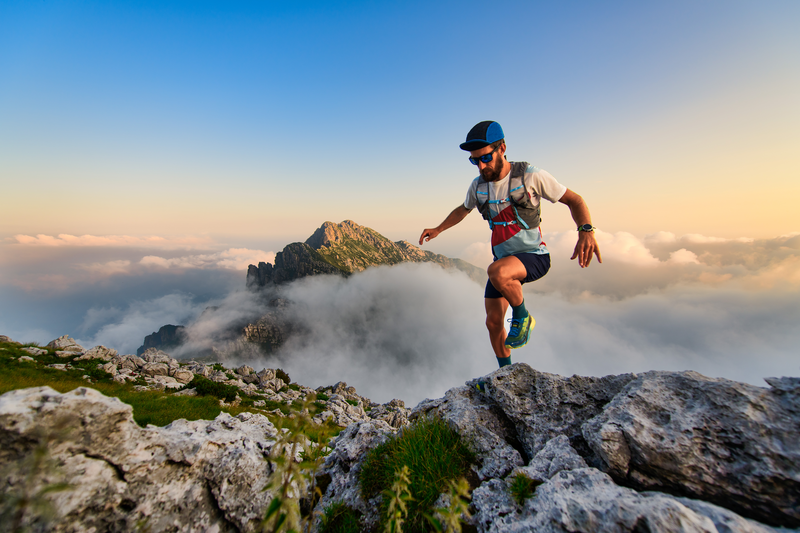
94% of researchers rate our articles as excellent or good
Learn more about the work of our research integrity team to safeguard the quality of each article we publish.
Find out more
ORIGINAL RESEARCH article
Front. Sustain. Food Syst. , 08 December 2022
Sec. Crop Biology and Sustainability
Volume 6 - 2022 | https://doi.org/10.3389/fsufs.2022.1073240
This article is part of the Research Topic Soil Microbiome Metabolomics: A Way Forward to Sustainable Intensification View all 8 articles
Introduction: Wheat (Triticum aestivum L.) is a strategic crop and one of the world's most essential cereals, providing most of the world's calories and protein needs. Drought stress is one of the main limitations for crop production such as wheat in arid and semi-arid regions. Plants can accumulate antioxidants, carbohydrates, and stress hormones that stimulate cell and molecular regeneration under stress conditions. Irrigation saves water, improves crop photosynthesis, and increases plant ability to absorb water and elements from soil. Therefore, irrigation at the right time or supplementary irrigation can help plant growth and crop yield under drought conditions. Appropriate nutrition with fertilizers increases plants' stress tolerance. Bio-fertilizers are restorative elements used in soil to improve tolerance to stresses such as drought stress. A well-known class of bio-fertilizers is plant growth promoting rhizobacteria (PGPR). These rhizosphere bacteria affect plant development and productivity by interacting with roots. Arbuscular mycorrhizal fungi (AMF) alleviate drought stress in plants by enhancing their ability to absorb water and nutrients from the soil. Seaweed extract bio-fertilizer is organic matter used to increase crop growth and soil fertility. This bio-fertilizer is utilized as growth stimulants and food supplements. Our research analyzed the effects of rhizobia and seaweed extracts on wheat's drought resistance mechanisms.
Materials and methods: This research was conducted in Iran in the crop years of 2017–2018 and 2018–2019 in the research farm of Kurdistan University Faculty of Agriculture located in Dehgolan with coordinates 47°18′ 55″ East and 35°19′ 10″ North with an altitude of 1866 meters above sea level, 45 kilometers east It was done on the wheat plant in Sanandaj city. The experiment was conducted in the form of a split-split plot in the form of a randomized complete block design with four replications. Irrigation treatments as the main factor (no irrigation or dry-land, one irrigation in the booting stage, two irrigations in the booting and spike stages), two wheat cultivars (Sardari and Sirvan) as secondary factors, and the application of biological fertilizers at eight levels including Mycorrhiza + Nitrozist and Phosphozist, Seaweed extract + Nitrozist and Phosphozist, Mycorrhiza + Seaweed extract, Mycorrhiza + Nitrozist and Phosphozist and no application of biological fertilizers (control) as Sub-sub-factors were considered.
Results and discussion: According to the study, when bio-fertilizer was applied with once and twice supplementary irrigation levels, leaf relative water content (RWC) and soluble protein content (SPC) increased, while lack of irrigation increased malondialdehyde (MDA). In both years, bio-fertilizers, especially their combinations, increased the amount and activity of enzymatic and non-enzymatic antioxidants, including peroxidase (POD), superoxide dismutase (SOD), phenol (Phe), flavonoid (Fla), and anthocyanin (Anth). Also, it enhanced the inhibition of free radicals by 2-2-Diphenyl picryl hydrazyl (DPPH) and cleared active oxygen species. It was found that malondialdehyde (MDA) levels were very low in wheat under two times irrigation with averages of 3.3909 and 3.3865 μmol g−1 FW. The results indicated a significant positive relationship between non-enzymatic and enzymatic antioxidants such as Phe, Fla, Anth, DPPH, POD, and SOD enzymes and their role in improving stress under dry-land conditions, especially in the Sardari variety. Biological fertilizers (Mycorrhiza + Nitrozist and Phosphozist + Seaweed extract) increased wheat yield compared to the control. Furthermore, Mycorrhiza + Nitrozist and Phosphozist + Seaweed extract improved grain yield by 8.04% and 6.96% in the 1st and 2nd years, respectively. Therefore, appropriate combinations of microorganisms, beneficial biological compounds, and supplementary irrigation can reduce the adverse effects of drought stress in arid and semi-arid regions.
Wheat (Triticum aestivum L.) is a strategic crop and one of the most important grains in the world, which provides about 20% of the calories needed by human and 40% of the protein needs of the world population (Li et al., 2022). Wheat planting is possible in a wide range of climatic conditions. A large part of the lands under wheat cultivation in Iran and many parts of the world is under drought conditions. Therefore, in these areas, wheat is faced water shortages at different stages of growth, limiting its production efficiency and resulting in the wheat yield reduction by 86% (Yang et al., 2021).
Drought stress disrupts plant water exchanges and membrane integrity and increases ion leakage. Germination, growth, production of photosynthetic pigments, and yield potential are reduced under water deficit conditions. Some plants can tolerate drought stress by employing and improving resistance mechanisms (Sanjari et al., 2021). Stresses can induce various cellular and molecular reactions in plant such as accumulation of enzymatic antioxidants, mainly including superoxide dismutase (SOD), catalase (CAT), ascorbate peroxidase (APX), POD, and non-enzymatic antioxidants, including ascorbic acid, carotenoids, phenol compounds, flavonoids along with compatible osmolytes such as proline, glycine betaine and soluble sugars and stress related hormones. These compounds prevent cellular damage against ROS. They also give electrons to ROS and eliminate free radicals, thus protecting cell membranes from damage caused by lipid peroxidation [reduction of malondialdehyde (MDA) production] and also play a key role in plant growth and development (Asghari et al., 2020; Hasanuzzaman et al., 2020; Chiappero et al., 2021).
Supplementary irrigation is carried out in environments with insufficient, uncertain, and unequal annual rainfall distribution (Chaganti et al., 2020) and can be considered as a way to reduce the effects of drought stress on plant growth and yield in these areas. Wang et al. (2021) stated that supplementary irrigation causes a significant increase in wheat grain yield in irrigated cultivars. Yan et al. (2022) stated that irrigation, especially drip irrigation (band tap), leads to saving water consumption, improving wheat plant photosynthesis, promoting dry matter accumulation, grain filling, and increasing wheat yield.
In addition to supplementary irrigation, proper nutrition plays an essential role in plant growth under drought stress. Appropriate nutrition can increase the ability of plants to tolerate environmental stressors (Zhou et al., 2021). Excessive use of chemical fertilizers may reduce the quality of crops, endanger human health, and in the long term, reduce the population of beneficial soil microorganisms. Bio-fertilizers have the potential of reducing demand for chemical fertilizers and increasing the population and diversity of soil microorganisms (Xu et al., 2020). Bio-fertilizers can improve soil fertility and increase the crop production so they can be a good candidate for reducing chemical fertilizers application (Sakara, 2020). Soil microorganisms and bio-fertilizers can also induce plant tolerance to environmental stresses (El-Esawi et al., 2017, 2019; Abd_Allah et al., 2018). Although using bio-fertilizers has a long history in agriculture, interest in these fertilizers has recently increased due to the growing awareness of the harmful effects of chemical fertilizers on the environment and the strong trend toward sustainable agriculture (Altieri, 2019). Inoculation of seeds with bio-fertilizers containing beneficial bacteria, especially bio-fertilizers containing plant growth promoting rhizobacteria (PGPR) improves the physicochemical, biochemical, and biological properties of the soil and improves its yield, enabling the plant to have a better response to environmental stressors and better growth and more performance (Benidire et al., 2021). Under drought stress conditions, PGPR increases the activity of plant antioxidant enzymes to prevent oxidative damage caused by ROS (Zhang et al., 2020). Therefore, using bio-fertilizers containing PGPR can reduce the use of chemical inputs (He et al., 2021).
The effect of Bio-fertilizers containing Rhizobium, Azospirillum, Arthrobacter, Bacillus, Pseudomonas, Enterobacter, and Burkholderia bacteria on plant growth has been proven and are known as PGPR growth-stimulating fertilizers (Goswani and Deka, 2020). Enterobacter cloacae and Serratia marcescens rhizobacteria increase nutrient availability to plants by dissolving insoluble phosphates and fixing nitrogen in the soil (Imade and Babalola, 2021; Safirzadeh et al., 2021). Bio-fertilizers containing Azospirillium stabilize nitrogen in the soil, and the role of these bacteria in reducing drought stress has been well-established (Raffi and Charyulu, 2021). It has been reported that bio-fertilizer containing Azospirillium bacteria increases yield and improves growth parameters of cereals, especially wheat (Raffi and Charyulu, 2021). Researchers reported that inoculating wheat seeds with PGPR bacteria results in exopolysaccharide (EPS) production. EPS is a mixture of polymers with a high molecular weight that sticks to surfaces. Together with other metabolites, EPS helps PGPR strains to survive under drought stress conditions. EPS are more stable if they adhere to the soil particles because they can quickly decompose if they are in their native state. EPS are natural humectants and help to retain water potential, thus its aggregation affects various aspects of soil properties, such as infiltration, root penetration, aeration, and runoff control (Rashid et al., 2021; Latif et al., 2022). In addition, EPS have several times more water-holding capacity than their mass. The production of these compounds enables bacteria to maintain a higher water content in the colonies' microenvironment and helps the wheat plant's survival by reducing the water potential (Rashid et al., 2021; Latif et al., 2022). Arbuscular mycorrhiza fungi (AMF) may have stimulating or inhibitory interactions with various microbes in the root rhizosphere region (Igiehon et al., 2021). AMFs cannot stabilize atmospheric nitrogen (N2) but interact positively with nitrogen stabilizers (Igiehon et al., 2021). Furthermore, AMF in the soil act as microbes that dissolve insoluble phosphates (Adeyemi et al., 2021). One of the obvious benefits of AMF is increasing the plant's ability to absorb phosphorus from the soil. This advantage makes AMF as a key component of sustainable agriculture, reducing the use of phosphorus fertilizers without reducing plant yield (Adeyemi et al., 2021).
Bio-fertilizer compounds of seaweed extract have microbial and antioxidant properties and are used as growth stimulants and dietary supplements. Using these fertilizers may stimulate the plant's immune mechanism and increases disease resistance (Ashour et al., 2020; Morais et al., 2020; Zaki et al., 2021). It has been shown that the bio-fertilizer of seaweed extract improves the growth, yield, and quality of agricultural products (Kumar and Dubey, 2020). The idea of a healthy lifestyle has led to a significant increase in the use of seaweed extract (Sumardianto et al., 2021).
In order to cope with the world-wide environmental stresses and to find solutions against their adverse effects, we investigated the beneficial soil microorganisms and biological compounds in the form of bio-fertilizers together with supplementary irrigation (as another factor to reduce the effects of drought stress) on the growth and yield of two wheat cultivars. Although use of bio-fertilizers has been studied widely so far, here we focused on the protective mechanisms of wheat against drought stress. We especially analyzed the effect of phosphorus and nitrogen solubilizing bacteria, mycorrhiza, and seaweed extract on wheat physiological reactions and the protective mechanisms that two wheat cultivars represent under water shortage conditions.
