- 1Institute of Environmental Sciences and Engineering, School of Civil and Environmental Engineering, National University of Sciences and Technology (NUST), Islamabad, Pakistan
- 2State Key Laboratory of Bioreactor Engineering, East China University of Science and Technology, Shanghai, China
- 3School of Food and Biological Engineering, Jiangsu University, Zhenjiang, China
- 4Technical University of Munich Campus Straubing for Biotechnology and Sustainability, Straubing, Germany
- 5German Institute of Food Technologies (DIL e.V.), Quakenbrück, Germany
- 6Food Microbiology and Biotechnology Laboratory, Food and Nutritional Sciences Program, North Carolina A&T State University, Greensboro, NC, United States
- 7School of Biochemistry and Biotechnology, University of the Punjab, Lahore, Pakistan
- 8Department of Food Science, Faculty of Agriculture, Zagazig University, Zagazig, Egypt
- 9School of Food Science and Engineering, South China University of Technology, Guangzhou, China
The extensive use of antibiotics and vaccines against microbial infections can result in long-term negative effects on humans and the environment. However, there are a number of plants that have antimicrobial effects against various disease-causing microbes such as bacteria, viruses, and fungi without negative side effects or harm to the environment. In this regard, four particular plants- Capsicum, Nigella sativa, Musa paradisiaca L., and Citrus limetta have been widely considered due to their excellent antimicrobial effect and ample availability. In this review, we discuss their antimicrobial effects due to the presence of thymoquinone, p-cymene, pinene, alkaloids, limonene, camphene, and melanin. These antimicrobial compounds disrupt the cell membrane of microbes, inhibit cellular division, and form biofilm in bacterial species, eventually reducing the number of microbes. Extraction of these compounds from the respective plants is carried out by different methods such as soxhlet, hydro-distillation, liquid-liquid extraction (LLE), pressurized liquid extraction (PLE), solid-phase extraction (SPE), supercritical fluid extraction (SFE), pulsed electric field (PEF), microwave-assisted extraction (MAE), enzyme-assisted extraction (EAE), ultrasound-assisted extraction (UAE), and high-voltage electrical discharge. Suitable selection of the extraction technique highly depends upon the associated advantages and disadvantages. In order to aid future study in this field, this review paper summarizes the advantages and disadvantages of each of these approaches. Additionally, the discussion covers how antimicrobial agents destroy harmful bacteria. Thus, this review offers in-depth knowledge to researchers on the antibacterial properties of Capsicum, Nigella sativa, Musa paradisiaca L. peels, and Citrus limetta.
Introduction
A global public health crisis has been caused by the rise in resistant bacteria over the past few decades (Dhingra et al., 2020; Hu et al., 2020; Ibrahim et al., 2021). Although antibiotics are used to treat illnesses by killing bacteria or limiting their growth, they can also have several negative effects (Gyawali and Ibrahim, 2014). For example, because some disease-causing bacteria have acquired resistance to particular antibiotics as a result of their widespread and sustained usage, these treatments are no longer as effective (Namita and Mukesh, 2012). In this regard, antimicrobial agents can eliminate resistant pathogenic microbes without imposing any known side effects. Antimicrobial agents disrupt the cellular structure of microbes, thus inhibiting various infectious diseases (Peterson, 2008) and are used to treat humans with diseases that are primarily due to non-human sources (Organization, 2019). According to WHO (world health organization), the overall consumption of antimicrobial agents is in between 4.4 and 64.4 Defined Daily Doses (DDD) per 1,000 persons per day. As per WHO data, the national estimates of antimicrobial consumption in 65 countries and areas: six from the Region of America, four from the African Region, three from the Eastern Mediterranean Region, 46 from the European Region: and six from the Western Pacific Region are presented in Figure 1 (Organization, 2018).
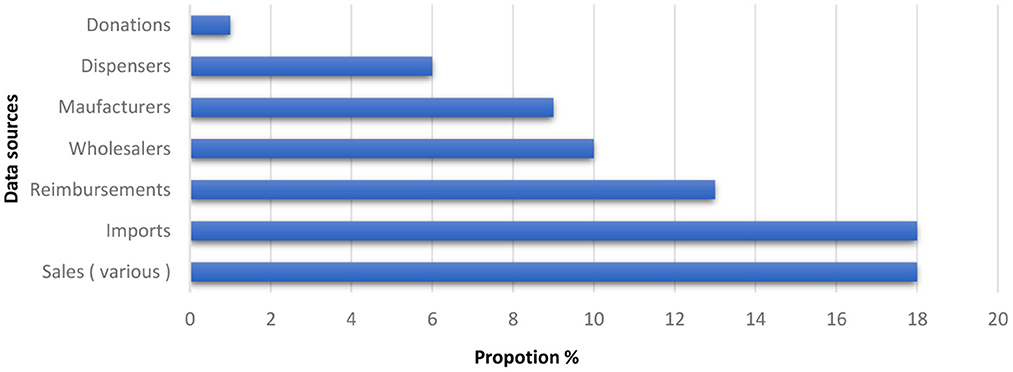
Figure 1. National estimates of antimicrobial consumption in 65 countries and areas (Organization, 2018).
According to WHO, residents of these low-income countries are more likely to die of communicable and infectious diseases such as malaria, tuberculosis, diarrhea, HIV/AIDS, etc. (Philip, 2017). Therefore, suitable preventive measures against these infectious and parasitic diseases should be adopted. These measures may include self-cleanliness, proper sanitation, safe food preparation and handling, and the use of antimicrobial agents to immunize the body against infectious and parasitic diseases (Mehta et al., 2014). Nature also plays its role in fighting against disease-causing microbes (Ibrahim et al., 2011; Bor et al., 2016). For example, many plants such as Nigella sativa, Capsicum, Musa paradisiaca L., and Citrus limetta have attracted tremendous public interest due to their efficient antimicrobial agents (Dutta et al., 2020b; El-Naggar et al., 2020; Ajijolakewu et al., 2021; Vanlalveni et al., 2021; Vijayakumar et al., 2021). Furthermore, whether in underdeveloped, developing, or developed regions of the world, these natural goods are widely accessible. Additionally, these items are cost-effective in their use because they don't need to be prepared using a time-consuming procedure in order to fight infectious bacteria (Ncube et al., 2008).
Nigella sativa, Capsicum, peels of Musa paradisiaca L., and Citrus limetta contain effective antimicrobial agents against various infectious microbes such as the following: pathogenic bacteria (gram-negative and gram-positive), including S. aureus, Candida albicans, E. coli, Gram-positive cocci, Microsporum canis, Trichophyton mentagrophytes, Trichophyton interdigitale, four species of Trichophyton rubrum, Staphylococcus epidermidis, Micrococcus luteus, Listeria monocytogene, Bacillus cereus, Vibrio parahaemolyticus, Pseudo. aeruginosa, Salmonella enteritidis, Sal. Typhimurium, and Shigella flexneri (Hanafy and Hatem, 1991; Hosseinzadeh et al., 2007; Hannan et al., 2008; Halawani, 2009; Chaieb et al., 2011; Alshareef, 2019). The microbes listed above are known to cause the following diseases: pneumonia, bloodstream infections, wound or surgical site infections, methicillin-resistant Staphylococcus aureus (MRSA), urinary tract infection, “traveler's diarrhea,” immunologic infections, diarrhea (sometimes bloody), fever, stomach cramps (Awaisheh and Ibrahim, 2009; Awaisheh et al., 2013; Blinov et al., 2022) and various others as reported in earlier conducted studies (Edelson and Unanue, 2000; Otto, 2009; Doernberg et al., 2017; Liu and Ji, 2018). These diseases can be prevented by using Nigella sativa, Capsicum, peels of Musa paradisiaca L., and Citrus limetta as antimicrobial agents. We have chosen to focus on these particular natural resources due to their global availability.
Worldwide, Capsicum grows on 1.93 million hectares of surface area. China is the world's largest producer of Capsicum at ~16 million tons annually. Mexico is the second largest producer at ~2.3 million tons, followed by Turkey and Indonesia with 2.2 and 1.8 million, respectively (Penella and Calatayud, 2018). Nigella sativa is produced mainly by India on 9,000 hectares. It is also produced in Pakistan, Sri Lanka, Nepal, Bangladesh, Egypt, and Iraq (Huchchannanavar et al., 2019). The annual global production of Musa paradisiaca L. is ~41.265 million tons per year with its primary producers being Brazil, China, Ecuador, the Philippines, and India (Preedy and Watson, 2019). Brazil is the largest Citrus limetta producing country globally with 15,912 tons followed by China with 5,450, the United States with 9,237 and the European Union (EU) with 5,999 thousand metric tons (Spreen, 2010). Annually, the global production of Musa paradisiaca L. is around 41.3 million tons and a large fraction of it is wasted as the peel. Notably, just in Brazil ~780 million tons of Musa paradisiaca L. -waste is produced annually (Martínez-Ruano et al., 2018). According to Prabhakar and Singh (2019), ~57% of processed Citrus limetta contributes to peel waste Usage of the endocarp of Musa paradisiaca L. and Citrus limetta creates solid waste problems due to the production of their peels, whereas Nigella sativa and Capsicum do not create such a problem. In this review, we address the handling of the huge solid waste problem produced by Musa paradisiaca L. and Citrus limetta by utilizing their peels as an antimicrobial agent against infectious diseases.
At present globally, a gradual increase in the wide spread of infectious diseases is quite evident. Therefore, it is need of time to adopt suitable preventive measures, such as regular use of antimicrobial agents. Therefore, it is proposed in this review paper that Capsicum, Nigella sativa, Musa paradisiaca L., and Citrus limetta on account of their abundant availability and antimicrobial constituents should be regarded as potential raw materials for the economical commercial production of various antimicrobial agents (Parthiban et al., 2011; Somda et al., 2011; Chitranshi et al., 2020). These natural materials are non-toxic, produce only organic food waste, and are easily available and completely safe for producing antimicrobial agents. Furthermore, the current study also discusses the antimicrobial compounds present in Capsicum, Nigella sativa, peels of Musa paradisiaca L., and Citrus limetta along with the methods employed for their extraction. Moreover, we also explain the overall antimicrobial mechanism of antimicrobial agents present in Capsicum, Nigella sativa, Musa paradisiaca L., and Citrus limetta. A schematic illustration of the content in this review is presented in Figure 2.
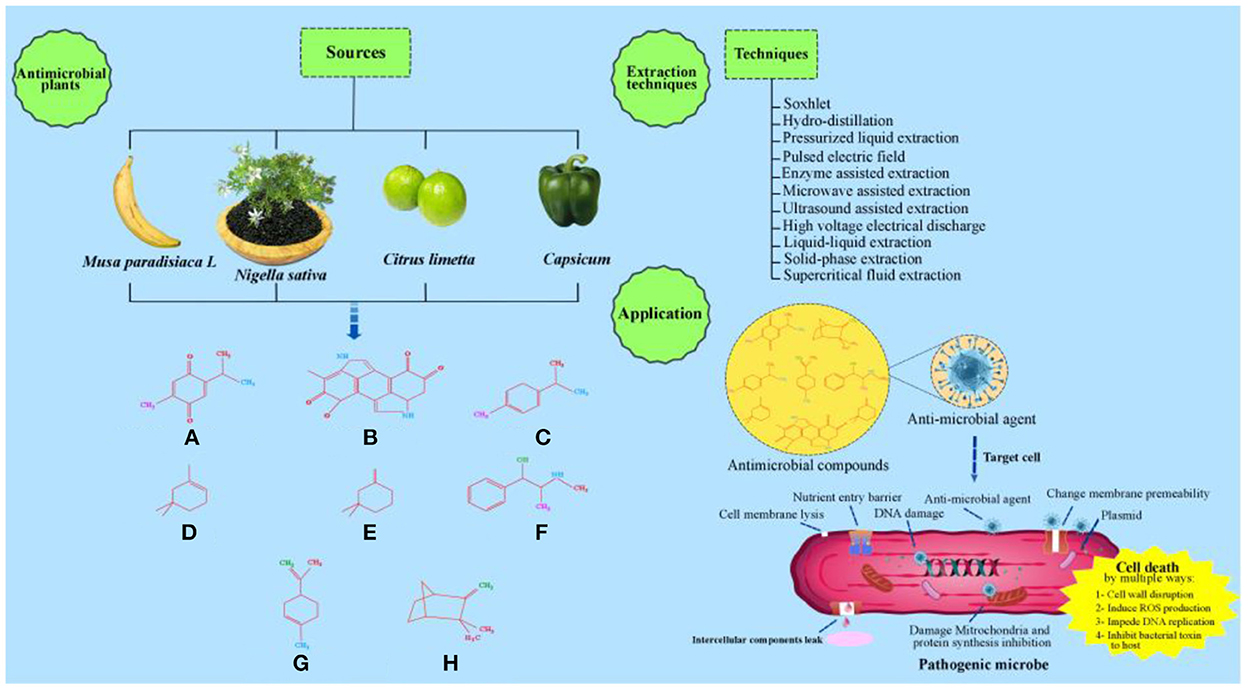
Figure 2. Schematic illustration of antimicrobial effects of Capsicum, Nigella sativa, Musa paradisiaca L and Citrus limetta. (A) Thymoquinone. (B) Melanin. (C) P-cymene. (D) a-Pinene. (E) b-Pinene. (F) Alkaloid. (G) Limonene. (H) Camphene.
Biological properties of plant
The history of plants and/or plant components' utilization to flavor and conserve food, and to prevent oxidation and treat health problems is dated back to thousands of years ago. Most interestingly, the knowledge of their biological properties has been transmitted over the centuries among human societies. Considering this fact, it has been stated that the phytochemicals are the valuable source of bioactive substances with significant antimicrobial and antioxidant properties (Silva and Fernandes, 2010; Radha and Laxmipriya, 2015).
Anti-microbial agents present in plants
It has long been believed that plants have therapeutic properties. Since prehistory, people have used hundreds of thousands of local plants in infusions and poultices to treat various ailments. The desire to find novel antimicrobials has been prompted by the rise of microbial strains that are resistant to antibiotics and the recurrence of newer, deadlier illnesses. To find possible pharmacological leads, the whole natural resource base is being quickly evaluated (Ref: Antimicrobial Agents from Plants Reshma Reghu, Pramod Sahadevan & Shiburaj Sugathan + Plant Products as Antimicrobial Agents Marjorie Murphy Cowan*). Many plants and/or plant components, including Nigella sativa, Capsicum, peels of Musa Paradisiaca L., and Citrus limetta are ascribed to the presence of anti-microbial agents, which will be discussed herein.
Antimicrobial agent in Nigella sativa
It contains more than 100 valuable elements. According to science N. sativa contains 20–85% of protein, 38.20% of fat, 7–94% of fiber, and 31.94% of total carbohydrates that have antimicrobial effects. Due to the presence of thymoquinone and thymohydroquinone in N. sativa it can fight against infectious disease-causing microbes (Bakal et al., 2017). The amount of the most significant bioactive component, thymoquinone, found in the volatile oil obtained from the seeds of N. sativa using various extraction techniques can range from 1.06 to 8.8 mg/g of oil, respectively (Yimer et al., 2019).
Antimicrobial agent in Capsicum
Capsaicin, also known as 8-methyl-N-vanillyl-6-nonenamide, is a compound found in Capsicum plants (chili peppers), which are used for food and medicine. Due to its antibacterial and anti-virulence properties, as well as its multiple pharmacological and physiological benefits (pain alleviation, cancer prevention, and advantageous cardiovascular, and gastrointestinal benefits; Marini et al., 2015), capsaicin has recently attracted a lot of attention. The capsaicinoids (2.5 mg/g; Othman et al., 2011) found in capsicum, which include more than 20 alkaloids (Bakht et al., 2020), are of major medical significance worldwide. Since capsicum has potent antibacterial effects, it is beneficial to utilize it in common food items.
Antimicrobial agent in Musa paradisiaca L.
Ethanol (0.5 g/100 g; Gorgus et al., 2016), which has a microbe-killing effect, is present in Musa paradisiaca L. at a concentration of 0.5 g/100 g. Secondary metabolites such as flavonoids, tannins, phlobatannins, alkaloids, glycosides, and terpenoids (Imam and Akter, 2011) have been found to be present in banana peel. Ighodaro (2012) evaluated the antibacterial effectiveness of banana peel extract against human pathogenic microorganisms and exhibited good inhibitory activity against S. aureus, Escherichia coli, and Proteus mirabilis. Although banana peel is regarded as waste, peel extracts can be utilized to create antibiotics (Saleem and Saeed, 2020).
Antimicrobial agent in Citrus limetta peel
Peel from Citrus limetta can be utilized to make solid waste management more efficient as well as to create long-lasting antibacterial products. Citrus peel has been discovered to have thousands more phenolic compounds than citrus pulp (Dutta et al., 2020a), and these phenolic compounds (444.55–502.54 mg/L; Buyukkurt et al., 2019) have antimicrobial properties. In a 2009 study, Kekuda et al. investigated the antifungal efficacy of the peels of three distinct citrus fruits, C. limetta, C. sinensis, and C. limon, against Aspergillus species, and found that C. limetta was best at preventing the growth of the fungus that was tested (Shabnam et al., 2013).
Plants and/or plant components derived antimicrobial compounds
Thymoquinone, melanin, P-cymene, a-pinene, b-pinene, alkaloid, limonene, and camphene are significantly present in Nigella sativa, Capsicum, Musa Paradisiaca L., and Citrus limetta. Moreover, these plant-derived chemicals are known to act as antimicrobial agents with numerous applications in the medical and food industries, which will be discussed in the subsequent section.
Thymoquinone
Thymoquinone is a compound that has remarkable anti-sepsis activity at a specific dose. Thymoquinone is the main component present in Nigella sativa that is found to be effective against the Avian influenza virus and coronavirus (Ulasli et al., 2014). Another study found that Nigella sativa consumption makes it difficult for the coronavirus to survive and replicate in the body (Ahmad et al., 2020). Thymoquinone is reported as an inhibitor for the treatment of coronavirus infections by blocking the entry of virus into the cell (Xu et al., 2021). Studies reported that thymoquinone can serve as an anticancer, anti-inflammatory, antioxidant and as an analgesic compound. Activator of transcription (JAK-STAT), janus kinase/signal transduction, mitogen-activated protein kinase (MAPK), and nuclear factor kappa beta (NF-κβ) signaling pathways can be inhibited by thymoquinone. Thymoquinone causes disruption in reactive oxygen species (ROS), pro-inflammatory cytokines, lipooxygenase (LOX) enzymes, cyclooxygenase (COX), myeloperoxidase, and elastase (Ali A. et al., 2021).
Melanin
Melanin is an efficient green agent that possesses anti-inflammatory, antioxidant, and antimicrobial activity with vast application in the biomedical field (Silvestri et al., 2017; Avossa et al., 2021). Antioxidants protect human bodies from free radicals, which may otherwise result in causing various diseases such as cancer and coronary heart disease (Hamid et al., 2010). Additionally, it is promising as an antibacterial agent against both gram-positive and gram-negative pathogens (Liu et al., 2014).
P-cymene
P-cymene is a natural compound found in many plants. It is used as an important agent in many drugs. It is widely present in more than 200 types of foods, including grape fruit, orange juice, mandarin, raspberries, carrots, butter, oregano, nutmeg, and almost every spice. P-cymene can serve as an analgesic, anti-inflammatory, antioxidant, and anti-tumor agent (Nickavar et al., 2014). P-cymene has anti-nociceptive, anticancer, antidiabetic, antiviral, antiparasitic, anti-inflammatory, antifungal, antioxidant, and antibacterial effects (Balahbib et al., 2021).
Pinene
Pinene is the natural compound found in citrus fruits. Pinene has various potential benefits such as it has anti-inflammatory, antimicrobial, antioxidant, and neuroprotective effects (Di Rauso Simeone et al., 2020). Pinene has anticoagulant, anti-inflammatory, anti-leishmania, antimalarial, antimicrobial, antioxidant, antitumor, analgesic, and antibiotic resistance modulation effects (Park et al., 2021). Pinene has the wound healing process by generating scars with effective tensile strength, accelerating wound closure, acting as an adhesive of primary intention, and contributing to collagen deposition (Salas-Oropeza et al., 2021).
Alkaloid
Alkaloid is a natural compound found in many plants. The presence of alkaloids in plants protects them from the destruction of various insects (Zhang et al., 2005). Indole alkaloids and isoquinoline alkaloids are the primary classes of substances having antibacterial action among the numerous alkaloids, including pyridine alkaloids, indole alkaloids, steroidal alkaloids, and other alkaloids. Alkaloids have antiviral, antibacterial, antifungal, cytotoxic, antioxidant, antifouling activities and anti-infective potential (Barghout et al., 2020; Diaz et al., 2020; Youssef et al., 2021).
Limonene
Limonene is a chemical found mostly in citrus fruits (Rodríguez et al., 2011). Limonene has several health benefits and belongs to the group of terpenes. These chemicals have strong aromas which protect plants from predators. Limonene also has anti-inflammatory and antioxidant effects (Miguel, 2010). Limonene can be used as a greener and safer alternative to market-available antimicrobial agents (Ibáñez et al., 2020).
