- 1Institute of Biological Sciences, Faculty of Science, Universiti Malaya, Kuala Lumpur, Malaysia
- 2Centre for Research in Biotechnology for Agriculture (CEBAR), Faculty of Science, Universiti Malaya, Kuala Lumpur, Malaysia
- 3Nutrition Technologies, Johor, Malaysia
- 4Institute of Bioproduct Development (IBD), Universiti Teknologi Malaysia (UTM), Skudai, Malaysia
- 5City of Scientific Research and Technology Application, New Borg El Arab, Egypt
Southeast Asia is a fertile land with a warm and humid climate which tends to accommodate various food crops. The development and advancement of the agricultural sector not only allows the countries in the region to feed the increasing population, but are also able to boost the nation's economy through exportation of the crops. Some of the well-known and economically-significant plant commodities found in the region include rice, oil palm, rubber, coconut, banana, sugarcane, pineapple, black pepper, maize, cocoa, durian, and jackfruit. Due to the high production of crops, Southeast Asia is able to stand among the top world producers of these commodities. Nevertheless, the widespread of pathogenic microorganisms has posed a serious threat to the industry over the years; with hundreds of millions of money wasted and total yield being lost due to the devastating diseases associated with each type of the plants. A lot of attention and effort have been continuously devoted to find effective plant management strategies to combat plant diseases, starting from traditional physical and chemical methods to the increasing discoveries on biological approaches made in recent decades. Due to the challenges and limitations faced by conventional approaches and the rising awareness toward the environment, more work has been focused on establishing the application of beneficial microorganisms to tackle plant diseases through direct mechanisms. Thus, by bringing the common plant commodities in Southeast Asia, their associated diseases and various physical, chemical and biological control measures together, this review aims to provide clearer insights and practical information to those who seek to limit the damages caused by plant diseases.
Introduction
According to the United Nations population forecast, the world's population is projected to reach 9.7 billion by 2050 (UN DESA, 2017). This rapid rise in global population during this climate change era brings about many challenges, particularly in relation to ensuring access to nutrient-rich foods and achieving food security. This may be more challenging to less-developed nations such as those in the Africa, Southeast Asia, and West Asia (Prosekov and Ivanova, 2018). In response, much attention has been given to the expansion and intensification of the agricultural sector. For example, it has been reported that as much as 25%−40% of the land has been devoted to crop cultivation in the Southeast Asian countries in order to cater for the demand of its people, as well as for economic growth through exportation of these valuable commodities (De Koninck and Rousseau, 2013).
Rice is the staple food for half the global population (Huang et al., 2020) with Southeast Asians accounted for 22% of this statistic, thereby making it as the dominant crop that is planted across the nations (Wailes and Chavez, 2012). The combined net rice export has risen from 15.5 million tons (in 2011) to 21.5 million tons (in 2021), with an annual growth of 3.3%. Overall, Southeast Asia has contributed to 53.3% of the total global rice volume over the last decades (Wailes and Chavez, 2012). Other than rice, Southeast Asia is also noted for various cash crop productions, of which the most notable ones include oil palm, rubber, sugarcane, banana, coconut, black pepper as well as cocoa. In Indonesia and Malaysia, the oil palm plantations covered nearly 16 million hectares of land while around 1 million hectare of rubber estates have been established in areas across Thailand, Laos, Vietnam, Cambodia, and Myanmar (Kenney-Lazar and Ishikawa, 2019).
Despite the growing trend in these crop plantations, the industry is constantly suffering from a wide range of issues where disease infection is one of the top hurdles. Since crops and plants are biological organisms, they are highly susceptible to stresses, damage, variations in the environment as well as invasions by other organisms. Diseases affecting plants have been the major reason behind the production and economic losses in agriculture which further threaten food security (Trebicki and Finlay, 2019; Fones et al., 2020). These diseases are often caused by pests and various microorganisms such as fungi, bacteria, and viruses which attack plant organs and eventually lead to plant death. In its worst condition especially during severe epidemics, the entire plantations can be easily wiped out which then negatively affects the industry as well as the whole nation.
Back then during the early agricultural era, plant diseases were seen as the God's wrath and apparent measures and management of plant diseases were very limited. Therefore, in the past when there were commonly lower yields and lack of food reserves, people were likely to experience disastrous effects as a result from food shortage such as the Bengal Famine in 1943 which was initiated by the rice brown spot. Although there has been increasing scientific contributions and advancements attempting to address the problem of plant diseases today, there is still 20%−30% of yield being lost every year which may reveal the insufficient knowledge on the causes and mechanisms behind the epidemic development, thus lacking appropriate and effective approaches to manage and eliminate the diseases (He et al., 2016). Additionally, chemical controls are known to have harmful impacts on human health and environment, thus it should not be seen as a long-term solution.
It is extremely crucial to identify and understand the causal agents and development of plant diseases in order to evaluate their economic impacts and minimize losses. The backgrounds of economically important crops in Southeast Asian countries are studied and disease management has been focused on using biological agents other than conventional control measures. Therefore, the aims of this paper are (i) to discuss some common diseases that affect important plant commodities in Southeast Asia and (ii) to provide an overall review on the treatments, covering both the traditional and biological aspects.
Plant commodities in Southeast Asia and association between climate change and diseases
Rice
The cultivation of rice or Oryza sativa was believed to begin around 8,000 to 6,000 BC and at a later time, rice field system was established in the lower and middle Yangtze River Valley in China where from there, the domestication spread to other regions (Cobo et al., 2019). Due to cultural and social significance, rice has remained as the most widely harvested and important staple food among the Southeast Asians. The areas for rice plantation encompass nearly 48 million hectares which account for almost 30% of world's rice harvest (IRRI, 2018). Southeast Asia has been producing more rice than what is demanded in the region which allows the stock levels to remain high and adequate. For example, Vietnam currently produces 44 million metric tons of rice a year (Benová et al., 2021; Connor et al., 2022), thus serves as the second largest rice exporter (Ishikawa-Ishiwata and Furuya, 2022). Nevertheless, despite the high amount for export, there is also a rise in the imports due to the substantially slowing down of growth and production. Farmers and scientists have expressed their concerns on this scenario which is believed to be caused by invasions of microorganisms brought upon by five major causal agents (Table 1). Among these, rice blast disease brought upon by Magnaporthe oryzae is one of the most serious (Figure 1). The production of rice and its disease susceptibility are further worsened by climate changes such as increased salinity, for example in Vietnam, 270,000 hectares of rice plantations were destroyed by a severe salt intrusion recorded in 2016, contributing to a loss of ~$455 million. Moreover, with every 1°C rise in temperature, the Philippines is expected to face 10% decrease in the rice crop yield due to the conducive environment for fungal spread (Redfern et al., 2012).
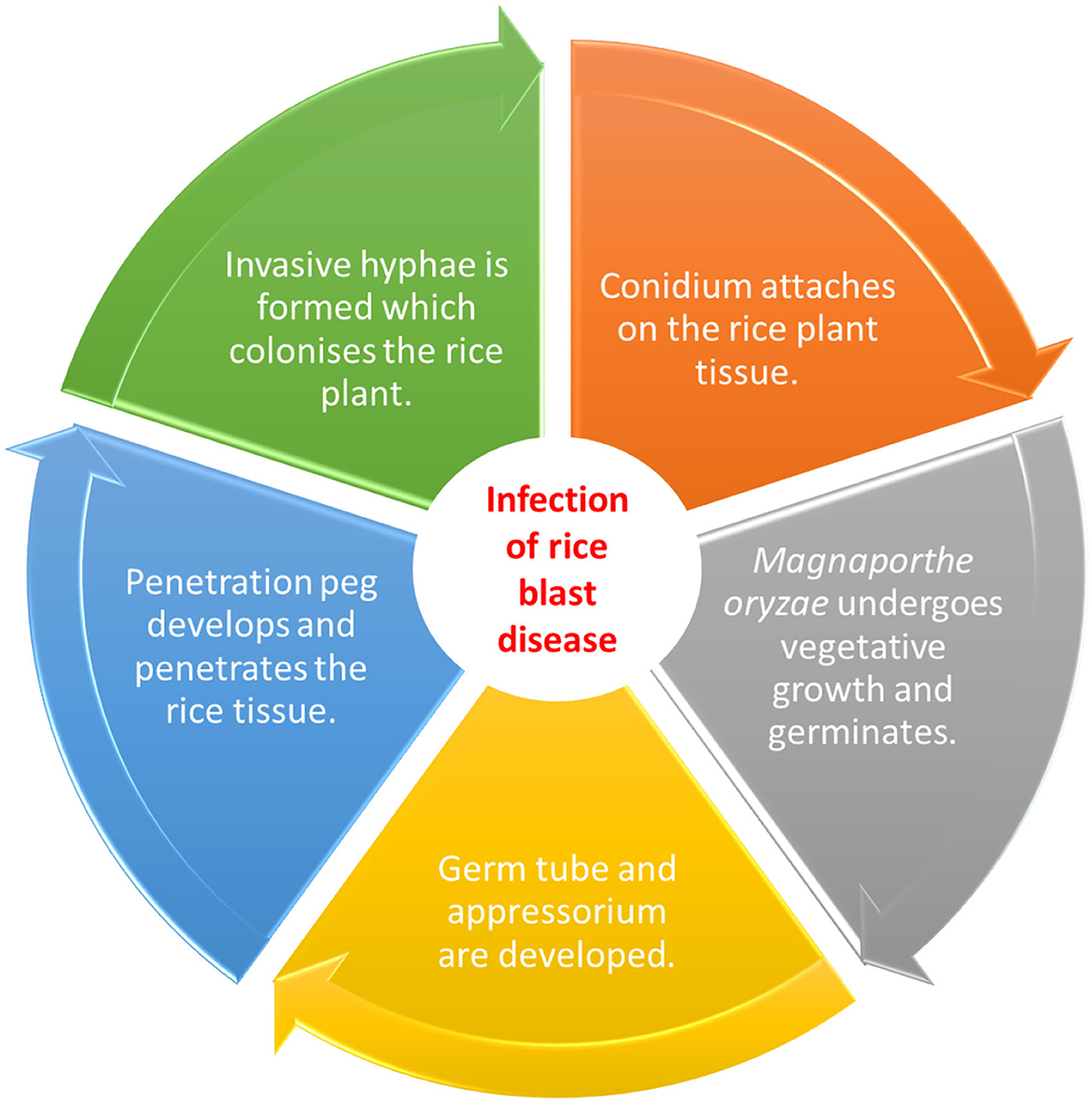
Figure 1. Invasion of Magnaporthe oryzae into rice plant, causing rice blast disease. The chart was illustrated based on the information obtained from Mentlak et al. (2012).
Oil palm
Oil palm or Elaeis guineensis was indigenous to Africa and later domesticated in Southeast Asia during the British colonial times. It is widely planted in Indonesia and Malaysia, whereby both countries contribute to ~84% of world's oil palm production (Wardhani and Rahadian, 2021). Oil palm demand and production have been on the rise due to its wide range of applications. The most frequently processed raw materials are palm oil and palm kernel oil, which are used to produce personal care and cosmetics, biofuel and cooking oil, animal feed, food, pharmaceuticals, and industrial products. In 2017 alone, the two major oil palm producers produced 36,000 and 21,000 metric tons respectively (Iskandar et al., 2018). Apart from internal consumption, Southeast Asia is also in the lead for oil palm exportation, with Indonesia and Malaysia exporting nearly 50 million metric tons in total during 2020 and 2021 (FAO, 2022). Nonetheless, the oil palm plantation is also prone to pathogenic attack whereby basal stem rot represents the highest threat potentially affecting more than 60 million mature oil palm (Siddiqui et al., 2021).
Basal stem rot
Basal stem rot or BSR disease has emerged as the most damaging palm disease in Southeast Asia where almost RM1.5 billion of losses were reported annually (Hushiarian et al., 2013). It is well-known to be caused by the pathogenic basidiomycete, Ganoderma boninense which caused up to 75% yield loss, especially in coastal areas and acidic soil (Maluin et al., 2020). The transmission of BSR is usually through root infection between palm trees as well as dispersion of fungal spores in the air. The infection by G. boninense can occur throughout the tree's life cycle with the earliest symptoms being detected on seedlings less than a year in nursery. Older trees are more prone to the attack and fungal infection can occur repeatedly (Hushiarian et al., 2013). BSR is characterized by stem and root decay where the basidiocarps of fungus emerge. Light brown lesions can be discovered on stem and trunk with visible hyphal growth and narrow, dark bands of tissues between lesions. The occurrence of BSR may rise dramatically with large decreases in suitable climate for palm growing which is likely to result in unsustainability of the oil palm industry after 2050 (Paterson, 2019). This is attributed by the ability of G. boninense to better adapt to the climate change as compared to the oil palm by strain selection that is more virulent. Lower crop yields will result from increased crop stress following excessive precipitation, and these diminished resistance to fungal invasion in oil palm will further exacerbate the spread of disease. In Malaysia, a substantial negative correlation between yearly average temperature, sea level rise and oil palm productivity is discovered whereby temperature increases of 1–4°C may result in 10%−41% decline in oil palm yield (Murphy et al., 2021).
