- 1School of Food Science and Engineering, South China University of Technology, Guangzhou, China
- 2Key Laboratory of South Subtropical Fruit Tree Biology and Genetic Resource Utilization, Ministry of Agriculture and Rural Affairs, Guangdong Province Key Laboratory of Tropical and Subtropical Fruit Tree Research, Institute of Fruit Tree Research, Guangdong Academy of Agricultural Sciences, Guangzhou, China
Introduction: Development of functional food ingredients with anti-obesity is a growing interest in the global food industry. Plantain (Musa spp. AAB), a special type of cooking/starchy banana, is widely growing in African and Latin American countries. The flour made from unripe plantain pulp, which is considered as a natural source of indigestible carbohydrates such as resistant starch (RS), could be used in the formulation of diverse functional foods due to its anti-obesity properties. However, the mechanisms underlying the anti-obesity properties of plantain flour are not explored.
Methods: In this study, we investigated the changes in serum hormone levels, liver transcriptome profiles, and the modulation of gut microbiota in high-fat-fed Sprague-Dawley (SD) rats. The male SD rats were divided into six groups, viz. two control groups [non-obese (NC) or obese (OC)] which were not given the supplementation, one positive control (PC) group which received orlistat supplementation (60 mg/kg body weight/day), and three groups of obese rats which were supplemented with unripe plantain flour (UPF) at a dosage (body weight/day) of 1.25 g/kg (low-dose, LD), 2.50 g/kg (intermediate-dose, MD) or 5.0 g/kg (high-dose, HD).
Results and discussion: It was found that UPF supplementation could lower the insulin levels of the obese rats. Moreover, UPF supplementation had a positive impact on gut microbiota, decreasing the relative abundances of Blautia, Parasutterella and Fusicatenibacter which were closely related to obesity, and increasing the relative abundances of probiotics (Allobaculum, Romboutsia, Staphylococcus, and Bacteroides). The spearman correlation analysis revealed that UPF supplementation reduced the relative abundance of Parasutterella and possibly decreased the blood sugar levels, leading to a decrease in the relative abundances of Blautia and Fusicatenibacter and a subsequent decrease in insulin levels. Furthermore, transcriptomic analysis of the liver tissues displayed that the peroxisome proliferator activated receptor-1α (PPAR) and AMP-activated protein kinase (AMPK) signaling pathway genes (Pparaa, Cpt1a, Prkaa1, Prkab1, Prkaa2, and Ppargc1a) were upregulated in those groups supplemented with UPF. These results indicated that UPF could mediate the glucolipid metabolism in the obese rats. Taken together, our findings suggested that the anti-obesity properties of UPF could be achieved by decreasing the insulin levels, positive-regulating of the gut microbiota composition as well as altering gene expression related to glucolipid metabolism.
Introduction
Obesity is a major public health problem in both developed and developing countries. The number of overweight and obese adults is at a record high, with 39–40% of adults being overweight or obese, and the incidence of these conditions is increasing in adolescents (Ruiz et al., 2019; Thompson et al., 2022). It is well known that being overweight or obese harms people's health, and consequently leads to numerous diseases, such as diabetes and cardiovascular diseases (Guo et al., 2021). Despite a global focus on reducing obesity, many people are still overweight or obese. There are many strategies for obesity treatment, including behavior therapy, dietary changes, physical activity, pharmacotherapies, and bariatric surgery etc. (Cerdo et al., 2019). However, bariatric surgery is not available or suitable for the majority of people who are suffering from overweight or obesity, and for some individuals the pharmacotherapies are costly and may have adverse effects (Golden, 2017; Nguyen and Varela, 2017; Bluher, 2019). Nevertheless, a number of strategies are recommended to reduce the increasing prevalence of obesity, which included regular physical activity, and the changes in dietary and lifestyle habits (Manna and Jain, 2015). Evidence is increasing from studies that plant-based dietary patterns, which are rich in fruits, vegetables, and whole grains, are valuable in preventing various chronic diseases, including obesity (Medina-Remon et al., 2018).
Banana (including plantains) is cultivated in tropical, subtropical and contains several bioactive compounds, such as phenolics, carotenoids, biogenic amines and phytosterols (Anyasi et al., 2013). Utilization of banana as an ingredient in different food products exerts a beneficial effect on health (Singh et al., 2016). There are high levels of resistant starch in unripe bananas, and the dry fruit contains more than 50% resistant starch content. Studies have shown that unripe banana flour helps to reduce the risk of non-communicable diseases, such as obesity, hyperglycaemia, and hyperlipidaemia (Hoffmann Sardá et al., 2016). Previous studies have shown that banana flour (rich in resistant starch) and banana resistant starch were effective in alleviating obesity (Tan and Wang, 2018; Fu et al., 2021). However, the anti-obesity properties vary depending on the banana cultivated groups. Two cultivated groups namely Cavendish (Musa AAA) and Dajiao (Musa ABB), were previously reported to have anti-obesity properties (Tan, 2019).
Plantain (Musa spp. AAB), is a special type of cooking/starchy banana, which is widely growing in African and Latin American countries. Plantain flour is an alternative source of indigestible carbohydrates (Garcia-Valle et al., 2019); in addition, it is also recognized as the source with the highest resistant starch (RS) content (Almanza-benitez et al., 2015). It has been reported that plantain flour can be blended with common flours, such as rice, wheat, and maize, to prepare bread (Juarez-Garcia et al., 2006), gluten-free pasta (Patiño-Rodríguez et al., 2018; Patino-Rodriguez et al., 2019), and cookies (García-Solís et al., 2018; Sanchez-Rivera et al., 2019). In addition to being an energy source in many people's daily diet, plantain flour can also improve gastrointestinal disease symptoms, lower blood sugar, and alleviate obesity and diabetes (Falcomer et al., 2019). Studies have shown that gluten-free snacks with high dietary fiber content and low predicted glycemic index can be developed using the unripe plantain flours as the main ingredient, and these gluten-free snacks may also be used as an alternative to reduce excess weight and obesity problems in the general population and celiac community (Flores-Silva et al., 2015). In addition, amelioration of acute pancreatitis by unripe plantain could play a key role in its management of diabetes and related complications, which indicates the potential of unripe plantain in the management of renal and liver complications arising from diabetes mellitus (Eleazu and Okafor, 2015). Therefore, plantain has considerable potential for use in banana food development.
Detailed data on the anti-obesity properties of unripe plantain flour (UPF), including its influence on body weight, food intake, and biochemical indexes, have been previously obtained (Han et al., 2021) (Supplementary Table 1). Here, the effects of UPF on gut microorganisms and a correlation analysis along with transcriptomic data from the liver fat metabolic pathway are reported. Results from this research on the anti-obesity properties of UPF and its mechanisms of action provide fundamental data on a natural food associated with weight loss and will benefit health food development.
Materials and methods
Preparation of plantain flour
Green mature unripe plantain (Musa spp. AAB group, French Horn subgroup) fruits were provided by the Institute of Fruit Tree Research, Guangdong Academy of Agricultural Sciences. The unripe plantain fruits were thoroughly cleaned, peeled, cut into 3-5 mm thick uniform slices, and soaked in 0.5 g/L citric acid solution for 10 minutes; then, the sliced pulp was freeze-dried in a vacuum freeze drier for 72 h. The dried materials were ground into flour and finally strained through a sieve to separate the granulates (diameter < 100 μm). The nutritional components of the plantain flour were analyzed using the following methods: Resistant starch content was determined using resistant starch assay kits (Megazyme K-RSTCL; Megazyme International Ireland Ltd.,Wicklow, Ireland). The total dietary fiber content was determined using a combination of enzymatic and gravimetric procedures according to GB 5009.88. The protein content, fat, and moisture content of the plantain flour were determined using the Kjeldahl method, the Soxhlet extractor method, and the ambient pressure drying method, respectively. The ash content was measured using a combination of combustion and gravimetric procedures. The chemical profile of the plantain flour is shown in Table 1.
Animals and experimental design
Male Sprague–Dawley (SD) rats (110 ± 10 g, 6 weeks old) were purchased from Guangdong Medical Laboratory Animal Center with the laboratory animal license number SCXK 2018-0002. The animals were provided with a normal chow diet (NCD: 55% nitrogen-free extract, 18% crude protein, 10% water, 8% ash, 4% crude fat, 5% crude fiber, 1.8% calcium, and 1.2% phosphorus, with a total calorific value of 327.6 kcal/100 g) and a high-fat diet (HFD: containing 64% NCD, 15.0% lard, 15.0% sucrose, 5% casein, 0.6% calcium hydrogen phosphate, and 0.4% stone flour, with a total calorific value of 404.84 kcal/100 g). All animals were raised in the SPF experimental animal room (constant temperature 21 ± 2 °C, relative humidity 50 ± 10%, and a 12 h light–dark cycle) of the Experimental Animal Center of South China Agricultural University with free access to water and food.
