- 1College of Pharmacy and Food Science, Zhuhai College of Science and Technology, Zhuhai, China
- 2School of Pharmacy, Macau University of Science and Technology, Taipa, Macau SAR, China
- 3College of Life Science, Jilin University, Changchun, China
The objectives of this study are to optimize the ultrasonic microwave synergistic extraction (UMSE) of anthocyanins from Lycium ruthenicum Murr (ALRM) by response surface methodology and also to investigate its antioxidant activity in vitro. Based on the single-factor experiments, we optimized the ALRM extraction process by response surface methodology assuming anthocyanin extraction rate as the response point and microwave power, ultrasonic power, extraction time, and liquid-to-material ratio as the investigating parameters as well as performed correlation analysis between DPPH·, ·OH, ABTS·, O radical scavenging potential, and ferric-reducing antioxidant power. The results showed that the influence on anthocyanin yield was in the order of liquid to material ratio > microwave power > ultrasonic power > extraction time; the elicited extraction conditions were: ultrasonic power 216.253 W, microwave power 89.311 W, time 26.141 min, liquid to material ratio 17.294 mL/g, the forecast content was 10.157 mg/g, while the actual value was consistent with the preview value. Meanwhile, ALRM obtainable by UMSE was identified as yielding favorable antioxidant properties firstly, when the concentration of purified ALRM (ALRM-1) was increased, the antioxidant capacity was strengthened. Theoretically, we provide a basis for the extraction procedure of ALRM and its antioxidant activity, which serves as a promising antioxidant and free radical scavenger.
Introduction
Lycium ruthenicum Murr (LRM) originates from the Solanaceae genus of Lycium and occurs abundantly along northern Shaanxi, Ningxia, Gansu, Qinghai, Xinjiang, and Tibet in China. LRM has a history of utilization in both Chinese Tibetan medicine and Uyghur medicine, which is known as “Punyak” in Tibetan medicine, documented in the Four Medical Classics of Tibetan Medicine as the principal treatment against heart fever, heart disease, irregular menstruation, menopause, and so forth. According to the Uyghur Pharmacopeia, the fruit and root bark of LRM were commonly prescribed for conditions such as urinary tract stones, ringworm and scabies, and bleeding gums, and in folklore as a tonic for strengthening as well as an antihypertensive (Guli et al., 2009) LRM with a sweet taste and neutral personality has many nutrients including proteins, polysaccharides, polyphenolic compounds, anthocyanins, flavonoids, trace elements, and other natural constituents (Li et al., 2010; Hang et al., 2016; Deng et al., 2021). Being safe and non-toxic natural pigments, anthocyanins have a variety of physiological features consisting of antioxidant, hypoglycemic, and antibacterial effects. With a deeper appreciation of Phyto-natural pigments, anthocyanins with distinct biological activities have promising prospects for exploitation (Duan et al., 2008; Tonon et al., 2010; Basharat et al., 2016; Li et al., 2017; Wang M. W. et al., 2022).
Since the introduction of the free radical theory of aging (Harman, 2009; LioChev, 2013; Shields et al., 2021), several types of antioxidants have been at the peak of scientific research, and natural antioxidants have the characteristics of good stability, safety, no side effects, and easy accessibility. Excessive free radicals in human cells, when not removed in time, might attack biological macromolecules within the body and organelles, causing a sequence of oxidative stimulation reactions which will accelerate the aging process and induce various diseases, like diabetes, cancer, coronary heart disease and Alzheimer's and other disorders (Poljsak et al., 2013; Viña et al., 2013, 2018; Lushchak, 2014; Boengler et al., 2017; Pomatto and Davies, 2018). Meanwhile, the chemically synthesized antioxidants in the market could eliminate free radicals and mitigate oxidative stress effectively, although these agents have adverse side effects on health. Consequently, efficient and low-toxic antioxidants from green and natural plants have become one of the essential avenues to locate cost-effective and sustainable antioxidants in the field of functional foods (Zhang et al., 2012; Fei et al., 2017; Kim et al., 2020; Zulfiqar and Ashraf, 2021), and in particular, they are of tremendous interest in plants with homologous medicine-food properties. Being a native plant component, LRM possesses a plentiful resource of anthocyanins, which have been mentioned in many existing reports (Coklar and Akbulut, 2017; Hu et al., 2021; Li et al., 2021; Lim et al., 2021; Wang et al., 2021) with certain antioxidant activity, but few reports have been available for the optimization of anthocyanin extraction from LRM.
The current extraction method commonly used for the extraction of ALRM is solvent-based, which is not only time-consuming but also inferior in terms of safety and the residual of external solvents (Cai et al., 2016; Farooq et al., 2020; Tan et al., 2022). Thus, a novel, high-efficiency, fail-safe anthocyanin extraction technique would be necessary. Ultrasonic Microwave Synergistic Extraction (UMSE) can have advantages in terms of low energy consumption, excellent extraction efficiency, short-term, high security, and so on (Chen et al., 2016; Kwansang et al., 2022). The objectives of this work were to optimize the process conditions for the extraction of ALRM by UMSE and to assess its feasibility via a response surface methodology (RSM) employing a Box-Behnken design (BBD) to evaluate the process conditions for the extraction of ALRM using the anthocyanin concentration as an indicator and LRM as a research target. Since little research has been reported on the antioxidant activity of ALRM, the magnitude of the antioxidant activity of anthocyanins, in a certain meaning, may also determine its application potential in food. It is based on the one-factor experiment, the process was optimized by general RSM, the purification of ALRM was performed by AB-8 resin, and eventually, the scavenging capability of free radicals and the reducing ability of ALRM and vitamin C (VC) resulted from UMSE were comparatively probed for its antioxidant activity in vitro to establish the foundation of the efficient exploitation of LRM by-products and to upgrade the added valuation of LRM. At the same time, it would provide a conceptual basis for the in-depth processing of LRM.
Materials and methods
Materials
LRM (Xinjiang Heiguo Wolfberry Biotechnology Co., Ltd., China) picked in July 2020 was authenticated by Runrong Zhang, senior engineer of traditional Chinese medicine, College of Pharmacy and Food Science, Zhuhai College of Science and Technology, Zhuhai, Guangdong Province, China.; 30% H2O2, Ferrous sulfate heptahydrate, Tris(hydroxymethyl)aminomethane (Tris), Biphenyl triol, Potassium persulfate, Salicylic acid, Potassium ferricyanide, Trichloroacetic acid Potassium chloride, Ferric chloride, Ethanol, Sodium acetate trihydrate, Hydrochloric acid, Citric acid (AR, Shantou Xilong Science Co., Ltd., China); DPPH (AR, Shanghai Aladdin Biochemical Technology Co., Ltd., China); ABTS and Ascorbic acid (AR, Shanghai Yuanye Biotechnology Co., Ltd., China).
