- 1LongPing Branch, College of Biology, Hunan University, Changsha, China
- 2Institute of Plant Protection, Hunan Academy of Agricultural Sciences, Changsha, China
The rice blast disease caused by Magnaporthe oryzae threatens global rice production yields. Tricyclazole and isoprothiolane are widely used fungicides with high activity against rice blast, and our previous study indicated the photosynthetic bacterium Rhodopseudomonas palustris PSB-06 significantly antagonizes rice blast. However the effect of combining these two chemical fungicides with PSB-06 on rice blast control is unclear. Here we test the control effect of photosynthetic bacteria PSB-06 combined with isoprothiolane and tricyclazole on rice blast. The growth of PSB-06 was unaffected by up to 1.25 mg/L of tricyclazole and 0.3 mg/L of isoprothiolane in the photosynthetic medium, indicated the two fungicides have no inhibition on PSB-06. The control efficiency in the field test reached 76.06% when PSB-06 was combined with isoprothiolane. This value was significantly higher than the individual efficiency of PSB-06 (67.99%) and tricyclazole (65.46%) and the combined control efficiency (72.20%) of those two antifungal agents. Our current findings highlighted the potential of combining R. palustris strain PSB-06 with isoprothiolane to control rice blast, providing environmental protection and reducing the use of fungicides.
Introduction
Rice blast is an important fungal disease causing significant annual losses to global rice production. It is caused by the filamentous fungus Magnaporthe oryzae (Talbot, 2003). In disease-endemic areas, the yield is generally reduced by 10–20%. Yield reduction reaches 40–50% in severe cases, and grains are not harvested in particularly heavy fields (Dean et al., 2012). Currently, the control of rice blast is mainly based on breeding and utilizing disease-resistant varieties along with supplementation by chemical fungicides. This results in the selection of disease-resistant types and drugs losing their effectiveness. Therefore, developing a new safe, efficient, economical, and convenient way of rice blast prevention and control is of great significance for ensuring food security.
A biological control refers to using living organisms or some metabolically active substances to prevent and control plant diseases, pests, and weeds (Kenneth, 1987). The current biological fungicides are primarily based on microbial fungicides (Fravel, 2005). A microbial fungicide is artificially modified or synthesized using microorganisms or their metabolites or their metabolites as precursors. These biocontrol factors include antagonistic microorganisms, antibiotics, and plant immunity inducers (Qiu et al., 2017). In recent years, the use of microbial fungicides to control rice blast has received increasing attention and has become a research hotspot in rice blast control. Trichoderma is a biocontrol fungus with abundant soil resources. It antagonizes various plant pathogens (Woo and Lorito, 2006). Trichoderma secretes extracellular enzymes, such as chitinase, cellulase, glucanase, protease, etc., while competing with pathogenic bacteria. These enzymes help dissolve the cell wall of pathogenic fungi, degrade cellulose and glucan in the environment, and acquire more nutrients while inhibiting the growth of pathogenic fungi (Vinalea et al., 2008). A series of studies have shown that Trichoderma is very good at inhibiting rice blast with tremendous control (Prabhakaran et al., 2015; Aravindan et al., 2016). In addition, biocontrol proteins like antimicrobial proteins, disease course-related proteins, and trigger proteinswere identified, produced, and applied (Chen M. J. et al., 2014) For example, the distinct antifungal proteins (AFPs) encoded by filamentous fungi exhibited antifungal activity against most fungi tested.
Photosynthetic bacteria are prokaryotic organisms that utilize light as their energy source. They use organic matter, sulfide, ammonia, etc., as hydrogen and carbon sources to perform photosynthesis under anaerobic conditions (Pirson, 1960; Olson, 2010). Photosynthetic bacteria are Gram-negative and belong to the bacterial phylum Eubacteria, order Rhodospirillales. It includes four families, 19 genera, and about 50 species (Olson, 2010). Photosynthetic bacteria are used in many fields due to their wide distribution, green environmental protection, simple production conditions, low cost, significant economic benefits, and convenient use (Idi, 2015). Our previous research identified the controlling effect exerted by the photosynthetic bacterium Rhodopseudomonas palustris strain PSB-06 on M. oryzae. Moreover, the secreted protein GroEL was significantly antagonistic and pathogenic to M. oryzae (Wu et al., 2021).
