- 1College of Agronomy, Federal University of Goiás, Goiânia, Brazil
- 2Doctorate and Master's Degree Graduation Program in Industrial Biotechnology, Universidade Positivo, Curitiba, Brazil
The aim of this study was to show how maltose production residues can be used to obtain natural pigments by Monascus ruber CCT 3802 in solid and submerged cultures. The microbial growth and the colour and heat stability characteristics of the pigments produced in both solid and submerged media, with different maltose syrup concentrations, were determined. The results showed that the addition of maltose provided significant increases in the velocity of microbial growth and production of red pigments. The highest radial growth velocity of Monascus ruber (0.1053 mm h−1) was obtained when cultivated in a medium containing 5 g L−1 of maltose syrup, corresponding to a 71.7% increase in growth as compared to the growth velocity in the control medium. Using submerged fermentation, the culture medium containing 10 g L−1 of maltose syrup provided the greatest concentrations of red pigments (14.54 AU510nm g−1 dry biomass) with an intense dark red colour, showing that Monascus ruber CCT 3802 had the capacity to assimilate the substrate and produce pigments. The red pigments produced in the cultures showed good heat stability with activation energies of 13.735 Kcal mol−1.
Introduction
Colour is one of the first sensory attributes used when choosing food. Although subjective, it is fundamental, since it influences consumer perception of other sensory characteristics such as aroma, flavour and texture (Spence, 2019). The transformation, modification and conservation operations applied during food processing cause changes in the colour (Paakki et al., 2019; Mehmood and Zeb, 2020), such that the industries have to intentionally add food additives such as dyestuffs to restore and/or highlight the sensory characteristics.
The search for foods containing no artificial additives has resulted in an increased production and application of natural pigments of vegetable, animal and microbial origin, to substitute the traditionally used artificial pigments (Sudhakar et al., 2016; Sen et al., 2019), and production, extraction and purification techniques have been developed to increase the productivity and sustainability of the natural pigments so obtained.
Pigment producing microorganisms can be cultivated under controlled conditions, independent of the environmental conditions (Kumar et al., 2015; Chatragadda and Dufossé, 2021). They show faster cell multiplication, can assimilate various substrates and nutrients and require little industrial area when compared to animals and vegetables (Cardoso et al., 2017; Heer and Sharma, 2017). Microbial pigments show greater light, temperature and pH stability, and, in general, are easily dissolved in water, to the contrary of vegetable origin pigments (Boo et al., 2012; Ramesh et al., 2019).
The genus Monascus stands out amongst the pigment producing microorganisms, producing yellow, orange and red pigments amongst other molecules of industrial interest (Seenivasan et al., 2020; Huang et al., 2021; Xu et al., 2021). These microorganisms are traditionally used as dyes in fermented foods, alcoholic beverages, rice, vegetables, meats and cheeses in countries such as China, Taiwan, Thailand, Japan, Indonesia and The Philippines (Ning et al., 2017; Sun et al., 2020; Xia et al., 2021).
Pigment production by Monascus species is usually carried out in solid media, but more recently the use of submerged culture has shown promise, with advantages such as a higher water content and more diluted nature of the culture medium and ease in controlling the pH and temperature, as well as guaranteeing nutrient homogeneity and having knowledge of the microbial kinetics behaviour (Costa et al., 2020; Ramesh et al., 2022; Liu et al., 2020).
The use of agro-industrial residues from the food industry is an opportunity to reduce the costs of producing microbial pigments (Usmani et al., 2020). Such substrates are attracting more and more attention, aimed at implementing the sustainability of industrial production (Costa et al., 2020), and the use of by-products from maltose production is an alternative for microbial pigment production. The objective of the work was the application of maltose residues to produce food pigments by Monascus ruber CCT 3802 and the determination of the thermal resistance of the pigments produced.
Materials and methods
Substrate
The maltose syrup used was donated by the Institute of Biological Phosphates (IFB) (Goiânia, Goiás, Brazil), and characterised according to Association of Official Analytical Chemists (2012). The moisture content was determined in an incubator (Tecnal TE-395, Piracicaba, Brazil) at 105°C to constant weight; the fixed mineral residue or ash content was determined in a muffler (EDG Forno Economic, São Carlos, Brazil) at 550 °C; the pH value using a potentiometer (Mettler Toledo, Brasília, Brazil); the total protein content quantified by the micro-Kjeldahl method with a conversion factor of 5.75 (Micro Kjeldahl Apparatus, Piracicaba, Brazil); the lipid content using the Soxhlet method (Nalgon, Brasília, Brazil); and the water activity (Aw) by direct reading in an Aqua Lab apparatus (Decagon Aqua Lab 3TE, São Paulo, Brazil). The reducing sugar content was determined using the 3,5-dinitrosalicylic acid method according to Miller (1959). All determinations were carried out in triplicate.
Microorganism
Monascus ruber CCT 3802 was obtained from the Tropical Culture Collection of the André Tosello Foundation (Campinas, São Paulo, Brazil) and maintained in malt extract agar (Nuclear, Brazil). Both test-tube slopes and Roux jars were inoculated, incubated at 30°C for 7 days and then maintained at 4°C.
