- 1Ornithology Department, National Museums of Kenya, Nairobi, Kenya
- 2Tambua Fish Farming Ventures, Mbale-Vihiga, Kenya
Waterbirds cause substantial fish-stock losses in open aquaculture systems, particularly in developing countries where fish-ponds are smaller and predator control methods largely manual or under-resourced. This study: (1) used three fish-pond treatment meassures to assess their efficiencies in deterring predation pressure by four piscivorous waterbird families in small tilapia and catfish farms in western Kenya; and (2) distinguished bird group(s) most effectively deterred by these measures. The treatment measures were: coarse-grid wire mesh barriers; finer-grid wire barriers; and enhanced pond fertilization. Twelve fish-ponds were randomly sampled to assess birds' pond-neigborhood assemblages and their predation deterrence responses to pond treatment effects. Bird species richness was not affected by pond cover status, enhanced pond fertilization or type of pond cover barrier. However, pond-cover status, singularly and interactively with enhanced fertilization, reduced bird encounter rates while cover barrier type did not. Conversely, cover status, cover barrier type and fertilization each separately but not interactively contributed to improved deterrence to bird predation rates overall. However, while predation by families of larger birds was effectively reduced by enhancing pond fertilization or cover barriers, predation by families of smaller birds was prevented only by fine-grid chicken-mesh barriers. These results demonstrate that using enhanced fertilization and physical barriers can significantly contribute to reduction in predation pressure on open-culture pond-fish by most piscivorous birds, but may not always be effective if used separately. Effectiveness of combination of measures chosen will depend on types of target bird species and their feeding habits. The results constitute additional knowledge on field techniques useful in diversifying solution options for minimizing impacts of vertebrate predation on pond-fish stocks toward promoting sustainable aquaculture production and improving rural human nutrition.
Introduction
Aquaculture continues to play an increasingly pivotal role in global food security and nutritional diversification (Garlock et al., 2022) and is now the fastest growing sector of food production. This popularity owes largely to human population growth in urban areas and a trending shift of protein intake away from red meat toward aquatic resources richer in macronutrients and lower in cholesterol (Godfray et al., 2009; Ariño et al., 2013). Inland finfish farming is a particularly significant agricultural sector in tropical regions as harvests and access to the traditional natural fish resources continue to dwindle from overfishing and impacts of civic infrastructural development (Kearney, 2010; FAO, 2016). According to FAO (2020), inland finfish aquaculture production in Africa almost tripled from an average of ~1.5 million metric tons between 1990 and 2009 to ~4.5 million metric tons between 2010 and 2018, which is among the fastest over that period.
In East Africa, aquaculture production is mainly undertaken by small-scale farmers, predominantly for subsistence but often also to supplement other mainstream sources of income (Babatunde et al., 2021). The farmers mainly use earthen-bottom ponds, although cage- and tank-fish systems are also gaining increasing prominence among some farmers (Obwanga et al., 2020). In 2018, Kenya's inland finfish production was equivalent to 0.1% of the global total, but in spite of this seemingly low volume, Kenya's share in this segment is among the top in sub-Saharan Africa (FAO, 2020). Such ventures provide considerable sustenance for rural fish farmers to compliment crop or livestock agriculture (Guillen et al., 2019). Additional benefits derived by inland fish farmers include creation of employment opportunities for aquaculture workers, and support for local economies through provision of a ready market for fish production inputs, as well as for local supplies of construction and maintenance infrastructure and equipment from industries (Kawarazuka and Bene, 2010).
Because of a relatively smaller scale of operation in Africa in comparison to the case in more developed economies, these inland aquaculture systems are largely characterized by open-system plans spread across the landscape. To most waterbird communities, particularly piscivorous and large predator species, such systems, just like rice paddies and other artificial wetlands, provide extensions of natural foraging habitats lost, destroyed or reduced due to anthropogenic disturbance (Fasola, 2011). In some cases, piscivorous birds may preferentially utilize and forage at aquaculture pond sites even if their natural habitat has not been lost and this is often because fish ponds provide more optimal foraging opportunities due to higher densities and availability of fish prey (Feaga et al., 2015; Burr et al., 2020). As a result, conflicts between fish farmers and piscivorous birds are common due to considerable losses resulting from predation pressure by such birds (Glahn et al., 1999; Werner et al., 2007; Nwadukwe and Arimoro, 2012; Ovegård et al., 2021). Otieno (2019) estimated that farmers may lose ~15% of their production of inland freshwater aquaculture finfish to piscivorous birds each season in the western Kenya region alone. In many parts of the world, additional potential impacts include fish die-offs as a result of disease transmission and parasitic infestation mediated by some piscivorous bird species (Walakira et al., 2014; Radwan, 2021). In developing countries, small-scale fish farmers' capacity to find solutions aimed at minimizing these losses are often undermined by challenges in accessing credit facilities to mobilize the requisite financial and infrastructural investments, and these are usually superimposed on the farmers' inherently low levels of operational scale (Quagrainie et al., 2010).
A wide range of measures have been proposed and applied for mitigating aquaculture fish losses through predation by piscivorous birds but erecting physical barriers to exclude bird access is widely recognized as the most effective option (USDA, 1994; EIFAC, 1998; Farrell and Leonard, 2001). Other measures that are less effective but widely applied include frightening birds, projecting sounds, using dogs, erecting human dummies or trapping and releasing the pest birds. However, each of these has cost implications for either installation, maintenance, replacement, or personnel time (Curtis et al., 1996). Although most of the measures have been widely applied with reasonable success in the more developed countries due to the large pond sizes that facilitate easier mechanized operations there (Martin and Hagar, 1990; USDA, 1994; EIFAC, 1998), there exists a regional divide, both economic and scale-wise for their potential applicability in the developing south where ponds are much smaller, isolated and rely on manual agronomic operations (Curtis et al., 1996). Additionally, lethal control options such as killing birds by shooting, trapping or mass poisoning, are untenable in most developing countries due to existing wildlife protection legislations (KLR, 2009). Thus, the bird-scaring option remains the most widely applied measure among most the fish farmers in Africa, for instance.