This research was carried out in the cropping years 2017-2018 and 2018-2019 in the research farm of the Faculty of Agriculture at the University of Kurdistan, located in Dehgolan plain (1,866 m above sea level with a longitude of 47°18′ 55′′ E and 35°19′ 10′′ N). This experiment was performed as a split-split plot in a randomized complete block design with four replications. Different levels of irrigation, including no irrigation (dryland), one irrigation (in the booting stage), and two irrigations (irrigation in flag leaf pod and spike stages), were considered as main plots. Wheat cultivars, including the Sirvan (an irrigated cultivar) and Sardari (a dryland cultivar drought tolerant cultivar), were assigned to sub-plots. Eight combinations of bio-fertilizer, including Mycorrhiza, Nitrozist and Phosphpzist, Seaweed extract, Mycorrhiza + Nitrozist and Phosphozist, Seaweed extract + Nitrozist, and Phosphozist, Mycorrhiza + Seaweed extract, Mycorrhiza + Nitrozist and Phosphozist, and no bio-fertilizers (control) were applied to sub-sub-plots. In this study, the area of sub-plots was 1.20 × 6. Each sub-sub-plot consisted of 8 planting rows with a length of 6 m and row spacing of 20 cm. The distance between the replicates and the main plots was 2 m2. The distance between sub-plots was not planted with three lines, and the distance between sub-sub plots was considered 20 cm (two planting lines).
Before conducting the farm experiments, field soil was sampled in both years to evaluate the physical and chemical parameters of the soil (Table 1). Table 2 also includes meteorological data for the test sites.
Table 2. Monthly precipitation, average maximum and minimum relative humidity (Max RHavg and Min RHavg), average maximum temperature (Max Tavg), average minimum temperature (Min Tavg) for the growing season in 2017–2018 and 2018–2019.
Seeds were inoculated with bio-fertilizers and sown in the farm in early November (both years) at 450 seeds per m2 at a depth of 3 cm. Wheat seeds were received from the Sararud Dryland Agricultural Research Center of Kermanshah Province. Nitrozist liquid bio-fertilizers include a Enterobacter cloacae strain and Phosphozist, is composed of a Serratia marcescens strain (Nozhan 1 and 2). According to the manufacturer's recommendation, 1 L of each biological fertilizer along with 7.5 L of water are required for every 60 kg of seeds. One thousand seeds of cultivars were calculated (42 g for Sardari and 38 g for Sirvan). The amount of seed required for four repetitions was 13.056 and 12 kg for Sardari and Siravan varieties, respectively. Therefore, seeds of Sardari variety, were mixed with 0.2176 ml of Nitrozist (or Phosphozist) and 1.632 L of water. For the Sirvan variety, 0.2 ml of Nitrozist (or Phosphozist bio-fertilizer) and 1.500 L of water was used. Seeds were Inoculated for 8 h and then dried under shade for 2 h and sown immediately. The Knowledge-based Gostar Nozhan 1 and 2 Companies (under the supervision of the Bojnourd University Science and Technology Park) has prepared Nitrozist and Phosphozist fertilizers. Mycorrhiza fungi consisted of spores (150 spores per gram of yeast), hyphae, and root parts, prepared by Knowledge-based Turan Shahroud Biotechnology Company (Under the supervision of Semnan Science and Technology Park). According to the company's recommendation, the amount used was 40 g m−2 of soil, which was used as a strip next to the seeds and as soil inoculation at planting time. Algae extract was sprayed in three growth stages during booting, spiking, and pollination using a hand sprayer. Seaweed extract (Ascophyllum nodosum) (Agri Techno Company, Spain), combined with 7% organic matter, 2.5% potassium, and 16% algae extract, was applied at sunset until wetting all the leaves. Three L of algae extract bio-fertilizer was applied per hectare according to the manufacturer's instructions. Before sowing, 20 kg ha−1 triple super-phosphate and 50 kg ha−1 urea fertilizers were used for chemical soil preparation. Due to the rapid growth of weeds inside and around the plots, manual weed control during the growing season was necessary. Harvest took place in mid-July.
We applied tape drip irrigation for this study. To calculate the irrigation water depth, random soil samples were taken from 0–30 cm of soil profile and quickly transferred to the laboratory in closed containers to maintain the initial moisture and weight. The samples were dried in an oven at 105°C for 24 h. The dry weight of the samples was determined, and the percentage of soil moisture content was obtained from equation 1. By dividing the result of initial soil weight minus soil dry weight on the initial soil weight and then using equation 2, the volume of irrigation water was estimated (Alizadeh and Kamali, 2008).
In the above equation, V is the volume of incoming water per plot (cm3), Fc is equal to the weight percentage of soil moisture at the field capacity, M is equal to the weight percentage of moisture at the time before irrigation, ρa is equivalent to the bulk density (g cm−3), A is equivalent to a plot area (m2), Ds is equivalent to root development depth (cm), and Ea is equivalent to irrigation efficiency estimated in this study 80–91% (Akhavan, 2015). The volume of irrigation water as the main component required in each stage of irrigation treatments was estimated through equations 1 and 2. In order to accurately control the volume of water entering each plot, a volume meter was used.
Evaluated traits include RWC, SOD, and POD activity, MDA, total Phe, Fla, Anth, TFAA, SPC content, DPPH, and grain yield. In each plot to measure the above traits, leaf samples were randomly selected from the mature and green flag leaves of five wheat plants 2 weeks after pollination. Except for grain yield, this coincided with the beginning of grain filling. The leaf samples were wrapped in aluminum foil, immediately placed in liquid nitrogen, transferred to the laboratory, and stored in a freezer at −40°C until testing, except for the RWC and grain yield.
Five fresh leaves to determine the RWC of the leaf 2 weeks after pollination, coinciding with the beginning of grain filling, were separated from the flag leaf of each plot, and four to five pieces with an area of 2 cm2 were measured immediately. Fresh leaves were distilled in water for 4 h and then weighed to record their saturated weight. Then, the samples were dried for 24 h in an oven at 72°C, and the dry leaf weight was recorded. The RWC of the leaf was obtained according to equation 3 (Barrs and Weatherley, 1962).
In this equation, FW is the initial fresh weight, and SW and DW are saturated and dry leaf weights in full amass.
In total 0.3 g of leaf tissue was ground in a porcelain pestle and mortar containing 5 ml of 0.1% trichloroacetic acid (TCA). The resulting extract was centrifuged at 10,000 rpm for 5 min in a refrigerated centrifuge at 4°C. In the following, 0.3 mL of the centrifugal solution was mixed with 1.2 ml of malondialdehyde solution containing 20% trichloroacetic acid (TCA) and 0.5% thiobarbituric acid (TBA). The resulting mixture was heated for 30 min at 95°C in a water bath. It was immediately cooled on ice and centrifuged for 10 min at 10,000 rpm at 25°C. The absorbance of this solution was measured using a UV-2100 spectrophotometer (SUV NEW JERSEYS 2100) at 532 nm. The absorption of other non-specific pigments was determined at 600 nm, subtracted from the resulting value, and calculated using a quenching coefficient of 155 mM cm−1 (Cakmak and Horst, 1991).
Where A532 is absorbance at 532 nm, A600 is absorbance at 600 nm, V represents sample volume (5 cc) and € and W are Extinction coefficient (155 mM cm−1) and Fresh weight of leaf (0.3 g), respectively.
In order to extract and measure the enzyme activity, frozen wheat leaves were ground and powdered using liquid nitrogen in a porcelain mortar. In total 0.5 g of the ground powder was transferred to 2 ml micro-tubes, and 1 ml of 50 mM potassium phosphate buffer (pH 7) was added. The tube was first vortexed and then centrifuged for 15 min at 14,000 rpm at 4°C. Then the supernatant was removed, and 1,000 μl of the supernatant was mixed with 350 μl of 50% glycerol. The mixture was transferred to 10 to 12 500 μl micro-tubes in 100 μl volumes. Then, the micro-tubes were stored in liquid nitrogen (Beauchamp and Fridovich, 1971).
In order to measure the activity of the SOD enzyme, the soluble proteins of the samples were extracted, and the protein concentration was determined by Bradford (1976) method. Subsequently, the activity of the SOD enzyme was measured according to Dhindsa and Matowe (1981). The method relies on inhibiting the SOD enzyme from nitroblutrazole (NBT) optical reduction as its basis for enzyme measurement. Briefly, the reaction solution, including 3 ml of 50 mM phosphate buffer pH 7.8, 13 mM mentionin, 75 μL NBT, 2 μM riboflavin, 0.1 ml EDTA, and 50 μL from the extracted protein extract, was mixed and poured into a micro-tube. Finally, riboflavin was added, and the microtubes were shaken for 10 min at a distance of 30 cm from a 15-watt fluorescent lamp. A sample without an enzyme was considered as a control. Absorption at 560 nm was measured with a NEW JERSEYS 2100 SUV spectrophotometer (UV-2100), and then, the activity of SOD was calculated using equation 5 in U mg−1 protein.
Amax = adsorption in the control sample.
To measure the peroxidase activity, 780 μl of 50 mM potassium phosphate buffer (pH 7) and 90 μl of Guaiacol (1%, 90 μl of 0.3% hydrogen peroxide) were mixed in a 1 ml cuvette on ice, and 40 μl of extracted protein was added to it. The absorption change curve was generated at 470 nm with a UV-2100 spectrophotometer (model SUV NEW JERSEYS 2100). Peroxidase activity was reported based on equation 6 in U min−1 mg−1 protein (Hemeda and Klein, 1990).
4 = Molecular coefficient of reaction components.
Vt = Volume of the reaction mixture.
Df = Diluting factor (sterile distilled water).
Vs = Volume of enzyme extract.
ε = Extinction coefficient (26.6 mM−1 cm−1).
T = Time (120 sec).
l = The length of the path of light passing through the reaction mixture.
The amount of total phenolic compounds was determined with the Folin-Ciocalteu reagent. One g of the leaf was ground in 10 ml of methanol for 2 min, and the solution was filtered through filter paper. To 0.5 ml of diluted extract (10 ml g−1 1:10) 5 ml of diluted fullene (1:10 diluted with distilled water), and then 4 ml of aqueous Na2CO3 (M1) was added, and the samples remained for 15 min at room temperature, and its adsorption was read at 760 nm using a NEW JERSEYS 2100 SUV spectrophotometer (UV-2100 model). The standard curve was prepared with different concentrations of gallic acid (150–1,000 μg ml−1 and 200–1,000 μg ml−1) in ethanol solution. Total Phe values equivalent to gallic acid (mg g−1 FW) were reported (Singleton et al., 1999).
In order to measure the amount of flavonoids, 0.5 g of the frozen flag leaf sample was ground with liquid nitrogen. Then, 10 ml of acidic methanol was added, and the solution was centrifuged for 15 min at 12,000 rpm. Then one ml of the solution was diluted with 4 ml of distilled water, and 0.3 ml of NaNO2 solution was used. The solution was kept at room temperature for 5 min, and 0.6 ml of AlCl3 was dissolved. After 6 min of remaining at room temperature, 2 ml of normal sodium was added. Finally, it was reduced to 10 ml, and a spectrophotometer read the absorbance of the solution at 510 nm (Zhishen et al., 1999).
In order to measure anthocyanin, acidic methanol was used for extraction. The resulting solution was centrifuged for 15 min at 4°C and 17000 rpm. The amount of total anthocyanin was measured using the adsorption difference method at different pH, and sample adsorption (A) was measured at 520 and 700 nm in buffers with pH equal to 1 and 4.5. It was necessary to prepare buffer one (pH 1) using a base solution containing 0.2 M potassium chloride (a gram of potassium chloride in one liter of distilled water, pH was adjusted to one by concentrated HCl). Buffer two (pH 4.5) with a base solution containing 0.2 M acetic acid (which required a gram of sodium acetate in 1 L of distilled water, pH with concentrated HCl was set to 4.5) was prepared. The dilution was determined by factor dilution or DF (1: 5 by volume). The amount of total anthocyanin was calculated according to the following formula (Giusti and Wrolstad, 2000). Anthocyanin content was expressed as mg g−1 FW and equivalent to cyaniding-3 glucoside.
Where:
A: The absorbance value of the sample.
ϵ: Absorbance of molar Cyanidin-3 glycoside (26,900 mol/cm).
L: Wide cuvette (1 cm).
MW: Molecular weight of Cyanidin-3-glycosides (449.2 g/mol).
DF: Diluent factor.