The protocol and design of the animal experiment, conducted in accordance with the method described for weight loss functions in Annex 8 issued by the Food and Drug Administration of China (Administration, 2012), are shown in Figure 1. After 6 weeks of experimentation, all rats were anesthetized and killed after fasting for 12 h. Blood samples were collected via the abdominal aorta and centrifuged at 3,000 r/min for 15 min at 4°C to obtain serum, which was stored at −80 °C until further analysis. The liver and intestinal tract contents were also obtained and stored at −80°C until further use.
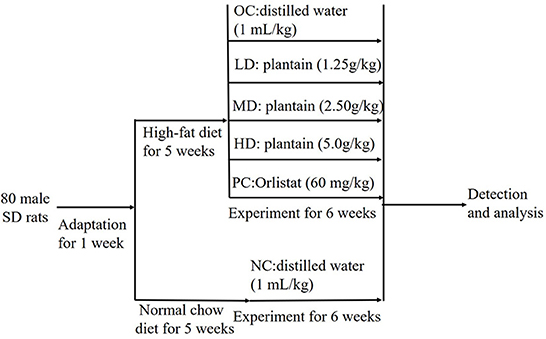
Figure 1. The protocol and design of animal experimental. NC, Normal control group; OC, Obesity control group; LD, Low-dose group; MD, Intermediate-dose group; HD, High-dose group; PC, Positive control group.
The animal study was reviewed and approved by the Experimental Animal Ethics Review Committee of South China Agricultural University. The ethical care and use of the laboratory animals were ensured according to the Guidelines for Animal Experimentation in Animal Research Laboratories.
Serum biochemistry
Serum leptin (LEP), insulin (INS), adiponectin (ADP), thyroxine (T4), and ghrelin levels were measured using ELISA kits (ColorfulGene Biological Technology Co., Ltd., Wuhan, China). All measurements were carried out according to the manufacturer's protocols.
DNA extraction and sequencing
DNA extraction and sequencing for the intestinal samples (n = 5) and the bioinformatics analysis were performed as previously described (Fu et al., 2021). Total genomic DNA from large intestine samples was extracted using the CTAB method, then, the V3–V4 hypervariable region of the microbiota 16S rRNA was amplified with the primers 341F (50- CCTAYGGGRBGCASCAG-30) and 806R (50-GGACTACNNGGGTATCTAAT-30). Purified amplicons were sequenced on an Ion S5TM XL platform (Thermo Scientific, MA, United States) by Nuohe Zhiyuan Technology Co., Ltd (Beijing, China).
Transcriptomics analysis
Total RNA was extracted from the liver tissue samples. RNA purity was assessed using a NanoPhotometer® spectrophotometer. Sequencing libraries were generated using the NEBNext® UltraTM RNA Library Prep Kit for Illumina® following the manufacturer's recommendations. The PCR products were purified, and the library quality was assessed. The library preparations were sequenced on an Illumina NovaSeq platform, and 150 bp paired-end reads were generated.
The reference genome index was built and paired-end clean reads were aligned to the reference genome using Hisat2 (v2.0.5). Differential expression analysis of the two conditions/groups (two biological replicates per condition) was performed using the DESeq2 R package (1.16.1). Gene Ontology (GO) enrichment analysis of differentially expressed genes was also conducted using the clusterProfiler R package. The clusterProfiler R package was used to test the statistical enrichment of differentially expressed genes according to Kyoto Encyclopedia of Genes and Genomes (KEGG) pathways.
Statistical analysis
Data are displayed as the mean ± standard deviation. One-way ANOVA and LSD tests were performed for significance analysis; the results were considered significant when p <0.05. All analyses were performed using IBM SPSS Statistics 22.0.
Results
Effects on the serum hormones of obese rats
The levels of serum hormones are shown in Table 2. The insulin levels were significantly higher in the OC group than the NC group (p < 0.05), which indicates that the HFD led to an increase in insulin secretion. After UPF intervention, the insulin levels were significantly lower in the LD and MD groups than the OC group (p<0.05). Unripe plantain has high levels of resistant starch, and many studies have shown that resistant starch has the ability to control the rise in the postprandial blood sugar level and reduce insulin secretion (Fuentes-Zaragoza et al., 2010). The differences in adiponectin between all groups were not significant. The ghrelin level was significantly higher in the OC group than the NC group (p<0.05), which indicated that the HFD may have led to increased ghrelin. As the dose of UPF was increased, the ghrelin levels in the three intervention groups decreased. This suggested that UPF possibly reduced ghrelin secretion. There were no significant differences in leptin and thyroxin levels between the OC and any of the UPF intervention groups. In summary, the insulin level in obese rats decreased after supplementation with UPF. This is in alignment with previously reported results showing a reduction in blood sugar and fat levels and the alleviation of liver function damage in obese rats following UPF intervention (Han et al., 2021).
Effects on intestinal flora
Diversity of the gut bacterial communities
Based on the alpha diversity analysis (Table 3), the values of Chao1 (p < 0.05) and ACE (p < 0.01) indexes were significantly reduced in the OC group compared with the NC group. However, the values of Shannon and Simpson indexes did not significantly different between these groups. It can be inferred that the obesity induced by an HFD may decrease the diversity of the gut microbiota. After UPF intervention, the Chao1 and ACE index values increased and showed in a dose-dependent manner. The values of Chao1 and ACE indexes increased significantly (p < 0.05) in the HD group compared with the OC group, indicating that UPF had the ability to increase the diversity of the intestinal flora.
In the beta diversity analysis (Figures 2A,B), the NC group showed a considerably different trend from that of the OC group, suggesting that the obesity induced by the HFD changed the overall structure of the gut microbiota. The HD and PC groups were clearly separated from the OC group (Figure 2A), whereas the LD and MD groups overlapped with the OC group. The three UPF intervention groups and the PC group were uniformly distributed between the NC and OC groups (Figure 2B). These results showed that the intestinal microbial community structure could be changed by UPF supplementation.
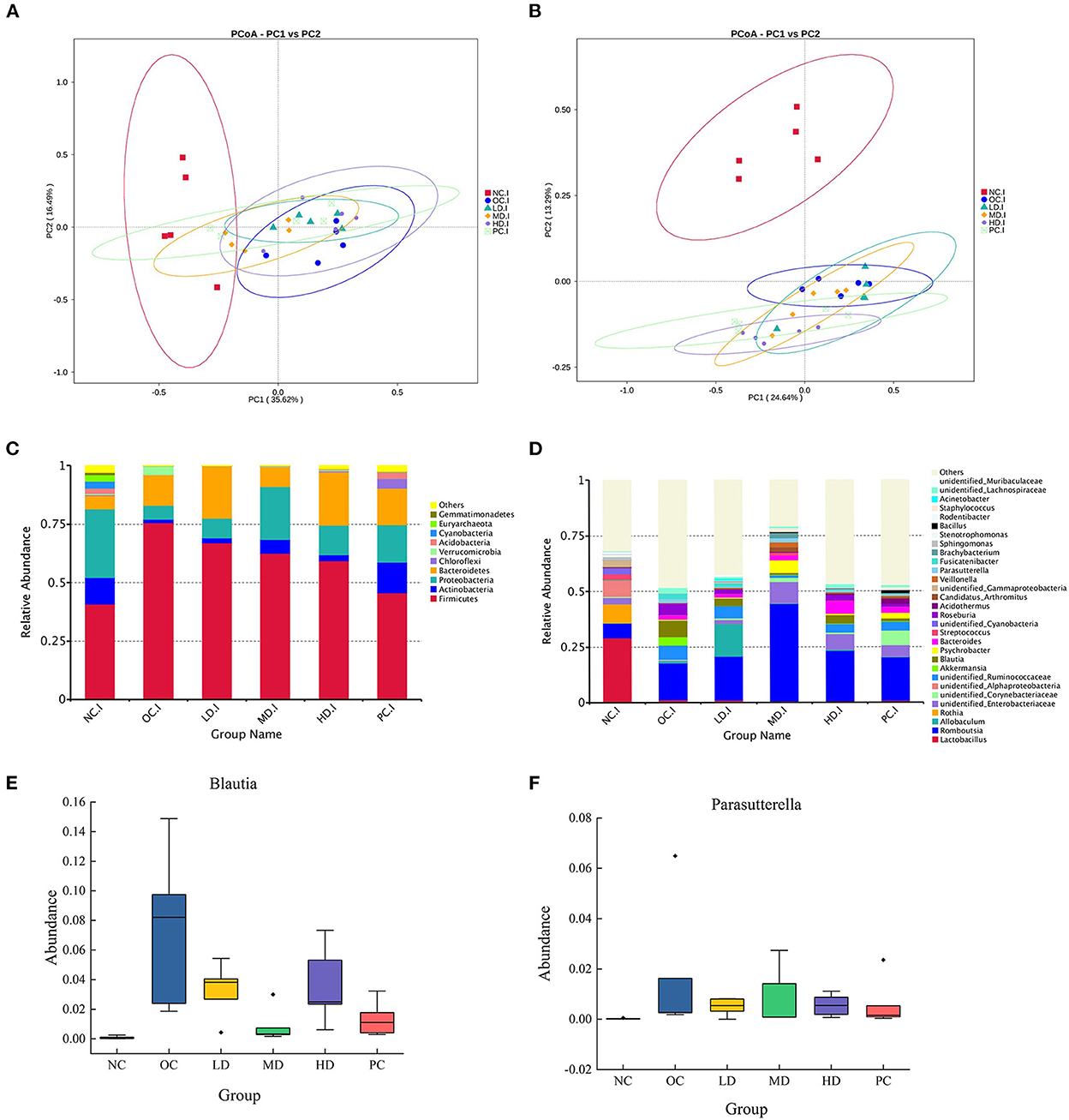
Figure 2. Plantain administration alters the composition of gut microbiota (A) principal co-ordinates analysis (PCoA) of gut microbiota based on Unweighted Unifrac distance (B) principal co-ordinates analysis (PCoA) based on Weighted Unifrac distance (C) relative abundance of gut microbiota at the phylum level (D) relative abundance of gut microbiota at the genus level (E) Blautia (F) Parasutterella. Differences were based on ANOVA analysis followed by Duncan test.