One other strategy which is less widespread but has been applied in parts of Uganda (Pers. com.) is boosting fertility of pond water using enhanced quantities of organic or synthetic chemicals. This is meant for enhancing primary production in the pond water column for supplementing fish nutrition while also helping to increase water turbidity thus making it more difficult for fish to be targeted by predators that rely on visual acuity to locate their aquatic prey. Enhancement of pond's fertility as a bird-predator deterrence measure is comparable to the aquashade technique mainly applied in temperate regions, involving application of a chemical agent to the pond water so as to reduce visibility of fish to birds (Jenkins and Smith, 1998). However, majority of small-holder fish farmers in Africa remain uninformed about the potential value of this technique for deterring piscivorous bird predation impact.
Although anecdotal and verbatim reports for Uganda exist, no quantitative study has previously been conducted in the East African region to assess the role of enhanced fish-pond fertilization on its own (Smith, 1968) or in combination with other measures such as physical barriers, as a means of mitigating predation pressure by piscivorous waterbirds.
The goal of this study was to assess relative effectiveness of three options for reducing predation pressure on pond-fish by four piscivorous bird families. The bird families were: hamerkop Scopus umbretta (Scopidae), herons (Ardeidae), Long-tailed cormorants Microcarbo africanus (Phalacrocorocidae), and kingfishers (Alcedinidae). The three conrtrol options included use of: a coarse-grid steel-mesh barrier, hereafter “steel-mesh”; a finer (chicken-grade) chain-link mesh barrier, hereafter “chicken-mesh”; and enhanced pond fertilization. This was to evaluate both viability and relative effectiveness of each of the options either singly or in combination. A previous study by Otieno (2019) identified these four bird families as the most abundant and frequent visitors to fish ponds in the study area, responsible for ~84% of all predation-related pond-fish losses in Kakamega County. Therefore, the main objectives of the study were to: (1) Survey assemblage patterns and visitation frequencies of the four bird families across the study ponds; (2) Record bird's fish capture rates across the ponds so as to assess relative effectiveness of the three measures, singly or in combination, for deterring overall fish depredation pressure posed by each of the four bird families; (3) Rank the four bird families in terms of their deterrence responses to the pond treatment measures. The study was conducted across twelve ponds belonging to small-scale freshwater fish farmers stocking Nile Tilapia (Oreochromis niloticus) and African Catfish (Clarias gariepinus) in western Kenya. Our hypotheses were that: (a) fish ponds with enhanced fertilization would record reduced bird assemblages and lower bird predation rates regardless of cover status and piscivorous bird family identity, and (b) fish ponds with cover barriers would exclude all piscivorous birds from accessing fish irrespective of fertilization status. The study is the first in this region to provide insight into the potential for combining enhanced fish-pond fertilization with physical barriers for controlling impacts of vertebrate predators on open aquaculture production facilities. Specifically, they demonstrate the significance of enhanced fertilization as a more practical and more affordable low-income equivalent to the aquashade technique that is often applied for the same purpose in larger farms in the more developed economies (Jenkins and Smith, 1998).
Materials and methods
Study area
The study was carried out between September 2020 to May 2021 across 12 small-scale freshwater ponds stocked with Nile Tilapia and African Catfish. The ponds were spread across two neighboring Counties of Kisumu (00°01′37″-00°25′46″S; 34°20′03″-34°24′09″E) and Vihiga (00°01′26″-00°12′24″ N; 34°32′24″-34°55′45″ E) in western Kenya (IEBC Kenya, 2012). The altitude of the area ranges from 1,400 to 1,800 m above sea level. Annual rainfall is 1,082 mm and mean temperature ranges from 17 to 32°C. In the study area, the dominant economic activities are subsistence crop and livestock farming but small-scale finfish farming has also gained prominence for the past 15 years after the Kenya Government and the World Bank jointly implemented an economic stimulus program to promote rural aquaculture across the country to boost farmer's income across the country (GoK, 2009). The study ponds had earthen-floors and each of them averaged 200–250 m2 in surface area, and water was maintained at nearly the same depth (1.0–1.3 m). Each pond was stocked at the rate of 1,000–1,200 fish, each bearing 90% tilapia and 10–15% catfish, a strategy cited by farmers to be useful in reducing predation risk posed by most vertebrate pests (Glahn et al., 2017), and in particular, loss of juvenile fish to predatory amphibians (Otieno et al., 2021). Fish in each pond were fed at least twice daily with commercially formulated feed pellets or fragments of Dagaa fish (Rastrineobola argentea) marketed specifically for this purpose (Were et al., 2006).
Experimental design
The bird-deterrent measures which constituted the pond treatment effects were: (a) pond cover status (covered vs. open); (b) pond-cover barrier type (chicken-mesh cover of 2.5 × 2.5 cm grid vs. a coarser-grid steel-mesh cover of 5.0 × 5.0 cm); and (c) enhanced pond fertilization (fertilized vs. unfertilized). Enhanced pond fertilization involved applying farm yard manure and commercial-grade Diammonium Phosphate containing both nitrogen (N) and phosphate (P205) nutrients in selected ponds (Boyd and Tucker, 1998). The combination of these treatments formed a basis for quasi-randomized block design of the experiment. In the enhanced pond fertilization treatment, synthetic or inorganic fertilizers were applied at the rate of 12 kg/ha and supplemented with 50 kg of farm yard manure, spreading the application over the fish grow-out period (Boyd, 2018). Thus, the pond treatment effects for each of the 12 ponds were: two replicate ponds covered with steel-mesh barrierr with enhanced fertilization; two replicate ponds covered with steel-mesh barriers without fertilization: two replicate ponds covered with chicken-mesh barriers with enhanced fertilization; two replicate ponds covered with chicken-mesh barriers without fertilization; two replicate ponds left open with enhanced fertilization; and two replicate ponds left open but without fertilization. The open ponds with no cover barriers and no fertilization served as experimental controls. To prevent fish capture by long-necked birds through open gaps, pond barrier covers were erected and held in place by sturdy stakes at heights averaging 30 cm above the water surface.
