- 1Department of Horticultural Science, Faculty of Agriculture, Agricultural Sciences and Natural Resources University of Khuzestan, Mollasani, Iran
- 2Department of Plant Protection, Agricultural Sciences and Natural Resources University of Khuzestan, Mollasani, Iran
The application of algae has been considered a key element for integrated disease management in sustainable agriculture. These organisms can act as a bio-stimulant for induction of resistance against a variety of abiotic and biotic agents that cause economical loss to crop production globally. Charcoal rot disease caused by Macrophomina phaseolina (Tassi) Goid. is one of the biotic agents restricting strawberry (Fragaria × ananassa Duch.) yield in many cultivation sites. Herein, the foliar application of brown alga (Sargassum angustifolium) was investigated for the reduction of the disease symptoms and improvement of vegetative and reproductive indices in strawberries under greenhouse conditions. The results showed that alga-treated infected plants showed symptom remission. Moreover, vegetative and reproductive indices of alga-treated plants were significantly improved. Biochemical analysis showed that in alga-treated infected plants the total phenol, flavonoids, and total antioxidant activity were significantly increased compared to non-treated infected plants. Furthermore, the content of defense-related enzymes, viz. phenylalanine ammonia-lyase and polyphenol oxidase, were significantly increased in the infected plants pre-treated with the alga extract. Foliar application of S. angustifolium extract can induce defense responses in strawberry plants infected by M. phaseolina leading to improved growth indices of the plants. It can be concluded that S. angustifolium extract is a promising source of bio-stimulants for induction of disease resistance against charcoal rot disease in strawberry cultivations.
Introduction
Strawberry (Fragaria × ananassa Duch.) belonging to the family Rosaceae, sub-family Rosoidea, and the genus Fragaria, is one of the most important small fruits of temperate regions, which is widely consumed in the world (Guttridge, 2019). Strawberry is an herbaceous and perennial plant with creeping stems (stolon), which is usually propagated at the commercial level through vegetative methods (production of stolon) (Caleb et al., 2016). Due to the presence of desired conditions for strawberry cultivation, Iran is one of the major producers of strawberries in the Middle East region (Tehranifar and Sarsaefi, 2002).
Strawberry fruit is non-perishable with a limited shelf life and is highly perishable against physical damage and fungal invasion (Figueroa et al., 2008). The charcoal rot disease caused by the fungus Macrophomina phaseolina (Tassi) Goid. is one of the most devastating diseases of strawberries globally (Zveibil and Freeman, 2005; Avilés et al., 2008; Baino et al., 2011; Hutton et al., 2013; Sánchez et al., 2013; Hajlaoui et al., 2015; Baggio et al., 2019). Damage caused by strawberry charcoal rot disease has been estimated to be 15–20% of yield reduction or 3–4 million dollars per year in the states located in the southeastern United States (Baggio et al., 2019). Every year, this disease destroys 2–5% of strawberry plants in the state of Florida, and if the climatic conditions are favorable, it destroys 80% of the field (Baggio et al., 2019). In Iran, M. phaseolina has been isolated from the crown and roots of strawberry plants resembling wilting and rotting symptoms in Kurdistan, Mazandaran, and Golestan provinces (Sharifi and Mahdavi, 2012). The fungus produces resistant structures called microsclerotia that can survive for long periods in soil and strawberry debris (Zveibil et al., 2012). These microsclerotia are usually the main source of new infections and their numbers increase in susceptible hosts in the soil and grow continuously for several seasons (Baggio et al., 2019).
The use of alga as fertilizer in agriculture has been common since ancient times in the Roman Empire (Pereira and Cotas, 2019). Alga extract is more useful than chemical fertilizer due to its biodegradable, non-toxic, and environmentally friendly properties. These are the most important reasons for using alga extract in recent years for sustainable agriculture in organic and integrated agriculture (Mukherjee and Patel, 2020). In the last decade, the use of natural plant biostimulants has become widespread (Drobek et al., 2019). Induction of plant defense mechanisms using polysaccharide or oligosaccharide extracted from the alga, which have a promising protective and strategic strategy (Benhamou and Rey, 2012). Alga extract has been used directly or mixed with soil as compost, which ultimately increases soil fertility (Khan et al., 2009; Craigie, 2011). The positive effects of alga extract on improving yield and improving the resistance level of garden and agricultural plants to biotic and abiotic stresses have been reported by several researchers (Park et al., 2005; Erulan et al., 2009; Mansori et al., 2015; Shukla et al., 2017, 2018; El-Sheekh et al., 2020, 2021; Mostafa et al., 2022). It was hypothesized that the use of macroalgal extract can reduce the damage of charcoal rot disease in strawberry plants. The goal of this study was to use the extract of brown alga (Sargassum angustifolium) for the reduction of M. phaseolina effects and improvement of growth indices in strawberry plants under greenhouse conditions.
Methods
Plant material and cultivation system
The certified Strawberry plantlets, cultivar Paros, were obtained from a commercial nursery in Sanandaj (Kurdistan province, western Iran). The plantlets were transferred into cylindrical plastic pots (20 cm × 18 cm) containing sterilized soil with a texture consisted of sand (37%), silt (39%), clay (20%), and organic matter (4.0%). The soil texture class was loam. No fertilization was applied to the pots during the experiment. A uonset greenhouse (height: 3 m, diameter: 6 m), with polyethylene cover and pad & fan cooling system located in Mollasani city [longitude (λ): 48.8648334, latitude (ϕ): 31.6242601], was used. The conditions of the greenhouse (temperature: 22 ± 3°C, relative humidity: 45 ± 5%, 12-h photoperiod) were regularly checked in terms of temperature and humidity. After 30 days, the first flowers were removed for better plant growth, and then the treatments were applied. The treatments were applied before the emergence of flowers, and after the fruits reached commercial maturity, they were harvested manually, wrapped in foil, instant frozen in liquid nitrogen, and stored at −70°C for further experiments.
Preparation of alga extract
The brown alga (S. angustifolium) was collected from the shores of Chabahar, southeastern Iran (25.300278°N 60.612778°E), and transferred to the laboratory. To remove impurities, the alga was first washed with deionized distilled water (ddH2O) and impurities were removed. After air-drying for 10 days, the tissues were then ground using a grinder. To prepare the aqueous solution, 50 g of alga powder and 500 mL of ddH2O were shacked at room temperature. Then it was boiled for 60 min and filtrated. A stock solution of the extract with a concentration of 5 mg/mL was prepared and used for the assay (Sivasankari et al., 2006; Ramarajan et al., 2012).