Composition and structure of the intestinal bacterial community
In this study, 35 phyla, 55 classes, 123 orders, 252 families, 620 genera, 449 species, and 4,350 OTUs were detected. The top 10 phyla in terms of relative abundance were Firmicutes (58.48%), Proteobacteria (15.86%), Bacteroidetes (14.86%), Actinobacteria (6.05%), Chloroflexi, Verrucomicrobia, Acidobacteria, Cyanobacteria, Euryarchaeota, and Gemmatimonadetes (Figure 2C). The relative abundance of Proteobacteria in the OC group (5.88%) was significantly (p<0.01) lower than that in the NC group (36.48%). In the MD group, the relative abundance of Proteobacteria was 22.50%, while in the HD group, the value was 17.37%, showing that the relative abundance of Proteobacteria was increased by UPF feeding (Figure 2C).
As shown in Figures 2D, 3A, the bacterial abundance profiles of the UPF group significantly differed from that of the OC group, showing that UPF intervention also improved the gut microbial composition at the genus level. The dominant bacteria in the NC group were Lactobacillus and Rothia (Figure 3A), with relative abundances of 29.15 and 8.60%, respectively. However, the abundance of these two genera was sharply decreased by the HFD. Lactobacillus and Rothia are probiotics (Liévin-Le Moal and Servin Alain, 2014; Qian et al., 2018); thus, it was deduced that the proportion of beneficial microorganisms was reduced by the HFD. In the OC group, Blautia and Parasutterella (Figures 2E,F) were found to have high relative abundances. It can be seen from Table 4 that the relative abundance of Allobaculum increased significantly in the LD group (p < 0.05), while the relative abundances of Romboutsia (p < 0.01) and Staphylococcus (p < 0.05) increased significantly in the MD group. Interestingly, Fusicatenibacter was not detected in the NC group but was detected in the OC group, with a relative abundance of 6.03%.
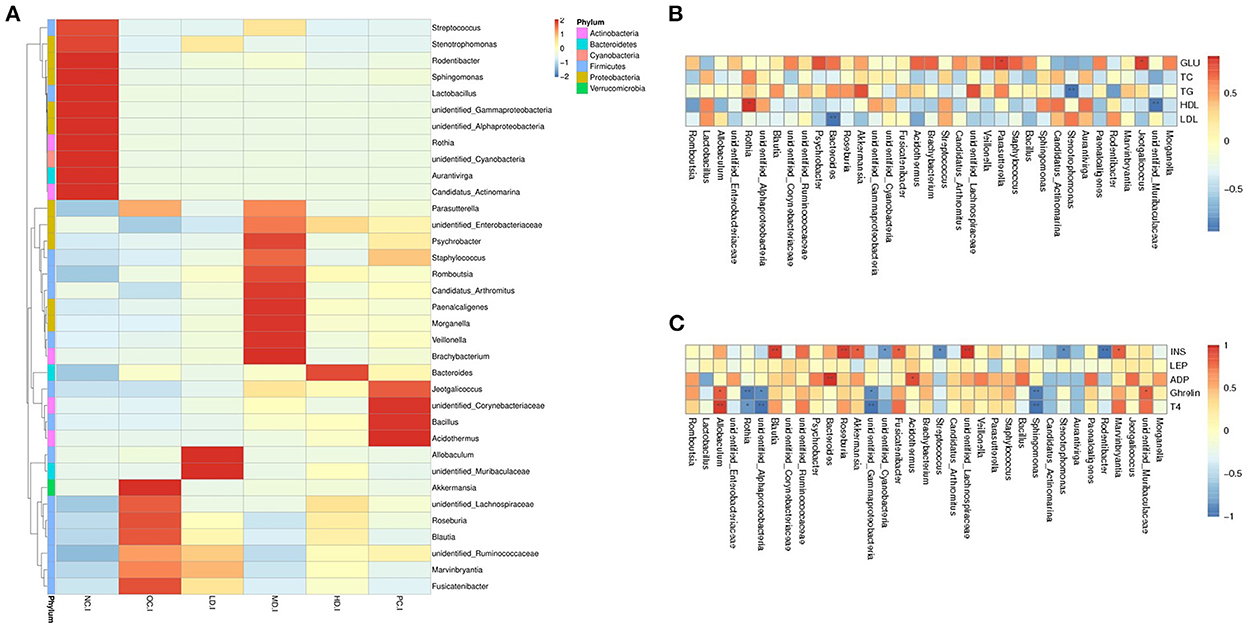
Figure 3. Spearman association analysis between gut microbiota and metabolic parameters at the genus level (A) heatmap analysis at the genus level (B) spearman association analysis between gut microbiota and glucolipid metabolism parameters and (C) serum hormone. *Correlation was significant at the 0.05 level, **correlation was significant at the 0.01 level.
The relative abundance of Fusicatenibacter decreased (p < 0.01) after UPF supplementation. The relative abundance of Bacteroides in the HD group was 5.66%, which was the highest of all groups (Figure 2D).
Association analysis between biological parameters and intestinal flora
Spearman correlation analysis was carried out to assess relationships between gut microbiota and blood biochemical indexes. Detailed data on the levels of blood sugar, total cholesterol, total triglycerides, and high- and low-density lipoproteins were reported by (Han et al., 2021); only the correlation results are shown here. It can be seen in Figure 3B that blood sugar was positively associated with the presence of Parasutterella and Jeotgalicoccus. The total triglyceride level was negatively correlated with the abundance of Stenotrophomonas. High-density lipoprotein showed a positive relationship with Rothia, while low-density lipoprotein was negatively related with Bacteroides. It could thus be inferred that HFD led to a decrease in the abundance of Rothia, causing a reduction in the high-density lipoprotein level in the OC group. The increase in Parasutterella abundance may have led to the increase in the blood sugar level in the OC group. Conversely, the effect of decreased blood sugar in the LD group may have resulted in the abatement of Parasutterella.
As indicated in Figure 3C, insulin was positively correlated with the presence of Roseburia, Blautia, Lachnospiraceae, Akkermansia, Fusicatenibacter, and Marvinbryantia and negatively associated with Rodentibacter, Stenotrophomonas, Streptococcus, and Cyanobacteria. Adiponectin had positively correlated with Bacteroides and Acidothermus. Finally, ghrelin and thyroxin were positively associated with Allobaculum and negatively correlated with Rothia, Sphingomonas, Gammaproteobacteria, and Alphaproteobacteria. These findings suggested that UPF feeding decreased the abundances of Blautia and Fusicatenibacter in the intestinal flora, leading to insulin abatement.
Analysis of differentially expressed genes in the liver
In this research, we analyzed the differentially expressed genes in liver samples after first confirming that the samples were of high quantity, quality, and biological reproducibility. As can be seen in the volcano map of differentially expressed genes (Figure 4), comparison of the OC group with the NC group revealed 5,755 differentially expressed genes in total, including 2,630 upregulated and 3,125 downregulated genes. Compared with the OC group, the LD, MD, HD, and PC groups had 1,457, 1,870, 2,538, and 2,580 upregulated genes, respectively, and 1,383, 1,740, 2,279, and 2,307 downregulated genes, respectively.