Sampling protocol and intensity
Fieldwork was conducted during one sampling day per week, consistently changing the day of each subsequent week and maintaining this systematic strategy during the 6-month sampling period between September 2020 to May 2021. This period transcended two consecutive seasons of the usual 6-month tilapia-catfish grow-out period. Sampling was conducted by two observers working simultaneously, each one sampling six replicate ponds. This yielded a total 24 sampling days (1 day * 4 weeks * 6 months) and a total 216 general sampling hours (24 days * 9 sampling h). Observations were made during three 3-h diurnal period blocks (06–09 h; 11–14 h; 16–19 h) on each sampling day, these periods being the peak feeding-activity times for most aquatic bird species and thus depicting optimal feeding behaviour (Bibby et al., 2000) and at the same time serving to minimize diurnal temporal bias on the observed activities. Records were taken on bird activity, with the aid of pairs of binoculers and telescopes from a distance, dividing observation session blocks into 20-min durations. Parameters measured and recorded at each pond were: observation-time period; bird's time-activity; bird species and family identity; and fish capture rates by individuals of each bird family (total number of fish capture attempts and number of fish capture successes). Thus, predation deterrence rates were evaluated as either total of all successful fish captures, or total of all failed attempts during an observation session (Cook, 1978; Jaccard and Jaccard, 2001). To ensure sampling independence and to minimize observer bias, pond-of-start as well as pond observer-assignments were alternated each sampling week (Fitzpatrick et al., 2009). Further, a distance of at least 50 m was maintained between study ponds to reduce potential bias related to localized inter-pond dispersal of birds (Sutherland, 2006), and observers themselves maintained a distance of at least 25 m from study ponds during surveys to avoid disturbing birds.
Data analyses
Analyses were performed to evaluate influences of the various fish pond treatment options on bird's daily encounter rates, species richness and fish capture success rates. Bird encounter rates were evaluated as mean number of times a species was sighted at a study pond per day of observation (daily occurrences) as well as per hour; species richness was determined as the cumulative number of unique species observed for each study pond over the entire study period (Ugland et al., 2003). Predation rates by bird families on fish in response to pond treatment measures were pooled for all the days of the whole study period and averaged per day as a predation deterrence failure (deterrence score = 0, equivalent to fish capture success) or as predation deterrence success (deterrence score = 1, equivalent to fish capture failure). Bird encounter rate data were first inspected for data dispersion using the AER package in R v. 4.0.2 (Faddy and Smith, 2011; R Development Core Team, 2020). Subsequent to confirmation of over-dispersion (z = −41.635, p = 1; α = −0.866) and to avoid committing a type-I error in conclusions drawn, the quasi-Poisson error distribution was chosen for fitting the generalized linear models (GLM). These were fitted with a log link function to test daily encounter rates' responses to the various pond treatment effects (cover status, cover barrier type, enhanced fertilization) within the lme4 package in R v. 4.0.2 (Ver Hoeff and Boveng, 2007). To model effects of the various pond treatment measures on bird's predation deterrence rates, binomial logistic regressions with logit link functions were applied in GLMs given that such data was of binary structure—deterrence success = 0 vs. deterrence failure = 1 (MacKenzie et al., 2017). Responses of bird assemblages and deterrence scores were tested first for each pond treatment measure separately, and then also for their interactive effects. This way, a quasi-randomized block design was achieved in the sampling protocol, with the non-covered, non-fertilized ponds serving as the control treatment effects (Ariel and Farrington, 2010).
Results
Bird encounter rates were significantly higher at fish ponds that were left open as compared to those that were covered (Table 1). However, neither type of cover barrier nor enhanced fertilization treatment had an important influence on encounter rates either in overall or at family or species levels (Figures 1A,B). Furthermore, Woodland Kingfisher Halcyon senegalensis was significantly the most frequently encountered at all ponds but Pied Kingfisher Ceryle rudis completely avoided ponds subjected to enhanced fertilization (Figure 1B). The combined effect of enhanced fertilization and cover barrier type also showed no notable influence on bird encounter rates although enhanced fertilization did have an interactive effect with cover status such that enhancing fertility of covered ponds appeared to limit bird visits, as opposed to enhancing the fertility of ponds with no cover barriers (Table 1).
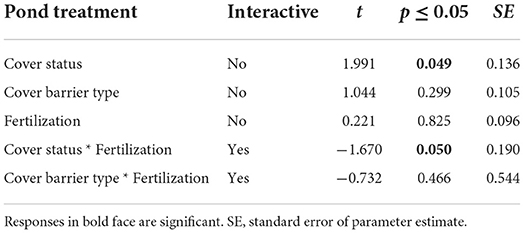
Table 1. Results of generalized quasi-Poisson linear models for separate and interactive influences of various pond treatment effects on bird encounter rates.
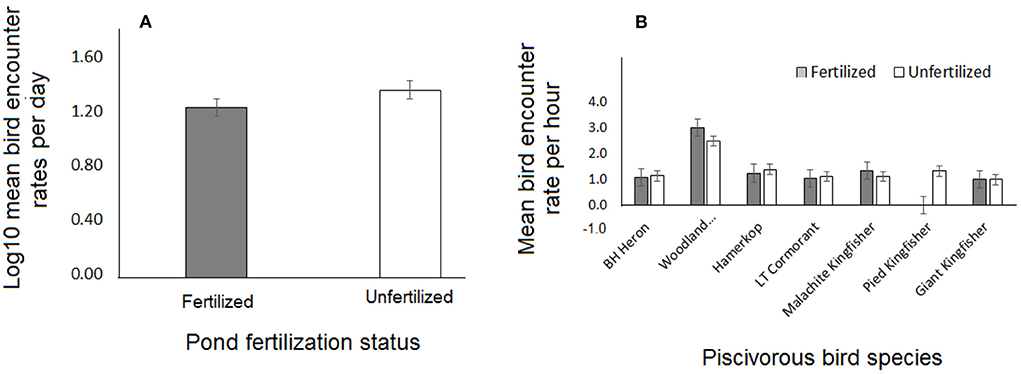
Figure 1. Effect of pond fertilization on encounter rates (A) Per diurnal day for piscivorous birds in general, and (B) Per diurnal hour for each species. BH Heron = Black-headed Heron; Woodland = Woodland Kingfisher; LT Cormorant = Long-tailed Cormorant.
Piscivorous bird species richness was generally not influenced by either ponds' cover status (t = 0.104, p = 0.198) or enhancing pond fertilization (t = −1.923, p = 0.068). However, ponds covered with chicken-mesh barriers attracted significantly the lowest variety of piscivorous species whereas steel-mesh covered ponds attracted the highest number of species (t = −3.292, p = 0.005) (Figure 2).