Fungal inoculation
A virulent isolate (MP) of M. phaseolina was prepared from the Fungal Culture Collection of Shiraz University (Shiraz, Iran). Fungal microsclerotia were obtained without culture, by placing a block of agar culture containing active fungal mycelium in a flask containing potato extract and dextrose (Short and Wyllie, 1978). The flask was incubated for 3 months at room temperature until thick tangled strands of microsclerotia were formed. These thick tangled strands were removed and washed 3 times with ddH2O and dried at 35°C and then it was ground gently with a mortar. Microsclerotia was mixed with 1,000 g of sterile sand and stored at 4°C. Before inoculation, this mixture was added to the soil required to be contaminated by the pathogen (Goudarzi et al., 2011).
Treatments
The treatments were divided into four groups: control, pathogen (M. phaseolina), alga (S. angustifolium), and alga-pathogen. Control included non-treated pathogen-free plants irrigated with 180 mL of ddH2O every 2 days. In the pathogen treatment, the soil next to the crown was replaced with 1,000 g of infested sand containing 100 viable of the fungus microsclerotia/g soil that was prepared in advance, and irrigation was applied. In case of non-inoculated plants, 1,000 g of pathogen-free sterilized sand was placed around their crown. In the alga-pathogen and alga treatment, 7.0 mL the algae extract (5 mg/mL concentration) was applied as a foliar spray every 3 days, and the application of this treatment continued until the appearance of flowers, and after the appearance of flowers and fruits, irrigation was conducted. The experiment was terminated 120 days post incubation in the greenhouse. A completely randomized design with total number of 4 replications per treatment was used and the experiments were performed two times.
Evaluation of disease severity
The disease severity caused by M. phaseolina in strawberry plants was visually evaluated for the inoculated plants 92 days post inoculation. To this end, each plant was inspected and scored from 0 to 5 according the developed symptoms (Table 1). The percentage of disease severity index (DSI) was then measured using Equation (1) which has been previously described by Camara et al. (2013):
In which DSI = disease severity index; e = class; Re = number of plants in class €; N = total number of plants.
Vegetative and reproductive indices
The vegetative and reproductive growth of strawberry plants were mainly determined according to Breen and Martin (1981). Vegetative traits included plant height, root length, aerial length, fresh weight of aerial organs, and dry weight of root. Also, reproductive indices included fruit number, fruit weight, fruit length and diameter, and fruit volume. Water displacement technique was used to measure the fruit size. The indices were measured at the end of the experiment.
Total phenol
To measure the amount of total phenol in the leaf, the Folin-Cicalito reagent was used (Singleton and Rossi, 1965). For this purpose, 1,500 μL of Folin-Cicalito reagent diluted with a ratio of one to ten (1:10) was added to 300 μL of the leaf homogenate (1 g) and placed at room temperature (25°C) for 5 min. 1,200 μL of 7.5% sodium carbonate were added to the resulting mixture and placed on a shaker in a dark place for 90 min. Six mL of ddH2O were added to the solution and finally, the absorbance number at 765 nm wavelength was recorded with a spectrophotometer (made in the USA—model 2100-UV). The amount of total phenol was calculated using the standard curve of gallic acid and the results were expressed in terms of mg of gallic acid per 100 g of fresh fruit weight. To prepare gallic acid standard solutions, 0.1 g of gallic acid was dissolved in ddH2O and the final volume was brought to 100 mL using distilled water. Then, to prepare 0, 10, 20, 30, 40, 80, 160, 320, and 480 mg/100 mL standards, 0, 0.1, 0.2, 0.3, 0.4, 8 0.0, 1.6, 3.2, and 4.8 mL of gallic acid solution were removed and made up to 10 mL using ddH2O. Then, 300 μL were taken from the standards, and the rest of the steps were carried out like the samples.
Flavonoids
For extraction, one g of strawberry leaf was kept with 8 mL of 80% methanol for 12 h at 4°C. Then it was centrifuged at 8,000 g for 25 min at 4°C. To measure the flavonoids, one mL of supernatant was mixed with 0.5 mL of 5% sodium nitrite. After 6 min, 0.5 mL of 10% aluminum chloride and after 6 min, 2 mL of one molar sodium hydroxide were added to the mixture and kept for 15 min. The absorption number at 510 nm wavelength was recorded with a spectrophotometer and the results were reported in terms of mg per 100 g of fresh fruit weight (Li et al., 2014).
Total antioxidant activity
The total antioxidant activity (TAA) was determined using the free radical reduction method (diphenylpicrylhydrazyl [DPPH]) with the method of Sanchez-Moreno (2002). First, DPPH solution with a concentration of 0.1 mM was obtained by dissolving 6 mg of DPPH in 100 mL of 80% methanol. This solution was prepared daily in a dark container to measure the inhibition percentage. To prepare the alcoholic extract, 0.3 g of leaf sample was pounded with 3 mL of solvent in the dark and shaken at 100°C for 30 min. After that, it was centrifuged for 10 min at 4°C at 5,000 rpm and the supernatant extract was used to measure TAA. For this purpose, 75 μL of the alcoholic extract was taken and 2,925 μL of DPPH solution was added to it. The samples were placed in the dark chamber for 30 min. The absorbance of the samples was measured at a wavelength of 517 nm (A517) using a spectrophotometer. The control sample was read using DPPH solution without adding the extract. Finally, using Equation (2), the inhibition percentage of the samples was calculated:
In which CA is the absorbance of the control and SA is the absorbance of the sample.
Polyphenol oxidase activity
To measure the activity of polyphenol oxidase (PPO) according to the proposed method (Worthington, 1988), one mL of 50 mM sodium phosphate buffer, one mL of 1 mM tyrosine, and 900 μL of ddH2O were placed into the cuvette and 100 μL of enzyme extract was added to the cuvette. It was added and the increase in absorbance at 280 nm wavelength was recorded using a spectrophotometer at 2, 4, 6, 8, 10, and 12 min. By placing the absorbance changes (the difference between the highest and the lowest number read during 12 min, which is linear) in Equation (3), the amount of enzyme activity was calculated in mg/g/min.
Phenylalanine ammonia-lyase activity
Phenylalanine ammonia-lyase (PAL) activity was measured using the method of Zucker (1968). First, one g of leaf tissue was crushed with 5 mL of sodium borate buffer [pH 8.8]. Then the prepared solution was centrifuged at 12,000 rpm for 10 min. Five hundred microliter of the centrifuged extract, two mL of sodium borate buffer with 500 μL of 20 mM phenylalanine solution were poured into a tube and placed in a hot water bath for one h at 37°C was placed. Enzyme activity was determined by measuring the absorbance of the solution at a wavelength of 290 nm for one h with an interval of 15 min and was reported as units per mg of enzyme extract protein (U/mg protein).
Data analysis
All data collected during the experiment were transferred into Excel software and prepared for statistical analysis. Data analysis was performed using SAS software (v. 9.4) (Yuan, 2011). The statistical design used in this study was a completely randomized design with 4 replications and the means were compared based on Duncan's multiple range test with an alpha error level of 5%. In the case of biochemical experiments, technical (sample) and biological (plant) replications were considered per treatment. To analyze the data from disease severity index, Kruskal–Wallis H test was used and the significance at P= 0.01 level was determined.