Solid state cultivation
Radial growth
The radial growth velocity of Monascus ruber CCT 3802 colonies in MEA was determined in media containing different maltose syrup concentrations (g L−1) (1.0; 2.5; 5.0; 10.0; 20.0 and 40.0). The culture media were autoclaved at 121°C for 15 min, poured into 100 mm diameter Petri dishes and allowed to solidify before inoculation. The inoculum was prepared by transferring three loopfuls of Monascus ruber CCT 3802, cultivated in MEA slopes and then transferred to Duran tubes containing 1 mL of 0.2% (w/v) bacteriological agar previously autoclaved at 121 °C for 15 min, thus forming a spore suspension. The solidified agar in the Petri dishes was inoculated with the spore suspension with the aid of 1.4 mm sterile micropipette tips. The tips were immersed in the spore suspension and then allowed to gently touch the centre of the plate.
After inoculation, three streaks were drawn on the bottom of the plate passing through the inoculation point. The plates were incubated at 30 °C and the diameters of the Monascus ruber CCT 3802 colonies measured every 24 h using a digital calliper (Universal, SP, Brazil). The radial growth velocity of the colonies cultivated in different maltose syrup concentrations was determined from the slope of the linear regression presented in Equation 1.
where, r(t) is the radius of the colony (mm); a is the linear regression constant; VRG is the radial growth velocity (mm h−1) and t is the cultivation time (h). Each trial was repeated five times.
Pigment extraction and quantification
At the end of cultivation, the Monascus ruber CCT 3802 colonies were scraped from the MEA surface, placed in 100 mL conical flasks containing 30 mL of 95% ethyl alcohol, and incubated in a water bath (Spencer, São Paulo, Brazil) at 40 °C with shaking at 100 rpm, for 2 h. The pigments were obtained by filtration through Whatman no. 1 filtre paper (Madiston, England) and submitted to scanning between 350 and 550 nm in a spectrophotometer. The yellow, orange and red pigments were quantified at the wavelengths of 400, 470, and 510 nm, respectively.
Biomass quantification
The Monascus ruber CCT 3802 colonies retained on the filtre paper were placed in 250 mL conical flasks containing 50 mL distilled water and autoclaved at 121°C for 5 min to completely dissolve the malt extract agar. The biomass was obtained by filtering through previously dried and weighed Whatman no. 1 filtre paper (Madiston, England), drying at 85°C for 24 h, cooling in a desiccator and weighing on an analytical balance. All determinations were carried out in quintuplet.
Submerged cultivation
Culture medium and preparation of inoculum
The culture medium was composed of (g L−1): 5.0 glycine; 5.0 K2HPO4; 5.0 KH2PO4; 0.1 CaCl2; 0.5 MgSO4.7H2O; 0.01 FeSO4.7H2O; 0.01 ZnSO4.7H2O and 0.03 MnSO4.H2O. The malt extract was added at concentrations of 10.0 to 50.0 g L−1. The cultures were prepared in 500 mL conical flasks containing 200 mL culture media, and inoculated with 10% (v/v) of spore solution (~ 4 x 106 spores mL−1). The initial pH value was adjusted to 6.5 using NaOH solutions and the flasks incubated in an orbital shaker (NI 1714 Shaker, São Paulo, Brazil) at 30 °C and 120 rpm. The cultivations took a mean time of 192 h, and 15 mL aliquots were removed every 24 h to determine the quantities of biomass, pigments and reducing sugars, and determine the CIELAB parameters.
Pigment quantification
Fifteen millilitre aliquots of culture media were filtered through Whatman no. 1 filtre paper (Madiston, England) and the filtrate scanned from 350 to 550 nm in a spectrophotometer (Cirrus 80, Piracicaba, Brazil). The yellow, orange and red pigments were quantified at 400, 470, and 510 nm, respectively.
Biomass quantification
Fifteen millilitre aliquots of culture media were filtered through Whatman no. 1 filtre paper (Madiston, England). The biomass retained was dried at 85°C for 24 h, cooled in a desiccator and weighed on an analytical balance.
Reducing sugar quantification
The reducing sugars were quantified in a spectrophotometer (Moltox, São Paulo, Brazil) using the 3,5-dinitrosalicylic acid method according to Miller (1959).
Determination of the colour parameters
The colour parameters L*, a* and b* were determined by a direct reading of the total transmittance in a colorimeter (ColorQuest® XE, HunterLab, Virginia, USA) using the CIELAB colour system. The saturation index or Chroma (C*) and the hue angle (hab) were determined from equations 2 and 3, respectively:
Thermal stability
The thermal stability of the red pigments was determined in a 500 mL jacketed glass flask containing 200 mL of pigment solution with the absorbance adjusted to 1 AU510nm. The thermal degradation constant (DC) was determined using the first order kinetic model expressed by equation 4.
where A is the absorbance (AU510nm), t the time (h) and DC the thermal degradation constant (h−1). Equation 4 can be linearized using the contour conditions: A=A0 when t=0 and A=A when t=t; resulting in equation 5.
where A is the absorbance at time t (AU510nm) and A0 is the initial absorbance (AU510nm). The half-life time (t1/2) was determined using equation 6, where A/A0 is equal to 2.
The activation energy (Ea) was determined using the Arrhenius model as shown in equation 7.
where Ea is the activation energy (Kcal mol−1), D0 the pre-exponential factor (h−1), R the universal gas constant (cal mol−1 K−1) and Te the temperature (K). Equation 8 was obtained by linearizing the Arrhenius model.
The angular coefficient of the linear regression of the natural logarithm of DC as a function of the inverse of the absolute temperature (in Kelvin degrees) multiplied by the ideal natural gas constant (1.987 cal mol−1 K−1), represents the value of the activation energy.