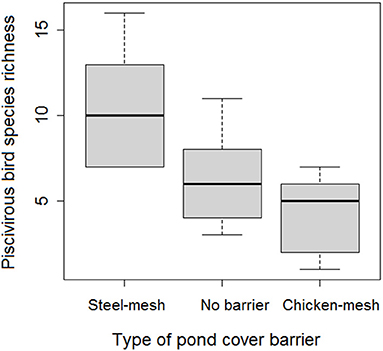
Figure 2. Comparison of piscivorous bird mean species richness across the three pond cover barrier types. Species richness was the average daily total number of species encountered across ponds covered by each cover barrier type over the entire study period.
When pond treatments were considered separately, the status of pond cover, type of pond cover barrier and enhanced fertilization, all had significant influences on the success of bird predation deterrence (Table 2).
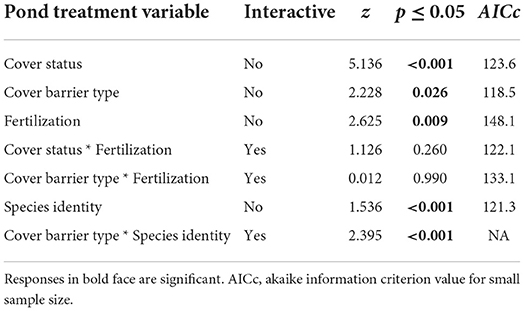
Table 2. Results of logistic regression models for separate and interactive influences of various pond treatment effects on the success of deterring birds against predation on pond fish.
Thus, as would be expected, when ponds were either open, unfertilized or covered only with the coarser-grid steel-mesh barriers, birds overall were less likely to be deterred from predating on pond-fish (Table 2; Figures 3A–D).
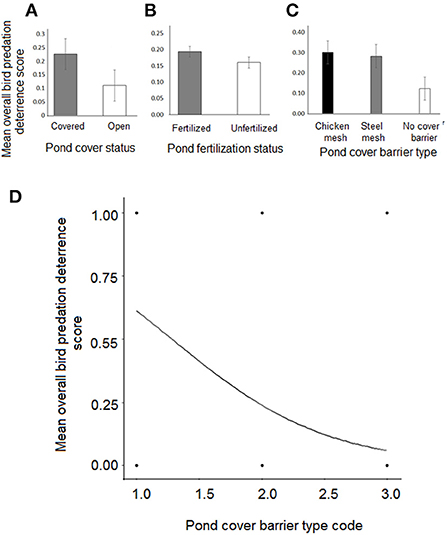
Figure 3. Effect of (A) Pond cover status, (B) Enhanced pond fertilization, and (C) Pond cover barrier type on bird predation deterrence success rate. (D) Logistic regression curve showing influence of pond cover barrier type on success rate of bird predation deterrence. Pond cover barrier type is coded on a rank score ranging from 1 = chicken-mesh; 2 = steel-mesh, to 3 = no cover barrier. Mean bird deterrence scores are daily averages for the total number of days of observation.
Considering fertilization alone and its influence on predation deterrence scores at bird family level, Hamerkop appeared most likely, but kingfishers least likely to be deterred by this control measure (Figure 4A), even though this effect was not statistically significant.
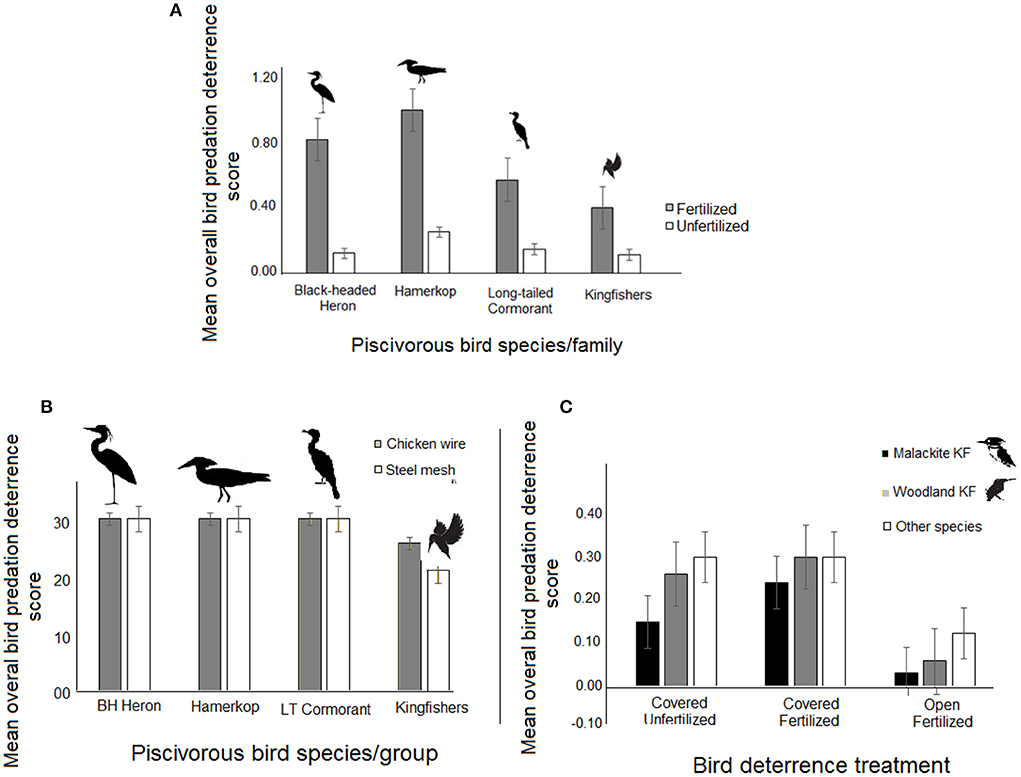
Figure 4. (A) Relative success rate of using pond fertilization alone as a measure in deterring in predation by individual families. (B) Influence of pond cover barrier type and (C) Influence of pond cover status and enhanced fertilization on predation deterrence success against kingfishers in comparison to the other bird species. BH Heron = Black-headed heron; LT Cormorant = Long-tailed Cormorant; KF = Kingfisher. Bird deterrence scores are daily averages for the total number of days of observation.