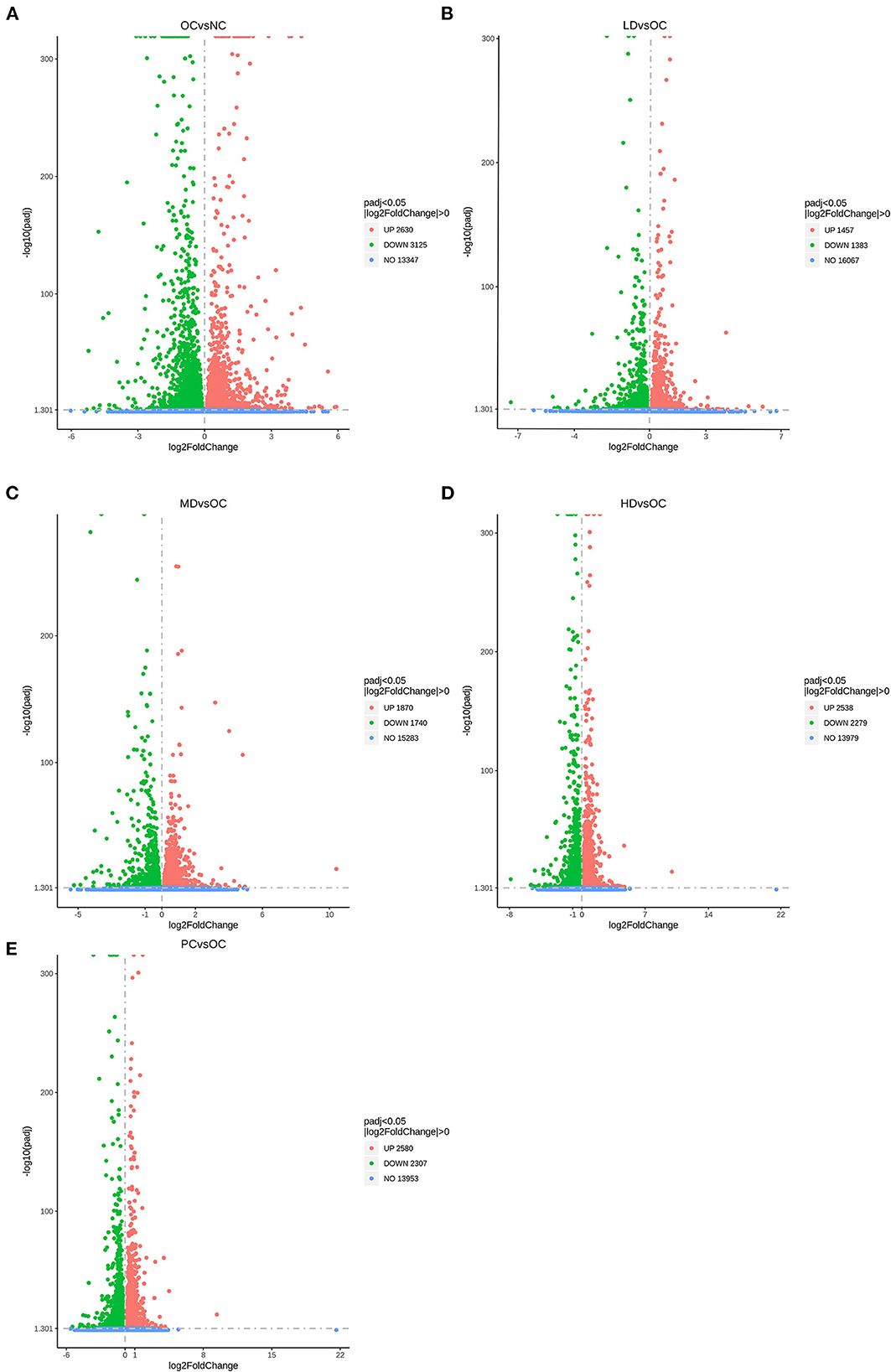
Figure 4. Differential expressed genes displayed by volcano plot (A) OC vs. NC (B) LD vs. OC (C) MD vs. OC (D) HD vs. OC (E) PC vs. OC. The dashed blue line represents the threshold line of differential gene screening criteria.
GO and KEGG functional analyses of differentially expressed genes
GO functional analysis
GO is a comprehensive database of gene function descriptions consisting of three categories: biological process (BP), cellular component (CC), and molecular function (MF) (Ashburner et al., 2000). The enrichment results from this research are illustrated in Figures 5A–E. Here, we found that several important GO categories were enriched, including the following metabolic processes: steroid metabolic process, organic acid catabolic process, carboxylic acid catabolic process, small molecule catabolic process, and fatty acid metabolic process.
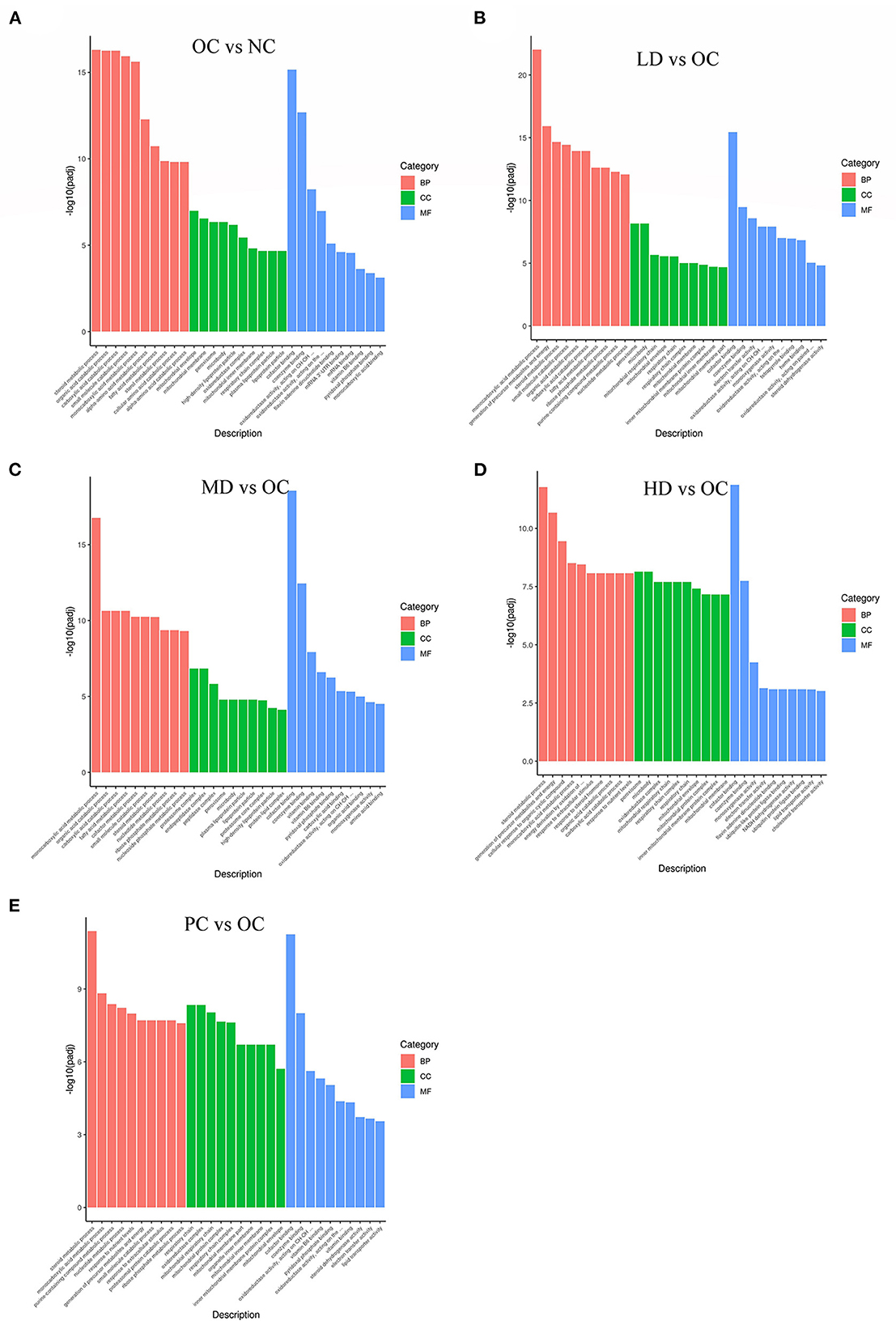
Figure 5. GO enrichment of differential genes (A) OC vs. NC (B) LD vs. OC (C) MD vs. OC (D) HD vs. OC (E) PC vs. OC. The abscissa is GO Term, and the ordinate is the significance level of GO Term enrichment, which is represented by –log10(padj), different colors represent different functional classifications.
The numbers of differentially expressed genes between the OC and NC groups that were enriched in the BP, CC, and MF categories were 609, 77, and 78, respectively. The numbers of differentially expressed genes in the other four comparison groups (in the BP, CC, and MF categories) were as follows: LD/OC (528, 51, 93); MD/OC (507, 53, 93); HD/OC (421, 79, 95); and PC/OC (430, 87, 82). The terms were mostly enriched in the BP category, followed by the CC and MF categories. Metabolic processes were prevalent in the BP category. Obesity is considered to be a chronic disease related to glucose and lipid metabolism. Therefore, the results of the GO functional analysis could form the basis for mechanistic studies of the anti-obesity properties of UPF.