Statistical analysis
The analysis of variance (ANOVA) was used to analyse the data and the minimal significant differences determined using Tukey's test at a significance level of 5% (p < 0.05) using the software STATISTICA 7.1. The Microsoft Excel® 2010 software was used to elaborate the graphs.
Results and discussion
Table 1 shows the composition of the substrate used in the experiments, characterised as high maltose content syrup (30 to 70 g L−1 of maltose). Figure 1 shows the radial growth curves of the M. ruber colonies cultivated in solid media containing different maltose syrup concentrations, and the visual aspect of these colonies can be seen in Figure 2. There was a gradual increase in growth of the Monascus ruber CCT 3802 colonies in the culture media containing maltose syrup as compared to the control (p ≤ 0.05). The addition of 5 g L−1 of maltose syrup resulted in higher radial growth velocities (0.1053 ± 0.0017 mm h−1), representing an increase of 71.7% as compared to the control trial (Table 2). The radial growth velocities of the colonies cultivated in culture media containing 10 to 40 g L−1 of maltose syrup showed a slight reduction as compared to the culture medium containing 5 g L−1, but still higher than that of the control experiment. This behaviour can be explained by the reduction in the water activity caused by the addition of more maltose syrup.
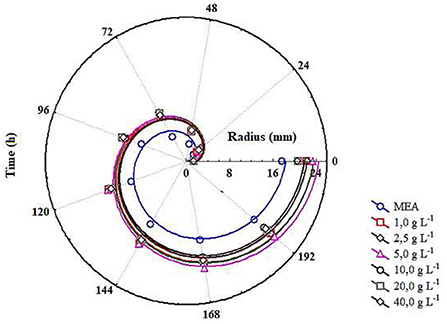
Figure 1. Radial growth curves of the colonies of Monascus ruber CCT 3802 grown in different concentrations of maltose.
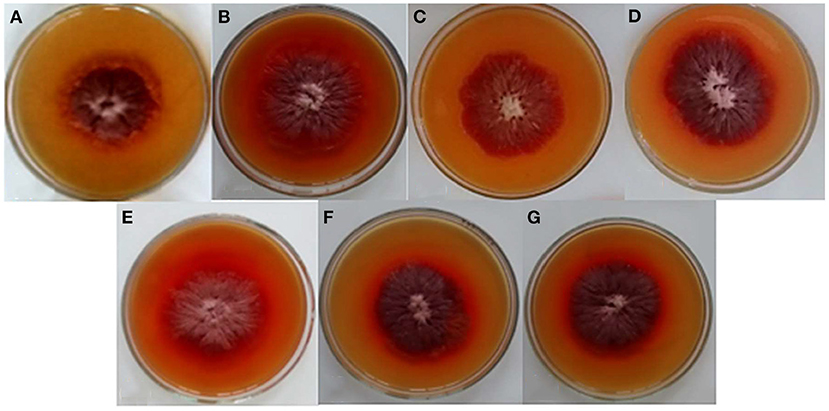
Figure 2. Visual aspect of the colonies of Monascus ruber CCT 3802 grown in different concentrations of maltose syrup. (A) MEA; (B) 1.0 g L−1 of maltose syrup; (C) 2.5 g L−1 of maltose syrup; (D) 5.0 g L−1 maltose syrup; (E) 10.0 g L−1 of maltose syrup; (F) 20.0 g L−1 of maltose syrup and (G) 40.0 g L−1 of maltose syrup.
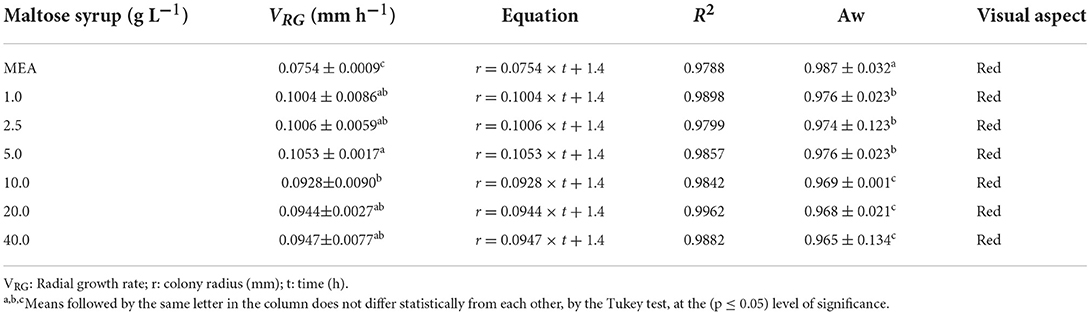
Table 2. Radial growth rate (VRG), equation of the colonies radius as a function of cultivation time, coefficient of determination (R2), water activity (Aw) and visual colour aspect of the colonies of Monascus ruber CCT 3802 grown in different concentrations of maltose syrup.
The radial growth velocity of the colonies is an indication of their capacity to assimilate and metabolise the nutrients present in the culture medium. The Monascus species used by Oliveira et al. (2016) presented radial growth velocities of 0.1383 mm h−1 when cultivated in a medium containing malt bagasse, and the species used by Carvalho et al. (2005) presented radial growth velocities between 0.095 and 0.129 mm h−1 when cultivated in culture media containing rice and cassava.