Interactive effects of enhanced pond fertilization with either pond cover status or with pond cover barrier type did not, however, show any effect on predation deterrence performance except for kingfishers (Table 2; Figure 4B). Further, the interactive effect of pond cover barrier type and species identity indicated kingfishers to be least likely than the other three bird families to be deterred from predation when steel-mesh barriers were used for covering fish ponds. In fact Woodland Kingfisher was only marginally deterred by steel-mesh coverings while Malachite Kingfisher (Chorythornis cristatus) was discouraged only by chicken-mesh cover barriers (Table 2; Figure 4C).
Discussion
Although bird encounter rates were higher at ponds without enhanced fertilization, type of cover barrier alone, regardless of fertilization, was not important in predicting bird encounter rates overall. Similarly, there was no notable influence of enhanced fertilization or cover barrier type on bird species richness at ponds. This is a manifestation that most piscivorous birds may use the aquaculture sites for purposes other than for foraging on fish. Such uses may include resting or coutrship displays for breeding purposes. In addition, except for kingfishers, the other three bird families especially hamerkop and herons, are not exclusively piscivorous and thus might visit the pond viscnity to forage on alternative prey resources such as arthropods, crustaceans, oligochaetes or amphibians (Wilson et al., 1987; Kopu, 1996; Burr et al., 2020). A high density of trees and suitable structures for roosting or alternative foraging opportunities can also enhance frequent visits by piscivorous birds near large aquaculture facilities since such a landscape character simulates the birds' own natural habitat (Sebasti'an-Gonz'alez and Green, 2013; Barrett et al., 2018; Otieno, 2019). Many species of waterbirds may thus be attracted to such sites in spite of the variety of artificial treatment effects that may be applied on individual ponds. Nevertheless, bird species richness was highest at pond covered with steel-mesh barriers in contrast to the fewest when ponds were covered with chicken-mesh barriers indicating that although fine-grid barriers may be less inviting to many species that may visit fish ponds, the coarser-grid steel mesh barriers being mouch more transparent, can still entice birds to approach ponds and attempt to catpure fish from below even if such attempts may not be successful.
In terms of bird predation deterrence, enhanced pond fertilization and use of pond cover barriers showed more profoundly impactful outcomes. Thus, when ponds were covered with barriers, especially the fine-grid chicken-mesh, or if they were treated with enhanced fertilization, bird's predation were significantly reduced. However, contrary to expectation, these measures were not necessarily successful in deterring predation by all piscivorous bird families when applied in combination. This apparent non-significance of this combined effect may be associated with feeding habits of kingfishers which, when analyzed separately, were not completely deterred by covering ponds except with the fine-grid chicken-mesh. Malachite Kingfisher, due to its relatively small size, was particularly undeterred by steel-mesh and continued to frequently visit and prey heavily on juvenile or immature fish (Laudelout and Libois, 2003; Vilches et al., 2013). These observations suggest that for most piscivorous birds, particularly the small-sized species, enhanced pond fertilization may produce positive results in deterring predation only as long as ponds are not left open and the only potentially successful alternative to fertilization is to deploy fine-grid barriers of at most the size of chicken-mesh. Many previous studies attest to this, showing that using physical barriers to cover ponds is the only measure that can guarantee against aquaculture fish loss to most vertebrate predators including piscivorous birds (Gorenzel et al., 1994; Farrell and Leonard, 2001; Nemtzov and Whittaker, 2003). Even partial barriers against predators may have significant positive impacts on fish production. For instance, Nwadukwe and Arimoro (2012) observed that when ponds were surrounded by open-top exclusion fencing using barrier netting, catfish nursery fingerling survival rates were c. 20% higher than for unfenced treatments due to predation by birds. Similar positive outcomes were reported by (Melotti et al., 1996) for exclusion netting against bird predation on Sea Bass (Dicentrarchus labrax). An alternative measure to physical exclusion barriers is to reduce fish visibility in the ponds by enhancing water fertility to stimulate phytoplankton growth and Boyd (2018) indicated that this measure may be applied on its own or in concert with varieties of physical barriers for successful results. However, enhancing pond fertilization need to be applied in such a way as to avoid compromising pond water's physico-chemical properties essential for maximal aquaculture production (Duan et al., 2011; Abdel-Tawwab et al., 2015).
Long-term effectiveness of the fine-grid barriers to exclude all access to fish by small bird species like kingfishers, however, also require designing these structures to be sturdier than the standard chicken-mesh, which is commercially provided only in gauges that are too flexible and may soon sag down or collapse under the weight of perching birds, as was occasionally witnessed during surveys, and is also prone to medium term degradation due to rusting. Therefore, a more effective design would be one of a higher tensile gauge. In addition, whether or not fertilization is simultaneously applied, mesh barriers should best be erected in inclined or dormed shapes to prevent accumulation of allotchthonous debris that might add to organic inputs into the water, or reduce light penetration and lower levels of dissolved oxygen, potentially undermining fish health and growth (Huntingford et al., 2010; Abdel-Tawwab et al., 2015).
In terms of relative suitabilities amongst the piscivorous bird families the findings of this study indicate that the larger-bodied piscivorous bird families, especially hamerkop and herons, which unlike kingfishers (Vilches et al., 2013), do not rely completely on visual acuity to catch their fish prey (Butler, 1997; Jepsen et al., 2018), are most likely to be successfully deterred from predating on pond fish when enhanced pond fertilization is applied even in the absence of preventive cover barriers. However, even for these bird families, use of physical barriers, at least of the steel-mesh grid size, remains the only non-lethal guarantee for totally preventing predation on pond-fish. Some species of heron, for instance, are able to prey on pond-fish even nocturnally (Laubhan et al., 1991; McNeil et al., 2011) while cormorants, having capacity for deep diving, are capable of capturing fish underwater without having to visually locate them (McKay et al., 2003) thus highlighting a possible limitation of enhanced fertilization alone. Both these cases illustrate that enhancing pond fertilization without deploying physical barriers may be useful primarily in situations where pond stocking densities are relatively low, water colums are deep enough to minimize activity of wading birds (Glahn et al., 2017) or where birds have alternative foraging opportunities within the viscinity, otherwise they would still be inclined to invest extraneous effort and time to capture fish (Gendron, 1986). Finally, by mediating reduced predation pressure from large wading piscivorous birds, physical barriers and enhanced fish-pond fertilization can also play a significantly instrumental role of protecting fish from diseases or parasites that can potentially be transmitted through feeding activities of some birds. For instance, Glahn et al. (2017) reported that disease and pathogen infection rates in catfish ponds in Mississippi were strongly attributable to Great Blue Heroms (Ardea herodias) and other large wading birds foraging in or around the aquaculture facilities.
