- 1Agroindustry Department, Agroindustrial Engineering Program, Research Group of Aprovechamiento de Subproductos Agroindustrial (ASUBAGROIN), Universidad del Cauca, Popayán, Colombia
- 2Agroindustry Department, Agroindustrial Engineering Program, Research Group of Ciencia y Tecnología de Biomoleculas de Interés Agroindustrial (CYTBIA), Universidad del Cauca, Popayán, Colombia
- 3School of Food Engineering, Faculty of Engineering, Research Group of Ingeniería de Procesos Agroalimentarios y Biotecnológicos (GIPAB), Universidad del Valle, Cali, Colombia
- 4Food Engineering Department, Food Packaging and Shelf Life Research Group (FP&SL), Universidad de Cartagena, Cartagena de Indias, Colombia
Introduction: The food industries play a fundamental role in feeding for the functions of animal metabolism. Fish feed extrusion cooking includes process-independent factors such as temperature (°C), screw speed (RPM), throughput, feed, and moisture content that influence the final product's nutritional value and physical properties. The evidence suggests that the application of hydrolyzed protein flour (HPH) is a crucial step for the techno-functional properties of the product. Therefore, this work aimed to study the effect of hydrolyzed protein meal from silage of trout (Oncorhynchus mykiss) on the parameters of the extrusion system and their physical transformations.
Methods: In this study, the influence of hydrolyzed protein meals ranges between 10 and 30% as a substitute for fish meals. The physical properties of the extrudate were monitored, evaluating the hardness, durability, buoyancy, expansion index, and apparent density.
Results: Consistent with this, parameters such as feed composition, screw speed, moisture content, and extrusion process affected the composition and properties of the final product.
Discussion: The physical properties indicated that the hydrolyzed protein flour presented cohesiveness and decreased the mean retention time in the extruder barrel and the specific mechanical energy (SME). Hydrolyzed protein flour during the extrusion process produces pellets with high durability and low hardness due to the high porosity presented, which allows for obtaining nutritional characteristics in the extruded product.
1. Introduction
Aquaculture (the farming of aquatic animals and plants) is a food activity that is dominating the world with a total production of more than 18.32 million tons (Tacon, 2020). Therefore, this sector requires quality food products for consumption with productive parameters. One of the critical sectors of aquaculture is the production of fish, such as trout, which requires considerable amounts of feed at a high cost. In this context, it is necessary to develop alternatives to replace commercial fish feed at least partially. One such alternative is fish silage. This type of fish food has certain physical properties, among which are hardness, durability, porosity, buoyancy, expansion rate, density, water absorption, and water solubility (Thomas and van der Poel, 1996; Cruz-Suárez et al., 2006; Chaabani et al., 2022). The physical quality tests of the diets must be conducted over some time, mainly if new ingredients, methodologies, or processing conditions are studied for the standardization of the product (Sørensen, 2012). In addition to nutritional aspects, fish feed has three main components within the pelleted feed, which are starch, lipids and protein of animal and/or vegetable origin, where their interaction influences the physical characteristics (Chen et al., 2010; Chaabani et al., 2022), such as porosity, good absorption, and a stable structure during storage, transport, and pneumatic feeding of the product (Aarseth, 2004; Aarseth et al., 2006; Draganovic et al., 2011).
Extrusion cooking of fish feed is a process that involves several variables and factors, both internal and external, to obtain the desired characteristics of the final product (Hoyos-Concha et al., 2022). During the process, independent parameters include temperature, process speed, throughput, feed composition, and feed moisture content. On the other hand, there are system variables also known as intermediate process variables such as specific mechanical energy and mean residence time that induces reactions that affect the nutritional value and physical properties of the final product (Choudhury and Gautam, 2003b; Choudhury et al., 2014). In this way, the composition of the feed plays a fundamental role in the variables of the process.
This study was conducted because, in the Department of Cauca (Colombia), there is high trout production, and several tons of waste are generated monthly. A strategy has been developed to take advantage of this waste and generate added value. Considering that fish feed has a high commercial cost, it is necessary to generate alternatives to reduce the price. In the present manuscript, an option to do so is proposed. The studies reported in this manuscript have served as the basis for scaling up the process at the pilot plant level. Therefore, this work aimed to study the effect of hydrolyzed protein meal from silage of trout (Oncorhynchus mykiss) on the parameters of the extrusion system and their physical transformations.
2. Methods
2.1. Materials
In the extrusion process, raw materials of hydrolyzed protein flour obtained from trout by chemical ensilage, fish flour (Siquality SA, Guayaquil-Ecuador), and cassava starch (sucre starch) were used. The proximate composition is presented in Table 1. Also, additives were used that were added to the diet such as vitamin core formulated for fish farming (Premex), Bentonite (Premex), calcium carbonate (CaCO3; analytical degree 99%, Carlo Erba), and sodium chloride (NaCl; analytical grade 99%, Carlo Erba).

Table 1. Means and standard deviations of total protein, ethereal extract, ash, fiber, free extract of nitrogen (ELN), total volatile nitrogen, pepsin digestibility, degree of hydrolysis, soluble protein, formic acid, and lactic acid of raw materials on a dry basis.
2.2. Materials and preparation of extruded product
The influence of hydrolyzed protein meal was investigated by using it as a replacement for fish meal, incorporating it in a range between 10 and 30%. The addition of supplements (vitamins, minerals, and starch) was based on the recommendations by the National Research Council (2011).
The diet formulation had a content of isoprotein and isoenergetic composition for omnivorous fish in the fattening stage. The flours were sieved with a No. 40 series Tyler sieve and blended in a Kitchen Aid mixer for 30 minu. They were later packed in polyethylene bags and refrigerated for 24 h, before being extruded. Raw materials required for the preparation of the diet are presented in Table 2.
2.3. Extrusion process of the feed
A compact single-screw extruder, Haake Polylab OS Rheomex 19/25 OS (Germany), was used, with a worm screw 475 mm long (L) and 19 mm in diameter (D) with a 25:1 L/D ratio. Their characteristics were a maximum temperature of 450°C, a total speed of 250 rpm and a maximum torque of 160 Nm, coupled to a Haake RheoDrive 4 system, with a motor power of 4 kW. The extruder barrel featured three independent heating or cooling zones; heating is conducted using electrical resistances and cooling through channels utilizing compressed air circulation. The compression ratio of the screw was 5:1, and the orifice diameter of the collet used was 5 mm. The equipment has three thermocouples to monitor the temperature of the barrel, an extra thermocouple placed in the matrix to measure the temperature of the product, and a device to measure the pressure. The feeding was done with the HAAKE Metering Feeder OS. The extruded samples were cut into pellets (length: 5 ± 0.1 mm) and dried in a BINDER brand dehumidifier (Germany) at 50°C until reaching a moisture content of 10 ± 0.5%. Finally, the dry product was stored in polyethylene bags for properties analysis (Hoyos-Concha et al., 2022).
2.4. Evaluation of parameters of the extrusion system
2.4.1. Mean residence time (MRT)
Mean residence time (MRT) was measured with a marker based on previous research (Lee and Mccarthy, 1996). 0.05 g of erythrosine dye (Sigma Aldrich) was mixed with 5 g of starch adjusted to the moisture of the diet. The marker was added when the extruder reached steady-state conditions. Samples were taken every 10 s for 3 min after the addition of the title. The samples obtained were reduced in size using an IKA brand mill for fine grinding MF 10 basic (Germany) and subsequently sieved on a standard ASTM No. 50 sieve. The color of the pulverized material was determined on a Konica Minolta CR-400 colorimeter (USA) in triplicate. The intensity of the red color (C) was determined (Equation 1).
where a* represents the redness value and b* is the yellowness color value. The value of b* does not contribute to the intensity of the red color, therefore, the expression (Equation 2) is simplified.
The value of a * means a measure of the intensity of the red color in the extrudate (Bi et al., 2007). The mean residence time (TMR) or (tm) is the mean residence time of a particle and was calculated according to what was proposed by Levenspiel (1999) (Equation 3).
where tm represents the mean residence time in hours, C is the concentration of the marker at time t (h) and Δt (h) the time interval between samplings.
2.4.2. Specific mechanical energy (SME)
A sample was taken when the extruder reached a state of equilibrium, with constant torque and die pressure values, for ~5 min. For each treatment, the SME was determined by the following equation (Pitts et al., 2014) (Equation 4).
where n is the screw speed (rpm), P the power (kW), τ the torque (Nm), nmax the maximum screw speed (rpm) and Qm the total mass flow (kg/h).
2.5. Evaluation of physical changes of the extrudate
2.5.1. Porosity
The samples were obtained from cross-sections made with Ultramicrotome, LKB, Bromma (Sweden), adjusted to a cut of 30 μm, from the extruded pellets to determine the porosity. The internal diameters of the chambers formed in the pellet were captured by a Nikon DS-2Mv 2Mp digital camera (Japan), coupled to the Nikon eclipse 80i optical microscope (Japan), and processed in the Image Pro-Plus Analyzer Software (Version 6.3, 2008). The percentage of porosity obtained from the different treatments was determined.