Results
Disease severity
The symptoms of the disease including blackening of root and crown, reduction of leaf area, leaf necrosis, reduction of fruit size, and decreased number of fruits were observed on M. phaseolina-inoculated plants 92 days post inoculation (dpi) (Figure 1a). Strawberry plants inoculated with the fungus were treated with the alga extract. In these plants, there are fewer symptoms compared to the non-treated plants (Figure 1b). Healthy strawberry plants treated with the alga extract showed improved vegetative growth (Figure 1c). Figure 1D shows the comparison of strawberry fruits among different treatments at 92 dpi. Accordingly, in plants treated with the alga extract, the size of the fruits was larger than those of non-treated plants (Figure 1d). In contrast, the infected plants which had not been treated with the alga extract produced the smallest fruits. Also, infected plants treated with the alga extract produced larger fruits compared to infected non-treated plants. Evaluation of disease severity showed that alga-treated infected plants had a significantly lower disease severity index (20%) than non-treated infected plants (80%). These results showed that foliar application of S. angustifolium extract to strawberry plants can reduce the disease severity caused by M. phaseolina.
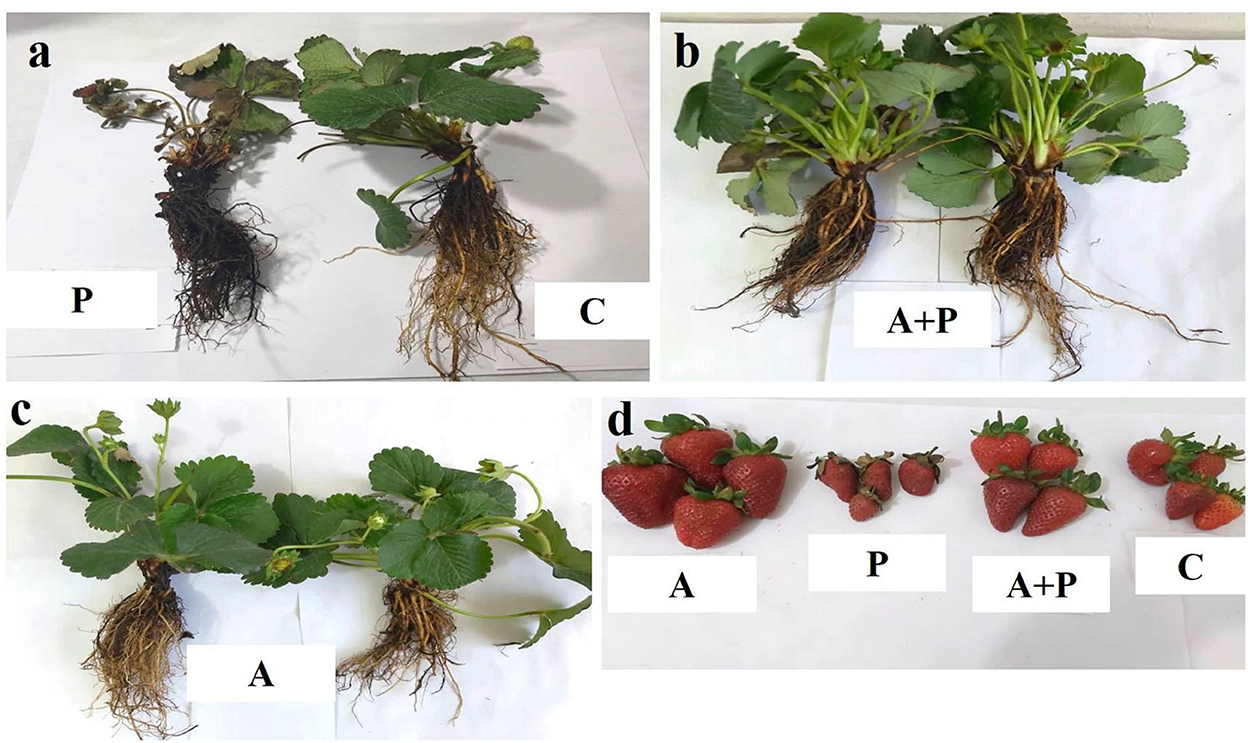
Figure 1. Phenotypic responses of strawberry plant to the fungal pathogen (M. phaseolina) among different treatments 92 days post inoculation. (a) Symptoms of charcoal rot in the pathogen-inoculated plant (P) compared to non-inoculated plant (C); (b) vegetative growth of infected plants pre-treated with the alga (S. angustifolium) extract (A + P); (c) vegetative growth of non-inoculated plants pre-treated with the alga extract (A); (d) comparison of fruits from plants under the different treatments.
Vegetative indices
Statistical analysis of the data from the effect of different treatments on strawberry plant length showed that the highest plant length (29.75 cm) was related to the plants under alga extract treatment, while the lowest plant length was found in non-treated infected plants (17 cm) (Table 2). Also, the length of the plant treated with alga extract was significantly higher than the other treatments, and the length of the infected plants was significantly lower than other treatments. The difference between the length values of non-infected alga-treated plants and infected alga-treated plants was not significant.
Similarly, the highest root length (18 cm) was found in non-infected alga-treated plants, while non-treated infected plants showed the lowest root length (8 cm) (Table 2). The root length of infected alga-treated plants was significantly higher than infected non-treated plants.
The highest value of aerial length (14 cm) was recorded for infected alga-treated plants (Table 2). The second highest value of aerial length (12 cm) belonged to non-inoculated alga treatment plants. The remaining plants did not show any significant difference in aerial length.
The non-inoculated alga-treated plants showed the highest fresh weight of the aerial organs (12.025 g), while non-treated infected plants exhibited the lowest fresh weight of aerial organs (5.84 g) (Table 2). Furthermore, this index in infected alga-treated plants was significantly higher than in non-treated infected plants.
The dry weight of roots in control plants was determined as the highest value (10.97 g), while non-treated infected plants showed the lowest value (5.58 g) (Table 2). Also, this index in alga-treated infected plants was significantly higher than in non-treated infected plants. The difference between the dry weight of roots of non-infected alga-treated plants and infected alga-treated plants was not significant.
Reproductive indices
Table 3 shows the effect of alga treatment on infected and non-infected strawberry plants. The mean number of fruits (5.50) in the alga-treated plants was significantly larger than that of other plants. Also, the significantly smallest mean number of fruits (3.00) was found in the non-treated infected plants compared to other treatments. Similarly, the highest value of fruit weight (8.86 g) and fruit volume (10.37 g/mL) were observed in alga-treated plants. These indices in non-treated infected plants were significantly lower than in plants under other treatments. Also, the highest fruit diameter was found in alga-treated plants and alga-treated infected plants (16.87 mm and 16.56 mm, respectively). The non-treated infected plants showed the lowest fruit diameter (12.5 mm) compared to other plants. The highest fruit length (27.287 mm) belonged to the alga-treated plants. The non-treated infected plants showed the lowest fruit diameter (12.50 mm) compared to plants under other treatments. There was no significant difference between the fruit diameter of alga-treated infected plants and control plants.