In total 0.1 g of frozen flag leaf sample was pulverized with liquid nitrogen to measure the content of total free amino acids. Then 10 cc of potassium phosphate buffer (pH = 6.8 0.5 M) was added, and the samples were centrifuged (refrigerated) at 4°C at 13,000 rpm for 20 min. After that, two cc of the centrifuged extract was removed and poured into glass tubes, and 400 μl of ninhydrin reagent was added to it and placed in a Ben Marie at 90°C for 5 min. After cooling the samples (for 30 min), light absorption was determined at a wavelength of 570 nm. By drawing a standard curve using Glycine, the number of free amino acids based on micrograms per wet weight was reported (Harding and MacLean, 1916).
As a test for antioxidant activity, diluted methanol extract from 5 times was placed into a test tube and the DPPH (2, 2-Diphenyl-Picryl-Hydrazyl) was monitored. Then, 2,000 μl of the pre-prepared DPPH solution was added to the desired extract. The resulting solution was shaken and kept at room temperature for 30 min. The adsorption was read at 515 nm in the spectrophotometer in the following. The control sample (blank) was prepared according to the above method, in which 50 μl of 80% methanol was used instead of the extract (Huang et al., 2005). Finally, the numbers read from the sample adsorption were converted to 5% by formula ratio inhibition in (relation 9), where Abs control is the amount of control adsorption, and Abs sample is the amount of sample adsorption. The percentage of free radical scavenging (DPPH) was calculated using the following formula.
In total 0.5 g of fresh leaves were ground in a porcelain mortar with liquid nitrogen and pulverized to measure soluble proteins content. Afterwards, 1.5 ml of potassium phosphate buffer (PH = 7) with sodium meta sulfide (0.01901 g per 100 - 0.01901 g−1 of the buffer) was added, and the leaf crushing was continued until homogenization. The resulting mixture was poured into 2 ml micro-tubes and centrifuged for 15 min at 15,000 rpm in a refrigerated centrifuge at 4°C. After centrifugation, the supernatant containing the soluble proteins of the sample was gently removed with a sampler, and 500 μl of the extract was mixed with 175 μl of 50% glycerol. This solution was divided into seven micro-tubes of 200 μl. The micro-tubes containing the extract were immediately transferred to a freezer at −40°C and kept until the protein content and enzymatic activity were determined. This method is based on binding Co-maxi Briant Blue G 250 dye in an acidic reagent to a protein molecule.
In total 0.01 g of Co-maxi Bryant Blue G 250 in 5 ml of 96% ethanol was well-dissolved in the dark with the help of a magnetic stirrer. Then 10 ml of 85% phosphoric acid was added drop by drop to the above mixture. After stirring, the final volume of the solution was distilled to 100 ml. Then, 40 μl of the extract was mixed with 960 μl of Bradford solution to measure the protein concentration. After 5 min, the absorption of soluble light at 595 nm was read with the UV-2100 spectrophotometer model SUV NEW JERSEYS 2100. Bovine Serum Albumin (BSA) was used to draw the standard curve. According to the standard curve and the amount of light absorption at the wavelength of 595 nm, the sample protein was determined in mg g−1 FW (Bradford, 1976).
To measure grain yield, one square meter (m2) plant in the middle of each plot, which represented the conditions of the whole plot, was harvested at full maturity (July to mid-July). In the harvested samples, after threshing the spikes, the grain was separated from the straw, the grain yield was weighed, and the grain yield was calculated in kg ha−1 (Bukhari et al., 2021).
Analysis of variance was performed by SAS statistical software version 9.4, and means were compared by the LSD method. Normality and variance uniformity of residuals were tested using Minitab (version 17) software. After performing the variance uniformity test of experimental errors with the Bartlett test, the Bartlett test showed that for all traits except soluble protein and total Phe, the variance was not uniform between 2 years; hence the data were analyzed separately by year. Graph Pad Prism software version 9 was used to draw graphs.
At the Dehgolan research station, the average maximum and minimum air temperature before the onset of winter in the 1st year was higher than in the 2nd year (Table 2). This subject is about damage to young plants at pre-winter sowing dates due to exposure to unexpected cold and the sensitivity of young seedlings to low temperatures without cover. Therefore, more cold in the 2nd year of the experiment will weaken the plants and eliminate them. As a result of meteorological data, the average relative humidity and rainfall were higher during sensitive growth stages of wheat in the 1st year (pollination, milking, grain filling, i.e., 11 May to 10 June) than those of the 2nd year. Therefore, the 1st year of autumn and winter was warmer, spring and summer were relatively cooler, and the wheat plant was in better condition in the 1st year (Table 2).
The analysis of variance of the leaf RWC data from the 1st and the 2nd year of the experiment showed that the simple effects of irrigation, cultivar, bio-fertilizer, and the double interaction of irrigation × bio-fertilizer are significant (Supplementary Tables 1, 2).
RWC for Sirvan was estimated as 78.17 and 73.06% for the 1st year and the 2nd year, respectively, which were lower than those of Sardari cultivar (with an average of 80.23 and 75.64%, respectively) (Figures 1A,B). Mean comparisons showed that water shortage in both years reduced the leaves RWC while increased irrigation times improved the plant water content. Also, bio-fertilizers significantly increased the RWC in all irrigation treatments. In both years, the lowest RWCs (with an average of 63.93 and 57.73%) were obtained under non-application of fertilizers treatment and dryland conditions. The highest RWC in the 1st and 2nd years (91.9 and 88.6%, respectively) was observed for the bio-fertilizers treatment (Mycorrhiza + Nitrozist and Phosphozist + Seaweed extract) under two irrigation conditions. In other words, the combined application of bio-fertilizers with two supplementary irrigations compared to dryland conditions without bio-fertilizer in the 1st and 2nd years increased the RWC by about 44 and 53%, respectively (Tables 3, 4).
Figure 1. Mean comparisons main effects of cultivars (A,B) on relative water content in wheat plant in the years 2017–2018 and 2018–2019. **0.001 < p ≤ 0.01.
Table 3. Mean comparisons of the dual interactions of irrigation levels and bio fertilizers on RWC, SOD, Anth, and TFAA traits in 2017–2018.
Table 4. Mean comparisons of the dual interactions of irrigation levels and bio fertilizers on RWC, Anth, TFAA, and DPPH traits in 2018–2019.
The fundamental impacts of irrigation levels, cultivars, and bio-fertilizers on MDA levels in the 1st and 2nd years of the experiment are shown in Supplementary Tables 1, 2. Supplementary irrigation decreased MDA content in wheat, with an average of 1.71616 and 1.8974 μmol g−1 FW in the 1st and 2nd years of the experiment, respectively, compared to no irrigation with an average of 3.3909 and 3.3865 μmol g−1 FW in the 1st and 2nd years of the trial (Figures 2A,B). The results of comparing the mean of the data revealed that the quantity of MDA in the 1st and 2nd years of the experiment in the Sirvan cultivar was 2.7554 and 2.7987 μmol g−1 FW, respectively, in comparison to the Sardari cultivar, which was 2.2873 and 2.4159 μmol g−1 FW, respectively (Figures 2C,D). The results (Figures 2E,F) also revealed that in the 1st and 2nd years, the non-fertilizer treatment produced the most MDA in wheat (an average of 2.6641 and 2.6577 μmol g−1 FW, respectively), while the applications of Mycorrhiza + Nitrozist and Phosphozist + Seaweed extract produced the least (an average of 2.3891 and 2.5601 μmol g−1 FW, respectively).
Figure 2. Mean comparisons of main effects of irrigation levels (A,B) on malondialdehyde, mean comparisons of main effects of cultivars (C,D) and bio-fertilizers (E,F) on malondialdehyde in wheat plant in the years 2017–2018 and 2018–2019. At p > 0.05, ns, not significant; and **0.001 < p ≤ 0.01.
Based on the analysis of variance (Supplementary Tables 1, 2), irrigation, cultivar, and bio-fertilizer significantly impacted wheat SOD activity in the 1st and 2nd years. In the 1st year of the experiment, the dual interaction of irrigation levels × bio-fertilizers affected the activity of the above-mentioned enzyme at a probability level of 1% (Supplementary Table 1). In the 1st year of the experiment (Table 3), it was found that SOD activity was high in dryland conditions and that supplementary irrigation, especially two irrigations, significantly reduced enzyme activity. At all irrigation levels, the application of bio-fertilizers, especially the combination of bio-fertilizers (Mycorrhiza + Nitrozist and Phosphozist + Seaweed extract), improved the enzyme activity compared to untreated plants (Table 3). In the 1st and 2nd years of the experiment (Figures 3B,C), the lowest SOD activity with an average (of 6.2892 and 7.6846, respectively) was observed in the Sirvan cultivar. In contrast, the highest enzyme activity was observed in the Sardari cultivar with an average (of 7.7106 and 9.9471, respectively), indicating a difference of 22.60 and 29.44%, respectively. A comparison of means in the 2nd year of the experiment showed (Figure 3A) that the highest amount of SOD was related to the control treatment of 11.9215 U mg−1 protein, and the lowest was related to two irrigations of 5.6976 U mg−1 protein. In wheat, a significant difference was found between bio-fertilizers (Mycorrhiza + Nitrozist and Phosphozist + Seaweed extract) (with an average of 8.9633 U mg−1 protein) and the control (with an average of 8.6354 U mg−1 protein) indicating 3.8% more SOD activity in the plant as compared to the control (without any bio-fertilizers) (Figure 3D).
Figure 3. Mean comparisons of main effects of irrigation levels (A) on malondialdehyde (2018–2019), main effects of cultivars (B,C) in the years 2017–2018 and 2018–2019, and bio-fertilizers (D) in the year 2018–2019 on superoxide dismutase activity in the wheat plant. At p > 0.05, ns, not significant; and **0.001 < p ≤ 0.01.
Based on the results of this study, in the 1st and 2nd years of the experiment, the effects of simple irrigation, cultivars, and bio-fertilizers on POD activity were notable (Supplementary Tables 1, 2). In the first-year experiment, the triple interaction of bio-fertilizer × irrigation levels × cultivar was significant at a 5% probability level (Supplementary Table 1). In the second-year experiment, the dual interactions of irrigation × cultivar and bio-fertilizer × irrigation were significant at the 1% probability level (Supplementary Table 2). Based on the results of the first-year experiment, the highest POD activity was observed in dryland conditions using Mycorrhiza + Nitrozist and Phosphozist + Seaweed extract in the Sardari cultivar (0.4625 U min−1 mg−1 protein) and the lowest (0.0646 U min−1 mg−1 protein) in two supplementary irrigation conditions in the Sirvan cultivar (Table 6). In the 2nd year of the experiment, the highest POD activity was obtained in Sardari and Sirvan cultivars in dryland conditions (0.4973 and 0.4853 U min−1 mg−1 protein, respectively), and the least amount was found under two irrigation conditions in Sirvan cultivars and Sardari (0.1116 and 0.1192 U min−1 mg−1 protein, respectively) were obtained (Table 5). The 2nd year of experimenting with different fertilizer treatments in two cultivars significantly increased POD activity. In both Sardari and Sirvan cultivars, the maximum activity of this enzyme was obtained in the simultaneous use of bio-fertilizers Mycorrhiza + Nitrozist and Phosphozist + Seaweed extract (0.3074 and 0.2712), and the lowest activity was obtained in the absence of fertilizer (0.2801 and 0.2681) (Table 10).
Table 5. Mean comparison of the dual interaction of irrigation levels and cultivar on Fla and Anth content, DPPH, and POD activity traits in 2 years of experiment (2017–2018 and 2018–2019).
Based on the results of a combined analysis of variance of the data, the interactions of irrigation levels × cultivar, irrigation levels × bio-fertilizer, and cultivar × bio-fertilizer on Phe content were significant (Supplementary Table 3). A comparison of means (Table 7) showed that the lowest amount of Phe (with an average of 69.8130 mg GAE g−1 FW) belonged to the Sirvan cultivar treated with twice irrigation and the highest (with an average of 25,725.6450 mg GAE g−1 FW) belonged to the Sardari cultivar under dryland conditions. The interaction of cultivars and bio-fertilizers on Phe content found that in both Sardari and Sirvan cultivars, the application of bio-fertilizers increased the amount of Phe than in the control treatment. The Sardari cultivar had the highest level (with an average of 173.4300 mg glycol acid in wet weight) under combined application of bio-fertilizers. Sirvan cultivar without application of bio-fertilizers had the lowest Phe content (average of 128.56) (Table 9). Interaction of irrigation levels × bio-fertilizer showed that the application of bio-fertilizers at all irrigation levels increased the content of Phe compounds in the plant. The lowest amount of Phe in the treatment of twice irrigation and non-application of bio-fertilizers with an average of 75.8370 mg GAE g−1 FW and the highest amount of Phe in dryland conditions and the application of bio-fertilizers Mycorrhiza + Nitrozist and Phosphozist + Seaweed extract with an average of 232.8850 mg GAE g−1 FW was obtained (Table 8).