Rubber
Hevea brasiliensis or the rubber tree is an economically significant plant commodity in the Southeast Asian countries due to its diversified advantages in products manufacturing. Native to the Amazon basin, rubber plantations have now been extensively established in the tropical regions, with Southeast Asia contributing to 97% of global natural rubber output with Thailand leading the pack, followed by Indonesia, Vietnam, and Malaysia (Mazlan et al., 2019). Serving as raw material for products ranging from gloves to car tire, Thailand has produced 4.37 million metric tons of natural rubber in 2020 while Indonesia, in the second lead producing 3.04 million metric tons (Wahab et al., 2022). Meanwhile, in Malaysia, 10.8% decline was observed in the production of natural rubber in September 2021 (41,180 tons) compared to the same month in 2020 (46,187 tons) in which disease infections may be a major factor (DOSM, 2021). The three major diseases affecting rubber plantation are depicted in Table 2 which indicated that disease occurrence and rubber yield are influenced by the rising temperature, drought, and heavy rainfall. Particularly, Phytophthora-related diseases are relatively common when there is too much of rain. Besides that, since latex harvesting is a major part in planting rubber trees, any factor that limits the water uptake by the tree such as drought and temperature rise will decrease the latex generation capability, thus contributing to serious economic losses (Pinizzotto et al., 2021).
Sugarcane
Sugarcane or Saccharum officinarum is a perennial grass that originated from New Guinea where the cultivation started 1,000 years ago. It was believed to have been hybridized with the wild type from India and China to produce the varieties that we have today (Wang et al., 2022). Sugarcane serves as one of the major sources of global sugar, with Thailand, Indonesia, and Vietnam leading the way in Southeast Asia (Voora et al., 2020). Nevertheless, despite the high output, the sugarcane industry in the region is constantly working to sustain the yield in the backdrop of several stresses, climatic changes and disease outbreaks (Solomon and Li, 2016). Climatic factors impact the sugarcane yield, where the increase of peak temperature demonstrated a detrimental effect on the productivity but its output is positively associated with the amount of rainfall (Guo et al., 2021). In response to global warming and drought threats, in Thailand, efforts are being taken to develop drought-resistant sugarcane cultivars that can withstand high water deficits (Khonghintaisong et al., 2021). Meanwhile, new high-yielding sugarcane varieties are being developed in Indonesia as part of the nation's crop improvement initiative (Riajaya, 2021; Riajaya et al., 2022).
Red rot
The red rot disease or also known as the cancer of sugarcane is among the most destructive diseases in the sugarcane industry, yielding around 18%−31% of losses in annual production. It usually results from fungal infection by Colletotrichum falcatum (sexual form: Glomerella tucumanensis, which is important for the fungus survival on rotting leaves and emergence of more aggressive pathogenic strains that cause periodic epidemics). The attack can occur as early as when germination begins, thus causing germination failure and death of germinated seedlings (Sharma et al., 2017). When the plant is infected, its sucrose content will decrease at a range of 31%−75%, depending on the levels of infection. Besides that, a decline in the cane weight (up to 83%) and in the juice extraction (between 24 and 90%) has been observed in red rot-infected sugarcanes (Viswanathan, 2021). Normally, all plant parts are subjected to the attack, especially during warm and wet conditions. The disease is easily identified by discoloration of leaves and reddening of interior tissues as well as development of interrupted red and white patches on the stem at a later stage (Huang et al., 2018).
Leaf scald
The leaf scald disease in the sugarcane industry was first reported in Taiwan in the 1980s and Guangxi, China later in 2015. Today, the disease is primarily distributed in Southeast Asia including the Philippines, Thailand, Myanmar, Vietnam, and Laos (Zhang et al., 2020). Also known as the leaf burning disease, the causal agent was identified as Gram-negative bacterium Xanthomonas albilineans which is capable of producing a gum (xanthan-like polymer) that obliterates the xylem elements, thus leading to desiccation and yellowing of the leaves (Legaz et al., 2018). Moreover, the bacteria can also produce a phytotoxin called albicidin, causing the most evident symptom of leaf scald disease through the formation of white “pencil lines” with yellow borders along the leaf veins, which subsequently results in tissue necrosis (Pieretti et al., 2015). Disease development often occurs during long periods of environmental stresses such as drought, waterlogging, and low temperature, which result in about 15%−20% of yield losses (Rott, 1995).
Coconut
Coconut (Cocos nucifera) plantations are widely distributed in the Southeast Asian countries, with the Philippines and Indonesia taking the lead in the world's coconut production. In 2020, the production of coconuts was 14.49 million metric tons in the Philippines while 16.82 million metric tons were recorded in Indonesia, contributing to 60% of global yield (FAO, 2022). Being a common yet economically important crop, a vast number of products can be extracted from the tree including oil, milk, sugar, and water taken from the fruit, brooms made from the branches while coconut flower can serve as a remedy for lifestyle diseases such as diabetes, cholesterol, and high blood pressure. Despite the high demand, the supply of coconuts is hampered due to various diseases affecting the coconut trees. On top of that, the plantation is severely affected by climate changes including elevated temperature and CO2 levels as well as increased precipitation. They are sensitive to heat stress particularly during pollen development as the morphology can be seriously altered, thus giving rise to a lower pollen viability. The coconut biomass is reported to have reduced by 10% at high temperature (3°C above ambient) while the cumulative effect of dryness and high temperatures resulted in 16% reduction in biomass (Hebbar et al., 2013).
Stem bleeding
Coconut trees infected by the fungus Thielaviopsis paradoxa would exhibit symptoms such as exudation of dark reddish-brown liquid from the longitudinal cracks in the bark. This fungus tends to be the main cause of most primary infections in the natural environment and is more common compared to its teleomorph stage, Ceratocystis paradoxa. Other features of infected trees may also include formation of wounds on the stem that are trickling down, upwards spread of lesions and rotting of tissues. Apart from coconut plantations, this fungal infection can also affect other crops grown in the tropical regions such as banana, pineapple, and sugarcane. Coconut stem bleeding was first reported in Sri Lanka and had nearly wiped out the PB-121 hybrid in Indonesia (Yu et al., 2012). This disease is likely to progress in a rapid manner during moist and favorable conditions (25–35°C), mainly in highly irrigated areas or after severe downpours that bring about water stagnation, nutritional imbalances, excess salinity, and plant stress (Nelson, 2005b).
Banana
The cultivation of bananas or Musa sp. is considered to have a long history in Southeast Asia and has remained the region's most economically significant crop. Among the banana varieties, Cavendish is the most commonly known cultivar which has been frequently produced for the large export market. In 2020, 90% of the Asian banana export came from the Philippines which is in the second lead after the largest global banana exporter, Ecuador (Viljoen et al., 2020). Malaysia has also become a big banana producer, with a yield of 350,493 metric tons in 2017, accounting for around a quarter of the country's total fruit production (Tumin and Shaharudin, 2019). However, since then, Malaysia's banana production has decreased and recorded only 312,968 metric tons of banana production in 2020 (FAO, 2022). Nevertheless, due to the popularity of a common snack made from the “plantain”' variety which is widely known as the “pisang goreng” or banana fritters among the Southeast Asians, the total banana consumption is significantly increasing every year despite the serious threat faced by banana farmers which is affecting the yield at the same time (Table 3). Furthermore, climate change may influence abiotic factors that increase or decrease the likelihood of a disease's emergence. The increased outbreak of the well-known black Sigatoka disease and infection development has illustrated a strong positive relationship with the temperature rise and increased leaf wetness duration, where the infection risk has gone up to 44.2% on average across the banana plantations since 1960s (Bebber, 2019).
Maize
Originally cultivated in Mexico by the native people 9,000 years ago, maize (Zea mays) is a thoroughly studied crop due to its agronomy, cytology, genetics and evolutionary history since its domestication (Strable and Scanlon, 2009). Maize is the third most important staple crop after rice and wheat and is widely farmed in Southeast Asia with Indonesia (41%) as the biggest production country, followed by the Philippines (29%), Thailand (13%) and Vietnam (12%) (Gianessi, 2014). Indonesia produced around 30 million metric tons of maize in 2018, and the country has planned to continuously increase its production by increasing the land area for maize cultivation through its maize extensification policy (Syahruddin et al., 2020). The increasing demand for maize not only comes from the changing dietary requirements in the rising populations, but is also driven by the increase in its usage as the main feed ingredient for livestock and poultry industry (Pasuquin et al., 2014). Despite the production in Southeast Asia has steadily increased every year, there is still a big disparity between yield and market demand due to disease outbreaks that are out of control. The situation is worsened by the change in climates whereby a 1.5–2°C increase of global warming, together with drought and aridity would cause 40%−80% of arable lands that are currently conducive for maize growth to disappear by 2030, thereby significantly decreasing its yield. It is projected that if the diseases can be adequately combated with proper adaptation strategies, there will be tremendous growth prospects with an average yield gain of 14% (Gianessi, 2014).
Downy mildew
The downy mildew disease is caused by the fungal-like protist plant pathogens, omycetes specifically Sclerophthora macrospora and Peronosclerospora philippinensis. The ubiquitous occurrence of the disease has been an important bottleneck for mass production of maize. Yield losses have been reported to be around 40%−60% but may reach up to 100% during favorable wet and humid conditions, with most disease distributions occurring in Indonesia, the Philippines, Cambodia, Thailand, and Laos (Sharma et al., 1993). Transmission of this disease occurs through the dispersal of airborne conidia. Higher risk of infection would occur at high temperatures, where larger-sized conidia are produced, but at lower temperatures (between 10 and 16°C), the rate of infection can be dramatically lower. The maize plants may experience different effects depending on its growth stage. For instance, when young seedlings are attacked, the plant fails to develop and produce fertile ears. On the other hand, stunted, malformed cobs with fewer grains can be formed on infected mature plants. Maize downy mildew can be characterized by the elongated chlorotic streaks with downy growth of conidia and conidiophores, pale yellow to whitish discoloration on leaf blade, distorted tassels, and aborted ears. As the infection progresses, the maize plant may experience death within 1 month (Rathore et al., 2002).
Brown spot
Caused by the fungus Physoderma maydis infection, maize brown spot is more common in regions with high rainfall and mean temperature. The disease was initially described in India in 1910 and later in the United States of America which had caused 5%−10% yield loss. Today, it is also widespread in Southeast Asia where maize production is growing (Robertson et al., 2015). The attack can be at any part of a maize plant but most infections occur at the leaves, especially during the tasseling stage, where the fungus attacks leaf sheaths, stalks and occasionally the outer husks. Brown spot disease manifests itself in the form of yellowish to brown oval spots that appear in alternate broad bands over the leaves. Dark purplish to black oval spots are developed on midrib whereas lesions on laminae remain as chlorotic spots. These can combine and cause stalk rotting and lodging in severe diseases (Subedi, 2015).
Cocoa
Theobroma cacao or commonly known as cocoa originated from the central and western Amazon region of South America. The cultivation of cocoa began 3,000 years ago by the Mayans, Toltecs, and Aztecs and later brought to Southeast Asia via the Philippines in the 1760s (Drenth and Guest, 2004). Now, Indonesia and Malaysia have become the leading cocoa bean producers, with Indonesia remaining at the third place of world's cocoa exporter after Ghana and Ivory Coast. Nevertheless, the production of cocoa has been gradually decreasing in recent years. Indonesia produced 325,000 metric tons of cocoa in 2014/2015 but the yield dropped to only 220,000 metric tons in 2018/2019, while for Malaysia, the output has decreased annually to just 3,000 metric tons from 100,000 metric tons over the past 20 years (ASEANToday., 2019). This decline was contributed by several factors, including the threats of diseases, which must be fully addressed in order to fulfill the increasing market demand (which is rising at a pace of 5% per annum). The production of cocoa is also influenced by the changing climates, for instance, high temperatures had been found to significantly reduce photosynthesis by damaging photosystem II and decreasing chlorophylls and pigment composition in young cocoa seedlings, thus impacting their survival (Mensah et al., 2022). Combinatorial effects occur when climate changes promote the survival and spread of pests and pathogens which further degrade the quality and yield (Delgado-Ospina et al., 2021). Apart from elevated temperature, strong storms have the potential to topple shade trees and seriously harm cocoa trees. The high wind sensitivity of cocoa leaves will potentially lead to poorer light absorption and increased plant stress which would reduce yields. In addition, strong winds at night make it easier for pathogens to spread over long distances (Asman et al., 2021).
Black pod
The fungus Phytophthora palmivora is the main causative agent of the common yet destructive black pod disease in the cocoa industry. It typically infects all parts of the cocoa plant at all growth phases throughout its life cycle, resulting in 20%−30% pod loss and annual death of 10% of trees due to black pod rot (Guest, 2007). Direct crop losses can reach up to 90% in areas with high humidity which favors the spread and development of fungal attack. The most recognized symptom of diseased cocoa plants is the appearance of black pods or pod rot. The infections can occur at the tip or stem end of the pods, but they are most common toward the soil (Acebo-Guerrero et al., 2012). Initially, the lesions begin as small dark spots on the pod which then grow rapidly to cover the whole surface and internal tissues (Guest, 2007). Cocoa beans become discolored and shriveled as a result of fungal invasion and the infected pods eventually become mummified. If the infestation occurs during the nursery stage, it will lead to seedling blight, thus jeopardizing the start point of cocoa culture. Besides, P. palmivora has a high degree of pathogenicity and tends to survive in the soil, primarily as chlamydospores. Hence, it can always be found in the canopy, ready to initiate the serious outbreaks at any time when condition is optimum (Acebo-Guerrero et al., 2012).