2.5.2. Hardness
According to the procedure described by the ASAE Standard (1998), the test was conducted, which indicates the compression testing conditions of food materials with a convex shape. For this, measurements were made using a universal testing machine, Shimadzu EZ-L (Japan), with the following configuration: Test, Compression; Units, International System (SI); Load transducer, 500 N; Compression device, 13mm diameter solid steel cylinder; Moving head moving speed, 10 mm/min. The samples to be analyzed were conditioned in a Binder Model KBF 240 climatic chamber (Germany) at 50% relative humidity and 25°C, for seven days. Thirty-five representative samples were processed per treatment, 25 for the test and 10 for the moisture content measurement, which was homogeneous in their dimensions. The study processed the information in the Trapezium2 software.
2.5.3. Durability
A New Holmen NHP 100 (UK) Portable Durabilimeter equipment was used for the durability test. For this, a sample of pellets was taken and sieved through a No 16 Tyler series sieve, eliminating impurities and fine particles, to take 100 g finally. According to the pellet size, the test was conducted for 60 s, the pellets were collected, and the weight of the whole or whole material was obtained. The durability will correspond to the percentage of material that remained intact for the initial weight (Equation 5).
where Pfpellets is the final weight of the pellets (g), Pipellets is the initial weight of the pellets (g).
2.5.4. Buoyancy
According to the methodology developed by Umar et al. (2013), it was conducted with modifications. In a 500 ml beaker, 400 ml of water were poured at 25°C, 100 pellets selected by quartering were taken, which were deposited in the water for 20 min. At the end of the time, the pellets that remained on the surface were quantified, and the percentage of buoyancy was determined (Equation 6).
Where Nf is the number of pellets floating after 20 min, Nt is the total number of pellets in the test.
2.5.5. Expansion index (EI)
The expansion index of the extruded products was determined according to the methodology described by Draganovic et al. (2011). The diameter of 10 pellets obtained by quartering from each treatment was determined with a Mitutoyo Co (Japan) digital vernier caliper. The average value was obtained and divided by the extruder nozzle diameter (5 mm; Equation 7).
where EI is the expansion index, Dp is the average diameter of the pellet (mm), and Db is the diameter of the nozzle (mm).
2.5.6. Apparent density
The apparent density of the extruded products was obtained assuming the cylindrical shape of each pellet, following the method proposed by Singh et al. (2012). Ten samples of pellets obtained by quartering of 5 mm in length were used, the mass was determined using a Kern AES 220-4 Analytical Balance (Germany). Height and diameter determination was performed using a Mitutoyo Co (Japan) digital vernier caliper. The bulk density of the samples was determined (Equation 8).
where DE is the density of the pellet (kg/m3), m is the mass (kg), r is the radius (m), and l is the length (m).
2.6. Statistical analysis
Table 3 shows the 2K factorial design to evaluate the parameters of temperature, screw speed, moisture content, and inclusion of hydrolyzed protein flour, with two levels each. The factorial experiment was extended by adding axial and central points to obtain a response surface design, with a main composite design of 31 treatments in total, distributed as follows: 16 treatments (1–16) corresponding to the factorial portion, eight treatments (17–24) corresponding to axial points and seven treatments (25–31) corresponding to central themes.
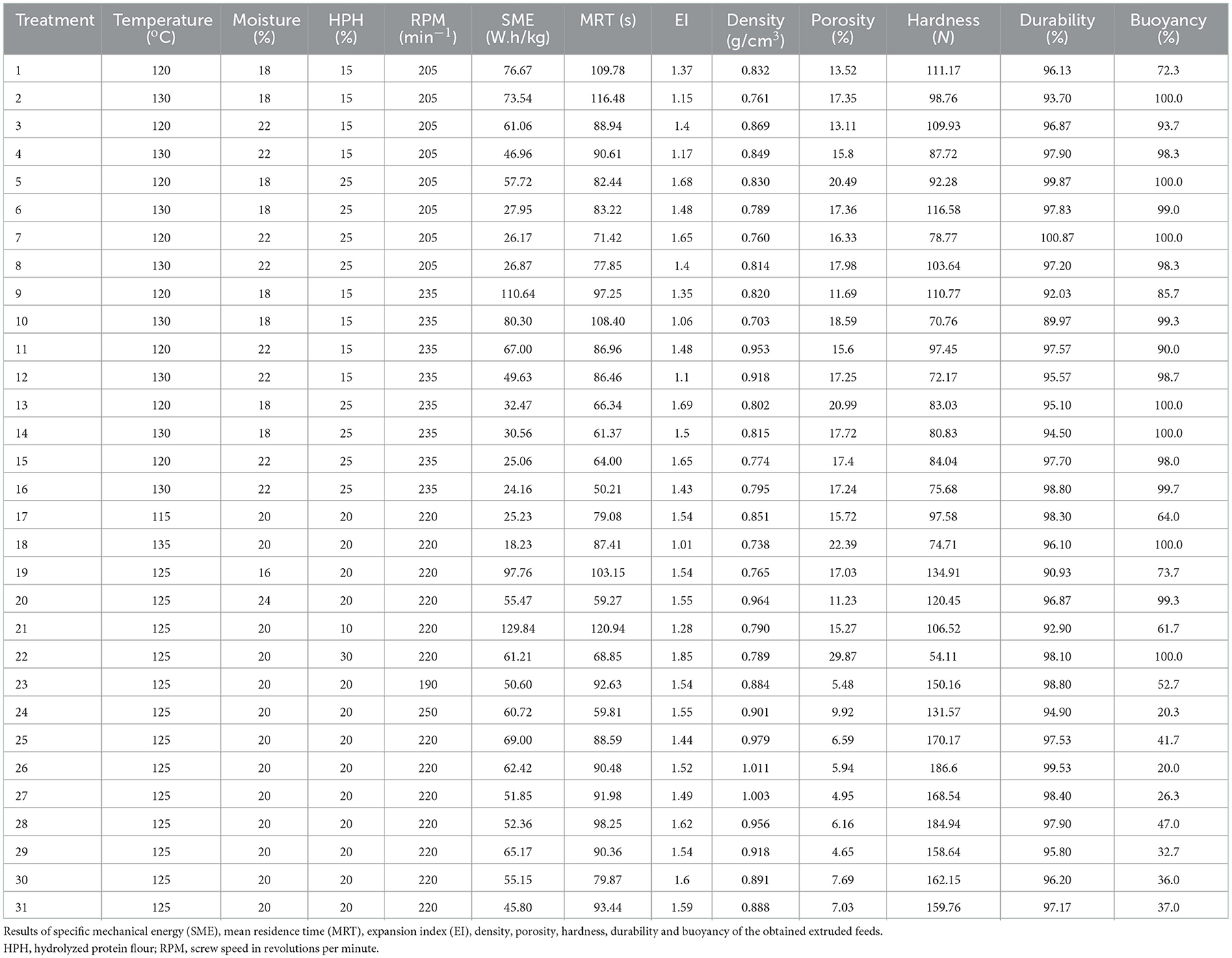
Table 3. Experimental design based on temperature, moisture, hydrolyzed protein flour (HPH) and screw speed (RMP).
The axial points were obtained by applying the term α = (F)¼, where F is the number of points used in the factorial portion of the design, where α = 2. The values of the axial points were extrapolated according to the coding presented in Table 5. Minitab 16 software was used for the response surface analysis.
3. Results
3.1. Extrusion system parameters
Table 4 presents the summary of the results obtained from the central composite design for each of the response variables. Once the fulfillment of the normality assumptions had been verified, the analysis of variance was carried out using linear regression. The non-significant terms were eliminated according to F and P-values, considering a significance level of 0.05. With the ANOVA adjusted for each of the variables, the regression coefficients were obtained for the construction of the mathematical models, corresponding to each response surface as a function of mean retention time (TMR), specific mechanical energy (SME), index of expansion (EI), density, porosity, hardness, durability and buoyancy, these results are presented in Table 3. All the models suggested a lack of acceptable fit (P-value > 0.05), and according to the regression analysis, it can also be stated that the effects considered in Table 4 explain the variability in a percentage higher than 70%. They were obtained in the data. Furthermore, the existence of curvature in the experimental plane evaluated can be inferred for all response variables due to the presence of quadratic terms with significant effect (P-value < 0.05).