Camphene
Camphene is a monoterpene found in many plants (Russo and Marcu, 2017). Terpenes are those chemicals that have strong aromas which protect plants from predators (Miguel, 2010). Camphene can fight against various deadly pathogens, such as Enterococcus spp. and S. aureus, methicillin-resistant Staphylococcus aureus (MRSA) and have an antifungal effect against Trichophyton mentagrophytes and several species of Paracoccidioides spp., Camphene have activity against Mycobacterium tuberculosis and vancomycin resistance Enterococcus spp. Isolates (de Freitas et al., 2020). Figure 3 shows the chemical structures of thymoquinone, melanin, P-cymene, a-pinene, b-pinene, alkaloid, limonene, and camphene.
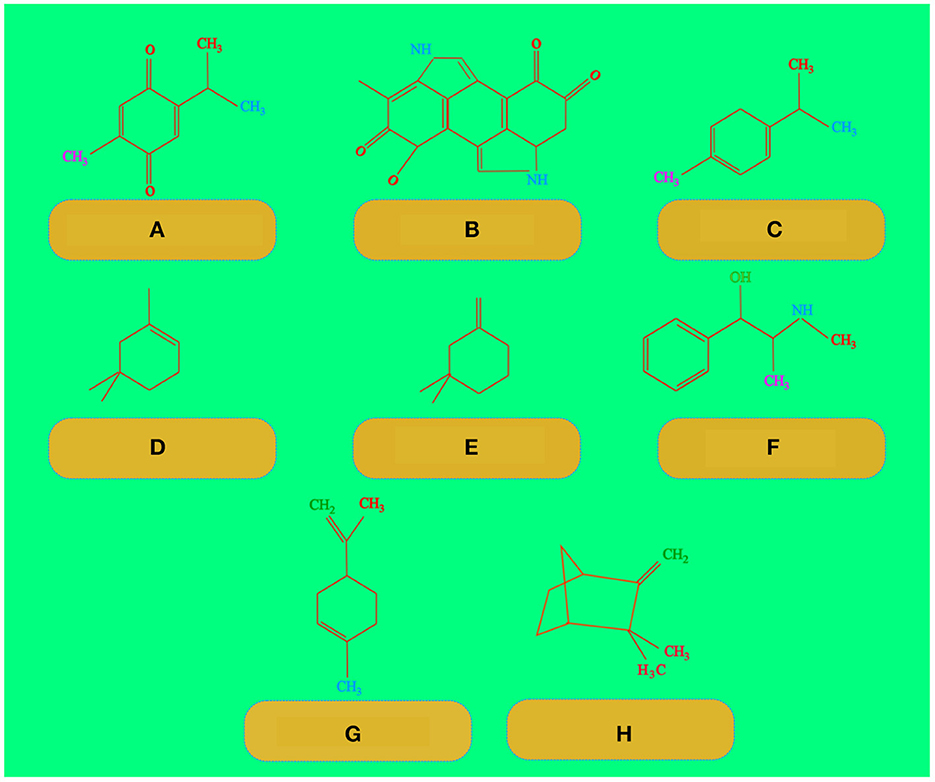
Figure 3. Chemical structures of (A) thymoquinone, (B) melanin, (C) P-cymene, (D) a-pinene, (E) b-pinene, (F) alkaloid, (G) limonene, and (H) camphene.
Table 1 shows the major antimicrobial compounds present in Nigella sativa and Capsicum along with the adopted methodology and important findings reported in previous research studies. Whereas, Table 2 shows the major antimicrobial compounds present in Musa paradisiaca L. and Citrus limetta along with the adopted methodology and important findings reported in previous research studies.
Extraction of antimicrobial compounds
The initial three basic processes in the extraction of antimicrobial compounds reported in the literature are (1) pretreatment, (2) extraction, and (3) purification.
Pretreatment
The first step in the extraction of antimicrobial agents is the preparation of materials employing methods such as washing, grinding, dewatering, heating and microfiltration. Pre-treatment consists of three additional steps: physical treatment, chemical treatment, and biological treatment.
Physical treatment
Physical treatment involves pre-washing, drying, grinding to get a homogeneous sample, heating, steaming, freeze-drying, and often boosting the kinetics of analytical extraction as well as increasing the contact of the sample surface with the solvent system. When making the extract from plant samples, proper precautions must be taken to ensure that any potential active ingredients are not lost, altered, or destroyed (Fabricant and Farnsworth, 2001; Cos et al., 2006; Sasidharan et al., 2011).
Chemical treatment
Chemical treatment involves the use of salts, acids, bases, and solvents. The chemical extraction method relies on the kinds of functional groups each component in the mixture possesses. Chemical processes can separate or purify materials when the right reagents are used. There are several chemical tests that can be used to identify the presence of alkaloids, flavonoids, tannins, saponins, flavones, sterols, and terpenes, which have been briefly discussed in the later part.
Identification of alkaloids
The extracts are individually filtered, dissolved in diluted hydrochloric acid, and analyzed for the presence of alkaloids. Typically, Mayers and Wagner's tests are used to identify alkaloids. In Mayer's test, the appearance of a yellow color on using Mayer reagent indicates the presence of alkaloids. Whereas, in Wagner's test a brown-reddish brown is developed upon using Wagner's reagent that indicates the presence of alkaloids (Trease and Evans, 1989; Wallis, 1989; Pandey and Tripathi, 2014; Beena et al., 2016; Dhawan and Gupta, 2017; Nortjie et al., 2022).
Identification of flavonoids
For the identification of flavonoids lead acetate and sulphuric acid test are usually performed. In Lead acetate test; the extracts are mixed with a few drops of a lead acetate solution. The presence of flavonoids is indicated by a precipitate that is yellow in color. In the sulfuric acid test; after a couple of drops of sulfuric acid are added to the extracts, an orange color appears, indicating the presence of flavonoids (Trease and Evans, 1989; Wallis, 1989; Pandey and Tripathi, 2014; Beena et al., 2016; Dhawan and Gupta, 2017; Nortjie et al., 2022).
Identification of steroids
Likewise, on adding a few drops of acetic anhydride to the extracts causes samples to turn violet then blue and lastly to green, which is a sign of the presence of steroids (Trease and Evans, 1989; Wallis, 1989; Pandey and Tripathi, 2014; Beena et al., 2016; Dhawan and Gupta, 2017; Nortjie et al., 2022).
Identification of terpenoids
The identification of terpenoids is usually done by Salkowski's test. This test is conducted by adding 3 mL of concentrated sulfuric acid to an extract of 5 mg of the targeted plant component. Eventually, the presence of terpenoids is indicated by the appearance of reddish-brown color (Trease and Evans, 1989; Wallis, 1989; Pandey and Tripathi, 2014; Beena et al., 2016; Dhawan and Gupta, 2017; Nortjie et al., 2022).
Identification of anthraquinones
For the identification of anthraquinones, Bontrager's test is usually performed. The Bontrager's test involves boiling 5 mg of the extract in a water bath for a short period of time with 10% HCl. After filtering, it is allowed to cool followed by the addition of CHCl3 in an equal amount to the filtrate. The liquid is then heated before a few drops of 10% NH3 are added. Anthraquinones are present when a pink color forms, indicating their presence (Trease and Evans, 1989; Wallis, 1989; Pandey and Tripathi, 2014; Beena et al., 2016; Dhawan and Gupta, 2017; Nortjie et al., 2022).
Identification of phenols
For the identification of phenols, ferric chloride test and lead acetate test are usually performed. In the Ferric chloride test; 10 mL extract is mixed with a few drops of ferric chloride. The presence of phenol is indicated by a bluish-black color. In the Lead acetate test; 10 mg of extract is added with a few drops of lead acetate solution. The presence of phenol is indicated by a yellow color (Trease and Evans, 1989; Wallis, 1989; Al-Owaisi et al., 2014; Pandey and Tripathi, 2014; Beena et al., 2016; Dhawan and Gupta, 2017; Nortjie et al., 2022).
Biological treatment
Biological treatment uses bacteria and fungi to extract antimicrobial agents. The antibacterial activity of an extract or a pure chemical can be assessed or screened using a number of biological laboratory techniques. Disk-diffusion and broth or agar dilution procedures are the most well-known and fundamental techniques (Magaldi et al., 2004; Pfaller et al., 2004; Roden et al., 2005; Das et al., 2010; Bensah and Mensah, 2013; Kokollari et al., 2015; Fierascu et al., 2019).
Disk-diffusion method
The underlying idea behind this procedure is that an antibiotic concentration gradient is created when an antibiotic-impregnated disc is placed on agar that has already been inoculated with the test bacterium. The antibiotic is present in high concentrations near the disk's edge and gradually decreases as the space between them expands until the or3ganism is no longer inhibited by the antibiotic, at which time it is free to proliferate. If the antibiotic suppresses bacterial growth, a clear zone or ring forms around the antibiotic disc incubated overnight (Digrak et al., 1999; Parekh et al., 2005; Klančnik et al., 2010; Novaković and Janković, 2011).
Broth and agar dilution
Agar dilution implies adding various quantities of the antimicrobial drug to a nutrient-rich agar medium before applying a set number of cells to the agar plate's surface. In broth dilution, Bacteria are introduced into a liquid growth medium while being exposed to various concentrations of an antimicrobial agent, which is frequently assessed in 96-well microtiter plate format. After 16–20 h of incubation, growth is evaluated and the MIC value is recorded. This 3-day regimen is only applicable to aerobic bacteria (Griffin et al., 2000; Wiegand et al., 2008; Wu C. et al., 2015; Albano et al., 2020).
Extraction techniques
The second step is the extraction of required compounds from the material. The best extraction process must involve the thorough extraction of all the necessary metabolites or chemicals. If it's going to be done repeatedly, it needs to be quick, easy, and repeatable. The choice of an appropriate extraction technique mostly depends on the work that has to be done and on the target metabolites to be known. There are various extraction processes like; Conventional techniques include solvent extract, soxhlet extraction, vortexing, maceration, centrifugation, hydro-distillation, cold pressing, and pressing and solid-liquid dynamic extraction. Non-conventional techniques include; pressurized liquid extraction, microwave-assisted method, ultrasound-assisted method, pulsed electric field, enzyme-assisted extraction, and supercritical fluid extraction. Conventional techniques are more time-consuming and less environmental friendly while non-conventional are fast and environment friendly techniques (Koubala et al., 2008; Azmir et al., 2013a,b; Deng et al., 2015).
Extraction technologies for extracting antimicrobial agents from Nigella sativa, Capsicum, peels of Musa paradisiaca L., and Citrus limetta
This review presents an analysis of several extraction techniques to extract antimicrobial agents from different plant sources, especially Nigella sativa, Capsicum, peels of Musa paradisiaca, and Citrus limetta.
Soxhlet extraction
This extraction technique involves putting solid material inside the thimble present in the extractor. The solvent condenses and fills the thimble as the vapor rises. This process of extraction is repeated again and again until the desired quantity is achieved. This technique was firstly used for the determination of fat in milk (Voon et al., 2012; Parashar et al., 2014; Chen and Urban, 2015). Five types of soxhlet techniques are mainly used for solvent extraction i.e., conventional soxhlet, high-pressure soxhlet extraction, ultrasound-assisted soxhlet extraction, automated soxhlet extraction, and microwave-assisted soxhlet extraction (Redfern et al., 2014; Wu C. et al., 2015; Wu G. et al., 2015). Conventional soxhlet extraction is a continuous process with slow processing time and an environmental unfriendly technique (Azmir et al., 2013b; Lopresto et al., 2014). High-pressure soxhlet extraction is used to fractionate polyethylene. The automated soxhlet technique saves time, sample consumption and is used for agricultural, food and industrial samples. Ultrasound-assisted soxhlet extraction is mainly used to extract fat from sunflowers, grapes and soya beans. However, this technique does not affect the quality of extracted oil due to the adoption of mild conditions in the process (De Castro and Priego-Capote, 2010; Parashar et al., 2014).
The soxhlet technique was used to extract 6.77 ± 1.2, 3.66 ± 1.43, and 4.81 ± 1.8% w/w of thymoquinone from Nigella sativa (Kausar et al., 2017). Capsaicin was extracted from Capsicum by using this technique (Chuichulcherm et al., 2013). Citrus limetta which is a major phyto compound was extracted from Capsicum by using this technique (Baranitharan et al., 2020).
Hydro distillation
Hydro distillation is a conventional method that is used for extracting bioactive compounds from different plant species. This process involves packing plant materials in a still chamber, adding an appropriate amount of water, and then heating it to a boil. Alternative methods include injecting direct steam straight into the plant sample. By using indirect cooling with water, the water and oil vapor combination is condensed. Oil and bioactive substances are automatically separated from the water in a separator when the condensed mixture flows from the condenser. Its usage is constrained by restrictions on high-temperature applications for heat-sensitive phenolic compounds, although it has several benefits such as the lack of organic solvents in the process, the requirement for no dehydration of the plant materials, and faster extraction times (Vankar, 2004; Sa-Nguanpuag et al., 2011; Erkan et al., 2012; Chenni et al., 2016; Elyemni et al., 2019).
Through this technique, thymoquinone and capsaicin were extracted from Nigella sativa (El Khoury et al., 2019) and Capsicum, respectively (Jiang et al., 2013). Hydro distillation is the most common method for obtaining thymoquinone from Nigella sativa (Liu et al., 2011; Oskouei et al., 2018).
Liquid–liquid extraction
In liquid-liquid extraction separation of required material from liquid solution is made through contact with another insoluble liquid. The required material is separated based on its solubility in different liquids (Chen et al., 2017; El Blidi et al., 2019; Goyal and Singh, 2022).
It is best suited for liquid samples. The technology is simple and can be used at ambient temperature. However, the technology is time-consuming, requires expensive chemicals, and takes a lot of labor (Espinosa-Alonso et al., 2006).
Liquid-liquid extraction is an energy e?cient technique (Blahušiak et al., 2018) and can be used in biorefineries for lipid extraction from microalgae (Du et al., 2017) and for fractionation of pyrolytic bio-oils (Cesari et al., 2019). Earlier research study also reports lignin recovery via liquid extraction (Stiefel et al., 2017). Moreover, the fractionation of citrus essential oils (separating the terpenic hydrocarbons and oxygenated compounds) is achieved through liquid-liquid extraction (Gonçalves et al., 2016).
Solid-phase extraction
The solid-phase extraction technique is the simplest technique for extracting and pre-concentrating trace levels of contaminants from samples. The extraction efficiency of solid-phase extraction is enhanced by using nanomaterials as sorbents which provide more pollutants capturing sites (Jacobsen et al., 2004; Silva et al., 2016; Azzouz et al., 2018).
The only difference between solid-phase extraction and liquid-liquid extraction is the medium used for separation; in solid-phase extraction, one phase is a liquid phase and the other is a solid phase. The solid surface consists of adsorbent material that absorbs specific required material through the solution. The separation rate of solid-phase extraction technique is faster than liquid-liquid extraction technique. It is a simple technique. It can be employed for polar compounds. It is not suitable for volatile samples (Abd-Talib et al., 2014). It is an expensive technique than the liquid-liquid extraction technique (Hernanz et al., 2008). Different methods were used to extract thymoquinone from Nigella sativa including Solid-phase extraction (Kausar et al., 2017).
Supercritical fluid extraction
Suppose the temperature and pressure of a specific liquid and gas are above the critical point. In that case, the supercritical fluid extraction technique should be used for extracting certain required materials from samples like essential oils. Certain parameters such as temperature, pressure, the flow rate of fluids, and the sample size significantly affect extraction yield (Santoyo et al., 2006; Ma et al., 2018; Yousefi et al., 2019; Zizovic, 2020; Santos et al., 2021).
The supercritical fluid extraction technique saves time, it is sustainable and suitable for volatile samples. However, it is not suitable for drugs and polar substances. It is also a costly technique (Mendiola et al., 2007; Abbas et al., 2008).
From mandarin, orange, lemon, grapefruit peel and sour orange peel phenolic compounds were extracted by using supercritical fluid extraction (Omar et al., 2013; Trabelsi et al., 2016). Super critical fluid extraction is used for obtaining thymoquinone from N. sativa (Liu et al., 2011; Oskouei et al., 2018).
Pressurized liquid extraction
Pressurized liquid extraction is mainly used for extracting phenolic compounds from plants. Liquid solvents are used in it with specific temperatures and pressure. It is suitable for solid samples and polar compounds. However, pressurized liquid extraction is time-consuming technology that requires less solvent. Also due to the 10 g maximum sample weight limit, it is not appropriate for situations where very low-level analyte detection is required (Jacobsen et al., 2004; Juan et al., 2010; Mustafa and Turner, 2011; Alañón et al., 2015; Alvarez-Rivera et al., 2020). It is mainly used to recover bioactive compounds from grapes (Pereira et al., 2019). Extraction of phenolic compounds (alkaloids) from Citrus limetta through pressurized liquid extraction was carried out by Olabinjo et al. (2020).
Pulsed electric field
The pulsed electric field is an efficient non-thermal technique that involves the application of high-voltage pulses. These pulses cause rupturing of plant materials thus extraction of required components occurred from plant cells. Though it is a sustainable technology, it takes a long time to process. This technology requires process parameters like energy inputs, treatment temperature and field strength to be maintained (Liang et al., 2002; Wu et al., 2005; Nguyen and Mittal, 2007; Puértolas et al., 2012; Garner, 2019). According to a study, Citrus limetta peels were subjected to pulsed electric fields in order to extract phenolic components (Luengo et al., 2013). In another study, phenolic compounds were extracted from orange, grapefruit, and lemon peel through a pulse electric field (El Kantar et al., 2018). Much more components were extracted from the N. sativa's oil when PEF was applied as a pretreatment. The major constituent was thymoquinone and cymene, whereas D-Limonene and β-Pinene were also present in small quantities (El-Dakhakhny et al., 2000; Bakhshabadi et al., 2018).
Enzyme-assisted extraction
Specific enzymes are used in enzyme-assisted extraction to target the cell walls of plant materials, destroying them and liberating antimicrobial compounds. The effectiveness of the procedure as a whole can be improved by combining this strategy with a number of other techniques (Nadar et al., 2018; Bilal et al., 2019; Habeebullah et al., 2020). For instance, Naghshineh et al. (2013) found enzymatic extraction with high pressure treatment led to a higher level of pectin extraction form lime peel powder (Naghshineh et al., 2013). This technology has high extraction rates and is sustainable. However, it has a high enzyme cost and is not appropriate for industrial use. Its operations must be handled with caution (Puri et al., 2012; Singh et al., 2016). Camphene is the predominant essential oil of Aristolochia manshurientsis Kom extracted from enzyme-assisted extraction followed by hydrodistillation (Zhao et al., 2018).
Microwave-assisted extraction
Organic compounds can be extracted from plant material with more ease and effectiveness using microwave technology than using traditional methods. It is a technology that saves time. It is a very efficient technology with a high yield. This technology is sustainable because it requires less heat (Huie, 2002; Kaufmann and Christen, 2002; Wang and Weller, 2006; Chen et al., 2007; Cravotto et al., 2008; Sticher, 2008; Zhang et al., 2009). It involves a simple and economical process as compared to supercritical fluid extraction. It has a difficult operation as compared to ultrasonic-assisted extraction (Hayat et al., 2009). Extraction of Capsaicin through Capsicum was done through microwave-assisted extraction by Chuichulcherm et al. (2013). A study reported that phenolic compounds (alkaloids) were extracted from Citrus limetta through microwave-assisted extraction (Arafat et al., 2020). In another study, phenolic compounds were extracted from orange peel through microwave-assisted extraction (Nayak et al., 2015). Microwave-assisted extraction is used for obtaining thymoquinone from N. sativa (Liu et al., 2011; Oskouei et al., 2018). Various alkaloids were extracted from plants using microwave-assisted extraction (Bergs et al., 2013; Ngo et al., 2017; Aqil et al., 2020).
Ultrasound-assisted extraction
Ultrasound-assisted extraction is a technique that involves throwing sound energy to plants and agitating it thus extracting the required materials through a plant cell. In this technology, low energy and chemicals are required. High yield can be obtained from it, and it is a time-saving technology. Proper optimization in ultrasound frequency, the nominal power of the device, input power, propagation of cycle, and system geometry is required for maximum yield (Azmir et al., 2013a; Barba et al., 2015; Sneha et al., 2022). Studies report the extraction of Capsaicin from Capsicum, phenolic compounds (alkaloids) from orange peel and citrus fruit peel through ultrasound-assisted extraction (Chuichulcherm et al., 2013; Omar et al., 2013; Boukroufa et al., 2015; Nishad et al., 2019). Different methods were used to extract thymoquinone from Nigella sativa including Ultrasound-assisted extraction (Kausar et al., 2017).