Analysis of variance in both years of the study showed (Supplementary Tables 1, 2) that the simple effects of irrigation levels, cultivar, and bio-fertilizer treatment on wheat Fla content were significant at a probability level of 1% (p ≤ 0.01). In the 1st year of the study (Supplementary Table 1), the interaction between irrigation and cultivar significantly affected Fla content at a probability level of 5% (p ≤ 0.05). Table 5 shows that in the 1st year of the experiment, the lowest amount of flavonoids in Sardari and Sirvan cultivars, with an average of 11.8486 and 9.0837 mg g−1 FW, was observed under the two irrigation conditions. In contrast, the highest amount of Fla, averaging 30.5156 and 25.6479 mg g−1 FW, belonged to non-irrigation treatment. Comparison of means (Figures 4C,D) revealed that Mycorrhiza + Nitrozist and Phosphozist + Seaweed extract in both years (with an average of 19.6292 and 21.1625 mg g−1 FW, respectively) increased the amount of Fla compared to the non-fertilizer treatment (with an average of 18.7931 and 20.394 mg g−1 FW, respectively), which had the lowest amount of Fla. The results of the 2nd year of the experiment showed that the highest amount of Fla (30.2959 mg g−1 FW) was obtained in dryland treatment, and the lowest amount of Fla was obtained in two irrigation conditions (11.3830 mg g−1 FW) (Figure 4A). In the 2nd year of the study, the Sardari cultivar had more Fla (22.12125 mg g−1 FW) than the Sirvan cultivar (18.6935 mg g−1 FW) (Figure 4B).
Figure 4. Mean comparisons of main effects of irrigation levels (A) and cultivars (B) in the year 2018–2019 and bio-fertilizers (C,D) on flavonoid content in wheat plants in the years 2017–2018 and 2018–2019. At p > 0.05, ns, not significant; and **0.001 < p ≤ 0.01.
The simple effects of irrigation levels, cultivars, and bio-fertilizers and the dual effects of irrigation levels × bio-fertilizers, and bio-fertilizer × cultivars were significant at a probability level of 1%, according to the results of the analysis of variance of the 1st- and 2nd-year data (Supplementary Tables 1, 2). The triple interaction of irrigation levels × bio-fertilizers × cultivars was significant at a probability level of 1% in the 1st year of the experiment (Supplementary Table 1). The results of the 2 years experiment revealed that reducing the number of irrigations increased the Anth content in both wheat cultivars, with the highest Anth content obtained in dryland conditions (Tables 5, 6). On the other hand, bio-fertilizers dramatically increased wheat Anth content at all irrigation levels (Tables 3, 4). Bio-fertilizer treatment enhanced Anth content in both cultivars in both years (Tables 6, 10). The results showed that the highest amount of Anth was obtained in the Sardari cultivar and the application of bio-fertilizers of Mycorrhiza + Nitrozist and Phosphozist + Seaweed extract in both years of the experiment. This quantity climbed by 104% in the 1st year compared to the control and 138% in the 2nd year (Tables 6, 10).
Table 6. Mean comparison of the interaction of Irrigation levels × Cultivars × Bio-fertilizers on POD activity and Anth content of wheat in 2017–2018.
According to the analysis of variance done in the 1st and 2nd years of the study (Supplementary Tables 1, 2) irrigation, and bio-fertilizers, as well as their interactions, exhibited statistical significance at a probability level of 1%. Based on the analysis of variance in the 1st year of the experiment, the simple effect of the cultivar on the concentration of free amino acids was shown to be significant at the 1% probability level (Supplementary Tables 1, 2). According to the mean comparison data, reducing the number of irrigations increased the content of TFAA in wheat. In both years, the highest amount of this trait was obtained in dryland conditions (Tables 3, 4). Using bio-fertilizers at all irrigation levels raised the TFAA content of the plant. The highest content of TFAA content was obtained in plants treated with the application of bio-fertilizers (Mycorrhiza + Nitrozist and Phosphozist + Seaweed extract) applied under dryland conditions, and supplementary irrigation reduced the values of the trait (Tables 3, 4). A means comparison in the 1st year of the experiment (Figure 5A) revealed that the Sardari cultivar (95.7236 mg g−1 FW) generated greater free amino acid content than the Sirvan cultivar (81.7172 mg g−1 FW).
Figure 5. Mean comparisons of main effects of irrigation levels (A) on 2-2-Diphenyl picryl hydrazyl (DPPH), mean comparisons of main effects of cultivars (B,C) on total free amino acids and 2-2-Diphenyl picryl hydrazyl (DPPH) and Mean comparisons of main effects of bio-fertilizers (D) on 2-2-Diphenyl picryl hydrazyl (DPPH) in wheat plant in years 2017–2018. **0.001 < p ≤ 0.01.
According to the analysis of variance of the experiment's 1st- and 2nd-year data, the separate effects of irrigation levels, cultivars, and bio-fertilizers on DPPH were significant at the level of 1% probability (Supplementary Tables 1, 2). Based on the variance analysis of the 2nd year's data, the interaction of irrigation levels × cultivar and the interaction of irrigation levels × bio-fertilizers on DPPH was significant at a 1% probability level (Supplementary Tables 1, 2). The application of bio-fertilizers in the 1st year of the experiment showed the highest amount of DPPH in the treatment of the combined application of Mycorrhiza + Nitrozist and Phosphozist + Seaweed extract with an average of 57.9221%. This year, the effect of applying these combined fertilizers (Mycorrhiza + Nitrozist and Phosphozist + Seaweed extract) increased DPPH by 14.94% in comparison to the control (with an average of 50.3946%) (Figure 5D). In the 1st year of the study, the amount of DPPH decreased with the number of irrigation. Therefore, two irrigation methods, compared to dryland conditions, showed a decrease of 57.88%. Drought stress increased DPPH in comparison to plants under double irrigation conditions (Figure 5B). Comparing the DPPH of the Sardari cultivar (58.7098%) with that of the Sirvan cultivar (50.1764%) in the 1st year of the experiment, we found that the Sardari cultivar had 17% more DPPH (Figure 5C). This superiority was also observed in the 2nd year (Table 5). In the 2nd year of the experiment, increasing irrigation frequency in both Sardari and Sirvan cultivars decreased DPPH (Table 5). In the 2nd year, the highest amount of DPPH (80.9933%) was obtained under dryland conditions and in the application of integrated bio-fertilizer treatment, and the lowest amount (43.7569%) was obtained under the conditions of double irrigation and non-use of bio-fertilizers (Table 4).
The results of a combined analysis of variance showed that the effects of simple irrigation and bio-fertilizer and the interaction effects of irrigation × cultivar and irrigation × bio-fertilizer on the SPC of leaves were significant (Supplementary Table 3). The results of comparing the mean of the data (Table 7) show that the highest amount of protein in both Sirvan and Sardari cultivars was related to two irrigations (11.21 and 9.89 mg g−1 FW, respectively) and the lowest amount in non-irrigation conditions (dryland) (5.69 and 6.47 mg g−1 FW, respectively) were obtained. As seen in Table 8, the application of bio-fertilizers, especially in the combined case, increased the content of soluble leaf proteins compared to the control treatment (no fertilizer use). When bio-fertilizer (Mycorrhiza + Nitrozist and Phosphozist + Seaweed extract) was used in two supplementary irrigation conditions, the SPC was up by 44.30 and 79.8% compared to one supplementary irrigation and no irrigation when bio-fertilizers were not used. Also, bio-fertilizers (Mycorrhiza + Nitrozist and Phosphozist + Seaweed extract) and supplemental irrigation increased the SPC by 29.55% compared to the treatment without irrigation (dryland and no bio-fertilizers) (Table 8).
Table 7. Mean comparisons of the dual interaction of cultivar and irrigation levels on phenol and soluble proteins content traits 2 years of experiment (2017–2018 and 2018–2019).
Table 8. Mean comparisons of the dual interactions of irrigation levels and bio-fertilizers on phenol and soluble proteins content traits in 2 years of experiment (2017–2018 and 2018–2019).
The two irrigation treatments had the highest grain yield in the 1st and 2nd year of the experiment, with an average of 6,250.38 and 5,927.19 kg ha−1, respectively. The dryland treatments had the lowest grain yield, with an average of 3,481.88 and 3,317.03 kg ha−1, respectively (Figures 6A,B). As seen in the experiment's 1st and 2nd years, grain yield was significantly different between Sardari and Sirvan cultivars. In both years of research, the Sirvan cultivar was found to have the most grain (Figures 6C,D). Based on the results presented in Figures 6A,B, grain yield was significantly affected by applying bio-fertilizers. All biofertilizers had different effects on grain yield in the 1st and 2nd years. Mycorrhiza + Nitrozist and Phosphozist + Seaweed extract increased grain yield by 8.456 and 6.9106%, respectively, compared to the control (Figures 6E,F).
Figure 6. Mean comparisons main effects of irrigation levels (A,B), cultivars (C,D) and bio-fertilizers (E,F) on grain yield in wheat plant in years 2017–2018 and 2018–2019. At p > 0.05, ns, not significant; and **0.001 < p ≤ 0.01.
One of the most severe abiotic stresses is drought stress, a severe obstacle to increasing agricultural production and has become more problematic today due to climate change (Naderi et al., 2020). Drought stress covers a wide range of plant responses, from cellular metabolism to changes in growth rate and crop yield (Anjum et al., 2017). These changes in the plant were associated with a decrease in its water content because the RWC reflects the water status of the plant and is one of the indicators of drought resistance (Khan and Bano, 2019; Rashid et al., 2021). In the present experiment, water shortage in both years reduced the leaves' RWC, while frequent irrigation improved plant water content (Figures 1A,B). In confirmation of our results, a study on wheat showed that drought stress reduced RWC, while under sufficient water supply conditions, a significant increase in RWC was observed (Wasaya et al., 2021).
In the present study, in addition to supplementary irrigation, using bio-fertilizers, especially the combination of Mycorrhiza + Nitrozist and Phosphozist + Seaweed extract, also enhanced RWC (Tables 3, 4). It could be due to the positive effects of these fertilizers on root volume and the ability of roots to absorb more water. Consistent with our results in other studies on the wheat plant, the application of PGPR bio-fertilizers has increased RWC under drought-stress conditions (Khan and Bano, 2019; Rashid et al., 2021). The existence of osmotic regulation mechanisms in wheat plants under drought stress, such as increasing the content of Anth (Tables 5, 6) and TFAA (Tables 3, 4), induces maintaining and higher keeping RWC in the plant. Increased content of soluble sugars, total free amino acids, and proline as osmotic protectors under drought stress conditions has been reported in other studies (Rady et al., 2020). Using bio-fertilizers has positive effects, such as enhancing the hydraulic conductivity of roots and plant organs, which, together with maintaining the pressure of cellular inflammation (turgor) as a result of the accumulation of compatible osmolytes, maintains RWC and resistance to drought stress. PGPR bio-fertilizers with in-dole acetic acid (IAA) excretion strengthen and expand the roots to further absorb water (Yasmin et al., 2017; Ambreetha et al., 2018). Bio-fertilizers containing PGPR also protect the plant from drying out by producing EPS from bacterial cells and improving water-holding capacity (Rashid et al., 2021). In addition, the increase of RWC by bio-fertilizers containing PGPR can be attributed to increasing water and nutrient uptake from the soil, production of volatile organic compounds, EPS, changes in the content of plant hormones such as abscise acid (ABA), in-dole acetate (IAA), gibberellin acid (GA) and ethylene (Oleńska et al., 2020). Fiorilli et al. (2022) stated that the higher RWC in wheat plants inoculated with mycorrhiza might be due to factors such as the high ability of mycelium hyphae to increase soil water availability, and water uptake with low potential in the rhizosphere region, greater hydraulic conductivity, enhanced uptake of nutrients, osmotic adjustment, and the higher ability to adjust stomata.