Pineapple
Scientifically known as Ananas comosus, pineapple is a tropical crop that was native to South America and later introduced to Asia in the 17th century. With production levels of 2.70 million tons and 2.45 million tons in 2020, the Philippines and Indonesia have surpassed Thailand as the leading pineapple producers in Southeast Asia (FAO, 2022). Because of its savory taste, pineapple is in high demand as a food source as well as medicine. In Malaysia, the MD2 pineapple has been identified as a high value pineapple variety for commercialization and export, and has been highlighted as a high-value crop in the country's National Agrofood Policy 2.0 (Bernama, 2021). However, despite the high popularity of pineapple, its cultivation is rather intensive as it takes 14–18 months for the plants to reach maturity for harvest (Reinhardt et al., 2018). In addition, pineapple production is also facing a huge threat from the natural environment and infection of diseases (Table 4). The increased pineapple disease outbreaks can be related to the prolonged droughts and elevated temperatures. Pineapple yields are also relatively sensitive to soil water-logging which eventually affects the quality of harvested fruits. Rainfall fluctuations are demonstrated to exhibit an impact on the pineapple productivity by lengthening the growth phases, increasing production costs, changing the number and quality of pineapples produced and particularly influencing the vegetative and yield formation stages of pineapple production (Williams et al., 2017).
Durian
Well-known as the king of fruits, durian or Durio sp. is a tropical fruit indigenous to Southeast Asia that is extensively distributed in Malaysia, Thailand, Indonesia, Vietnam, and the Philippines. Around 600,000 and 300,000 metric tons of production came from Thailand and Malaysia, respectively in 2019, while in the following year, Indonesia recorded an output of 1.19 million metric tons, placing them among the top global durian producers and exporters (FAO, 2022). Despite its many variations which provide distinct taste enjoyment, durian has a notorious reputation in some parts of the world for its strong pungent smell. Yet, many people still favor the fruit, especially in the Asian market with Singapore, China, and Hong Kong as the top receiving markets (Safari et al., 2021). Typically, the growth of durian trees is mostly favored during hot and humid tropical conditions with high annual rainfall. In Indonesia and Malaysia, the production often occurs in short seasons of 2–3 months despite having two fruiting seasons as the plantations are subjected to different monsoon seasons (Drenth and Guest, 2004). Thus, due to its high demand, this tropical fruit has attracted a premium price in the current market. Climate vagaries can threaten the supply of durian to support the high-demand market. In the Philippines, the durian production has declined to 70,064 metric tons due to the destructed farms caused by typhoon. Insufficient water also leads to yield loss as a typical young durian tree requires an annual rainfall of 1,500 mm to grow optimally due to its shallow root system, while excessive water may disrupt the flowering stage. In addition, animal pollinators are also needed to facilitate the reproduction of trees, including durian. Thus, the decline of forest-dwelling animals such as fruit bats due to deforestation or hunting by the local communities will hamper the yield of durian, especially when these important durian pollinators face a population threat (Mohd-Azlan et al., 2022).
Patch canker
Patch canker is a widespread and devastating disease that can affect almost 30% of durian trees in an area. It is caused by a soilborne pathogen, P. palmivora. High rainfall is unsurprisingly conducive for the spread and development of this disease within durian plantations. It has been reported that 20%−25% of yield was lost from disease outbreak due to the failure to control the fungal spread (Drenth and Guest, 2004). The symptoms are characterized by reddish-brown discoloration of outer bark and sapwood and areas of necrosis on the bark. Although evident symptoms can be found around the base of main trunk and on lower branches, they are more prominent at the point where main and lateral stem branching occur (Sivapalan et al., 1997). The leaves turn yellow and drop, followed by a burst of shooting. Patch canker can cause durian trees to become debilitated and finally die if the disease is not well controlled (Lim and Sangchote, 2003).
Jackfruit
Jackfruit or Artocarpus heterophyllus, the biggest tree-borne fruit native to South and Southeast Asia is widely cultivated in Thailand, Vietnam, the Philippines, Indonesia, and Malaysia (Sidhu, 2012). Weighing up to 100 pounds, a single jackfruit can feed a whole family due to its large size and the different ways people have learnt to prepare it. The tree's early life is frost-sensitive and cannot withstand drought, allowing it to only thrive in humid tropical and near tropical climates. Climatic change can result in the loss of ~4.5% of arable lands to grow jackfruits. Within the 3-year period between 2015 and 2017, the yield of jackfruit has accounted for 3.7 million tons (Altendorf, 2018). Jackfruit has been a popular and extensively consumed fruit in Southeast Asia, where it is served in a variety of ways in many households, including curry, juice, cream, and cakes. Even its seeds are edible with a sweet milky taste. Recently, due to its high fiber content and meat-like texture, many start-up companies have proposed jackfruit as a plant-based meat substitute and the idea has gained much popularity in the western countries (Ong et al., 2021; Keerthana Priya et al., 2022). Nevertheless, there is still a constant threat of diseases that concern the jackfruit growers, which largely depends on the temperature, rainfall and relative humidity.
Rhizopus fruit rot
Caused by Rhizopus stolonifer, Rhizopus artocarpi, and Rhizopus oryzae, this fruit rot disease is a severe danger to the jackfruit industry and occurs frequently in locations with high rainfall or after stormy periods. Whenever the flowering and fruiting season coincides with warm, humid, and wet weather, there is likely to be a total loss of fruits in the plantation. The fungus can attack the jackfruit at any point of its development, causing harm to flowering branches or stalks of the fragile fruits, resulting in soft rot (Sidhu, 2012). Around 15%−32% of losses can incur from the disease outbreak. Most commonly, the infection takes place at male inflorescence and young fruits. Recognizable symptoms of Rhizopus fruit rot include the initial formation of soft, watery brown patches on blossoms or fruits, followed by powdery, fuzzy-looking masses of black spores and white fungal mycelia covering the whole surface of fruit. Although optimum condition is required for the development of disease, Rhizopus spp. can survive in decaying plant litter or in the soil, thus it is capable of initiating a new infection at any time and no jackfruit varieties have been suggested to be significantly resistant to the disease (Nelson, 2005a).
Black pepper
Piper nigrum or commonly recognized as the black pepper is an important and widely used spice ingredient in the Southeast Asian cuisines. Believed to have come from the state of Kerala in South-western India, it has dominated the world's spice trade volume by 34% with the annual quantity growth rate of 3.6% (Nair, 2020). The biggest producers and exporters of black pepper are Indonesia, Malaysia, and Vietnam, which together with Sri Lanka and Brazil make up the International Pepper Community (IPC). Vietnam alone contributed to 42% of global pepper production and dominated the global pepper market (Korah and Mohankumar, 2021). The cultivation of black pepper is essentially crucial as it requires heavy and well-distributed rainfall and high temperature for maximum productivity. Besides, disease infection is also a major factor which is affecting the yield of black pepper and the situation is further exacerbated by the unpredictable weather events. Thus, much attention is highly needed to decrease the spread and incidence of diseases, as well as to combat the effects brought upon by climate change.
Anthracnose
Anthracnose or the black berries disease is a common fungal disease which not only affects black pepper, but also causes serious losses to other crop plantations such as rubber and tobacco. Caused by Colletotrichum gloeosporioides, the severity of disease varies from 28 to 34%, thus causing production losses that range from 1.9 to 5.9% (Nair et al., 1987). The fungal infection can happen at any plant part including foliage stem, spikes, and berries (Biju et al., 2013). Increased incidence of anthracnose has been reported particularly during the post-monsoon season which tremendously reduced the black pepper production. The fungus tends to favor warm and humid conditions (27°C and 80% humidity) and may be inactive during dry season (Roberts, 2001). Anthracnose can be identified by looking for symptoms such as necrosis at the tips of berries stalk and appearance of dark lesions on infected berries that crack and fall off (Zakaria and Mohd Noor, 2020).
Foot rot
Induced by fungus, Phytophthora capsici, the disease is also referred as sudden wilt where an epidemic in Sarawak, Malaysia during the mid-1950s had resulted in a total crop failure (Holliday, 1963), while in Vietnam, up to 15%−20% of yield loss has also been reported (Drenth and Guest, 2004). The soilborne fungus is likely to be active in wet soil and the disease spreads rapidly after prolonged wet periods with temperature between 24 and 29°C, thus making control measures to be difficult. Some farmers have reported that the outbreak tends to arise only at the fruiting stage of black pepper plants and once infected, the black pepper plants may die within 2–3 weeks in the rain (Truong et al., 2008). The disease can also be transmitted to nearby plants within 1 or 2 months (Tang Ton and Buu, 2011). Currently, no effective result has been obtained from any specific pesticides to decrease the occurrence of foot rot disease. Visible symptoms often start with formation of dark spots on leaf margins which will then be defoliated when the fungus spreads to the entire laminar. The infected stems will wilt and berries turn black, while the roots also deteriorate and eventually lead to the death of the plant (Zakaria and Mohd Noor, 2020).
Wheat
Although wheat has been grown massively in Southeast Asia in the past decades, it is now not a significant crop in the area. However, changing circumstances in some countries such as the need to diversify crop production have resulted in increasing attention being directed to its possibilities. The production of wheat or scientifically known as Triticum sp. is small in Southeast Asia but the grains play an important part of diets in the region, where the demand continues to trend higher based on longer term shifts in consumption from rice to wheat, as diet diversify. The wheat crop is relatively resistant to drought, thus able to survive in areas with cool and dry climates. The production system is often characterized by the adequate supply of water, appropriate plant nutrition as well as timely sowing. Southeast Asia's total estimated wheat grain production potential is >3 tons per hectare (Aggarwal, 1988). Worldwide productivity of wheat plants is significantly impacted by major diseases caused by pathogens that can infect the root, leaf, and head of the plant. Depending on the variety, the severity of the disease and the favorable climate for disease growth, root diseases can result in losses of 3%−4%. In addition, numerous studies revealed that the fungus Rhizoctonia solani and the root disease both generate annual output losses of 25%−100% of the world's wheat supply (Abass et al., 2021). The population of plant pathogen has been significantly impacted by climate change, in which the germination and survival of pathogens are significantly influenced by temperature and water. The rise in rainfall, accompanied with an increase in air temperature of 0.8–1.1°C is well-suited for the pathogen life cycle and thus facilitating colonization of crop debris and access to specific susceptible hosts. High temperatures also increase transpiration which raises the risk of drought and lowers wheat productivity. At the moment, 15% of wheat productivity is impacted by drought. According to predictions, there would be a serious water shortage in the next 20 to 30 years for every 2°C change in temperature and the lack of water during milking and grain filling will reduce wheat production (Elahi et al., 2022).
Damping off
Damping off is caused by Sclerotia rolfsii and R. solani. The fungal pathogens are soil-borne and widely distributed, which can survive for a long period of time, thus causing its control to be difficult. When wheat crops are infected with damping off disease, failure of seeds to emerge out occurs with the appearance of light brown to red water-soaked roots and stem that eventually lead to drying and collapse of wheat plants. The seeds may also rot before the germination due to the fungal invasion (Muimba-Kankolongo, 2018). Damping off often affects around 5%−80% of seedlings, thus causing heavy economic and yield losses to the farmers (Jay et al., 2017).
Soybean
Soybean or Glycine max is a type of legume with sweet flavor that is native to East Asia. It was initially domestically farmed in 1100 BC and was first mentioned in Chinese literature in 2850 BC. Soybeans had already been cultivated in Japan by the first century AD and were also produced in Indonesia, the Philippines, Vietnam, Thailand, Malaysia, Burma, Nepal, and India. It has a high protein content and is often used as an alternative source to meat to produce various food such as tempeh and tofu. Despite the soaring protein demand, many Southeast Asian countries do not have soybean production and tend to import soybeans from other countries including Argentina, the United States, and Brazil. Due to the lack of soybean crushing facilities in Southeast Asia, soybean meal imports stand a larger proportion than the whole soybeans, which take up 9.4 million tons in 2018 and are projected to increase to 11.9 million tons in 2028. Countries like the Philippines and Thailand have minimal soybean production which only supplies to 2% of the consumers while Vietnam's domestic production meets roughly 4% of the needs (Lee and Hansen, 2019). With the minimal production, concerns are still high on the potential of disease outbreak in soybeans. On top of that, climate change also reduces the production of soybean seeds and nitrogen balance, with 5% loss is foreseen to occur between 2050 and 2100 as a result of the drier and hotter environment.
Soybean rust
The soybean rust disease, caused by Phakopsora pachyrhizi presents as one of the major threats to the soybean production as a result of its rapid spore dispersion by wind. Uredinia develop 5–7 days after infection under ideal circumstances; with a temperature range between 15 and 28°C and moisture on the leaf surface for 6–12 h. Urediniospores can be formed 2 days later. For spore germination and leaf infection, a relative humidity between 75 and 80% is required. After the start of sporulation, a single pustule can continually produce hundreds of urediniospores for around 3 weeks. New infections then develop close to the original disease site as a result of the urediniospores being spread by the wind. As long as the urediniospores are shielded from ultraviolet radiation, they can be carried across great distances or stay viable in the air for several days, leading to new infections outside the immediate area. Until the soybean plant is defoliated or environmental factors no longer favor disease growth, the disease cycle will persist (Murithi et al., 2016). Hence, obtaining soybean resistance is challenging because of the pathogen's great genetic diversity. While soybean rust infection can cause symptoms at any stage of plant growth, losses are most frequently linked to the blooming through pod filling stages. Typically, lesions with one or more yellowish brown to cream uredinia and gray-green, tan to dark brown, or reddish-brown colorations appear on the lower side of leaf. Infection with soybean rust reduces yields, mostly by reducing the photosynthetic activity of infected leaves. This is brought on by a decrease in the amount of green leaf area as a result of lesion formation and early defoliation, which reduces the amount of dry matter accumulated, the quantity of full pods, the size, and weight of seeds. In countries where soybean rust is established, losses can range from 10 to 80% and the severity differs by the susceptibility of soybean variety, climate, and growing season (Sweets et al., 2022).