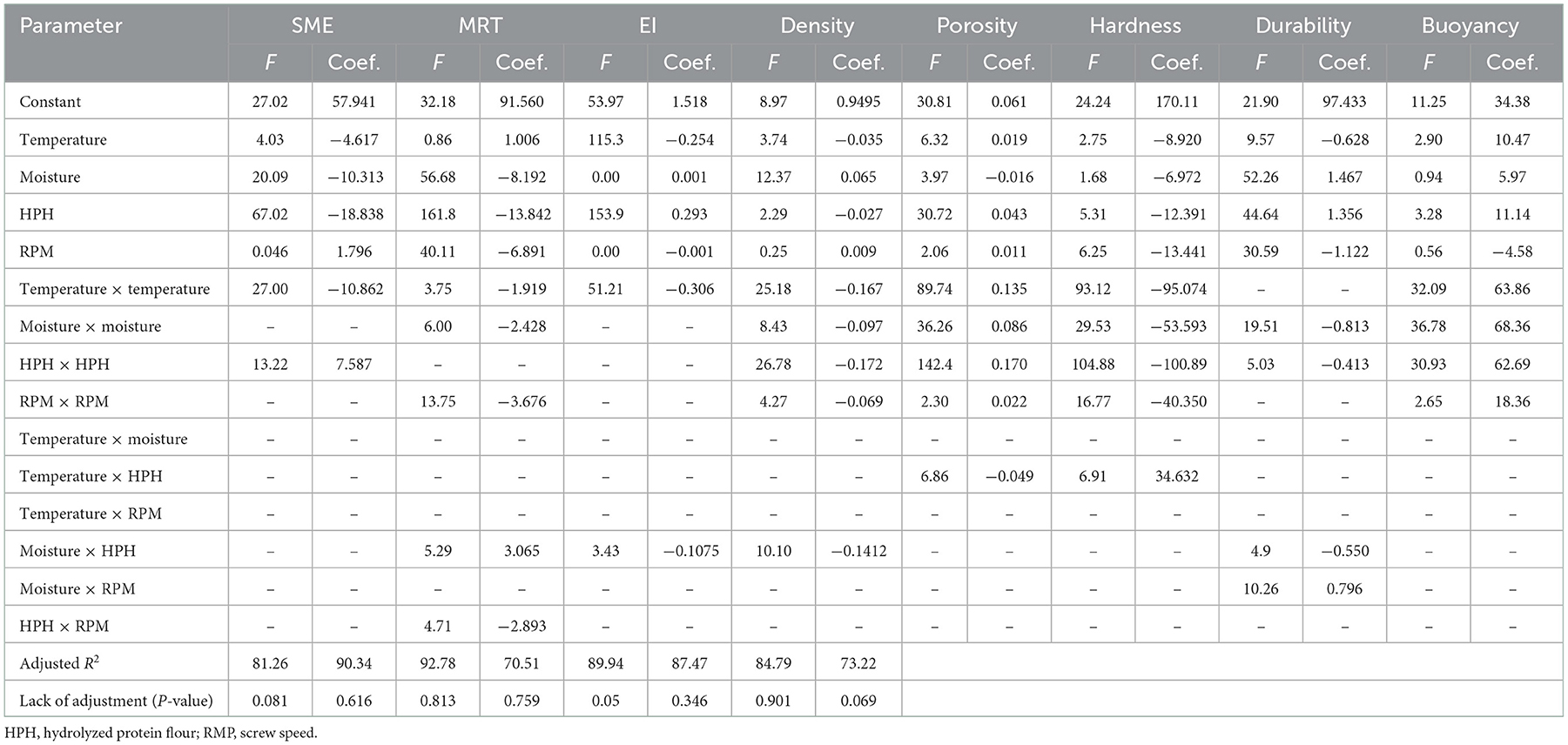
Table 4. Regression analysis of specific mechanical energy (SME), mean residence time (MRT), expansion index (EI), density, porosity, hardness, durability, and buoyancy of the obtained extruded feeds.
3.1.1. Specific mechanical energy (SME)
The F values estimated in the ANOVA (Table 4) indicate that the factors hydrolyzed protein flour (67.02) and moisture content (20.09), followed by the quadratic effects of temperature (27.10) and hydrolyzed protein flour (13.22), has the most significant influence on SME. In Table 5, it is verified that the regression coefficient of the screw speed has a positive effect on the SME (1.796). This fact means that the SME increased by increasing the screw speed. Although the impact of screw speed is low, some authors attribute the change to an increase in torque in the equipment's motor. Factors such as engine torque, die pressure, and SME were closely related. Besides, Figure 1 shows the effects of HPH, moisture content and temperature on SME.
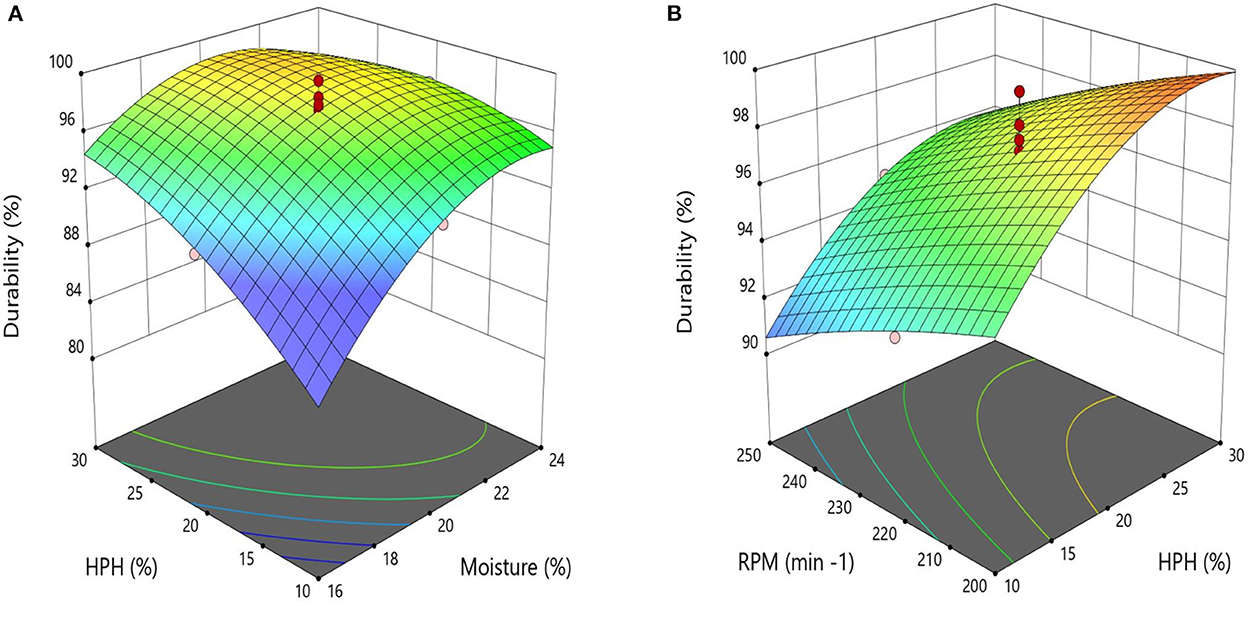
Figure 1. Effect of HPH, moisture and temperature on EME. (A) Moisture vs. HPH (temperature 125°C and screw speed 220 RPM). (B) HPH vs. temperature (screw speed 220 RPM and humidity 20%).
According to Figure 1A, it can be verified that SME decreased when there were a high concentration of moisture and hydrolyzed protein. However, this decrease is much more pronounced because of the hydrolyzed protein compared to the impact that the change in moisture content in the mixture could generate. On the other hand, in Figure 1A, it is observed that as the concentration of hydrolyzed protein decreases, the SME increases, which can be explained by a higher concentration of fishmeal (high molecular weight protein). The effect of temperature and hydrolyzed protein can be seen in Figure 1B that hydrolyzed protein has a notable impact on the impact of weather on SME. As in the previous case, it can be verified that with the increase in the content of hydrolyzed protein flour, the SME decreased, independent of the evaluated temperature.
3.1.2. Retention mean time (TMR)
The F values estimated in the ANOVA (Table 4) show that the individual factors of hydrolyzed protein flour (161.82) and moisture (56.68) had a more significant influence on the TMR. Similarly, there was essential participation of the pure quadratic term of the screw speed (13.75) and the effect of hydrolyzed protein flour with moisture and screw speed. Figure 2 shows the impact of HPH, moisture content and screw speed on TMR.
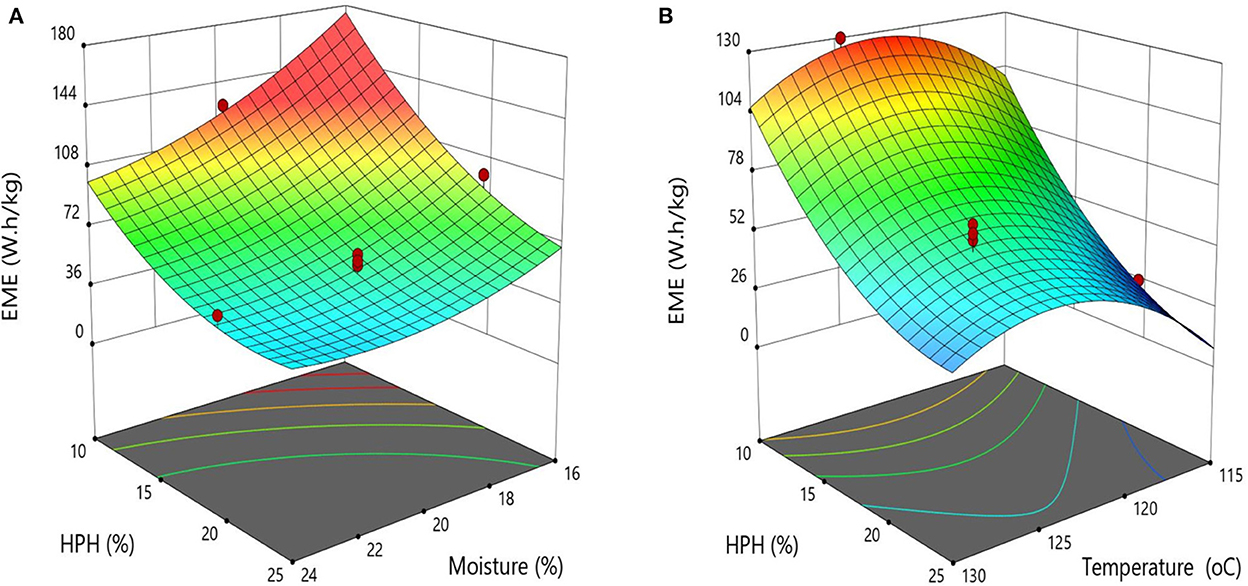
Figure 2. Effect of HPH, moisture and screw speed on TMR. (A) Moisture vs. HPH (temperature 125°C and screw speed 220 RPM). (B) Screw speed vs. HPH (moisture 20% and temperature 125°C).