Extraction and purification of anthocyanins
The leaves of LRM were removed, dried to constant weight at a constant temperature of 35°C, crushed, and sieved to obtain the dry LRM powder. 1.000 g of LRM dried powder was weighed accurately and placed in a round-bottom flask; 1% HCl-70% ethanol (1:99, v/v) supplemented with ultrasonic microwave co-extraction at constant temperature and avoiding light; the supernatant was collected by centrifugation at 6,000 r/min for 10 min; acidic ethanol was fixed to 50 mL, and then the extract was obtained. The extracts were determined for content, and the optimized extraction method was screened by RSM based on one-factor experiments. According to the optimal conditions of RSM, ALRM concentrates were obtained and purified by adsorption on AB-8 macroporous resin and concentrated and dried under reduced pressure. It was finally purified to yield ALRM-1, and the extraction and purification procedure is shown in Figure 1.
Determination of anthocyanin content
The absorbance of anthocyanin extracts in pH 1.0 and 4.5 buffer solution was measured by scanning the extracts with a UV spectrophotometer in the range of 400–700 nm, and the wavelength (527 nm) at which the maximum difference in absorbance occurred was the best wavelength for the determination. The extracts were taken as 500 μL and fixed to 25 mL with pH 1.0 and 4.5 buffers, respectively, mixed uniformly, and then equilibrated in a constant temperature water bath at 37°C for 30 min as the experimental group. The extract was treated with the same conditions using 1% acidic ethanol instead of the extract and equilibrated for 30 min as the blank cohort. Once the equilibration was finished, the absorbance at 527 and 700 nm, respectively, were measured. The total anthocyanin content was calculated according to the following formula. In which, MW: molar mass of Cyanidin-3-O-glucoside chloride 449.2 g/mol; DF: dilution factor, 50; V: volume of extraction solution, 50 mL; ε: molar extinction coefficient 26,900 L/(mol-cm); L: light range cm, 1 cm; m: mass of LRM (g).
One-factor experiment for anthocyanin extraction
Effect of ultrasonic power on extraction
The extraction of ALRM was carried out at microwave power 130 w and ultrasonic power 100, 150, 200, 250, and 300 w for 20 min at a liquid-to-material ratio of 20:1 mL/g, respectively, with 70% ethanol aqueous solution at an acidity of 1% as the extraction solvent.
Effect of microwave power on extraction
The extraction of ALRM was carried out with an acidic 1% aqueous 70% ethanol solution as the extraction solvent at an ultrasonic power of 200 w, an extraction temperature of 50°C, and an extraction solution to a liquid-to-material of 20:1 mL/g for 20 min at different microwave powers of 50, 90, 130, 170, and 210 w.
Effect of extraction time on extraction
The extracting solvent was 70% ethanol aqueous solution with an acidity of 1%, ultrasonic power of 200 w, and microwave power of 130 w. The extraction temperature was set at 50°C, and ALRM was extracted for 20 min at different liquid-to-material ratios of 10:1, 15:1, 20:1, 25:1, and 30:1 mL/g.
Effect of liquid-to-material ratio on extraction
The extraction was carried out at 50°C at different liquid-to-material ratios of 10:1, 15:1, 20:1, 25:1, and 30:1 mL/g for 20 min each while using 70% ethanol aqueous solution with 1% acidity as the extraction solvent and 200 w ultrasonic power and 130 w microwave power.
Design of RSM
The method in this subsection incorporates the RSM from the literature (Song et al., 2011) with appropriate modifications. To further investigate the interaction effect relationship between the variables, response surface analysis was used to screen the optimal extraction process. The initial study of individual factors was the guiding scope for response surface optimization. Based on the BBD principle, four influencing factors of content: ultrasonic power, microwave power, extraction time, and liquid-to-material ratio were selected for a 4-factor, 3-level RSM. The factor levels of RSM are shown in Table 1. The BBD combination principle was implemented to design a response surface optimization test using anthocyanin content as the response value Table 2. The experimental data were analyzed using Design-Expert 10.0.3 software to obtain the quadratic multinomial regression equation: content (mg/g) = 10.0194 + 0.211833* A-0.269417 * B + 0.10325 * C + 0.389167 * D + 0.478 * AB+0.17275* AC + 0.78425 * AD-0.21125 * BC + 0.299 * BD + 0.47975 * CD - 0.927367 * A ∧ 2-0.748492 * B ∧ 2-0.841492 * C ∧ 2-0.815117 * D ∧ 2 (coding system) (A :the ultrasonic power/(W), B: the microwave power/(W), C : the extraction time/(min), D : the liquid-to-material ratio/(mL/g)). [The RSM was firstly examined by determining a good one-way experimental design, and the results of the experiment were used to determine the high, medium, and low levels (1, 0, −1) of each factor (independent variable) separately.]
Determination of the color value of ALRM after purification
The absorbance at 527 nm of the purified anthocyanin was measured by dissolving 0.01 g in 100 mL of pH = 3.0 buffer, and the absorbance was stabilized at 0.2–0.7 in the following formula.
Where CV is the color value; A is the absorbance value (OD527nm); F is the dilution multiple; W is the sample mass (g).
In vitro antioxidant effect of ALRM
Measurement of DPPH· radical scavenging rate
Antioxidant sample and VC control solvent preparation: ALRM-1 sample solution and VC standard solution at concentrations of 4, 2, 1, 0.5, 0.25, 0.1, and 0.05 mg/mL were prepared for determination. Weighed 7.886 mg of DPPH·, fix the volume of anhydrous ethanol into a 100 mL brown volumetric flask, sonicate to dissolve it fully, to prepare 0.2 mmol/L of anhydrous ethanol DPPH· solution (Solution A), shake well, and protect from light. One milliliter of ALRM-1 solution was mixed thoroughly with solution A, protected from light for 1 h and the absorbance was measured at 517 nm as A1. An equal amount of anhydrous ethanol was substituted for solution A. The solution was mixed thoroughly and left to stand for 1 h protected from light, and the absorbance was measured as A2 as above. An equal amount of distilled water was pipetted in place of the anthocyanin solution and mixed thoroughly with solution A. The absorbance was measured for A3 after standing for 1 h protected from light. VC was used as the standard solution for the control experiment. The above method was drawn from that in the literature (Mi et al., 2019), which is suitably modified.
Measurement of ·OH radical scavenging rate
After weighing 2.502 g of Ferrous sulfate heptahydrate and fixed in distilled water to 1 L, the solution was dissolved by sonication to obtain a 9 mmol/L Ferrous sulfate solution (Solution A). Weigh 1.243 g salicylic acid, fix the volume to 100 mL with anhydrous ethanol, dissolve it by sonication, fill with anhydrous ethanol and fix the volume to obtain a 9 mmol/L salicylic acid solution (Solution B). After pipetting 90 μL of 30% hydrogen peroxide solution in 100 mL of distilled water and fixing the volume, obtain a 9 mmol/L hydrogen peroxide solution (Solution C). The absorbance of 1 mL of ALRM-1 solution was determined as A1 at 510 nm by adding 1 mL of solutions A, B, and C in sequence and mixing thoroughly, and then standing in a water bath at 37°C for 30 min. Distilled water was substituted for the sample solution and the absorbance value measured by repeating the operation was A2. Two milliliter of distilled water and 1 mL of anhydrous ethanol were substituted for solutions A, B, and C as well as the absorbance value measured by repeating the operation was A3. VC solution was substituted for the sample as a control experiment. The above method was drawn from that in the literature (Wang and Jiao, 2000), which is suitably modified.