Water scarcity stress and often abiotic stresses induce oxidative stress in plants by disrupting electron transfer and the function of optical photosystems, which in turn increases the amount of ROS such as superoxide, H2O2, and OH−. ROS reduces photosynthesis, plant growth, and yield by oxidizing lipids in biological membranes, damaging chloroplast structure and reducing photosynthetic pigment content in plants (Anjum et al., 2017). In the present study, the amount of H2O2 (data not shown) and MDA (Figures 2A,B) was increased in dryland and water shortage conditions. At the same time, the application of supplementary irrigation and bio-fertilizers decreased MDA in wheat (Figures 2A,B,E,F). Under stress conditions, ROS are produced, and ROS-induced oxidative damage to membrane lipids produces MDA, which is the product of membrane lipid peroxidation (Naliwajski and Skłodowska, 2021). Elkelish et al. (2021), in a study, stated that drought stress increased H2O2 in wheat. Increasing membrane lipid peroxidation decreased the membrane stability index, led to membrane electrolyte leakage, and enhansed the production of MDA. An increase in protective antioxidants limits the overproduction of toxic molecules (ROS) under stress conditions. Plants need to eliminate and detoxify ROS as part of their drought tolerance, and the production of enzymatic and non-enzymatic antioxidants plays a role in plants' defense against drought. Plants have a wide range of antioxidants, each specific to a specific ROS (Baruah et al., 2021). The first plant defense system against oxidative stress is SOD, which continuously detoxifies excess O−2 in various organs such as chloroplasts, apoplasts, cytosols, mitochondria, and peroxisomes and converts O−2 to H2O2 (García et al., 2020). Then other enzymes, such as CAT (peroxisomes and mitochondria) and peroxidase (cell walls), can then convert H2O2 to H2O and O2 (Liu et al., 2019; Xie et al., 2019). The second group of antioxidants is non-enzymatic such as ascorbic acid, carotenoids, phenolic compounds, Fla, and Anth (Chiappero et al., 2021). Wheat plants also increased their antioxidant activity in response to drought stress and to counteract it. In contrast, in more favorable conditions of water access and supplemental irrigation, the reduced production of ROS and their destructive effects did not require an increase in the production and activity of these enzymes (Tables 3, 5, 6, 8). Secondary metabolites such as Phe, Fla, and Anth, while acting as antioxidants in inhibiting ROS, can act as an osmotic solution in drought or water scarcity conditions and accumulate in young and growing leaves (Baruah et al., 2021). Osmolytes and secondary metabolites such as soluble carbohydrates, soluble proteins, and proline play key roles in osmotic regulation under stress conditions (Amjad et al., 2021; Noreen et al., 2021). In a study, (Naderi et al., 2020) reported that the wheat plant reduced its ROS by using defense systems such as antioxidants and phenolic compounds and then used mechanisms such as increasing lignin to strengthen cell walls, which can be effective in improving wheat drought tolerance.
The present study showed that bio-fertilizers reduced wheat's H2O2 (Najafi vafa et al., 2022) and MDA (Figures 2E,F) content. The amount of H2O2 indicates the balance between the production and decomposition of ROS. Bio-fertilizers increased the percentage of DPPH (Figure 5D and Table 4). A reduction in H2O2 and MDA content was also achieved by raising the amounts and activities of non-enzymatic antioxidants such as Phe (Tables 8, 9), Fla (Figures 4C,D) and enzymatic antioxidants such as POD (Table 3) and SOD (Tables 6, 10) due to the bio-fertilizer application. Increased DPPH under stress conditions has produced a positive property for eliminating O−2 radicals (Patil et al., 2021). As a confirmation of the results of the present study, another study on wheat has shown that PGPR application under drought stress conditions decreases membrane lipid peroxidation by reducing H2O2 and MDA, maintaining cellular redox balance and, therefore, preventing electrolyte leakage in treated plants (Ahmad et al., 2019; Omara et al., 2022). Benaffari et al. (2022) reported that the production of secondary metabolites in quinoa inoculated with mycorrhiza fungi under drought stress improves the plant's capacity to inhibit ROS. They believe that in inoculated plants, increasing the activity of SOD, APX, POX, and PPO enzymes in leaves and roots with less H2O2 and MDA content than in uninoculated plants protects the plant against drought stress. Bitaraf et al. (2020) and Zou et al. (2021) stated that wheat plants treated with Glomus mosseae mycorrhiza under water deficiency had a higher content of non-enzymatic antioxidant secondary metabolites such as Fla, Anth, and total Phe compounds than uninoculated wheat. Pan et al. (2019) observed that inoculating corn with Azospirillium significantly reduced the amount of MDA in the plant. This shows that Azospirillium reduces oxidative stress and subsequent MDA, which ensures the integrity of the membrane and prevents the leakage of critical ions. In confirmation of the results of our research, (Ansari et al., 2021) also stated that the biochemical parameter of MDA in wheat fertilized with bio-fertilizers containing PGPR was significantly lower compared to the control (non-inoculation). In a study by Trivedi et al. (2018), MDA content in maize plants under drought stress increased in comparison to plants under a supplementary irrigation regime. In this study, the use of algae extracts compared to the control plant caused a significant reduction in MDA content, O−2 concentration, hydroxyl radical, and H2O2 content, which is completely consistent with the results of the present study.
Table 9. Mean comparisons of the dual interactions of cultivar and bio-fertilizers on Phenol content in 2 years of experiment (2017–2018 and 2018–2019).
Table 10. Mean comparisons of the dual interactions of bio-fertilizers and cultivar on anthocyanin content and peroxidase activity in 2018–2019.
A study on the wheat plant (Spagnoletti et al., 2021) showed that under stress conditions, the amount of SOD activity in plants inoculated with AMF was higher than inoculated plants (control). Under stress conditions, inoculating wheat plants with AMF activated the expression of genes encoding the synthesis of antioxidant enzymes such as SOD, POD, and CAT (Zou et al., 2021). In other studies, inoculation of wheat plants with PGPRs under drought stress conditions increased the activity of antioxidant enzymes SOD, POD, APX, CAT, and GR compared to the control (non-inoculation with PGPRs) (Kumar and Dubey, 2020; Zhang et al., 2020; Rashid et al., 2021). Also, Hussein et al. (2021), during a study on maize, stated that foliar application of algae extract on maize increased antioxidant enzymes such as SOD, POD, and CAT and non-enzymatic enzymes such as Phe. Latique et al. (2017) and Latique et al. (2021) stated in a study that foliar application of seaweed extract on the wheat plant under stress conditions improved SOD activity. They attributed the increase to the presence of some biochemical compounds such as polyphenols, sugars, and proteins soluble in algae extract. These compounds stimulated and increased the necessary antioxidant enzymes and reduced cell damage caused by ROS.
Flavonoids are antioxidants that, by reducing ROS, reduce the adverse effects on DNA, lipids, and proteins and increase plant tolerance to stress conditions (Baruah et al., 2021). The increase of secondary metabolites such as Fla in plants inoculated with arbuscular mycorrhiza is due to increased activity of phenylalanine ammonia-lyase (PAL) (Mirjani et al., 2019) and the involvement of gene expression in the synthesis of Fla (Aseel et al., 2019). Under stress, ROSs are destroyed by Fla, which are antioxidants. Fla stimulates mycorrhiza fungi activity; Fla causes spore germination, mycorrhiza hyphae growth, and arbuscular formation in the host plant's roots (Mirjani et al., 2019). In another study, the total Phe content of maize inoculated with MAF increased under stress, indicating that these fungi destroy ROS by producing compounds such as Phe and protecting the cellular structures and function of the host plant (Begum et al., 2019). Foliar application of maize with bio-fertilizer of seaweed extracts under stress conditions also increased soluble sugars, free amino acids, phenolic compounds, and Fla, which reduced electrolyte membrane leakage and oxidative damage by reducing oxygen free radicals (Baltazar et al., 2021; Shukla and Prithiviraj, 2021).
Studies have shown that the response of plants to drought stress in terms of the SPC of wheat leaves is variable and can be rising, decreasing, or unchanged. As in the study of Drooger et al. (2019) on different wheat cultivars, the amount of SPC with increasing drought stress in different wheat cultivars first went up to a 50% stress level and then showed a decreasing trend. In other words, it can be stated that increasing the activity of antioxidant enzymes under water stress may prevent the breakdown of plant proteins under drought stress (Drooger et al., 2019). Amjad et al. (2021) also reported that inoculation of wheat plants with AMF under drought stress enhanced the SPC of a wheat leaf. The study of different crops has well-proven the positive effects of seed inoculation with bio-fertilizers containing bacteria on the increase of the SPC (Pan et al., 2019), which is completely consistent with the results of the present experiment (Tables 7, 8).
Cell membrane integrity, the content of pigments and intracellular compounds, plant hydrogen bonds, osmotic regulation, and photosynthetic activity are significantly affected by dehydration stress, which ultimately reduces plant growth and yield (Anjum et al., 2017). In the present experiment, wheat grain yield decreased due to drought stress and irrigation and application of bio-fertilizers, especially the combination of bio-fertilizers used in the experiment, improved grain yield (Figures 6A,B,E,F). As a result of inoculating wheat seeds with EPS-producing rhizobacterial strains, the amounts and activities of non-enzymatic antioxidants such as Phe (Tables 8, 9) and Fla (Figures 4C,D), and enzymatic antioxidants such as SOD (Table 10), POD (Tables 6, 10) and DPPH (Figure 5D and Table 4) increased significantly. Also, inoculation significantly reduced leaf H2O2 and MDA contents compared with non-inoculated conditions (Figures 2E,F). This study showed that EPS-producing PGPR changed the defense reactions of plants, which could indicate its protective function. These physiological mechanisms are possibly controlled by signaling events that occur during the interactions between plants and EPS-generating rhizobacteria.
Drought stress, while impairing plant metabolic activity by reducing access to water, also reduces nutrient uptake, ultimately affecting crop growth and yield. The present study showed that drought stress in different growth stages significantly changed wheat growth, yield, and quality traits due to reduced water absorption, lower mobility of food reserves, and changes in enzyme activity, reduction and delay in germination. In addition, it reduced wheat plant growth by decreasing cell division and cell length, decreasing photosynthesis rate, and increasing ROS accumulation (Batool et al., 2022; Javed et al., 2022). Drought stress causes a reduction in stem growth, leaf area, stomatal closure, reduced photosynthesis, reduced growth material production, and disturbance in carbon metabolism and carbohydrate source, which leads to partial stomatal closure with less carbon dioxide availability. Furthermore, drought causes the production of ROS, which leads to membrane damage. As a result, it causes a decrease in the development of vegetative and reproductive organs, which can ultimately lead to a decrease in wheat plant performance in water-limited (dryland) conditions (Hussein et al., 2022). However, there is a great variation among genotypes in terms of their response to drought stress. Therefore, determining the effects of drought stress on yield and quality traits helps to select superior genotypes (Javed et al., 2022). Gui et al. (2021) showed that wheat's total biomass and grain yield decrease significantly with the occurrence and increase of stress. Other studies have shown that inoculation of wheat with AMF increased its growth and yield, which was associated with the expansion of the root system to access minerals and water, which increased plant resistance to drought stress (Ye et al., 2019; Tarnabi et al., 2020). Also, other research found that drought stress decreased wheat yield, but using PGPR bio-fertilizers improved wheat yield in drought-stress conditions (Akhtar et al., 2021). These researchers attributed the improvement in grain yield to increasing the amount and activity of antioxidants, establishing a more appropriate relationship between plant and soil for water and nutrient absorption, maintaining membrane stability (decreased electrolyte leakage), and increasing chlorophyll content (Akhtar et al., 2021). Inoculation of wheat seeds with bio-fertilizers containing PGPR caused tolerance to drought stress in wheat plants. It is possible to develop tolerance to drought stress in wheat plants inoculated with bio-fertilizers containing PGPR due to various direct or indirect mechanisms, including facilitation of water and nutrient absorption, induction of plant antioxidant systems, growth regulators, phytohormones such as IAA, osmolytes, the activity of different enzymes removing ROS and increasing soluble carbohydrates, increased the growth and photosynthetic efficiency of inoculated wheat plants. These changes can be a defense response and a reason for increasing drought stress tolerance in wheat plants inoculated with bio-fertilizers containing PGPR (Rashid et al., 2021; Latif et al., 2022). These bio-fertilizers contain bacterial strains commonly found in the wheat plant rhizosphere, which interact with the roots of the wheat plant and affect their growth and productivity. Bio-fertilizers containing PGPR had positive effects on various characteristics of wheat plants, such as biological nitrogen fixation, dissolution of inorganic phosphate compounds, and mineralization of organic phosphate compounds. The secretion of various plant hormones and vitamins, the limitation of ethylene synthesis, and the secretion of antibiotics increased the absorption of water and nutrients, tolerance to drought, resistance to disease, and the growth of wheat plants (Sedri et al., 2022). PGPR makes nutrients available to the rhizosphere by producing and releasing organic acids to dissolve phosphorus and potassium, improves nutrient absorption, and with oxidation reactions, converts nutrients from inaccessible shape to accessible form (Hungria et al., 2021). Ahmad et al. (2020) and Mousavi et al. (2020) reported that fertilizers containing PGPR increased corn, millet, and beans yield, which corresponds to our findings. Different plant species and varieties have different reactions to environmental conditions. In the present experiment, Sardari had a higher RWC than Sirvan in water limit conditions (Tables 3, 4). Higher RWC indicates existing water in the plant leaf and more resistance to stress conditions (Khan and Bano, 2019; Rashid et al., 2021). In a study on 14 wheat genotypes, the Galaxy-2013 genotype had the highest RWC and tangible superiority over other cultivars in severe drought stress conditions. In our research, Sedaris's cultivar due to a series of defensive mechanisms such as the further activity of antioxidant enzymes of SOD (Figures 3B,C) and POD (Tables 5, 10) as well as more non-enzymatic antioxidants including Phe (Tables 7, 9), Fla (Figure 4B) and Anth (Tables 5, 10) and higher osmotic adjustment (Figures 1A,B, 5A, Table 5) and better inhibit active oxygen radicals (Figure 5C, Tables 5, 6) had more resistance to dry stress. Drought-resistant species maintain more RWC in stress conditions, which can be a desirable trait in drought-resistant cultivars, which causes their openings to be tied in dry stress conditions and reduce transpiration, which affects the absorption and transfer of minerals (Wasaya et al., 2021). The higher RWC can be for two cases: these cultivars with their characteristics and by closing their stomata and less transpiration in dry stress conditions, keep their water content at high levels of water, and or these cultivars can absorb water from more deep of soil and transfer to air organs and maintain their RWC at high levels (Singh et al., 2021; El-Borhamy et al., 2022; Lotfi et al., 2022). Genotypes with higher RWC under stress conditions can have high stomata conductivity and photosynthesis and, as a result, have high drought resistance (Anwar et al., 2022). In our study, despite the highest capability of Sardari's cultivar than Sirvan's cultivar in drought resistance, Sirvan produced more grain yield. Najafi vafa et al. (2022) attributed this advantage to the higher content of photosynthetic pigments, higher photosynthetic power, and more yield potential for Sirvan compared with Sardari's cultivar.