Biochemical factors
The results showed that alga-treated plants had a significant effect on the total phenol, flavonoids, and TAA of strawberries (Figure 2). Alga-treated infected plants had significantly more phenol content (1.60 mg/g) and flavonoids (1.44 mg/g) than plants under other treatments (Figures 2A,B). The lowest phenol content and flavonoids were found in control plants (0.93 mg/g and 0.51 mg/g, respectively). Phenol content and flavonoids were not significantly different between alga-treated and non-treated infected plants (Figures 2A,B). The highest level of TAA (0.2) was recorded for alga-treated infected plants (Figure 2C). In contrast, non-treated infected plants showed the lowest level of TAA (0.06).
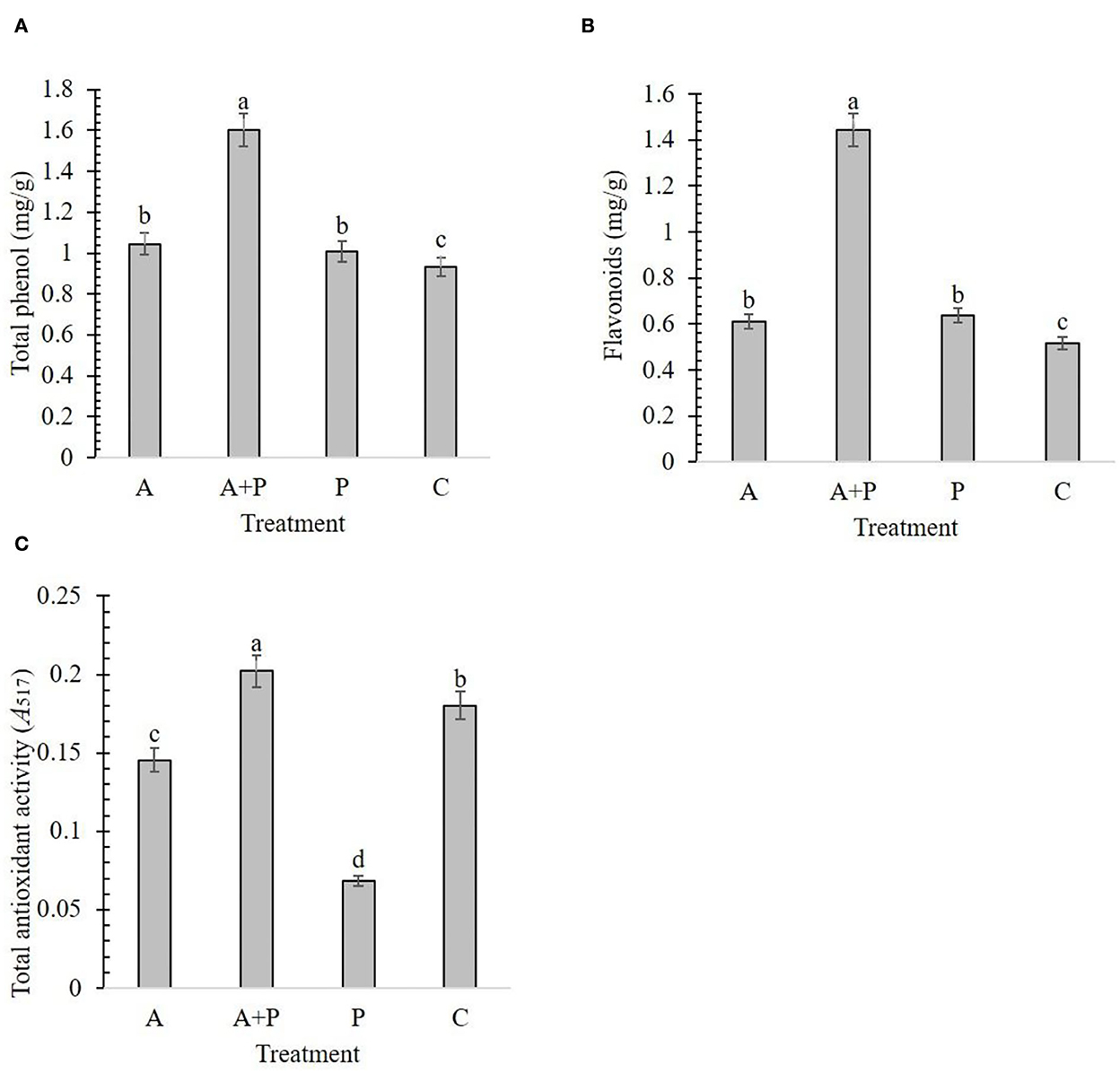
Figure 2. Effect of different treatments [A, alga (S. angustifolium) extract; A + P, pathogen (M. phaseolina)-inoculated pre-treated with the alga extract; P, pathogen-inoculated; C, control] and on some biochemical factors of the strawberry plant including total phenol (A), flavonoids (B), and total antioxidant activity (C). Letters on bars show the significant difference between the treatments according to the results of Duncan's multiple range test.
Enzyme activity
The results from the effect of different treatments on the PAL content in strawberry plants showed that the alga-treated infected plants had the highest enzyme content (0.89 U/mg) found while the lowest amount of PAL enzyme was recorded for alga-treated non-infected plants (0.54 U/mg) (Figure 3A). The content of the PAL enzyme was not significantly different between non-treated infected and control plants (Figure 3A).