KEGG functional classification
KEGG is a database resource that can be used to understand the high-level functions and utility of biological systems, such as cells, organisms, and ecosystems, from molecular-level information, especially large-scale molecular datasets generated by genome sequencing and other high-throughput experimental technologies (KEGG, 2021). As shown in Figures 6A–E, 2,454 unigenes were classified as being related to the metabolic pathways of obesity, including fatty acid metabolism, amino acid metabolism, and carbon metabolism. The pathways with the highest representation were the peroxisome proliferator activated receptor-1α (PPAR) signaling pathway (56 unigenes, 2.28%); fatty acid degradation (36 unigenes, 1.47%); pyruvate metabolism (30 unigenes, 1.22%); valine, leucine, and isoleucine degradation (41 unigenes, 1.67%); retinol metabolism (42 unigenes, 1.71%); protein processing in endoplasmic reticulum (94 unigenes, 3.83%); non-alcoholic fatty liver disease (NAFLD) (86 unigenes, 3.50%); steroid hormone biosynthesis (41 unigenes, 1.67%); complement and coagulation cascades (51 unigenes, 2.08%); and carbon metabolism (69 unigenes, 2.81%). These classifications provided some explanation of the weight-loss impacts of UPF.
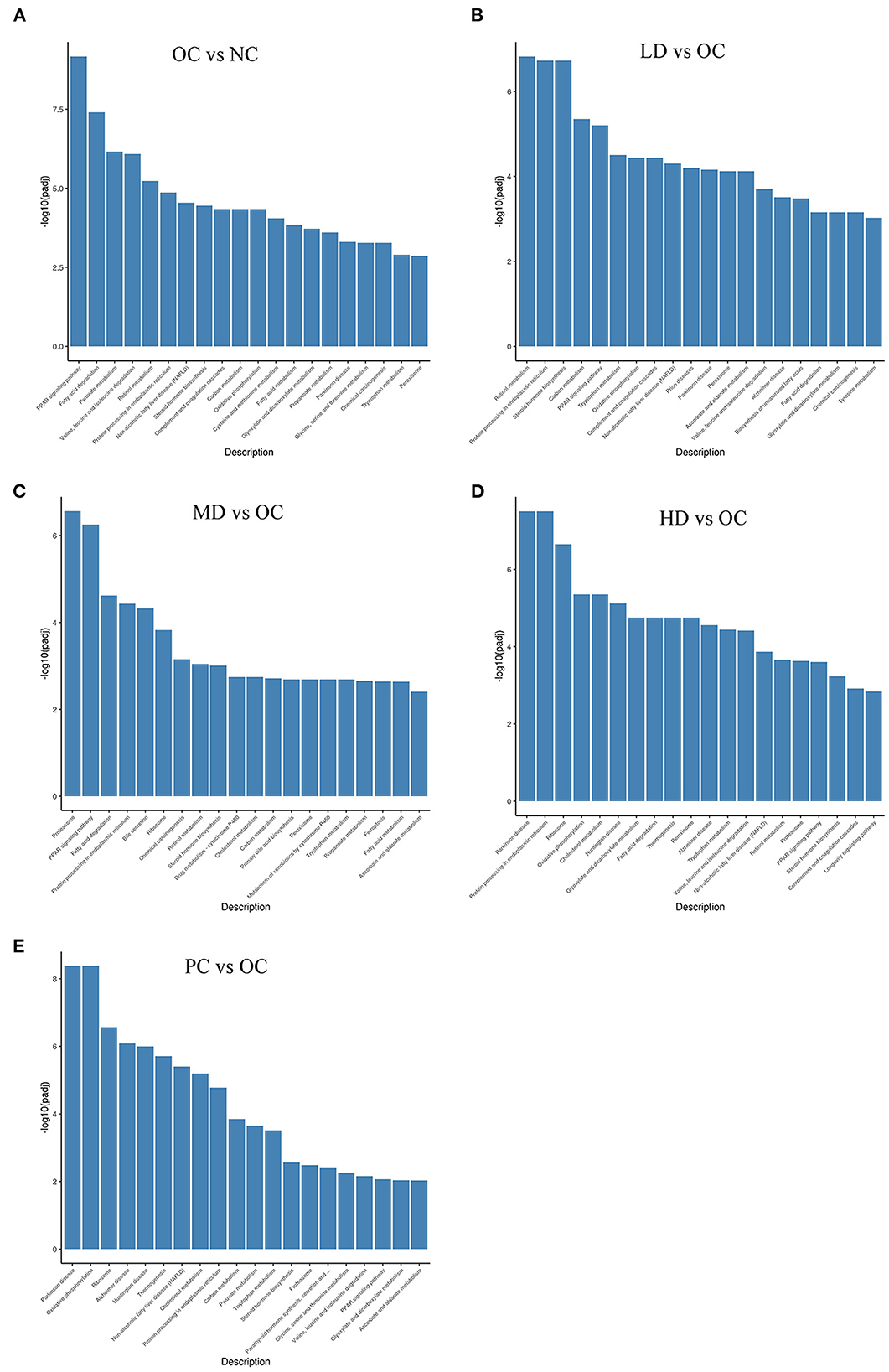
Figure 6. KEGG enrichment of differential genes (A) OC vs. NC (B) LD vs. OC (C) MD vs. OC (D) HD vs. OC (E) PC vs. OC. The abscissa is KEGG pathway, and the ordinate is the significant level of pathway enrichment.
The KEGG classification of enzymes and differentially expressed genes that were significantly enriched in the analysis gives some indication of the metabolic pathways that were affected by UPF. In terms of Pparaa gene expression in lipid metabolism (Table 5), the OC group showed a decreasing trend compared with the NC group. However, the expression of the Pparaa gene expression was significantly increased in the four intervention groups (LD, MD, HD, and PC) compared with the OC group. Moreover, Cpt1a gene expression was significantly decreased in the OC group compared with the NC group. Cpt1a gene expression was significantly increased in the LD, MD, HD, and PC groups compared with the OC group. These findings suggested that a UPF-based diet might increase Pparaa genes expression and, subsequently, the expression of Cpt1a genes, accelerating the oxidative decomposition of fatty acids and reducing the triglyceride content in the liver.
The results of the statistical analysis of the differentially expressed genes were shown in Table 5. The expression levels of the genes encoding three Amp-activated protein kinase (AMPK) subunits was significantly decreased in OC group compared with the NC group. However, the level of Prkaa2 subunit was significantly increased in the four intervention groups (LD, MD, HD, and PC) compared with the OC group. In addition, the expression of Prkab1 increased in the MD group, while that of Prkaa1 increased in the HD group. The expression levels of the Ppargc1a gene, which is related to peroxisome proliferator-activated receptor-1α (PGC1α), were lower in the OC group than the NC group, while the level was highest in the LD group followed by the HD group. It was inferred that obesity likely reduced the expression of AMPK-related genes (Prkaa1, Prkaa2, and Prkab1) and decreased Ppargc1a expression, which weakened the absorption of glucose in the body. The anti-obesity properties of UPF may have resulted from the following: (1) the increased activity of AMPK and increased expression of PGC1α—which may have led to an improvement in the body's ability to utilize glucose and decreased blood sugar levels in obese rats, helping to reducing the insulin resistance—or (2) its impact on the process of glucose metabolism, which further affects energy metabolism.
Discussion
Obesity is a metabolic disease that manifests as an increase in weight due to the accumulation of body fat (Nguyen and El-Serag, 2010). UPF contains high levels of resistant starch. In addition, our former studies have shown that resistant starch isolated from bananas can help to alleviate obesity (Fu et al., 2021). Therefore, UPF can be used a health food material with anti-obesity properties. The effects of UPF on the intestinal flora and liver transcriptome were examined here to explore the mechanisms underlying the anti-obesity properties of UPF.
Here, a UPF-supplemented diet was shown to improve dyslipidemia and repair the liver injury induced by an HFD (Han et al., 2021) (Supplementary Table 1). This could possibly be attributed to the effect of the resistant starch and dietary fiber found in UPF on lipid metabolism, studies have shown that dietary RS can reduce serum total cholesterol and triacylglycerol concentrations and fat accretion, and the increasing fiber intake can lower blood pressure and serum cholesterol levels (de Deckere et al., 1993; Nugent, 2005; Anderson et al., 2009). Obesity increases the free fatty acid levels in blood plasma and inhibits glucose transport, leading to insulin resistance (Felber and Gola, 2002). Insulin resistance is a condition in which cells become resistant to the effects of insulin, with disruption in glucose absorption, metabolism, and storage (Kahn and Flier, 2000). Hence, excess insulin is secreted, which causes hyperinsulinemia. In this research, the insulin level of the OC group was significantly higher in the OC group than the NC group. Insulin levels decreased after UPF intervention, indicating that insulin sensitivity was increased while insulin resistance was reduced.