Conclusion
This study has shown that totally excluding piscivorous birds through fine-grid pond cover barriers would be the most effective in eliminating predation pressure on aquaculture fish, but that on their own, enhanced fertilization or coarser-grid cover barriers would not be effective against all birds. The chicken barrier will exclude even the small-bodied piscivorous birds that are most significant predators of juvenile fish in breeding ponds. But even though chicken-mesh size barriers were sufficiently effective even on their own, steel-mesh barriers when deployed together with enhanced pond fertilization can be almost equally effective as an alternative combined predation deterrence strategy against all species. For large piscivorous bird species alone, the steel-mesh can also be totally effective even without pond fertilization (Alceste, 2019) as long as they are erected high above the pond water surface. If ponds are to be left open, enhancing fertilization is a reasonable but less effective predation deterrence measure against birds that rely on sight to capture fish prey, although this will still not limit the variety of bird species visiting aquaculture pond viscinity for purposes other than capturing fish. Despite chicken-mesh cover barriers being the most effective for preventing bird predation and the cheapest option, it is commercially available only in indurable designs that can drive up production costs owing to the need for frequent maintenance and replacement from damage from birds' perching. Similarly, steel-mesh may be sturdier in design and last longer but bears a heavier initial cost for acquisition and installation. Inorganic fertilizer prices are often also volatile and unpredictable therefore, for many farmers in this region or those with many large ponds, this option may, similarly, still present a production cost challenge. Therefore, the decision as to whichever one or combination of measures to adopt will ultimately depend on individuals farmers' own circumstances and budgets. Nonetheless, many of these farmer are already using organic or farmyard manure to boost plankton productivity in the ponds and to supplement commercial fish feeds (Fermon, 2011). These farmers need only to increase the use of such organic manures to realize the additional benefit of mitigating bird predation pressure. Such use of farmyard manure to supplement fish feeds is already practiced across many small aquaculture systems in southern parts of Asia and is considered as a significant form of sustainable fish farming, commonly referred to as integrated aquaculture (Bhatt et al., 2011). In order to further help fish farmers make optimal choices, future research should consider incorporating economic variables in designing an integrated model for a decision support tool that will allow for flexibility and versatility, taking into account individual farmers' own capital and labor endowments balanced against expected economic returns on investment. Given the growing importance of aquatic food in human diets and global food chains (Garlock et al., 2022), such a tool would be a significant aid to small-scale fish farmers and would help in boosting their efforts and contribution to local and national food security through aquaculture production. Similarly, because the present study did not quantify the role of dissolved oxygen levels in influencing bird predation rates, future investigation on this theme should include this linkage as a way of evaluating optimal fertilization levels that will avert potentially detrimental effects on fish health and overall fish-pond productivity.
Data availability statement
The raw data supporting the conclusions of this article will be made available by the authors, without undue reservation.
Ethics statement
This study did not require any ethics approval because it was purely observational from a distance and did not involve any direct handling, any direct contact with or manipulation of any regulated animals or manipulation of their wild or natural habitat, or long continuous surveillance that might interfere with their natural behavior or welfare.
Author contributions
NO: research conceptualization, data collection, data analysis, and manuscript writing and submission. ES: data collection, data collation, manuscript edit, and approval. Both authors approved the submitted version.
Funding
Funding for the project were provided to the lead author by the Waterbird Society through the Waterbird Research Grant Award Scheme, Grant Details: WBS/WRA-2020. The funding agency was not involved in the study design, collection, analysis, interpretation of data, the writing of this article, or the decision to submit it for publication.
Acknowledgments
We thank the three fish farmers in Tambua Ward of Vihiga County and one farmer in Chulaimbo Region, Kisumu West Ward of Kusmu County, Kenya, who permitted us access to conduct research on their aquaculture their ponds; the County Fisheries Ministries in Kisumu and Vihiga for study approvals and to the Local Administrative Officials for additional grassroots liaison support. The National Museums of Kenya is thanked for hosting the project and availing office space, administrative and computing infrastructure to facilitate effective management and execution of the project. Funds for the project were provided by the Waterbird Society through the Waterbird Research Grant Award Scheme.
Conflict of interest
The authors declare that the research was conducted in the absence of any commercial or financial relationships that could be construed as a potential conflict of interest.
Publisher's note
All claims expressed in this article are solely those of the authors and do not necessarily represent those of their affiliated organizations, or those of the publisher, the editors and the reviewers. Any product that may be evaluated in this article, or claim that may be made by its manufacturer, is not guaranteed or endorsed by the publisher.
References
Abdel-Tawwab, M., Hagras, A. E., Allah, H., Elbaghdady, M., and Monier, M. N. (2015). Effects of dissolved oxygen and fish size on Nile tilapia, Oreochromis niloticus (L.): growth performance, whole-body composition, and innate immunity. Aquacul. Int. 23, 1261–1274. doi: 10.1007/s10499-015-9882-y
Alceste, C. C. (2019). Advice for Managing Predatory Birds, Part 1. FL: Global Aquaculture Advocate. Available online at: https://www.globalseafood.org/advocate/advice-managing-~predatory-birds-part-1/ (accessed June 30, 2022).
Ariel, B., and Farrington, D. P. (2010). “Randomized block designs”, in Handbook of Quantitative Criminology, ed. A. Piquero, and D. Weisburd (New York, NY: Springer), 437–564.
Ariño, A., Beltrán, A., Herrera, J. A., and Roncalés, P. (2013). “Fish and seafood: nutritional value” in Encyclopedia of Human Nutrition. 3rd Edn, ed. B. Caballero (New York, NY: Academic Press), 254–261.