How climate change affects the production of commodities?
The production of crops in Southeast Asia can be highly dependent on the climate due to its long coastline, seasonal monsoon patterns and a heavy resilience on natural resources (Nor Diana et al., 2022). When climate changes, they could pose challenges to the agricultural sectors and threaten the food security (EPA, 2017). Climate change takes in changes exceeding the average atmospheric condition that are consequences of natural causes including orbit of Earth's revolution, volcanic activities, crustal movements and anthropogenic activities. Despite being a constant process on Earth, the pace of this variation has risen manifolds whereby the average temperature has increased by 0.9°C since the 19th century, dominantly due to global warming and greenhouse gas emissions. As a consequence of the remarkable rise in temperature, increased events of droughts, floods, irregular patterns of precipitation and heat waves are happening across all nations. If the current situation continues to worsen, a decline in the yield of the primary cereal crops (20%−45% in maize, 5%−50% in wheat and 20%−30% in rice productions) is foreseen by the year 2100 (Arora, 2019).
Climate change impacts agriculture through various ways. Beyond the optimum growth temperature, global warming is likely to decrease the crop yields by interfering with their growth developments and ability to absorb nutrients or moisture. Rate of evaporation from the soil increases while crops transpire at a higher rate, thus losing more water content from the leaves. The combined effect is known as evapotranspiration (Cline, 2008). An increased level of carbon dioxide (CO2) also affects crop productions as it is often associated with reduced nutritional levels such as protein and nitrogen contents in alfafa and soybean plants, causing quality losses. Besides that, under the warm temperature, wetter climates and elevated CO2 levels, a number of weeds, pests, and fungi could thrive, hence exposing continuous threats to the crops (EPA, 2017). The widespread of pathogens and diseases can be worsened by the changing climate when heat waves and drought decrease the resistance of crops to specific diseases and through increased pathogenicity of pests caused by mutation induced by environmental stresses (Gornall et al., 2010).
Changes in the physical climate is likely to result in the changes in plant morphology, physiology, and chemistry due to increasing CO2 concentrations that boost diseases severity. The disease outbreak may significantly hinder the limited range of crops that are adapted to the changing climate. Crop losses due to increased disease transmission under changed climate can be evaluated by various interacting factors. For instance, altered physiology and morphology of crops as well as elevated CO2 may change the interception of light and precipitation, modify canopy structure and microclimate to affect disease epidemiology (Chakraborty et al., 2000). Apart from CO2 concentrations, changes in temperature and precipitation regimes due to climate change tend to modify the growth stage, development rate and pathogenicity of infectious pathogens as well as the resistance of host plants. It may also cause a shift in the geographical distribution of pathogens and favor the dormant agents that could induce an epidemic. Evidences have shown that increased severity of bacterial diseases are most likely to happen as a result of temperature change as it governs the reproduction and evolution of pathogens (Gautam et al., 2013). In Malaysia, it has been reported that a 4.4% of decline in grain mass and as much as 9.6%−10.0% drop in grain yield per 1°C rise occur when temperature reaches above 25°C (Alam et al., 2017). Notably, oil palm production reduces from 2 to 5% for each 10°C increase in temperature. The climate inconsistency has created significant impacts in agriculture with 13%−80% reduction in rice yield and 10%−30% decline in other crop productivities (Nor Diana et al., 2022).
Evidence of decreased crop productivity in Southeast Asia (2010 – 2020)
The decrease in available agricultural area due to the increase in population growth, coupled with the problems brought upon by climate change has unfortunately taken a toll on crop productivity. Although crop production is one of the dominant contributor to climate change, it is also highly susceptible to the effects whereby any slight change in the climate parameters will incur a significant impact on crop output, pest susceptibility and pathogen mortality. Figure 2 shows the yield of top six major crops in Southeast Asia from 2010 until 2020. It can be observed that the yield of oil palm, rubber, sugarcane, and banana is showing a decreasing trend in most of the producing countries, despite the technological advances and automation processes that have been adapted by the agricultural sector. The disease triangle (Figure 3) in an agricultural process is made up of three components, namely pathogen, host, and environment where the interaction among them is what distinguishes an infection from one that does not exist. Pathogen presence and virulence are influenced by several factors including genome structure, reproductive systems and capacity for horizontal gene transfer, genetic variation within population and effective population size.
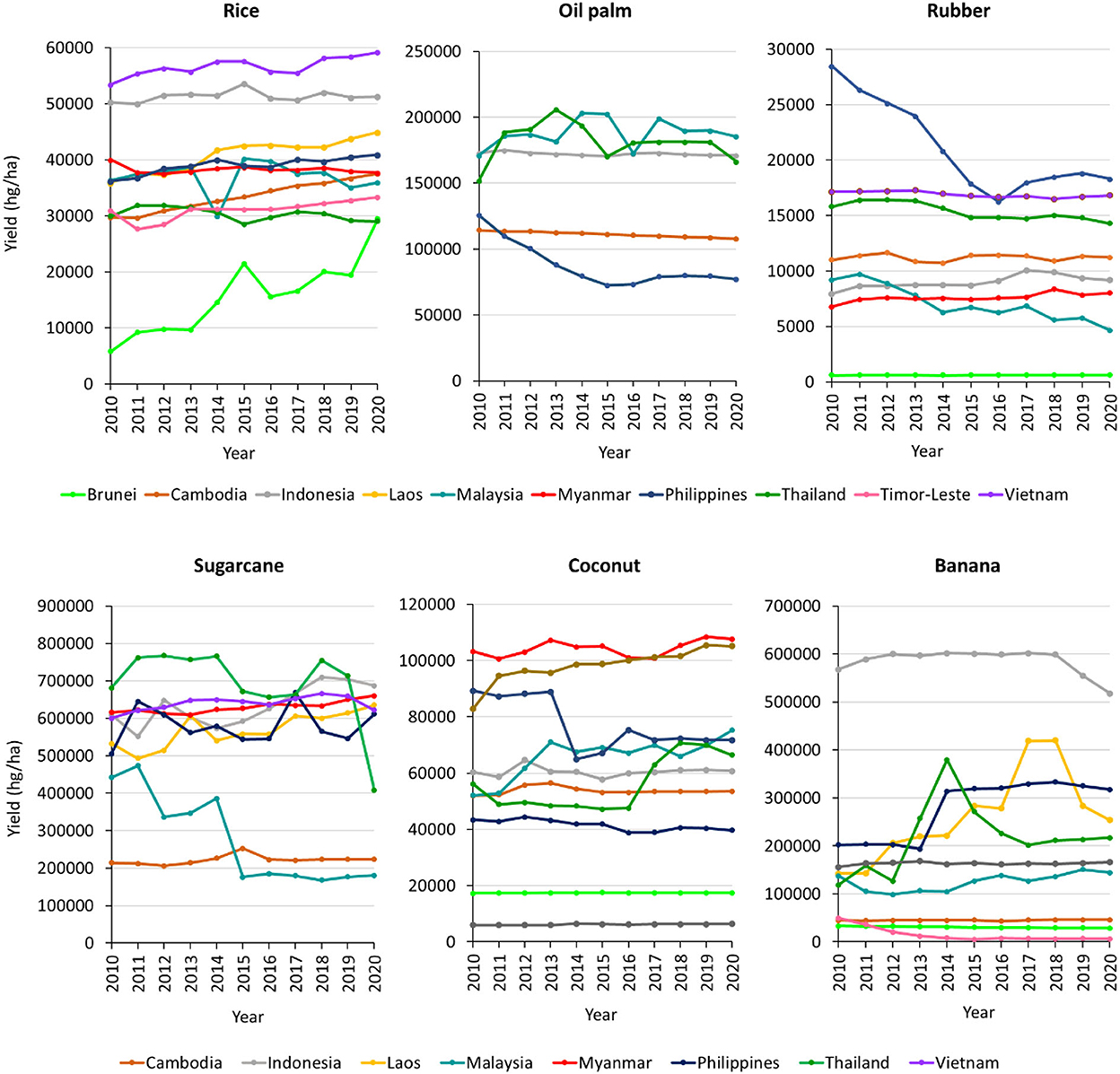
Figure 2. Productivity of top six major crops in Southeast Asia (rice, oil palm, rubber, sugarcane, coconut, and banana) measured in terms of their yield (production per unit of area harvested). These graphs were plotted based on the data provided by FAO (2022).
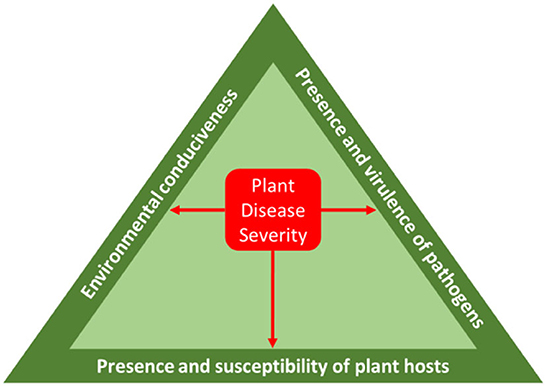
Figure 3. The disease triangle. Redrawn based on Fones et al. (2020).
Several plant diseases tend to have a massive outbreak when climate changes. This is because plant pathogens, similar to all species, exhibit particular tolerances or requirements for specific environmental conditions. Their ecological niches are often defined by these tolerances, which establishes the geographic regions and time periods during which encourage the growth and attack of pests and pathogens (Chaloner et al., 2021). A major example for devastating impacts of climate change is the occurrence of floods resulted from the rise of sea level which eventually leads to disappearance of low-lying regions and tremendous agricultural losses. Different types of crops will respond to flooding in a different way but they are all vulnerable to the effects. For instance, as a semi-aquatic crop species, rice tends to be more tolerant to flooding compared to other plants while small grains including wheat may be able to withstand at least a day of low-level flooding or 2 days of completely saturated soils. The excessive water on the soil surface leads to oxygen deficiency, causing root rot pathogens such as Fusarium spp. and Pythium spp. which flourish in these conditions, to be more likely to infect plants. Under wet conditions, root rots progress into mesocotyl and crown rots, thereby making a bad situation worse.
Besides flooding, drought is another major threat to plants, whereby the insufficiencies in water levels result in plants losing their biological processes and becoming more vulnerable to diseases and pests. The blast disease, caused by M. oryzae can severely affect the rainfed rice that has experienced intermittent and repeated drought. Evidences have depicted that the occurrence of drought is likely to influence the expression of plant resistance genes or pathogen effectors, leading to partial degradation of several important resistance genes. A rise in abscisic acid (ABA) is induced by the effects of drought, where ABA generally boosts stomatal closure and other pre-penetration defenses but it does not work for rice blast fungus since it does not require stomata for penetration. However, ABA pathway activation in response to drought could impede plant defense, verifying the down-regulated plant immunity response (Bidzinski et al., 2016).
Meanwhile, according to Abubakar et al. (2021), the repurcussions of climate change on oil palm production in Southeast Asia are of great concern. The incidences of heat wave and temperature rise would accelerate soil water evaporation, rendering the soils to be drier and accentuating the effects of water scarcity. Further exacerbating this problem, the projected temperature for the Southeast Asia region in 2050 as well as 2100 have also been reported to be extreme and unsuitable for oil palm plantation (Paterson, 2021). In addition, the increase in temperature would also affect the pollination activity and population of oil palm pollinators such as the Elaeidobius kamerunicus weevils, which in turn would significantly hamper the yield of oil palm fresh fruit bunches (FFB) (Harun and Noor, 2002). Besides that, the worsening heat wave due to global warming also weakens plants' natural defenses. This is because the extreme high temperature may suppress the production of salicyclic acid where in normal cases, this natural plant immune system is activated to keep the pathogens from spreading. Rising CO2 may promote the production of plant biomass but consequently the high concentration of carbohydrates in the plant tissue will stimulate the development of biotrophic fungi like rust, powdery mildew and leaf spots, while increased plant density tends to prolong leaf surface moisture and regulate temperature which will enhance the likelihood of foliar pathogen infection (Jankar et al., 2020). Over time, it can be more difficult to control and suppress pathogen invasion as they are also experiencing evolutions in order to adapt to the changing environment. For instance, wheat rust which is devastating to the wheat production is becoming more aggressive to survive in the warmer climates. Researchers, farmers and regulatory bodies are constantly finding a way to combat the problems to anticipate the future in sustaining food security.
Despite that, the yield of staple food such as rice is increasing, due to the increased efforts and monetory inputs invested for research and development of high-yielding and stress-tolerant rice varieties (Dam et al., 2021; Dar et al., 2021; Ho et al., 2022). Similarly, the yield of coconut is also on the increasing trend, due to the rise in its production and land use conversion (Zhang and Su, 2020) since it is one of most important cash crops that thrives well in hot environment (Moreno et al., 2020).