The pigments produced by the Monascus ruber colonies were extracted and quantified using a spectrophotometer and the cells used to quantify the biomass. The scans showed absorbance peaks in the regions of 400 and 510 nm, indicating the presence of yellow and red pigments (Figure 3), results corroborated by the results of the colour analysis shown in Table 3. It can be seen that the pigments produced by Monascus ruber cultivated in culture media containing maltose presented smaller values for L* and larger values for a*, b*, C and hab when compared to the control, showing that the addition of syrup, in addition to increasing the pigment concentration, intensified the red colour of the pigments.
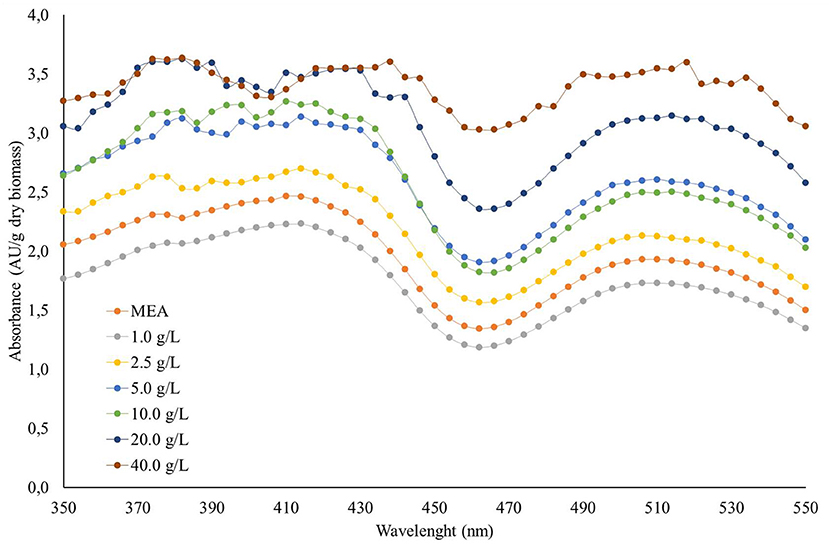
Figure 3. Scanning spectrum of pigments produced by the colonies of Monascus ruber CCT 3802 grown in different concentrations of maltose syrup.
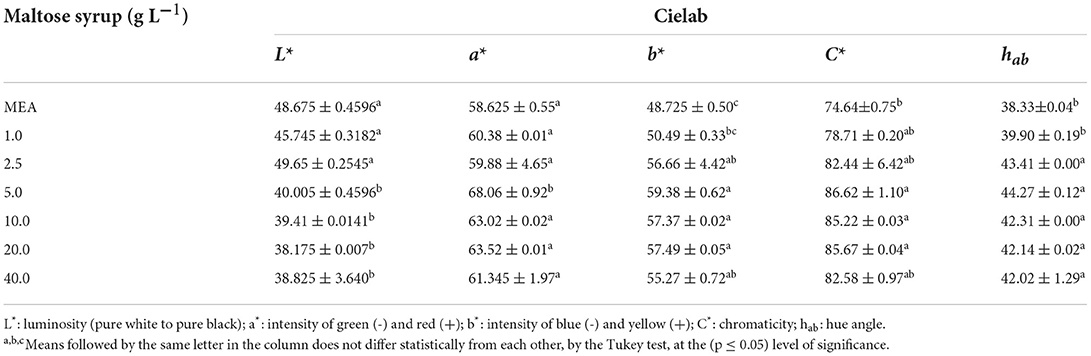
Table 3. Mean and standard deviation of the colour parameters of the pigments produced by Monascus ruber CCT 3802 grown in different concentrations of maltose syrup.
Since the addition of maltose to the solid culture media presented positive effects on the growth and red pigment production, it was decided to study the behaviour of Monascus ruber in submerged cultures. The cultures showed a 24-h lag phase and pigment production was intensified between 48 and 120 h of cultivation, a behaviour justified by the fungus using the carbon and nitrogen sources in the initial growth phase, whereas the secondary metabolites were produced in the exponential and stationary phases. The culture media containing 40 and 50 g L−1 of maltose showed higher biomass concentrations of 8.29 and 9.32 g L−1, respectively (Figures 4A,B), and higher absorbance values were observed for the cultures developed in media containing 10 and 20 g L−1 of maltose syrup, reaching values of 14.54 and 12.47 AU510nm, respectively (Figure 4C). These results demonstrate that the addition of maltose syrup presented a significant effect on cell growth and pigment production, also showing that concentrations of up to 20 g L−1 intensified the production of pigments, even with smaller cell concentrations, demonstrating greater pigment production specific velocities.