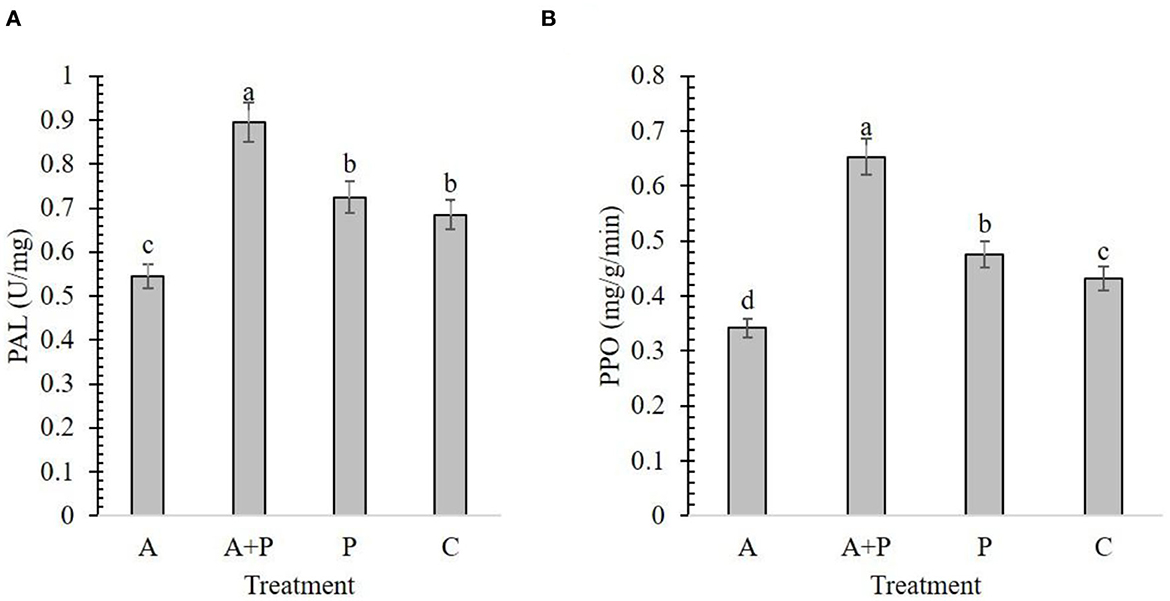
Figure 3. Effect of different treatments [A, alga (S. angustifolium) extract; A + P, pathogen (M. phaseolina)-inoculated pre-treated with the alga extract; P, pathogen-inoculated; C, control] and on the activity of strawberry enzymes including PAL (A) and PPO (B). Letters on bars show the significant difference between the treatments according to the results of Duncan's multiple range test.
In the alga-treated infected plants, the highest amount (0.65 mg/g/min) of PPO enzyme was found while the lowest value was determined in the alga-treated non-infected plants (0.34 mg/g/min) (Figure 3B). These results showed that foliar application of alga extract can significantly increase the content of PAL and PPO in infected plants.
Discussion
Algae affect crops to increase plant growth, plantlet growth, and secondary root growth. They can also improve nutrient composition, fruit set, pest and disease resistance, improve stress response (drought, salinity, and temperature) (Mukherjee and Patel, 2020). It has been shown that foliar spray of alga extract increases the absorption of nutrients, promoting growth and root development in various crops, such as corn (Jeannin et al., 1991), tomato (Crouch and van Staden, 1992), Arabidopsis (Rayorath et al., 2008), grape (Mugnai et al., 2008), strawberry (Alam et al., 2013), spinach (Fan et al., 2013), okra (Zodape et al., 2008), olive (Chouliaras et al., 2009) and broccoli (Mattner et al., 2013). It has also increased the consumption of nutrients such as nitrogen, phosphorus, potassium, calcium, sulfur, and micronutrients such as magnesium, zinc, manganese, and iron (Crouch and van Staden, 1992; Mancuso et al., 2006; Rathore et al., 2009; Zodape et al., 2011). Similarly, our results showed that the foliar application of a brown alga improved the vegetative indices of strawberry plants including height, root length and fresh weight of aerial organs (Table 2). Additionally, strawberry fruit indices including number, weight, diameter and length showed a significant increase in alga-treated plants (Table 3) demonstrating the positive effect of macroalgal treatment on growth and yield of strawberry plants. Although the positive effect of an alga (Ascophyllum sp.) extract on strawberry growth has been reported previously by Alam et al. (2013), here we reported a new alga species (S. angustifolium) with a beneficial effect. The antifungal activity of seaweeds has been reported against phytopathogenic fungi including Fusarium oxysporum (El-Sheekh et al., 2020), F. solani (El-Sheekh et al., 2021) and F. oxysporum f. sp. lycopersici (Mostafa et al., 2022). Particularly, El-Sheekh et al. (2021) demonstrated that the mycelial growth of M. phaseolina was inhibited when cucumber plants were treated with the extract of green seaweeds, Ulva fasciata, and Enteromorpha flexuosa. Herein, however, the anti-fungal effect of brown alga, S. angustifolium, was shown indirectly as foliar treatment of the algal extract could reduce the disease severity caused by M. phaseolina in strawberry plants. Marine algae metabolites contain bioactive molecules with anti-fungal, anti-viral, anti-bacterial, and anti-protozoal properties. Usually, metabolites isolated from brown, red, and green algae are stronger than antimicrobial chemicals (Ben Salah et al., 2018). In our experiment, the brown alga and the pathogen were applied in different sites (shoot and crown, respectively), therefore, the reduced disease severity of M. phaseolina in alga-treated strawberry plants is not probably due to the anti-fungi metabolites within S. angustifolium extract. Alternatively, induced resistance might be the main mechanism by which the alga-treated strawberry plants encounter M. phaseolina invasion.
Moreover, algae are a source of stimulants due to the presence of several different polysaccharide compounds. These polysaccharides are involved in primary signaling processes through the activation of plant secondary metabolic pathways and the mobilization of messenger molecules to activate the defense response in the host plant (Paulert et al., 2009; Sharma et al., 2014). Alga polysaccharides make plants resistant to plant pathogens (Mercier et al., 2001; Sangha et al., 2015). The increase in total phenol and flavonoids are general defense responses against biotic agents such as plant pathogens (Wallis and Galarneau, 2020). Similarly, our results demonstrated that foliar application of S. angustifolium extract can significantly increase total phenol and flavonoids within strawberry plants (Figure 2) which probably contributes to induced resistance against M. phaseolina leading to symptom remission of infected plants (Figure 1). Generally, free radicals are produced as a defensive response to pathogen infection in plant cells which trigger the systemic acquired resistance (SAR) within plant (Agrios, 2005; Wendehenne et al., 2014). The antioxidant activity, however, suppress the oxidation by scavenging the free radicals (Larson, 1995). In our experiment, the alga-treated infected plants exhibited a significant increase in total antioxidant activity (Figure 2C) suggesting that a non-SAR pathway, i.e., induced systemic resistance (ISR) (Heil and Bostock, 2002), is involved in strawberry plant defense against the fungal pathogen. Enzymes such as PAL and PPO are major biomolecules that have a key role in the induced resistance of plants challenged by a pathogen (Ngadze et al., 2012). Herein, the alga-treated infected plants showed a significant increase in the content of two defense-related enzymes, viz. PAL and PPO (Figure 3), suggesting the role of induced resistance in the reduction of disease severity caused by M. phaseolina. Taken together, foliar application of S. angustifolium extract can elicit the defense responses of strawberry plants challenged by M. phaseolina leading to improved growth indices of the plants. Further experiments are required to examine the other application ways to find the optimum method for the alga usage in strawberry greenhouses facing charcoal rot disease.