High-voltage electrical discharge
A high-voltage electrical discharge is a non-thermal method that relies on ambient temperature and uses pulsed rapid discharge voltages. It is a technique that saves time and uses less energy. However, at the industrial and pilot level, this technology has not yet been utilized (Barba et al., 2015; Li et al., 2019; Rajha et al., 2019; Žuntar et al., 2019). Extraction of phenolic compounds (alkaloids) from grapefruit peel (El Kantar et al., 2019) and from the mandarin peel (Buniowska et al., 2015), through high voltage electric discharge was studied in respective studies.
Comparative analysis of different extraction technologies for extracting bioactive compounds
Selecting an extraction technique presents one of the biggest challenges in getting bioactive compounds from plants because many factors need to be taken into account, including the solvent's polarity, the chemical makeup of the bioactive compound, yield, selectivity, temperature, solubility, extraction time, and cost. Natural plant extracts can be obtained in a variety of ways, but the elements of the process profoundly affect the final product's quality. Among the extraction techniques, the supercritical extraction and/or the extraction utilizing pressurized fluids can be highlighted. In these procedures, a highly compressible fluid is used as a solvent in low- or mid-temperature conditions. The utilization of supercritical fluids is a possible technique for the extraction and fractionation of natural resources, particularly for food and pharmaceuticals. The key advantages of supercritical fluid extraction include high selectivity, ease of solvent removal, and use of mild temperatures (Correa et al., 2017). Using Capsicum frutescens L. (Chuichulcherm et al., 2013), investigated the extraction of capsaicinoids using microwave-assisted extraction (MAE) and ultrasound-assisted extraction (UAE), in comparison to the conventional soxhlet method. They concluded that the UAE approach was the best technique for extracting capsaicinoids from C. frutescens. Therefore, even though the amounts of capsaicinoids in MAE and UAE were 5.28 and 4.01 mg/g of C. frutescens, respectively, UAE needed the least amount of energy and was deemed the best approach. The low-quality oil produced by traditional solvent extraction methods necessitates intensive purifying procedures, which cause thermal deterioration and the loss of important components. There has been a shift toward more environmentally friendly extraction techniques, such supercritical fluid extraction, as a result of the use of organic solvents and the need to recover the solvent (SFE). SFE is evolving into a successful and widely utilized method for extracting valuable natural compounds from challenging materials (Ghahramanloo et al., 2017). Particularly, maceration, microwave-assisted extraction (MAE) and ultrasound-assisted extraction (UAE) have been considered as popular extraction techniques; Zhang and his coworkers concluded that MAE was the most effective with a yield of 7.04 ± 0.14 mg/g chelerythrine and 17.10 ± 0.4 mg/g sanguinarine with 5 min of extraction time (Zhang et al., 2005). High hydrostatic pressure (HHP), ultra-sonics and pulsed electric fields (PEF) were used for extracting bioactive compounds from grapes and the finding of this study was; the total phenolic content of samples was 50% higher than in the control samples (Corrales et al., 2008). Typically, phenolics have antibacterial and antioxidant properties. Due to their unique molecular structure, can offer superior platforms for making antimicrobial products (Siddiqui et al., 2022). High pressure (HP), pulsed electric fields (PEF), and low pasteurization (LPT) were used for extracting, pulsed electric field and low pasteurization; the former was the most effective treatment for the extraction of bioactive components from orange juice (Plaza et al., 2011). Conventional soxhlet extraction, MAE and ultrasound assisted extraction (UAE) were used for extracting bioactive compounds from agiorgitico red grape pomace. This study concluded that UAE was the most effective one (Drosou et al., 2015). There have been several research investigations on the extraction of bioactive substances using traditional and non-conventional (green) methods, including soxhlet extraction, microwave, supercritical fluid extraction, ultrasound extraction, etc. Each methodology has pros and cons. For instance, the greatest yield (26.52%) and total phenolic content (15,263.32 mg Eq gallic/100 g DW) from citrus reticulata blanco cv. sainampueng peel were obtained via the extraction of bioactive components under the ideal UAE conditions. UAE therefore demonstrated greater extraction efficiency in terms of yield under the same extraction circumstances, which was 1.77 times higher than MAE (Saini et al., 2019).
Purification
Purification of antimicrobial agents is generally the final step. There are various purification methods that can be used to purify antimicrobial agents. The choice of a specific purification method in any study depends on the degree of purification required for specific antimicrobial agents. In the case of polar compounds, purification is done mainly by filtration, ultrafiltration, paper chromatography, thin-layer chromatography, gas chromatography and high-performance liquid chromatography. Whereas, non-polar compound purification is mainly performed by; liquid-liquid extraction, reversed phase purification chromatography and through Hydrophobic interaction chromatography (Thumar et al., 2010; Galanakis, 2012; Ingle et al., 2017; Tang et al., 2018; Martinenghi et al., 2020).
Filtration
Filtration is a technique that involves pouring a combination of solid and liquid onto a membrane or a filter paper, which permits the passage of liquid (the filtrate) and results in the collection of the solid, this process is used to separate solids from liquids. During filtration, the size of the materials that can be removed relies on the filter's pore size. With just one filter, this technique can filter out a variety of undesirable particles of various sizes and is comparatively inexpensive (McGaw et al., 2002; Jin et al., 2007; Mushore and Matuvhunye, 2013; Sahle and Okbatinsae, 2017).
Ultrafiltration
Ultrafiltration (UF) is a type of membrane filtration, that forces a liquid against a semi-permeable membrane by hydrostatic pressure. Water and solutes <0.005 micro meter pass through the barrier, whereas suspended solids and solutes >0.1 micro meter are trapped. Compared to traditional methods, ultrafiltration technology can accomplish more work in 50% less space (Pampanin et al., 2012; Wang et al., 2013; Tang et al., 2018; Kellogg et al., 2019; Martinenghi et al., 2020).
Paper chromatography
In this method, separations are performed using a piece of paper that serves as both a support and a medium for separation. The filter paper is placed in the chromatographic chamber with the solvent, and the sample is put close to the bottom of the filter paper. By means of capillary action, the solvent flows while also transferring soluble molecules. Paper chromatography has the benefit that separations can be done easily on sheets of filter paper, which serves as both a substrate and a medium for separation. The high degree of reproducibility of Rf (retention factor) values can also be obtained on paper through this technique (Thumar et al., 2010; Galanakis, 2012; Ingle et al., 2017; Muhamad et al., 2017; Tang et al., 2018; Martinenghi et al., 2020).
Thin-layer chromatography
Using this method, samples are separated based on their interactions with a thin layer of adsorbent that is adhered to the plate of low molecular weight substances. Compounds are separated using various adsorbents. The particular benefit of TLC over paper chromatography is its adaptability, speed, and sensitivity (Thumar et al., 2010; Galanakis, 2012; Tang et al., 2018; Martinenghi et al., 2020).
Gas chromatography
Utilizing this method, volatile substances are separated. Through its distribution in the gas phase, the chemical species' rate of kinetics is identified. While the liquid phase is still, the gas phase is moving. The chemical species' rate of migration is estimated based on how widely distributed it is in the gas phase. For instance, a species that disperses 100% of itself into the gas phase will migrate at the same rate as the gas that is flowing, whereas a species that disperses 100% of itself into the stationary phase will not move at all. In gas chromatography, a sample is vaporized and then injected into the chromatographic column's head. The flow of the inert, gaseous mobile phase carries the sample through the column. A liquid stationary phase that is adsorbed onto the surface of an inert solid is present in the column itself (Thumar et al., 2010; Galanakis, 2012; Ingle et al., 2017; Tang et al., 2018; Sreedharan et al., 2019; Martinenghi et al., 2020).
High-performance liquid chromatography
HPLC is an analytical technique used for the separation and measurement of organic and inorganic solutes in any samples, particularly biological, pharmaceutical, food, environmental, industrial, etc. This method divides compounds by how they interact with the solvent of the mobile phase and the solid particles of a densely packed column. To help in identifying the chemicals, the Diode Array Detector examines the analytes' absorption spectra (Thumar et al., 2010; Galanakis, 2012; Ingle et al., 2017; Tang et al., 2018; Martinenghi et al., 2020).
Reverse-phase chromatography
In reverse-phase chromatography (RPC), molecules are separated from one another through hydrophobic interactions between the ligands attached to the stationary phase and the solute molecules in the mobile phase of the liquid chromatography. Better solubility for polar analytes is provided by reverse-phase chromatography, which also uses non-toxic solvents, provides a means for removing impurities and mobile phase additives, and provides rapid sample recovery with minimal solvent evaporation (Nair and Kanfer, 2006; Thumar et al., 2010; Galanakis, 2012; Tang et al., 2018; Martinenghi et al., 2020).
Hydrophobic interaction chromatography
Based on their hydrophobicity, molecules are separated using hydrophobic interaction chromatography (HIC). Due to the use of less denaturing conditions and matrices, HIC is a good separation approach for removing proteins while preserving biological activity. This technique can improve the mass spectrometry's sensitivity of detection. When compared to reversed-phase liquid chromatography, the flow rate in this approach can be larger because the mobile phase's viscosity is low. Low viscosity mobile phases enable the use of columns that are twice as long as those required for reverse phase HPLC. The sample separates very quickly due to the eluent's viscosity, which is also correlated with a lower separation impedance. Compared to reverse phase HPLC, basic solutes can be loaded more readily (Thumar et al., 2010; Galanakis, 2012; Sagar et al., 2012; Tang et al., 2018; Martinenghi et al., 2020).
Antibiotic resistance mechanisms in disease causing bacteria
Microbial infections are on the rise globally, and the human population is at risk due to microbes because these microbes are the major cause of worldwide mortalities, as shown in Table 3. Basically, disease causing bacteria have resistance against various antibiotics due to myriad mechanisms. These mechanisms are: (1) Bacteria can have resistance through transformation, transduction, and conjugation phenomenon. (2) Through the processes of phosphorylation, adenylation, or acetylation bacteria deactivate the antibiotics. (3) Bacteria prevent the interaction of drugs and antibiotics. (4) They can cause efflux of the antibiotic from the cell (Munita and Arias, 2016; Reygaert, 2018; Abushaheen et al., 2020). Recently, resistance to antibiotics has been the biggest challenge which threatens human communities. Meanwhile, the occurrence of the evolution of resistance endangers the effectiveness of existing antibiotic drugs (Baym et al., 2016; Marston et al., 2016). Therefore, several strategies have been proposed to overcome the antimicrobial resistance. One of the recommended methods to achieve this goal is the usage of natural compounds that are derived from plants. These plant extracts seem to be ideally suited to overcome the emergence of antibiotic resistance in multidrug resistance bacteria (Ncube et al., 2008; Rossiter et al., 2017).
Anti-microbial properties of Capsicum, Nigella sativa, peels of Musa paradisiaca L., and Citrus limetta
Plants have a plethora of constituents that impose antimicrobial effects (Gyawali et al., 2011; Mickymaray et al., 2016; Dewapriya et al., 2018; Casciaro et al., 2019; Ali M. Y. et al., 2021). Two major groups of antibiotics extracted from plants are; (1) phytoanticipins and (2) phytoalexins. Phytoanticipins inhibit microbial actions, whereas Phytoalexins are generally antioxidants (Sukalingam et al., 2017, 2018). Moreover, various secondary plant metabolites are known to cause a significant antimicrobial effect which will be discussed in later section.
Antimicrobial mechanism of bioactive compounds
These secondary metabolites are grouped into three types; (1) phenolic compounds, (2) terpenes, and (3) alkaloids (Crozier et al., 2006; Mickymaray, 2019; Roaa, 2020). These antimicrobial extracts cause cell wall disruption and lysis, cell wall construction, inhibit biofilm formation, inhibit microbial DNA replication, inhibit energy synthesis, inhibit bacterial toxins to the host, and induce reactive oxygen species production. Despite all these effects, these antimicrobials also prevent antimicrobial resistance and synergetics to antibiotics, which helps kill pathogenic organisms (Tariq et al., 2019; Zheng et al., 2020; Vaou et al., 2022), as shown in Figure 4.
Promote cell wall disruption and lysis
Cell disruption mechanism takes place via two approaches that are mechanical and non-mechanical approach (Figure 5). The detail of the antimicrobial mechanism is given in separate subsequent paragraphs. Firstly, compounds that functionalized this disruption are delineated as follows.
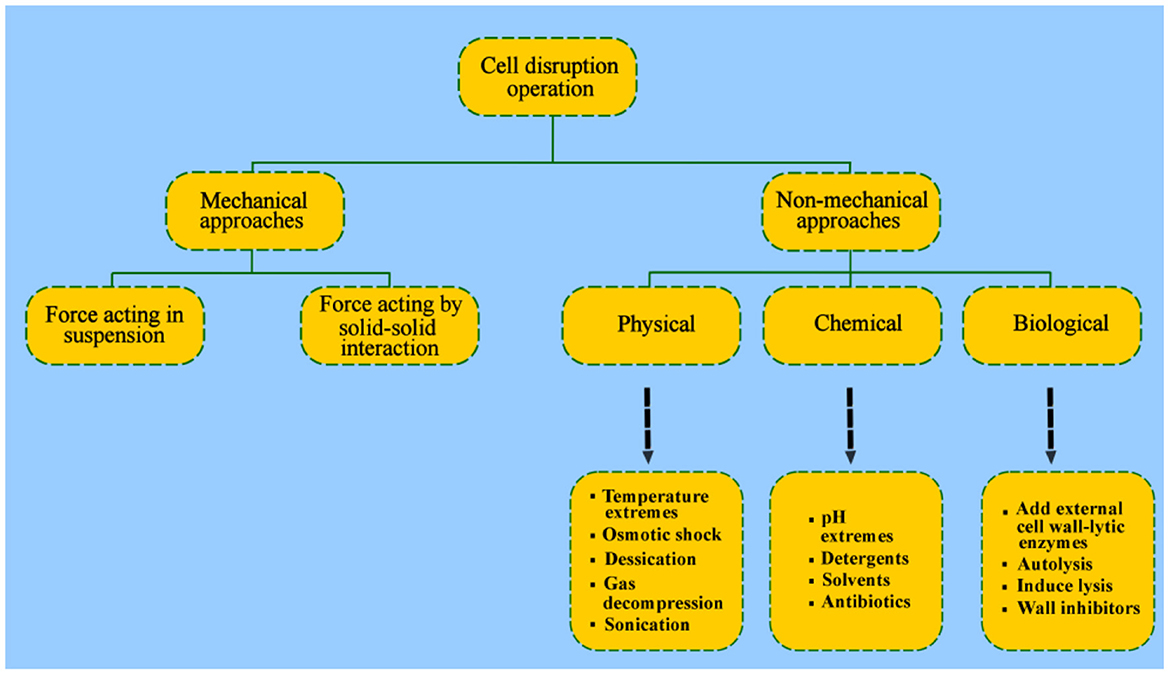
Figure 5. Antimicrobial mechanism by promoting cell wall disruption and lysis (Moo-Young, 2019).
Phenolic compounds belong to the family of aromatics containing a hydroxyl functional group, which in reaction with the microorganisms disrupts their cell wall (Ganesan and Xu, 2017a, 2018). These aromatics are moved to the microbe's cell surface, and thus they disrupt their cell walls. Flavonoids are phenolic compounds that form a complex relationship with the bacterial cell wall and thus disrupt its structure (Ganesan and Xu, 2017b,c). Rutin, naringenin, sophoraflavanone, and tiliroside are the flavonoids that disrupt S. aureus and S. mutans microbes (Tsuchiya and Iinuma, 2000; Sanver et al., 2016). Terpenes contain isoprene that disrupts microbial membranes (Guimarães et al., 2019; Moghrovyan et al., 2019).
Induction of reactive oxygen species production
Reactive oxygen species are formed by the partial reduction of molecular oxygen that helps in antimicrobial activity and provides a defense mechanism against various microbes. The method of catechins involves augmentation of the production of oxidative stress that disrupts cell walls (Fathima and Rao, 2016; Van Acker and Coenye, 2017; Zou et al., 2017). Sources of reactive oxygen species, antioxidant defenses, and subsequent biological effects depending on the level of reactive oxygen species production are shown in Figure 6.
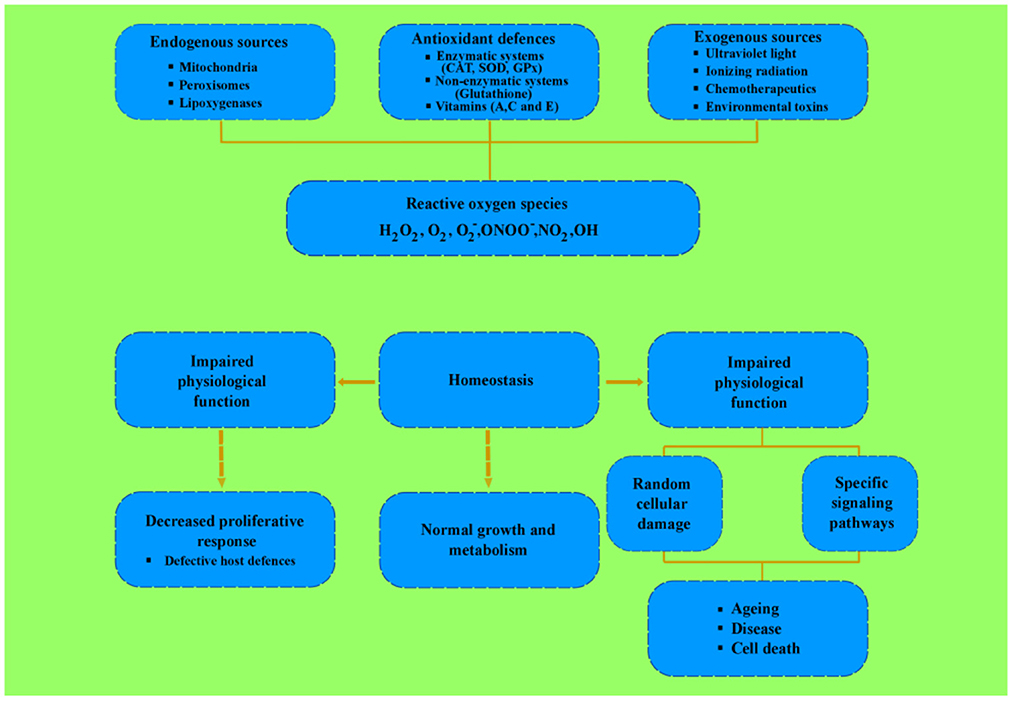
Figure 6. Endogenous and exogenous sources of ROS, antioxidant defenses, and biological effects that depends on ROS production.
Inhibition of biofilm formation
Biofilms developed by bacteria are 100–1,000 times more resistant to the antimicrobial drugs (Kahaliw et al., 2017; Mishra et al., 2020). In some studies, it was indicated that flavonoids aggregate the multicellular composites; thus, inhibiting bacteria growth. Flavonoids such as; (1) galangin, (2) isovitexin, (3) 3-O-octanoyl-epicatechin, and (4) 5, 7, and 40-trihydroxyflavanol cause the aggregation of S. aureus and S. mutans; therefore inhibiting their growths (Awolola et al., 2014; Rabin et al., 2015).
Inhibition of cell wall construction
The bacterial cell wall is the main target of any antimicrobial agent because the cell wall is the main source of biosynthesis of lipids, respiration and the transport mechanism. For all these functions, membrane integrity plays a major role in bacterial species, so the membrane disruption leads to bacterial death (Lazar and Walker, 2002; Klis et al., 2006; Reygaert, 2014).
Inhibition of bacterial toxins against the host
Catechins and some other flavonoids cause disruption of bacteria cell walls thus resulting in an inability to discharge toxins (Lee et al., 2011; Chang et al., 2019). Compounds such as catechins, pinocembrin, kaempferol, kaempferol-3-O-rutinoside, gallocatechin gallate, quercetin glycoside, genistein, and proanthocyanidins neutralize bacterial toxic factors initiating from N. gonorrhoeae, V. cholerae, E. coli, B. anthracis, V. vulnificus, S. aureus, and C. botulinum (Shahnazari et al., 2011; Ahmed et al., 2016).
Inhibition of energy synthesis
The production of energy or adenosine triphosphate (ATP) is important for the development of bacteria because it is the main source of a living system. The reaction of flavonoids such as isobavachalcone and 6-prenylapigenin with S. aureus results in disruption of the bacterial cell wall (Freudenberg and Mager, 1971; Kuete et al., 2011; Xie et al., 2015).
Inhibition of microbial DNA replication
Antimicrobial agents inhibit DNA replication thus stopping the further division of microbes. Moreover, bioactive compounds present in plants such as kaempferol, quercetin, apigenin, genistein, nobiletin, myricetin, chrysin, tangeritin, and 3, 6, 7, 30, 40-pentahydroxyflavone are DNA disrupters (Gotoh et al., 2008; van Eijk et al., 2017; Vijayakumar et al., 2018).