3.2. Evaluation of the physical changes of the extrudate
3.2.1. Expansion index (EI)
The F values estimated in the ANOVA (Table 5) indicated that the hydrolyzed protein flour factors (153.9) and temperature (115.3) exerted a more substantial influence on the response variable. Furthermore, the only interaction with a significant effect was moisture and hydrolyzed protein flour (3.43).
According to Table 4, the EI ranged between 1.01 and 1.85. Figure 3A shows that the increase in hydrolyzed protein concentration provoked an increase in EI.
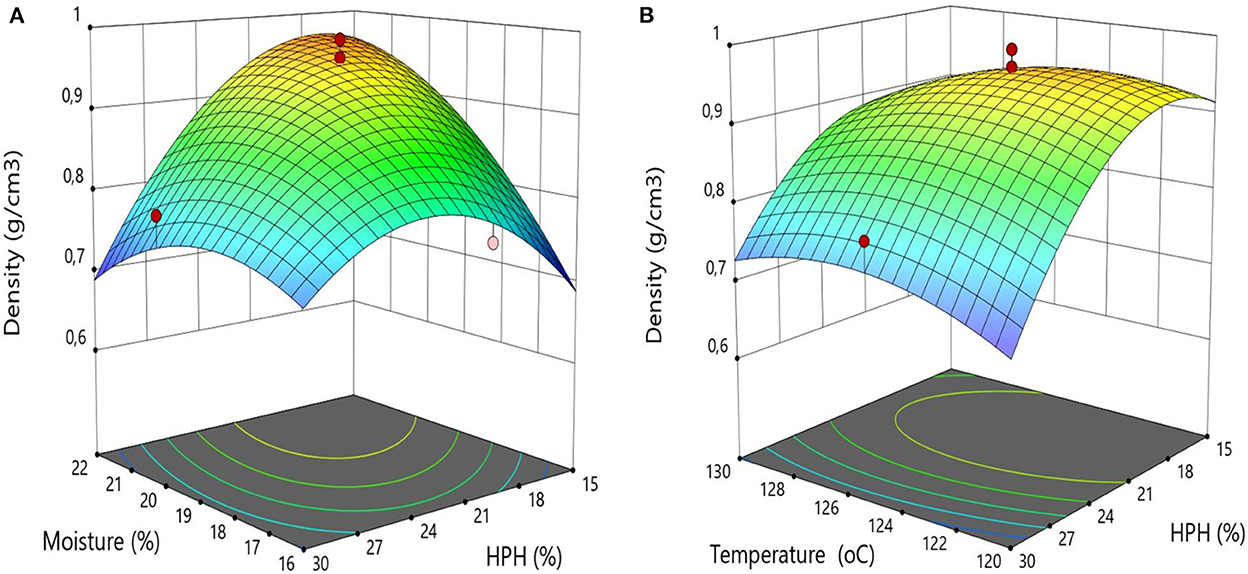
Figure 3. Effect of HPH, moisture and temperature on EI. (A) Moisture vs. HPH (temperature 125°c and screw speed 220 RPM). (B) HPH vs. temperature (screw speed 220 RPM and moisture 20%).
3.2.2. Density
Figure 4 shows the effect of HPH, moisture content and temperature on density. Figure 4A shows that the product's density decreased with the concentration of hydrolyzed protein flour. In Figure 4B, it is observed that the hydrolyzed protein flour had a more significant influence on the change in the density of the product obtained compared to the effect that the temperature change can exert. However, the interaction of temperature and hydrolyzed protein flour increased density when extrusion conditions converge on an intermediate temperature profile of 128°C and a concentration of hydrolyzed protein flour of 20%. On the other hand, the change in density attributable to screw speed is low. In Table 5, it is observed that the terms that represent the screw speed, independent (F = 0.25) and pure quadratic (F = 4.27), contributed little to the change in density.
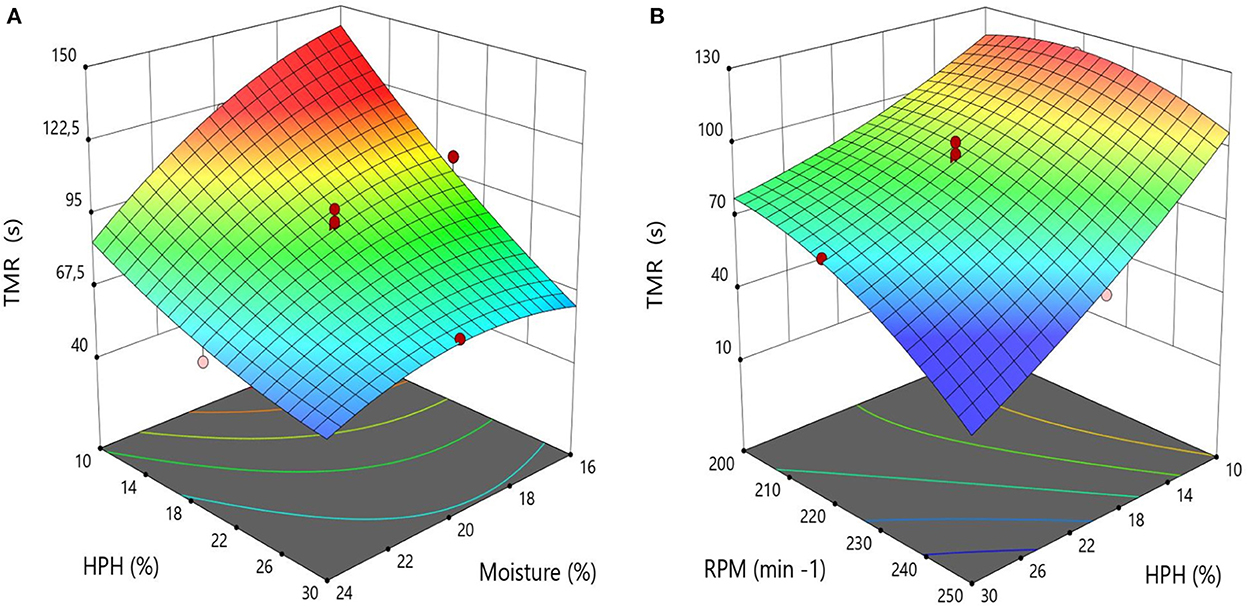
Figure 4. Effect of HPH, moisture and temperature on density. (A) Moisture vs. HPH (temperature 125°C and screw speed 220 RPM). (B) HPH vs. temperature (screw speed 220 RPM and moisture 20%).
3.2.3. Hardness
In Figure 5A, it is observed that there is a more significant effect of the concentration of hydrolyzed protein flour on hardness compared to the impact that a change in moisture content could exert during the extrusion process. It was also found that the lowest level of hardness of the extrudates was achieved when the content of hydrolyzed protein flour was at its maximum concentration (30%). Secondly, in Figure 5B, a more significant influence is corroborated by the variation of hydrolyzed protein flour in the mixture, compared to the impact generated by temperature. However, when the temperature in the extruder barrel was low, and the concentration of hydrolyzed protein flour was high (30%), the hardness of the material showed an apparent decrease. Table 5 shows the effect of screw speed and the regression coefficient for this parameter.