Measurement of ABTS· radical scavenging rate
An accurate amount of ABTS 0.192 g was weighed, dissolved by ultrasonication in distilled water, and fixed in a 50 mL brown volumetric flask (Solution A). Then weighed 67 mg of potassium persulphate was dissolved by ultrasonication in distilled water, and fixed in a 50 mL volumetric flask (solution B).Solution A: solution B was thoroughly mixed at room temperature and protected from light for 16 h. Combine solution A: solution B = 1:1 and mix thoroughly, then react for 16 h at room temperature and avoid light to obtain ABTS· Master which was diluted with phosphate buffer to give an absorbance value of 0.70 ± 0.02 at 734 nm and stored away from light. Take 1 mL of ALRM-1 solution, add 3 mL of ABTS· solution, react at room temperature and avoid light for 1 h. Measure the absorbance value at 734 nm and record it as A1. Replace ABTS· solution with solution B and repeat the operation to calculate the absorbance value as A2. Alternatively, 1 mL of distilled water was used in place of the ALRM-1 solution and the absorbance value was recorded as A3. VC solution was used in place of the sample for the control experiment. The calculated formula is the same as for the DPPH· radical scavenging rate (%). The above method was drawn from that in the literature (Huang et al., 2022), which is suitably modified.
Measurement of O radical scavenging rate
Precisely weighed 1.21 g Tris, dissolved by ultrasonication, and fixed in distilled water to 100 mL to obtain 0.1 mol/L Tris solution. Take concentrated hydrochloric acid and dilute it to 0.1 mol/L HCl solution. Dilute 0.1 mol/L Tris to 50 mmol/L and adjust pH = 8.2 with 0.1 mol/L HCl solution to arrive at Tris-HCl buffer (Solution A). A dilution of 0.1 mol/L HCl was taken to obtain a 1 mmol/L HCl solution, then 73 mg of biphenyl triol was added, dissolved by sonication, and shaken to obtain a 60 mmol/L biphenyl triol solution (solution B). Concentrated hydrochloric acid was removed and allowed to dilute to a 10 mol/L HCl solution. Then it was added to 2.25 mL of solution A, 2 mL of ALRM-1 solution, and 50 μL of solution B. The reaction was terminated by adding 20 μL of 10 mol/L HCl immediately after 4 min. The absorbance was measured at 325 nm as A1. Two milliliter of distilled water was used in place of the anthocyanin sample and the absorbance was repeated as A2. 1 mmol/L HCl solution was used in place of solution B and the absorbance was repeated as A3. VC solution was substituted for the sample as a control experiment. The calculated formula is the same as for the ·OH radical scavenging rate (%). The above method was drawn from that in the literature (Liu et al., 2017), which is suitably modified.
Measurement of ferric-reducing antioxidant power
Then added 2.5 mL of phosphate buffer solution (0.2 mol/L, pH = 6.6) and 2.5 mL of 1% Potassium ferricyanide solution, mixed well, cooled rapidly after the reaction at 50°C for 20 min, added 2.5 mL of 10% trichloroacetic acid solution, and centrifuged at 4,000 r/min for 15 min. 2.5 mL, add 2.5 mL of distilled water and 0.5 mL of 0.1% Ferric chloride solution, mix well and react for 10 min, measure the absorbance value at 700 nm, replace the anthocyanin sample solution with distilled water and repeat the operation as a blank group. The VC solution was applied instead of the sample as the control experiment. The above method was drawn from that in the literature (Wang Y. et al., 2022), which is suitably modified.
Statistical analysis
Data are shown as mean ± SEM, and statistical analysis was performed using Origin 2021, Graphpad Prism 8, Design-Expert 10.0.3, and EXCEL 2019. To compare the differences between multiple groups, a one-way analysis of variance (ANOVA) was performed in this software. P < 0.05 indicates statistical significance.
Results
Analysis of one-factor experiment
Analysis of ultrasound power on extraction
As shown in Figure 2A, the extraction efficiency is increased because of the cavitation effect of ultrasound, which accelerates the rupture of plant cells (Maran et al., 2013), thus having a wider contact area and increasing the rate of solvent penetration into the system so that the anthocyanins can be adequately solubilized. Eventually, 150, 200, and 250 w were picked as the three levels of subsequent RSM.
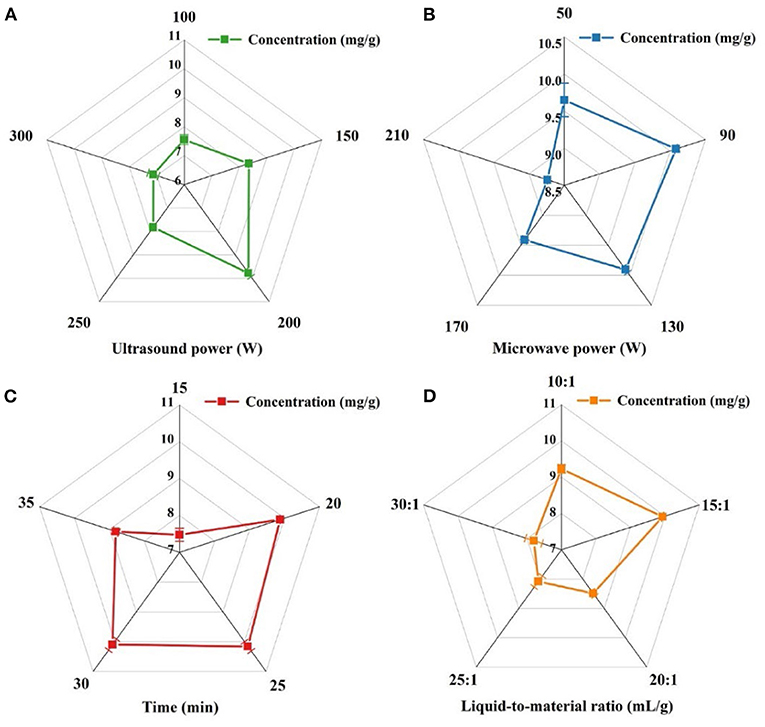
Figure 2. Effect of ultrasonic power (A), microwave power (B), extraction time (C), and liquid-to-material ratio (D) on the extraction rate of LRM.
Analysis of microwave power on extraction
As shown in Figure 2B. An extraction rate of anthocyanins was found to increase with increasing microwave power when the microwave power was increased to within 100 W. The absorption of microwave energy led to accelerated thermal heating and increased internal pressure, which prompted the rupture of the plant materials. It is because, with the increment of microwave power, the collapse of the cell barrier structure was ruptured (Zhang et al., 2008; Yan et al., 2010). With microwave power of more than 100 W, the extraction rate becomes smaller, and microwave energy may provide too much energy to the system, affecting intermolecular interactions. Based on the experimental results, three microwave powers, 50, 100, and 150 W were chosen to make the highest extraction rate of anthocyanins as the three levels of microwave power for the subsequent RSM.
Analysis of extraction time on extraction
For the variation of extraction time from 15 to 25 min, as shown in Figure 2C, the extraction rate increased with increasing extraction time because more energy was absorbed by the solution as time increased, leading to heat build-up in the solution and increasing the mass transfer rate (Marana et al., 2013). However, extraction rates decreased for extraction times >25 min, which may be due to longer sonication times and microwave times leading to anthocyanin degradation (Chen et al., 2015). Therefore, the three points with the highest extraction rates, 20, 25, and 30 min, were selected as the three levels of extraction time for subsequent RSM.