Antimicrobial mechanism of antimicrobial agents
Antimicrobial mechanisms of antimicrobial agents present in Nigella sativa, Capsicum, Musa paradisiaca L., and Citrus limetta are discussed below;
Antimicrobial mechanism of thymoquinone
Thymoquinone plays a major role in fighting against microbes. Basically, thymoquinone causes resistance in the biofilm formation in bacterial species because biofilm formation is the important activity of microbes in their virulence strategy. Thymoquinone also causes hindrance in the oxidative activity of microbes thus reducing the number of microbes (Harzallah et al., 2011; Forouzanfar et al., 2014; Khan, 2018; Fan et al., 2021).
Microbes cause many infectious diseases like AIDS, dengue, MERS, MRSA, and VRE, etc. Thymoquinone prevents the murine cytomegalovirus (CMV) replication in the liver and spleen of the infected mice. Medication with thymoquinone increases the number of CD4+ T cells which plays a major role in building the immune system. Thymoquinone increases levels of serum interferon-γ (IFN-γ) and macrophages. Thymoquinone also fights against various fungal pathogens like Candida albicans and Aspergillus fumigatus (Khan, 2018; Wang et al., 2021; Qureshi et al., 2022).
Antimicrobial mechanism of melanin
Melanin constitutes a heterogenous group of phenolic polymers, which plays a major role in fighting against microbes. Melanin is an important antimicrobial agent which stops the proliferation of pathogens around the infected area. Melanin can fight against food pathogens; thus, contributes a major role in the food and health sector. Melanin also helped in antimicrobial drug discovery. Melanin extracted from hair and further dopped in metal ions demonstrated the potential of antibacterial activity (Mackintosh, 2001; Alviano et al., 2004; Nosanchuk and Casadevall, 2006; Eliato et al., 2021).
Antimicrobial mechanism of P-cymene
P-cymene is an important antimicrobial agent which is found in more than 100 plants and contributes to the food and medicine sector. P-cymene is used in the preparation of fungicides and pesticides. P-cymene affects the bacterial membrane, pH and ATP thus contributing to the file of antimicrobes. Studies reported that p-cymene inhibits the growth of E. coli, L. monocytogenes, S. enterica, S. aureus, Vibrio parahaemolyticus, and Streptococcus mutans (Kordali et al., 2008; Marchese et al., 2017; Miladi et al., 2017; Balahbib et al., 2021).
Antimicrobial mechanism of pinene
Pinene belongs to the group of terpenes and has antimicrobial properties. Pinene inhibits microorganisms by interfering in their metabolic process and modulation of gene (Tyc et al., 2017; do Amaral et al., 2020). Pinene has its antimicrobial activity (Dhar et al., 2014; Zhang et al., 2021) against C. neoformans, C. neoformans phospholipase and esterase, C. Albicans, R. oryzae, and MRSA (Silva et al., 2012; Kovač et al., 2015).
Antimicrobial mechanism of alkaloid
Alkaloids disrupt the cell membrane of microbes, inhibit cellular division, inhibit efflux pump, and inhibit biofilm formation thus act as a lead compound in the development of antimicrobial agents. Studies reported that alkaloids can kill Pseudomonas aeruginosa, Streptococcus mutans, faecalis and pyogenes, Lactobacillus acidophilus, Candida albicans, and Staphylococcus aureus (Orhan et al., 2010; Özçelik et al., 2011; Mittal and Jaitak, 2019; Othman et al., 2019; Zielińska et al., 2019; Huang et al., 2022).
Antimicrobial mechanism of limonene
Limonene belongs to the family of monoterpenes. Limonene by accumulating in the membrane of microbes causes disruption in it. Limonene is also one of the main causes of the dissipation of proton motive force in microbes. Limonene being an antimicrobial agent has a broad application in the food sector. Studies reported that limonene can kill gram-positive and gram-negative bacterial species. Limonene is also found to be effective against fungal pathogens (Cai et al., 2019; Han et al., 2019, 2020; Gupta et al., 2021).
Antimicrobial mechanism of camphene
Camphene is a lipophilic antimicrobial compound that by penetrating in cell membranes of bacterial species becomes the reason of cell death. Camphene inhibits microorganism by disruption in many cell activities, and the breakdown of the membrane thus cellular components and ions start leaking from the bacterial body. Also, camphene is the major source of depletion of ATP in bacterial species. Studies reported that camphene has antibacterial, antiviral, and antifungal activities (Zhou et al., 2016; Er et al., 2018; de Freitas et al., 2020; Hachlafi et al., 2021).
Conclusion
Microbial diseases are a major threat to the human population. The worldwide death rates due to microbes are: Pseudomonas aeruginosa 61%, E. coli 4.2%, Staphylococcus epidermidis 20%, Candida albicans 24%, Shigella flexneri 13.2%, Sal. Typhimurium 3 million each year, and Vibrio alginolyticus 50%. However, these death rates can be reduced by using antimicrobial agents on a regular basis. This review evaluated the antimicrobial effects of four plant resources- Capsicum, Nigella sativa, Musa paradisiaca L., and Citrus limetta. These natural resources contain thymoquinone, p-cymene, pinene, alkaloid, limonene, camphene, and melanin, which serve as antimicrobial agents by disrupting the cell membrane of microbes, inhibiting the cellular division and inhibiting the formation of biofilm in bacterial species, thus reducing the number of microbes. Soxhlet, hydro-distillation, solid-phase extraction (SPE), liquid-liquid extraction (LLE), pressurized liquid extraction (PLE), supercritical fluid extraction (SFE), pulsed electric field (PEF), microwave-assisted extraction (MAE), enzyme-assisted extraction (EAE), ultrasound-assisted extraction (UAE), and high-voltage electrical discharge are the various techniques used for extracting critical antimicrobial agents from Capsicum, Nigella sativa, peels of Musa paradisiaca L., and Citrus limetta. A brief description of all these techniques was given along with their advantages and disadvantages in order to ease the selection of appropriate technology for the extraction of naturally available antimicrobial compounds.
Author contributions
SB, WZ, MH, and AM equally contributed to the concept of the study, its framework, the coordination and activities of subgroups, and writing of the manuscript. MM, MG, MI, SS, SI, SU-R, and SK equally contributed to the activities of subgroups and the review of the manuscript. All authors contributed to the article and approved the submitted version.
Funding
This work was funded in part by the USDA/NIFA through the Agricultural Research Program at North Carolina Agricultural and Technical State University (Evans-Allen Program, project number NC.X-291-5-15-170-1), by an 1890 Capacity Building Program Grant (No. 2020-38821-31113/project accession No. 021765), and (Project Nos. NC.X337-5-21-170-1 and NC.X341-5-21-170-1) from the National Institute of Food and Agriculture (NIFA). SI would like to acknowledge the support of the Agricultural Research Station at North Carolina Agricultural and Technical State University (Greensboro, NC, United States).
Acknowledgments
We would like to acknowledge the support of the Agricultural Research Station at North Carolina Agricultural and Technical State University (Greensboro, NC, United States).
Conflict of interest
The authors declare that the research was conducted in the absence of any commercial or financial relationships that could be construed as a potential conflict of interest.
Publisher's note
All claims expressed in this article are solely those of the authors and do not necessarily represent those of their affiliated organizations, or those of the publisher, the editors and the reviewers. Any product that may be evaluated in this article, or claim that may be made by its manufacturer, is not guaranteed or endorsed by the publisher.
References
Abbas, K. M., Abdulamir, A., and Abas, H. (2008). A review on supercritical fluid extraction as new analytical method. Am. J. Biochem. Biotechnol. 4, 345–353. doi: 10.3844/ajbbsp.2008.345.353
Abd-Talib, N., Mohd-Setapar, S. H., and Khamis, A. K. (2014). The benefits and limitations of methods development in solid phase extraction: mini review. J. Teknol. 69, 3177. doi: 10.11113/jt.v69.3177
Abushaheen, M. A., Fatani, A. J., Alosaimi, M., Mansy, W., George, M., Acharya, S., et al. (2020). Antimicrobial resistance, mechanisms and its clinical significance. Dis. Mon. 66, 100971. doi: 10.1016/j.disamonth.2020.100971
Ahmad, A., Rehman, M. U., and Ahmad, P. (2020). Covid-19 and thymoquinone: connecting the dots. Phytother. Res. 2020, ptr.6793. doi: 10.1002/ptr.6793
Ahmad, I., and Beg, A. Z. (2001). Antimicrobial and phytochemical studies on 45 Indian medicinal plants against multi-drug resistant human pathogens. J. Ethnopharmacol. 74, 113–123. doi: 10.1016/S0378-8741(00)00335-4
Ahmed, S. I., Hayat, M. Q., Tahir, M., Mansoor, Q., Ismail, M., and Keck, K. (2016). Pharmacologically active flavonoids from the anticancer, antioxidant and antimicrobial extracts of Cassia angustifolia Vahl. BMC Complement. Med. Ther. 16, 1–9. doi: 10.1186/s12906-016-1443-z
Ajijolakewu, K. A., Ayoola, A. S., Agbabiaka, T. O., Folashade, R. Z., Nike, R. A., Olusegun, J. O., et al. (2021). A review of the ethnomedicinal, antimicrobial, and phytochemical properties of Musa paradisiaca (plantain). Bull. Natl. Res. Cent. 45, 1–17. doi: 10.1186/s42269-021-00549-3
Akpan, A., and Morgan, R. (2002). Oral candidiasis. Postgrad. Med. J. 78, 455–459. doi: 10.1136/pmj.78.922.455
Alañón, M. E., García-Ruiz, A., Díaz-Maroto, M. C., Pérez-Coello, M. S., and Moreno-Arribas, M. V. (2015). Antimicrobial and antioxidant activity of pressurized liquid extracts from oenological woods. Food Control 50, 581–588. doi: 10.1016/j.foodcont.2014.09.043
Albano, M., Karau, M. J., Schuetz, A. N., and Patel, R. (2020). Comparison of agar dilution to broth microdilution for testing in vitro activity of cefiderocol against Gram-negative bacilli. J. Clin. Microbiol. 59, e00966–e00920. doi: 10.1128/JCM.00966-20
Ali, A., Wu, H., Ponnampalam, E. N., Cottrell, J. J., Dunshea, F. R., and Suleria, H. A. (2021). Comprehensive profiling of most widely used spices for their phenolic compounds through lc-esi-qtof-ms2 and their antioxidant potential. Antioxidants 10, 721. doi: 10.3390/antiox10050721
Ali, B., and Blunden, G. (2003). Pharmacological and toxicological properties of Nigella sativa. Phytother. Res. 17, 299–305. doi: 10.1002/ptr.1309
Ali, M. Y., Akter, Z., Mei, Z., Mousumi, T., and Md Asaduzzaman, K. (2021). Thymoquinone in autoimmune diseases: therapeutic potential and molecular mechanisms. Biomed. Pharmacother. 134, 111–157. doi: 10.1016/j.biopha.2020.111157
Al-Owaisi, M., Al-Hadiwi, N., and Khan, S. A. (2014). GC-MS analysis, determination of total phenolics, flavonoid content and free radical scavenging activities of various crude extracts of Moringa peregrina (Forssk). Fiori leaves. Asian Pac. J. Trop. Biomed. 4, 964–970. doi: 10.12980/APJTB.4.201414B295
Alshareef, I. A. A. (2019). Antimicrobial Activity of Nigella sativa Seeds Extracts Against Staphylococcus aureus Nasal Isolates among Sudan University of Science and Technology Students. Avaialble online at: http://repository.sustech.edu/handle/123456789/23477 (accessed September 16, 2020).
Al-Snafi, A. E. (2016). Medicinal plants with antimicrobial activities (part 2): plant based review. Sch. Acad. J. Pharm. 5, 208–239. doi: 10.21276/sajp.2016.5.6.2
Alvarez-Rivera, G., Bueno, M., Ballesteros-Vivas, D., Mendiola, J. A., and Ibanez, E. (2020). “Pressurized liquid extraction,” in Liquid-Phase Extraction, ed. C. Poole (Amsterdam: Elsevier), 375–398.
Alviano, D. S., Franzen, A. J., Travassos, L. R., Holandino, C., Rozental, S., Ejzemberg, R., et al. (2004). Melanin from Fonsecaea pedrosoi induces production of human antifungal antibodies and enhances the antimicrobial efficacy of phagocytes. Infect. Immun. 72, 229–237. doi: 10.1128/IAI.72.1.229-237.2004
Aqil, M., Chaudhuri, A., and Qadir, A. (2020). Herbal cosmeceuticals: new opportunities in cosmetology. Trends Phytochem. Res. 4, 117–142. Available online at: https://www.researchgate.net/publication/348648864_Herbal_cosmeceuticals_New_opportunities_in_cosmetology
Arafat, Y., Altemimi, A., Ibrahim, S. A., and Laxmikant, S. B. (2020). Valorization of sweet lime peel for the extraction of essential oil by solvent free microwave extraction enhanced with ultrasound pretreatment. Molecules 25, 4072. doi: 10.3390/molecules25184072
Avossa, J., Pota, G., Vitiello, G., Di Napoli, M., Pezzella, A., Errico, G. D., et al. (2021). Multifunctional mats by antimicrobial nanoparticles decoration for bioinspired smart wound dressing solutions. Mater. Sci. Eng. C. 123, 111954. doi: 10.1016/j.msec.2021.111954
Awaisheh, S. S., Al-Nabulsi, A. A., Osaili, T. M., Ibrahim, S., and Holley, R. (2013). Inhibition of Cronobacter sakazakii by heat labile bacteriocins produced by probiotic LAB isolated from healthy infants. J. Food Sci. 78, M1416–M1420. doi: 10.1111/1750-3841.12209
Awaisheh, S. S., and Ibrahim, S. A. (2009). Screening of antibacterial activity of lactic acid bacteria against different pathogens found in vacuum-packaged meat products. Foodborne Pathog. Dis. 6, 1125–1132. doi: 10.1089/fpd.2009.0272
Awolola, G. V., Koorbanally, N. A., Chenia, H., Francis, O. S., and Himansu, B. (2014). Antibacterial and anti-biofilm activity of flavonoids and triterpenes isolated from the extracts of Ficus sansibarica Warb. subsp. sansibarica (Moraceae) extracts. Afr. J. Tradit. Complement. Altern. Med. 11, 124–131. doi: 10.4314/ajtcam.v11i3.19
Azmir, J., Zaidul, I., Rahman, M., Sahena, F., Jahurul, M. H. A., Ghafoor, K., et al. (2013a). Techniques for extraction of bioactive compounds from plant materials: a review. J. Food Eng. 117, 426–436.
Azmir, J., Zaidul, I., Rahman, M., Sahena, F., Jahurul, M. H. A., Ghafoor, K., et al. (2013b). Techniques for extraction of bioactive compounds from plant materials: a review. J. Food Eng. 117, 426–436. doi: 10.1016/j.jfoodeng.2013.01.014
Azzouz, A., Kailasa, S. K., Lee, S., Andrés, S., Rascón, J., Ballesteros, E., et al. (2018). Review of nanomaterials as sorbents in solid-phase extraction for environmental samples. Trends Analyt. Chem. 108, 347–369. doi: 10.1016/j.trac.2018.08.009
Bakal, S. N., Bereswill, S., and Heimesaat, M. M. (2017). Finding novel antibiotic substances from medicinal plants—antimicrobial properties of Nigella sativa directed against multidrug resistant bacteria. Eur. J. Immunol. 7, 92–98. doi: 10.1556/1886.2017.00001
Bakathir, H. A., and Abbas, N. A. (2011). Detection of the antibacterial effect of nigella sativa ground seedswith water. Afr. J. Tradit. Complement. Altern. Med. 8, 159–164. doi: 10.4314/ajtcam.v8i2.63203
Bakhshabadi, H., Mirzaei, H., Ghodsvali, A., Jafari, S. M., and Ziaiifar, A. M. (2018). The influence of pulsed electric fields and microwave pretreatments on some selected physicochemical properties of oil extracted from black cumin seed. Food Sci. Nutr. 6, 111–118. doi: 10.1002/fsn3.535
Bakht, J., Noor, N., Iqbal, A., and Shafi, M. (2020). Antimicrobial activity of different solvent extracted samples from the leaves and fruits of Capsicum annuum. Pak. J. Pharm. Sci. 33, 27–32.
Balahbib, A., El Omari, N., Hachlafi, N. E., Zengin, G., and Bouyahya, A. (2021). Health beneficial and pharmacological properties of p-cymene. Food Chem. Toxicol. 153, 112259. doi: 10.1016/j.fct.2021.112259
Baranitharan, M., Krishnappa, K., and Elumalai, K. (2020). Citrus limetta (Risso)-borne compound as novel mosquitocides: effectiveness against medical pest and acute toxicity on non-target fauna. S. Afr. J. Bot. 128, 218–224. doi: 10.1016/j.sajb.2019.11.014
Barba, F., Puértolas, E., and Brncic, M. (2015). Food Waste Recovery Processing Technologies and Industrial Techniques, ed C. M. Galanakis. (Amsterdam: Elsevier). ISBN: 9780128225929
Barghout, N., Chebata, N., Moumene, S., and Seddik, K. (2020). Antioxidant and antimicrobial effect of alkaloid bulbs extract of Polianthes tuberosa L. (Amaryllidaceae) cultivated in Algeria. J. Drug Deliv. Ther. 10, 44–48. doi: 10.22270/jddt.v10i4.4134
Baym, M., Stone, L. K., and Kishony, R. (2016). Multidrug evolutionary strategies to reverse antibiotic resistance. Science 351, 3292. doi: 10.1126/science.aad3292
Beena, P., Rajesh, K. J., and Arul, B. (2016). Preliminary phytochemical screening of Cicer arietinum in folklore medicine for hepatoprotection. J. Innov. Pharm. Biol. Sci. 3, 153–159. Available online at: https://scholar.google.com/scholar?hl=en&as_sdt=0%2C5&q=Beena%2C+P.%2C+Rajesh%2C+K.+J.%2C+and+Arul%2C+B.+%282016%29.+Preliminary+phytochemical+screening+of+Cicer+arietinum+in+folklore+medicine+for+hepatoprotection.+J.+Innov.+Pharm.+Biol.+Sci.+3%2C+153%E2%80%93159&btnG=
Bensah, E. C., and Mensah, M. (2013). Chemical pretreatment methods for the production of cellulosic ethanol: technologies and innovations. Int. J. Chem. Eng. 2013, 719607. doi: 10.1155/2013/719607
Bergs, D., Merz, J., Delp, A., Joehnck, M., Martin, G., and Schembecker, G. (2013). A standard procedure for the selection of solvents for natural plant extraction in the early stages of process development. Chem. Eng. Technol. 36, 1739–1748. doi: 10.1002/ceat.201300276
Bilal, M., Adeel, M., Rasheed, T., Zhao, Y., and Iqbal, H. M. (2019). Emerging contaminants of high concern and their enzyme-assisted biodegradation–a review. Environ. Int. 124, 336–353. doi: 10.1016/j.envint.2019.01.011
Blahušiak, M., Kiss, A. A., Babic, K., Kersten, R. A., Bargeman, G., Schuur, B., et al. (2018). Insights into the selection and design of fluid separation processes. Sep. Purif. Technol. 194, 301–318. doi: 10.1016/j.seppur.2017.10.026
Blinov, A. V., Siddiqui, S. A., Blinova, A. A., Khramtsov, A. G., Oboturova, N. P., Nagdalian, A. A., et al. (2022). Analysis of the dispersed composition of milk using photon correlation spectroscopy. J. Food Compos. Anal. 108, 104414. doi: 10.1016/j.jfca.2022.104414
Bor, T., Aljaloud, S. O., Gyawali, R., and Ibrahim, S. A. (2016). Antimicrobials From Herbs, Spices, and Plants Fruits, Vegetables, and Herbs (Amsterdam: Elsevier), 551–578. doi: 10.1016/B978-0-12-802972-5.00026-3
Boukroufa, M., Boutekedjiret, C., Petigny, L., Rakotomanomana, N., and Chemat, F. (2015). Bio-refinery of orange peels waste: a new concept based on integrated green and solvent free extraction processes using ultrasound and microwave techniques to obtain essential oil, polyphenols and pectin. Ultrason. Sonochem. 24, 72–79. doi: 10.1016/j.ultsonch.2014.11.015
Brooks, A. (2008). Ethanol production potential of local yeast strains isolated from ripe banana peels. Afr. J. Biotechnol. 7, 3749–3752. Available online at: https://www.ajol.info/index.php/ajb/article/view/59424
Buniowska, M., Carbonell-Capella, J., Zulueta, A., Frigola, A., and Esteve, M. J. (2015). Bioaccessibility of bioactive compounds and antioxidant capacity from orange peel after pulsed electric fields and high voltage electrical discharges. Int. J. Food Process. Technol. 1, 17. doi: 10.15406/mojfpt.2015.01.00017
Buyukkurt, O. K., Guclu, G., Kelebek, H., and Selli, S. (2019). Characterization of phenolic compounds in sweet lime (Citrus limetta) peel and freshly squeezed juices by LC-DAD-ESI-MS/MS and their antioxidant activity. J. Food Meas. Charact. 13, 3242–3249. doi: 10.1007/s11694-019-00246-w
Cai, R., Hu, M., Zhang, Y., Niu, C., Yue, T., Yuan, Y., et al. (2019). Antifungal activity and mechanism of citral, limonene and eugenol against Zygosaccharomyces rouxii. LWT 106, 50–56. doi: 10.1016/j.lwt.2019.02.059
Cao, H., Lai, Y., Bougouffa, S., Xu, Z., and Yan, A. (2017). Comparative genome and transcriptome analysis reveals distinctive surface characteristics and unique physiological potentials of Pseudomonas aeruginosa ATCC 27853. BMC Genom. 18, 459. doi: 10.1186/s12864-017-3842-z
Careaga, M., Fernández, E., Dorantes, L., Mota, L., Jaramillo, M. E., and Hernandez-Sanchez, H. (2003). Antibacterial activity of Capsicum extract against Salmonella typhimurium and Pseudomonas aeruginosa inoculated in raw beef meat. Int. J. Food Microbiol. 83, 331–335. doi: 10.1016/S0168-1605(02)00382-3
Casciaro, B., Calcaterra, A., Cappiello, F., Mangoni, M. L., Botta, B., and Quaglio, D. (2019). Nigritanine as a new potential antimicrobial alkaloid for the treatment of Staphylococcus aureus-induced infections. Toxins 11, 511. doi: 10.3390/toxins11090511
Cesari, L., Canabady-Rochelle, L., and Mutelet, F. (2019). Separation of phenols from lignin pyrolysis oil using ionic liquid. Sep. Purif. Technol. 209, 528–534. doi: 10.1016/j.seppur.2018.07.083
Chaieb, K., Kouidhi, B., Jrah, H., Mahdouani, K., and Bakhrouf, A. (2011). Antibacterial activity of thymoquinone, an active principle of Nigella sativa and its potency to prevent bacterial biofilm formation. BMC Complement. Med. Ther. 11, 29. doi: 10.1186/1472-6882-11-29
Chang, E. H., Huang, J., Lin, Z., and Brown, A. C. (2019). Catechin-mediated restructuring of a bacterial toxin inhibits activity. Biochim. Biophys. Acta Gen. Subj. 1863, 191–198. doi: 10.1016/j.bbagen.2018.10.011
Chen, F., Jia, J., Zhang, Q., Gu, H., and Yang, L. (2017). A modified approach for isolation of essential oil from fruit of Amorpha fruticosa Linn using microwave-assisted hydrodistillation concatenated liquid-liquid extraction. J. Chromatogr. A 1524, 254–265. doi: 10.1016/j.chroma.2017.10.008
Chen, S. Y., and Urban, P. L. (2015). On-line monitoring of Soxhlet extraction by chromatography and mass spectrometry to reveal temporal extract profiles. Anal. Chim. Acta. 881, 74–81. doi: 10.1016/j.aca.2015.05.003
Chen, Y., Xie, M. Y., and Gong, X. F. (2007). Microwave-assisted extraction used for the isolation of total triterpenoid saponins from Ganoderma atrum. J. Food Eng. 81, 162–170. doi: 10.1016/j.jfoodeng.2006.10.018
Chenni, M., El Abed, D., Rakotomanomana, N., Fernandez, X., and Chemat, F. (2016). Comparative study of essential oils extracted from Egyptian basil leaves (Ocimum basilicum L.) using hydro-distillation and solvent-free microwave extraction. Molecules 21, 113. doi: 10.3390/molecules21010113
Chitranshi, S., Dubey, N., and Sajjad, M. (2020). Sustainable botanical products for safe post-harvest management of perishable produce: a review. J. Hortic. Postharvest Res. 3, 125–140. doi: 10.22077/JHPR.2019.2703.1083
Chuichulcherm, S., Prommakort, S., Srinophakun, P., and Thanapimmetha, A. (2013). Optimization of capsaicin purification from Capsicum frutescens Linn. with column chromatography using Taguchi design. Ind. Crops Prod. 44, 473–479. doi: 10.1016/j.indcrop.2012.10.007
Coburn, B., Grassl, G. A., and Finlay, B. (2007). Salmonella, the host and disease: a brief review. Immunol. Cell Biol. 85, 112–118. doi: 10.1038/sj.icb.7100007
Centers for Disease Control Prevention (2011). Gram-Negative Bacteria Infections in Healthcare Settings. Available online at: https://www.cdc.gov/hai/organisms/gram-negative-bacteria.html (accessed October 8, 2020).