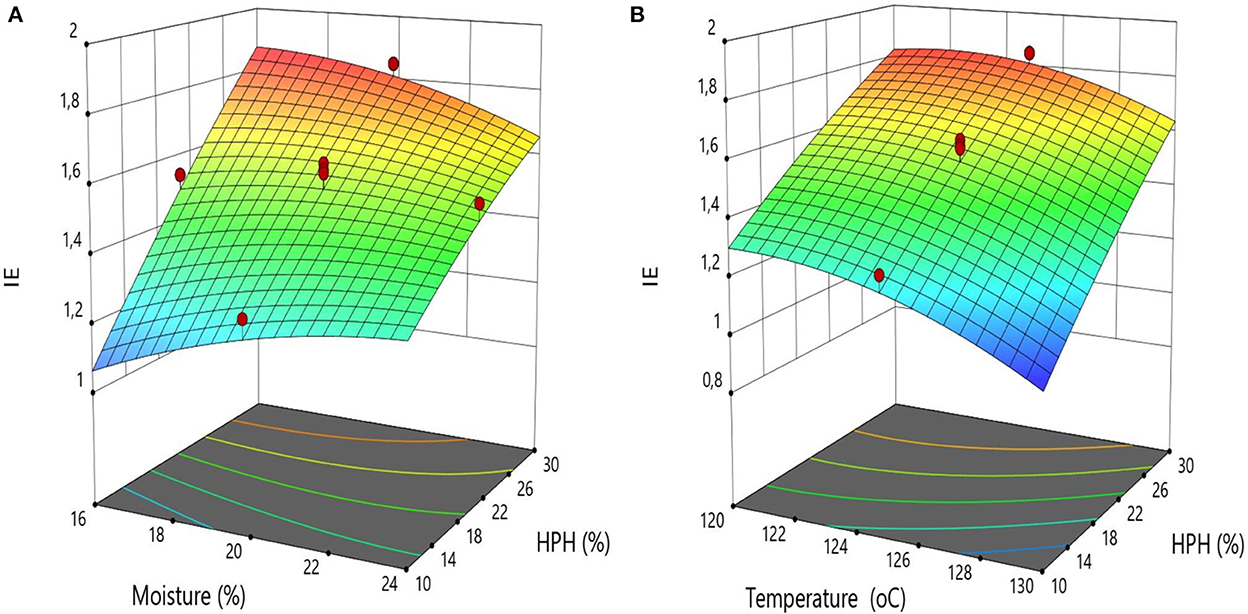
Figure 5. Effect of HPH, moisture and temperature on hardness. (A) Moisture vs. HPH (temperature 125°C and screw speed 220 RPM). (B) HPH vs. temperature (screw speed 220 RPM and moisture 20%).
3.2.4. Durability
Table 5 shows the F values estimated in the ANOVA as moisture content (52.26) and hydrolyzed protein meal (44.64) as independent terms with more influence on durability. Figure 6A shows that the maximum durability of the pellets was obtained when the moisture content was between 20 and 24% and the concentration of hydrolyzed protein flour was kept between 25 and 30%. In Figure 6B, the high durability of the pellets can be observed when the screw speed was 190 rpm, while the content of hydrolyzed protein flour in the mixture was high (30%).
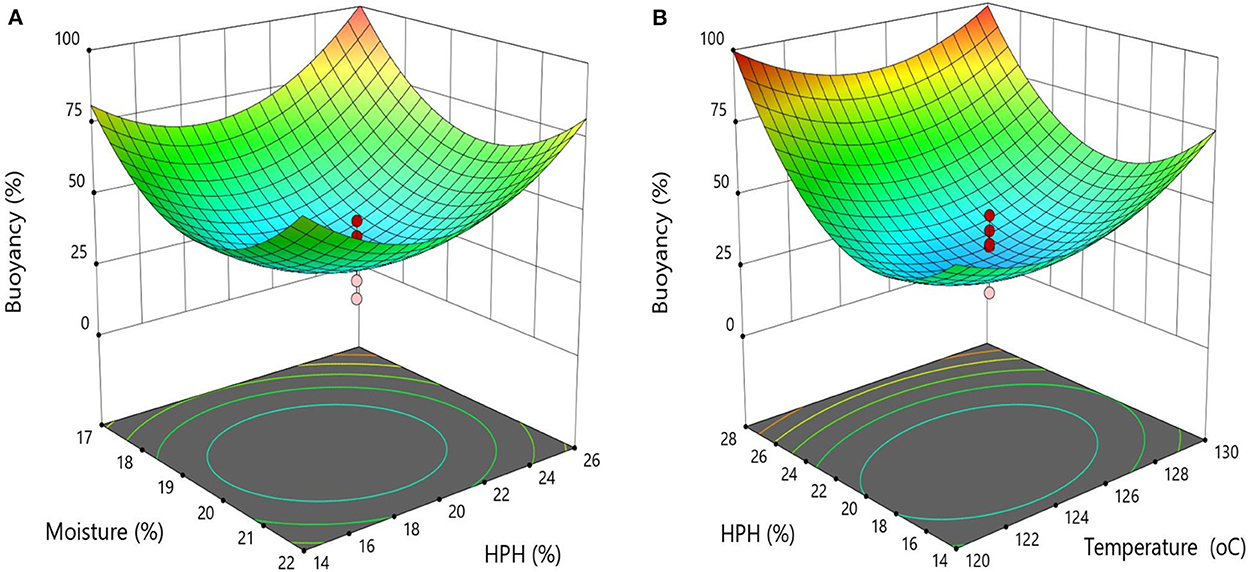
Figure 6. Effect of HPH, moisture and screw speed on durability. (A) HPH vs. Moisture (temperature 125°C and screw speed 220 RPM). (B) HPH vs. screw speed (temperature 125°C and moisture 20%).
3.2.5. Buoyancy
The values of F estimated in the ANOVA (Table 5) indicate that the pure quadratic terms of temperature (32.09), moisture content (36.78) and hydrolyzed protein flour (30.93) were those that had the most significant influence on buoyancy. Figure 7 shows the effect of HPH, moisture content and temperature on buoyancy. According to Figure 7A, the maximum percentage of buoyancy of the pellets was reached when the extrusion process was carried out with 24% moisture content and 30% hydrolyzed protein flour. These observations agree with the minimum density (Figure 4A) achieved at said extrusion conditions.
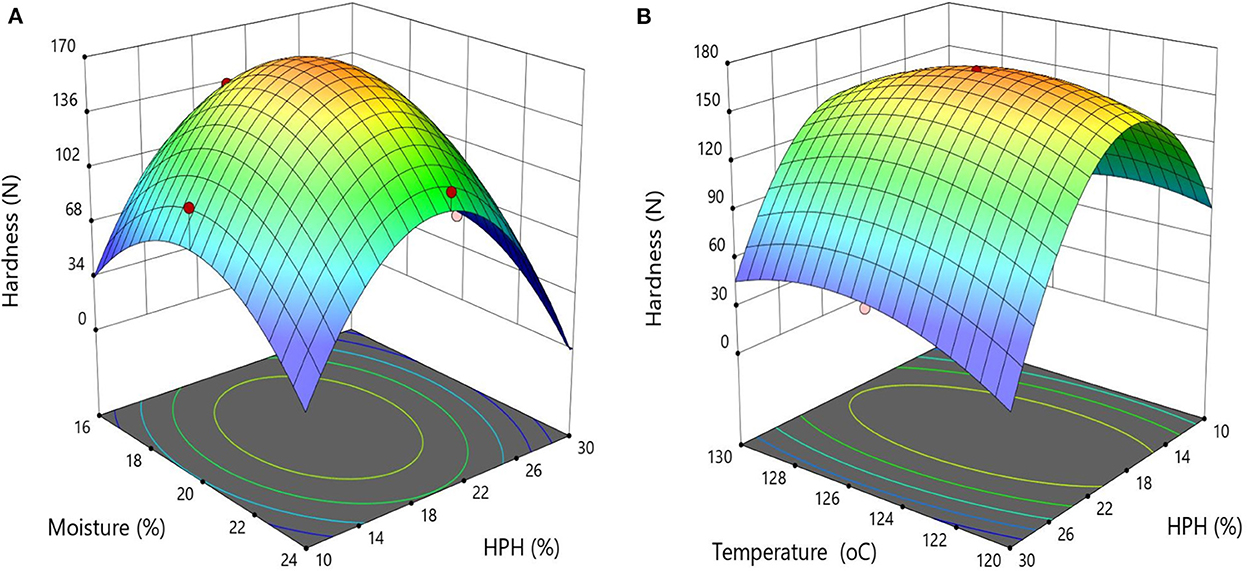
Figure 7. Effect of HPH, moisture and temperature on buoyancy. (A) Moisture vs. HPH (temperature 125°C and screw speed 220 RPM). (B) HPH vs. temperature (screw speed 220 RPM and moisture 20%).
4. Discussions
4.1. Extrusion system parameters
4.1.1. Specific mechanical energy (SME)
According to Figure 1A, it can be verified that SME decreased when there was a high concentration of moisture and hydrolyzed protein simultaneously. However, this decrease was much more pronounced because of the hydrolyzed protein than the impact that the change in moisture content in the mixture could generate. On the other hand, in Figure 1A, it is observed that, as the concentration of hydrolyzed protein decreased, the SME increased; this behavior could be attributed to a higher concentration of fish meal (high molecular weight protein). The effect of temperature and hydrolyzed protein can be seen in Figure 1B that hydrolyzed protein had a notable impact on the impact of weather on SME. As in the previous case, it can be verified that with the increase in the content of hydrolyzed protein flour, the SME decreased, independent of the evaluated temperature. The decrease in SME at low or elevated temperatures was probably due to reduced viscosity in the mixture fed to the extruder (Singha et al., 2018). At the same time, they were related to the level of transformation of the product obtained and its characteristics such as expansion, density, geometric and texture characteristics (Pansawat et al., 2008).
Similar studies reported that the SME applied to a mixture in specific parameters was affected by the complex viscosity of the mixture during the fusion process and the mixture by the torque needed to turn the screws. Therefore, a mixture with higher viscosity and a lower processing temperature needs a higher torque and, by extension, a higher SME than mixtures in conditions where the viscosity was lower (Ma et al., 2019; Thompson and Williams, 2021).