Strategies to mitigate climate change effects for increasing crop productivity in Southeast Asia
Despite the booming effects brought by the agricultural sector to the economy in Southeast Asian countries, this particular sector can be very vulnerable to the changes in the climate. Significant and devastating impacts such as entire crop loss can constantly threaten the sector. Hence, appropriate mitigation strategies are required in order to sustain crop productivity, to lower the vulnerability and to improve the resilience of agricultural system to climate change. The adaptations may include both technological and non-technological solutions to produce more food, reduce or share risks and enhance governance. Various agricultural management practices have been optimized, among which are on-farm practices and biophysical measures such as increased soil organic matter, improved cropland management, multiple cropping, crop-livestock mixed system and agroecological approaches. For instance, soil management is crucial in curbing the effect of climate change on crops whereby hedgerow planting, vegetation cover and contour plowing are efficient to prevent wind-induced soil erosion. Changing tillage practice and shifting to zero tillage with residue retention help cropping system to adapt to water stress, untimed rainfall, and high temperature. Besides that, crop diversification enhances the resistance to climate change by improving the ability of crops to suppress disease outbreaks while decreasing the rate of pathogen spread, thus buffering crop yield under climatic stress. It is considered as one of the effective approaches in achieving sustainable agricultural development as it minimizes environmental pollutions, while securing food supply opportunities.
The introduction of short duration crop varieties may also help in curtailing the serious impacts of climate risk. Farmers can practice planting leguminous crops with the main crop to supply nitrogen to the soil which is lost due to soil erosion or flooding. The system of rice intensification (SRI) is a set of crop, soil, and water management practices in which 8–15 days old seedlings are transplanted singly and irrigated intermittently to keep rice fields not only moist but aerated. This measure successfully increases the crop productivity by more than 10% with less water consumption (25%−47% less water). The rice plants grown in SRI practices tend to have stronger tillers and root systems and are more resistant to biotic and abiotic stresses due to climate change (Aryal et al., 2020). It reduces methane production and emissions by using less water and alternating wet and dry situations, serving as a holistic approach to a sustainable farming. With this system, it has yielded 50 to 100% more outputs than the conventional rice production.
Besides that, the utilization of biotechnological tools to improve plant genomes is also one of the exciting strategies that are currently being employed to help combat the effects of climate change. Genetic modification is a strategy to allow plants to exhibit more tolerance or better resistance to unfavorable environments. For instance, marker-assisted selection (MAS) has been employed in rice cultivation to overcome the submergence issues during flooding. Although rice is technically grown in semi-aquatic condition, the growth and development of most rice cultivars is severely obstructed and the rice plants will eventually die under 1 week of complete submergence, leading to annual loss of US$1 billion. However, some cultivars such as Oryzae sativa ssp. indica cultivar FR13A tend to tolerate better and can survive despite being completely submerged for 2 weeks. This desired trait is attributed by the quantitative trait locus Submergence 1 (Sub1) near the centromere of chromosome 9, with three genes being identified (Sub1A, Sub1B, and Sub1C) (Xu and Mackill, 1996). Among these three Sub1 genes, only Sub1A gene is highly variable across the rice varieties and it exists in two alleles, namely Sub1A-1 (tolerance-specific) and Sub1A-2 (intolerance-specific). Hence, evidences have illustrated that the overexpression of Sub1A1 in the submergence-intolerant rice can significantly enhance the plant's tolerance in the face of flooding (Xu et al., 2006). The locus is then introgressed into the Asian rice cultivar using MAS, producing a new variety with unaffected yield and is submergence-tolerant. This approach serves as a solution against destructive floods and thus improving food security (Xu et al., 2006). Compared to the traditional breeding and selection techniques, genetic tools may provide more efficient and desirable results to the agricultural industry. However, concerns may rise as this genome application can be tedious, expensive and somehow is not environmentally-friendly when there is an unintentional mix between the edited and wild types, which may potentially bring harm to the ecosystem.
Another approach of climate change adaptation is through implementation of national policies such as the National Agrofood Policy 2.0 adopted by the Malaysian government. It aims to transform the agrofood sector into a sustainable, competitive, and high-technology field, while boosting the nation's economic growth to strengthen the wellbeing of people. Shifting toward a sustainable food system which is adapted to climate change allows the well development of agrifood sector without taking a cost to the environment. Through the practice of smart agriculture and embracement of modernization, crop producers are able to gain better control over pests and disease threats, thus opting for a more predictable and efficient process. These strategies are likely to look into enhancing production volume, improving self-sufficiency levels, enhancing efficiency of natural resource and improving the livelihood and income levels of farmers in recent years (MAFI., 2021).
Control of crop's diseases
The high incidence of various plant pathogens constantly threatening the crop plantations in Southeast Asian regions has resulted in devastating economic and production losses. Hence, farmers and scientists are continuously seeking for effective treatments or preventive approaches to hinder the transmission of pathogens and minimize the disease outbreaks. Various crop management strategies are extremely essential and have been proposed in order to sustain crop production. During the past decades when there were less development and advanced technology in the agricultural sector, these plant diseases were usually controlled physically or through modification of cultural practices (Howard, 1996). Removal of infected parts, pruning, adequate irrigation system, crop rotation, and plant spacing are some common physical and cultural controls to lower the intensity of plant diseases (Cruz-Lacierda and Erazo-Pagador, 2001). These techniques rely on the simple principle of tackling the physiological tolerance of pathogenic causative agents to harsh conditions (such as high or low temperatures and a lack of moisture), to stop the spread of spores, remove pathogen sources or prevent close contact between the pathogens and host plants by establishing physical barriers (Cruz-Lacierda and Erazo-Pagador, 2001).
Chemical control of plant diseases
Later, chemical fertilizers were found to provide better performance in reducing crop losses, especially with the development of several specific-action fungicides or host-defense inducers. These chemical agents are capable of hindering the growth or killing the disease-causing pathogens through direct mechanisms, which in turn increase their effectiveness. A variety of chemicals have been formulated to control different plant pathogens, such as the widely used fungicides like sulfur, copper, captan, thiram, hexaconazole, mancozeb, and maneb. The mode of application is usually through spraying on seeds, foliage, flowers, fruits or via soil drenching (Hirooka and Ishii, 2013). Other than fungicides or bactericides to kill pathogens, chemical fertilizers containing nitrogen, phosphorus, and potassium or plant hormones such as salicylic acid are also popular as a preventive strategy which promote plant growth and allow plants to develop the required resistance against pathogen attacks (Lefevere et al., 2020).
However, despite the development of numerous disease treatments, there is still 20%−30% of crop production being lost annually. This may be due to the limitations in the control efficacy shown by traditional methods due to multiple drawbacks. These strategies have become less dependable as a result of adverse environmental effects of chemical agents and the evolution of pathogen resistance. This is made worse by the global climate change which causes disease control to be more complicated and difficult. The sustainable application of chemicals has resulted in the rapid development of pathogens which are now more robust and resilient. Moreover, environmentalists and nature conservationists often criticized the use of chemical agents which contaminates the soil, water, and other vegetations, thus decreasing their dependency. Plant breeding or the use of resistant cultivars has been thought to be a stable and long-lasting solution which may minimize the use of chemical controls. Plants' innate immune systems allow them to recognize the pathogens and breeding for long-lasting resistance by combining multiple and diverse resistance genes may help to enhance crop performance, but barriers to this approach may be created on the basis of public perception toward genetically modified organisms (GMOs) as well as the high cost and technology involved (Adhikari et al., 2020). Hence, the establishment of more environmentally-friendly, economical and effective bio-controls are highly anticipated.
Disease control through sustainable and eco-friendly measures
Many beneficial microorganisms including fungi and bacteria have been shown to be efficient in impeding and suppressing the growth of disease-causing pathogens. For example, Pseudomonas spp., Bacillus spp., Trichoderma spp., Acinetobacter spp., Streptomyces spp., Aspergillus spp., yeasts, and lactic acid bacteria have been successfully used as bio-control agents. The control efficacy by these microbes is often due to their ability to produce various antimicrobial metabolites, volatile organic compounds (VOCs), siderophores, 2, 4-diacetylphloroglucinol (DAPG) and numerous cell-wall degrading enzymes (CWDEs) including cellulase, chitinase, protease, and β-1, 3-glucanases which are essential in breaking down the fungal chitinous cell wall (He et al., 2021). These biological control agents (BCAs) alter the interaction among plants, pathogens, and the environments, thus resulting in biological and physical cascades that affect pathogen fitness, plant health, and ecological function. Compared to the control measures aforementioned, BCAs tend to target specific pathogens and therefore contribute fewer negative impacts on the ecosystem.
The control of plant diseases by BCA can be defined through several mechanisms including pathogen suppression, compounds priming, and ecosystem regulation. Some microorganisms produce secondary compounds that directly kill the pathogens while some rely on the pathogens for energy and living environment, which then suppress the development of pathogens. For instance, pathogen causing powdery mildew in cucumber is suppressed by the compounds produced by Pseudozyma flocculosa that induce a rapid cell collapse. Besides that, some compounds produced by the beneficial microbes are responsible for the observed systemic acquired resistance after the host plant is infected by pathogens, thus strengthening the plant's immune response. Treating potatoes with fatty acids results in 39%– 82% of protection against Phytophthora infestans. Often, the outbreak of plant diseases is due to the imbalance ecosystem. By increasing the amount and diversity of beneficial microbes in agricultural lands, good environmental quality, and functional ecosystem are maintained for the growth and immunity development of plants (He et al., 2021). Because of these attributes, increased interests have been displayed in discovering biocontrol agents with enhanced effectiveness in controlling plant diseases for food crop sustainability. The control measures based on different plant diseases are summarized in Supplementary Table 1 according to physical, chemical, and biological aspects.
Disease control through genetic engineering
Apart from the conventional control measures, recent focus has been placed on genetic engineering (GE) by modifying the genes of crops or pest to combat the rise of plant disease outbreaks. This is because GE tends to reduce the time to identify desirable traits and allow a more precise alteration of plant's traits which usually requires months to years, in place of traditional plant breeding and selection methods. The widely used approach is through developing crops with herbicide or insecticide resistances as these traits enable the farmers to use herbicides that offer more effective weed control. For example, the biotechnology Bt crops such as maize and cotton were genetically engineered to express the insecticidal proteins from soil bacterium Bacillus thuringiensis. The foreign bacterial genes encoding for the harmful protein are inserted into the plant genes, hence the plants will be able to produce the same toxin that kills the unwanted pests. Besides modifying the plant's gene, farmers also practice genetic pest management (GPM) by releasing altered pest species to mate with the wild-type pests in order to reduce its population. This is achieved by the combined effects of genetic drift, bottlenecking, and selection of the modified insects which will then decrease their fitness in the wild (Leftwich et al., 2021).
Other than introducing a target gene encoding for the desired trait, gene editing using CRISPR-Cas system can also help in plant disease control. Successful research has been made on CRISPR-Cas9 which facilitated targeted mutagenesis efficiently and precisely in plants to elevate resistance to fungal diseases. It has been reported that the knockout of a susceptibility gene known as mildew resistance locus O (MLO), i.e., SlMlo1 gene in tomato plants resulted in a complete resistance to Oidium neolycopersici, i.e., the powdery mildew causing pathogen (Pramanik et al., 2021). Besides tomato plants, rice has also displayed increased resistance to M. oryzae which causes rice blast disease, through RNAi-mediated knockdown of OsERF922, which encodes for the APETELA2/ethylene response factor (AP2/ERF) type transcription factor (Liu et al., 2012). Recently, a triple mutant rice exhibiting blast-resistant phenotype was developed using CRISPR/Cas9-mediated gene editing, to knockout three broad-spectrum blast-resistant genes, namely Bsr-d1, Pi21, and ERF922 (Zhou et al., 2022). The study also reported that the Pi21 and ERF922 knockout mutants also exhibited increased resistance to bacterial blight, due to the activation of genes involved in salicylic acid and jasmonic acid pathways. However, despite the efficacy of genome editing in controlling plant diseases, there are still some limitations such as the off-target mutations brought upon by CRISPR-Cas9 system which can cause non-specific changes in the genome sequences that eventually affect protein structure and function in plants. When infections rapidly evolve, this would result in a higher genetic diversity of the pathogen population, thus single gene editing-based disease resistance can be easily overcome. Combinatorial genome editing, which involves the interaction of the host plant's resistance gene and the pathogen effector, may be employed to address this issue. This had been demonstrated when the Phytophthora sojae effector Avr genes and soybean Rps genes were edited to lessen the outbreak of Phytophthora root and stem rots in soybean (Paul et al., 2021).
Conclusion
Crop plantations in Southeast Asia have been consistently facing substantial disease concerns due to the ubiquitous pathogenic microorganisms, causing severe losses in terms of economy and production as revealed in the review. Hence, plant management strategies are critical for reducing losses and an integrated approach is usually the best option. Concerns should, however, be directed more toward green and low-cost strategies, with future work focusing on the establishment of new biological control approaches with higher efficacy to overcome the obstacles and limitations that now exist in conventional methods.
Author contributions
MRSZ, JEE, and SRAMR conceived and designed the research. JAL conducted the literature search and wrote the manuscript draft. JSY, JAL, MRSZ, JEE, HAEE, and SRAMR revised the draft and proofread the final manuscript. All authors have read and agreed to the published version of the manuscript.
Funding
This project was funded by Universiti Malaya (grant no. IIRG009A-19FNW) and Institute of Bioproduct Development (IBD), Universiti Teknologi Malaysia, UTM (industrial fund with cost centers: R.J130000.7609.4C395 and R.J130000.7609.4C284).