Currently, chemical control, biological control, and breeding of disease-resistant varieties are the main methods for controlling rice blast, along with cultivation management and other technical means (Jia, 2013). Chemical fungicides are the mainstream choice for rice blast control. Tricyclazole and Isoprothiolane exhibit excellent management of rice blast (Uchida and Fukada, 1983; Shiba and Nagata, 2009). Tricyclazole inhibits the formation of melanin in the rice blast pathogen, thereby inhibiting the germination of conidia and the formation of appressorium of the rice blast pathogen. Subsequently, the invasion of the pathogen is prevented, and the plant is protected. Isoprothiolane acts similarly to organophosphorus fungicides to a certain extent. Its primary mechanism of action is to inhibit the transmethylation of phospholipid choline before synthesis.
Here, the controlling potential of strain PSB-06 was evaluated against M. oryzae infection. Further, the combined use of PSB-06 and isopropanethiole or tricyclazole was tested against M. oryzae in greenhouse and field trials. The biological agents exerted their antibacterial activity to a greater extent under the action of pesticides. They competed for nutrients required for fungal growth and reduced the use of pesticides to achieve a pollution-free environment to the greatest extent.
Materials and methods
Bacterial strains and culture media
Photosynthetic bacterial PSB-06 and M. oryzae strain Guy11 were provided by the Institute of Plant Protection, Hunan Academy of Agricultural Sciences. PSB-06 was grown in the photosynthetic medium (PM) at 30°C under 6500 lx illumination. The M. oryzae Guy11 strain was cultured on complete medium (CM) agar plates for 3-7 days at 28°C (Talbot et al., 1993). Guy11 was inoculated onto SDC medium plates for conidiation. The concentration was adjusted to 5 × 104 spores/mL before use.
Sensitivity test of M. oryzae to isoprothiolane and tricyclazole
The growth rate assay method was used to identify the sensitivity of M. oryzae to isoprothiolane and tricyclazole. Fungi blocks of 4 mm diameter were cut and inoculated into the medium plates with five concentration gradients of 1, 2, 3, 4, and 5 mg/L. The plates were cultivated in the dark at 28°C for seven days to measure the diameter and calculate the relative inhibition rate.
The calculation formula was:
Inhibition rate = (colony diameter of the control group - colony diameter of the treatment group) /colony diameter of the control group × 100%.
Sensitivity test of appressorium formation to isoprothiolane and tricyclazole
For conidiation, 10-day-old conidia were collected with 5 ml of distilled water, filtered through three layers of lens paper and counted with a haemacytometer under a microscope. Conidial germination and appressorium formation were measured on a hydrophobic surface, 30 μL conidia containing 1, 2, 3, 4, and 5 mg/L fungicide, 5‰ (v/v) methanol as control. Appressorium formation rate was counted at 12 h post-inoculation (hpi) under the microscope, more than 200 appressoria were counted for each strain, Photographs were taken at 24 hpi. The experiments were repeated three times.
Effect of isoprothiolane or tricyclazole on PSB-06
After culturing PSB-06 for 5 days, 1 mL of the culture was added to a 120-mL serum bottle containing 100 mL of the photosynthetic medium. Cultures were diluted to 2 × 109 CFU /mL before use (Zhang et al., 2020). To the flasks, isoprothiolane was added to final concentrations of 1, 0.5, and 0.3 mg/L; tricyclazole was added to final concentrations of 2.5, 1.25, and 0.8 mg/L. The mixed cultures were incubated under anaerobic conditions at 30°C and 6,500 lx illumination. After 2, 4, and 6 days, the absorbance of the cultures in the flasks of each treatment group was measured at 600 nm using a microplate spectrophotometer (BioTek Instruments, VT, USA). At 6 days post-infection (6 dpi) (the first day after inoculation), The contents were dispersed on a solid medium plate of photosynthetic bacteria, and the colonies were counted after seven days. The growth of the photosynthetic bacteria under different treatment conditions was determined by the optical density of the culture. The number of colonies was counted to represent the number of viable bacteria. Experiments were independently repeated at least thrice.
Greenhouse experiments
The plant infection test was performed on 4-leaf-sized susceptible rice seedlings from O. sativa cultivar CO39 using 5 mL of the conidial suspension. The experimental treatment was divided into six groups: Group 1: Ck; Group 2: 2 × 106 CFU/mL of PSB-06; Group 3: isoprothiolane to achieve a final concentration of 0.3 mg/L; Group 4: tricyclazole to reach a final concentration of 0.8 mg/L; Group 5: 2 × 106 CFU/mL of PSB-06 + isoprothiolane to achieve a final concentration of 0.3 mg/L; Group 6: 2 × 106 CFU/mL of PSB-06 + tricyclazole to reach a final concentration of 0.8 mg/L. The inoculated rice was incubated at 28°C and 90% humidity, cultivated in the dark for 24 h and then transferred back to another moist chamber with a photoperiod of 12 h under fluorescent lights. The disease severity was evaluated at 7 days after inoculation (Chen Y. et al., 2014). Approximately 6 cm long diseased rice blades were photographed to evaluate the virulence of different group.