The use of low temperatures avoided the gelatinization of the starch and its interaction with the protein. In addition, the plasticizing effect of the proteins allowed the mobility and fluidity of the mixture through the extruder (Samuelsen and Oterhals, 2016). On the other hand, elevated temperatures also decreased SME, but the behavior was probably due to the rapid dextrinization of carbohydrates and the breakdown of previously formed protein complexes. This effect also facilitated the movement of the melted mixture through the extruder (Day and Swanson, 2013; Miranda et al., 2014). The increase in SME at an intermediate temperature condition could be explained by the rise in the viscosity of the molten material (Pansawat et al., 2008).
4.1.2. Retention mean time (TMR)
Regarding Figure 2A, it can verify that the TMR had a more significant change when the concentration of hydrolyzed protein flour decreased, keeping the moisture content low. The concentration of hydrolyzed protein and the indirect effect of the concentration of fish meal present in the mixture could explain this behavior. A low concentration of hydrolyzed protein (low molecular weight protein) was related to a higher concentration of fishmeal (high molecular weight protein), which can absorb and retain water more strongly and limits the efficient distribution of moisture available, resulting in a decrease in plasticizer in the mix and addition in TMR (Jean et al., 2005; Singh et al., 2012; Samuelsen et al., 2014). By increasing the concentration of hydrolyzed protein and the mixture's moisture content, the TMR's lowest value was recorded. In this case, the protein and the moisture content function as plasticizers facilitating the mobility of the mixture in the extruder. However, excess moisture and a high level of hydrolysis of fish protein decreased viscosity so much that it was difficult for the material to exit the extruder (Choudhury and Gautam, 2003a). In Figure 2B, it was observed that the high screw speeds decreased the TMR, according to that reported by Yu et al. (2014), presenting a more efficient heat dissipation, greater pumping force, and more significant shear, giving way to better mixing of the material and a short TMR (Kumar et al., 2006). A drop in TMR was observed at extreme temperatures and slightly increased when the process was at intermediate temperatures. The fall of the TMR may be a function of a loss of the mixture's viscosity inside the extruder, facilitating the transit of the material through the barrel. Said thickness loss occurred when low molecular weight protein concentration and its plasticizing effect increased (Samuelsen et al., 2014) at the low-temperature point. On the other hand, increasing the temperature to the maximum level also generated a drop in the viscosity of the molten material, requiring less effort to exit the extruder (Kumar et al., 2006).
4.2. Evaluation of the physical changes of the extrudate
4.2.1. Expansion index (EI)
This behavior could be associated with the low molecular weight of the hydrolyzed protein. Although this type of protein allowed a uniform moisture distribution, it also facilitated the gelatinization of starches in the hydrothermal process (Samuelsen et al., 2014). At the same time, these proteins provided sufficient material for interaction between components, facilitating a nucleation effect for the formation of cells, regulating the release of water vapor, and maintaining the expanded structure at the exit of the extruder (Singh and Muthukumarappan, 2016).
Figure 3B shows the change in EI due to the change in hydrolyzed protein and temperature concentration, finding that the effect of the concentration of hydrolyzed protein flour was more significant (Table 5). This figure verifies that maintaining a concentration of hydrolyzed protein flour equal to 30% and conducting the extrusion at temperatures below 120°C were sufficient conditions to achieve a higher EI. This fact was probably because the temperatures were sufficient to achieve gelatinization of the available starch (Dileep et al., 2009) and facilitate the interaction between proteins-carbohydrates through interactions by hydrogen bonds, ionic bonds, and hydrophobic interactions (Samuelsen and Oterhals, 2016), generating stable networks or structures at the exit of the extruder, facilitating an increase in EI. On the other hand, if the temperature was increased beyond 120°C, it contributed to a decrease in the viscosity of the molten material, the product of the excessive degradation of the components in the mixture, causing low stability of the networks formed at the exit from the extruder and deficiencies in the filling of the barrel, all these mechanisms generated a consequent decrease in EI (Pansawat et al., 2008; Valenzuela et al., 2017).
On the other hand, it has been reported that a low screw speed reduces shear forces, generates less pressure inside the barrel in the extruder and decreases radial expansion (Majumdar and Singh, 2014). Excess screw speed produces a structural collapse of the extruded food material, reducing the EI (Valenzuela et al., 2017).
4.2.2. Density
The apparent density (g/cm3) quantifies the mass of the material per unit volume of the extrudates. It measures the extrudates' internal structure (Kannadhason et al., 2010). The molten mixture suffers expansion as it exits the extruder. According to Figure 4A, the density of the product decreased with the concentration of hydrolyzed protein flour, which made the moisture present in the mixture easily distributed, favoring the gelatinization of the starch, interactions with protein and the nucleation effect with a increase in pressure (Dileep et al., 2009).
When making a comparison of the results of EI and density, it can be stated that achieving a very low density does not necessarily imply a maximum expansion, as observed in Figures 3A, 4A, where the highest level of increase was completed at the point hydrolyzed protein flour 30% and moisture content 16%. Density and EI were negatively correlated in any extrusion process, behavior related to the distribution of cells inside the extrudate, size, and thickness of the walls that they form makeup (Zarzycki et al., 2015).
The lowest level of density was achieved at a low screw speed and a maximum concentration of hydrolyzed protein flour in the mixture, indicating that a low screw speed was sufficient to facilitate a kneading of the mix that allowed the interaction between the low molecular weight proteins and available carbohydrates, promoted the shear effect, the pressure inside the extruder and the distribution of moisture content (Cheftel et al., 2014).
4.2.3. Hardness
The hardness is considered a property that allows information about the physical quality of the pellets (Samuelsen and Oterhals, 2016) and is positively correlated with increased density and durability (Majumdar and Singh, 2014). The behavior of Figure 5 could be attributed to a higher concentration of low molecular weight protein, which favored a higher level of starch gelatinization, with the generation of expanded, porous, and low-density structures (Ozer et al., 2004), characteristics that were inversely correlated to hardness.
Zhang et al. (2021) reported that at low humidity levels, the hardness of the product decreased as the cooking temperature increased. In addition, as the extrudate emerged from the cooling nozzle, there was a tendency to swell slightly, weakening the structure and leading to lower hardness.
4.2.4. Durability
The durability of an extrudate is related to the ability of the solid material to maintain a complete structure when subjected to external mechanical stress, such as wear due to fragmentation and abrasion (Kaliyan and Vance, 2009; Samuelsen et al., 2013). Figure 6 shows the effect of HPH, moisture content, and screw speed on durability.
According to Figure 6A, the maximum durability of the pellets was obtained when the moisture content was between 20 and 24%, and the concentration of hydrolyzed protein flour was kept between 25 and 30%. These combinations could be constituted in an adequate ratio of components for adequate mixing of the molten material, homogeneity, and increasing durability (Samuelsen et al., 2014). The decrease in durability in the pellets occurred when the process was carried out at low levels of hydrolyzed protein flour (10%) and low moisture contents (16%), due to the high molecular weight of fishmeal, which had a great influence on the result, since it prevented the starch from achieving an effective gelatinization process, avoiding the interaction of components during the fusion of the mixture (Samuelsen et al., 2013; Ah-Hen et al., 2014).
In the Figure 6B, this behavior could be explained by the effectiveness of the shear generated under these extrusion conditions, which may be sufficient to allow the components in the mixture to achieve their melting temperature, with the consequent formation of a durable structure (Samuelsen et al., 2014).
Also, the durability of the pellets reached its lowest level when the screw speed was increased (250 rpm), and the concentration of hydrolyzed protein flour in the mixture was decreased (10%). This behavior was influenced by the high concentration of high molecular weight proteins (heavy flour) in the protein fraction of the mixture, limiting the availability of moisture, which means that the starches were unable to gelatinize, as explained above. In addition, it prevented fusion, shear, and coupling of low molecular weight proteins, causing a decrease in the durability of the material (Jobling et al., 2007).
Ishak et al. (2022) studied different starch sources influencing the physical parameters of extruded foods prepared for the Malaysian mahseer, Tor tambroides. Forty percent protein and 23.4% starch were used in the methodology, and they were extruded using a single screw extruder (120 rpm speed, 2 mm diameter die head). Among the results, it was found that the starches from three local crops (sago, Metroxylon sagu; manioc, Manihot esculenta; and taro, Colocasia esculenta) produced extrudates with a quality like the extrudates of corn starch in terms of expansion ratio, durability index, durability index, and buoyancy index. However, electron microscopy indicated cassava starch extrudates had the best external appearance.
By verifying the effect of temperature on its regression coefficient (Table 5), its negative effect concerning the durability of the pellet can be established. This behavior could be attributed to the fact that the temperature profiles close to 115°C were sufficient to ensure that the molten mixture that passes through the extruder could induce proteins to quickly join with the rest of the components in the mixture, improving the durability of the pellets (Kaliyan and Vance, 2009).