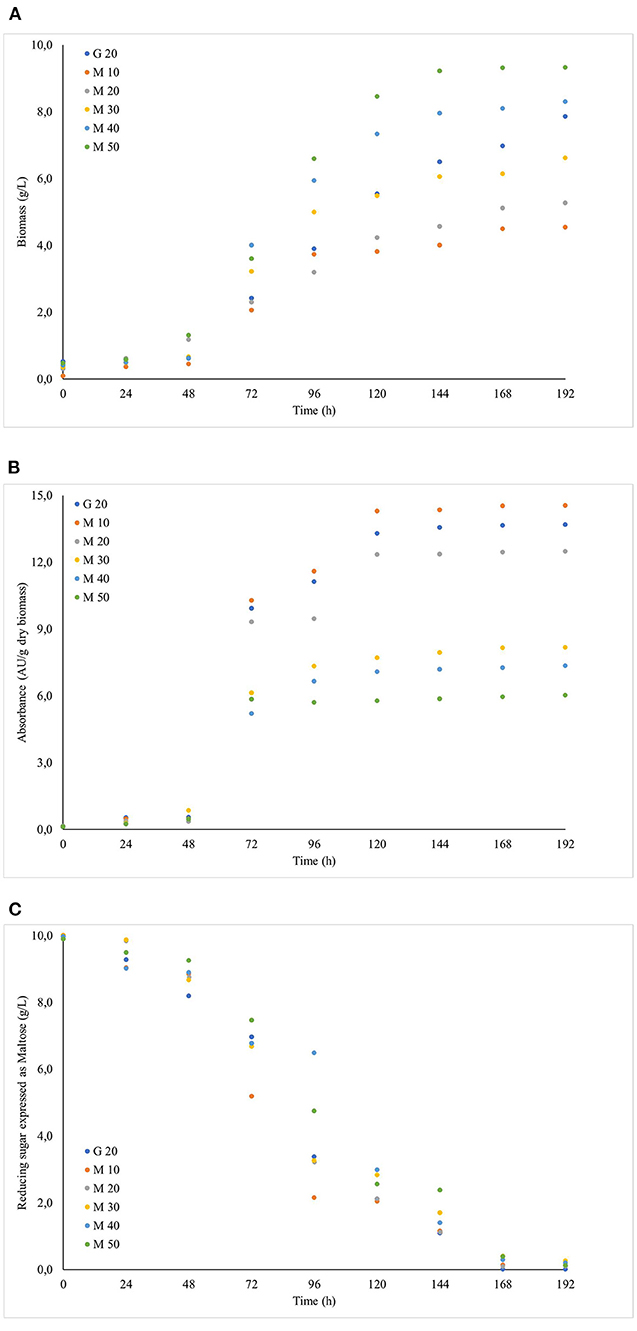
Figure 4. Evolution of the cultivation of Monascus ruber CCT 3802 grown in different concentrations of maltose syrup: (A) Biomass, (B) Reducing sugar and (C) Absorbance units.
Jiefeng et al. (2010) obtained maximum biomass production of 4.4 g L−1 and 1.6 AU of red pigments in flasks containing glucose as the substrate, shaken at 120 rpm. Meinicke et al. (2012) evaluated the potential of glycerol for the production of red pigments by Monascus ruber in submerged fermentation and obtained a maximum production of 7.38 AU with 8.34 g L−1 of biomass. Thus it was concluded that the biotechnological production of molecules with greater value, such as red pigments, is possible using agro-industrial residues as the substrates.
Table 4 shows the CIELAB colour parameters of the pigments produced by Monascus ruber CCT 3802 with different maltose syrup concentrations, and Figure 5 shows some images of the cultures. It can be seen that all the responses increased with increase in maltose concentration, indicating a positive effect on the pigment colour intensity. Smaller maltose concentrations favoured the production of larger amounts of pigments, but with less colour intensity.
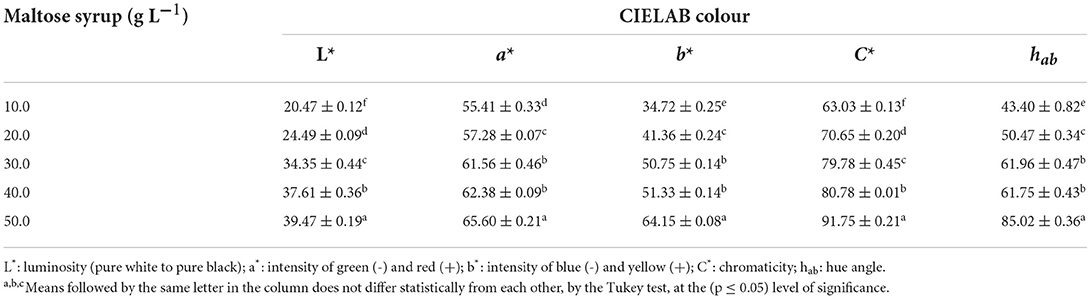
Table 4. CIELAB colour parameters of the pigments produced by Monascus ruber CCT 3802 grown in different concentrations of maltose syrup.
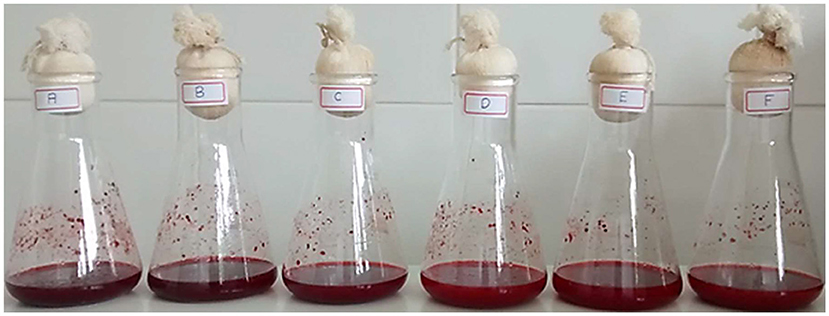
Figure 5. Pigment production in shaken flasks in 192 h of cultivation from Monascus ruber CCT 3802 cultures in shaken flasks in different concentrations of maltose syrup. (A) 20 g L−1 of glucose; (B) 10 g L−1 maltose syrup; (C) 20 g L−1 maltose syrup; (D) 30 g L−1 maltose syrup; (E) 40 g L−1 maltose syrup and (F) 50 g L−1 maltose syrup.