Field experiments
The field experiment (2021) was conducted in Chunhua (Hunan Province, China). The Nongxiang 42 cultivar rice variety was selected as the control target during the investigation. The soil was cultivated and fertilized according to appropriate agronomic guidelines. The trials included 7 treatment regimens, each with 4 replicates. Each area was 50 square meters. Using the random square method, the following processing method was used: Treatment 1: Control (water); Treatment 2: 2 × 106 CFU/mL of PSB-06; Treatment 3: 6 × 106 CFU/mL of PSB-06; Treatment 4: 1 mg/L of isoprothiolane; Treatment 5: 2.5 mg/L of tricyclazole; Treatment 6: 6 × 106 CFU/mL of PSB-06 + 0.8 mg/L of isoprothiolane; Treatment 7: 6 × 106 CFU/mL of PSB-06 + 2 mg/L of tricyclazole. The treatment was administered using a hand-held sprayer. According to local weather conditions, the fungicide were sprayed three times in a row, every 15 days apart. No other fungicides were applied for controlling rice blast in the experimental field. Experiments were independently repeated at least thrice.
After 15 days of the last spray, 50 rice plants were randomly selected from each experimental area for individual testing. Disease severity and control efficacy were calculated as follows:
Disease severity (%) = [R (number of diseased plants with this index × disease index) /(total number of plants investigated × highest disease index)] × 100.
Control efficacy (%) = [(disease severity of control - disease severity of treated group) /disease severity of control] × 100.
Statistical analysis
Statistical data analysis was carried out using the SPSS software (version 17.0, SPSS, Inc., Chicago, IL, USA). One-way analysis of variance (ANOVA) was used to analyze the biocontrol effects. Student's t-test was used to determine any significance between means. p < 0.01 indicated statistical significance.
Results
Sensitivity test of M. oryzae to isoprothiolane and tricyclazole
The effect of different concentrations of isoprothiolane or tricyclazole was further evaluated on the growth of M. oryzae. Our results showed that the growth inhibition rate of tricyclazole and isoprothiolane on Magnaporthe oryzae exceeded 50% at the concentration of 3 mg/L (Figure 1A)and 4 mg/L (Figure 1B), respectively, the two fungicides have the same and significant inhibitory effect on the growth of rice blast.
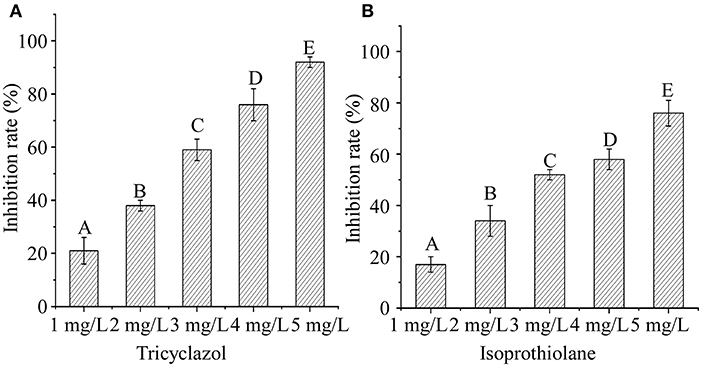
Figure 1. Sensitivity test of M. oryzae to isoprothiolane and tricyclazole. (A,B) The growth inhibition rate is estimated relative to the growth rate of each untreated control. [Inhibition rate = (the diameter of untreated strain – the diameter of treated strain)/(the diameter of untreated strain × 100%)]. Values in columns followed by the same superscript letters indicate no significant difference according to the multiple Duncan test (P < 0.01). Three repeats were performed and similar results obtained.
Sensitivity test of appressorium formation to isoprothiolane and tricyclazole
Conidia, play an important role during M. oryzae infection, we test the sensitivity of appressorium formation to isoprothiolane and tricyclazole. Under fungicide treatment, the biological morphology of conidia did not not changed (Figure 2A). Then we counted the rate of appressorium formation. Compared with the control group (98%), the rate of appressorium formation was 38 and 59% after treatment with tricyclazole and isoprothiolane at the concentration of 1 mg/l, and the inhibition rate of two fungicides on appressorium formation was the same at the concentration of 5 mg/l (Figures 2B,C). The experimental results showed that the two fungicides had significant inhibition on appressorium formation.