Analysis of liquid-to-material ratio on extraction
Figure 2D shows that the extraction rate reaches a maximum when the liquid-to-material ratio is 15:1, due to the acceleration of anthocyanin release to the solvent as the liquid-to-material ratio increases and there is a higher osmotic pressure inside and outside the cell (Yan et al., 2010). However, as the liquid-to-material ratio gradually increased, the extraction rate decreased, due to the saturated solvent limiting the rate of mass transfer (Marana et al., 2013) and the consequent increase in viscosity of the solvent, which was in a position to limit the formation of cavities due to sonication, resulting in a decrease in extraction rate (Chen et al., 2015). Hence, 10:1, 15:1, and 20:1 have been chosen as the three levels for the following RSM liquid-to-material ratio.
RSM results and ANOVA
Based on the results of the one-way test, the four-factor, the three-level experiment was selected, as shown in Table 2. An analysis of variance for the regression equation is shown in Table 3. Where the F-value can be used to test the level of significance of the effect of each variable on the response value, the larger the F-value, the more significant the corresponding variable is. The model is considered to be statistically significant when the probability of significance test for the model is P < 0.05. The difference in lack of fit was P = 0.3260 > 0.05, which was not significant, explaining that the model fits well and that the experimental error was small, which implied that the model could be implemented for the determination of ALRM. From Tables 1–3, it can be seen that the process conditions affect the size of the content in the following order: D > B > A > C, i.e., liquid-to-material ratio > microwave power > ultrasonic power > extraction time. The coefficient of determination R2 of the model was 0.9692, indicating a high significance of the model, while = 0.9384, which was able to explain 93.84% of the variation of the experimental response values, and was also close to the predicted correlation coefficient Pred R2, indicating that this experimental model fits well with the real data and has practical guidance significance, thus the model can be adapted to analyze and predict the optimal extraction process for the contents. It can be possible to conduct analysis and predict the optimal extraction process.
The influence of the interaction of different process conditions on the content is shown in Figures 3–8. As can be seen from Figure 3, the trend of the influence of ultrasonic power and microwave power interaction on the content is a parabolic surface distribution, with the content increasing and then decreasing with the increase of both ultrasonic power and microwave power. In comparison, the microwave power caused a greater fluctuation in the surface, indicating that the microwave power made a stronger contribution to the effect of the interaction and had a greater impact on the content. The product content was significantly increased when moderate conditions were taken: ultrasonic power 175–225 W and microwave power 70–100 W range. Figure 4 shows that the interaction surface between ultrasonic power and time has good symmetry, indicating that the two contribute equally to the content of the interaction. The optimized process conditions for content considering only the influence of the two are concentrated around the combination of ultrasound power of 200 W and time of 25 min level. The longitudinal span of the ultrasound power-liquid ratio interaction surface shown in Figure 5 is large and the contours are significantly elliptical, indicating that the interaction between the two has a significant effect on the content. When the ultrasonic power was set at 200–230 W and the liquid-to-material ratio was set at 15–20 mL/g, the extraction process was optimized for the content under the interaction between the two.
As can be seen from Figure 6, when the microwave power is <90 W, there is a positive correlation between the content and the microwave power, while when the microwave power is >90 W, the correlation turns around and the microwave power is taken around 90 W as the critical optimum process parameter for the content. In the interaction shown in Figure 7, the effect of microwave power and liquid ratio on the content of the first rise and then fall, and the liquid ratio is a sensitive factor for the content, to moderate conditions: microwave power 70–100 W, the liquid ratio of 14–18 mL/g or so, to promote the product content. Figure 8 shows that the time-to-liquid ratio interaction caused large fluctuations in the surface, and the contours showed a significant ellipse, indicating that the interaction between the two had a significant effect on the content. The combination of the time level of 25 min and the liquid-to-material ratio level of around 16 mL/g increased the content level.
Optimal process conditions and validation experiments
The average content was 10.535 mg/g, which was close to the predicted result of the model, indicating that the RSM model is a valid method to optimize the extraction process. The results were close to the predictions of the model, indicating that the method of optimizing the extraction process based on the RSM model was effective and feasible. To fully consider the effect of the interaction between the four factors on the content of ALRM-1, and with the maximum content as the optimization target, according to the results of the Design-Expert 10.0.3 software, combined with the feasibility of the actual process settings, the optimal extraction process solution for the content of ALRM-1 under the interaction of the four factors: ultrasonic power, microwave power, time and liquid to material ratio was: ultrasonic power. Consequently, the model predicted ALRM content of 10.157 mg/g under the following conditions: ultrasound power 216 W, microwave power 89 W, extraction time 26 min, liquid to material ratio 17:1 mL/g.
He et al. (2020) investigated the extraction rate of ALRM using hydrochloric acid-ethanol extraction at pH = 30 under different extraction solvent concentrations, material ratios, extraction times, extraction temperatures, and with or without degreasing. The optimum conditions for the extraction of anthocyanins with ethanol were 80% hydrochloric acid-ethanol at pH = 30, a material ratio of 1:15, an extraction time of 5 h, and an extraction temperature of 40°C. The extraction rate of anthocyanins reached 0.978% without degreasing the LRM. Hang et al. (2018) extracted ALRM at an ultrasonic power of 120 W and 40°C for 25 min with an ethanol feed-liquid ratio of 1:10 g/mL and could reach 9.024 mg/g. In the above regard, the extraction efficiency of the ultrasonic microwave co-extraction method was more than that of the ultrasonic-assisted method and the leaching assay.
Analysis of the color value of ALRM after purification
Table 4 shows that the purified anthocyanins were in the form of a purplish red powder with a color value of 57, which was significantly higher than the crude unpurified anthocyanin product, after purification by the macroporous resin AB-8. The sensory evaluation revealed that the unpurified product was more viscous than the purified product, and the comparative table showed that the purified ALRM-1 contained four times as much color value as before purification, uncovering that the AB-8 macroporous resin bears the responsibility of effectively improving the purity of ALRM.
Analysis of ALRM in vitro antioxidant
Figures 9A–E show the in vitro antioxidant capacity of ALRM-1 compared with VC. The anthocyanins were investigated for their ability to scavenge DPPH·, ·OH, ABTS·, O, and its ferric-reducing antioxidant power (FRAP), and VC was used as a positive control. The obtained results demonstrated that the antioxidant capacity of ALRM-1 progressively intensified with increasingly elevated concentrations in the range of 0.05–4 mg/mL. At a concentration range of 4 mg/mL, ALRM-1 was noted to hold a moderate level of antioxidant properties. The scavenging ability of anthocyanins for DPPH· radicals was slightly outweighed by that of VC, and those for DPPH·, ·OH, ABTS·, O radicals, and the FRAP were on par with the corresponding concentrations of VC.
