiles. For example, the Highveld recorded lower temperatures and high, varying, rainfall patterns. This is contrary to the higher temperatures and lower, constant, rainfall recorded in the Waterberg region. The average rainfall in each region, however, remains lower than the country's annual average of 495 mm. This observation supports the assertion by Mukheibir (2008) that inland temperature is expected to increase by ~2–3°C compared to the 1.5°C increase expected in the coastal areas, thus lowering the region's precipitation intensity, frequency, and duration. Whereas the unpredictable variations and increased rainfall intensities will exert pressure the finite water resources in the country, Eskom (2012) and Nkhonjera (2017) contended that climate variabilities in the Waterberg and Highveld regions would intensify the demand for water from the Olifants and Mokolo catchments. This is likely to affect both water-dependent ecological activities and socio-economic sectors, predominantly energy and agriculture, in a negative manner.
Conclusion and Recommendations
The present paper assessed the interactions between the elements of water, energy and climate change, the result of which is considered one of the emerging threats to the attainment of the country's energy and water security. The study shows that the intertwined relationship between the water, energy and climate change sectors in South Africa is inevitable, pointing to the existence of a WECC nexus. This is confirmed by the data used to assess several key interfaces between the sectors of WECC. Despite the paper attesting that data is paramount for the evaluation of nexus interlinkages, the study also revealed limitations in the existing systems and processes for measuring and availing data which links some of the WECC components in South Africa. The inadequacies in these systems are aggravated by the limited coordination and integration of approaches for the management of this nexus. Any gap in the data management system associated with the WECC nexus has the potential to derail the country's plans to manage this nexus effectively and in an integrated manner. South Africa should, therefore, move toward the improvement of management systems to ensure data is collected, stored, accessible, and used to inform the emergence of an institutional and policy framework for sustainable resource management.
While the nexus approach indicates that the water, energy and climate change sectors are equally important and should be managed as such, the current situation in the country presents South Africa with an opportunity to assess this nexus within the context of water resource management. This is key for the enhanced understanding and governance of the WECC nexus, largely due to the crosscutting role that water, despite its finite status, continues to play across various sectors in the country. This paper recommends that a water-centric approach be adopted to enhance the establishment of an evidence-based institutional and policy framework to respond to the socio-economic challenges associated with the WECC nexus.
Data Availability Statement
The data was made available specifically for this project. Requests to access these datasets should be directed to SMM at steviemathetsa@gmail.com.
Author Contributions
SMM collected the data and wrote the article in consultation with MDS and ITR who were the supervisors of the PhD research project. All authors contributed to the article and approved the submitted version.
Funding
The study was undertaken as part of the PhD project titled Water-Energy and Climate Change Nexus in South Africa: Application of the Integrated Water Resource Management (IWRM) approach for Sustainable Development which was sponsored by Eskom (Research Number: N.RA.60008.R02.009) and the National Research Fund's Global Grand Challenge, under the funding instrument Global Change Social Science Research Programme (Grant No: 129481).
Author Disclaimer
The views presented in this paper are those of the authors and therefore does not reflect the position of Eskom.
Conflict of Interest
At the time of writing this article, SMM was employed by Eskom.
The remaining authors declare that the research was conducted in the absence of any commercial or financial relationships that could be construed as a potential conflict of interest.
Publisher's Note
All claims expressed in this article are solely those of the authors and do not necessarily represent those of their affiliated organizations, or those of the publisher, the editors and the reviewers. Any product that may be evaluated in this article, or claim that may be made by its manufacturer, is not guaranteed or endorsed by the publisher.
Acknowledgments
The authors would like to thank Eskom SOC (Ltd), the South African Weather Services, and the Department of Water and Sanitation for providing the study data.
References
Aboelgna, H. T., Khalifa, M., Mcnamara, I., Ribbe, L., and Sycz, J. (2018). The Water-Energy-Food Security Nexus, A review of Nexus literature and ongoing Nexus initiatives for policymakers. Bonn, Germany: Nexus Regional Dialogue Programme (NRD).