The gut microbiota produces a large number of enzymes that hydrolyze various complex polysaccharides. In particular, resistant starch is fermented by gut microbiota in the large intestine, which produces short-chain fatty acids (SCFAs) and provides nutrients to the gut microbiota (Cherbuy et al., 2019). Obesity causes disorder in the intestinal flora and further impacts body metabolism. Here, the UPF intervention led to structural changes in the gut microbial community and increased the cluster diversity as indicated by the increase in the Chao1 and ACE index values (p < 0.05).
At the phylum level, the relative abundance of Proteobacteria increased with UPF feeding. At the genus level, the relative abundances of Blautia and Parasutterella were high in the OC group. The relative abundance of Allobaculum increased in the LD group (p < 0.05), whereas those of Romboutsia (p < 0.01) and Staphylococcus (p < 0.05) increased in the MD group. Interestingly, Fusicatenibacter was not detected in the NC group but was enriched in the OC group. The abundance of Fusicatenibacter decreased (p < 0.01) after the UPF diet intervention. Bacteroides was the most abundant probiotic in the HD group (5.66%).
Studies have shown that Blautia is positively related to weight, including waist circumference, body mass index, and blood fat levels, which includes measures of low-density lipoprotein (LDL), total triglyceride (TG), and total cholesterol (TC) (Zeng et al., 2019). The increase in the relative abundance of Parasutterella could be a potential indicator of malnutrition and metabolic abnormality associated with obesity (Lee et al., 2020). Allobaculum species are involved in SCFA production. Plantain feeding led to the enrichment of SCFA-producing microorganisms (Zhang, X. et al., 2015). Romboutsia is negatively related to body weight, fasting blood glucose, and insulin (Liu et al., 2018). It uses glucose to produce acetic acid and iso-butyric acid, which is beneficial for fat loss. In one study, Staphylococcus was shown to have a high relative abundance in thin adults in a comparison of its abundance in the intestinal flora of fat and thin adults, indicating that the enrichment of Staphylococcus may have anti-obesity properties (Vasana et al., 2018).
Fusicatenibacter showed a positive correlation with lipid metabolic indexes (TC and TG) (Huang et al., 2020). The UPF intervention significantly reduced the abundance of Fusicatenibacter, indicating that UPF had a beneficial influence on lipid metabolism. Similar to previous investigations (Amabebe et al., 2020), the gut microbiota enriched by an HFD were predominated by Firmicutes (Clostridium), Prevotella, and Methanobrevibacter, while probiotics such Bacteroides, Bifidobacterium, Lactobacillus, and Akkermansia were absent.
The relative abundance of Bacteroides in the HD group was increased, indicating that UPF had the capacity to increase probiotic proliferation. During the microbial fermentation of RS in the colon, lactic acid, acetate, propionate, and butyrate are generated (Bojarczuk et al., 2022). Data suggest that propionic acid and acetic acid can lower the pH in the large intestine. A low pH in the intestinal environment promotes the proliferation of the beneficial bacteria that make up the intestinal microbiota as well as protect it from the growth of pathogenic microorganisms (Shen et al., 2017). Moreover, RS can be used as a substrate for the growth of probiotic microorganisms because it almost completely passes through the small intestine (Sajilata et al., 2006). In summary, UPF can reduce the abundances of Blautia, Parasutterella, and Fusicatenibacter while increasing the abundance of beneficial bacteria such as Allobaculum, Romboutsia, Staphylococcus, and Bacteroides.
Spearman correlation analysis revealed the relationships between the intestinal flora and metabolic indexes. The mechanism underlying the anti-obesity properties of UPF is likely as follows: the depression of blood sugar levels may result from the decreased relative abundance of Parasutterella, a decrease in the abundances of Blautia and Fusicatenibacter may cause insulin abatement, and an increase in the abundance of Bacteroides may lead to adiponectin augmentation. Through the regulation of gut microorganisms, UPF alleviates the disorder of sugar and fat metabolism associated with obesity. Moreover, it improves hormone secretion in the host.
Pparaa and Cpt1a expression was higher in the UPF intervention groups than that in the OC group. PPARα plays a key role in fatty acid metabolism (Ricote and Glass, 2007). Mitochondrial β oxidation, microparticle ω oxidation, and peroxidase β oxidation are the three pathways of fatty acid metabolism. PPARα participates in the metabolic regulation all these pathways (Pawlak et al., 2015). Mitochondria are the main sites of fatty acid oxidation in animal cells. When fatty acids enter mitochondria, a transport medium is needed; this role is played by carnitine palmitoyl transferase (CPT1), of which CPT1A is an hypotype (McGarry and Brown, 1997). Fatty acids are restricted from entering the mitochondria if CPT1A activity is low. If this occurs, the fatty acids are converted to free fatty acids (FFAs). However, excessive FFA levels lead to insulin resistance and the accumulation of triglycerides in the liver (Levin et al., 2007). The possible regulatory mechanisms of UPF include the increased expression of the Pparaa and Cpt1a genes, which could accelerate fatty acid oxidation and decrease the triglyceride content.
AMPK, described as a “cellular energy receptor”, is a key enzyme (Woods et al., 2003). Activated AMPK can upregulate the expression of downstream PGC1α (Woods et al., 2003). In the glucose metabolic process, PGC1α can promote the expression of glucose transporter 4 (GLUT4) and subsequently increase the ability of the body to utilize glucose (Jäger et al., 2007; Chopra et al., 2012). In this study (Table 5), the expression of the AMPK gene (Prkaa1, Prkab1, and Prkaa2) was downregulated in the OC group compared with the NC group. UPF supplementation contributed to an increase in the expression of AMPK (Prkaa1, Prkab1, and Prkaa2) and PGC1α (Ppargc1a), thereby reducing glucose absorption. These effects assisted in the abatement of blood sugar and the loss of body fat and weight.
We analyzed the mechanisms underlying the anti-obesity properties of UPF. The mechanism of regulation lipid metabolism by UPF (rich in RS) was likely related to the RS fermentation in the large intestine and the production of SCFAs. SCFAs turned out to promote the release of the L-cells to secret the putative satiety hormones such as intestinal peptide YY (PYY), glucagon-like peptide-1 (GLP-1) hormones, suggesting that RS might augment satiety (Zhang, L. et al., 2015). On the other hand, RS in the diet may assist in the management of body weight via its potential effects on delaying the delivery of glucose as fuel with subsequent fat utilization, which may increase fat oxidation to promote fat mobilization (Birt et al., 2013). However, it should be noted that the UPF was a mixture; thus, elucidating the specific substance responsible for the observed effects is a matter worthy of further exploration.
Conclusion
In this study, the UPF intervention caused changes in the hormone levels by decreasing insulin levels, and increased the diversity of gut microbes and modulate the gut microbiota composition (such as the improvement in the levels of Allobaculum, Romboutsia, Staphylococcus, and Bacteroides, as well as the decrement in the levels of Fusicatenibacter). Importantly, UPF supplementation promoted glucolipid metabolism through PPAR signaling pathway (upregulation of Pparaa and Cpt1a expression) and AMPK signaling pathway (upregulation of Prkaa1, Prkab1, Prkaa2, and Ppargc1a) mechanism, improved glycemic control, and decreased insulin resistance, suggesting that the anti-obesity properties of UPF might at least partially be mediated by these genes and pathways. Hence, UPF could be a potential food ingredient for the prevention of obesity-induced by a high-fat diet and the consumption of UPF could potentially aid in improving metabolic health.
Data availability statement
The datasets presented in this study can be found in online repositories. The names of the repository/repositories and accession number(s) can be found below: https://www.ncbi.nlm.nih.gov/-SRR21422979-SRR21423008 and https://www.ncbi.nlm.nih.gov/geo/-GSM6574159-GSM6574176.
Ethics statement
The animal study was reviewed and approved by the Experimental Animal Ethics Review Committee of South China Agricultural University (SCXK 2019-0048). Ethical care and use of the laboratory animals were ensured according to the Guidelines for Animal Experimentation in Animal Research Laboratories.
Author contributions
Funding acquisition: JW and OS. Methodology: JF, JX, and ST. Project administration: GY, JW, and OS. Writing—original draft: JF and JX. Writing—review and editing: JF, QS, and OS. All authors contributed to the article and approved the submitted version.
Funding
This project was financially supported by National Key R&D Program of China (2019YFD1000203), Science Foundation of Guangdong Province (2018A030313026), GDAAS (R2020PY-JX002), the earmarked fund for CARS (CARS-31-01), Laboratory of Lingnan Modern Agriculture Project (NT2021004), Maoming Branch Grant (No. 2021TDQD003), and the Fundamental Research Funds for the Central Universities (2022ZYGXZR102).