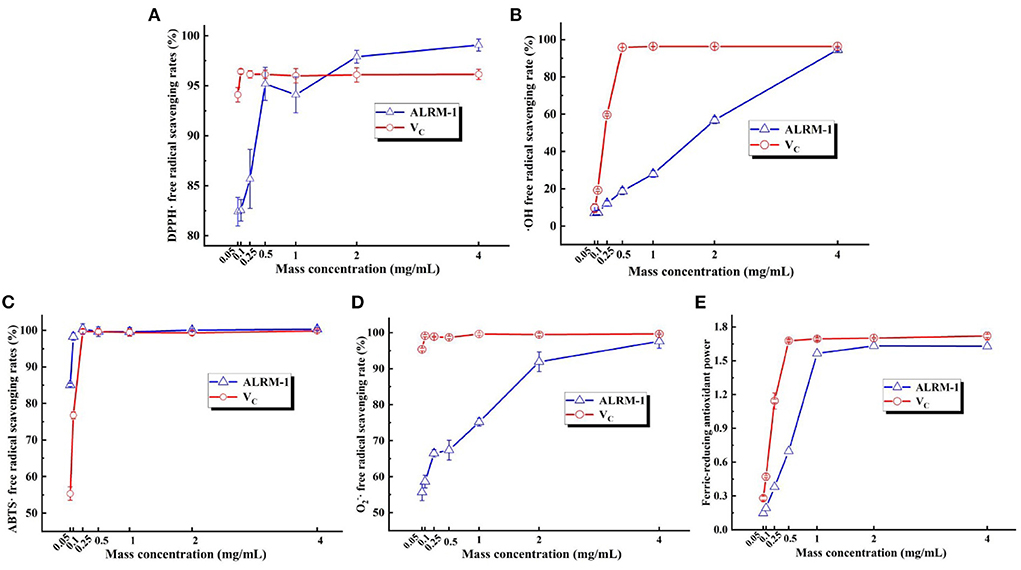
Figure 9. The scavenging radical capacity of ALRM-1 [(A) comparison of the scavenging rate of DPPH· radicals by VC and ALRM-1; (B) comparison of the scavenging rate of ·OH radicals by VC and ALRM-1; (C) comparison of the scavenging rate of ABTS· radicals by VC and ALRM-1; (D) comparison of the scavenging rate of O radicals by VC and ALRM-1; (E) comparison of FRAP by VC and ALRM-1].
Discussion
LRM of the genus Lycium, family Solanaceae, is a characteristic medicinal plant of western China and is widely used in traditional Chinese medicine. Anthocyanins are the main active ingredients in LRM, which have pharmacological effects such as anti-oxidant, anti-aging, cardiovascular protection, anti-cancer, and hyperglycemia (Tan et al., 2022). As the active ingredients in LRM are complex, finding a proven extraction method to separate and purify the active ingredients in Chinese medicine and ultimately obtain effective monomers is an important element in the field of Chinese medicine research. Traditional methods of extracting Chinese medicine ingredients, such as maceration and decoction, have an insufficient performance to meet the needs of modern pharmaceutical production due to poor selectivity, a limited extraction range, easy leaching of a large number of impurities, as well as easy decomposition of active ingredients, which directly affects the quality, production efficiency and economic efficiency of medicines (Marana et al., 2014; Cheng et al., 2015; Xu et al., 2015; Jin et al., 2019). Combining the advantages of both ultrasound and microwave, UMSE utilizes the heating effect of microwave and the cavitation effect of ultrasound. The high thermal energy and ultrasonic vibrations cause the plant cells to rupture and release the bioactive substances into the surrounding solvent. UMSE has the characteristics of low energy consumption, high extraction efficiency, short time, and convenient and secure operation (Lin et al., 2022). In this study, a new anthocyanin extraction process has been optimized for its rational exploitation, and the extraction rate is higher than the traditional process, which will have a rational basis for the development and exploitation of anthocyanin pigments in food, health products, and cosmetics.
As people's living standards and health awareness continue to rise, more and more attention is being paid to health and wellness products. LRM, as a characteristic plant with both medicinal and edible values, is now widely used in food, medicine, cosmetics, and other fields and has a large economic value. The common free radicals include superoxide radicals, lipid peroxide, hydrogen peroxide, singlet oxygen, and hydroxyl radicals. Free radicals are highly biologically active as well as can damage DNA, proteins, and lipids, causing cell mutation, senescence, and apoptosis, suggesting that the gradual accumulation of oxidized cells is a fundamental driver of cellular senescence (Feng et al., 2021; Kucukler et al., 2021). The mechanism of their associated free radical doctrine is shown in Figure 10 The use of antioxidants as natural and synthetic functional food ingredients and dietary supplements have become increasingly popular in recent years. Due to an imbalance in the ratio of antioxidants to free radicals, oxidative reactions can damage proteins, lipids, and DNA. Appropriate methods focusing on the kinetics of reactions involving antioxidants and addressing the mechanisms of antioxidant activity are needed to screen plant-derived compounds for their antioxidant capacity (Gulcin, 2020; Zhong et al., 2022). This paper explores the antioxidant activity of anthocyanins, a highly valuable natural beneficial ingredient unique to LRM, and will add a new theoretical basis for their functional activity and provide a basis for the development and application of functional foods.
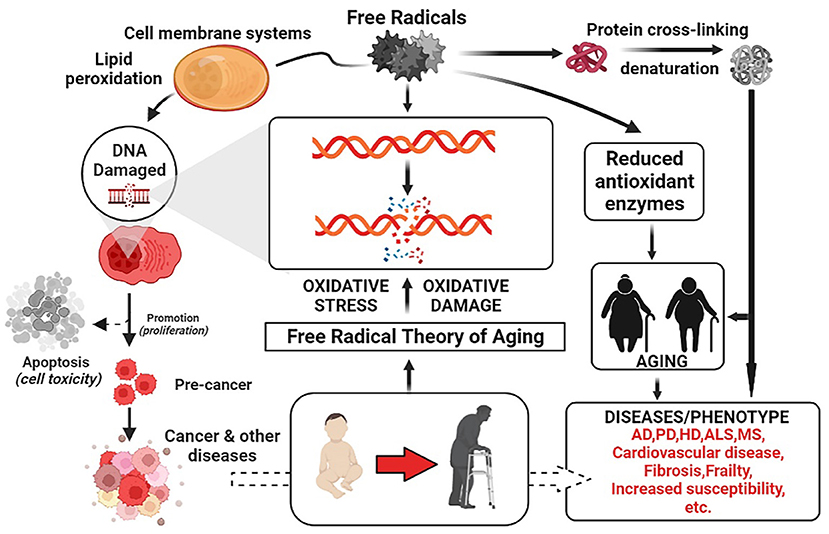
Figure 10. Mechanisms of the free radical doctrine of aging (The figure was created employing online software named BioRender.com).