The pigments produced in submerged culture were submitted to a determination of their thermal stability by obtaining the thermal degradation constant (DC), half-life time (t1/2) and activation energy (Ea). Figure 6 shows the behaviour of ln (A/A0) as a function of the thermal treatment time at each temperature applied, and Table 5 shows the responses obtained for DC and t1/2. As expected, the exposure time and increase in temperature showed an effect on the colour degradation of the pigments according to a first order model, with a linear coefficient between 0.97 and 0.99. The thermal degradation constant of the pigments increased from 0.0008 h−1 at 50 °C to 0.0084 h−1 at 90 °C, showing an approximately 10-times increase. The experimental data were shown to adjust to the Arrhenius model with an R2 of 0.9901 (Figure 7), and an Ea of 13.7355 Kcal mol was obtained by multiplying the angular coefficient of the linear regression by the ideal gas constant of 1.987 cal mol−1 K−1. Levenspiel (1986) pointed out that reactions with high activation energies are much more temperature sensitive and that the Arrhenius model is the first adequate approximation to determine the effect of temperature on the kinetic equation, as well as adjusting the experimental data well in a wide temperature range, a behaviour verified in the present study.
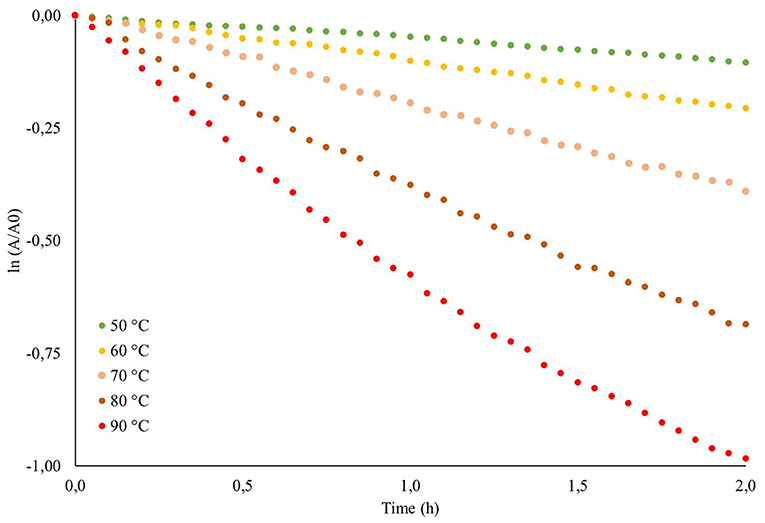
Figure 6. ln(A/A0) of the red pigments produced in the submerged cultivation from Monascus ruber CCT 3802 as a function of the heat treatment time under different temperatures.
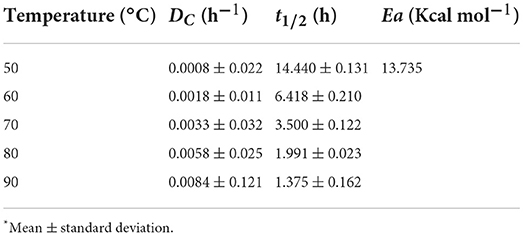
Table 5. DC, t1/2 and Ea of the red pigments produced in cultivation submerged by Monascus ruber CCT 3802 under different heat treatments.
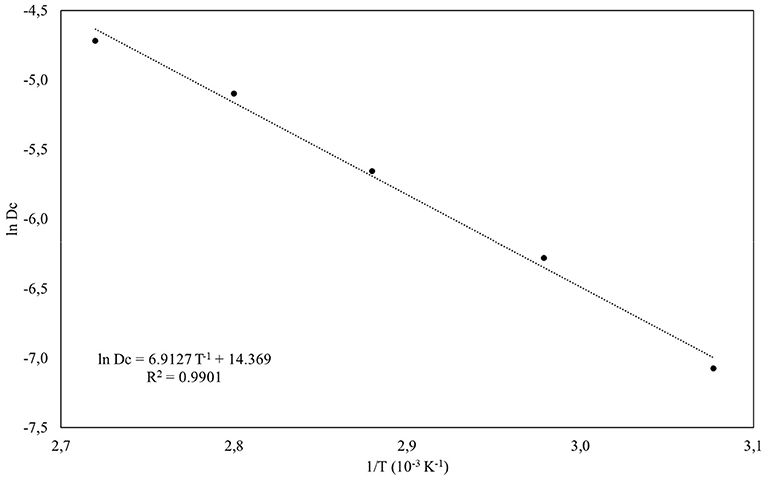
Figure 7. Activation energy (Ea) stimation of the pigments produced by Monascus ruber CCT 3802 in submerged culture by Arrhenius model.
The temperature, pH, presence of oxygen and other factors interfere directly in the stability of natural pigments. Knowledge and quantification concerning the action mode and intensity of these factors would contribute to obtaining information about the production conditions, extraction, conservation and application of this molecule in a way that would maintain its properties. Vendruscolo et al. (2013) developed stability studies concerning the red pigments produced by Monascus sp. and reported values for DC and t1/2 of 0.171 h−1 and 4.104 h, respectively, when determined at 90 °C and pH 6.5, whereas when determined at pH 4.5 the values were 0.947 h−1 and 0.739 h, indicating a reduction in the stability. This shows that the pigments obtained in the present study were less susceptible to thermal degradation. Similar behaviour was observed by Jung et al. (2005) for red pigments and by Ou et al. (2009) for monacolin k, both studies being developed with Monascus sp.