Corrales, M., Toepfl, S., Butz, P., Knorr, D., and Tauscher, B. (2008). Extraction of anthocyanins from grape by-products assisted by ultrasonics, high hydrostatic pressure or pulsed electric fields: a comparison. Innov. Food Sci. Emerg. Technol. 9, 85–91. doi: 10.1016/j.ifset.2007.06.002
Correa, M., Bombardelli, M. C., Fontana, P. D., Bovo, F., Messias-Reason, I. J., Maurer, J. B. B., et al. (2017). Bioactivity of extracts of Musa paradisiaca L. obtained with compressed propane and supercritical CO2. J. Supercrit. Fluids 122, 63–69. doi: 10.1016/j.supflu.2016.12.004
Cos, P., Vlietinck, A. J., Berghe, D. V., and Maes, L. (2006). Anti-infective potential of natural products: how to develop a stronger in vitro ‘proof-of-concept'. J. Ethnopharmacol. 106, 290–302. doi: 10.1016/j.jep.2006.04.003
Cravotto, G., Boffa, L., Mantegna, S., Perego, P., Avogadro, M., and Cintas, P. (2008). Improved extraction of vegetable oils under high-intensity ultrasound and/or microwaves. Ultrason. Sonochem. 15, 898–902. doi: 10.1016/j.ultsonch.2007.10.009
Crozier, A., Jaganath, I. B., and Clifford, M. N. (2006). Phenols, polyphenols and tannins: an overview. Plant Second. Metabolit. 1, 1–25. doi: 10.1002/9780470988558.ch1
Das, J., Deka, M., and Gogoi, K. (2018). Antimicrobial activity of chilli extracts (Capsicum Chinense) against food borne pathogens Escherichia coli and Staphylococcus aureus. Int. J. Res. Anal. 5, 717–720. Available online at: https://www.ijrar.org/papers/IJRAR1944311.pdf
Das, K., Tiwari, R. K. S., and Shrivastava, D. K. (2010). Techniques for evaluation of medicinal plant products as antimicrobial agent: current methods and future trends. J. Med. Plants Res 4, 104–111. Available online at: https://www.researchgate.net/publication/330556242_Techniques_for_evaluation_of_medicinal_plant_products_as_antimicrobial_agent_Current_methods_and_future_trends
De Castro, M. L., and Priego-Capote, F. (2010). Soxhlet extraction: past and present panacea. J. Chromatogr. A. 1217, 2383–2389. doi: 10.1016/j.chroma.2009.11.027
de Freitas, B. C., Queiroz, P. A., Baldin, V. P., Vandresen, F., Caleffi-Ferracioli, K. R., and Siqueira, V. L. D. (2020). Camphene-based derivatives as potential antibacterial agents against Staphylococcus aureus and Enterococcus spp. Future Microbiol. 15, 1527–1534. doi: 10.2217/fmb-2020-0131
Deng, Q., Zinoviadou, K. G., Galanakis, C. M., Orlien, V., Grimi, N., Vorobiev, E., et al. (2015). The effects of conventional and non-conventional processing on glucosinolates and its derived forms, isothiocyanates: extraction, degradation, and applications. Food Eng. Rev. 7, 357–381. doi: 10.1007/s12393-014-9104-9
Dewapriya, P., Khalil, Z. G., Prasad, P., Cruz-Morales, E., Marcellin, R., and Capon, J. (2018). Talaropeptides AD: structure and biosynthesis of extensively N-methylated linear peptides from an Australian marine tunicate-derived Talaromyces sp. Front. Chem. 6, 394. doi: 10.3389/fchem.2018.00394
Dhar, P., Chan, P., Cohen, D. T., Khawam, F., Gibbons, S., Snyder-Leiby, T., et al. (2014). Synthesis, antimicrobial evaluation, and structure–activity relationship of α-pinene derivatives. J. Agric. Food Chem 62, 3548–3552. doi: 10.1021/jf403586t
Dhawan, D., and Gupta, J. (2017). Research article comparison of different solvents for phytochemical extraction potential from datura metel plant leaves. Int. J. Biol. Chem. 11, 17–22. doi: 10.3923/ijbc.2017.17.22
Dhingra, S., Rahman, N. A. A., Peile, E., Rahman, M., Sartelli, M., Hassali, M. A., et al. (2020). Microbial resistance movements: an overview of global public health threats posed by antimicrobial resistance, and how best to counter. Front. Public Health 8, 535668. doi: 10.3389/fpubh.2020.535668
Di Rauso Simeone, G., Di Matteo, A., Rao, M. A., Rao, M. A., and Vaio, C. D. (2020). Variations of peel essential oils during fruit ripening in four lemon [Citrus limon (L.) Burm. F.] cultivars. J. Sci. Food Agric. 100, 193–200. doi: 10.1002/jsfa.10016
Diaz, Y. H., Arranz, J., Fernández, R., Fidalgo, L. M., Jaén-Batista, D. G., and da Silva, C. F. (2020). Trypanocidal potentialities of skimmianine an alkaloid isolated from Zanthoxylum pistaciifolium griseb leaves. Pharmacogenomics J. 12, 322. Available online at: https://www.researchgate.net/publication/343770096_Trypanocidal_Potentialities_of_Skimmianine_an_Alkaloid_Isolated_from_Zanthoxylum_pistaciifolium_Griseb_Leaves
Digrak, M., Alma, M. H., Ilcim, A., and Sen, S. (1999). Antibacterial and antifungal effects of various commercial plant extracts. Pharm. Biol. 37, 216–220. doi: 10.1076/phbi.37.3.216.6307
do Amaral, F. L. E., Farias, T. C., de Brito, R. C., de Melo, T. R., Ferreira, P. B., Lima, Z. N., et al. (2020). Effect of the association and evaluation of the induction to adaptation of the (+)-α-pinene with commercial antimicrobials against strains of Escherichia coli. Curr. Top. Med. Chem. 20, 2300–2307. doi: 10.2174/1568026620666200820150425
Doernberg, S. B., Lodise, T. P., Thaden, J., Corey, G. R., Miller, L. G., and Holland, T. L. (2017). Gram-positive bacterial infections: research priorities, accomplishments, and future directions of the Antibacterial Resistance Leadership Group. Clin. Infect. Dis. 64, S24–S29. doi: 10.1093/cid/ciw828
Dorantes, L., Colmenero, R., Hernandez, H., Jaramillo, M. E., Fernandez, E., and Solano, C. (2000). Inhibition of growth of some foodborne pathogenic bacteria by Capsicum annum extracts. Int. J. Food Microbiol. 57, 125–128. doi: 10.1016/S0168-1605(00)00216-6
Drosou, C., Kyriakopoulou, K., Bimpilas, A., Tsimogiannis, D., and Krokida, M. (2015). A comparative study on different extraction techniques to recover red grape pomace polyphenols from vinification byproducts. Ind. Crops Prod. 75, 141–149. doi: 10.1016/j.indcrop.2015.05.063
Du, Y., Schuur, B., and Brilman, D. (2017). Maximizing lipid yield in Neochloris oleoabundans algae extraction by stressing and using multiple extraction stages with N-ethylbutylamine as switchable solvent. J. Ind. Eng. Chem. 56, 8073–8080. doi: 10.1021/acs.iecr.7b01032
Duncan-Lowey, J. K., Wiscovitch, A. L., Wood, T., Wood, E. T., Goldberg, B. M., and Russo, B. C. (2020). Shigella flexneri disruption of cellular tension promotes intercellular spread. Cell Rep. 33, 108409. doi: 10.1016/j.celrep.2020.108409
Dutta, T., Ghosh, N. N., Das, M., Adhikary, R., Mandal, V., and Chattopadhyay, A. P. (2020a). Green synthesis of antibacterial and antifungal silver nanoparticles using Citrus limetta peel extract: experimental and theoretical studies. J. Environ. Chem. Eng. 2020, 104019.
Dutta, T., Ghosh, N. N., Das, M., Adhikary, R., Mandal, V., and Chattopadhyay, A. P. (2020b). Green synthesis of antibacterial and antifungal silver nanoparticles using Citrus limetta peel extract: experimental and theoretical studies. J. Environ. Chem. Eng. 8, 104019. doi: 10.1016/j.jece.2020.104019
Edelson, B. T., and Unanue, E. (2000). Immunity to listeria infection. Curr. Opin. Immunol. 12, 425–431. doi: 10.1016/S0952-7915(00)00112-6
El Blidi, L., Saleh, J., Ben Ghanem, O., El-Harbawi, M., Lvque, J. M., and Hadj-Kali, M. K. (2019). Synthesis, characterization, and antimicrobial toxicity study of dicyanamide-based ionic liquids and their application to liquid–liquid extraction. J. Chem. Eng. Data 65, 34–42. doi: 10.1021/acs.jced.9b00654
El Kantar, S., Boussetta, N., Lebovka, N., Louka, N., and Vorobiev, E. (2018). Pulsed electric field treatment of citrus fruits: improvement of juice and polyphenols extraction. Innov. Food Sci. Emerg. Technol. 46, 153–161. doi: 10.1016/j.ifset.2017.09.024
El Kantar, S., Rajha, H. N., Boussetta, N., Maroun, G. R., and Louka, N. (2019). Green extraction of polyphenols from grapefruit peels using high voltage electrical discharges, deep eutectic solvents and aqueous glycerol. Food Chem. 295, 165–171. doi: 10.1016/j.foodchem.2019.05.111
El Khoury, R., Michael Jubeli, R., El Beyrouthy, M., Ali Tfayli, P. H. D., and Roger Lteif, P. H. D. (2019). Phytochemical screening and antityrosinase activity of carvacrol, thymoquinone, and four essential oils of Lebanese plants. J. Cosmet. Dermatol. 18, 944–952. doi: 10.1111/jocd.12754
El-Dakhakhny, M., Barakat, M., Abd El-Halim, M., and Aly, S. M. (2000). Effects of Nigella sativa oil on gastric secretion and ethanol induced ulcer in rats. J. Ethnopharmacol. 72, 299–304. doi: 10.1016/S0378-8741(00)00235-X
Eliato, T. R., Smith, J. T., Tian, Z., Kim, E. S., Hwang, W., Andam, C. P., et al. (2021). Melanin pigments extracted from horsehair as antibacterial agents. J. Mater. Chem. B 9, 1536–1545. doi: 10.1039/D0TB02475A
El-Kamali, H., Ahmed, A., Mohammed, A., Ahmed, A. H., Mohammed, B. A., and Yahia, A. M. (1998). Antibacterial properties of essential oils from Nigella sativa seeds, Cymbopogon citratus leaves and Pulicaria undulata aerial parts. Fitoterapia 69, 77–78.
El-Naggar, M. E., Soliman, R. A., Morsy, O., and Abdel-Aziz, M. S. (2020). Nanoemulsion of Capsicum fruit extract as an eco-friendly antimicrobial agent for production of medical bandages. Biocatal. Agric. Biotechnol. 23, 101516. doi: 10.1016/j.bcab.2020.101516
Elyemni, M., Louaste, B., Nechad, I., Taleb, M., Chaouch, M., and Eloutassi, N. (2019). Extraction of essential oils of Rosmarinus officinalis L. by two different methods: hydrodistillation and microwave assisted hydrodistillation. Sci. World J. 45, 3659432. doi: 10.1155/2019/3659432
Er, Y., Sivri, N., and Mirik, M. (2018). Antimicrobial activity of essential oil against Rhizobium (Agrobacterium) vitis using agar well and disc diffusion method. J. Bacteriol. 8, 1–11. doi: 10.3923/bj.2018.1.11
Erkan, N., Tao, Z., Rupasinghe, H. V., Uysal, B., and Oksal, B. S. (2012). Antibacterial activities of essential oils extracted from leaves of Murraya koenigii by solvent-free microwave extraction and hydro-distillation. Nat. Prod. Commun. 7, 1934578X1200700139. doi: 10.1177/1934578X1200700139
Espinosa-Alonso, L. G., Lygin, A., Widholm, J., Valverde, M. E., and Paredes-Lopez, O. (2006). Polyphenols in wild and weedy Mexican common beans (Phaseolus vulgaris L.). J. Agric. Food Chem. 54, 4436–4444. doi: 10.1021/jf060185e
Fabricant, D. S., and Farnsworth, N. R. (2001). The value of plants used in traditional medicine for drug discovery. Environ. Health Perspect. 109, 69–75. doi: 10.1289/ehp.01109s169
Fan, Q., Yuan, Y., Jia, H., Zeng, X., Wang, Z., Hu, Z., et al. (2021). Antimicrobial and anti-biofilm activity of thymoquinone against Shigella flexneri. Appl. Microbiol. Biotechnol. 105, 4709–4718. doi: 10.1007/s00253-021-11295-x
Fathima, A., and Rao, J. (2016). Selective toxicity of Catechin—a natural flavonoid towards bacteria. Appl. Microbiol. Biotechnol. 100, 6395–6402. doi: 10.1007/s00253-016-7492-x
Fidrianny, I., Rizki, K., and Insanu, M. (2014). In vitro antioxidant activities from various extracts of banana peels using ABTS, DPPH assays and correlation with phenolic, flavonoid, carotenoid content. Int. J. Pharm. Pharm. 6, 299–303. Available online at: https://www.semanticscholar.org/paper/IN-VITRO-ANTIOXIDANT-ACTIVITIES-FROM-VARIOUS-OF-AND-Fidrianny-KikiRizki/eef056225ea55e8107a1d0b664f03ec836590bb5
Fierascu, R. C., Fierascu, I., Avramescu, S., and Sieniawska, E. (2019). Recovery of natural antioxidants from agro-industrial side streams through advanced extraction techniques. Molecules 24, 4212. doi: 10.3390/molecules24234212
Forouzanfar, F., Bazzaz, B. S. F., and Hosseinzadeh, H. (2014). Black cumin (Nigella sativa) and its constituent (thymoquinone): a review on antimicrobial effects. Iran J. Basic Med. Sci. 17, 929. doi: 10.22038/ijbms.2015.3849
Freudenberg, H., and Mager, J. (1971). Studies on the mechanism of the inhibition of protein synthesis induced by intracellular ATP depletion. Biochim. Biophy. Acta Protein Synth. 232, 537–555. doi: 10.1016/0005-2787(71)90608-3
Galanakis, C. (2012). Recovery of high added-value components from food wastes: conventional, emerging technologies and commercialized applications. Trends Food Sci. Technol. 26, 68–87. doi: 10.1016/j.tifs.2012.03.003
Ganesan, K., and Xu, B. (2017a). A critical review on polyphenols and health benefits of black soybeans. Nutrients 9, 455. doi: 10.3390/nu9050455
Ganesan, K., and Xu, B. (2017b). Polyphenol-rich dry common beans (Phaseolus vulgaris L.) and their health benefits. Int. J. Mol. Sci. 18, 2331. doi: 10.3390/ijms18112331
Ganesan, K., and Xu, B. (2017c). Polyphenol-rich lentils and their health promoting effects. Int. J. Mol. Sci. 18, 2390. doi: 10.3390/ijms18112390
Ganesan, K., and Xu, B. (2018). A critical review on phytochemical profile and health promoting effects of mung bean (Vigna radiata). Food Sci. Hum. Wellness. 7, 11–33. doi: 10.1016/j.fshw.2017.11.002
Garner, A. L. (2019). Pulsed electric field inactivation of microorganisms: from fundamental biophysics to synergistic treatments. Appl. Microbiol. Biotechnol. 103, 7917–7929. doi: 10.1007/s00253-019-10067-y
Ghahramanloo, K. H., Kamalidehghan, B., Javar, H. A., Widodo, R. T., Majidzadeh, K., and Noordin, M. I. (2017). Comparative analysis of essential oil composition of Iranian and Indian Nigella sativa L. extracted using supercritical fluid extraction and solvent extraction. Drug Des. Devel. Ther. 11, 2221. doi: 10.2147/DDDT.S87251
Gonçalves, D., Emil, M., Cristina, E. T., Koshima, C., and Elisabete, C. (2016). Fractionation of citrus essential oil by liquid–liquid extraction using a perforated rotating disc contactor. Sep. Purif. Technol. 163, 247–257. doi: 10.1016/j.seppur.2016.03.002
Gorgus, E., Hittinger, M., and Schrenk, D. (2016). Estimates of ethanol exposure in children from food not labeled as alcohol-containing. J. Anal. Toxicol. 40, 537–542. doi: 10.1093/jat/bkw046
Gotoh, H., Zhang, Y., Dallo, S. F., Hong, S., Kasaraneni, N., and Weitao, T. (2008). Pseudomonas aeruginosa, under DNA replication inhibition, tends to form biofilms via Arr. Res. Microbiol. 159, 294–302. doi: 10.1016/j.resmic.2008.02.002
Goyal, S., and Singh, J. (2022). Biphasic liquid-liquid extraction of biosurfactant from Lactobacillus delbrueckii. Braz. Arch. Biol. Technol. 65, 2022210325. doi: 10.1590/1678-4324-2022210325
Griffin, S. G., Markham, J. L., and Leach, D. N. (2000). An agar dilution method for the determination of the minimum inhibitory concentration of essential oils. J. Essent. Oil Res. 12, 249–255. doi: 10.1080/10412905.2000.9699509
Guimarães, A. C., Meireles, L. M., and Lemos, M. F. (2019). Antibacterial activity of terpenes and terpenoids present in essential oils. Molecules 24, 2471. doi: 10.3390/molecules24132471
Gupta, A., Jeyakumar, E., and Lawrence, R. (2021). Journey of limonene as an antimicrobial agent. J. Pure Appl. Microbiol. 15, 1094–1110. doi: 10.22207/JPAM.15.3.01
Gyawali, R., and Ibrahim, S. A. (2014). Natural products as antimicrobial agents. Food Control 46, 412–429. doi: 10.1016/j.foodcont.2014.05.047
Gyawali, R., Ibrahim, S. A., Abu Hasfa, S. H., Smqadri, S. Q., and Haik, Y. (2011). Antimicrobial activity of copper alone and in combination with lactic acid against Escherichia coli O157: H7 in laboratory medium and on the surface of lettuce and tomatoes. J. Pathog. 2011, 650968. doi: 10.4061/2011/650968
Habeebullah, S. F., Alagarsamy, S., Sattari, Z., Al-Haddad, S., Fakhraldeen, S., Al-Ghunaim, A., et al. (2020). Enzyme-assisted extraction of bioactive compounds from brown seaweeds and characterization. J. Appl. Phycol. 32, 615–629. doi: 10.1007/s10811-019-01906-6
Hachlafi, N. E., Aanniz, T., Menyiy, N. E., Baaboua, A. E., Omari, N. E., Balahbib, A., et al. (2021). In vitro and in vivo biological investigations of camphene and its mechanism insights: a review. Food Rev. Int. 2021, 1–28. doi: 10.1080/87559129.2021.1936007
Halawani, E. (2009). Antibacterial activity of thymoquinone and thymohydroquinone of Nigella sativa L. and their interaction with some antibiotics. Adv. Biol. Regul. 3, 148–152. Available online at: https://www.semanticscholar.org/paper/Antibacterial-Activity-of-Thymoquinone-and-of-L.-Halawani-Arabia/ac785b435940dab993e677f7ca4f6fd8a5bcc469
Hamid, A. A., Aiyelaagbe, O. O., Usman, L. A., Ameen, O. M., and Lawal, A. (2010). Antioxidants: its medicinal and pharmacological applications. Afr. J. Pure Appl Chem. 4, 142–151. Available online at: https://www.academia.edu/15038001/Antioxidants_Its_medicinal_and_pharmacological_applications
Han, Y., Sun, Z., and Chen, W. (2019). Antimicrobial susceptibility and antibacterial mechanism of limonene against Listeria monocytogenes. Molecules 25, 33.