Studies have found that Xia and Zhao (2014) extracted the polysaccharides, flavonoids, and polyphenols of LRM, and determined the scavenging ability of these three components on diphenyl bitter acyl (DPPH·), hydroxyl radicals (·OH), and superoxide anion radicals (O), as well as on mitochondrial swelling and malondialdehyde (MDA) content in mouse liver, to evaluate the antioxidant activity of the functional components of LRM and their protective effects on the antioxidant activity and mitochondrial protective effects of the functional components of LRM were evaluated. The contents of all were found to be 30.6, 21.3, and 49.5 mg/g, respectively, as well as the ability of flavonoids and polyphenols to scavenge DPPH·, ·OH, and O radicals was stronger than that of polysaccharides. The mitochondrial swelling induced by FeSO4-VC was significantly inhibited by LRM flavonoids (P < 0.01) and polyphenols (P < 0.01), and the mitochondrial MDA content was significantly reduced by LRM flavonoids and polyphenols (P < 0.05). The polysaccharides, flavonoids, and polyphenols of LRM had antioxidant activity, and the protective effect of flavonoids and polyphenols on mitochondria was stronger than that of polysaccharides. Exercise fatigue may arise because free radicals are not scavenged in time causing damage to the organism, and energy substances are depleted and the accumulation of metabolites; simultaneously, liver and muscle glycogen also directly affect exercise endurance and fatigue level. Gavage of polysaccharides from LRM (PLRM) reduces lipid peroxide content in mouse liver tissue, increases serum superoxide dismutase (SOD) values, and has a beneficial impact on maintaining high levels of liver and muscle glycogen content. PLRM has a protective effect on acrylamide (AA)-induced liver injury in rats (Wang et al., 2020). It proved that its flavonoids have antioxidant activity in a certain concentration range. The above indicates that the active ingredients in LRM have antioxidant effects, and LRM is rich in active ingredients, including anthocyanins, but little research has been done on its antioxidant activity, so it is of reference value to investigate the antioxidant function of LRAM obtained from the UMSE method in this work.
As the market for synthetic pigments will shrink with the increasing requirements for food safety, anthocyanins are a natural pigment, and in the future natural pigments will be more popular in the market and offer great potential in the food industry, health products, and cosmetics. As the main component of LRM, anthocyanins have been studied in terms of the chemical composition and pharmacological properties of ALRM, but there are still many issues for further research. For example, research on the extraction methodology of ALRM is mainly focused on traditional extraction methods, and there is less research on new extraction methods in recent years, which should increase the content of its potent substances as an important way to obtain natural pharmaceutical ingredients. In addition, less research has been done on the antioxidant aspects of LRM, and this is a direction that needs to be addressed in future research to develop and study the antioxidant functions of ALRM and its products more thoroughly and comprehensively. This paper focuses on the optimization of ALRM extraction (UMSE) and in vitro antioxidant aspects, with a view to its subsequent widespread application and improved utilization.
Conclusions
The quadratic regression model for the yield of anthocyanins has been established by acquiring ALRM through UMSE with the RSM for the first time. The optimum process conditions were obtained as ultrasonic power of 216 W, microwave power of 89 W, time of 26 min, and the liquid-to-material ratio of 17:1 mL/g. Three sets of validation experiments were carried out under these conditions, yielding 10.535 mg/g of anthocyanins, which equates to approximately the theoretical maximum yield of 10.157 mg/g. It has been suggested that ALRM purified with AB-8 obtained by UMSE has the capability of scavenging DPPH·, ·OH, ABTS·, O radicals, and the FRAP. In addition, its ABTS free radical scavenging properties, outperform that of VC. Hence, it may serve as a reference value and conceptual foundation for the exploitation of professional functional components of ALRM.
Data availability statement
The raw data supporting the conclusions of this article will be made available by the authors, without undue reservation.
Author contributions
Conceptualization and investigation: CY. Data curation and supervision: WZ. Formal analysis: YD, CY, YZ, and DY. Funding acquisition and resources: YD and WZ. Methodology: YD, CY, and DY. Project administration: YD, WZ, YZ, and DY. Software: YD and DY. Visualization: YD, YZ, and DY. Writing—original draft: YZ. Writing—review and editing: DY. All authors contributed to the article and approved the submitted version.
Conflict of interest
The authors declare that the research was conducted in the absence of any commercial or financial relationships that could be construed as a potential conflict of interest.
Publisher's note
All claims expressed in this article are solely those of the authors and do not necessarily represent those of their affiliated organizations, or those of the publisher, the editors and the reviewers. Any product that may be evaluated in this article, or claim that may be made by its manufacturer, is not guaranteed or endorsed by the publisher.
References
Basharat, Y., Khalid, G., Ali, A. W., and Preeti, S. (2016). Health benefits of anthocyanins and their encapsulation for potential use in food systems: a review. Crit. Rev. Food Sci. Nutr. 56, 2223–2230. doi: 10.1080/10408398.2013.805316
Boengler, K., Kosiol, M., Mayr, M., Schulz, R., and Rohrbach, S. (2017). Mitochondria and ageing: role in heart, skeletal muscle and adipose tissue. J. Cachexia Sarcop. Muscle. 8, 349–369. doi: 10.1002/jcsm.12178
Cai, Z., Qu, Z. Q., Lan, Y., Zhao, S. J., Ma, X. H., Wan, Q., et al. (2016). Conventional, ultrasound-assisted, and accelerated-solvent extractions of anthocyanins from purple sweet potatoes. Food Chem. 197, 266–272. doi: 10.1016/j.foodchem.2015.10.110
Chen, C., You, L. J., Abbasi, A. M., Fu, X., and Liu, R. H. (2015). Optimization for ultrasound extraction of polysaccharides from mulberry fruits with antioxidant and hyperglycemic activity in vitro. Carbohyd. Polym. 130, 122–132. doi: 10.1016/j.carbpol.2015.05.003
Chen, F. L., Zhang, X. L., Zhang, Q., Du, X. Q., Yang, L., Zu, Y. G., et al. (2016). Simultaneous synergistic microwave–ultrasonic extraction and hydrolysis for preparation of trans-resveratrol in tree peony seed oil-extracted residues using imidazolium-based ionic liquid. Indust. Crops Prod. 94, 266–280. doi: 10.1016/j.indcrop.2016.08.048
Cheng, Z. Y., Song, H., Yang, Y., Liu, Y. J., Liu, Y., Hu, Z. G., et al. (2015). Optimization of microwave-assisted enzymatic extraction of polysaccharides from the fruit of Schisandra chinensis Baill. Int. J. Biol. Macromol. 76, 161–168. doi: 10.1016/j.ijbiomac.2015.01.048
Coklar, H., and Akbulut, M. (2017). Anthocyanins and phenolic compounds of Mahonia aquifolium berries and their contributions to antioxidant activity. J. Funct. Foods. 35, 166–174. doi: 10.1016/j.jff.2017.05.037
Deng, K., Ouyang, J., Hu, N., and Wang, H. L. (2021). Effect of structural difference on stability and cellular antioxidative activity of anthocyanins from Lycium ruthenicum Murr. Natl. Prod. Res. Dev. 34, 034–002. doi: 10.16333/j.1001-6880.2022.2.005
Duan, X. W., Su, X. G., Shi, J., You, Y. L., Zhao, M. M., Li, Y. B., et al. (2008). Inhibitory effect of anthocyanin extract from the seed coat of black bean on pericarp browning and lipid peroxidation of litchi fruit during storage. J. Food Biochem. 32, 415–430. doi: 10.1111/j.1745-4514.2008.00158.x
Farooq, S., Shah, M. A., Siddiqui, M. W., Dar, B. N., Mir, S. A., Ali, A., et al. (2020). Recent trends in extraction techniques of anthocyanins from plant materials. J. Food Meas. Characteriz. 14, 3508–3519. doi: 10.1007/s11694-020-00598-8
Fei, T. Y., Fei, J., Huang, F., Xie, T. P., Xu, J. F., Zhou, Y., et al. (2017). The anti-aging and anti-oxidation effects of tea water extract in Caenorhabditis elegans. Exp. Gerontol. 97, 86–96. doi: 10.1016/j.exger.2017.07.015
Feng, D., He, R., Jiang, Y. J., Wang, Y. J., and Yan, W. J. (2021). Research progress on the anti-aging function of Cistanches. J. Food Safety Qual. 12, 4429–4437. doi: 10.19812/j.cnki.jfsq11-5956/ts.2021.11.018
Gulcin, I. (2020). Antioxidants, and antioxidant methods: an updated overview. Arch. Toxicol. 94, 651–715. doi: 10.1007/s00204-020-02689-3
Guli, D. N., Jia, Q. Z., Tao, D. Y., and Chen, Y. (2009). Effect of blackberry pigment on hypoxia tolerance and swimming endurance at ambient pressure in mice. Lishizhen Med. Materia Med. Res. 20, 2682–2683.