Conclusion
The addition of maltose syrup as a substrate exerted a significant influence on the microbial growth and red pigment production in both solid and submerged cultures, showing that this substrate could be used to obtain pigments produced by Monascus species. The results indicated that Monascus assimilated the nutrients from the culture medium with the production of red pigments in the two cultivation methods used. The red pigments produced showed good thermal stability and could therefore be applied in processes involving heating. The red pigments produced in this study were less susceptible to degradation at 90 °C than others reported in the literature.
Data availability statement
The original contributions presented in the study are included in the article/supplementary material, further inquiries can be directed to the corresponding author.
Author contributions
CO carried out the experiments. FV conceived and designed the experiments, contributed with the reagents, material, analytical tools, and supervised the trials. LC and CO analyzed and interpreted the data. LC and FV wrote the paper. All authors contributed to the article and approved the submitted version.
Acknowledgments
The authors are grateful to the Coordenação de Aperfeiçoamento de Pessoal de Nível Superior (CAPES – Committee for Professional Training of Higher Education Staff), for the research scholarship granted.
Conflict of interest
The authors declare that the research was conducted in the absence of any commercial or financial relationships that could be construed as a potential conflict of interest.
Publisher's note
All claims expressed in this article are solely those of the authors and do not necessarily represent those of their affiliated organizations, or those of the publisher, the editors and the reviewers. Any product that may be evaluated in this article, or claim that may be made by its manufacturer, is not guaranteed or endorsed by the publisher.
References
Association of Official Analytical Chemists. (2012). Official Methods of Analysis of the Association of Official Analytical Chemists, 16th Edn. Washington: AOAC. p. 850.
Boo, H. O., Hwang, S. J., Bae, C. S., Park, S. H., Heo, B. G., and Gorinstein, S. (2012). Extraction and characterization of some natural plant pigments. Ind. Crops Prod. 40, 129–135. doi: 10.1016/j.indcrop.2012.02.042
Cardoso, L. A. C., Kanno, K. Y. F., and Karp, S. G. (2017). Microbial production of carotenoids - a review. Afr. J. Biotechnol. 16, 139–146. doi: 10.5897/AJB2016.15763
Carvalho, J. C., Oishi, B. O., Pandey, A., and Soccol, C. R. (2005). Biopigments from monascus: strain selection, citrinin production and color stability. Braz. Arch Biol. Technol. 48, 885-894. doi: 10.1590/S1516-89132005000800004
Chatragadda, R., and Dufossé, L. (2021). Ecological and biotechnological aspects of pigmented microbes: a way forward in development of food and pharmaceutical grade pigments. Microorganisms. 9, 637. doi: 10.3390/microorganisms9030637
Costa, J. P. V., Oliveira, C. F. D., and Vendruscolo, F. (2020). Cheese whey as a potential substrate for Monascus pigments production. AIMS Agri. Food. 5, 785–798. doi: 10.3934/agrfood.2020.4.785
Heer, K., and Sharma, S. (2017). Microbial pigments as a natural color: a review. Int. J. Pharm. Sci. 8, 1913–1922.
Huang, Z. R., Chen, M., Guo, W. L., Li, T. T., Liu, B., Bai, W. D., et al. (2021). Monascus purpureus-fermented common buckwheat protects against dyslipidemia and non-alcoholic fatty liver disease through the regulation of liver metabolome and intestinal microbiome. Food Res. Int. 136, 109511, doi: 10.1016/j.foodres.2020.109511
Jiefeng, L., Yiran, R., and Shanjing, Y. (2010). Repeated-batch cultivation of encapsulated Monascus purpureus by polyelectrolyte complex for natural pigment production. Chin. J. Chem. Eng. 5, 1013–1017. doi: 10.1016/S1004-9541(09)60161-0
Jung, H., Kim, C., and Shin, C. S. (2005). Enhanced photostability of Monascus pigments derived with various amino acids via fermentation. J. Agric. Food Chem. 53, 7108–7114. doi: 10.1021/jf0510283
Kumar, A., Vishwakarma, H. S., Singh, J., Dwvedi, S., and Kumar, M. (2015). Microbial pigments: production and their applications in various industries. Int. J. Pharm. Chem. Biol. Sci. 5, 203–212.
Levenspiel, O. (1986). Ingenieria de las Reacciones Químicas. Editorial Reverté S. A., Barcelona, Espanã. p. 634.