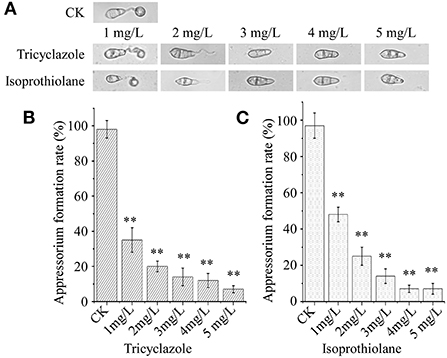
Figure 2. Fungicide inhibits appressorium formation. (A) Morphological observations of conidia. (B,C) Biostatistics of appressorium formation, formation rate = normally formed appressorium / total appressorium × 100% (p < 0.01). CK: 5‰ (v/v) methanol.
Effect of isoprothiolane or tricyclazole on strain PSB-06
The photosynthetic bacteria were co-cultured with different concentrations of the two fungicides. The growth of the PSB-06 was not inhibited at isoprothiolane concentrations lower than 0.3 mg/L (Table 1) and tricyclazole concentrations lower than 1.25 mg/L (Table 2). The optical densities (OD600) were 0.749, 1.319, 0.801, and 0.809 at 6 dpi, respectively. Further, the number of CFU of PSB-06 detected on the plate was similar to the optical density result. With increasing concentrations, both fungicides significantly inhibited the growth of PSB-06. At the working concentration of tricyclazole (2.5 mg/L) and isoprothiolane (1 mg/L), the optical densities of the PSB-06 were 0.231 and 0.475 at 6 dpi, respectively.
Plant infection assays
It was interesting to understand the effect of combining PSB-06 with the two fungicides and check the antagonism against rice blast. Hence, different concentrations of the suspensions (PSB-06 and fungicides) were sprayed on 2-week-old rice seedlings. After seven days post-infection, the spores produced tiny and restricted lesions on the rice leaves in the different treatment groups compared with the control group (Figure 3A). Statistical analysis showed that the lesion density (5 cm2) was only 11 after the combined treatment of isoprothiolane with PSB-06. Moreover, the controlling effect was higher than in other treatment groups (Figure 3B). Thus, the fungicide mixed with PSB-06 exerted better control.
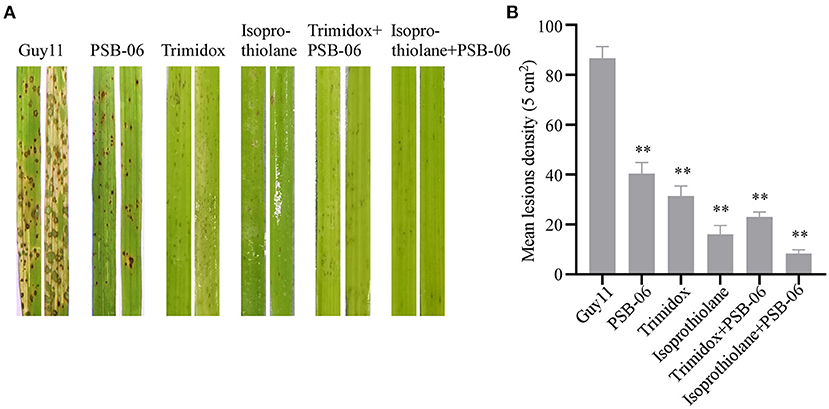
Figure 3. Pathogenicity assay under different treatments. (A) Leaf spraying assay, four milliliters of conidia suspension (5 × 104 spores/ml) of each strain were sprayed on two-week old rice seedlings. Diseased leaves were photographed at 7 day after inoculation. (B) Lesion density was performed by counting lesion numbers of unit area.