On the other hand, if the temperature profiles used in the process were high (135°C). At the same time, the content of hydrolyzed protein flour was low (10%), extrudates of a non-cohesive nature were generated and, therefore, pellets of low durability (Jobling et al., 2007; Chevanan et al., 2008).
4.2.5. Buoyancy
Buoyancy is the number of floating pellets suspended in the aqueous medium, observed for some time. Furthermore, the buoyancy of the pellets has been inversely associated with density, stability to water, higher absorption, and low solubility. In this sense, the buoyancy of the pellets is guaranteed if the density is below 750 kg/m3. Authors such as de Cruz et al. (2015) stated that the pellets float if the thickness was below 530 kg/m3, while authors such as Mjoun and Rosentrater (2011) stated that there was a guaranteed buoyancy if the pellets have a density lower than 1 g/ml. These variations could be a function of the dimensions of the pellets and their composition (Vassallo et al., 2005).
Regarding the effect of the speed of the screw and according to its regression coefficient of the model, it has a significant quadratic effect on the change in buoyancy (2.65). However, its impact was positive and of low influence on others. Variables considered buoyancy increased at the extreme levels of this parameter and decreased at intermediate values. This behavior was that reported with the density analysis (Table 5), where its quadratic effect, apart from being significant, its influence was negative, being inversely correlated variables (Chevanan et al., 2008; Draganovic et al., 2011; Mjoun and Rosentrater, 2011; Ah-Hen et al., 2014; de Cruz et al., 2015).
5. Conclusions
Regarding the cohesion capacity (crosslink), the physical properties of the granules, such as buoyancy, expansion index, porosity, hardness, and durability, were studied. Hydrolyzed protein flour had cohesive and plasticizing capacity. The plasticizing capacity generated a reduction in the average retention time in the extruder cylinder and a decrease in the specific mechanical energy. Although HPH had a cohesive action, the increase in mass flow due to the plasticizing effect impacts the SME, reducing its value. Durability was the response variable that showed a positive correlation. In the study, high-durability pellets were achieved due to the cohesiveness of the hydrolyzed protein flour, but at the same, time low hardness due to the high porosity achieved. Therefore, porosity was one of the response variables in which the addition of hydrolyzed protein flour had a higher incidence. The fish feed obtained in this work has a high potential to replace, at least partially, commercial fishmeal, having a positive impact on production costs. It has also served as the basis for scaling production to a semi-industrial scale in the Department of Cauca, Colombia.
Data availability statement
The original contributions presented in the study are included in the article/supplementary material, further inquiries can be directed to the corresponding authors.
Ethics statement
The animal study was reviewed and approved by Comité de ética de la Universidad del Cauca.
Author contributions
Conceptualization, formal analysis, funding acquisition, investigation, and methodology: JH-C. Data curation: JH-C and DR-A. Project administration: JH-C and HV-C. Resources and validation: JH-C, HV-C, and AF-Q. Software and writing—original draft: JH-C and RO-T. Supervision: HV-C and AF-Q. Visualization: JH-C and AF-Q. Writing—review and editing: JH-C, DR-A, and RO-T. All authors have read and agreed to the published version of the manuscript.
Acknowledgments
The authors thank Universidad del Cauca and the financial support of the General System of Royalties through project ID 3883.
Conflict of interest
The authors declare that the research was conducted in the absence of any commercial or financial relationships that could be construed as a potential conflict of interest.
Publisher's note
All claims expressed in this article are solely those of the authors and do not necessarily represent those of their affiliated organizations, or those of the publisher, the editors and the reviewers. Any product that may be evaluated in this article, or claim that may be made by its manufacturer, is not guaranteed or endorsed by the publisher.
References
Aarseth, K. A. (2004). Attrition of feed pellets during pneumatic conveying: the influence of velocity and bend radius. Biosyst. Eng. 89, 197–213. doi: 10.1016/j.biosystemseng.2004.06.008
Aarseth, K. A., Perez, V., Bøe, J. K., and Jeksrud, W. K. (2006). Reliable pneumatic conveying of fish feed. Aquacult. Eng. 35, 14–25. doi: 10.1016/j.aquaeng.2005.06.006
Ah-Hen, K., Lehnebach, G., Lemus, R., Zura, L., Leyton, P., Vega, A., and Figuerola, F. (2014). Evaluation of different starch sources in extruded feed for Atlantic salmon. Aquacult. Nutr. 20, 183–191. doi: 10.1111/anu.12064
ASAE Standard. (1998). Compression test of Food Materials of Convex Shape. St. Joseph, MI: American Society of Agricultural Engineering, S368.3, 580–587.
Bi, C., Jiang, B., and Li, A. (2007). Digital image processing method for measuring the residence time distribution in a plasticating extruder. Polym. Eng. Sci. 47, 1108–1113. doi: 10.1002/pen.20793
Chaabani, A., Labonne, L., Durrieu, V., Rouilly, A., Skiba, F., and Evon, P. (2022). Preconditioner influence on twin-screw extrusion cooking of starch-based feed pellets: the example of fish feed. Aquacult. Eng. 102268. doi: 10.1016/j.aquaeng.2022.102268
Cheftel, J., Kitagawa, M., and Quéguiner, C. (2014). New protein texturization processes by extrusion cooking at high moisture levels. Food Rev. Int. 8, 235–275. doi: 10.1080/87559129209540940
Chen, F. L., Wei, Y. M., Zhang, B., and Ojokoh, A. O. (2010). System parameters and product properties response of soybean protein extruded at wide moisture range. J. Food Eng. 96, 208–213. doi: 10.1016/j.jfoodeng.2009.07.014
Chevanan, N., Rosentrater, K. A., and Muthukumarappan, K. (2008). Effect of DDGS, moisture content, and screw speed on physical properties of extrudates in single-screw extrusion. Cereal Chem. 85, 132–139. doi: 10.1094/CCHEM-85-2-0132
Choudhury, G. S., and Gautam, A. (2003a). Hydrolyzed fish muscle as a modifier of rice flour extrudate characteristics. J. Food Sci. 68, 1713–1721. doi: 10.1111/j.1365-2621.2003.tb12318.x
Choudhury, G. S., and Gautam, A. (2003b). Effects of hydrolysed fish muscle on intermediate process variables during twin-screw extrusion of rice flour. Lebensm. Wiss. Technol. 36,667–678. doi: 10.1016/S0023-6438(03)00087-2
Choudhury, M., Chakraborty, R., and Chaudhuri, U. (2014). Thermal and microstructural property of extruded snack: an overview. J. Eng. Appl. 4, 9–18. doi: 10.1021/acsomega.1c02650
Cruz-Suárez, L. E., Ruiz-Díaz, P. P., Cota-Cerecer, E., Guajardo-Barbosa, C., Tapia-Salazar, M., and Ricque-Marie, D. (2006). Revisión sobre Algunas Características Físicas y Control de Calidad de Alimentos Comerciales para Camarón en México. San Nicolás de los Garza: Universidad Autónoma de Nuevo León, 330–370.
Day, L., and Swanson, B. G. (2013). Functionality of protein-fortified extrudates. Compr. Rev. Food Sci. Food Saf. 12, 546–564. doi: 10.1111/1541-4337.12023
de Cruz, C., Kamarudin, M., Saad, C., and Ramezani-Fard, E. (2015). Effects of extruder die temperature on the physical properties of extruded fish pellets containing taro and broken rice starch. Anim. Feed Sci. Technol. 199, 137–145. doi: 10.1016/j.anifeedsci.2014.11.010
Dileep, A., Shamasundar, B., Binsi, P., and Howell, N. (2009). Composition and quality of rice flour - fish mince based extruded products with emphasis on thermal properties of rice flour. J. Texture Stud. 41,190–207. doi: 10.1111/j.1745-4603.2010.00221.x
Draganovic, V., Van Der Goot, A., Boom, R., and Jonkers. (2011). Assessment of the effects of fish meal, wheat gluten, soy protein concentrate and feed moisture on extruder system parameters and the technical quality of fish feed. Anim. Feed Sci. Technol. 165, 238–250. doi: 10.1016/j.anifeedsci.2011.03.004
Hoyos-Concha, J. L., Villada-Castillo, H. S., Fernández-Quintero, A., and Ortega-Toro, R. (2022). Effect of the addition of high-protein hydrolyzed flour from Oncorhynchus mykiss byproducts on the properties of an extruded feed. ACS Omega 7, 2554–2564.
Ishak, S. D., Yusof, Y. A., and Kamarudin, M. S. (2022). Different starch sources affect physical characteristics of extruded feeds prepared for the Malaysian mahseer, Tor tambroides. J. Appl. Aquacult. 34, 1–18. doi: 10.1080/10454438.2020.1822979
Jean, I., Work, R., Camire, M., Briggs, J., Barrett, A., and Bushway, A. (2005). Selected properties of extruded potato and chicken meat. J. Food Sci. 61, 783–789. doi: 10.1111/j.1365-2621.1996.tb12203.x
Jobling, M., Gomes, E., and Dias, J. (2007). “Feed types, manufacture, and ingredients.” in Food Intake in Fish, eds D. Houlihan, T. Boujard, and M. Jobling (Oxford: Blackwell Science Ltd), 5025–5048.