According to the result of the present study, supplementary irrigation affected the traits of RWC, SPC, enzymatic and non-enzymatic antioxidants, caused reduced damage due to oxidative stresses, reduced the amounts of MDA, and increased plant growth and grain yield in both wheat cultivars. The consumption of biological fertilizers reduced the harmful effects of water scarcity stress and improved grain yield by increasing RWC, SPC, enzymatic antioxidants activity (SOD and POD) and content of non-enzymatic antioxidants (Phe, Fla, Anth) and more DPPH and decreased contents of MDA. Sardari's resistance to drought stress was higher than Sirvan's cultivar and responded better to the consumption of bio-fertilizers, although it had a lower yield than Sirvan. Therefore, it is reasonable to suggest applying a combination of supplemental irrigation and bio-fertilizers as an efficient technique to lessen the negative impacts of drought stress in arid and semi-arid environments.
The datasets used and/or analyzed during the current study are available from the corresponding author on reasonable request.
YS: supervisor of student in the Ph.D. thesis project, designing farm experiments, managing laboratory works, guiding and helping to statistical analysis of data, and writing the manuscript. ZNV: performing practical works in the farm and the laboratory, statistical analysis of data obtained from the experiment, writing the manuscript, and plotting the figures related to the manuscript. GM and GH: advisor of student in the Ph.D. thesis project, guiding and helping to statistical analysis of data, and writing the manuscript. All authors contributed to the article and approved the submitted version.
The authors declare that the research was conducted in the absence of any commercial or financial relationships that could be construed as a potential conflict of interest.
All claims expressed in this article are solely those of the authors and do not necessarily represent those of their affiliated organizations, or those of the publisher, the editors and the reviewers. Any product that may be evaluated in this article, or claim that may be made by its manufacturer, is not guaranteed or endorsed by the publisher.
The Supplementary Material for this article can be found online at: https://www.frontiersin.org/articles/10.3389/fsufs.2022.1073240/full#supplementary-material
Abd_Allah, E. F., Alqarawi, A. A., Hashem, A., Radhakrishnan, R., Al-Huqail, A. A., Al-Otibi, F. O. N., et al. (2018). Endophytic bacterium Bacillus subtilis (BERA 71) improves salt tolerance in chickpea plants by regulating the plant defense mechanisms. J. Plant Interact. 13, 37–44. doi: 10.1080/17429145.2017.1414321
Adeyemi, N. O., Atayese, M. O., Sakariyawo, O. S., Azeez, J. O., Olubode, A. A., Ridwan, M., et al. (2021). Influence of different arbuscular mycorrhiza fungi isolates in enhancing growth, phosphorus uptake and grain yield of soybean in a phosphorus deficient soil under field conditions. Commun. Soil Sci. Plant Anal. 52, 1171–1183. doi: 10.1080/00103624.2021.1879117
Ahmad, R., Hussain, S., Anjum, M. A., Khalid, M. F., Saqib, M., Zakir, I., et al. (2019). “Oxidative stress and antioxidant defense mechanisms in plants under salt stress,” in Plant Abiotic Stress Tolerance (Springer, Cham), 191–205. doi: 10.1007/978-3-030-06118-0_8
Ahmad, Z., Waraich, E. A., Barutçular, C., Hossain, A., Erman, M., ÇiG, F., et al. (2020). Enhancing drought tolerance in wheat through improving morpho-physiological and antioxidants activities of plants by the supplementation of foliar silicon. Phyton. Int. J. Exp. Bot. 89, 1–11. doi: 10.32604/phyton.2020.09143
Akhavan, K. (2015). Ministry of jihad agriculture, agricultural research, education and extension organization, agricultural research and training center and natural resources of Ardabil Province. Tech. J. Appl. Irrig. Sys. Strip. Diameters (Type) Wheat Agri. 83, 2–22.
Akhtar, N., Ilyas, N., Hayat, R., Yasmin, H., Noureldeen, A., and Ahmad, P. (2021). Synergistic effects of plant growth promoting rhizobacteria and silicon dioxide nano-particles for amelioration of drought stress in wheat. Plant Physiol. Biochem. 166, 160–176. doi: 10.1016/j.plaphy.2021.05.039
Alizadeh, A., and Kamali, G. H. (2008). Plant Water Requirement in Iran. Astan Ghods Razavi Publ. 2, 227.
Altieri, M. A. (2019). Agro-ecology: The Science of Sustainable Agriculture. Boca Raton, FL: CRC Press. doi: 10.1201/9780429495465
Ambreetha, S., Chinnadurai, C., Marimuthu, P., and Balachandar, D. (2018). Plant-associated Bacillus modulates the expression of auxin-responsive genes of rice and modifies the root architecture. Rhizosphere. 5, 57–66. doi: 10.1016/j.rhisph.2017.12.001
Amjad, S. F., Mansoora, N., Din, I. U., Khalid Iqbal, R., Jatoi, G. H., Murtaza, G., et al. (2021). Application of zinc fertilizer and mycorrhiza inoculation on physio-biochemical parameters of wheat grown under water-stressed environment. Sustainability. 13, 1–14. doi: 10.3390/su131911007
Anjum, S. A., Ashraf, U., Tanveer, M., Khan, I., Hussain, S., Shahzad, B., et al. (2017). Drought induced changes in growth, osmolyte accumulation and antioxidant metabolism of three maize hybrids. Front. Plant Sci. 8, 1–12. doi: 10.3389/fpls.2017.00069
Ansari, F. A., Jabeen, M., and Ahmad, I. (2021). Pseudomonas azotoformans FAP5, a novel biofilm-forming PGPR strain, alleviates drought stress in wheat plant. Int. J. Environ. Sci. Technol. 18, 3855–3870. doi: 10.1007/s13762-020-03045-9
Anwar, M., Saleem, M. A., Dan, M., Malik, W., Ul-Allah, S., Ahmad, M. Q., et al. (2022). Morphological, physiological and molecular assessment of cotton for drought tolerance under field conditions. Saudi J. Biol. Sci. 29, 444–452. doi: 10.1016/j.sjbs.2021.09.009
Aseel, D. G., Rashad, Y. M., and Hammad, S. M. (2019). Arbuscular mycorrhiza fungi trigger transcriptional expression of flavonoid and chromogenic acid biosynthetic pathways genes in tomato against Tomato Mosaic Virus. Sci. Rep. 9, 1–10. doi: 10.1038/s41598-019-46281-x
Asghari, B., Khademian, R., and Sedaghati, B. (2020). Plant growth promoting rhizobacteria (PGPR) confer drought resistance and stimulate biosynthesis of secondary metabolites in pennyroyal (Mentha pulegium L.) under water shortage condition. Sci Hortic. 263, 1–10. doi: 10.1016/j.scienta.2019.109132
Ashour, M., El-Shafei, A. A., Khairy, H. M., Abd-Elkader, D. Y., Mattar, M. A., Alataway, A., et al. (2020). Effect of Pterocladia capillacea seaweed extracts on growth parameters and biochemical constituents of Jew's Mallow. Agron. 10, 1–18. doi: 10.3390/agronomy10030420
Baltazar, M., Correia, S., Guinan, K. J., Sujeeth, N., Bragança, R., and Gonçalves, B. (2021). Recent advances in the molecular effects of bio-stimulants in plants: An overview. Biomolog 11, 1–27. doi: 10.3390/biom11081096
Barrs, H. D., and Weatherley, P. E. (1962). A re-examination of the relative turgidity technique for estimating water deficits in leaves. Aust. J. Biol. Sci. 15, 413–428. doi: 10.1071/BI9620413
Baruah, I., Sarki, Y. N., Chikkaputtaiah, C., and Baruah, G. (2021). “Role of PGPR in conferring drought stress tolerance in rice,” in Antioxidants in Plant-Microbe Interaction, eds. A. K. Singh, S. Kumar, T. Sinha (Singapore: Springer), 425–448. doi: 10.1007/978-981-16-1350-0_20
Batool, M., El-Badri, A. M., Hassan, M. U., Haiyun, Y., Chunyun, W., Zhenkun, Y., et al. (2022). Drought stress in Brassica napus: Effects, tolerance mechanisms, and management strategies. J. Plant Growth Regul. 9, 1–25. doi: 10.1007/s00344-021-10542-9
Beauchamp, C., and Fridovich, I. (1971). Superoxide dismutase: improved assays and an assay applicable to acrylamide gels. Anal. Biochem. 44, 276–287. doi: 10.1016/0003-2697(71)90370-8
Begum, N., Ahanger, M. A., Su, Y., Lei, Y., Mustafa, N. S. A., Ahmad, P., et al. (2019). Improved drought tolerance by AMF inoculation in maize (Zea mays) involves physiological and biochemical implications. Plants. 8, 1–20. doi: 10.3390/plants8120579
Benaffari, W., Boutasknit, A., Anli, M., Ait-El-Mokhtar, M., Ait-Rahou, Y., Ben-Laouane, R., et al. (2022). The native arbuscular mycorrhiza fungi and vermicomposting-based organic amendments enhance soil fertility, growth performance, and the drought stress tolerance of quinoa. Plants. 11, 1–27. doi: 10.3390/plants11030393
Benidire, L., Madline, A., Pereira, S. I. A., Castro, P. M. L., and Boularbah, A. (2021). Synergistic effect of organ mineral amendments and plant growth-promoting rhizobacteria (PGPR) on the establishment of vegetation cover and amelioration of mine tailings. Chemosphere. 262, 1–50. doi: 10.1016/j.chemosphere.2020.127803
Bitaraf, N., Saadatmand, S., Mehregan, I., Ahmadvand, R., and Ebadi, M. (2020). Evaluation of mitigation effects of Glomus mosseae on Triticum aestivum L., cv. Chamran under drought stress. Period. Tche Quim. 17, 1033–1045. doi: 10.52571/PTQ.v17.n34.2020.1065_P34_pgs_1033_1045.pdf
Bradford, M. M. (1976). A rapid and sensitive method for the quantitation of microgram quantities of protein utilizing the principle of protein-dye binding. Ann. Clin. Biochem. 72, 248–254. doi: 10.1016/0003-2697(76)90527-3
Bremner, J. M. (1965). Total nitrogen. Methods Soil Anal. 9, 1149–1178. doi: 10.2134/agronmonogr9.2.c32
Bukhari, M. A., Ahmad, Z., Ashraf, M. Y., Afzal, M., Nawaz, F., Nafees, M., et al. (2021). Silicon mitigates drought stress in wheat (Triticum aestivum L.) through improving photosynthetic pigments, biochemical and yield characters. Silicon. 13, 4757–4772. doi: 10.1007/s12633-020-00797-4
Cakmak, I., and Horst, W. J. (1991). Effect of aluminum on lipid peroxidation, superoxide dismutase, catalase and peroxidase activities in root tips of soybean (Glycine max). Physiol. Plant. 83, 463–468. doi: 10.1111/j.1399-3054.1991.tb00121.x
Carter, M. R., and Gregorich, E. G. (2006). Soil Sampling and Methods of Analysis. Boca Raton, FL: Canadian Society of Science; CRC Press. doi: 10.1201/9781420005271
Chaganti, V. N., Ganjegunte, G., Niu, G., Ulery, A., Flynn, R., Enciso, J. M., et al. (2020). Effects of treated urban wastewater irrigation on bioenergy sorghum and soil quality. Agric. Manage. Water. 228, 1–12. doi: 10.1016/j.agwat.2019.105894
Chapman, H. D., and Pratt, P. F. (1961). Methods of analysis for soils, plants, and waters. Soil Analy. 93, 68.