Hang, Y. Y., Chen, H., Ye, Y., Qin, Y. T., Liu, H. C., Ma, W. T., et al. (2016). Study on extraction and purification of anthocyanin from Lycium ruthenicum Murr. J. Food Safety Qual. 7, 3372–3739. doi: 10.19812/j.cnki.jfsq11-5956/ts.2016.09.051
Hang, Y. Y., Li, Y., Shu, T., Chen, H., Cao, X. H., Wang, X., and Wang, S. L. (2018). Extraction and antioxidative ability of Lycium ruthenicum Murr anthocyanins. Food Sci. Technol. 43, 237–242. doi: 10.13684/j.cnki.spkj.2018.05.043
Harman, D. (2009). About “Origin and evolution of the free radical theory of aging: a brief personal history, 1954-2009”. Biogerontology. 10, 783. doi: 10.1007/s10522-009-9253-z
He, P., Kong, D. S., Wang, L., and Liu, R. M. (2020). Optimizing the extraction technology of anthocyanin from fruit of lycium ruthenicum by orthogonal design. Chin. Wild Plant Resour. 39, 33–37. doi: 10.3969/j.issn.1006-9690.2020.02.006
Hu, A., Hao, S., Zheng, J., Chen, L., and Sun, P. (2021). Multi-frequency ultrasonic extraction of anthocyanins from blueberry pomace and evaluation of its antioxidant activity. J. AOAC Int. 104, 811–817. doi: 10.1093/jaoacint/qsaa150
Huang, X. H., Feng, L. X., Li, J. H., Zhang, H. Y., Zang, Q. M., Zhang, Y. W., et al. (2022). Study on antioxidant activity from different medicinal parts of Picrasma quassioides. Feed Res. 45, 67–70. doi: 10.13557/j.cnki.issn1002-2813.2022.15.015
Jin, W. B., Zhou, T., and Li, G. K. (2019). Recent advances of modern sample preparation techniques for traditional Chinese medicines. Chromatography A. 1606, 460377. doi: 10.1016/j.chroma.2019.460377
Kim, J., Pälijärvi, M., Karonen, M., and Salminen, J. (2020). Distribution of enzymatic and alkaline oxidative activities of phenolic compounds in plants. Phytochemistry. 179, 112501. doi: 10.1016/j.phytochem.2020.112501
Kucukler, S., Benzer, F., Yildirim, S., Gur, C., Kandemir, F. M., Bengu, A. S., et al. (2021). Protective effects of chrysin against oxidative stress and inflammation induced by Lead Acetate in Rat Kidneys: a biochemical and histopathological approach. Biol. Trace Element Res. 199, 1501–1514. doi: 10.1007/s12011-020-02268-8
Kwansang, J., Chen, C. J., and Chaiprateep, E. (2022). Optimization of water-based ultrasonic-microwave assisted extraction (UMAE) of bioactive compounds from Garcinia mangostana pericarp. J. Compl. Integr. Med. 19, 219–225. doi: 10.1515/jcim-2021-0454
Li, D. T., Wang, P. P., Luo, Y. H., Zhao, M. Y., and Chen, F. (2017). Health benefits of anthocyanins and molecular mechanisms: Update from the recent decade. Crit. Rev. Food Sci. Nutr. 57, 1729–1741. doi: 10.1080/10408398.2015.1030064
Li, J., Li, S. Z., Feng, W. J., and Yuan, H. (2010). In vitro antioxidant and free radical scavenging activities of total flavonoids from the leaves of Lycium ruthenicum Murr. Food Sci. 31, 259–262. doi: 10.7506/spkx1002-6630-201013059
Li, X., Zhu, F. Y., and Zeng, Z. W. (2021). Effects of different extraction methods on antioxidant properties of blueberry anthocyanins. Open Chem. 19, 138–148. doi: 10.1515/chem-2020-0052
Lim, Y. J., Kwon, S., Qu, S. S., Kim, D., and Eom, S. H. (2021). Antioxidant contributors in seed, seed coat, and cotyledon of γ-ray-induced soybean mutant lines with different seed coat colors. Antioxidants. 10, 353. doi: 10.3390/antiox10030353
Lin, S. X., Meng, X. J., Tan, C., Tong, Y. Q., Wan, M., Wang, M., et al. (2022). Composition and antioxidant activity of anthocyanins from Aronia melanocarpa extracted using an ultrasonic-microwave-assisted natural deep eutectic solvent extraction method. Ultras. Sonochem. 89, 106102. doi: 10.1016/j.ultsonch.2022.106102
LioChev, S. I. (2013). Reactive oxygen species and the free radical theory of aging. Free Rad. Biol. Med. 60, 1–4. doi: 10.1016/j.freeradbiomed.2013.02.011
Liu, S. J., Luo, Q. Q., Pan, Y. T., Liu, Z. Y., Su, Y. C., Lin, J. F., et al. (2017). Studies on antioxidative activities in vitro of the extracts by different EtOH precipitation from Perna viridis. Food Ferment. Indust. 43, 187–192. doi: 10.13995/j.cnki.11-1802/ts.201706031
Lushchak, V. I. (2014). Free radicals, reactive oxygen species, oxidative stress, and its classification. Chemico-Biol. Inter. 224, 164–175. doi: 10.1016/j.cbi.2014.10.016
Maran, J. P., Mekala, V., and Manikandan, S. (2013). Modeling, and optimization of ultrasound-assisted extraction of polysaccharide from Cucurbita moschata. Carbohyd. Polym. 92, 2018–2026. doi: 10.1016/j.carbpol.2012.11.086
Marana, J. P., Sivakumara, V., Thirugnanasambandhama, K., and Sridharb, R. (2013). Optimization of microwave assisted extraction of pectin from orange peel. Carbohyd. Polym. 97, 703–709. doi: 10.1016/j.carbpol.2013.05.052
Marana, J. P., Sivakumara, V., Thirugnanasambandhama, K., and Sridharb, R. (2014). Microwave assisted extraction of pectin from waste Citrullus lanatus fruit rinds. Carbohyd. Polym. 101, 786–791. doi: 10.1016/j.carbpol.2013.09.062
Mi, X, Liu, S. X., Zeng, Q., and Xu, D. (2019). Optimization of ultrasound-assisted extraction of carotenoids from persimmon peel by response surface methodology and its antioxidant activity in vitro. China Condiment. 44, 26–31. doi: 10.3969/j.issn.1000-9973.2019.11.006
Poljsak, B., Šuput, D., and Milisav, I. (2013). Achieving the balance between ros and antioxidants: when to use the synthetic antioxidants. Oxid. Med. Cell. Long. 2013, 956792. doi: 10.1155/2013/956792