Albrecht, T. R., Crootof, A., and Scott, C. A. (2008). The water-energy-food nexus: A systematic review of methods for nexus assessment. Environ. Res. Lett. 13, 043022. doi: 10.1088/1748-9326/aaa9c6
Bindra, S. P., Hamid, A., Salem, H., Hamuda, K., and Abulifa, S. (2014). Sustainable integrated water resources management for energy production and food security in Libya. Procedia Technology. 12, 747–752. doi: 10.1016/j.protcy.2013.12.558
Biswas, A.K. (2008). Integrated Water Resources Management: Is it working? Int. J. Water Resour. Dev. 24, 5–22. doi: 10.1080/07900620701871718
Bizikova, L., Roy, D., Swanson, D., Venema, H. D., and Mccandless, M. (2013). The Water-Energy-Food Security Nexus: Towards a practical planning and decision-support framework for landscape investment and risk management. International Institute of Sustainable Development Report. Available online at: http://www.cilt.uct.ac.za/sites/default/files/image_tool/images/91/Bizikova%20et%20al.%20wef_nexus_2013%20IISD.pdf (accessed February 22, 2019).
Bizikova, L., Roy, D., Venema, D. H., Mccandless, M., Darren Swanson Khachtryan, A., Borden, C., et al. (2014). The Water-Energy-Food Nexus and Agricultural Investment: A sustainable development guidebook. International Institute for Sustainable Development (IISD).
Bless, C., Higson-Smith, C., and Sithole, S. L. (2013). Fundamentals of Social Research Methods: An African Perspective. 5th edition. South Africa: Juta & Co.
Cammerman, N. (2009). Integrated Water Resource Management And The Water, Energy, Climate Change Nexus: A Discussion Report. Masters Thesis. Australia: University of Queensland.
Carpenter, A. M. (2015). Water availability and policies for the coal power sector. United Kingdom: IEA Clean Coal Centre.
Carter, S., and Gulati, M. (2014). Climate change, the food energy water nexus and food security in South Africa: understanding the food-energy-water nexus. WWF-SA 1–17.
Claasen, M. (2013). Integrated water resource management in South Africa. Int. J. Water Resour. Dev. 1 323–338. doi: 10.7564/13-IJWG12
Cullis, J. D. S., Walker, N. J., Ahjum, F., and Rodrigues, D. J. (2018). Modelling the water-energy nexus: should variability in water supply impact on decision making for future energy supply options? Int. Associat. Hydrol Sci. 376, 3–8. doi: 10.5194/piahs-376-3-2018
de Strasser, L., Lipponen, A., Howells, M., Stec, S., and Bréthaut, C. (2016). A methodology to assess the water energy food ecosystems nexus in transboundary river basins. Water. 8.1–29. doi: 10.3390/w8020059
Department of Environmental Affairs (DEA) (2015). South Africa's Greenhouse Gas Inventory report 2000-2015. Available online at: https://www.environment.gov.za/sites/default/files/reports/GHG-National-Inventory-Report-SouthAfrica-2000-2015.pdf (accessed July 26, 2019).
Department of Water and Sanitation (DWS) (2004). National Water Resource Strategy (NWRS). Water for Equitable and Sustainable Future. Pretoria, South Africa: Government printers
Department of Water and Sanitation (DWS) (2014). National Water Resource Strategy (NWRS). Water for Equitable and Sustainable Future. Pretoria, South Africa: Government printers.
Eskom (2012). Water, Energy and Development. Johannesburg, South Africa: Sustainability Group, Research, Testing and Development. p. 6.
Eskom (2019). Geographical Information Systems Center of Excellence. Available online at: http://www.eskom.co.za/Whatweredoing/ElectricityGeneration/Pages/Electricity_Generation.aspx (accessed March 26, 2019).
Etikan, I., Musa, S. A., and Alkasim, R. S. (2016). Comparison of convenience sampling and purposive sampling. Am J Theor Appl Stat. 5, 1–4. doi: 10.11648/j.ajtas.20160501.11
Fatch, J. J. (2009). Local participation in transboundary water resources management: The case of Limpopo. MSc Thesis. Basin, Zimbabwe: University of Zimbabwe.