Han, Y., Sun, Z., and Chen, W. (2020). Antimicrobial susceptibility and antibacterial mechanism of limonene against Listeria monocytogenes. Molecules 25, 33. doi: 10.3390/molecules25010033
Hanafy, M., and Hatem, M. (1991). Studies on the antimicrobial activity of Nigella sativa seed (black cumin). J. Ethnopharmacol. 34, 275–278. doi: 10.1016/0378-8741(91)90047-H
Hannan, A., Saleem, S., Chaudhary, S., Barkaat, M., and Arshad, M. U. (2008). Anti bacterial activity of Nigella sativa against clinical isolates of methicillin resistant Staphylococcus aureus. J. Ayub. Med. Coll. 20, 72–74. Available online at: https://pubmed.ncbi.nlm.nih.gov/19610522/#:~:text=Results%3A%20All%20tested%20strains%20of,has%20inhibitory%20effect%20on%20MRSA
Harzallah, H. J., Kouidhi, B., Flamini, G., Bakhrouf, A., and Mahjoub, T. (2011). Chemical composition, antimicrobial potential against cariogenic bacteria and cytotoxic activity of Tunisian Nigella sativa essential oil and thymoquinone. Food Chem. 129, 1469–1474. doi: 10.1016/j.foodchem.2011.05.117
Hayat, K., Hussain, S., Abbas, S., Xia, S., Jia, C., Zhang, X., et al. (2009). Optimized microwave-assisted extraction of phenolic acids from citrus mandarin peels and evaluation of antioxidant activity in vitro. Sep. Purif. Technol. 70, 63–70. doi: 10.1016/j.seppur.2009.08.012
Hernanz, D., Gallo, V., Recamales, A., Antonio, J., Martínez, M., and Heredia, F. J. (2008). Comparison of the effectiveness of solid-phase and ultrasound-mediated liquid–liquid extractions to determine the volatile compounds of wine. Talanta 76, 929–935. doi: 10.1016/j.talanta.2008.04.049
Hosseinzadeh, H., Fazly Bazzaz, B., and Haghi, M. (2007). Antibacterial activity of total extracts and essential oil of Nigella sativa L. seeds in mice. Pharmacologyonline 2, 429–435. doi: 10.1211/002235702777503000
Hu, X. Y., Logue, M., and Robinson, N. (2020). Antimicrobial resistance is a global problem–a UK perspective. Eur. J. Intern. Med. 36, 101136. doi: 10.1016/j.eujim.2020.101136
Huang, W., Wang, Y., Tian, W., Cui, X., Tu, P., Li, J., et al. (2022). Biosynthesis investigations of terpenoid, alkaloid, and flavonoid antimicrobial agents derived from medicinal plants. Antibiotics 11, 1380. doi: 10.3390/antibiotics11101380
Huchchannanavar, S., Yogesh, L., and Prashant, S. (2019). The black seed Nigella sativa: a wonder seed. Int. J. Chem. Stud. 7, 1320–1324. Available online at: https://icarkvkballari.org/wp-content/uploads/2022/06/SH1.pdf
Huie, C. W. (2002). A review of modern sample-preparation techniques for the extraction and analysis of medicinal plants. Anal. Bioanal. Chem. 373, 23–30. doi: 10.1007/s00216-002-1265-3
Ibáñez, M. D., Sanchez-Ballester, N. M., and Blázquez, M. A. (2020). Encapsulated limonene: a pleasant lemon-like aroma with promising application in the agri-food industry. A review. Molecules 25, 2598. doi: 10.3390/molecules25112598
Ibrahim, S. A., Ayivi, R. D., Zimmerman, T., Siddiqui, S. A., Altemimi, A. B., Fidan, H., et al. (2021). Lactic acid bacteria as antimicrobial agents: food safety and microbial food spoilage prevention. Foods 10, 3131. doi: 10.3390/foods10123131
Ibrahim, S. A., Yang, G., Song, D., and Tse, T. S. (2011). Antimicrobial effect of guava on Escherichia coli O157: H7 and Salmonella typhimurium in liquid medium. Int. J. Food Prop. 14, 102–109. doi: 10.1080/10942910903147833
Ighodaro, O. M. (2012). Evaluation study on Nigerian species of Musa paradisiaca peels. Researcher 4, 17–20. Available online at: http://www.sciencepub.net/researcher/research0408/004_10621research0408_17_20.pdf
Imam, M. Z., and Akter, S. (2011). Musa paradisiaca L. and Musa sapientum L.: a phytochemical and pharmacological review. J. Appl. Pharm. Sci. 5, 14–20. Available online at: https://japsonline.com/abstract.php?article_id=78&sts=2
Ingle, K. P., Deshmukh, A. G., Padole, D. A., Dudhare, M. S., Moharil, M. P., et al. (2017). Phytochemicals: extraction methods, identification and detection of bioactive compounds from plant extracts. J. Pharmacogn. Phytochem. 6, 32–36. Available online at: https://www.semanticscholar.org/paper/Phytochemicals%3A-Extraction-methods%2C-identification-Ingle-Deshmukh/c761e169d3630b80c98a778560bcd0591e8f2c89
Jacobsen, A. M., Halling-Sørensen, B., Ingerslev, F., and Hansen, S. H. (2004). Simultaneous extraction of tetracycline, macrolide and sulfonamide antibiotics from agricultural soils using pressurised liquid extraction, followed by solid-phase extraction and liquid chromatography–tandem mass spectrometry. J. Chromatogr. A 1038, 157–170. doi: 10.1016/j.chroma.2004.03.034
Javed, S., Ahmad, R., Shahzad, K., and Saleem, Y. (2013). Chemical constituents, antimicrobial and antioxidant activity of essential oil of Citrus limetta var. Mitha (sweet lime) peel in Pakistan. Afr. J. Microbiol. Res. 7, 3071–3077. doi: 10.5897/AJMR12.1254
Jessberger, N., Dietrich, R., and Märtlbauer, E. (2020). The Bacillus cereus food infection as multifactorial process. Toxins 12, 701. doi: 10.3390/toxins12110701
Jiang, Z. T., Feng, X., Li, R., and Wang, Y. (2013). Composition comparison of essential oils extracted by classical hydro distillation and microwave-assisted Hydrodistillation from Pimenta dioica. J. Essent. Oil-Bear. Plants. 16, 45–50. doi: 10.1080/0972060X.2013.764178
Jin, G., Wenling, T., and Lu, P. (2007). Isolation of pathogenic fungi of post-harvest Lingwuchangzao and screen of antibiosis material. J. Northwest Sci-Tech Uni. Agric. For. 35, 81–86. Available online at: https://agris.fao.org/agris-search/search.do?recordID=CN2008001488
Juan, C., Moltó, J. C., Mañes, J., and Font, G. (2010). Determination of macrolide and lincosamide antibiotics by pressurised liquid extraction and liquid chromatography-tandem mass spectrometry in meat and milk. Food Control 21, 1703–1709. doi: 10.1016/j.foodcont.2010.05.004
Jung, T., Jung, Y., Ahn, J., and Yang, S. (2020). Continuous, rapid concentration of foodborne bacteria (Staphylococcus aureus, Salmonella typhimurium, and Listeria monocytogenes) using magnetophoresis-based microfluidic device. Food Control 2020, 107229. doi: 10.1016/j.foodcont.2020.107229
Kahaliw, W., Aseffa, A., Abebe, M., Teferi, M., and Engidawork, E. (2017). Evaluation of the antimycobacterial activity of crude extracts and solvent fractions of selected Ethiopian medicinal plants. BMC Complement. Med. Ther. 17, 143. doi: 10.1186/s12906-017-1563-0
Kang, C. I., Kim, S. H., Kim, H. B., Myoung-Don, O. H., Eui-Chong, K., and Kang-Won, C. (2003). Pseudomonas aeruginosa bacteremia: risk factors for mortality and influence of delayed receipt of effective antimicrobial therapy on clinical outcome. Clin. Infect. Dis. 37, 745–751. doi: 10.1086/377200
Kardjeva, V., Summerbell, R., Kantardjiev, T., Devliotou-Panagiotidou, D., Sotiriou, E., and Gräser, Y. (2006). Forty-eight-hour diagnosis of onychomycosis with subtyping of Trichophyton rubrum strains. J. Clin. Microbiol. 44, 1419–1427. doi: 10.1128/JCM.44.4.1419-1427.2006
Karuppiah, P., and Mustaffa, M. (2013). Antibacterial and antioxidant activities of Musa sp. leaf extracts against multidrug resistant clinical pathogens causing nosocomial infection. Asian Pac. J. Trop. Biomed. 3, 737–742. doi: 10.1016/S2221-1691(13)60148-3
Kaufmann, B., and Christen, P. (2002). Recent extraction techniques for natural products: microwave-assisted extraction and pressurised solvent extraction. Phytochem. Anal. Int. J. Chem. Technol. 13, 105–113. doi: 10.1002/pca.631
Kausar, H., Abidin, L., and Mujeeb, M. (2017). Comparative assessment of extraction methods and quantitative estimation of thymoquinone in the seeds of Nigella sativa L. by HPLC. Int. J. Pharmacogn. Phytochem. Res. 9, 1425–1428. doi: 10.25258/phyto.v9i12.11186
Kellogg, J. J., Paine, M. F., McCune, J. S., Oberlies, N. H., and Cech, N. B. (2019). Selection and characterization of botanical natural products for research studies: a NaPDI center recommended approach. Nat. Prod. Rep. 36, 1196–1221. doi: 10.1039/C8NP00065D
Khalil, I. A., Troeger, C., Blacker, B., Qadri, F., Riddle, M. S., and Ryan, E. T. (2018). Morbidity and mortality due to shigella and enterotoxigenic Escherichia coli diarrhoea: the Global Burden of Disease Study 1990–2016. Lancet Infect. Dis. 18, 1229–1240. doi: 10.1016/S1473-3099(18)30475-4
Khan, M., Khan, M., Qais, F., Ahmad, I., and Althubiani, A. S. (2017). In vitro antibacterial activity of citrus limetta peel extracts against biofilm forming MDR gram negative bacterial pathogens. Univ. J. Phytochem. Ayurvedic Heights. 2, 4–11. Available online at: https://www.researchgate.net/publication/322144990_In_vitro_Antibacterial_Activity_of_Citrus_limetta_Peel_Extracts_against_Biofilm_Forming_MDR_Gram_Negative_Bacterial_Pathogens
Khan, M. A. (2018). Antimicrobial Action of Thymoquinone Molecular and Therapeutic actions of Thymoquinone (Berlin: Springer), 57–64. doi: 10.1007/978-981-10-8800-1_5
Klančnik, A., Piskernik, S., Jeršek, B., and MoŽina, S. S. (2010). Evaluation of diffusion and dilution methods to determine the antibacterial activity of plant extracts. J. Microbiol. Methods 81, 121–126. doi: 10.1016/j.mimet.2010.02.004
Klis, F. M., Boorsma, A., and De Groot, P. W. (2006). Cell wall construction in Saccharomyces cerevisiae. Yeast 23, 185–202. doi: 10.1002/yea.1349
Koffi-Nevry, R., Kouassi, K. C., Nanga, Z., Koussémon, M., and Loukou, G. Y. (2012). Antibacterial activity of two bell pepper extracts: Capsicum annuum L. and Capsicum frutescens. Int. J. Food Prop. 15, 961–971. doi: 10.1080/10942912.2010.509896
Kokollari, F., Daka, A., Blyta, Y., Ismajli, F., and Haxhijaha-Lulaj, K. (2015). Tinea corporis, caused by microsporum canis-a case report from Kosovo. Arch. Med. Res. 69, 345. doi: 10.5455/medarh.2015.69.345-346
Kordali, S., Cakir, A., Ozer, H., Cakmakci, R., Kesdek, M., and Mete, E. (2008). Antifungal, phytotoxic and insecticidal properties of essential oil isolated from Turkish Origanum acutidens and its three components, carvacrol, thymol and p-cymene. Bioresour. Technol. 99, 8788–8795. doi: 10.1016/j.biortech.2008.04.048
Koubala, B., Mbome, L., Kansci, G., Crepeau, M. J., Thibault, J. F., and Ralet, M. C. (2008). Physicochemical properties of pectins from ambarella peels (Spondias cytherea) obtained using different extraction conditions. Food Chem. 106, 1202–1207. doi: 10.1016/j.foodchem.2007.07.065
Kovač, J., Šimunović, K., Wu, Z., Klančnik, A., Bucar, F., Zhang, Q., et al. (2015). Antibiotic resistance modulation and modes of action of (-)-α-pinene in Campylobacter jejuni. PLoS ONE 10, e0122871. doi: 10.1371/journal.pone.0122871
Kuete, V., Ango, P. Y., Fotso, G. W., Dzoyem, J. P., Wouking, A. G., Ngadjui, B. T., et al. (2011). Antimicrobial activities of the methanol extract and compounds from Artocarpus communis (Moraceae). BMC Complement. Med. Ther. 11, 42. doi: 10.1186/1472-6882-11-42
Lazar, K., and Walker, S. (2002). Substrate analogues to study cell-wall biosynthesis and its inhibition. Curr. Opin Chem. Biol. 6, 786–793. doi: 10.1016/S1367-5931(02)00355-1
Lee, J. H., Regmi, S. C., Kim, J. A., Yun, H., Chang-Soo, L., and Lee, J. (2011). Apple flavonoid phloretin inhibits Escherichia coli O157: H7 biofilm formation and ameliorates colon inflammation in rats. Infect. Immun. 79, 4819–4827. doi: 10.1128/IAI.05580-11
Li, Z., Fan, Y., and Xi, J. (2019). Recent advances in high voltage electric discharge extraction of bioactive ingredients from plant materials. Food Chem. 277, 246–260. doi: 10.1016/j.foodchem.2018.10.119
Liang, Z., Mittal, G. S., and Griffiths, M. W. (2002). Inactivation of Salmonella Typhimurium in orange juice containing antimicrobial agents by pulsed electric field. J. Food Prot. 65, 1081–1087. doi: 10.4315/0362-028X-65.7.1081
Liu, X. M., Abd El-Aty, A., and Shim, J. H. (2011). Various extraction and analytical techniques for isolation and identification of secondary metabolites from Nigella sativa seeds. Mini. Rev. Med. Chem. 11, 947–955. doi: 10.2174/138955711797068472
Liu, Y., Ai, K., and Lu, L. (2014). Polydopamine and its derivative materials: synthesis and promising applications in energy, environmental, and biomedical fields. Chem. Rev. 114, 5057–5115. doi: 10.1021/cr400407a
Liu, Y., and Ji, P. (2018). Dietary factors in prevention of pediatric Escherichia coli infection: a model using domestic piglets. ILAR J. 59, 338–351. doi: 10.1093/ilar/ilz005
Lopresto, C. G., Petrillo, F., Casazza, A. A., Aliakbarian, B., Perego, P., and Calabrò, V. (2014). A non-conventional method to extract D-limonene from waste lemon peels and comparison with traditional Soxhlet extraction. Sep. Purif. Technol. 137, 13–20. doi: 10.1016/j.seppur.2014.09.015
Luengo, E., Álvarez, I., and Raso, J. (2013). Improving the pressing extraction of polyphenols of orange peel by pulsed electric fields. Innov. Food Sci. Emerg. Technol. 17, 79–84. doi: 10.1016/j.ifset.2012.10.005
Ma, J., Xu, R. R., Lu, Y., Ren, D. F., and Lu, J. (2018). Composition, antimicrobial and antioxidant activity of supercritical fluid extract of Elsholtzia ciliata. J. Essent. Oil Bear. Pl. 21, 556–562. doi: 10.1080/0972060X.2017.1409657
Mackintosh, J. A. (2001). The antimicrobial properties of melanocytes, melanosomes and melanin and the evolution of black skin. J. Theor Biol. 211, 101–113. doi: 10.1006/jtbi.2001.2331
Magaldi, S., Mata-Essayag, S., De Capriles, C. H., Pérez, C., Colella, M. T., Olaizola, C., et al. (2004). Well diffusion for antifungal susceptibility testing. Int. J. Infect. Dis. 8, 39–45. doi: 10.1016/j.ijid.2003.03.002
Magill, S. S., Edwards, J. R., Bamberg, W., McAllister-Hollod, L., Nadle, J., Wilson, L. E., et al. (2014). Multistate point-prevalence survey of health care–associated infections. N. Engl. J. Med. 370, 1198–1208. doi: 10.1056/NEJMoa1306801
Makvana, S., and Krilov, L. R. (2015). Escherichia coli infections. J. Pediatr. Rev. 36, 167–170. doi: 10.1542/pir.36.4.167
Marchese, A., Arciola, C. R., Barbieri, R., Izadi, M., Jafari, N. J., Daglia, M., et al. (2017). Update on monoterpenes as antimicrobial agents: a particular focus on p-cymene. Materials 10, 947. doi: 10.3390/ma10080947
Marini, E., Magi, G., Mingoia, M., Pugnaloni, A., and Facinelli, B. (2015). Antimicrobial and anti-virulence activity of capsaicin against erythromycin-resistant, cell-invasive group a streptococci. Front. Microbiol 6, 1281. doi: 10.3389/fmicb.2015.01281
Marston, H. D., Dixon, D. M., Knisely, J. M., Palmore, T. N., and Fauci, A. S. (2016). Antimicrobial resistance. J. Am. Med. Assoc. 316, 1193–1204. doi: 10.1001/jama.2016.11764
Martinenghi, L. D., Jønsson, R., Lund, T., and Jenssen, H. (2020). Isolation, purification, and antimicrobial characterization of cannabidiolic acid and cannabidiol from Cannabis sativa L. Biomolecules 10, 900. doi: 10.3390/biom10060900
Martínez-Ruano, J. A., Caballero-Galván, A. S., Restrepo-Serna, D., Restrepo-Serna, D. L., and Cardona, C. A. (2018). Techno-economic and environmental assessment of biogas production from banana peel (Musa paradisiaca) in a biorefinery concept. Environ. Sci. Pollut. Res. 25, 35971–35980. doi: 10.1007/s11356-018-1848-y
McDowell, R. H., Sands, E. M., and Friedman, H. (2020). Bacillus cereus. StatPearls. Available online at: https://www.ncbi.nlm.nih.gov/books/NBK459121 (accessed July 2, 2021).