Field experiments
The field test results were consistent with the greenhouse test; each treatment significantly reduced disease occurrence (Table 3). The preventive effect of combining PSB-06 and isoprothiolane or PSB-06 and tricyclazole was higher than that of the individual treatment. The treatment group 5 (2 × 106 CFU/mL of PSB-06 + 0.8 mg/L isoprothiolane) was the most efficient and reduced by 76.06%. The effect of group 6 (2 × 106 CFU/mL of PSB-06 + 2.5 mg/L tricyclazole) was 72.02%, The least efficient preparation was 2 × 106 CFU/ml PSB-06, resulting in a control efficacy of 47.28%, The effect of high concentration treatment is higher than that of low concentration when applied separately. The controlling effect of the group with a higher concentration (6 × 106 CFU/mL) of PSB-06 was 67.99%, and that of the individual fungicides isoprothiolane and tricyclazole was 70.07 and 65.46%, respectively. These results indicate that bactericide and PSB-06 have synergistic bacteriostatic effect, and the synergistic effect of the two fungicides and PSB-06 could control rice blast more effectively.
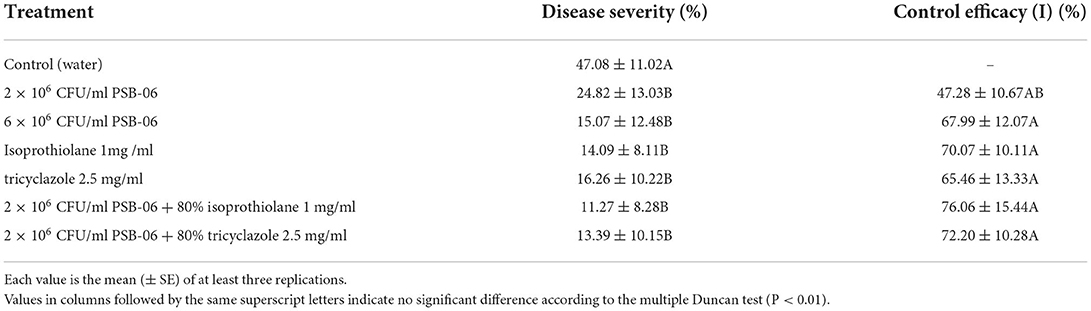
Table 3. Effect of combinations of PSB-06 and Isoprothiolane or Tricyclazole on controlling rice blast in the field plots.
Discussion
Rice blast causes great harm to agricultural production, and fungicides are the first line of treatment for controlling rice blast. However, fungicides cannot be degraded in time. For example, 4 to 11 distinct fungicidal compounds were detected in 81 samples from vineyards sprayed with fungicides. The ecotoxicological potential of some mixtures in the water stream exceeded the threshold set by the unified principle (Bundschuhab et al., 2016). In Japan, large amounts of pentachloronitrobenzene (PCNB) and major biodegradation products, pentachloroaniline (PCA) and pentachlorothioanisole (PCTA), were detected in agricultural areas (Fushiwaki et al., 1990). Fungicides are generally toxic and pose a threat to human health. Fungicidal residues in the environment can contaminate water, soil, and food. Hence, it is essential to choose green control methods.
Biological control is convenient for local production and local application. Biological control measures have a wide range of applications and broad development prospects compared with chemical control. These measures protect and improve farmland ecological environment, do not pollute the environment, reduce the remaining amount of residual poison, and are safe for humans and animals. Moreover, they benefit by delaying the occurrence and development of fungal drug resistance. They also have a continuous and lasting inhibitory effect on some fungi when used constantly. Numerous examples demonstrate the advantages of combining biological and chemical control methods to achieve more effective sterilization and less environmental pollution in the recent upsurge of integrated disease management. Combining azoxystrobin with Bacillus subtilis under controlled conditions showed better results against P. xanthii than azoxystrobin alone (Gilardi et al., 2008). Two botanical fungicides, Wanis 20 EC and Damet 50 EC, combined with Pseudomonas fluorescens 1, reduced the wilt incidence significantly under greenhouse (64%) and field conditions (75%) (Akila et al., 2011). In addition, combined use accelerates the degradation of fungicides. Photosynthetic bacteria significantly degrade organophosphorus fungicides and chemical fungicides containing ester bonds.
In this study, the effects of two fungicides were analyzed on strain PSB-06. Tricyclazole (1.25 mg/L) or isoprothiolane (0.3 mg/L) did not inhibit the growth of strain PSB-06 (Tables 1, 2). Zhang et al. found that photosynthetic bacteria effectively degraded the organophosphorus fungicide methamidophos. The degradation rate of the non-organophosphorus fungicide fenpromethrin reached 67.43% (Zhang et al., 2009a,b, 2014; Kim et al., 2011). Therefore, photosynthetic bacteria were selected as the biological control agent.