Conclusions
Algal metabolites are a valuable source of nutrients and elicitors for the improvement of plant growth and encountering biotic agents. Brown alga (S. angustifolium) is one of the beneficial macroalgae that has been used in disease management programs. Foliar application of S. angustifolium extract can improve both vegetative and reproductive indices of strawberry plants. Furthermore, it can reduce the charcoal rot severity caused by M. phaseolina through an increase in the level of total phenol, flavonoids, TAA, and defense-related enzymes (PAL and PPO) within the plants. It is recommended that the macroalga is used against charcoal rot disease in strawberry greenhouses.
Data availability statement
The raw data supporting the conclusions of this article will be made available by the authors, without undue reservation.
Author contributions
ST mainly conducted this work, performed the experiments, and collected the data. MR-J conceived the study. MG analyzed the data. MR-J and MG mainly wrote the manuscript. All authors edited and approved the final version of the manuscript.
Funding
This study was funded by the Agricultural Sciences and Natural Resources University of Khuzestan.
Acknowledgments
The authors appreciate the staff of Central Laboratory in Agricultural Sciences and Natural Resources University, for their technical assistance.
Conflict of interest
The authors declare that the research was conducted in the absence of any commercial or financial relationships that could be construed as a potential conflict of interest.
Publisher's note
All claims expressed in this article are solely those of the authors and do not necessarily represent those of their affiliated organizations, or those of the publisher, the editors and the reviewers. Any product that may be evaluated in this article, or claim that may be made by its manufacturer, is not guaranteed or endorsed by the publisher.
Abbreviations
TAA, Total antioxidant activity; PAL, Phenylalanine ammonia-lyase; PPO, Polyphenol oxidase activity; S. angustifolium, Sargassum angustifolium; M. phaseolina, Macrophomina phaseolina; °C, Celsius Degree; g, Gram; cm, Centimeter; mm, Millimeter; mg, Milligram; min, Minute; h, Hour; μL, Microliter; l, Liter; ddH2O, Deionized distilled water; mL, Milliliter; g, Gravity; rpm, Centrifugal rotation speed; DPPH, Diphenylpicrylhydrazyl; mM, Millimolar; nm, Nanometer; A, Absorbance; CA, Control absorbance; SA, Sample absorbance; U, Unite; dpi, Days post inoculation; C, Control; P, Pathogen; A, Alga.
References
Alam, M. Z., Braun, G., Norrie, J., and Hodges, D. M. (2013). Effect of Ascophyllum extract application on plant growth, fruit yield and soil microbial communities of strawberry. Can. J. Plant. Sci. 93, 23–36. doi: 10.4141/cjps2011-260
Avilés, M., Castillo, S., Bascon, J., Zea-Bonilla, T., Martín-Sánchez, P. M., and Pérez-Jiménez, R. M. (2008). First report of Macrophomina phaseolina causing crown and root rot of strawberry in Spain. Plant Pathol. 57, 382–382. doi: 10.1111/j.1365-3059.2007.01717.x
Baggio, J. S., Cordova, L. G., and Peres, N. A. (2019). Sources of inoculum and survival of Macrophomina phaseolina in Florida strawberry fields. Plant Dis. 103, 2417–2424. doi: 10.1094/PDIS-03-19-0510-RE
Baino, O. M., Salazar, S. M., Ramallo, A. C., and Kirschbaum, D. S. (2011). First report of Macrophomina phaseolina causing strawberry crown and root rot in northwestern Argentina. Plant Dis. 95, 1477–1477. doi: 10.1094/PDIS-03-11-0193
Ben Salah, I., Aghrouss, S., Douira, A., Aissam, S., El Alaoui-Talibi, Z., Filali-Maltouf, A., et al. (2018). Seaweed polysaccharides as bio-elicitors of natural defenses in olive trees against Verticillium wilt of olive. J. Plant Interact. 13, 248–255. doi: 10.1080/17429145.2018.1471528
Benhamou, N., and Rey, P. (2012). Stimulators of natural plant defenses: a new phytosanitary strategy in the context of sustainable ecoproduction: II. Interest of the SND in crop protection. Phytoprotection 92, 24–35. doi: 10.7202/1013299ar
Breen, P. J., and Martin, L. W. (1981). Vegetative and reproductive growth responses of three strawberry cultivars to nitrogen. J. Amer. Soc. Hort. Sci. 106, 266–272. doi: 10.21273/JASHS.106.3.266
Caleb, O. J., Wegner, G., Rolleczek, C., Herppich, W. B., Geyer, M., and Mahajan, P. V. (2016). Hot water dipping: impact on postharvest quality, individual sugars, and bioactive compounds during storage of ‘Sonata’ strawberry. Sci. Hortic. 210, 150–157. doi: 10.1016/j.scienta.2016.07.021
Camara, M., Mbaye, A. A., Noba, K., Samb, P. I., Diao, S., and Cilas, C. (2013). Field screening of tomato genotypes for resistance to tomato yellow leaf curl virus (TYLCV) disease in Senegal. Crop Prot. 44, 59–65. doi: 10.1016/j.cropro.2012.10.007
Chouliaras, V., Tasioula, M., Chatzissavvidis, C., Therios, I., and Tsabolatidou, E. (2009). The effects of a seaweed extract in addition to nitrogen and boron fertilization on productivity, fruit maturation, leaf nutritional status and oil quality of the olive (Olea europaea L.) cultivar Koroneiki. J. Sci. Food Agric. 89, 984–988. doi: 10.1002/jsfa.3543
Craigie, J. S. (2011). Seaweed extract stimuli in plant science and agriculture. J. Appl. Phycol. 23, 371–393. doi: 10.1007/s10811-010-9560-4
Crouch, I. J., and van Staden, J. (1992). Effect of seaweed concentrate on the establishment and yield of greenhouse tomato plants. J. Appl. Phycol. 4, 291–296. doi: 10.1007/BF02185785
Drobek, M., Frac, M., and Cybulska, J. (2019). Plant biostimulants: Importance of the quality and yield of horticultural crops and the improvement of plant tolerance to abiotic stress—a review. Agronomy 9, 335. doi: 10.3390/agronomy9060335
El-Sheekh, M. M., Ahmed, A. Y., Soliman, A. S., Abdel-Ghafour, S. E., and Sobhy, H. M. (2021). Biological control of soil borne cucumber diseases using green marine macroalgae. Egypt. J. Biol. Pest Control 31, 1–7. doi: 10.1186/s41938-021-00421-6
El-Sheekh, M. M., Mousa, A. S. H., and Farghl, A. A. (2020). Biological control of Fusarium wilt disease of tomato plants using seaweed extracts. Arab. J. Sci. Eng. 45, 4557–4570. doi: 10.1007/s13369-020-04518-2
Erulan, V., Soundarapandian, P., Thirumaran, G., and Ananthan, G. (2009). Studies on the effect of Sargassum polycystum (C, Agardh 1824) extract on the growth and biochemical composition of Cajanus cajan (L.) Mill sp. Am. Eurasian J. Agric. Environ. Sci. 6, 392–399.