Fulazzaky, M. A. (2014). Challenges of integrated water resources management in Indonesia. Water. 6, 2000–2020. doi: 10.3390/w6072000
Funke, N., Oelofse, S. S. H., Hattings, H., Ashton, P. J., and Turton, A. R. (2007). IWRM in developing countries: lessons from the Mhlatuze catchment in South Africa. Physics and Chemistry of the Earth. 32, 1237–1245. doi: 10.1016/j.pce.2007.07.018
Global Water Partnership (2000). Integrated Water Resources Management. Stockholm, Sweden: Report by Technical Advisory Committee. TAC background papers no. 4.
Gobin, A., Sparks, D., Okedi, J., Armitage, N., and Ahjum, F. (2019). Assessing the energy and carbon footprints of exploiting and treating brackish groundwater in Cape Town. Water SA. 45, 63–74. doi: 10.4314/wsa.v45i1.08
Gulati, M., Jacobs, I., Jooste, A., Naidoo, D., and Fakir, S. (2013). The water-energy-food security nexus: challenges and opportunities for food security in South Africa. Aquatic Procedia. 1, 150–164. doi: 10.1016/j.aqpro.2013.07.013
Head, B., and Cammerman, N. (2010). The Water-Energy Nexus, A Policy Challenge for Knowledge and Policy. Australia: Urban Water Security Research Alliance, Technical Report No. 39.
Hejazi, M. I., Edmonds, J., Clarke, L., Kyle, P., Davies, E., Chaturvedi, V., et al. (2014). Integrated assessment of global water scarcity over 21st century under multiple climate change mitigation policies. Hydrol. Earth Syst. Sci. 18, 2859–2883. doi: 10.5194/hess-18-2859-2014
Hussey, K., and Pittock, J. (2012). The Energy-Water Nexus: Managing the Links between Energy and Water for Sustainable Future. Ecol. Soc. 17, 31–39. doi: 10.5751/ES-04641-170131
Intergovernmental Panel on Climate Change (IPCC) (2014). Climate Change 2014: Mitigation of Climate Change. Contribution of Working Group III to the Fifth Assessment Report of the Intergovernmental Panel on Climate Change, Edenhofer, O., Pichs-Madruga, R., Sokona, Y., Farahani, E., Kadner, S., Seyboth, K., Adler, A., et al. (eds.). Cambridge, United Kingdom and New York, NY, USA: Cambridge University Press.
Leendertse, K., Mitchell, S. A. M., and Harlin, J. (2008). IWRM and the environment: A view on their interaction and examples where IWRM led to better environmental management in developing countries. Water SA 34, 691–698. doi: 10.4314/wsa.v34i6.183671
Liu, J., Yang, H., Cudennec, C., Gain, A. K., Hoff, H., Lawford, R., et al. (2017). Challenges in operationalizing the water–energy–food nexus. Hydrological Sci. J. 62, 1714–1720. doi: 10.1080/02626667.2017.1353695
Mabhaudhi, T., Mpandeli, S., Madhlopa, A., Modi, A. T., Backeberg, G., and Nhamo, L. (2016). Southern Africa's water-energy nexus: towards regional integration and development. Water. 8, 1–21. doi: 10.3390/w8060235
Mathetsa, S. M., Simatele, M. D., Rampedi, I. T., and Gericke, G. (2019). Perspectives on integrated water resource management and its relevance in understanding the water-energy-climate change nexus in South Africa. J. Energy South. Africa. 30, 11–21. doi: 10.17159/2413-3051/2019/v30i3a5654
McCartney, M., and Brunner, J. (2020). Improved water management is central to solving the water-energy-food trilemma in Lao PDR. Int. J. Water Resour. Dev. doi: 10.1080/07900627.2020.1754175
Mukheibir, P. (2008). Water Resources Management Strategies for adaptation to climate-induced impacts in South Africa. Water Resources Management. 22, 1259–1276. doi: 10.1007/s11269-007-9224-6
Nhamo, L., Ndlela, B., Nhemachena, C., Mabhaudhi, T., Mpandeli, S., and Matchaya, G. (2018). The water-energy-food nexus: Climate risks and opportunities in Southern Africa. Water. 10, 567–584. doi: 10.3390/w10050567
Nkhonjera, K. G. (2017). Understanding the impact of climate change on the dwindling water resources of South Africa, focusing mainly on Olifants River basin: A review. Environ Sci Policy. 71, 19–29. doi: 10.1016/j.envsci.2017.02.004
Pahl-Wostl, C., Bhaduri, A., and Bruns, A. (2018). Editorial special issue: The nexus of water, energy and food-An environmental governance perspective. Environ Sci Policy. (2018) 90, 161–163. doi: 10.1016/j.envsci.2018.06.021
Pahl-Wostl, C., Gorris, P., Jager, N., Gorris, P., Koch, L., Lebel, L., et al. (2020). Scale-related governance challenges in the water–energy–food nexus: toward a diagnostic approach. Sustain. Sci. 16, 615–629. doi: 10.1007/s11625-020-00888-6
Pather, V. Eskom and Water. In: Proceedings of the 2004 Water Institute of Southern Africa (WISA) Biennial Conference. Johannesburg: Water Institute of Southern Africa (2004).