Acknowledgments
The authors thank Universiti Malaya (UM) and Universiti Teknologi Malaysia (UTM) for the facilities provided. The authors would also like to express their deepest gratitude to Nutrition Technologies, Malaysia for its support throughout the work of literature review and preparation of the review draft, prior to publication.
Conflict of interest
SRAMR, JEE, and MRSZ were employed by Nutrition Technologies.
The remaining authors declare that the research was conducted in the absence of any commercial or financial relationships that could be construed as a potential conflict of interest.
Publisher's note
All claims expressed in this article are solely those of the authors and do not necessarily represent those of their affiliated organizations, or those of the publisher, the editors and the reviewers. Any product that may be evaluated in this article, or claim that may be made by its manufacturer, is not guaranteed or endorsed by the publisher.
Supplementary material
The Supplementary Material for this article can be found online at: https://www.frontiersin.org/articles/10.3389/fsufs.2022.1030540/full#supplementary-material
References
Abass, M. H., Madhi, Q. H., and Matrood, A. A. A. (2021). Identity and prevalence of wheat damping-off fungal pathogens in different fields of Basrah and Maysan provinces. Bull. Natl. Res. Centre 45, 1–12. doi: 10.1186/s42269-021-00506-0
Abubakar, A., Ishak, M. Y., and Makmom, A. A. (2021). Impacts of and adaptation to climate change on the oil palm in Malaysia: a systematic review. Environ. Sci. Pollut. Res. 28, 54339–54361. doi: 10.1007/s11356-021-15890-3
Acebo-Guerrero, Y., Hernández-Rodríguez, A., Heydrich-Pérez, M., El Jaziri, M., and Hernández-Lauzardo, A. N. (2012). Management of black pod rot in cacao (Theobroma cacao L.): a review. Fruits 67, 41–48. doi: 10.1051/fruits/2011065
Adhikari, B., Pangomm, K., Veerana, M., Mitra, S., and Park, G. (2020). Plant disease control by non-thermal atmospheric-pressure plasma. Front. Plant Sci. 11, 77. doi: 10.3389/fpls.2020.00077
Aggarwal, P. K. (1988). Wheat production in Southeast Asia: potential and constraints. Outlook Agric. 17, 49–53. doi: 10.1177/003072708801700202
Alam, M., Siwar, C., Murad, M. W., and Toriman, M. (2017). Impacts of climate change on agriculture and food security issues in Malaysia: an empirical study on farm level assessment. World Appl. Sci. J. 14, 431–442.
Altendorf, S. (2018). Minor tropical fruits. Food Outlook. FAO, 67–75. Available online at: http://www.fao.org/fileadmin/templates/est/COMM_MARKETS_MONITORING/Tropical_Fruits/Documents/Minor_Tropical_Fruits_FoodOutlook_1_2018.pdf (accessed October 28, 2022).
Altendorf, S. (2019). Banana Fusarium Wilt Tropical Race 4: A Mounting Threat to Global Banana Markets. FAO Food Outlook, Rome: FAO.
Anderson, J., Pegg, K. G., Scott, C., and Drenth, A. (2012). Phosphonate applied as a pre-plant dip controls Phytophthora cinnamomi root and heart rot in susceptible pineapple hybrids. Australs. Plant Pathol. 41, 59–68. doi: 10.1007/s13313-011-0090-6
Arora, N. K. (2019). Impact of Climate Change on Agriculture Production and its Sustainable Solutions. New York, NY: Springer. doi: 10.1007/s42398-019-00078-w
Aryal, J. P., Sapkota, T. B., Khurana, R., Khatri-Chhetri, A., Rahut, D. B., Jat, M. L., et al. (2020). Climate change and agriculture in South Asia: adaptation options in smallholder production systems. Environ. Dev. Sustain. 22, 5045–5075. doi: 10.1007/s10668-019-00414-4
ASEANToday. (2019). ASEAN's top cocoa bean producers are struggling to cope with increasing demand for chocolate. ASEAN Today. Available online at: https://www.aseantoday.com/2019/06/aseans-top-cocoa-bean-producers-are-struggling-to-cope-with-increasing-demand-for-chocolate/ (accessed May 12, 2022).
Asman, A., Bin Purung, M. H., Lambert, S., Amiruddin, A., and Rosmana, A. (2021). Effect of rootstock and scion on resistance of cocoa clones to vascular streak dieback caused by Ceratobasidium theobromae. Ann. Agric. Sci. 66, 25–30. doi: 10.1016/j.aoas.2021.02.005
Barnwal, M. K., Kotasthane, A., Magculia, N., Mukherjee, P. K., Savary, S., Sharma, A. K., et al. (2013). A review on crop losses, epidemiology and disease management of rice brown spot to identify research priorities and knowledge gaps. Eur. J. Plant Pathol. 136, 443–457. doi: 10.1007/s10658-013-0195-6
Bebber, D. P. (2019). Climate change effects on Black Sigatoka disease of banana. Philos. Trans. R. Soc. B 374, 20180269. doi: 10.1098/rstb.2018.0269
Benová, D., Mareš, K., Hutla, P., Ivanova, T., Banout, J., Kolaríková, M., et al. (2021). Energy potential of agri residual biomass in Southeast Asia with the focus on Vietnam. Agronomy 11, 169. doi: 10.3390/agronomy11010169
Bernama (2021). National Agrofood Policy 2, 0. Boosts Country's Pineapple Industry. Petaling Jaya: theSun.
Bidzinski, P., Ballini, E., Ducasse, A., Michel, C., Zuluaga, P., Genga, A., et al. (2016). Transcriptional basis of drought-induced susceptibility to the rice blast fungus Magnaporthe oryzae. Front. Plant Sci. 7, 1558. doi: 10.3389/fpls.2016.01558
Bigirimana, V. D. P., Hua, G. K., Nyamangyoku, O. I., and Höfte, M. (2015). Rice sheath rot: an emerging ubiquitous destructive disease complex. Front. Plant Sci. 6, 1066. doi: 10.3389/fpls.2015.01066
Biju, C., Praveena, R., Ankegowda, S., Darshana, C., and Jashmi, K. (2013). Epidemiological Studies of Black Pepper Anthracnose (Colletotrichum gloeosporioides) (New Delhi).
Blomme, G., Dita, M., Jacobsen, K. S., Pérez Vicente, L., Molina, A., Ocimati, W., et al. (2017). Bacterial diseases of bananas and enset: current state of knowledge and integrated approaches toward sustainable management. Front. Plant Sci. 8, 1290. doi: 10.3389/fpls.2017.01290
Chakraborty, S., Tiedemann, A., and Teng, P. S. (2000). Climate change: potential impact on plant diseases. Environ. Pollut. 108, 317–326. doi: 10.1016/S0269-7491(99)00210-9
Chaloner, T. M., Gurr, S. J., and Bebber, D. P. (2021). Plant pathogen infection risk tracks global crop yields under climate change. Nat. Clim. Change 11, 710–715. doi: 10.1038/s41558-021-01104-8
Cline, W. R. (2008). Global Warming and Agriculture, Finance & Development, 0045(001), A007. Retrieved from: https://www.elibrary.imf.org/view/journals/022/0045/001/article-A007-en.xml (accessed December 8, 2022).
Cobo, J. M., Fort, J., and Isern, N. (2019). The spread of domesticated rice in eastern and southeastern Asia was mainly demic. J. Archaeol. Sci. 101, 123–130. doi: 10.1016/j.jas.2018.12.001
Connor, M., Cuong, O. Q., Demont, M., Sander, B. O., and Nelson, K. (2022). The influence of climate change knowledge on consumer valuation of sustainably produced rice in Vietnam. Sustain. Prod. Consum. 31, 1–12. doi: 10.1016/j.spc.2022.01.034
Cruz-Lacierda, E. R., and Erazo-Pagador, G. E. (2001). “Physical, environmental, and chemical methods of disease prevention and control,” in Health Management in Aquaculture, eds G. D. Lio-Po, C. R. Lavilla, and E. R. CruzLacierda (Tigbauan: Aquaculture Department, Southeast Asian Fisheries Development Center), 97–110.
Dam, T. H. T., Tur-Cardona, J., Speelman, S., Amjath-Babu, T., Sam, A. S., Zander, P., et al. (2021). Incremental and transformative adaptation preferences of rice farmers against increasing soil salinity-Evidence from choice experiments in north central Vietnam. Agric. Syst. 190, 103090. doi: 10.1016/j.agsy.2021.103090
Dar, M. H., Bano, D. A., Waza, S. A., Zaidi, N. W., Majid, A., Shikari, A. B., et al. (2021). Abiotic stress tolerance-progress and pathways of sustainable rice production. Sustainability 13, 2078. doi: 10.3390/su13042078
De Koninck, R., and Rousseau, J.-F. (2013). Southeast Asian agricultures: why such rapid growth? LEspace Geogr. 42, 143–164. doi: 10.3917/eg.422.0143
Dean, R., Van Kan, J. A., Pretorius, Z. A., Hammond-Kosack, K. E., Di Pietro, A., Spanu, P. D., et al. (2012). The top 10 fungal pathogens in molecular plant pathology. Mol. Plant Pathol. 13, 414–430. doi: 10.1111/j.1364-3703.2011.00783.x
Delgado-Ospina, J., Molina-Hernández, J. B., Chaves-López, C., Romanazzi, G., and Paparella, A. (2021). The role of fungi in the cocoa production chain and the challenge of climate change. J. Fungi 7, 202. doi: 10.3390/jof7030202
Denny, T. (2007). “Plant pathogenic Ralstonia species,” in Plant-Associated Bacteria, ed S. S. Gnanamanickam (Dordrecht: Springer), 573–644. doi: 10.1007/978-1-4020-4538-7_16
Dita, M., Barquero, M., Heck, D., Mizubuti, E. S., and Staver, C. P. (2018). Fusarium wilt of banana: current knowledge on epidemiology and research needs toward sustainable disease management. Front. Plant Sci. 9, 1468. doi: 10.3389/fpls.2018.01468
DOSM (2021). Monthly Rubber Statistics Malaysia, September 2021. Malaysia: Department of Statistics Malaysia.
Drenth, A., and Guest, D. I. (2004). Diversity and Management of Phytophthora in Southeast Asia. Bruce: Australian Centre for International Agricultural Research (ACIAR).
Elahi, I., Saeed, U., Wadood, A., Abbas, A., Nawaz, H., Jabbar, S., et al. (2022). “Effect of climate change on wheat productivity,” in Wheat Chapter 8, ed M. R. Ansari (London: IntechOpen). doi: 10.5772/intechopen.103780
EPA (2017). Climate impacts on agriculture and food supply. United States Environmental Protection Agency. Available online at: https://19january2017snapshot.epa.gov/climate-impacts/climate-impacts-agriculture-and-food-supply_html (accessed October 29, 2022).
FAO (2022). FAOSTAT - Crops and Livestock Products. Food and Agriculture Organization of the United Nations. Available online at: https://www.fao.org/faostat/en/#data/QCL (accessed March 26 2022).
Fones, H. N., Bebber, D. P., Chaloner, T. M., Kay, W. T., Steinberg, G., Gurr, S. J., et al. (2020). Threats to global food security from emerging fungal and oomycete crop pathogens. Nat. Food 1, 332–342. doi: 10.1038/s43016-020-0075-0
Gautam, H., Bhardwaj, M., and Kumar, R. (2013). Climate change and its impact on plant diseases. Curr. Sci. 105, 1685–1691.
Gianessi, L. P. (2014). “Importance of pesticides for growing maize in South and South East Asia,” in International Pesticide Benefit Case Study Vol. 107 (Washington, DC), 1–4.
Gornall, J., Betts, R., Burke, E., Clark, R., Camp, J., Willett, K., et al. (2010). Implications of climate change for agricultural productivity in the early twenty-first century. Philos. Trans. R. Soc. B Biol. Sci. 365, 2973–2989. doi: 10.1098/rstb.2010.0158
Guest, D. (2007). Black pod: diverse pathogens with a global impact on cocoa yield. Phytopathology 97, 1650–1653. doi: 10.1094/PHYTO-97-12-1650
Guo, H., Huang, Z., Tan, M., Ruan, H., Awe, G. O., Are, K. S., et al. (2021). Crop resilience to climate change: a study of spatio-temporal variability of sugarcane yield in a subtropical region, China. Smart Agric. Technol. 1, 100014. doi: 10.1016/j.atech.2021.100014
Harun, M. H., and Noor, M. R. M. (2002). Fruit set and oil palm bunch components. J. Oil Palm Res. 14, 24–33.
He, D.-C., He, M.-H., Amalin, D. M., Liu, W., Alvindia, D. G., Zhan, J., et al. (2021). Biological control of plant diseases: an evolutionary and eco-Economic consideration. Pathogens 10, 1311. doi: 10.3390/pathogens10101311
He, D.-C., Zhan, J.-S., and Xie, L.-H. (2016). Problems, challenges and future of plant disease management: from an ecological point of view. J. Integr. Agric. 15, 705–715. doi: 10.1016/S2095-3119(15)61300-4
Hebbar, K. B., Balasimha, D., and Thomas, G. V. (2013). “Plantation crops response to climate change: coconut perspective,” in Climate-Resilient Horticulture: Adaptation and Mitigation Strategies, eds H. Singh, and N. Rao (New Delhi: Springer), 177–187. doi: 10.1007/978-81-322-0974-4_16
Hirooka, T., and Ishii, H. (2013). Chemical control of plant diseases. J. Gen. Plant Pathol. 79, 390–401. doi: 10.1007/s10327-013-0470-6
Ho, T. D., Tsusaka, T. W., Kuwornu, J. K., Datta, A., and Nguyen, L. T. (2022). Do rice varieties matter? Climate change adaptation and livelihood diversification among rural smallholder households in the Mekong Delta region of Vietnam. Mitig. Adapt. Strateg. Glob. Chang. 27, 1–33. doi: 10.1007/s11027-021-09978-x
Holliday, P. (1963). Foot rot of Piper nigrum L. (Phytophthora palmivora). Phytopathol. Pap. 6, 1–62.