Fan, D., Hodges, D. M., Critchley, A. T., and Prithiviraj, B. (2013). A commercial extract of brown macroagla (Ascophyllum nodosum) affects yield and the nutritional quality of spinach in vitro. Commun. Soil. Sci. Plant Anal. 44, 1873–1884. doi: 10.1080/00103624.2013.790404
Figueroa, C. R., Pimentel, P., Gaete-Eastman, C., Moya, M., Herrera, R., Caligari, P. D., et al. (2008). Softening rate of the Chilean strawberry (Fragaria chiloensis) fruit reflects the expression of polygalacturonase and pectate lyase genes. Postharvest Biol. Technol. 49, 210–220. doi: 10.1016/j.postharvbio.2008.01.018
Goudarzi, A., Banihashemi, Z., and Maftoun, M. (2011). Effect of salt and water stress on root infection by Macrophomina phaseolina and ion composition in shoot in sorghum. Iran. J. Plant Pathol. 47, 69–83.
Guttridge, C. G. (2019). “Fragaria × ananassa: En. Strawberry; Fr. Fraise; Ge. Erdbeere; Sp. Fresón,” in CRC Handbook of Flowering, ed A. H. Halevy (Boca Raton, FL: CRC Press), 16–33.
Hajlaoui, M. R., Mnari-Hattab, M., Sayeh, M., Zarrouk, I., Jemmali, A., and Koike, S. T. (2015). First report of Macrophomina phaseolina causing charcoal rot of strawberry in Tunisia. New Dis. Rep. 32, 2044–20588. doi: 10.5197/j.2044-0588.2015.032.014
Heil, M., and Bostock, R. M. (2002). Induced systemic resistance (ISR) against pathogens in the context of induced plant defences. Ann. Bot. 89, 5032512. doi: 10.1093/aob/mcf076
Hutton, D. G., Gomez, A. O., and Mattner, S. W. (2013). Macrophomina phaseolina and its association with strawberry crown rot in Australia. Int. J. Fruit Sci. 13, 1492155. doi: 10.1080/15538362.2012.698143
Jeannin, I., Lescure, J. C., and Morot-Gaudry, J. F. (1991). The effects of aqueous seaweed sprays on the growth of maize. Bot. Mar. 334, 469–473. doi: 10.1515/botm.1991.34.6.469
Khan, W., Rayirath, U. P., Subramanian, S., Jithesh, M. N, Rayorath, P., Mark Hodges, D., et al. (2009). Seaweed extracts as bio-stimulants of plant growth and development. J. Plant Growth Regul. 28, 386–399. doi: 10.1007/s00344-009-9103-x
Larson, R. A. (1995). Plant defenses against oxidative stress. Arch. Insect Biochem. Physiol. 29, 175–186. doi: 10.1002/arch.940290207
Li, D., Luo, Z., Mou, W., Wang, Y., Ying, T., and Mao, L. (2014). ABA and UV-C effects on quality, antioxidant capacity and anthocyanin contents of strawberry fruit (Fragaria ananassa Duch.). Postharvest Biol. Technol. 90, 56–62. doi: 10.1016/j.postharvbio.2013.12.006
Mancuso, S., Azzarello, E., Mugnai, S., and Briand, X. (2006). Marine bioactive substances (IPA extract) improve foliar ion uptake and water stress tolerance in potted Vitis vinifera plants. Adv. Hortic. Sci. 20, 156–161. doi: 10.1400/53262
Mansori, M., Chernane, H., Latique, S., Benaliat, A., Hsissou, D., and El Kaoua, M. (2015). Seaweed extract effect on water deficit and antioxidative mechanisms in bean plants (Phaseolus vulgaris L.). J. Appl. Phycol. 27, 1689–1698. doi: 10.1007/s10811-014-0455-7
Mattner, S. W., Wite, D., Riches, D. A., Porter, I. J., and Arioli, T. (2013). The effect of kelp extract on seedling establishment of broccoli on contrasting soil types in southern Victoria. Aust. Biol. Agric. Hortic. 29, 258–270. doi: 10.1080/01448765.2013.830276
Mercier, L., Laftte, C., Borderies, G., Briand, X., Esquerré-Tugayé, M. T., and Fournier, J. (2001). The algal polysaccharide carrageenans can act as an elicitor of plant defence. New Phytol. 149, 43–51. doi: 10.1046/j.1469-8137.2001.00011.x
Mostafa, Y. S., Alamri, S. A., Alrumman, S. A., Hashem, M., Taher, M. A., and Baka, Z. A. (2022). In vitro and in vivo biocontrol of tomato Fusarium wilt by extracts from brown, red, and green macroalgae. Agriculture 12, 345. doi: 10.3390/agriculture12030345
Mugnai, S., Azzarello, E., Pandolf, C., Salamagne, S., Briand, X., and Mancuso, S. (2008). Enhancement of ammonium and potassium root infuxes by the application of marine bioactive substances positively affects Vitis vinifera plant growth. J. Appl. Phycol. 20, 177–182. doi: 10.1007/s10811-007-9203-6
Mukherjee, A., and Patel, J. S. (2020). Seaweed extract: biostimulator of plant defense and plant productivity. Int. J. Environ. Sci. Technol. 17, 553–558. doi: 10.1007/s13762-019-02442-z
Ngadze, E., Icishahayo, D., Coutinho, T. A., and Van der Waals, J. E. (2012). Role of polyphenol oxidase, peroxidase, phenylalanine ammonia lyase, chlorogenic acid, and total soluble phenols in resistance of potatoes to soft rot. Plant Dis. 96, 186–192. doi: 10.1094/PDIS-02-11-0149
Park, P. J., Heo, S. J., Park, E. J., Kim, S. K., Byun, H. G., Jeon, B. T., et al. (2005). Reactive oxygen scavenging effect of enzymatic extracts from Sargassum thunbergii. J. Agric. Food. Chem. 53, 6666–6672. doi: 10.1021/jf050582+
Paulert, R., Talamini, V., Cassolato, J. E. F., Duarte, M. E. R., Noseda, M. D., Smania, A., et al. (2009). Effects of sulfated polysaccharide and alcoholic extracts from green alga Ulva fasciata on anthracnose severity and growth of common bean (Phaseolus vulgaris L.). J. Plant Dis. Prot. 116, 263–270. doi: 10.1007/BF03356321
Pereira, L., and Cotas, J. (2019). “Historical use of seaweed as an agricultural fertilizer in the European Atlantic area,” in Seaweeds as Plant Fertilizer, Agricultural Biostimulants and Animal Fodder, eds K. Bahcevandziev and L. Pereira, (Boca Raton, FL: CRC Press), 1–22. doi: 10.1201/9780429487156-1
Ramarajan, S., Joseph, L. H., and Ganthi, A. S. (2012). Effect of seaweed liquid fertilizer on the germination and pigment concentration of soybean. J. Crop Sci. Technol. 1, 1–5.