Conflict of interest
The authors declare that the research was conducted in the absence of any commercial or financial relationships that could be construed as a potential conflict of interest.
Publisher's note
All claims expressed in this article are solely those of the authors and do not necessarily represent those of their affiliated organizations, or those of the publisher, the editors and the reviewers. Any product that may be evaluated in this article, or claim that may be made by its manufacturer, is not guaranteed or endorsed by the publisher.
Supplementary material
The Supplementary Material for this article can be found online at: https://www.frontiersin.org/articles/10.3389/fsufs.2022.1027762/full#supplementary-material
References
Administration (2012). National Food and Drug Administration[online]. Available online at: http://www.cfda.com.cn/newsdetail.aspx?id=53366 (accessed May 2022).
Almanza-benitez, S., Osorio-díaz, P., Méndez-Montealvo, G., Islas-Hernández, J. J., and Bello-Perez, L. A. (2015). Addition of acid-treated unripe plantain flour modified the starch digestibility, indigestible carbohydrate content and antioxidant capacity of semolina spaghetti. LWT Food Sci. Technol. 62, 1127–1133. doi: 10.1016/j.lwt.2015.02.031
Amabebe, E., Robert, F. O., Agbalalah, T., and Orubu, E. S. F. (2020). Microbial dysbiosis-induced obesity: role of gut microbiota in homoeostasis of energy metabolism. Br. J. Nutr. 123, 1127–1137. doi: 10.1017/S0007114520000380
Anderson, J. W., Baird, P., Davis, R. H., Ferreri, S. Jr, Knudtson, M., Koraym, A., et al. (2009). Health benefits of dietary fiber. Nutr. Rev. 67, 188–205. doi: 10.1111/j.1753-4887.2009.00189.x
Anyasi, T. A., Jideani, A. I. O., and Mchau, G. R. A. (2013). Functional properties and postharvest utilization of commercial and noncommercial banana cultivars. Compr. Rev. Food Sci. Food Saf. 12, 509–522. doi: 10.1111/1541-4337.12025
Ashburner, M., Ball, C. A., Blake, J. A., Botstein, D., Butler, H., Cherry, J. M., et al. (2000). Gene Ontology: tool for the unification of biology. Nat. Genet. 25, 25–29. doi: 10.1038/75556
Birt, D. F., Boylston, T., Hendrich, S., Jane, J. L., Hollis, J., Li, L., et al. (2013). Resistant starch: promise for improving human health. Adv. Nutr. 4, 587–601. doi: 10.3945/an.113.004325
Bluher, M. (2019). Obesity: global epidemiology and pathogenesis. Nat. Rev. Endocrinol. 15, 288–298. doi: 10.1038/s41574-019-0176-8
Bojarczuk, A., Skapska, S., Mousavi Khaneghah, A., and Marszałek, K. (2022). Health benefits of resistant starch: a review of the literature. J. Funct. Foods 93, 105094. doi: 10.1016/j.jff.2022.105094
Cerdo, T., Garcia-Santos, J. A., M, G. B., and Campoy, C. (2019). The role of probiotics and prebiotics in the prevention and treatment of obesity. Nutrients 11, 635. doi: 10.3390/nu11030635
Cherbuy, C., Bellet, D., Robert, V., Mayeur, C., Schwiertz, A., and Langella, P. (2019). Modulation of the caecal gut microbiota of mice by dietary supplement containing resistant starch: impact is donor-dependent. Front. Microbiol. 10, 1234. doi: 10.3389/fmicb.2019.01234
Chopra, I., Li, H. F., Wang, H., and Webster, K. A. (2012). Phosphorylation of the insulin receptor by AMP-activated protein kinase (AMPK) promotes ligand-independent activation of the insulin signalling pathway in rodent muscle. Diabetologia 55, 783–794. doi: 10.1007/s00125-011-2407-y
de Deckere, E. A., Kloots, W. J., and Van Amelsvoort, J. M. (1993). Resistant starch decreases serum total cholesterol and triacylglycerol concentrations in rats. J. Nutr. 123, 2142–2151.
Eleazu, C. O., and Okafor, P. (2015). Use of unripe plantain (Musa paradisiaca) in the management of diabetes and hepatic dysfunction in streptozotocin induced diabetes in rats. Interv. Med. Appl. Sci. 7, 9–16. doi: 10.1556/IMAS.7.2015.1.2
Falcomer, A. L., Riquette, R. F., de Lima, B. R., Ginani, V. C., and Zandonadi, R. P. (2019). Health benefits of green banana consumption: a systematic review. Nutrients 11, 1222. doi: 10.3390/nu11061222
Felber, J. P., and Gola, A. (2002). Pathways from obesity to diabetes. Int. J. Obes. 26, S39–S45. doi: 10.1038/sj.ijo.0802126
Flores-Silva, P. C., Rodriguez-Ambriz, S. L., and Bello-Perez, L. A. (2015). Gluten-free snacks using plantain-chickpea and maize blend: chemical composition, starch digestibility, and predicted glycemic index. J. Food Sci. 80, C961–C966. doi: 10.1111/1750-3841.12865
Fu, J., Wang, Y., Tan, S., and Wang, J. (2021). Effects of banana resistant starch on the biochemical indexes and intestinal flora of obese rats induced by a high-fat diet and their correlation analysis. Front. Bioeng. Biotechnol. 9, 575724. doi: 10.3389/fbioe.2021.575724
Fuentes-Zaragoza, E., Riquelme-Navarrete, M. J., Sánchez-Zapata, E., and Pérez-Álvarez, J. A. (2010). Resistant starch as functional ingredient: a review. Food Res. Int. 43, 931–942. doi: 10.1016/j.foodres.2010.02.004
García-Solís, S. E., Bello-Pérez, L. A., Agama-Acevedo, E., and Flores-Silva, P. C. (2018). Plantain flour: A potential nutraceutical ingredient to increase fiber and reduce starch digestibility of gluten-free cookies. Starch–Stärke 70, 170070. doi: 10.1002/star.201700107
Garcia-Valle, D. E., Bello-Perez, L. A., Flores-Silva, P. C., Agama-Acevedo, E., and Tovar, J. (2019). Extruded unripe plantain flour as an indigestible carbohydrate-rich ingredient. Front. Nutr. 6, 2. doi: 10.3389/fnut.2019.00002
Golden, A. (2017). Current pharmacotherapies for obesity: a practical perspective. J. Am. Assoc. Nurse. Pract. 29, S43–S52. doi: 10.1002/2327-6924.12519
Guo, J., Tan, L., and Kong, L. (2021). Impact of dietary intake of resistant starch on obesity and associated metabolic profiles in human: a systematic review of the literature. Crit. Rev. Food Sci. Nutr. 61, 889–905. doi: 10.1080/10408398.2020.1747391
Han, Z., Xiao, J., Ji, J., Guo, Y., Wang, J., and Sheng, O. (2021). Antiobesity effects of plantain flour on obese rats induced by high-fat diet. Food Sci. Technol. 42, 318–324.
Hoffmann Sardá, F. A., Giuntini, E. B., Gomez, M. L. P. A., Lui, M. C. Y., Negrini, J. A. E., Tadini, C. C., et al. (2016). Impact of resistant starch from unripe banana flour on hunger, satiety, and glucose homeostasis in healthy volunteers. J. Funct. Foods 24, 63–74. doi: 10.1016/j.jff.2016.04.001
Huang, K., Yu, W., Li, S., Guan, X., Liu, J., Song, H., et al. (2020). Effect of embryo-remaining oat rice on the lipid profile and intestinal microbiota in high-fat diet fed rats. Food Res. Int. 129, 108816. doi: 10.1016/j.foodres.2019.108816
Jäger, S., Handschin, C., St.-Pierre, J., and Spiegelman Bruce, M. (2007). AMP-activated protein kinase (AMPK) action in skeletal muscle via direct phosphorylation of PGC-1α. Proc. Nat. Acad. Sci. 104, 12017–12022. doi: 10.1073/pnas.0705070104
Juarez-Garcia, E., Agama-Acevedo, E., Sáyago-Ayerdi, S. G., Rodríguez-Ambriz, S. L., and Bello-Pérez, L. A. (2006). Composition, digestibility and application in breadmaking of banana flour. Plant Foods Hum. Nutr. 61, 131–137. doi: 10.1007/s11130-006-0020-x
Kahn, B. B., and Flier, J. S. (2000). Obesity and insulin resistance. J. Clin. Invest. 106, 473–481. doi: 10.1172/JCI10842
KEGG (2021). Available online at: https://www.kegg.jp/ (accessed may 2022).