Babatunde, A., Robertson-Andersson, D., Moodley, G., and Taylor, S. (2021). Aquaculture in Africa: a comparative review of Egypt, Nigeria, and Uganda vis-a-vis South Africa. Rev. Fish. Sci. Aquacul. 29, 167–197. doi: 10.1080/23308249.2020.1795615
Barrett, L. T., Swearer, S. E., and Dempster, T. (2018). Impacts of marine and freshwater aquaculture on wildlife: a global meta-analysis. Rev. Aquacul. 11, 1022–1044. doi: 10.1111/raq.12277
Bhatt, B. P., Bujarbaruah, K. M., Vinod, K., and Karunakaran, M. (2011). Integrated fish farming for nutritional security in eastern Himalayas. India J. Appl. Aquacul. 23, 157–165. doi: 10.1080/10454438.2011.581585
Bibby, C. J., Burgess, N. D., and Hill, D. A. L. (2000). Bird Census Techniques. 2nd Edn. London: Academic Press.
Boyd, C. E., and Tucker, C. S. (1998). Pond Aquaculture and Water Quality Management. Boston, MA: Kluwer Academic Publishers. Available online at: https://link.springer.com/book/10.1007/978-1-4615-5407-3 (accessed March 10, 2022).
Burr, P., Avery, J. I., Street, G. M., Strickland, B. K., and Dorr, B. S. (2020). Piscivorous bird use of aquaculture and natural water bodies in Mississippi. J. Wildl. Manag. 84, 1–10. doi: 10.1002/jwmg.21948
Butler, R. W. (1997). The Great Blue Heron: A Natural History and Ecology of A Seashore Sentinel. Vancouver: University of British Columbia Press. Available online at: https://www.nhbs.com/the-great-blue-heron-book (accessed April 18, 2022).
Cook (1978). Foraging behaviour and food of Grey Herons Ardea cinerea on the Ythan Estuary. Bird Stud. 25, 17–22. doi: 10.1080/00063657809476570
Curtis, K. S., Pitt, W. C., and Canover, M. R. (1996). Overview of Techniques for Reducing Bird Predation at Aquaculture Facilities. Publication 12 of the Jack Berryman Institute. Logan: Utah State University. Available online at: https://digitalcommons.usu.edu/extension_histall/1011 (accessed June 30, 2022).
Duan, Y., Dong, X., Zhang, X., and Miao, Z. (2011). Effects of dissolved oxygen concentration and stocking density on the growth, energy budget and body composition of juvenile Japanese flounder, Paralichthys olivaceus (Temminck and Schlegel). Aquacul. Res. 42, 407–416. doi: 10.1111/j.1365-2109.2010.02635.x
EIFAC (1998). Report of the EIFAC Working Party on Prevention and Control of Bird Predation in Aquaculture and Fisheries Operations. EIFAC Technical Paper (51). Rome: Food and Agriculture Organization. Available online at: https://www.fao.org/documents/card/en/c/fbf302bf-da1a-54f1-adc0-f7523800c728/ (accessed June 5, 2022).
Faddy, M. J., and Smith, M. D. (2011). Analysis of count data with covariate dependence in both mean and variance. J. Appl. Stat. 38, 2683–2694. doi: 10.1080/02664763.2011.567250
FAO (2016). The State of World Fisheries and Aquaculture for 2016: Contributing to Food Security and Nutrition for All. Rome: FAO Fisheries Department. Available online at: http://www.fao.org/3/a-i5555e.pdf (accessed June 5, 2022).
FAO (2020). The State of World Fisheries and Aquaculture 2020: Sustainability in Action. Rome: FAO Fisheries Department. Available online at: https://www.fao.org/publications/sofia/2020/en/ (accessed June 30, 2022).
Farrell, P., and Leonard, B. (2001). Observations on the survival of the Yabby, Cherax destructor, in ponds where access by piscivorous birds is inhibited. J. Appl. Aquacul. 11, 75–80. doi: 10.1300/J028v11n03_07
Fasola, M. (2011). Consequences of rice agriculture for waterbird population size and dynamics. Waterbirds 33, 160–166. doi: 10.1675/063.033.s112
Feaga, J., Vilella, F., Kaminski, R., and Davis, B. (2015). Waterbird use of catfish ponds and migratory bird habitat initiative wetlands in Mississippi. Waterbirds 38, 269–281. doi: 10.1675/063.038.0307
Fermon, Y. (2011). Subsistence Fish Farming in Africa: A Technical Manual. Bagnolet: Action Contre la Faim, (ACF) International. Available online at: https://www.actionagainsthunger.org/publication/2013/10/subsistence-fish-farming-africa-technical-manual (accessed June 30, 2022).
Fitzpatrick, M. C., Presisser, E. L., Ellison, E. M., and Elkinton, J. J. (2009). Observer bias and the detection of low-density populations. Ecol. Applic. 19, 1673–1679. doi: 10.1890/09-0265.1
Garlock, T., Asche, F., Anderson, J., Ceballos, A., Love, D., Osmundsen, T., et al. (2022). Aquaculture: the missing contributor in the food security agenda. Glob. Food Sec. 32, 100620. doi: 10.1016/j.gfs.2022.100620
Gendron, R. P. (1986). Searching for cryptic prey: evidence for optimal search rates and the formation of search images in quail. Anim. Behav. 34, 898–912. doi: 10.1016/S0003-3472(86)80076-8
Glahn, J. F., Dorr, B., Harrel, J. B., and Khoo, L. (2017). Foraging ecology and depredation management of Great Blue Herons at Mississippi catfish f arms. J. Wildl. Manag. 66, 194–201. doi: 10.2307/3802885
Glahn, J. F., Reinhold, D. S., and Smith, P. (1999). Wading bird depredations on channel catfish Ictalurus punctatus in northwest Mississippi. J. World Aquacul. Soc. 30, 107–114. doi: 10.1111/j.1749-7345.1999.tb00323.x
Godfray, C. H., Averayd, C. P., Garnnett, T., Hall, J. W., Timothy, J. K., Lorimer, J., et al. (2009). Meat consumption, health, and the environment. Science 361, eaam5324. doi: 10.1126/science.aam5324
GoK (2009). Economic Stimulus Programme: Overcoming Today's Challenges for a Better Tomorrow. Nairobi: Government of the Republic of Kenya, Ministry of Finance and Planning.