Rathore, S. S., Chaudhary, D. R., and Boricha, G. N. (2009). Effect of seaweed extract on the growth, yield and nutrient uptake of soybean (Glycine max) under rainfed conditions. S. Afr. J. Bot. 75, 351–355. doi: 10.1016/j.sajb.2008.10.009
Rayorath, P., Jithesh, M. N., Farid, A., Khan, W., Palanisamy, R., Hankins, S. D., et al. (2008). Rapid bioassays to evaluate the plant growth promoting activity of Ascophyllum nodosum (L.) Le Jol. using a model plant, Arabidopsis thaliana (L.) Heynh. J. Appl. Phycol. 20, 423–429. doi: 10.1007/s10811-007-9280-6
Sánchez, S., Gambardella, M., Henríquez, J. L., and Díaz, I. (2013). First report of crown rot of strawberry caused by Macrophomina phaseolina in Chile. Plant Dis. 97, 996–996. doi: 10.1094/PDIS-12-12-1121-PDN
Sanchez-Moreno, C. (2002). Methods used to evaluate the free radical scavenging activity in food and biological systems. Food Sc. Technol. 8, 121–137. doi: 10.1177/1082013202008003770
Sangha, J. S., Kandasamy, S., Khan, W., Bahia, N. S., Singh, R. P., Critchley, A. T., et al. (2015). λ-carrageenan suppresses tomato chlorotic dwarf viroid (TCDVd) replication and symptom expression in tomatoes. Mar. Drugs 13, 2875–2889. doi: 10.3390/md13052875
Sharifi, K., and Mahdavi, M. (2012). First report of strawberry crown and root rot caused by Macrophomina phaseolina in Iran. Iran. J. Plant Pathol. 47, 279–480.
Sharma, H. S., Fleming, C., Selby, C., Rao, J. R., and Martin, T. (2014). Plant biostimulants: a review on the processing of macroalgae and use of extracts for crop management to reduce abiotic and biotic stresses. J. Appl. Phycol. 26, 465–490. doi: 10.1007/s10811-013-0101-9
Short, G. E., and Wyllie, T. D. (1978). Inoculum potential of Macrophomina phaseolina. Phytopathology 68, 742–746. doi: 10.1094/Phyto-68-742
Shukla, P. S., Borza, T., Critchley, A. T., Hiltz, D., Norrie, J., and Prithiviraj, B. (2018). Ascophyllum nodosum extract mitigates salinity stress in Arabidopsis thaliana by modulating the expression of miRNA involved in stress tolerance and nutrient acquisition. PLoS ONE 13, 206–221. doi: 10.1371/journal.pone.0206221
Shukla, P. S., Shotton, K., Norman, E., Neily, W., Critchley, A. T., and Prithiviraj, B. (2017). Seaweed extract improve drought tolerance of soybean by regulating stress-response genes. AoB Plants 10, plx051. doi: 10.1093/aobpla/plx051
Singleton, V. L., and Rossi, J. A. (1965). Colorimetry of total phenolics with phosphomolybdic–phosphotungstic acid reagents. Am. J. Enol. Vitic. 16, 144–158.
Sivasankari, S., Venkatesalu, V., Anantharaj, M., and Chandrasekaran, M. (2006). Effect of seaweed extracts on the growth and biochemical constituents of Vigna sinensis. Bioresour. Technol. 97, 1745–1751. doi: 10.1016/j.biortech.2005.06.016
Tehranifar, A., and Sarsaefi, M. (2002). Strawberry growing in Iran. Acta Hort. 567, 547–549. doi: 10.17660/ActaHortic.2002.567.118
Wallis, C. M., and Galarneau, E. R. A. (2020). Phenolic compound induction in plant-microbe and plant-insect interactions: a meta-analysis. Front. Plant Sci. 11, 580753. doi: 10.3389/fpls.2020.580753
Wendehenne, D., Gao, Q. M., Kachroo, A., and Kachroo, P. (2014). Free radical-mediated systemic immunity in plants. Curr. Opin. Plant Biol. 20, 127–134. doi: 10.1016/j.pbi.2014.05.012
Worthington, C. C. (1988). Worthington Enzyme Manual: Enzymes and Related Biochemicals. Lakewood, NJ: Worthington Biochemical Corporation.
Yuan, Y. (2011). Multiple imputation using SAS software. J. Stat. Softw. 45, 1–25. doi: 10.18637/jss.v045.i01
Zodape, S. T., Gupta, A., Bhandari, S. C., Rawat, U. S., Chaudhary, D. R., Eswaran, K., et al. (2011). Foliar application of seaweed sap as bio stimulant for enhancement of yield and quality of tomato (Lycopersicon esculentum Mill.). J. Sci. Ind. Res. 70, 215–219.
Zodape, S. T., Kawarkhe, V. J., Patolia, J. S., and Warade, A. D. (2008). Effect of liquid seaweed fertilizer on yield and quality of okra (Abelmoschus esculentus L.). J. Sci. Ind. Res. 67, 1115–1117.
Zucker, M. (1968). Sequential induction of phenylalanine ammonia-lyase and a lyase-inactivating system in potato tuber disks. Plant Physiol. 43, 365–374. doi: 10.1104/pp.43.3.365
Zveibil, A., and Freeman, S. (2005). First report of crown and root rot in strawberry caused by Macrophomina phaseolina in Israel. Plant Dis. 89, 1014–1014. doi: 10.1094/PD-89-1014C
Keywords: seaweed extract, charcoal rot disease, induced resistance, growth index, greenhouse cultivation system
Citation: Tajdinian S, Rahmati-Joneidabad M and Ghodoum Parizipour MH (2022) Macroalgal treatment to alleviate the strawberry yield loss caused by Macrophomina phaseolina (Tassi) Goid. in greenhouse cultivation system. Front. Sustain. Food Syst. 6:1089553. doi: 10.3389/fsufs.2022.1089553
Received: 04 November 2022; Accepted: 05 December 2022;
Published: 20 December 2022.
Edited by:
Abdelilah Meddich, Cadi Ayyad University, MoroccoReviewed by:
Mohamed Anli, Cadi Ayyad University, MoroccoEl Kaoua Mimoun, Cadi Ayyad University, Morocco
Copyright © 2022 Tajdinian, Rahmati-Joneidabad and Ghodoum Parizipour. This is an open-access article distributed under the terms of the Creative Commons Attribution License (CC BY). The use, distribution or reproduction in other forums is permitted, provided the original author(s) and the copyright owner(s) are credited and that the original publication in this journal is cited, in accordance with accepted academic practice. No use, distribution or reproduction is permitted which does not comply with these terms.
*Correspondence: Mohamad Hamed Ghodoum Parizipour, cGFyaXppQGFzbnJ1a2guYWMuaXI=