Liu, J., Luo, Y., Guo, T., Tang, C., Chai, X., Zhao, W., et al. (2020). Cost-effective pigment production by Monascus purpureus using rice straw hydrolysate as substrate in submerged fermentation. J. Biosci. Bioeng. 129, 229–236. doi: 10.1016/j.jbiosc.2019.08.007
Mehmood, A., and Zeb, A. (2020). Effects of different cooking techniques on bioactive contents of leafy vegetables. Int J Gastron Food Sci. 22, 100246. doi: 10.1016/j.ijgfs.2020.100246
Meinicke, R. M., Vendruscolo, F., Moritz, D. E., Oliveira, D., Schmidell, W., Samohyl, R. W., et al. (2012). Potencial use of glycerol as substrate for the production of red pigments by Monascus ruber in submerged fermentation. Biocatal. Agric. Biotechnol. 1, 238–242. doi: 10.1016/j.bcab.2012.03.001
Miller, G. L. (1959). Use of dinitrosalicilic acid reagent for determination of reducing sugar. Anal. Chem. 31, 426–428. doi: 10.1021/ac60147a030
Ning, Z. Q., Cui, H., Xu, Y., Huang, Z. B., Tu, Z., and Li, Y. P. (2017). Deleting the citrinin biosynthesis-related gene, ctnE, to greatly reduce citrinin production in Monascus aurantiacus Li AS3.4384. Int. J. Food Microbiol. 241, 325–330. doi: 10.1016/j.ijfoodmicro.2016.11.004
Oliveira, C. F. D., Vendruscolo, F., Costa, J. P. V., and Araújo, W. D. B. (2016). Bagaço de malte como substrato para produção de biopigmentos produzidos por Monascus ruber CCT 3802. Revista de Agricultura Neotropical. 3, 6–9. doi: 10.32404/rean.v3i3.1200
Ou, H. P., Wang, C. C. R., and Lai, L. S. (2009). Thermal degradation kinetics analysis of monacolin K in Monascus-fermented products. Food Sci Technol. 42, 292–296. doi: 10.1016/j.lwt.2008.05.021
Paakki, M., Aaltojärvi, I., Sandell, M., and Hopia, A. (2019). The importance of the visual aesthetics of colours in food at a workday lunch. Int. J. Gastron. Food Sci. 16, 100131. doi: 10.1016/j.ijgfs.2018.12.001
Ramesh, C., Prasastha, V. R., Venkatachalam, M., and Dufoss,é, L. (2022). Natural substrates and culture conditions to produce pigments from potential microbes in submerged fermentation. Fermentation. 8, 460. doi: 10.3390/fermentation8090460
Ramesh, C., Vinithkumar, N. V., Kirubagaran, R., Venil, C. K., and Dufoss,é, L. (2019). Multifaceted applications of microbial pigments: current knowledge, challenges and future directions for Public Health implications. Microorganisms. 7, 186. doi: 10.3390/microorganisms7070186
Seenivasan, A., Satya, E. J., Sankar, P., Gummadi, S. N., Panda, T., and Venkateswarlu, C. (2020). Metabolic pathway analysis and dynamic macroscopic model development for lovastatin production by Monascus purpureus using metabolic footprinting concept. Biochem. Eng. J. 154, 107437. doi: 10.1016/j.bej.2019.107437
Sen, T., Barrow, C. J., and Deshmukh, S. K. (2019). Microbial pigments in the food indsutry – Challenges and the way forward. Front. Nutrition. 6, 7. doi: 10.3389/fnut.2019.00007
Spence, C. (2019). On the changing colour of food and drink. Int. J. Gastron. Food Sci. 17, 100161. doi: 10.1016/j.ijgfs.2019.100161
Sudhakar, P., Latha, P., and Reddy, P. V. (2016). “Chapter 15 - Plant pigments,” in Sudhakar, P., Latha, P., and Reddy, P. V.Phenotyping Crop Plants for Physiological and Biochemical Traits. p. 121–127. Academic Press. doi: 10.1016/B978-0-12-804073-7.00015-6
Sun, C., Wu, X., Chen, X., Li, X., Zheng, Z., and Jiang, S. (2020). Production and characterization of okara dietary fiber produced by fermentation with Monascus anka. Food Chem. 136, 126243. doi: 10.1016/j.foodchem.2020.126243
Usmani, Z., Sharma, M., Sudheer, S., Gupta, V. K., and Bhat, R. (2020). Engineered microbes for pigment production using waste biomass. Curr. Genom. 21, 80–95. doi: 10.2174/1389202921999200330152007
Vendruscolo, F., Muller, B. L., Moritz, D. E., Oliveira, D., Schmidell, W., and Ninow, J. L. (2013). Thermal stability of natural pigments produced by Monascus ruber in submerged fermentation. Biocatal. Agric. Biotechnol. 2, 278–284. doi: 10.1016/j.bcab.2013.03.008
Xia, Y., Yuan, R., Weng, S., Wang, G., Xiong, Z., Zhang, H., et al (2021). Proteolysis, lipolysis, texture and sensory properties of cheese ripened by Monascus fumeus. Food Res. Int. 137, 109657. doi: 10.1016/j.foodres.2020.109657
Keywords: microbial pigments, thermal stability, radial growth rate, monascus pigments, Monascus ruber
Citation: Oliveira CFD, Cardoso LAdC and Vendruscolo F (2022) Production of pigments by Monascus ruber CCT0302 in culture media containing maltose as substrate. Front. Sustain. Food Syst. 6:1029017. doi: 10.3389/fsufs.2022.1029017
Received: 26 August 2022; Accepted: 28 October 2022;
Published: 25 November 2022.
Edited by:
Laurent Dufossé, Université de la Réunion, FranceReviewed by:
Zhilong Wang, Shanghai Jiao Tong University, ChinaRamesh Chatragadda, Council of Scientific and Industrial Research (CSIR), India
Copyright © 2022 Oliveira, Cardoso and Vendruscolo. This is an open-access article distributed under the terms of the Creative Commons Attribution License (CC BY). The use, distribution or reproduction in other forums is permitted, provided the original author(s) and the copyright owner(s) are credited and that the original publication in this journal is cited, in accordance with accepted academic practice. No use, distribution or reproduction is permitted which does not comply with these terms.
*Correspondence: Francielo Vendruscolo, ZnJhbmNpZWxvdmVuZHJ1c2NvbG9AdWZnLmJy