As shown as our results, tricyclazole and isoprothiolane have significant inhibitory effect on the growth of Magnaporthe oryzae at the concentration of 3 mg/L and 4 mg/L, meanwhile, the concentration of the two fungicides was 5 mg/L, the rate of appressorium germination was only 7%, since conidia play an important role during M. oryzae Infection. Hence, they were found suitable for controlling rice blast. However, the present study demonstrated a reduced effect of fungicides in controlling rice blast when used individually. Combining the fungicides with the biocontrol bacterium PSB-06 caused a more significant inhibition of rice blast. The greenhouse and the field experiment results demonstrated a higher control on the rice blast when strain PSB-06 was combined with isoprothiolane compared to their individual treatments. The controlling effect of PSB-06 (6.7 × 106 CFU/mL) on rice blast in the field was 67.99 ±12.07%. This value was lower than that of isoprothiolane (1 mg/mL) (70.07%) and higher than that of tricyclazole (2.5 mg/mL) (65.46%). Co-spraying PSB-06 with a low concentration of isoprothiolane (1 mg/mL) significantly improved the controlling efficiency of rice blast. Thus, to achieve the best biological control, temporary mixing is recommended before spraying. The critical factor for biological control is the efficiency and sustainability by which the agents subsequently inhibit or kill the pathogens; they act on their own or the plant (Kim et al., 2011; Vrieze et al., 2018). The ability to colonize vegetation is key to functioning. PSB-06 grows in a photogenic mode by collecting light energy and separating carbon from carbon dioxide. So, it can adapt to the complex natural field environment with its advantages (Liu et al., 2016).
Our previous study identified a significant increment in the contents of SOD, POD, and chitin after rice was treated with the photosynthetic bacterium. They played an essential role in plant stress resistance (Wu et al., 2021). Su et al. also found that photosynthetic bacteria colonized the leaf periphery of tobacco, producing two plant hormones, 3-indoleacetic acid (3-IAA) and 5-aminolevulinic acid (5-ALA), which promoted plant growth and development (Su et al., 2015, 2017). The controlling efficiency of the current experiment was remarkable. The combination of the antibacterial mechanism of the fungicide and PSB-06 strain improved the controlling effect of rice blast disease.
Combining chemical and biological control is promising. Biological agents exert their antibacterial activity to a greater extent under the action of fungicides. The photosynthetic bacterium PSB-06 was more effective when combined with two fungicides and had better application. It effectively controlled rice blast and reduced the application amount of chemical pesticides to achieve the harmony of green chemistry, high efficiency, and environmental protection.
Data availability statement
The original contributions presented in the study are included in the article/supplementary material, further inquiries can be directed to the corresponding author/s.
Author contributions
YC, DZ, and YL conceived the project. YC, XW, CC, QH, CL, XZ, and XT prepared the samples and performed the research and analyzed the data. YC and XW were major contributor in writing the manuscript. DZ and YL revised the manuscript. All authors read and approved the final manuscript.
Funding
This research was supported by the Natural Science Foundation of China (31972323), the Science and Technology Innovation Program of Hunan Province (2021RC3114), the Natural Science Foundation of Hunan Province (2020JJ3024), the Natural Science Foundation of Hainan Province (321MS107), the Agricultural Science and Technology Innovation Program of Hunan Province (2021CX35, 2021CX71, and 2022CX65).
Conflict of interest
The authors declare that the research was conducted in the absence of any commercial or financial relationships that could be construed as a potential conflict of interest.
Publisher's note
All claims expressed in this article are solely those of the authors and do not necessarily represent those of their affiliated organizations, or those of the publisher, the editors and the reviewers. Any product that may be evaluated in this article, or claim that may be made by its manufacturer, is not guaranteed or endorsed by the publisher.
References
Akila, R., Rajendran, R, Harish, S., Saveetha, K., Raguchander, T, and Samiyappan, R. (2011). Combined application of botanical formulations and biocontrol agents for the management of Fusarium oxysporum f. sp. cubense (Foc) causing Fusarium wilt in banana. Biol. Control. 57, 175–183. doi: 10.1016/j.biocontrol.2011.02.010
Aravindan, S., Yadav, M. K., and Sharma, P. (2016). Biological control of rice blast disease with Trichoderma spp. under upland rice System. Environ. Ecol. 53, 167–173.