McGaw, L. J., Jäger, A. K., Van Staden, J., and Eloff, J. N. (2002). Variation in antibacterial activity of Schotia species. S. Afr. J. Bot. 68, 41–46. doi: 10.1016/S0254-6299(15)30438-5
Mehta, Y., Gupta, A., Todi, S., Bhattacharya, P. K., and Ramasubban, S. (2014). Guidelines for prevention of hospital acquired infections. Ind. J. Crit. Care Med. 18, 149. doi: 10.4103/0972-5229.128705
Mendiola, J. A., Herrero, M., Cifuentes, A., and Ibañez, E. (2007). Use of compressed fluids for sample preparation: food applications. J. Chromatogr. A 1152, 234–246. doi: 10.1016/j.chroma.2007.02.046
Mickymaray, S. (2019). Efficacy and mechanism of traditional medicinal plants and bioactive compounds against clinically important pathogens. Antibiotics 8, 257. doi: 10.3390/antibiotics8040257
Mickymaray, S., Al Aboody, M. S., Rath, P. K., Annamalai, P., and Nooruddin, T. (2016). Screening and antibacterial efficacy of selected Indian medicinal plants. Asian Pac. J. Trop. Biomed. 6, 185–191. doi: 10.1016/j.apjtb.2015.12.005
Miguel, M. G. (2010). Antioxidant and anti-inflammatory activities of essential oils: a short review. Molecules 15, 9252–9287. doi: 10.3390/molecules15129252
Miladi, H., Zmantar, T., Kouidhi, B., Al Qurashi, Y. M. A., Bakhrouf, A., Chaabouni, Y., et al. (2017). Synergistic effect of eugenol, carvacrol, thymol, p-cymene and γ-terpinene on inhibition of drug resistance and biofilm formation of oral bacteria. Microb. Pathog. 112, 156–163. doi: 10.1016/j.micpath.2017.09.057
Mishra, R., Panda, A. K., De Mandal, S., Shakeel, M., Bisht, S. S., and Khan, J. (2020). Natural anti-biofilm agents: strategies to control biofilm-forming pathogens. Front. Microbiol. 11, 566325. doi: 10.3389/fmicb.2020.566325
Mittal, R. P., and Jaitak, V. (2019). Plant-derived natural alkaloids as new antimicrobial and adjuvant agents in existing antimicrobial therapy. Curr. Drug Targets. 20, 1409–1433. doi: 10.2174/1389450120666190618124224
Moghrovyan, A., Sahakyan, N., Babayan, A., Petrosyan, M., and Trchounian, A. (2019). Essential oil and ethanol extract of oregano (Origanum vulgare L.) from Armenian flora as a natural source of terpenes, flavonoids and other phytochemicals with antiradical, antioxidant, metal chelating, tyrosinase inhibitory and antibacterial activity. Curr. Pharm. Des. 25, 1809–1816. doi: 10.2174/1381612825666190702095612
Moo-Young, M. (2019). Comprehensive Biotechnology. Elsevier. Available online at: https://www.elsevier.com/books/comprehensive-biotechnology/moo-young/978-0-444-64046-8 (accessed July 5, 2021).
Morsi, N. M. (2000). Antimicrobial effect of crude extracts of Nigella sativa on multiple antibiotics-resistant bacteria. Acta Biochim. Pol. 49, 63–74. Available online at: https://pubmed.ncbi.nlm.nih.gov/10997492/#:~:text=Different%20crude%20extracts%20of%20Nigella,antibiotics%2C%20specially%20the%20gramnegative%20ones
Mügge, C., Haustein, U. F., and Nenoff, P. (2006). Onychomykosen—eine retrospektive Untersuchung zum Erregerspektrum. Journal der Deutschen Dermatologischen Gesellschaft. 4, 1. doi: 10.1111/j.1610-0387.2006.05877_1.x
Muhamad, I. I., Hassan, N. D., Mamat, S. N., Nawi, N. M., Rashid, W. A., and Tan, N. A. (2017). Extraction Technologies and Solvents of Phytocompounds From Plant Materials: Physicochemical Characterization and Identification of Ingredients and Bioactive Compounds From Plant Extract Using Various Instrumentations. In Ingredients Extraction by Physicochemical Methods in Food. (Cambridge, MA: Academic Press), 523–560. doi: 10.1016/B978-0-12-811521-3.00014-4
Munita, J. M., and Arias, C. A. (2016). Mechanisms of antibiotic resistance. Virulence mechanisms of bacterial pathogens. Microbiol. Spectr. 8, 481–511. doi: 10.1128/9781555819286.ch17
Mushore, J., and Matuvhunye, M. (2013). Antibacterial properties of Mangifera indica on Staphylococcus aureus. African J. Clin. Exp. Microbiol. 14, 62–74.i2.4. doi: 10.4314/ajcem.v14i2.4
Mustafa, A., and Turner, C. (2011). Pressurized liquid extraction as a green approach in food and herbal plants extraction: a review. Anal. Chim. Acta. 703, 8–18. doi: 10.1016/j.aca.2011.07.018
Nadar, S. S., Rao, P., and Rathod, V. K. (2018). Enzyme assisted extraction of biomolecules as an approach to novel extraction technology: a review. Food Res. Int. 108, 309–330. doi: 10.1016/j.foodres.2018.03.006
Naghshineh, M., Olsen, K., and Georgiou, C. A. (2013). Sustainable production of pectin from lime peel by high hydrostatic pressure treatment. Food Chem. 136, 472–478. doi: 10.1016/j.foodchem.2012.08.036
Nair, V. D., and Kanfer, I. (2006). High-performance liquid chromatographic method for the quantitative determination of hypoxoside in African potato (Hypoxis hemerocallidea) and in commercial products containing the plant material and/or its extracts. J. Agric. Food Chem. 54, 2816–2821. doi: 10.1021/jf052418s
Namita, P., and Mukesh, R. (2012). Medicinal plants used as antimicrobial agents: a review. Int. Res. J. Pharm. 3, 31–40. Available online at: https://www.researchgate.net/publication/285309397_Medicinal_plants_used_as_antimicrobial_agents_A_review
Nayak, B., Dahmoune, F., Moussi, K., Dairi, S., Aoun, O., and Khodir, M. (2015). Comparison of microwave, ultrasound and accelerated-assisted solvent extraction for recovery of polyphenols from Citrus sinensis peels. Food Chem. 187, 507–516. doi: 10.1016/j.foodchem.2015.04.081
Ncube, N. S., Afolayan, A. J., and Okoh, A. I. (2008). Assessment techniques of antimicrobial properties of natural compounds of plant origin: current methods and future trends. Afr. J. Biotechnol. 7, 613. doi: 10.5897/AJB07.613
Ngo, T. V., Scarlett, C. J., Bowyer, M. C., Ngo, P. D., and Vuong, Q. V. (2017). Impact of different extraction solvents on bioactive compounds and antioxidant capacity from the root of Salacia chinensis L. J. Food Qual. 2017, 9305047. doi: 10.1155/2017/9305047
Nguyen, P., and Mittal, G. S. (2007). Inactivation of naturally occurring microorganisms in tomato juice using pulsed electric field (PEF) with and without antimicrobials. Chem. Eng. Proces. 46, 360–365. doi: 10.1016/j.cep.2006.07.010
Nickavar, B., Adeli, A., and Nickavar, A. (2014). TLC-bioautography and GC-MS analyses for detection and identification of antioxidant constituents of Trachyspermum copticum essential oil. Iran. J. Pharm. Sci. 13, 127–133. Available online at: https://pubmed.ncbi.nlm.nih.gov/24734063/
Nishad, J., Saha, S., and Kaur, C. (2019). Enzyme-and ultrasound-assisted extractions of polyphenols from Citrus sinensis (cv. Malta) peel: a comparative study. J. Food Process. Preserv. 43, e14046. doi: 10.1111/jfpp.14046
Nortjie, E., Basitere, M., Moyo, D., and Nyamukamba, P. (2022). Extraction methods, quantitative and qualitative phytochemical screening of medicinal plants for antimicrobial textiles: a review. Plants 11, 2011. doi: 10.3390/plants11152011
Nosanchuk, J. D., and Casadevall, A. (2006). Impact of melanin on microbial virulence and clinical resistance to antimicrobial compounds. Antimicrob. Agents Chemother. 50, 3519–3528. doi: 10.1128/AAC.00545-06
Novaković, M., and Janković, S. (2011). The methodology of determining antibacterial activity of plant extracts with disk-diffusion method in vitro. Racion. Ter. 3, 33–37. doi: 10.5937/racter1102033N
Okorondu, S., Mepba, H., Okorondu, M., and Nwachukwu, I. (2010). Antibacterial properties of Musa paradisiaca peel extract. Curr. Trends Microbiol. 6, 21–26. Available online at: https://www.cabdirect.org/
Olabinjo, O. O., Ogunlowo, A. S., and Oliveira, A. L. (2020). Optimization of pressurized liquid extraction of essential oil from Citrus sinesis peels. Int. J. Agric. Eng. 22, 255–263. Available online at: https://cigrjournal.org/index.php/Ejounral/article/view/5541
Omar, J., Alonso, I., Garaikoetxea, A., and Etxebarria, N. (2013). Optimization of focused ultrasound extraction (FUSE) and supercritical fluid extraction (SFE) of citrus peel volatile oils and antioxidants. Food Anal. Methods. 6, 1244–1252. doi: 10.1007/s12161-012-9536-x
Omolo, M. A., Wong, Z. Z., Mergen, K., Reiland, H. A., Case, K. A., and Baumler, D. J. (2014). Antimicrobial properties of chili peppers. J. Infect. Dis. 8, 145. doi: 10.4172/2332-0877.1000145
Organization W. H. (2018). WHO Report on Surveillance of Antibiotic Consumption: Early Implementation. Available online at: https://www.who.int/publications/i/item/who-report-on-surveillance-of-antibiotic-consumption (accessed May 1, 2020).
Organization W. H. (2019). Critically Important Antimicrobials for Human Medicine. Available online at: https://www.who.int/home/cms-decommissioning (accessed April 3, 2020).
Orhan, D. D., Özçelik, B., Özgen, S., and Ergun, F. (2010). Antibacterial, antifungal, and antiviral activities of some flavonoids. Microbiol. Res. 165, 496–504. doi: 10.1016/j.micres.2009.09.002
Oskouei, Z., Akaberi, M., and Hosseinzadeh, H. (2018). A glance at black cumin (Nigella sativa) and its active constituent, thymoquinone, in ischemia: a review. Iran. J. Basic Med. Sci. 21, 1200. doi: 10.22038/ijbms.2018.31703.7630
Othman, L., Sleiman, A., and Abdel-Massih, R. M. (2019). Antimicrobial activity of polyphenols and alkaloids in middle eastern plants. Front. Microbiol. 10, 911. doi: 10.3389/fmicb.2019.00911
Othman, Z. A. A., Ahmed, Y. B. H., Habila, M. A., and Ghafar, A. A. (2011). Determination of capsaicin and dihydrocapsaicin in Capsicum fruit samples using high performance liquid chromatography. Molecules 16, 8919–8929. doi: 10.3390/molecules16108919
Otto, M. (2009). Staphylococcus epidermidis—the'accidental'pathogen. Nat. Rev. Microbiol. 7, 555–567. doi: 10.1038/nrmicro2182
Özçelik, B., Kartal, M., and Orhan, I. (2011). Cytotoxicity, antiviral and antimicrobial activities of alkaloids, flavonoids, and phenolic acids. Pharm. Biol. 49, 396–402. doi: 10.3109/13880209.2010.519390
Pampanin, D. M., Larssen, E., Provan, F., Sivertsvik, M., Ruoff, P., and Sydnes, M. O. (2012). Detection of small bioactive peptides from Atlantic herring (Clupea harengus L.). Peptides 34, 423–426. doi: 10.1016/j.peptides.2012.02.005
Pandey, A., and Tripathi, S. (2014). Concept of standardization, extraction and pre phytochemical screening strategies for herbal drug. J. Pharmacog. Phytochem. 2, 115–119. Available online at: https://www.phytojournal.com/archives?year=2014&vol=2&issue=5&ArticleId=255
Parashar, S., Sharma, H., and Garg, M. (2014). Antimicrobial and antioxidant activities of fruits and vegetable peels: a review. J. Pharmacog. Phytochem. 3, 160–164. Available online at: https://www.phytojournal.com/archives/2014.v3.i1.329/antimicrobial-and-antioxidant-activities-of-fruits-and-vegetable-peels-a-review
Parekh, J., Jadeja, D., and Chanda, S. (2005). Efficacy of aqueous and methanol extracts of some medicinal plants for potential antibacterial activity. Turk. J. Biol. 29, 203–210. Available online at: https://www.researchgate.net/publication/239604762_Efficacy_of_Aqueous_and_Methanol_Extracts_of_Some_Medicinal_Plants_for_Potential_Antibacterial_ Activity#:~:text=The%20methanol%20extracts%20were%20more,subtilis%2C% 20followed%20by%20S
Park, B. B., An, J. Y., and Park, S. (2021). Recent studies on pinene and its biological and pharmacological activities. EXCLI J. 20, 812–818. doi: 10.17179/excli2021-3714
Parthiban, R., Sivarajan, M., and Sukumar, M. (2011). Ethanol Production From Banana Peel Waste Using Saccharomyces cerevisiae. Chennai: IET. doi: 10.1049/cp.2011.0357
Penella, C., and Calatayud, A. (2018). Pepper crop under climate change: grafting as an environmental friendly strategy. Climate Resilient Agri. 2018, 129–155. doi: 10.5772/intechopen.72361
Pereira, D. T. V., Tarone, A. G., Cazarin, C., Gerardo, C. C., Barbero, F., and Martínez, J. (2019). Pressurized liquid extraction of bioactive compounds from grape marc. J. Food Eng. 240, 105–113. doi: 10.1016/j.jfoodeng.2018.07.019
Peterson, L. (2008). Currently available antimicrobial agents and their potential for use as monotherapy. Clin. Microbiol. Infect. 14, 30–45. doi: 10.1111/j.1469-0691.2008.02125.x
Pfaller, M. A., Sheehan, D. J., and Rex, J. H. (2004). Determination of fungicidal activities against yeasts and molds: lessons learned from bactericidal testing and the need for standardization. Clin. Microbiol. Rev. 17, 268–280. doi: 10.1128/CMR.17.2.268-280.2004
Philip, S. (2017). Diseases of Poverty and the 10/90 Gap Fighting the Diseases of Poverty. (London: Routledge), 126–140. Available online at: https://www.who.int/intellectualproperty/submissions/InternationalPolicyNetwork.pdf (accessed April 8, 2020).
Plaza, L., Sánchez-Moreno, C., De Ancos, B., Elez-Martínez, P., Martín-Belloso, O., and Cano, M. P. (2011). Carotenoid and flavanone content during refrigerated storage of orange juice processed by high-pressure, pulsed electric fields and low pasteurization. LWT Food Sci. Technol. 44, 834–839. doi: 10.1016/j.lwt.2010.12.013
Prabhakar, V., and Singh, S. (2019). Preliminary assessment for the potential of Citrus limetta peel waste for bioethanol production under indian conditions. Int. J. Food Sci. 7, 16–18. Available online at: https://ijari.org/assets/papers/7/1/IJARI-CV-19-03-102.pdf
Prakash, A., Mathur, K., Vishwakarma, A., and Mishra, B. (2013). Comparative Assay of Antioxidant and Antibacterial Properties of Indian Culinary Seasonal Fruit Peel Extracts Obtained from Vellore, Tamilnadu. Available online at: https://www.researchgate.net/publication/247779038 (accessed August 7, 2020).
Preedy, V., and Watson, R. (2019). Flour and Breads and Their Fortification in Health and Disease Prevention. Cambridge, MA: Academic Press. Available online at: https://www.sciencedirect.com/book/9780128146392 (accessed August 8, 2020).
Puértolas, E., Luengo, E., Álvarez., I, and Raso, J. (2012). Improving mass transfer to soften tissues by pulsed electric fields: fundamentals and applications. Annu. Rev. Food Sci. Technol. 3, 263–282. doi: 10.1146/annurev-food-022811-101208
Puri, M., Sharma, D., and Barrow, C. J. (2012). Enzyme-assisted extraction of bioactives from plants. Trends Biotechnol. 30, 37–44. doi: 10.1016/j.tibtech.2011.06.014
Qureshi, K. A., Imtiaz, M., Parvez, A., Rai, P. K., Jaremko, M., Emwas, A. H., et al. (2022). In vitro and in silico approaches for the evaluation of antimicrobial activity, time-kill kinetics, and anti-biofilm potential of thymoquinone (2-Methyl-5-propan-2-ylcyclohexa-2, 5-diene-1, 4-dione) against selected human pathogens. Antibiotics 11, 79. doi: 10.3390/antibiotics11010079
Rabin, N., Zheng, Y., Opoku-Temeng, C., Du, Y., Bonsu, E., and Sintim, H. O. (2015). Agents that inhibit bacterial biofilm formation. Future Med. Chem. 7, 647–671. doi: 10.4155/fmc.15.7
Radha, M. H., and Laxmipriya, N. P. (2015). Evaluation of biological properties and clinical effectiveness of Aloe vera: a systematic review. J. Trad. Complement. Med. 5, 21–26. doi: 10.1016/j.jtcme.2014.10.006
Rajha, H. N., Abi-Khattar, A. M., El Kantar, S., Boussetta, N., Lebovka, N., Maroun, R. G., et al. (2019). Comparison of aqueous extraction efficiency and biological activities of polyphenols from pomegranate peels assisted by infrared, ultrasound, pulsed electric fields and high-voltage electrical discharges. Innov. Food Sci. Emerg. Technol. 58, 102212. doi: 10.1016/j.ifset.2019.102212
Redfern, J., Kinninmonth, M., Burdass, D., and Verran, J. (2014). Using soxhlet ethanol extraction to produce and test plant material (essential oils) for their antimicrobial properties. J. Microbiol. Biol. Educ. 15, 45–46. doi: 10.1128/jmbe.v15i1.656
Reilly, G., Reilly, C., Smith, E., and Baker-Austin, C. (2011). Vibrio alginolyticus-associated wound infection acquired in British waters, Guernsey. Eurosurveillance 16, 19994. doi: 10.2807/ese.16.42.19994-en
Reygaert, W. C. (2014). The antimicrobial possibilities of green tea. Front. Microbiol. 5, 434. doi: 10.3389/fmicb.2014.00434
Reygaert, W. C. (2018). An overview of the antimicrobial resistance mechanisms of bacteria. AIMS Microbiol. 4, 482. doi: 10.3934/microbiol.2018.3.482
Roaa, M. H. (2020). A review article: the importance of the major groups of plants secondary metabolism phenols, alkaloids, and terpenes. Int. J. Res. Appl. Sci. Biotechnol. 7, 354–358. doi: 10.31033/ijrasb.7.5.47
Roden, M., Zaoutis, T. E., Buchanan, W. L., Christine, C., Chu, J. H., Kontoyiannis, P. D., et al. (2005). Epidemiology and outcome of zygomycosis: a review of 929 reported cases. Clin. Infect. Dis. 41, 634–653. doi: 10.1086/432579
Rodríguez, A., Andrés, V. S., Cervera, M., Zacarías, L., Palou, L., and López, M. M. (2011). The monoterpene limonene in orange peels attracts pests and microorganisms. Plant Signal. Behav. 6, 1820–1823. doi: 10.4161/psb.6.11.16980
Rossiter, S. E., Fletcher, M. H., and Wuest, W. M. (2017). Natural products as platforms to overcome antibiotic resistance. Chem Rev. 117, 12415–12474. doi: 10.1021/acs.chemrev.7b00283
Roy, J., Shakleya, D. M., Callery, P., and Thomas, J. G. (2006). Chemical constituents and antimicrobial activity of a traditional herbal medicine containing garlic and black cumin. Afr. J. Tradit. Complement. Altern. Med. 3, 1–7. doi: 10.4314/ajtcam.v3i2.31151
Russo, E. B., and Marcu, J. (2017). Cannabis pharmacology: the usual suspects and a few promising leads. Adv. Pharm. J. 80, 67–134. doi: 10.1016/bs.apha.2017.03.004
Sagar, S., Gehring, C., and Minneman, K. P. (2012). “Methods to isolate and identify new plant signaling peptides,” in Plant Signaling Peptides, eds. H. R. Irving and C. Gehring (Berlin; Heidelberg: Springer), 217–239. doi: 10.1007/978-3-642-27603-3_12
Sahle, T., and Okbatinsae, G. (2017). Phytochemical investigation and antimicrobial activity of the fruit extract of Solanum incanum grown in Eritrea. Ornament. Med. Plants 1, 15–25. Available online at: http://www.iaees.org/publications/journals/omp/articles/2017-1(1)/antimicrobial-activity-of-fruit-extract-of-Solanum-incanum.pdf
Saini, A., Panesar, P. S., and Bera, M. B. (2019). Comparative study on the extraction and quantification of polyphenols from citrus peels using maceration and ultrasonic technique. Curr. Res. Nutr. Food Sci. 7, 678. doi: 10.12944/CRNFSJ.7.3.08
Salas-Oropeza, J., Jimenez-Estrada, M., Perez-Torres, A., Jarquin-Yañez, K., and Canales-Martinez, M. M. (2021). Wound healing activity of α-pinene and α-phellandrene. Molecules 26, 2488. doi: 10.3390/molecules26092488
Saleem, M., and Saeed, M. (2020). Potential application of waste fruit peels (orange, yellow lemon and banana) as wide range natural antimicrobial agent. J. King Saud Univ. Sci. 32, 805–810. doi: 10.1016/j.jksus.2019.02.013
Sa-Nguanpuag, K., Kanlayanarat, S., Srilaong, V., Tanprasert, K., and Techavuthiporn, C. (2011). Ginger (Zingiber officinale) oil as an antimicrobial agent for minimally processed produce: a case study in shredded green papaya. Int. J. Agric. Biol. 13, 895–901. Available online at: https://www.researchgate.net/publication/263618928
Santos, P. H., Kammers, J. C., Silva, A. P., Oliveira, J. V., and Hense, H. (2021). Antioxidant and antibacterial compounds from feijoa leaf extracts obtained by pressurized liquid extraction and supercritical fluid extraction. Food Chem. 344, 128620. doi: 10.1016/j.foodchem.2020.128620
Santoyo, S., Lloria, R., Jaime, L., Ibanez, E., Senorans, F. J., and Reglero, G. (2006). Supercritical fluid extraction of antioxidant and antimicrobial compounds from Laurus nobilis L. chemical and functional characterization. Eur. Food Res. Technol. 222, 565–571. doi: 10.1007/s00217-005-0027-9
Sanver, D., Murray, B. S., Sadeghpour, A., Rappolt, M., and Nelson, A. L. (2016). Experimental modeling of flavonoid–biomembrane interactions. Langmuir 32, 13234–13243. doi: 10.1021/acs.langmuir.6b02219
Sasidharan, S., Chen, Y., Saravanan, D., Sundram, K. M., and Latha, L. Y. (2011). Extraction, isolation and characterization of bioactive compounds from plants' extracts. Afr. J. Tradit. Complement. Altern. Med. 8, 60483. doi: 10.4314/ajtcam.v8i1.60483
Shabnam, J., Rauf, A., Khurram, S., Shaista, N., Salman, S., and Yasar, S. (2013). Chemical constituents, antimicrobial and antioxidant activity of essential oil of Citrus limetta var. Mitha (sweet lime) peel in Pakistan. Afr. J. Microbiol. Res. 7, 3071–3077.