Kaliyan, N., and Vance, R. (2009). Factors affecting strength and durability of densified biomass products. Biomass Bioenergy 33, 337–359. doi: 10.1016/j.biombioe.2008.08.005
Kannadhason, S., Rosentrater, K., Muthukumarappan, K., and Brown, M. (2010). Twin screw extrusion of DDGS-bBased aquaculture feeds. J. Aquacult. Soc. 41,1–15. doi: 10.1111/j.1749-7345.2009.00328.x
Kumar, A., Ganjyal, G. M., Jones, D. D., and Hanna, M. A. (2006). Digital image processing for measurement of residence time distribution in a laboratory extruder. J. Food Eng. 75, 237–244. doi: 10.1016/j.jfoodeng.2005.04.025
Lee, S. Y., and Mccarthy, K. L. (1996). Effect of screw configuration and speed on RTD and expansion of rice extrudate. J. Food Process Eng. 19, 153–170. doi: 10.1111/j.1745-4530.1996.tb00387.x
Levenspiel, O. (1999). Chemical reaction engineering. Ind. Eng. Chem. Res. 38, 4140–4143. doi: 10.1021/ie990488g
Ma, X., Huang, S., Lowinger, M. B., Liu, X., Lu, X., Su, Y., and Williams, R. O. III (2019). Influence of mechanical and thermal energy on nifedipine amorphous solid dispersions prepared by hot melt extrusion: preparation and physical stability. Int. J. Pharm. 561, 324–334. doi: 10.1016/j.ijpharm.2019.03.014
Majumdar, R. K., and Singh, R. K. R. (2014). The effect of extrusion conditions on the physicochemical properties and sensory characteristics of fish-based expanded snacks. J. Food Process. Preserv. 38, 864–879. doi: 10.1111/jfpp.12041
Miranda, J., Gomez, C., Castro, J., Ramirez, B., Vivar, M., Morales, I., et al. (2014). Effect of extrusion temperature, moisture content and screw speed on the functional properties of aquaculture balanced feed. Emir. J. Food Agric. 26, 659–671. doi: 10.9755/ejfa.v26i8.17133
Mjoun, K., and Rosentrater, K. A. (2011). Extruded aquafeeds containing distillers dried grains with solubles: effects on extrudate properties and processing behaviour. J. Sci. Food Agric. 91, 2865–2874. doi: 10.1002/jsfa.4536
National Research Council. (2011). Nutrient Requirements of Fish and Shrimp. Washington, DC: National Academies Press.
Ozer, E. A., Ibanoglu, S., Ainsworth, P., and Yagmur, C. (2004). Expansion characteristics of a nutritious extruded snack food using response surface methodology. Eur. Food Res. Technol. 218, 474–479. doi: 10.1007/s00217-004-0884-7
Pansawat, N., Jangchud, K., Jangchud, A., Wuttijumnong, P., Saalia, F. K., Eitenmiller, R. R., and Phillips, R. D. (2008). Effects of extrusion conditions on secondary extrusion variables and physical properties of fish, rice-based snacks. LWT Food Sci. Technol. 41, 632–641. doi: 10.1016/j.lwt.2007.05.010
Pitts, K. F., Favaro, J., Austin, P., and Day, L. (2014). Co-effect of salt and sugar on extrusion processing, rheology, structure, and fracture mechanical properties of wheat-corn blend. J. Food Eng. 127, 58–66. doi: 10.1016/j.jfoodeng.2013.11.026
Samuelsen, T., Mjøs, S., and Oterhals, Å. (2013). Impact of variability in fishmeal physicochemical properties on the extrusion process, starch gelatinization and pellet durability and hardness. Anim. Feed Sci. Technol. 179, 77–84. doi: 10.1016/j.anifeedsci.2012.10.009
Samuelsen, T. A., Mjøs, S. A., and Oterhals, Å. (2014). Influence of type of raw material on fishmeal physicochemical properties, the extrusion process, starch gelatinization and physical quality of fish feed. Aquacult. Nutr. 20, 410–420. doi: 10.1111/anu.12093
Samuelsen, T. A., and Oterhals, A. (2016). Water-soluble protein level in fishmeal affects extrusion behaviour, phase transitions and physical quality of feed. Aquacult. Nutr. 22, 120–133. doi: 10.1111/anu.12235
Singh, R. K. R., Majumdar, R. K., and Venkateshwarlu, G. (2012). Optimum extrusion-cooking conditions for improving physical properties of fish-cereal based snacks by response surface methodology. J. Food Sci. Technol. 51, 1827–1836. doi: 10.1007/s13197-012-0725-9
Singh, S., and Muthukumarappan, K. (2016). Effect of feed moisture, extrusion temperature and screw speed on properties of soy white flakes based aquafeed: a response surface analysis. J. Sci. Food Agric. 96, 2220–2229. doi: 10.1002/jsfa.7339
Singha, P., Muthukumarappan, K., and Krishnan, P. (2018). Influence of processing conditions on apparent viscosity and system parameters during extrusion of distiller's dried grains-based snacks. Food Sci. Nutr. 6, 101–110. doi: 10.1002/fsn3.534
Sørensen, M. (2012). A review of the effects of ingredient composition and processing conditions on the physical qualities of extruded high-energy fish feed as measured by prevailing methods. Aquacult. Nutr. 18, 233–248. doi: 10.1111/j.1365-2095.2011.00924.x
Tacon, A. G. (2020). Trends in global aquaculture and aquafeed production: 2000–2017. Rev. Fish. Sci. Aquacult. 28, 43–56. doi: 10.1080/23308249.2019.1649634
Thomas, M., and van der Poel, A. F. B. (1996). Physical quality of pelleted animal feed 1. Criteria for pellet quality. Anim. Feed Sci. Technol. 61, 89–112. doi: 10.1016/0377-8401(96)00949-2
Thompson, S. A., and Williams, R. O. III (2021). Specific mechanical energy-an essential parameter in the processing of amorphous solid dispersions. Adv. Drug Deliv. Rev. 173, 374–393. doi: 10.1016/j.addr.2021.03.006
Umar, S., Kamarudin, M. S., and Ramezani-Fard, E. (2013). Physical properties of extruded aquafeed with a combination of sago and tapioca starches at different moisture contents. Anim. Feed Sci. Technol. 183, 51–55. doi: 10.1016/j.anifeedsci.2013.03.009
Valenzuela, J., Gutiérrez, R., Pacheo, R., Lugo, M., Valdez, J., Reyes, C., et al. (2017). Botanas expandidas a base de mezclas de harinas de calamar, maíz y papa: efecto de las variables del proceso sobre propiedades fisicoquímicas. CyTA J. Food 15, 118–124. doi: 10.1080/19476337.2016.1219391
Vassallo, P., Doglioli, A., Rinaldi, F., and Beiso, I. (2005). Determination of physical properties of feed pellets for Mediterranean aquaculture. Aquac. Res. 37, 1–23. doi: 10.1111/j.1365-2109.2005.01403.x
Yu, L., Meng, Y., Ramaswamy, H., and Boye, J. (2014). Residence time distribution of soy protein isolate, and corn flour feed mix in a twin-screw extruder. J. Food Process. Preserv. 38, 573–584. doi: 10.1111/jfpp.12005
Zarzycki, P., Kasprzak, M., Rzedzicki, Z., Sobota, A., Wirkijowska, A., and Sykut-Domańska, E. (2015). Effect of blend moisture and extrusion temperature on physical properties of everlasting pea-wheat extrudates. J. Food Sci. Technol. 52, 6663–6670. doi: 10.1007/s13197-015-1754-y
Keywords: pellets, Oncorhynchus mykiss, cohesiveness, chemical silage, durability, specific mechanical energy, mean residence time
Citation: Hoyos-Concha JL, Villada-Castillo HS, Roa-Acosta DF, Fernández-Quintero A and Ortega-Toro R (2023) Extrusion parameters and physical transformations of an extrudate for fish: Effect of the addition of hydrolyzed protein flour from by-products of Oncorhynchus mykiss. Front. Sustain. Food Syst. 6:1077274. doi: 10.3389/fsufs.2022.1077274
Received: 22 October 2022; Accepted: 30 November 2022;
Published: 24 January 2023.
Edited by:
Samuel Ayofemi Olalekan Adeyeye, Hindustan University, IndiaReviewed by:
Emmanuel Oke, Federal University of Agriculture, Abeokuta, NigeriaWasiu Awoyale, International Institute of Tropical Agriculture (IITA), Nigeria
Abiodun Aderoju Adeola, Federal University of Agriculture, Abeokuta, Nigeria
Copyright © 2023 Hoyos-Concha, Villada-Castillo, Roa-Acosta, Fernández-Quintero and Ortega-Toro. This is an open-access article distributed under the terms of the Creative Commons Attribution License (CC BY). The use, distribution or reproduction in other forums is permitted, provided the original author(s) and the copyright owner(s) are credited and that the original publication in this journal is cited, in accordance with accepted academic practice. No use, distribution or reproduction is permitted which does not comply with these terms.
*Correspondence: José Luis Hoyos-Concha, jlhoyos@unicauca.edu.co; Rodrigo Ortega-Toro,
rortegap1@unicartagena.edu.co