Chiappero, J., del Rosario Cappellari, L., Palermo, T. B., Giordano, W., Khan, N., and Banchio, E. (2021). Antioxidant status of medicinal and aromatic plants under the influence of growth-promoting rhizobacteria and osmotic stress. Ind. Crops Prod. 167, 1–14. doi: 10.1016/j.indcrop.2021.113541
Dhindsa, R. S., and Matowe, W. (1981). Drought tolerance in two mosses: correlated with enzymatic defense against lipid peroxidation. J. Exp. Bot. 32, 79–91. doi: 10.1093/jxb/32.1.79
Drooger, H., Fakheri, B., Mehdi Nejad, N., and Mohammadi, R. (2019). Evaluation of some biochemical traits in several crops and wild wheat under drought stress. Environ. Stresses. Agric. Sci. 12, 696–685.
El-Borhamy, A., Khedr, R. A., and El-Mansoury, M. (2022). Physiological, Biochemical and Agronomic Response of Some Flax Cultivars to Water Deficit under Clay Soil Conditions in North Delta. J. Adv. Agric. Res. 27, 351–365. doi: 10.21608/jalexu.2022.139346.1065
El-Esawi, M. A., Al-Ghamdi, A. A., Ali, H. M., and Alayafi, A. A. (2019). Azospirillum lipoferum FK1 confers improved salt tolerance in chickpea (Cicer arietinum L.) by modulating osmolytes, antioxidant machinery and stress-related genes expression. Environ. Exp. Bot. 159, 55–65. doi: 10.1016/j.envexpbot.2018.12.001
El-Esawi, M. A., Elansary, H. O., El-Shanhorey, N. A., Abdel-Hamid, A. M., Ali, H. M., and Elshikh, M. S. (2017). Salicylic acid-regulated antioxidant mechanisms and gene expression enhance rosemary performance under saline conditions. Front. Physiol. 8, 1–14. doi: 10.3389/fphys.2017.00716
Elkelish, A., El-Mogy, M. M., Niedbała, G., Piekutowska, M., Atia, M. A., Hamada, M., et al. (2021). Roles of Exogenous α-lipoic acid and cysteine in mitigation of drought stress and restoration of grain quality in wheat. Plants. 10, 1–25. doi: 10.3390/plants10112318
Fiorilli, V., Maghrebi, M., Novero, M., Votta, C., Mazzarella, T., Buffoni, B., et al. (2022). Arbuscular mycorrhiza symbiosis differentially affects the nutritional status of two durum wheat genotypes under drought conditions. Plants. 11, 1–21. doi: 10.3390/plants11060804
García, G., Clemente-Moreno, M. J., Díaz-Vivancos, P., García, M., and Hernández, J. A. (2020). The apoplastic and symplastic antioxidant system in onion: Response to long-term salt stress. Antioxid. 9, 1–17. doi: 10.3390/antiox9010067
Gee, G. W., and Bauder, J. W. (1986). “Particle size analysis,” in Methods of Soil Analysis, Agronomy Monograph, 2nd Edn. eds. A. Klute (Madison, WI: ASA and SSSA), 9, 383–411. doi: 10.2136/sssabookser5.1.2ed.c15
Giusti, M. M., and Wrolstad, R. E. (2000). “Characterization and measurement with UV-visible spectroscopy. Unit F2. 2, Ch. 2,” in Current Protocols in Food Analytical Chemistry, eds. S. King, M. Gates and L. Scalettar (New York: Wiley), 1–13.
Goswani, M., and Deka, S. (2020). Plant growth-promoting rhizobacteria alleviators of abiotic stresses in soil: a review. Pedosphere. 30, 40–61. doi: 10.1016/S1002-0160(19)60839-8
Gui, Y. W., Sheteiwy, M. S., Zhu, S. G., Batool, A., and Xiong, Y. C. (2021). Differentiate effects of non-hydraulic and hydraulic root signaling on yield and water use efficiency in diploid and tetraploid wheat under drought stress. Environ. Exp. Bot. 181, 1–10. doi: 10.1016/j.envexpbot.2020.104287
Harding, V. J., and MacLean, R. M. (1916). The ninhydrin reaction with amines and amides. J. Biol. Chem. 25, 337–350. doi: 10.1016/S0021-9258(18)87507-X
Hasanuzzaman, M., Bhuyan, M. H. M. B., Zulfiqar, F., Raza, A., Mohsin, S. M., Mahmud, J. A., et al. (2020). Reactive oxygen species and antioxidant defense in plants under abiotic stress: revisiting the crucial role of a universal defense regulator. Antioxid. 9, 1–52. doi: 10.3390/antiox9080681
He, A., Niu, S., Yang, D., Ren, W., Zhao, L., Sun, Y., et al. (2021). Two PGPR strains from the rhizosphere of Haloxylon ammo dendron promoted growth and enhanced drought tolerance of ryegrass. Plant Physiol. Biochem. 161, 74–85. doi: 10.1016/j.plaphy.2021.02.003
Hemeda, H. M., and Klein, B. P. (1990). Effects of naturally occurring antioxidants on peroxidase activity of vegetable extracts. J. Food Sci. 55, 184–185. doi: 10.1111/j.1365-2621.1990.tb06048.x
Huang, D., Ou, B., and Prior, R. L. (2005). The chemistry behind antioxidant capacity assays. J. Agric. Food Chem. 53, 1841–1856. doi: 10.1021/jf030723c
Hungria, M., Rondina, A. B. L., Nunes, A. L. P., Araujo, R. S., and Nogueira, M. A. (2021). Seed leaf-spray inoculation of PGPR in brachiarias (Urochloa spp.) as an economic and environmental opportunity to improve plant growth, forage yield and nutrient status. Plant Soil. 463, 171–186. doi: 10.1007/s11104-021-04908-x
Hussein, H. A. A., Alshammari, S. O., Kenawy, S. K., Elkady, F. M., and Badawy, A. A. (2022). Grain-priming with L-arginine improves the growth performance of wheat (Triticum aestivum L.) plants under drought stress. Plants 11, 1–15. doi: 10.3390/plants11091219
Hussein, M. H., Eltanahy, E., Al Bakry, A. F., Elsafty, N., and Elshamy, M. M. (2021). Seaweed extracts as prospective plant growth bio-stimulant and salinity stress alleviator for Vigna sinensis and Zea mays. J. Appl. Phycol. 33, 1273–1291. doi: 10.1007/s10811-020-02330-x
Igiehon, N. O., Babalola, O. O., Cheseto, X., and Torto, B. (2021). Effects of rhizobia and arbuscular mycorrhiza fungi on yield, size distribution and fatty acid of soybean seeds grown under drought stress. Microbiol. Res. 242, 99–113. doi: 10.1016/j.micres.2020.126640
Imade, E. E., and Babalola, O. O. (2021). Biotechnological utilization: the role of Zea mays rhizospheric bacteria in ecosystem sustainability. Appl. Microbiol. Biotechnol. 105, 4487–4500. doi: 10.1007/s00253-021-11351-6
Javed, A., Ahmad, N., Ahmed, J., Hameed, A., Ashraf, M. A., Zafar, S., et al. (2022). Grain yield, chlorophyll and protein contents of elite wheat genotypes under drought stress. J. King Saud Univ. Sci. 34, 1–8. doi: 10.1016/j.jksus.2022.102279
Khan, N., and Bano, A. (2019). Exo-polysaccharide producing rhizobacteria and their impact on growth and drought tolerance of wheat grown under rain-fed conditions. PLoS ONE. 14, 1–19. doi: 10.1371/journal.pone.0222302
Kumar, A., and Dubey, A. (2020). Rhizosphere micro-biome: Engineering bacterial competitiveness for enhancing crop production. J. Adv. Res. 24, 337–352. doi: 10.1016/j.jare.2020.04.014
Latif, M., Bukhari, S. A. H., Alrajhi, A. A., Alotaibi, F. S., Ahmad, M., Shahzad, A. N., et al. (2022). Inducing drought tolerance in wheat through exopolysaccharide-producing rhizobacteria. Agronomy 12, 1–18. doi: 10.3390/agronomy12051140
Latique, S., Mohamed Aymen, E., Halima, C., Chérif, H., and Mimoun, E. K. (2017). Alleviation of salt stress in durum wheat (Triticum durum L.) seedlings through the application of liquid seaweed extracts of Fucus spiralis. Commun. Soil Sci. Plant Anal. 48, 2582–2593. doi: 10.1080/00103624.2017.1416136
Latique, S., Mrid, R. B., Kabach, I., Kchikich, A., Sammama, H., and Yasri, A. (2021). Foliar Application of Ulva rigida Water Extracts Improves Salinity Tolerance in Wheat (Triticum durum L.). Agronomy. 11, 1–17. doi: 10.3390/agronomy11020265
Li, F., Zhao, A., Cui, C., Dong, J., Gao, X., Rustgi, S., et al. (2022). Progress in genetic studies of traits related to the nutritional value of wheat. Adv. Agron. 35, 1–79. doi: 10.1016/bs.agron.2022.07.002
Lindsay, W. L., and Norvell, W. (1978). Development of a DTPA soil test for zinc, iron, manganese, and copper. Soil Sci. Soc. Am. J. 42, 421–428. doi: 10.2136/sssaj1978.03615995004200030009x
Liu, J., Hasanuzzaman, M., Wen, H., Zhang, J., Peng, T., Sun, H., et al. (2019). High temperature and drought stress cause abscisic acid and reactive oxygen species accumulation and suppress seed germination growth in rice. Protoplasma. 256, 1217–1227. doi: 10.1007/s00709-019-01354-6
Lotfi, R., Abbasi, A., Kalaji, H. M., Eskandari, I., Sedghieh, V., Khorsandi, H., et al. (2022). The role of potassium on drought resistance of winter wheat cultivars under cold dry land conditions: Probed by chlorophyll a fluorescence. Plant Physiol. Biochem. 182, 45–54. doi: 10.1016/j.plaphy.2022.04.010
McLean, E. O. (1983). Soil pH and lime requirement. Methods Soil Anal. 9, 199–224. doi: 10.2134/agronmonogr9.2.2ed.c12
Mirjani, L., Salimi, A., Matinizadeh, M., Razavi, K., and Shahbazi, M. (2019). The role of arbuscular mycorrhiza fungi on acclimatization of micro propagated plantlet Satureja khuzistanica Jam. by ameliorating of antioxidant activity and expression of PAL gene. Sci. Hortic. 253, 364–370. doi: 10.1016/j.scienta.2019.04.060
Morais, T., Inácio, A., Coutinho, T., Ministro, M., Cotas, J., and Pereira, L. (2020). Seaweed potential in the animal feed: A review. J. Mar. Sci. Eng. 8, 1–24. doi: 10.3390/jmse8080559
Mousavi, S. A., Shokuhfar, A., Lak, S., Mojaddam, M., and Alavifazel, M. (2020). Integrated application of bio-char and bio-fertilizer improves yield and yield components of cowpea under water-deficient stress. Ital. J. Agron. 15, 94–101. doi: 10.4081/ija.2020.1581
Naderi, S., Fakheri, B. A., Maali-Amiri, R., and Mahdinezhad, N. (2020). Tolerance responses in wheat landrace Bolani are related to enhance metabolic adjustments under drought stress. Plant Physiol. Biochem. 150, 244–253. doi: 10.1016/j.plaphy.2020.03.002
Najafi vafa, Z., Sohrabi, Y., Mirzaghaderi, G. H., and Heidari, G. H. (2022). Soil microorganisms and seaweed application with supplementary irrigation improved physiological traits and yield of two dry land wheat cultivars. Front. Plant Sci. 13, 1–23. doi: 10.3389/fpls.2022.855090
Naliwajski, M., and Skłodowska, M. (2021). The relationship between the antioxidant system and proline metabolism in the leaves of cucumber plants acclimated to salt stress. Cells. 10, 1–15. doi: 10.3390/cells10030609
Noreen, S., Sultan, M., Akhter, M. S., Shah, K. H., Ummara, U., Manzoor, H., et al. (2021). Foliar fertigation of ascorbic acid and zinc improves growth, antioxidant enzyme activity and harvest index in barley (Hordeum vulgare L.) grown under salt stress. Plant Physiol. Biochem. 158, 244–254. doi: 10.1016/j.plaphy.2020.11.007