Pollard, S., and Du Toit, D. (2008). Integrated water resource management in complex systems: How the catchment management strategies seek to achieve sustainability and equity in water resources in South Africa. Water SA. 34, 671–680. doi: 10.4314/wsa.v34i6.183668
Prasad, G., Boulle, M., Boyd, A., Rahlao, S., Wlokas, H., and Yaholnitsky, I. (2012). Energy, water and climate change in Southern Africa. What are the issues that need further investment and research? Cape Town, South Africa: Energy Research Centre, University of Cape Town.
Rasul, G., and Sharma, B. (2015). The nexus approach to water-energy-food security: an option for adaptation to climate change. Climate Policy. 16, 682–702. doi: 10.1080/14693062.2015.1029865
Seymore, R., Inglesi-Lotz, R., and Blignau, J. (2014). A greenhouse gas emissions inventory for South Africa: a comparative analysis. Renew. Sust. Energ. Rev. 34, 371–379. doi: 10.1016/j.rser.2014.03.028
South African Weather Services (SAWS) (2019). Personal Communication. Eco Glades block 1b, Eco Park, Corner Olievenhoutbosch and Ribbon Grass Streets, Centurion, South Africa.
Sparks, D., Madhlopa, A., Keen, S., Moorlach, M., Dane, A., Krog, P., et al. (2014). Renewable energy choices and their water requirements in South Africa. J. Energy in Southern Africa. 25, 80–92. doi: 10.17159/2413-3051/2014/v25i4a2241
World Bank (2017). Modeling the Water-Energy Nexus: How Do Water Constraints Affect Energy Planning in South Africa?. Washington DC: World Bank. Available online at: http://documents.worldbank.org/curated/en/873091468155720710/pdf/851260BRI0Live00Box382147B00PUBLIC0.pdf (accessed December 06, 2018).
Keywords: water, energy, climate change, nexus, South Africa
Citation: Mathetsa SM, Simatele MD and Rampedi IT (2022) A Water-Centric Approach in the Assessment and Governance of the Water-Energy-Climate Change Nexus in South Africa. Front. Sustain. Food Syst. 6:691704. doi: 10.3389/fsufs.2022.691704
Received: 15 April 2021; Accepted: 02 March 2022;
Published: 13 April 2022.
Edited by:
Wenfeng Liu, China Agricultural University, ChinaReviewed by:
Sisira S. Withanachchi, University of Kassel, GermanyRabi Mohtar, Texas A&M University, United States
Copyright © 2022 Mathetsa, Simatele and Rampedi. This is an open-access article distributed under the terms of the Creative Commons Attribution License (CC BY). The use, distribution or reproduction in other forums is permitted, provided the original author(s) and the copyright owner(s) are credited and that the original publication in this journal is cited, in accordance with accepted academic practice. No use, distribution or reproduction is permitted which does not comply with these terms.
*Correspondence: Steven Matome Mathetsa, steviemathetsa@gmail.com