Howard, R. J. (1996). Cultural control of plant diseases: a historical perspective. Can. J. Plant Pathol. 18, 145–150. doi: 10.1080/07060669609500639
Huang, S., Wang, P., Yamaji, N., and Ma, J. F. (2020). Plant nutrition for human nutrition: hints from rice research and future perspectives. Mol. Plant 13, 825–835. doi: 10.1016/j.molp.2020.05.007
Huang, Y.-K., Li, W.-F., Zhang, R.-Y., and Wang, X.-Y, eds (2018). “Diagnosis and control of sugarcane important diseases,” in Color Illustration of Diagnosis and Control for Modern Sugarcane Diseases, Pests, and Weeds (New York, NY: Springer), 1–103. doi: 10.1007/978-981-13-1319-6_1
Hushiarian, R., Yusof, N. A., and Dutse, S. W. (2013). Detection and control of Ganoderma boninense: strategies and perspectives. Springerplus 2, 1–12. doi: 10.1186/2193-1801-2-555
IRRI (2018). Regional Strategy for Southeast Asia 2020-2025. Philippines: International Rice Research Institute.
IRRI (2022). Rice Knowledge Bank. Philippines: International Rice Research Institute. Available online at: http://www.knowledgebank.irri.org/ (accessed May 12, 2022).
Ishikawa-Ishiwata, Y., and Furuya, J. (2022). “Economic evaluation and climate change adaptation measures for rice production in vietnam using a supply and demand model: special emphasis on the Mekong River Delta region in Vietnam,” in Interlocal Adaptations to Climate Change in East and Southeast Asia, eds T. Ito, M. Tamura, A. Kotera, and Y. Ishikawa-Ishiwata (Cham: Springer), 45–53. doi: 10.1007/978-3-030-81207-2_4
Iskandar, M. J., Baharum, A., Anuar, F. H., and Othaman, R. (2018). Palm oil industry in South East Asia and the effluent treatment technology—a review. Environ. Technol. Innov. 9, 169–185. doi: 10.1016/j.eti.2017.11.003
Jamil, F. N., Tang, C.-N., Saidi, N. B., Lai, K.-S., and Baharum, N. A. (2019). “Fusarium wilt in banana: epidemics and management strategies,” in Horticultural Crops, eds H. K. Baimey, N. Hamamouch, and Y. A. Kolombia (London: IntechOpen), 229–331.
Jankar, K., Mane, S., Tatte, R., and Kose, P. (2020). Plant disease management strategies under changing climate scenario. J. Pharmacogn. Phytochem. 9, 950–954.
Jay, R., Carolyne, D., Andr,é, A., Marie-Hélène, R., Jean-Pierre, S., Vincent, C., et al. (2017). Integrated management of damping-off diseases. A review. Agron. Sustain. Dev. 37, 10–35. doi: 10.1007/s13593-017-0417-y
Jiang, N., Yan, J., Liang, Y., Shi, Y., He, Z., Wu, Y., et al. (2020). Resistance genes and their interactions with bacterial blight/leaf streak pathogens (Xanthomonas oryzae) in rice (Oryza sativa L.)—an updated review. Rice 13, 1–12. doi: 10.1186/s12284-019-0358-y
Jinji, P., Xin, Z., Yangxian, Q., Yixian, X., Huiqiang, Z., He, Z., et al. (2007). First record of Corynespora leaf fall disease of Hevea rubber tree in China. Australas. Plant Dis. Notes 2, 35–36. doi: 10.1071/DN07017
Joy, P. P., and Sindhu, G. (2012). Diseases of Pineapple (Ananas comosus) – Pathogens, Symptoms, Infection, spread and Management Pineapple Research Station (Kerala Agricultural University): Kerala Agricultural University. Available online at: https://kau.in/sites/default/files/documents/diseases_of_pineapple.pdf (accessed March 26, 2022).
Kado, C. I. (2003). Pink Disease of Pineapple. Saint Paul: The American Phytopathological Society. doi: 10.1094/APSnetFeature-2003-0303
Kaneshiro, W. S., Burger, M., Vine, B. G., De Silva, A. S., and Alvarez, A. M. (2008). Characterization of Erwinia chrysanthemi from a bacterial heart rot of pineapple outbreak in Hawaii. Plant Dis. 92, 1444–1450. doi: 10.1094/PDIS-92-10-1444
Keerthana Priya, R., Rawson, A., Vidhyalakshmi, R., and Jagan Mohan, R. (2022). Development of vegan sausage using banana floret (Musa paradisiaca) and jackfruit (Artocarpus heterophyllus Lam.) as a meat substitute: evaluation of textural, physico-chemical and sensory characteristics. J. Food Process. Preserv. 46, e16118. doi: 10.1111/jfpp.16118
Kenney-Lazar, M., and Ishikawa, N. (2019). Mega-plantations in southeast Asia: landscapes of displacement. Environ. Soc. 10, 63–82. doi: 10.3167/ares.2019.100105
Khonghintaisong, J., Songsri, P., and Jongrungklang, N. (2021). Understanding growth rate patterns among different drought resistant sugarcane cultivars during plant and ratoon crops encountered water deficit at early growth stage under natural field conditions. Agronomy 11, 2083. doi: 10.3390/agronomy11102083
Khoshkdaman, M., Mousanejad, S., Elahinia, S. A., Ebadi, A. A., and Padasht-Dehkaei, F. (2021). Sheath blight development and yield loss on rice in different epidemiological conditions. J. Plant Pathol. 103, 87–96. doi: 10.1007/s42161-020-00653-9
Korah, G., and Mohankumar, D. (2021). Black pepper price and its determinants: a panel data analysis using different estimators. J. Contemp. Issues Bus. Gov. 27, 383. doi: 10.47750/cibg.2021.27.03.052
Lee, T., and Hansen, J. (2019). Southeast Asia's Growing Meat Demand and its Implications for Feedstuffs Imports. Amber Waves: The Economics of Food, Farming, Natural Resources, and Rural America.
Lefevere, H., Bauters, L., and Gheysen, G. (2020). Salicylic acid biosynthesis in plants. Front. Plant Sci. 11, 338. doi: 10.3389/fpls.2020.00338
Leftwich, P. T., Spurgin, L. G., Harvey-Samuel, T., Thomas, C. J., Paladino, L. C., Edgington, M. P., et al. (2021). Genetic pest management and the background genetics of release strains. Philos. Trans. R. Soc. B 376, 20190805. doi: 10.1098/rstb.2019.0805
Legaz, M. E., Sánchez-Elordi, E., Santiago, R., De Armas, R., Fontaniella, R., Millanes, B., et al. (2018). “Metabolic responses of sugarcane plants upon different plant-pathogen interactions,” in Plant Metabolites and Regulation Under Environmental Stress, eds P. Ahmad, M. A. Ahanger, V. P. Singh, D. K. Tripathi, P. Alam, and M. N. Alyemeni (Amsterdam: Elsevier), 241–280. doi: 10.1016/B978-0-12-812689-9.00013-3
Lim, T., and Sangchote, S. (2003). 10 Diseases of Durian. Dis. Trop. Fruit Crops 241, 241–251. doi: 10.1079/9780851993904.0241
Liu, D., Chen, X., Liu, J., Ye, J., and Guo, Z. (2012). The rice ERF transcription factor OsERF922 negatively regulates resistance to Magnaporthe oryzae and salt tolerance. J. Exp. Bot. 63, 3899–3911. doi: 10.1093/jxb/ers079
Liyanage, K., Khan, S., Mortimer, P., Hyde, K., Xu, J., Brooks, S., et al. (2016). Powdery mildew disease of rubber tree. For. Pathol. 46, 90–103. doi: 10.1111/efp.12271
Liyanage, K. K., Khan, S., Brooks, S., Mortimer, P. E., Karunarathna, S. C., Xu, J., et al. (2018). Morpho-molecular characterization of two Ampelomyces spp. (Pleosporales) strains mycoparasites of powdery mildew of Hevea brasiliensis. Front. Microbiol. 9, 12. doi: 10.3389/fmicb.2018.00012
MAFI. (2021). Executive Summary National Agrofood Policy 2021-2030 (NAP 2, 0.) - Agrofood Modernisation: Safeguarding the Future of National Food Security, ed. P.a.S.P. Division. (Putrajaya, Malaysia: Ministry of Agriculture and Food Industries of Malaysia).
Maluin, F. N., Hussein, M. Z., and Idris, A. S. (2020). An overview of the oil palm industry: challenges and some emerging opportunities for nanotechnology development. Agronomy 10, 356. doi: 10.3390/agronomy10030356
Mazlan, S., Jaafar, N. M., Wahab, A., Rajandas, H., and Zulperi, D. (2019). Major diseases of rubber (Hevea brasiliensis) in Malaysia. Pertanika J. Sch. Res. Rev. 5, 10–21.
Mehta, S., Singh, B., Dhakate, P., Rahman, M., and Islam, M. A. (2019). “Rice, marker-assisted breeding, and disease resistance,” in Disease Resistance in Crop Plants, ed S. H. Wani (New York, NY: Springer), 83–111. doi: 10.1007/978-3-030-20728-1_5
Mensah, E. O., Asare, R., Vaast, P., Amoatey, C. A., Markussen, B., Owusu, K., et al. (2022). Limited effects of shade on physiological performances of cocoa (Theobroma cacao L.) under elevated temperature. Environ. Exp. Bot. 201, 104983. doi: 10.1016/j.envexpbot.2022.104983
Mentlak, T. A., Kombrink, A., Shinya, T., Ryder, L. S., Otomo, I., Saitoh, H., et al. (2012). Effector-mediated suppression of chitin-triggered immunity by Magnaporthe oryzae is necessary for rice blast disease. Plant Cell 24, 322–335. doi: 10.1105/tpc.111.092957
Mohd-Azlan, J., Yong, J. Y., Hazzrol, N. N. M., Pengiran, P., Atong, A., Aziz, S. A., et al. (2022). Local hunting practices and perceptions regarding the distribution and ecological role of the Large Flying Fox (Chiroptera: Pteropodidae: Pteropus vampyrus) in western Sarawak, Malaysian Borneo. J. Threat. Taxa 14, 20387–20399. doi: 10.11609/jott.6977.14.1.20387-20399
Moreno, M. L., Kuwornu, J. K., and Szabo, S. (2020). Overview and constraints of the coconut supply chain in the Philippines. Int. J. Fruit Sci. 20, S524–S541. doi: 10.1080/15538362.2020.1746727
Muimba-Kankolongo, A. (2018). Food Crop Production by Smallholder Farmers in Southern Africa: Challenges and Opportunities for Improvement. Cambridge, MA: Academic Press. doi: 10.1016/B978-0-12-814383-4.00013-X
Murithi, H., Beed, F., Tukamuhabwa, P., Thomma, B., and Joosten, M. (2016). Soybean production in eastern and southern Africa and threat of yield loss due to soybean rust caused by Phakopsora pachyrhizi. Plant Pathol. 65, 176–188. doi: 10.1111/ppa.12457
Murphy, D. J., Goggin, K., and Paterson, R. R. M. (2021). Oil palm in the 2020s and beyond: challenges and solutions. CABI Agric. Biosci. 2, 1–22. doi: 10.1186/s43170-021-00058-3
Nair, K. P. (2020). The Geography of Black Pepper (Piper nigrum): The “King” of Spices–Volume 1. New York, NY: Springer Nature. doi: 10.1007/978-3-030-52865-2
Nair, P., Sasikumaran, S., and Pillai, V. (1987). Time of application of fungicides for control of anthracnose disease of pepper. Agric. Res. J. Kerala 25, 136–139.
Nelson, S. (2005a). Rhizopus Rot of Jackfruit. College of Tropical Agriculture and Human Resources (University of Hawai'i). Available online at: https://scholarspace.manoa.hawaii.edu/server/api/core/bitstreams/8233f00f-0614-4d51-93c2-b0d85b9b9920/content (accessed December 8, 2022).
Nelson, S. (2005b). Stem Bleeding of Coconut Palm. College of Tropical Agriculture and Human Resources (University of Hawai'i). Available online at: https://scholarspace.manoa.hawaii.edu/server/api/core/bitstreams/8656b6a1-a895-4839-8ab1-75dc7d2a8898/content (accessed December 8, 2022).
Nor Diana, M. I., Zulkepli, N. A., Siwar, C., and Zainol, M. R. (2022). Farmers' adaptation strategies to climate change in Southeast Asia: a systematic literature review. Sustainability 14, 3639. doi: 10.3390/su14063639
Nurulnahar, E., Adam, P., Mazidah, M., Roslan, I., and Rafii, Y. M. (2020). Rice blast disease in Malaysia: options for its control. J. Trop. Agric. Food Sci. 48, 11–23.