Pomatto, L. C., and Davies, K. J. (2018). Adaptive homeostasis and the free radical theory of ageing. Free Rad. Biol. Med. 124, 420–430. doi: 10.1016/j.freeradbiomed.2018.06.016
Shields, H. J., Traa, A., and Raamsdonk, J. M. (2021). Beneficial and detrimental effects of reactive oxygen species on lifespan: a comprehensive review of comparative and experimental studies. Front. Cell Dev. Biol. 9, 628157. doi: 10.3389/fcell.2021.628157
Song, Y., Du, B. J., Zhou, T., Han, B., Yu, F., Yang, R., et al. (2011). Optimization of extraction process by response surface methodology and preliminary structural analysis of polysaccharides from defatted peanut (Arachis hypogaea) cakes. Carbohyd. Res. 346, 305–310. doi: 10.1016/j.carres.2010.11.019
Tan, J. Q., Han, Y. M., Han, B., Qi, X. M., Cai, X., Ge, S. Q., et al. (2022). Extraction and purification of anthocyanins: a review. J. Agric. Food Res. 8, 100306. doi: 10.1016/j.jafr.2022.100306
Tonon, R., Brabet, C., and Hubinger, M. D. (2010). Anthocyanin stability and antioxidant activity of spray-dried açai (Euterpe oleracea Mart.) juice produced with different carrier agents. Food Res. Int. 43, 907–914. doi: 10.1016/j.foodres.2009.12.013
Viña, J., Borras, C., Abdelaziz, K. M., Garcia-Valles, R., and Gomez-Cabrera, M. C. (2013). The free radical theory of aging revisited: the cell signaling disruption theory of aging. Antioxid. Redox Signal. 19, 779–787. doi: 10.1089/ars.2012.5111
Viña, J., Borras, C., and Gomez-Cabrera, M. C. (2018). A free radical theory of frailty. Free Radical Biol. Med. (2018) 124, 358–363.doi: 10.1016/j.freeradbiomed.2018.06.028
Wang, L., Ye, W. J., and Wu, H. J. (2020). Protective Effect of Lycium barbarum polysaccharide on hepatic injury by acrylamide in rats. Sci. Technol. Food Industry. 41, 1–7. doi: 10.13386/j.issn1002-0306.2020.14.046
Wang, M. W., Zhang, Z. Y., Sun, H. J., He, S. D., Liu, S. Y., Zhang, T., et al. (2022). Research progress of anthocyanin prebiotic activity: a review. Phytomedicine. 102, 154145. doi: 10.1016/j.phymed.2022.154145
Wang, S. Y., and Jiao, H. (2000). Scavenging capacity of berry crops on superoxide radicals, hydrogen peroxide, hydroxyl radicals, and singlet oxygen. Agric. Food Chem. 48, 5677–5684. doi: 10.1021/jf000766i
Wang, Y., Deng, H., Huang, D. C., Zeng, X. H., Zhang, X., and He, Q. P. (2022). Effect of drying methods on yield and antioxidant activity of fat-soluble antioxidant components from Rosmarinus officinalis. China Oils Fats. 47, 87–91. doi: 10.19902/j.cnki.zgyz.1003-7969.210379
Wang, Y. W., Ye, Y., Wang, L., Yin, W., and Liang, J. (2021). Antioxidant activity and subcritical water extraction of anthocyanin from raspberry process optimization by response surface methodology. Food Biosci. 44, 101394. doi: 10.1016/j.fbio.2021.101394
Xia, N., and Zhao, L. F. (2014). Study on the antioxidant activity and protective effect on mitochondria of Lycium ruthenicum functional ingredient. Sci. Technol. Food Industry. 35, 162–166.+175. doi: 10.13386/j.issn1002-0306.2014.22.027
Xu, Y. Q., Zhang, L., Yang, Y., Song, X. M., and Yu, Z. Y. (2015). Optimization of ultrasound-assisted compound enzymatic extraction and characterization of polysaccharides from blackcurrant. Carbohyd. Polym. 117, 895–902. doi: 10.1016/j.carbpol.2014.10.032
Yan, M. M., Liu, W., Fu, Y. J., Zu, Y. G., Chen, C. Y., Luo, M., et al. (2010). Optimisation of the microwave-assisted extraction process for four main astragalosides in Radix Astragali. Food Chem. 119, 1663–1670. doi: 10.1016/j.foodchem.2009.09.021
Zhang, B., Yang, R. Y., and Liu, C. Z. (2008). Microwave-assisted extraction of chlorogenic acid from flower buds of Lonicera japonica Thunb. Separ. Purif. Technol. 62, 480–483. doi: 10.1016/j.seppur.2008.02.013
Zhang, Y., Zheng, B. D., Tian, Y. T., and Huang, S. Y. (2012). Microwave-assisted extraction and anti-oxidation activity of polyphenols from lotus (Nelumbo nucifera Gaertn.) seeds. Food Sci. Biotechnol. 21, 1577–1584. doi: 10.1007/s10068-012-0210-2
Zhong, W. T., Yang, C. M., Zhang, Y. Z., Liu, Y. M., and Yang, D. S. (2022). The chemical profiling and anticancer potential of functional polysaccharides from flos sophorae immaturus. Molecules. 27, 5978. doi: 10.3390/molecules27185978
Keywords: LRM, anthocyanin extraction, response surface optimization, ultrasonic microwave synergistic extraction, antioxidant activity
Citation: Dong Y, Zhong W, Yang C, Zhang Y and Yang D (2022) Study on anthocyanins from Lycium ruthenicum Murr via ultrasonic microwave synergistic extraction and its antioxidant properties. Front. Sustain. Food Syst. 6:1052499. doi: 10.3389/fsufs.2022.1052499
Received: 24 September 2022; Accepted: 18 October 2022;
Published: 04 November 2022.
Edited by:
Philippa Chinyere Ojimelukwe, Michael Okpara University of Agriculture, NigeriaReviewed by:
Selin Sahin Sevgili, Istanbul University, TurkeyAhmad Cheikhyoussef, University of Namibia, Namibia
Copyright © 2022 Dong, Zhong, Yang, Zhang and Yang. This is an open-access article distributed under the terms of the Creative Commons Attribution License (CC BY). The use, distribution or reproduction in other forums is permitted, provided the original author(s) and the copyright owner(s) are credited and that the original publication in this journal is cited, in accordance with accepted academic practice. No use, distribution or reproduction is permitted which does not comply with these terms.
*Correspondence: Dongsheng Yang, eWRzQHpjc3QuZWR1LmNu
†These authors have contributed equally to this work