Shahnazari, S., Namolovan, A., Mogridge, J., Kim, P. K., and Brumell, J. H. (2011). Bacterial toxins can inhibit host cell autophagy through cAMP generation. Autophagy 7, 957–965. doi: 10.4161/auto.7.9.16435
Shakya, A., Luitel, B., Kumari, P., Dahal, P. R., and Chaudhary, R. (2019). Comparative study of antibacterial activity of juice and peel extract of citrus fruits. J. Gen. Microbiol. 6, 82–88. doi: 10.3126/tujm.v6i0.26589
Siddiqui, S. A., Bahmid, N. A., Taha, A., Khalifa, I., Khan, S., Rostamabadi, H., et al. (2022). Recent advances in food applications of phenolic-loaded micro/nanodelivery systems. Crit. Rev. Food Sci. Nutr. 2022, 1–21. doi: 10.1080/10408398.2022.2056870
Silva, A. C., Lopes, P. M., Azevedo, M., Cristina, D., Costa, M., Alviano, C. S., et al. (2012). Biological activities of a-pinene and β-pinene enantiomers. Molecules 17, 6305–6316. doi: 10.3390/molecules17066305
Silva, N., and Fernandes, A. (2010). Biological properties of medicinal plants: a review of their antimicrobial activity. J. Venom. Anim. Toxins Incl. Trop. Dis. 16, 6. doi: 10.1590/S1678-91992010000300006
Silva, S., Costa, E. M., Mendes, M., Morais, R. M., Calhau, C., and Pintado, M. M. (2016). Antimicrobial, antiadhesive and antibiofilm activity of an ethanolic, anthocyanin-rich blueberry extract purified by solid phase extraction. J. Appl. Microbiol. 121, 693–703. doi: 10.1111/jam.13215
Silvestri, B., Vitiello, G., Luciani, G., Pezzella, A., and Ischia, M. D. (2017). Probing the eumelanin–silica interface in chemically engineered bulk hybrid nanoparticles for targeted subcellular antioxidant protection. ACS Appl. Mater. Interfaces 9, 37615–37622. doi: 10.1021/acsami.7b11839
Singh, G., Verma, A., and Kumar, V. (2016). Catalytic properties, functional attributes and industrial applications of β-glucosidases. Biotechnol. J. 6, 3. doi: 10.1007/s13205-015-0328-z
Sizar, O., and Unakal, C. (2020). Gram Positive Bacteria. StatPearls. Available online at: https://www.ncbi.nlm.nih.gov/books/NBK470553/ (accessed October 4, 2021).
Sneha, K., Narayanankutty, A., Job, J. T., Olatunji, O. J., Alfarhan, A., Famurewa, A. C., et al. (2022). Antimicrobial and larvicidal activities of different ocimum essential oils extracted by ultrasound-assisted hydrodistillation. Molecules 27, 1456. doi: 10.3390/molecules27051456
Somda, M., Savadogo, A., Ouattara, C., Ouattara, A. S., and Traore, A. S. (2011). Thermotolerant and alcohol-tolerant yeasts targeted to optimize hydrolyzation from mango peel for high bioethanol production. Asian J. Biotechnol. 3, 77–83. doi: 10.3923/ajbkr.2011.77.83
Spreen, T. (2010). The world citrus industry. Soils Plant Growth Crop Prod. 3, 249–269. Available online at: http://www.eolss.net/sample-chapters/c10/e1-05a-39-00.pdf
Sreedharan, S., Gothe, A., Aier, K., Shivasharanappa, K., Kumar, K. P., and Patil, S. J. (2019). Research article bioactive molecules and antimicrobial studies of indian traditional medicinal plant Rhus semialata seeds. Res. J. Med. Plants. 13, 10–17. doi: 10.3923/rjmp.2019.10.17
Stiefel, S., Di Marino, D., Eggert, A., Leitner, W., Jupke, A., and Wessling, M. (2017). Liquid/liquid extraction of biomass-derived lignin from lignocellulosic pretreatments. Green Chem. 19, 93–97. doi: 10.1039/C6GC02270G
Sukalingam, K., Ganesan, K., and Xu, B. (2017). Trianthema portulacastrum L. (giant pigweed): phytochemistry and pharmacological properties. Phytochem. Rev. 16, 461–478. doi: 10.1007/s11101-017-9493-5
Sukalingam, K., Ganesan, K., and Xu, B. (2018). Protective effect of aqueous extract from the leaves of Justicia tranquebariesis against thioacetamide-induced oxidative stress and hepatic fibrosis in rats. Antioxidants 7, 78. doi: 10.3390/antiox7070078
Tang, S. S., Prodhan, Z. H., Biswas, S. K., Le, C. F., and Sekaran, S. D. (2018). Antimicrobial peptides from different plant sources: isolation, characterisation, and purification. Phytochem 154, 94–105. doi: 10.1016/j.phytochem.2018.07.002
Tano, K., Nervy, R., and Koussemoun, M. (2008). The effects of different storage temperatures on the quality of fresh bell pepper (Capsicum annum L.). J. Agric. Food Chem. 3, 157–162. Available online at: https://medwelljournals.com/abstract/?doi=aj.2008.157.162
Tariq, S., Wani, S., Rasool, W., Shafi, K., Bhat, M. A., Prabhakar, A., et al. (2019). A comprehensive review of the antibacterial, antifungal and antiviral potential of essential oils and their chemical constituents against drug-resistant microbial pathogens. Microb. Pathog. 134, 103580. doi: 10.1016/j.micpath.2019.103580
Tellez, G., Jaeger, L., Dean, C., William, J. D., and Hargis, B. M. (1993). Effect of prolonged administration of dietary capsaicin on Salmonella enteritidis infection in leghorn chicks. Avian Dis. 7, 143–148. doi: 10.2307/1591467
Thumar, J. T., Dhulia, K., and Singh, S. P. (2010). Isolation and partial purification of an antimicrobial agent from halotolerant alkaliphilic Streptomyces aburaviensis strain Kut-8. World J. Microbiol. Biotechnol. 26, 2081–2087. doi: 10.1007/s11274-010-0394-7
Trabelsi, D., Aydi, A., Zibetti, A. W., Cricchio, V., Langa, E., Abderrabba, M., et al. (2016). Supercritical extraction from Citrus aurantium amara peels using CO2 with ethanol as co-solvent. J. Supercrit. Fluids. 117, 33–39. doi: 10.1016/j.supflu.2016.07.003
Trease, G. E., and Evans, W. C. (1989). Textbook of Pharmacognosy, 13th Edn. London; Toronto, ON; Tokyo: Bailiere Tindall, 200–1.
Tsuchiya, H., and Iinuma, M. (2000). Reduction of membrane fluidity by antibacterial sophoraflavanone G isolated from Sophora exigua. Phytomedicine 7, 161–165. doi: 10.1016/S0944-7113(00)80089-6
Turnidge, J. D., Kotsanas, D., Munckhof, W., Nimmo, G. R., Coombs, G. W., Murray, R. J., et al. (2009). Staphylococcus aureus bacteraemia: a major cause of mortality in Australia and New Zealand. Med. J. Aust. 191, 368–373. doi: 10.5694/j.1326-5377.2009.tb02841.x
Tyc, O., Song, C., Dickschat, J. S., Vos, M., and Garbeva, P. (2017). The ecological role of volatile and soluble secondary metabolites produced by soil bacteria. Trends Microbiol. 25, 280–292. doi: 10.1016/j.tim.2016.12.002
Ulasli, M., Gurses, S. A., Bayraktar, R., Oztuzcu, S., Igci, M., Igci, Y. Z., et al. (2014). The effects of Nigella sativa (Ns), Anthemis hyalina (Ah) and Citrus sinensis (Cs) extracts on the replication of coronavirus and the expression of TRP genes family. Mol. Biol. Rep. 41, 1703–1711. doi: 10.1007/s11033-014-3019-7
Van Acker, H., and Coenye, T. (2017). The role of reactive oxygen species in antibiotic-mediated killing of bacteria. Trends Microbiol. 25, 456–466. doi: 10.1016/j.tim.2016.12.008
van Eijk, E., Wittekoek, B., Kuijper, E. J., and Smits, W. K. (2017). DNA replication proteins as potential targets for antimicrobials in drug-resistant bacterial pathogens. J. Antimicrob. Chemother. 72, 1275–1284. doi: 10.1093/jac/dkw548
Vankar, P. (2004). Essential oils and fragrances from natural sources. Resonance 9, 30–41. doi: 10.1007/BF02834854
Vanlalveni, C., Lallianrawna, S., Biswas, A., Changmai, B., and Rokhum, S. L. (2021). Green synthesis of silver nanoparticles using plant extracts and their antimicrobial activities: a review of recent literature. RSC Adv. 11, 2804–2837. doi: 10.1039/D0RA09941D
Vaou, N., Stavropoulou, E., Voidarou, C., Tsakris, Z., Rozos, G., Tsigalou, C., et al. (2022). Interactions between medical plant-derived bioactive compounds: focus on antimicrobial combination effects. Antibiotics 11, 1014. doi: 10.3390/antibiotics11081014
Vijayakumar, R., Sandle, T., Al-Aboody, M., Mickymaray, S., Premanathan, M., and Alsagaby, S. A. (2018). Distribution of biocide resistant genes and biocides susceptibility in multidrug-resistant Klebsiella pneumoniae, Pseudomonas aeruginosa and Acinetobacter baumannii—a first report from the Kingdom of Saudi Arabia. J. Infect. Public Health. 11, 812–816. doi: 10.1016/j.jiph.2018.05.011
Vijayakumar, S., Divya, M., Vaseeharan, B., Shreema, K., Ranjan, S., and Dasgupta, N. (2021). Biological compound capping of silver nanoparticle with the seed extracts of blackcumin (Nigella sativa): a potential antibacterial, antidiabetic, anti-inflammatory, and antioxidant. J. Inorg. Organomet Polym. Mater. 31, 624–635. doi: 10.1007/s10904-020-01713-4
Voon, H. C., Bhat, R., and Rusul, G. (2012). Flower extracts and their essential oils as potential antimicrobial agents for food uses and pharmaceutical applications. Compr. Rev. Food Sci. Food Saf. 11, 34–55. doi: 10.1111/j.1541-4337.2011.00169.x
Wallis, T. E. (1989). Text Book of Pharmacognosy. (Delhi: CBS Publishers and Distributors), 356–549.
Wang, H., Liu, Y. M., Qi, Z. M., Wang, S. Y., Liu, S. X., Li, X., et al. (2013). An overview on natural polysaccharides with antioxidant properties. Curr. Med. Chem. 20, 2899–2913. doi: 10.2174/0929867311320230006
Wang, L., and Weller, C. (2006). Recent advances in extraction of nutraceuticals from plants. Trends Food Sci. Technol. 17, 300–312. doi: 10.1016/j.tifs.2005.12.004
Wang, S., Deng, H., Wang, Y., Rui, W., Zhao, P., Yong, Q., et al. (2021). Antimicrobial activity and action mechanism of thymoquinone against Bacillus cereus and its spores. Foods 10, 3048. doi: 10.3390/foods10123048
Wiegand, I., Hilpert, K., and Hancock, R. E. (2008). Agar and broth dilution methods to determine the minimal inhibitory concentration (MIC) of antimicrobial substances. Nat. Protoc. 3, 163–175. doi: 10.1038/nprot.2007.521
Wu, C., Wang, F., Liu, J., Zou, Y., and Chen, X. (2015). A comparison of volatile fractions obtained from Lonicera macranthoides via different extraction processes: ultrasound, microwave, Soxhlet extraction, hydrodistillation, and cold maceration. Integr.Med. Res. 4, 171–177. doi: 10.1016/j.imr.2015.06.001
Wu, G., Yang, Q., Long, M., Guo, L., Li, B., Meng, Y., et al. (2015). Evaluation of agar dilution and broth microdilution methods to determine the disinfectant susceptibility. J. Antibiot. 68, 661–665. doi: 10.1038/ja.2015.51
Wu, Y., Mittal, G. S., and Griffiths, M. W. (2005). Effect of pulsed electric field on the inactivation of microorganisms in grape juices with and without antimicrobials. Biosyst. Engin. 90, 1–7. doi: 10.1016/j.biosystemseng.2004.07.012
Xie, Y., Yang, W., Tang, F., Chen, X., and Ren, L. (2015). Antibacterial activities of flavonoids: structure-activity relationship and mechanism. Curr. Med. Chem. 22, 132–149. doi: 10.2174/0929867321666140916113443
Xu, H., Liu, B., Xiao, Z., Gao, J., Akhtar, J., Xiang, B., et al. (2021). Computational and experimental studies reveal that thymoquinone blocks the entry of coronaviruses into in vitro cells. J. Infect. Dis. 10, 483–494. doi: 10.1007/s40121-021-00400-2
Yimer, E. M., Tuem, K. B., Karim, A., Ur-Rehman, N., and Anwar, F. (2019). Nigella sativa L. (black cumin): a promising natural remedy for wide range of illnesses. Evid Based Complement. Altern. Med. 2019, 1528635. doi: 10.1155/2019/1528635
Yousefi, M., Rahimi-Nasrabadi, M., Pourmortazavi, S., Jesionowski, T., Ehrlich, H., Mirsadeghi, S., et al. (2019). Supercritical fluid extraction of essential oils. Trends Anal. Chem. 118, 182–193. doi: 10.1016/j.trac.2019.05.038
Youssef, F. S., Alshammari, E., and Ashour, M. (2021). Bioactive alkaloids from Genus Aspergillus: mechanistic interpretation of their antimicrobial and potential SARS-CoV-2 inhibitory activity using molecular modelling. Int. J. Mol. Sci. 22, 1866. doi: 10.3390/ijms22041866
Zhang, F., Chen, B., Xiao, S., and Shou-zhuo, Y. (2005). Optimization and comparison of different extraction techniques for sanguinarine and chelerythrine in fruits of Macleaya cordata (Willd) R. Br. Sep. Purif. Technol. 42, 283–290. doi: 10.1016/j.seppur.2004.09.002
Zhang, F., Yang, Y., Su, P., and Guo, Z. (2009). Microwave-assisted extraction of rutin and quercetin from the stalks of Euonymus alatus (Thunb.) Sieb. Phytochem. Anal. 20, 33–37. doi: 10.1002/pca.1088
Zhang, L., Feng, X. Z., Xiao, Z. Q., Fan, G. R., Chen, S. X., Liao, S. L., et al. (2021). Design, synthesis, antibacterial, antifungal and anticancer evaluations of novel β-pinene quaternary ammonium salts. Int. J. Mol. Sci. 22, 11299. doi: 10.3390/ijms222011299
Zhang, M., Jiang, L., Li, F., and Wang, B. (2019). Simultaneous dermatophytosis and keratomycosis caused by Trichophyton interdigitale infection: a case report and literature review. BMC Infect. Dis. 19, 1–8. doi: 10.1186/s12879-019-4612-0
Zhao, X., Xin, G., Zhao, L., Xiao, Z., and Xue, B. (2018). “Composition of the essential oil of Aristolochia manshurientsis Kom,” in IOP Conference Series: Materials Science and Engineering, vol. 322 (Bristol: IOP Publishing), 22007. doi: 10.1088/1757-899X/322/2/022007
Zheng, D., Huang, C., Huang, H., Zhao, Y., Khan, M. R. U., Zhao, H., et al. (2020). Antibacterial mechanism of curcumin: a review. Chem. Biodivers. 17, e2000171. doi: 10.1002/cbdv.202000171
Zhou, L. J., Li, F. R., Huang, L. J., Yang, Z. R., Yuan, S., and Bai, L. H. (2016). Antifungal activity of eucalyptus oil against rice blast fungi and the possible mechanism of gene expression pattern. Molecules 21, 621. doi: 10.3390/molecules21050621
Zielińska, S., Wójciak-Kosior, M., and Dziagwa-Becker, M. (2019). The activity of isoquinoline alkaloids and extracts from Chelidonium majus against pathogenic bacteria and Candida sp. Toxins 11, 406. doi: 10.3390/toxins11070406
Zizovic, I. (2020). Supercritical fluid applications in the design of novel antimicrobial materials. Molecules 25, 2491. doi: 10.3390/molecules25112491
Zou, Z., Chang, H., Li, H., and Wang, S. (2017). Induction of reactive oxygen species: an emerging approach for cancer therapy. Apoptosis 22, 1321–1335. doi: 10.1007/s10495-017-1424-9
Keywords: antimicrobial agent, antimicrobial mechanism, solid waste management, extraction techniques, Capsicum, Nigella sativa, Musa paradisiaca L., Citrus limetta
Citation: Bhatti SA, Hussain MH, Mohsin MZ, Mohsin A, Zaman WQ, Guo M, Iqbal MW, Siddiqui SA, Ibrahim SA, Ur-Rehman S and Korma SA (2022) Evaluation of the antimicrobial effects of Capsicum, Nigella sativa, Musa paradisiaca L., and Citrus limetta: A review. Front. Sustain. Food Syst. 6:1043823. doi: 10.3389/fsufs.2022.1043823
Received: 14 September 2022; Accepted: 10 November 2022;
Published: 19 December 2022.
Edited by:
Rabia Siddique, Government College University, Faisalabad, PakistanReviewed by:
Ayyaz Ahmad, Muhammad Nawaz Sharif University of Engineering & Technology, PakistanMuhammad Adil Farooq, Khwaja Fareed University of Engineering and Information Technology (KFUEIT), Pakistan
Akhtar Ali, The University of Melbourne, Australia
Murtaza Ali, Foshan University, China
Copyright © 2022 Bhatti, Hussain, Mohsin, Mohsin, Zaman, Guo, Iqbal, Siddiqui, Ibrahim, Ur-Rehman and Korma. This is an open-access article distributed under the terms of the Creative Commons Attribution License (CC BY). The use, distribution or reproduction in other forums is permitted, provided the original author(s) and the copyright owner(s) are credited and that the original publication in this journal is cited, in accordance with accepted academic practice. No use, distribution or reproduction is permitted which does not comply with these terms.
*Correspondence: Waqas Qamar Zaman, ZHJ3YXFhcyYjeDAwMDQwO2llc2UubnVzdC5lZHUucGs=; Salam A. Ibrahim, aWJyYWgwMDEmI3gwMDA0MDtuY2F0LmVkdQ==
†These authors have contributed equally to this work