Oleńska, E., Małek, W., Wójcik, M., Swiecicka, I., Thijs, S., and Vangronsveld, J. (2020). Beneficial features of plant growth-promoting rhizobacteria for improving plant growth and health in challenging conditions: A methodical review. Sci. Total Environ. 743, 1–54. doi: 10.1016/j.scitotenv.2020.140682
Olsen, S. R., and Sommers, L. E. (1982). “Phosphorus,” in Methods of soil Analysis: Chemical and microbiological Properties, part 2, eds. A. Klute (Madison WI, USA: American Society of Agronomy and Soils Science Society of America), 403–430. doi: 10.2134/agronmonogr9.2.2ed.c24
Omara, A. E. D., Hafez, E. M., Osman, H. S., Rashwan, E., El-Said, M. A., Alharbi, K., et al. (2022). Collaborative impact of compost and beneficial rhizobacteria on soil properties, physiological attributes, and productivity of wheat subjected to deficit irrigation in salt affected soil. Plants. 11, 1–19. doi: 10.3390/plants11070877
Pan, J., Peng, F., Xue, X., You, Q., Zhang, W., Wang, T., et al. (2019). The growth promotion of two salt-tolerant plant groups with PGPR inoculation: a meta-analysis. Sustainability. 11, 1–14. doi: 10.3390/su11020378
Patil, P., Gupta, A. K., Bains, N. S., and Kaur, K. (2021). Variability in enzymatic and non-enzymatic antioxidants in wheat (Triticum aestivum L.) genotypes. Plant Physiol. Rep. 26, 428–442. doi: 10.1007/s40502-021-00584-2
Rady, M. O., Semida, W. M., Abd El-Mageed, T. A., Howladar, S. M., and Shaaban, A. (2020). Foliage applied selenium improves photosynthetic efficiency, antioxidant potential and wheat productivity under drought stress. Int. J. Agric. Biol. 24, 1293–1300. doi: 10.17957/IJAB/15.1562
Raffi, M. M., and Charyulu, P. B. B. N. (2021). “Azospirillum bio-fertilizer for sustainable cereal crop production: Current status,” in Recent Developments in Applied Microbiology and Biochemistry, ed B. Viswanath (Massachusetts: Academic Press; University of Cambridge). doi: 10.1016/B978-0-12-821406-0.00018-7
Rashid, U., Yasmin, H., Hassan, M. N., Naz, R., Nosheen, A., Sajjad, M., et al. (2021). Drought-tolerant Bacillus megaterium isolated from semi-arid conditions induces systemic tolerance of wheat under drought conditions. Plant Cell Rep. 41, 549–569. doi: 10.1007/s00299-020-02640-x
Safirzadeh, S., Chorom, M., and Enayatizamir, N. (2021). Speciation and fractionation of phosphorus affected by Enterobacter cloacae in the rhizosphere of sugarcane (Saccharum officinarum L.). J. Soil Sci. Plant Nutr. 21, 187–199. doi: 10.1007/s42729-020-00352-5
Sakara, H. M. (2020). Bio-fortification of Potato Plants with different Nitrogen Rates and Applied Iron Forms in Presence and Absent of Azotobacter sp. Egypt. J. Soil Sci. 60, 449–467. doi: 10.21608/ejss.2020.43816.1386
Sanjari, S., Shobbar, Z. S., Ghanati, F., Afshari-Behbahanizadeh, S., Farajpour, M., Jokar, M., et al. (2021). Molecular, chemical, and physiological analyses of sorghum leaf wax under post-flowering drought stress. Plant Physiol. Biochem. 159, 383–391. doi: 10.1016/j.plaphy.2021.01.001
Sedri, M. H., Niedbała, G., Roohi, E., Niazian, M., Szulc, P., Rahmani, H. A., et al. (2022). Comparative analysis of plant growth-promoting rhizobacteria (PGPR) and chemical fertilizers on quantitative and qualitative characteristics of rainfed wheat. Agronomy. 12, 1–14. doi: 10.3390/agronomy12071524
Shukla, P. S., and Prithiviraj, B. (2021). Ascophyllum nodosum bio-stimulant improves the growth of Zea mays grown under phosphorus impoverished conditions. Front. Plant Sci. 11, 1–17. doi: 10.3389/fpls.2020.601843
Singh, C. K., Rajkumar, B. K., and Kumar, V. (2021). Differential responses of antioxidants and osmolytes in upland cotton (Gossypium hirsutum) cultivars contrasting in drought tolerance. Plant Stress. 2, 1–9. doi: 10.1016/j.stress.2021.100031
Singleton, G. R., Leirs, H., Hinds, L. A., and Zhang, Z. (1999). “Ecologically-based management of rodent pests–re-evaluating our approach to an old problem,” in Ecologically-based management of rodent pests. Australian Centre for International Agricultural Research (ACIAR) (Canberra) 17–29.
Spagnoletti, F. N., Carmona, M., Balestrasse, K., Chiocchio, V., Giacometti, R., and Lavado, R. S. (2021). The arbuscular mycorrhiza fungus Rhizophagus intra-radices reduces the root rot caused by Fusarium pseudograminearum in wheat. Rhizosphere. 19, 1–18. doi: 10.1016/j.rhisph.2021.100369
Sumardianto, R., PH, A., AD, R., and Rianingsih, L. (2021). Phenol content and antioxidant activity in seaweed fermented with lactic acid bacteria. Food Res. 5, 7–13. doi: 10.26656/fr.2017.5(S3).006
Tandon, H. L. S. (1998). Method of Analysis of Soil, Plant, Waters and Fertilizer. New Delhi, India: Development and Consultation Organization 144.
Tarnabi, Z., Iranbakhsh, A., Mehregan, I., and Ahmadvand, R. (2020). Impact of arbuscular mycorrhiza fungi (AMF) on gene expression of some cell wall and membrane elements of wheat (Triticum aestivum L.) under water deficit using transcript-me analysis. Physiol. Mol. Biol. Plants. 26, 143–162. doi: 10.1007/s12298-019-00727-8
Trivedi, K., Vijay Anand, K. G., Kubavat, D., Patidar, R., and Ghosh, A. (2018). Drought alleviatory potential of Kappaphycus seaweed extract and the role of the quaternary ammonium compounds as its constituents towards imparting drought tolerance in Zea mays L. J. Appl. Phycol. 30, 2001–2015. doi: 10.1007/s10811-017-1375-0
Wang, J., Barański, M., Hasanaliyeva, G., Korkut, R., Kalee, H. A., Leifert, A., et al. (2021). Effect of irrigation, fertilizer type and variety on grain yield and nutritional quality of spelt wheat (Triticum spelta) grown under semi-arid conditions. Food Chem. 358, 1–11. doi: 10.1016/j.foodchem.2021.129826
Wasaya, A., Manzoor, S., Yasir, T. A., Sarwar, N., Mubeen, K., Ismail, I. A., et al. (2021). Evaluation of fourteen bread wheat (Triticum aestivum L.) genotypes by observing gas exchange parameters, relative water and chlorophyll content, and yield attributes under drought stress. Sustainability. 13, 1–25. doi: 10.3390/su13094799
Xie, Z., Chu, Y., Zhang, W., Lang, D., and Zhang, X. (2019). Bacillus pumilus alleviates drought stress and increases metabolite accumulation in Glycyrrhizin uralensis Fisch. Environ. Exp. Bot. 158, 99–106. doi: 10.1016/j.envexpbot.2018.11.021
Xu, Q., Ling, N., Chen, H., Duan, Y., Wang, S., Shen, Q., et al. (2020). Long-term chemical-only fertilization induces a diversity decline and deep selection on the soil bacteria. Msystems. 5, 1–10. doi: 10.1128/mSystems.00337-20
Yan, S., Wu, Y., Fan, J., Zhang, F., Guo, J., Zheng, J., et al. (2022). Optimization of drip irrigation and fertilization regimes to enhance winter wheat grain yield by improving post-anthesis dry matter accumulation and translocation in northwest China. Agric. Water. Manage. 271, 1–11. doi: 10.1016/j.agwat.2022.107782
Yang, H., Hu, W., Zhao, J., Huang, X., Zheng, T., and Fan, G. (2021). Genetic improvement combined with seed ethephon priming improved grain yield and drought resistance of wheat exposed to soil water deficit at tillering stage. J. Plant Growth Regul. 95, 399–419. doi: 10.1007/s10725-021-00749-x
Yasmin, H., Nosheen, A., Naz, R., Bano, A., and Keyani, R. (2017). L-tryptophan-assisted PGPR-mediated induction of drought tolerance in maize (Zea mays L.). J. Plant Interact. 12, 567–578. doi: 10.1080/17429145.2017.1402212
Ye, L., Zhao, X., Bao, E., Cao, K., and Zou, Z. (2019). Effects of arbuscular mycorrhiza fungi on watermelon growth, elemental uptake, antioxidant, and photosystem II activities and stress-response gene expressions under salinity-alkalinity stresses. Front. Plant Sci. 10, 1–12. doi: 10.3389/fpls.2019.00863
Zaki, M. A., Ashour, M., Heneash, A. M. M., Mabrouk, M. M., Alprol, A. E., Khairy, H. M., et al. (2021). Potential applications of native cyan bacterium isolate (Arthrospira platensis NIOF17/003) for biodiesel production and utilization of its byproduct in marine rotifer (Brachionus plicatilis) production. Sustainability. 13, 1–16. doi: 10.3390/su13041769
Zhang, M., Yang, L., Hao, R., Bai, X., Wang, Y., and Yu, X. (2020). Drought-tolerant plant growth-promoting rhizobacteria isolated from jujube (Ziziphus jujuba) and their potential to enhance drought tolerance. J. Plant Soil. 452, 423–440. doi: 10.1007/s11104-020-04582-5
Zhishen, J., Mengcheng, T., and Jianming, W. (1999). The determination of flavonoid contents in mulberry and their scavenging effects on superoxide radicals. Food Chem. 64, 555–559. doi: 10.1016/S0308-8146(98)00102-2
Zhou, L., Li, C., White, J. F., and Johnson, R. D. (2021). Synergism between calcium nitrate applications and fungal endophytes to increase sugar concentration in Festuca sinensis under cold stress. PeerJ. 9, 1–25. doi: 10.7717/peerj.10568
Keywords: antioxidant, drought stress, malondialdehyde, osmolytes, seaweed extract
Citation: Najafi Vafa Z, Sohrabi Y, Mirzaghaderi G and Heidari G (2022) The effect of rhizobia in improving the protective mechanisms of wheat under drought and supplementary irrigation conditions. Front. Sustain. Food Syst. 6:1073240. doi: 10.3389/fsufs.2022.1073240
Received: 18 October 2022; Accepted: 18 November 2022;
Published: 08 December 2022.
Edited by:
Javid A. Parray, GDC Eidgah Srinagar, IndiaReviewed by:
Solmaz Najafi, Yüzüncü Yil University, TurkeyCopyright © 2022 Najafi Vafa, Sohrabi, Mirzaghaderi and Heidari. This is an open-access article distributed under the terms of the Creative Commons Attribution License (CC BY). The use, distribution or reproduction in other forums is permitted, provided the original author(s) and the copyright owner(s) are credited and that the original publication in this journal is cited, in accordance with accepted academic practice. No use, distribution or reproduction is permitted which does not comply with these terms.
*Correspondence: Yousef Sohrabi, eS5zb2hyYWJpQHVvay5hYy5pcg==
Disclaimer: All claims expressed in this article are solely those of the authors and do not necessarily represent those of their affiliated organizations, or those of the publisher, the editors and the reviewers. Any product that may be evaluated in this article or claim that may be made by its manufacturer is not guaranteed or endorsed by the publisher.
Research integrity at Frontiers
Learn more about the work of our research integrity team to safeguard the quality of each article we publish.