Bundschuhab, M., Elsaessera, D., Stanga, C., and Schulz, R. (2016). Mitigation of fungicide pollution in detention ponds and vegetated ditches within a vine-growing area in Germany. Ecol. Eng. 89, 121–130. doi: 10.1016/j.ecoleng.2015.12.015
Chen, M. J., Zhang, C. Z, Zi, Q., Qiu, D. W., Liu, W. X., and Zeng, H. M. (2014). A novel elicitor identified from Magnaporthe oryzae triggers defense responses in tobacco and rice. Plant Cell Rep. 33, 1865–1879. doi: 10.1007/s00299-014-1663-y
Chen, Y., Zuo, R. F., Zhu, Q., Sun, Y., Li, M. Y., Dong, Y. H., et al. (2014). MoLys2 is necessary for growth, conidiogenesis, lysine biosynthesis, and pathogenicity in Magnaporthe oryzae. Fungal Genet. Biol. 67, 51–57. doi: 10.1016/j.fgb.2014.04.001
Dean, R., Kan, J. A. L., Pretorius, Z. A., Kosack, K. E. H., Pietro, A. D., Spanu, P. D., et al. (2012). The Top 10 fungal pathogens in molecular plant pathology. Mol Plant Pathol. 13, 414–430. doi: 10.1111/j.1364-3703.2011.00783.x
Fravel, D. R. (2005). Commercialization and implementation of biocontrol. Ann Rev Phytopathol. 43, 337–359. doi: 10.1146/annurev.phyto.43.032904.092924
Fushiwaki, Y., Tase, N., Saeki, A., and Urano, K. (1990). Pollution by the fungicide pentachloronitrobenzene in an intensive farming area in Japan. Sci. Total Environm. 92, 55–67. doi: 10.1016/0048-9697(90)90321-K
Gilardi, G., Manker, D. C., and Gulino, M. L. (2008). Efficacy of the biocontrol agents Bacillus subtilis and Ampelomyces quisqualis applied in combination with fungicides against powdery mildew of zucchini. J. Plant Dis. Protect. 115, 208–213. doi: 10.1007/BF03356265
Idi, A. (2015). Nor MHM,Wahab MFA, Ibrahim Z. Photosynthetic bacteria: an eco-friendly and cheap tool for bioremediation. Rev. Environ. Sci. Bio/Technol. 14, 271–285. doi: 10.1007/s11157-014-9355-1
Kenneth, F. B. (1987). Evolving concepts of biological control of plant pathogens. Ann Rev Phytopathol. 25, 67–85. doi: 10.1146/annurev.py.25.090187.000435
Kim, Y. C., Leveau, L., Gardener, B. B. M., Pierson, E. A., and Pierson, L. S. (2011). The multifactorial basis for plant health promotion by plant-associated bacteria. Appl. Environ. Microbiol. 77, 1548–1555. doi: 10.1128/AEM.01867-10
Liu, F. G., Tang, X. Q., Sheng, X. Z., Xing, J., and Zhan, W. B. (2016). DNA vaccine encoding molecular chaperone GroEL of Edwardsiella tarda confers protective efficacy against edwardsiellosis. Mol. Immunol. 79, 55–65. doi: 10.1016/j.molimm.2016.09.024
Olson, J. M. (2010). Chlorophyll Organization and Function in Green Photosynthetic Bacteria*. Photochem. Photobiol. 67, 61–75. doi: 10.1111/j.1751-1097.1998.tb05166.x
Prabhakaran, N., Prameeladevi, T., Sathiyabama, M., and Kamil, D. (2015). Screening of different Trichoderma species against agriculturally important foliar plant pathogens. J. Environ. Biol. 36, 191–198.