Lee, S., Knotts, T. A., Goodson, M. L., Barboza, M., Wudeck, E., England, G., et al. (2020). Metabolic responses to butyrate supplementation in lf- and hf-fed mice are cohort-dependent and associated with changes in composition and function of the gut microbiota. Nutrients 12, 3524. doi: 10.3390/nu12113524
Levin, M. C., Monetti, M., Watt, M. J., Sajan, M. P., Stevens, R. D., Bain, J. R., et al. (2007). Increased lipid accumulation and insulin resistance in transgenic mice expressing DGAT2 in glycolytic (type II) muscle. Am. J. Physiol. Endocrinol. Metab. 293, E1772–E1781. doi: 10.1152/ajpendo.00158.2007
Liévin-Le Moal, V., and Servin Alain, L. (2014). Anti-infective activities of Lactobacillus strains in the human intestinal microbiota: from probiotics to gastrointestinal anti-infectious biotherapeutic agents. Clin. Microbiol. Rev. 27, 167–199. doi: 10.1128/CMR.00080-13
Liu, J., Yue, S., Yang, Z., Feng, W., Meng, X., Wang, A., et al. (2018). Oral hydroxysafflor yellow A reduces obesity in mice by modulating the gut microbiota and serum metabolism. Pharmacol. Res. 134, 40–50. doi: 10.1016/j.phrs.2018.05.012
Manna, P., and Jain, S. K. (2015). Obesity, oxidative stress, adipose tissue dysfunction, and the associated health risks: causes and therapeutic strategies. Metab. Syndr. Relat. Disord. 13, 423–444. doi: 10.1089/met.2015.0095
McGarry, J. D., and Brown, N. F. (1997). The mitochondrial carnitine palmitoyltransferase system—from concept to molecular analysis. Eur. J. Biochem. 244, 1–14. doi: 10.1111/j.1432-1033.1997.00001.x
Medina-Remon, A., Kirwan, R., Lamuela-Raventos, R. M., and Estruch, R. (2018). Dietary patterns and the risk of obesity, type 2 diabetes mellitus, cardiovascular diseases, asthma, and neurodegenerative diseases. Crit. Rev. Food Sci. Nutr. 58, 262–296. doi: 10.1080/10408398.2016.1158690
Nguyen, D. M., and El-Serag, H. B. (2010). The epidemiology of obesity. Gastroenterol. Clin. North Am. 39, 1. doi: 10.1016/j.gtc.2009.12.014
Nguyen, N. T., and Varela, J. E. (2017). Bariatric surgery for obesity and metabolic disorders: state of the art. Nat. Rev. Gastroenterol. Hepatol. 14, 160–169. doi: 10.1038/nrgastro.2016.170
Nugent, A. P. (2005). Health properties of resistant starch. Nutr. Bull. 30, 27–54. doi: 10.1111/j.1467-3010.2005.00481.x
Patino-Rodriguez, O., Agama-Acevedo, E., Pacheco-Vargas, G., Alvarez-Ramirez, J., and Bello-Perez, L. A. (2019). Physicochemical, microstructural and digestibility analysis of gluten-free spaghetti of whole unripe plantain flour. Food Chem. 298, 125085. doi: 10.1016/j.foodchem.2019.125085
Patiño-Rodríguez, O., Bello-Pérez, L. A., Flores-Silva, P. C., Sánchez-Rivera, M. M., and Romero-Bastida, C. A. (2018). Physicochemical properties and metabolomic profile of gluten-free spaghetti prepared with unripe plantain flours. LWT 90, 297–302. doi: 10.1016/j.lwt.2017.12.025
Pawlak, M., Lefebvre, P., and Staels, B. (2015). Molecular mechanism of PPARα action and its impact on lipid metabolism, inflammation and fibrosis in non-alcoholic fatty liver disease. J. Hepatol. 62, 720–733. doi: 10.1016/j.jhep.2014.10.039
Qian, L., Gao, R., Hong, L., Pan, C., Li, H., Huang, J., et al. (2018). Association analysis of dietary habits with gut microbiota of a native Chinese community. Exp. Ther. Med. 16, 856–866. doi: 10.3892/etm.2018.6249
Ricote, M., and Glass, C. K. (2007). PPARs and molecular mechanisms of transrepression. Biochim. Biophy. Acta (BBA) Mole. Cell Biol. Lipids 1771, 926–935. doi: 10.1016/j.bbalip.2007.02.013
Ruiz, L. D., Zuelch, M. L., Dimitratos, S. M., and Scherr, R. E. (2019). adolescent obesity: diet quality, psychosocial health, and cardiometabolic risk factors. Nutrients 12. doi: 10.3390/nu12010043
Sajilata, M. G., Singhal, R. S., and Kulkarni, P. R. (2006). Resistant starch—a review. Compr. Rev. Food Sci. Food Saf. 5, 1–17. doi: 10.1111/j.1541-4337.2006.tb00076.x
Sanchez-Rivera, M. M., Bello-Perez, L. A., Tovar, J., Martinez, M. M., and Agama-Acevedo, E. (2019). Esterified plantain flour for the production of cookies rich in indigestible carbohydrates. Food Chem. 292, 1–5. doi: 10.1016/j.foodchem.2019.04.007
Shen, D., Bai, H., Li, Z., Yu, Y., Zhang, H., and Chen, L. (2017). Positive effects of resistant starch supplementation on bowel function in healthy adults: a systematic review and meta-analysis of randomized controlled trials. Int. J. Food Sci. Nutr. 68, 149–157. doi: 10.1080/09637486.2016.1226275
Singh, B., Singh, J. P., Kaur, A., and Singh, N. (2016). Bioactive compounds in banana and their associated health benefits—a review. Food Chem. 206, 1–11. doi: 10.1016/j.foodchem.2016.03.033
Tan, S. (2019). Study on Weight-loss Function Evaluation and Mechanism of Seven Materials Including Banana Powder. Postgraduate, South China University of Technology.
Tan, S., and Wang, J. (2018). Weight losing function of five products including banana powder. Food Sci. Technol. 43, 61–66. doi: 10.13684/j.cnki.spkj.2018.12.012
Thompson, M. S., Hui Yan, T., Saari, N., and Sarbini, S. R. (2022). A review: resistant starch, a promising prebiotic for obesity and weight management. Food Biosci. 50, 101965. doi: 10.1016/j.fbio.2022.101965
Vasana, J., Niwed, K., Kongkiat, K., Eleni, G., and Siam, P. (2018). Comparison of gut microbiota between lean and obese adult thai individuals. Microbiol. Biotechnol. Lett. 46, 277–287. doi: 10.4014/mbl.1711.11003
Woods, A., Vertommen, D., Neumann, D., Türk, R., Bayliss, J., Schlattner, U., et al. (2003). Identification of phosphorylation sites in AMP-activated protein Kinase (AMPK) for upstream AMPK Kinases and study of their roles by site-directed mutagenesis. J. Biol. Chem. 278, 28434–28442. doi: 10.1074/jbc.M303946200
Zeng, Q., Li, D., He, Y., Li, Y., Yang, Z., Zhao, X., et al. (2019). Discrepant gut microbiota markers for the classification of obesity-related metabolic abnormalities. Sci. Rep. 9, 13424. doi: 10.1038/s41598-019-49462-w
Zhang, L., Li, H. T., Shen, L., Fang, Q. C., Qian, L. L., and Jia, W. P. (2015). Effect of dietary resistant starch on prevention and treatment of obesity-related diseases and its possible mechanisms. Biomed. Environ. Sci. 28, 291–297.
Keywords: anti-obesity, plantain flour, intestinal flora, lipid metabolism, hormones, metabolic pathway, obesity
Citation: Fu J, Xiao J, Tu S, Sheng Q, Yi G, Wang J and Sheng O (2022) Plantain flour: A potential anti-obesity ingredient for intestinal flora regulation and improved hormone secretion. Front. Sustain. Food Syst. 6:1027762. doi: 10.3389/fsufs.2022.1027762
Received: 25 August 2022; Accepted: 10 November 2022;
Published: 02 December 2022.
Edited by:
Melissa Pflugh Prescott, University of Illinois at Urbana-Champaign, United StatesReviewed by:
Chayon Goswami, Bangladesh Agricultural University, BangladeshTonna Ashim Anyasi, Agricultural Research Council of South Africa (ARC-SA), South Africa
Copyright © 2022 Fu, Xiao, Tu, Sheng, Yi, Wang and Sheng. This is an open-access article distributed under the terms of the Creative Commons Attribution License (CC BY). The use, distribution or reproduction in other forums is permitted, provided the original author(s) and the copyright owner(s) are credited and that the original publication in this journal is cited, in accordance with accepted academic practice. No use, distribution or reproduction is permitted which does not comply with these terms.
*Correspondence: Juan Wang, d2FuZ2p1YW4mI3gwMDA0MDtzY3V0LmVkdS5jbg==; Ou Sheng, c2hlbmdvdTYmI3gwMDA0MDsxMjYuY29t
†These authors have contributed equally to this work