Ong, S., Loo, L., Pang, M., Tan, R., Teng, Y., Lou, X., et al. (2021). Decompartmentalisation as a simple color manipulation of plant-based marbling meat alternatives. Biomaterials 277, 121107. doi: 10.1016/j.biomaterials.2021.121107
Parthasarathy, S., Thiribhuvanamala, G., and Prabakar, K. (2020). Diseases of Field Crops and Their Management. Boca Raton, FL: CRC Press. doi: 10.1201/9781003084198
Pasuquin, J., Pampolino, M., Witt, C., Dobermann, A., Oberthür, T., Fisher, M. J., et al. (2014). Closing yield gaps in maize production in Southeast Asia through site-specific nutrient management. Field Crops Res. 156, 219–230. doi: 10.1016/j.fcr.2013.11.016
Paterson, R. R. M. (2019). Ganoderma boninense disease of oil palm to significantly reduce production after 2050 in Sumatra if projected climate change occurs. Microorganisms 7, 24. doi: 10.3390/microorganisms7010024
Paterson, R. R. M. (2021). Longitudinal trends of future suitable climate for conserving oil palm indicates refuges in tropical south-east Asia with comparisons to Africa and South America. Pac. Conserv. Biol. 28, 57–67. doi: 10.1071/PC20067
Paul, N. C., Park, S.-W., Liu, H., Choi, S., Ma, J., Maccready, J. S., et al. (2021). Plant and fungal genome editing to enhance plant disease resistance using the CRISPR/Cas9 system. Front. Plant Sci. 12, 700925. doi: 10.3389/fpls.2021.700925
Pieretti, I., Pesic, A., Petras, D., Royer, M., Süssmuth, R. D., Cociancich, S., et al. (2015). What makes Xanthomonas albilineans unique amongst xanthomonads? Front. Plant Sci. 6, 289. doi: 10.3389/fpls.2015.00289
Pinizzotto, S., Aziz, A., Gitz, V., Sainte-Beuve, J., Nair, L., Gohet, E., et al. (2021). “Natural rubber systems and climate change,” in Proceedings and Extended Abstracts from the Online Workshop, 23–25 June 2020. Montpellier: CGIAR.
Ploetz, R. (2001). “Black sigatoka of banana. The Plant Health Instructor. Available online at: https://www.apsnet.org/edcenter/apsnetfeatures/Pages/BlackSigatoka.aspx (accessed October 28, 2022).
Pramanik, D., Shelake, R. M., Park, J., Kim, M. J., Hwang, I., Park, Y., et al. (2021). CRISPR/Cas9-mediated generation of pathogen-resistant tomato against tomato yellow leaf curl virus and powdery mildew. Int. J. Mol. Sci. 22, 1878. doi: 10.3390/ijms22041878
Prosekov, A. Y., and Ivanova, S. A. (2018). Food security: the challenge of the present. Geoforum 91, 73–77. doi: 10.1016/j.geoforum.2018.02.030
Rathore, R., Trivedi, A., and Mathur, K. (2002). “Rajasthan downy mildew of maize: the problem and management perspectives”, in: Proceedings of the 8th Asian Regional Maize Workshop, Bangkok. Thailand, 366–379.
Ray, J. D., Subandiyah, S., Rincon-Florez, V. A., Prakoso, A. B., Mudita, I., Carvalhais, L. C., et al. (2021). Geographic expansion of banana Blood disease in Southeast Asia. Plant Dis. 105, 2792–2800. doi: 10.1094/PDIS-01-21-0149-RE
Redfern, S. K., Azzu, N., and Binamira, J. S. (2012). “Rice in Southeast Asia: facing risks and vulnerabilities to respond to climate change,” in Build Resilience Adapt Climate Change Agri Sector, Vol. 23, (Rome), 1–14.
Reinhardt, D. H. R., Bartholomew, D. P., Souza, F. V. D., Carvalho, A. C. P. P. D., Pádua, T. R. P. D., Junghans, D. T., et al. (2018). Advances in pineapple plant propagation. Rev. Bras. Frutic. 40, 1–22. doi: 10.1590/0100-29452018302
Riajaya, P. D. (2021). Yields of promising sugarcane clones under three different planting arrangements. AGRIVITA J. Agric. Sci. 44, 119–129. doi: 10.17503/agrivita.v44i1.2797
Riajaya, P. D., Hariyono, B., Cholid, M., Kadarwati, F. T., and Santoso, B. (2022). Growth and yield potential of new sugarcane varieties during plant and first ratoon crops. Sustainability 14, 14396. doi: 10.3390/su142114396
Roberts, P. (2001). Anthracnose caused by Colletotrichum sp. on pepper. Gainesville, FL: University of Florida Cooperative Extension Service, Institute of Food and Agricultural Science.
Robertson, A. E., Jesse, L., Munkvold, G., Rojas, E. S., and Mueller, D. S. (2015). Physoderma brown spot and stalk rot of corn caused by Physoderma maydis in Iowa. Plant Health Prog. 16, 90–92. doi: 10.1094/PHP-BR-15-0003
Rott, P. (1995). Leaf Scald of Sugarcane. French Agricultural Research Centre for International Development (CIRAD). Available online at: https://agritrop.cirad.fr/389753/1/document_389753.pdf (accessed December 8, 2022).
Safari, S., Razali, N. A., Ibrahim, W. M. W., and Rahim, M. S. A. (2021). From farm to China: a case study of Malaysian frozen whole durian export supply chain. Econ. Technol. Manag. Rev. 16, 1–20.
Sharma, G., Singh, J., Arya, A., and Sharma, S. (2017). Biology and management of sugarcane red rot: a review. Plant Arch. 17, 775–784.
Sharma, R., De Leon, C., and Payak, M. (1993). Diseases of maize in South and South-East Asia: problems and progress. Crop Prot. 12, 414–422. doi: 10.1016/0261-2194(93)90002-Z
Siddiqui, Y., Surendran, A., Paterson, R. R. M., Ali, A., and Ahmad, K. (2021). Current strategies and perspectives in detection and control of basal stem rot of oil palm. Saudi J. Biol. Sci. 28, 2840–2849. doi: 10.1016/j.sjbs.2021.02.016
Sidhu, A. S. (2012). Jackfruit Improvement in the Asia-Pacific Region: A Status Report. Bangkok: APAARI.
Singh, P., Mazumdar, P., Harikrishna, J. A., and Babu, S. (2019). Sheath blight of rice: a review and identification of priorities for future research. Planta 250, 1387–1407. doi: 10.1007/s00425-019-03246-8
Sivapalan, A., Hj Hamdan, F., and Junaidy, M. (1997). Patch canker of Durio zibethinus caused by Phytophthora palmivora in Brunei Darussalam. Plant Dis. 81, 113–113. doi: 10.1094/PDIS.1997.81.1.113C
Solomon, S., and Li, Y.-R. (2016). The Sugar Industry of Asian Region. New York, NY: Springer. doi: 10.1007/s12355-016-0500-8
Strable, J., and Scanlon, M. J. (2009). Maize (Zea mays): a model organism for basic and applied research in plant biology. Cold Spring Harb. Protoc. 2009, pdb.emo132. doi: 10.1101/pdb.emo132
Subedi, S. (2015). A review on important maize diseases and their management in Nepal. J. Maize Res. Dev. 1, 28–52. doi: 10.3126/jmrd.v1i1.14242
Surendhar, M., Anbuselvam, Y., and Johnny, J. (2021). Status of rice brown spot (Helminthosporium oryza) management in India: a review. Agric. Rev. 43, 1–6. doi: 10.18805/ag.R-2111
Sweets, L. E., Wrather, J. A., and Wright, S. (2022). Soybean rust. MU Extension, University Of Missouri-Columbia. Available online at: https://mospace.umsystem.edu/xmlui/bitstream/handle/10355/91197/g4442.pdf?sequence=1&isAllowed=y (accessed December 8, 2022).
Syahruddin, K., Azrai, M., Nur, A., and Wu, W. (2020). “A review of maize production and breeding in Indonesia,” in: IOP Conference Series: Earth and Environmental Science. Bristol: IOP Publishing. doi: 10.1088/1755-1315/484/1/012040
Tang Ton, N., and Buu, B. C. (2011). “How to prevent the most serious diseases of black pepper (Piper nigrum L.)-a case study of Vietnam”, in: IPC Annual Meeting in Lombok, Indonesia.
Trebicki, P., and Finlay, K. (2019). Pests and Diseases Under Climate Change; its Threat to Food Security. Chichester: John Wiley and Sons Ltd. doi: 10.1002/9781119180661.ch11
Truong, N.-V., Burgess, L., and Liew, E. (2008). Prevalence and aetiology of Phytophthora foot rot of black pepper in Vietnam. Austrlas. Plant Pathol. 37, 431–442. doi: 10.1071/AP08034
Tumin, S., and Shaharudin, A. A. (2019). Banana: The world's Most Popular Fruit. Kuala Lumpur: Khazanah Research Institute, 1–13.
UN DESA (2017). World population projected to reach 9, 8. billion in 2050, and 11.2 billion in 2100. UN DESA| United Nations Department of economic and social affairs. Available online at: https://www.un.org (accessed October 28, 2022).
Viljoen, A., Ma, L.-J., and Molina, A. B. (2020). “Fusarium wilt (Panama disease) and monoculture banana production: resurgence of a century-old disease,” Emerging Plant Diseases and Global Food Security, eds J. B. Ristaino and A. Records (Minnesota: APS Press), 159–184. doi: 10.1094/9780890546383.008
Viswanathan, R. (2021). Red rot of Sugarcane (Colletotrichum falcatum Went) (Wallingford). doi: 10.1079/PAVSNNR202116023
Wahab, S. A., Zaki, N. M., Rasidi, N. M. F. M., Nor, N. M., and Wahab, S. (2022). The influence of knowledge management, knowledge workers and industrial revolution 4.0 towards competitiveness in the rubber-based industry: a literature review. Asian J. Res. Bus. Manag. 4, 122–135. doi: 10.55057/ajrbm.2022.4.1.11
Wang, T., Fang, J., and Zhang, J. (2022). Advances in sugarcane genomics and genetics. Sugar Tech. 24, 354–368. doi: 10.1007/s12355-021-01065-4
Wardhani, R., and Rahadian, Y. (2021). Sustainability strategy of Indonesian and Malaysian palm oil industry: a qualitative analysis. Sustain. Account. Manag. Policy J. 12, 1077–1107. doi: 10.1108/SAMPJ-07-2020-0259
Williams, P. A., Crespo, O., Atkinson, C. J., and Essegbey, G. O. (2017). Impact of climate variability on pineapple production in Ghana. Agric. Food Secur. 6, 1–14. doi: 10.1186/s40066-017-0104-x
Xu, K., and Mackill, D. J. (1996). A major locus for submergence tolerance mapped on rice chromosome 9. Mol. Breed. 2, 219–224. doi: 10.1007/BF00564199
Xu, K., Xu, X., Fukao, T., Canlas, P., Maghirang-Rodriguez, R., Heuer, S., et al. (2006). Sub1A is an ethylene-response-factor-like gene that confers submergence tolerance to rice. Nature 442, 705–708. doi: 10.1038/nature04920
Yu, F.-Y., Niu, X.-Q., Tang, Q.-H., Zhu, H., Song, W.-W., Qin, W.-Q., et al. (2012). First report of stem bleeding in coconut caused by Ceratocystis paradoxa in Hainan, China. Plant Dis. 96, 290–290. doi: 10.1094/PDIS-10-11-0840
Zakaria, S. N. S., and Mohd Noor, N. (2020). A review on major fungus associated with black pepper (Piper nigrum L.) diseases in Malaysia. Int. J. Sci. Eng. Res. 11, 319–324.
Zhang, H.-L., Ntambo, M. S., Rott, P. C., Chen, G., Chen, L.-L., Huang, M.-T., et al. (2020). Complete genome sequence reveals evolutionary and comparative genomic features of Xanthomonas albilineans causing sugarcane leaf scald. Microorganisms 8, 182. doi: 10.3390/microorganisms8020182
Zhang, J., and Su, F. (2020). Land use change in the major bays along the coast of the South China Sea in Southeast Asia from 1988 to 2018. Land 9, 30. doi: 10.3390/land9010030
Keywords: plant diseases, yield losses, biological control, plant management strategies, Southeast Asia (SEA)
Citation: Lim JA, Yaacob JS, Mohd Rasli SRA, Eyahmalay JE, El Enshasy HA and Zakaria MRS (2023) Mitigating the repercussions of climate change on diseases affecting important crop commodities in Southeast Asia, for food security and environmental sustainability—A review. Front. Sustain. Food Syst. 6:1030540. doi: 10.3389/fsufs.2022.1030540
Received: 29 August 2022; Accepted: 21 November 2022;
Published: 16 January 2023.
Edited by:
Beixin Mo, Shenzhen University, ChinaReviewed by:
Phetole Mangena, University of Limpopo, South AfricaRasappa Viswanathan, Indian Council of Agricultural Research (ICAR), India
Copyright © 2023 Lim, Yaacob, Mohd Rasli, Eyahmalay, El Enshasy and Zakaria. This is an open-access article distributed under the terms of the Creative Commons Attribution License (CC BY). The use, distribution or reproduction in other forums is permitted, provided the original author(s) and the copyright owner(s) are credited and that the original publication in this journal is cited, in accordance with accepted academic practice. No use, distribution or reproduction is permitted which does not comply with these terms.
*Correspondence: Jamilah Syafawati Yaacob, jamilahsyafawati@um.edu.my; Siti Rasila Ainaa Mohd Rasli, rasila@nutrition-technologies.com