Qiu, D. W., Dong, Y. J., Zhang, Y., Li, S. P., and Shi, F. C. (2017). Plant immunity inducer development and application. Mol Plant-microbe Interact. 30, 355–360. doi: 10.1094/MPMI-11-16-0231-CR
Shiba, Y., and Nagata, T. (2009). The mode of action of tricyclazole in controlling rice blast. Ann. Phytopathol. Soc. Japan. 47, 662–667. doi: 10.3186/jjphytopath.47.662
Su, P., Feng, T. Z., Zhou, X. G., Zhang, S. B., Zhang, Y., Cheng, J. E., et al. (2015). Isolation of Rhp-PSP, a member of YER057c/YjgF/UK114 protein family with antiviral properties, from the photosynthetic bacterium Rhodopseudomonas palustris strain JSC-3b. Sci. Rep. 5, 16–121. doi: 10.1038/srep16121
Su, P., Tan, X. Q., Li, C. G., Zhang, D. Y., Cheng, J. E., Zhang, S. B., et al. (2017). Photosynthetic bacterium Rhodopseudomonas palustris GJ-22 induces systemic resistance against viruses. Microb. Biotechnol. 10, 612–624. doi: 10.1111/1751-7915.12704
Talbot, N. J. (2003). On the trail of a cereal killer: Exploring the biology of Magnaporthe grisea. Ann. Rev. Microbiol. 57, 177–202. doi: 10.1146/annurev.micro.57.030502.090957
Talbot, N. J., Ebbole, D. J., and Hamer, J. E (1993). Identification and characterization of MPG1, gene involved in pathogenicity from the rice blast fungus Magnaporthe grisea. Plant Cell Online. 5, 1575–1590. doi: 10.1105/tpc.5.11.1575
Uchida, M., and Fukada, M. (1983). Effect of a Rice Blast Controlling Agent, Isoprothiolane, on Nilaparvata Lugens Stal with Different Levels of Susceptibility to Diazinon. Pest Resist. Pest. 421–428. doi: 10.1007/978-1-4684-4466-7_18
Vinalea, F., Sivasithamparamb, K., Ghisalbertic, E. L., Marraa, R., Woo, S. L., Loritoa, M., et al. (2008). Trichoderma–plant–pathogen interactions. Soil Biol. Biochem. 40, 1–10. doi: 10.1016/j.soilbio.2007.07.002
Vrieze, D. E., Germanier, F., Vuille, N., and Weisskopf, L. (2018). Combining different potato-associated pseudomonas strains for improved biocontrol of phytophthora infestans. Front. Microbiol. 9, 2018. doi: 10.3389/fmicb.2018.02573
Woo, S. L., and Lorito, M. R. (2006). The molecular biology of the interactions betweentrichoderma spp, phytopathogenic fungi, and plants. Phytopathology. 96, 181–185. doi: 10.1094/PHYTO-96-0181
Wu, X. Y., Chen, Y., Li, C. G., Zhang, X., Tan, X. Q., Lv, L., et al. (2021). GroEL protein from the potential biocontrol agent Rhodopseudomonas palustris enhances resistance to rice blast disease. Pest Manage. Sci. 77, 5443–5435. doi: 10.1002/ps.6584
Zhang, S. B., Zhang, D. Y., Liu, Y., Luo, X. W., Cheng, F. X., Luo, Y. H., et al. (2009a). Degradation characteristics and pathway of fenpropathrin by Rhodopseudomonas sp. strain PSB07-6. Fresenius Environ. Bull. 18, 2060–2065.
Zhang, X., Li, X. N., Chen, Y., Tan, X. Q., Su, P., Zhang, D. Y., et al. (2020). Integrated control of potato late blight with a combination of the photosynthetic bacterium Rhodopseudomonas palustris strain GJ-22 and fungicides. BioControl. 65, 635–345. doi: 10.1007/s10526-020-10026-x
Zhang, S. B, Zhu, C. H., Liu, Y, and Zhang, D. Y. (2014). Biodegradation of fenpropathrin by a novel Rhodopseudomonas sp. strain PSB07-8. Int. J. Environ. Eng. 6, 55–67. doi: 10.1504/IJEE.2014.057833
Keywords: Magnaporthe oryzae, Rhodopseudomonas palustris, tricyclazole, isoprothiolane, combined application
Citation: Wu X, Chen Y, Chen C, Huang Q, Qin Y, Zhang X, Li C, Tan X, Liu Y and Zhang D (2022) Combining the microbial agent Rhodopseudomonas palustris strain PSB-06 with fungicides for controlling rice blast. Front. Sustain. Food Syst. 6:1072156. doi: 10.3389/fsufs.2022.1072156
Received: 17 October 2022; Accepted: 02 November 2022;
Published: 17 November 2022.
Edited by:
Wei Li, Hunan Agricultural University, ChinaReviewed by:
Delong Li, Qingdao Agricultural University, ChinaWeixiao Yin, Huazhong Agricultural University, China
Yu Chen, Anhui Agricultural University, China
Copyright © 2022 Wu, Chen, Chen, Huang, Qin, Zhang, Li, Tan, Liu and Zhang. This is an open-access article distributed under the terms of the Creative Commons Attribution License (CC BY). The use, distribution or reproduction in other forums is permitted, provided the original author(s) and the copyright owner(s) are credited and that the original publication in this journal is cited, in accordance with accepted academic practice. No use, distribution or reproduction is permitted which does not comply with these terms.
*Correspondence: Yue Chen, hnsfcy@126.com; Yong Liu, haoasliu@163.com; Deyong Zhang, dyzhang78@163.com