Gorenzel, W. P., Conte, E. S., and Salmon, T. P. (1994). “Bird damage at aquaculture facilities), in The Handbook: Prevention and Control of Wildlife Damage, eds. S. E. Hygnstrom, R. M. Timm, and G. E. Larson (Lincoln: University of Nebraska), 5–18. Available online at: https://digitalcommons.unl.edu/icwdmhandbook/57 (accessed June 30, 2022).
Guillen, J., Natale, F., Garvalho, N., Casey, J., Hofher, J., Druoon, G., et al. (2019). Global seafood consumption footprint. Ambio 48, 111–122. doi: 10.1007/s13280-018-1060-9
Huntingford, F. A., Andrew, G., Mackenzie, S., Morera, D., Coyle, S. M., Pilarczyk, M., et al. (2010). Coping strategies in a strong schooling fish, the common carp (Cyprinus carpioL.). J. Fish Biol. 76, 1576–1591. doi: 10.1111/j.1095-8649.2010.02582.x
IEBC Kenya (2012). Preliminary Report on the First Review Relating to the Delimitation of Boundaries of Constituencies and Wards. Nairobi: IEBC, Government of the Republic of Kenya. Available online at: https://academia-ke.org/library/download/iebc-preliminary-report-on-the-1st-review-~of-delimitation-of-boundaries-of-constituencies-and-wards-2012-pdf/ (accessed April 14, 2022).
Jaccard, J., and Jaccard, J. (2001). Interaction Effects in Logistic Regression. Thousand Oaks: Sage New York. Available online at: https://methods.sagepub.com/book/interaction-effects-in-logistic-regression (accessed May 15, 2022).
Jenkins, W. A., and Smith, T. I. J. (1998). Aquashade fails to control avian predators of pond-cultured juvenile sunshine bass (Morone chrysops) females and M. saxatilis males. J. Appl. Aquacul. 8, 63–69. doi: 10.1300/J028v08n02_06
Jepsen, N., Flávio, H., and Koed, A. (2018). The impact of cormorant predation on Atlantic salmon and Sea trout smolt survival. Fish. Manag. Ecolog. 26, 183–186. doi: 10.1111/fme.12329
Kawarazuka, N., and Bene, C. (2010). Linking small-scale fisheries and aquaculture to household nutritional security: an overview. Food Sec. 2, 343–357. doi: 10.1007/s12571-010-0079-y
Kearney, J. (2010). Food consumption trends and drivers. Phil. Trans. Roy. Soic. B Biolog. Sci. 365, 2793–2807. doi: 10.1098/rstb.2010.0149
KLR (2009). Laws of Kenya: Chapter 376 - The Kenya Wildlife Service and Management Act. Nairobi: National Council for Law Reporting.
Kopu, G. (1996). Breeding and feeding ecology of the Reed Cormorant Phalacrocorax africanus in the Free State, South Africa. Act. Ornithol. 31, 89–99.
Laubhan, M. K., Rundle, W. D., Swartz, B. I., and Reid, F. A. (1991). Diurnal activity patterns and foraging success of Yellow-Crowned Night-Herons in seasonally flooded wetlands. Wils. Bull. 103, 212–277.
Laudelout, A., and Libois, F. R. (2003). “On the feeding ecology of the pied kingfisher, Ceryle rudis at Lake Nokoué, Benin. Is there competition with fishermen?” in Interactions Between Fish and Birds: Implications for Management, ed. I. G. Cowx (Oxford: Blackwell Science), 165–177.
MacKenzie, D. I., Nichols, J., Royle, J. A., Pollock, K. H., Bailey, L. L., and Hines, J. E. (2017). Occupancy Simulation and Modelling - Inferring Patterns and Dynamics of Species Occurrence. 2nd Edn. New York, NY: Academic Press.
Martin, L. R., and Hagar, S. (1990). Bird control on containment pond sites. Proc. Vertebrate Pest Conf. 14, 307–310.
McKay, H. V., Russell, I. C., Rehfisch, M. M., Armitage, M., Packer, J., and Parrott, D. (2003). “Pilot trials to assess the efficacy of fish refuges in reducing the impact of cormorants on inland fisheries”, in Interactions Between Fish and Birds: Implications for Management, ed I. G. Cowx (New York, NY: Blackwell Publishing Ltd.), 278–287.
McNeil, R., Benoit, R., and DesGranges, J. (2011). Daytime and night-time activity at a breeding colony of Great Blue Herons in a non-tidal environment. Can. J. Zool. 71, 1075–1078. doi: 10.1139/z93-145
Melotti, P., Roncarati, A., Dees, A., and Mordenti, O. (1996). “Impact of bird predation on the intensive rearingof seabass and sea bream,” in Proc. Int. Workshopon Sea Bass and Sea Bream Culture: Problems and Prospects, Verona. 16–18 October 1996, 301–314.
Nemtzov, S., and Whittaker, L. O. (2003). The use of netting over fish ponds as a hazard to waterbirds. Waterbirds 46, 416–423. doi: 10.1675/1524-4695(2003)026[0416:TUONOF]2.0.CO;2
Nwadukwe, F. O., and Arimoro, F. O. (2012). The use of plastlon net fence as predator control in catfish nursery ponds. World J. Fish Mar. Sci. 4, 232–236. doi: 10.5829/idosi.wjfms.2012.04.03.61265
Obwanga, B. K., Soma, A., Ingasia, O., Ayuya, E., Rurangwa, D., van Wonderen, D. G., et al. (2020). Exploring Enabling Factors for Commercializing the Aquaculture Sector in Kenya. 3R Research report 011. Wageningen: Wageningen University and Research.
Otieno, N. E. (2019). Economic impact of predatory piscivorous birds on small-scale aquaculture farms in Kenya. Aquacul. Rep. 15, 1–10. doi: 10.1016/j.aqrep.2019.100220
Otieno, N. E., Wasonga, D. V., and Imboko, D. (2021). Pond-adjacent grass height and pond proximity to water influence predation risk of pond fish by amphibians. Hydrobiologia 848, 1795–1809. doi: 10.1007/s10750-021-04551-8
Ovegård, M., Jepsen, N., Nord, M., and Petersson, E. (2021). Cormorant predation effects on fish populations: a global meta-analysis. Fish Fisher. 22, 1–18. doi: 10.1111/faf,.12540
Quagrainie, K. K., Ngugi, C., and Amisah, S. (2010). Analysis of the use of credit facilities by small-scale fish farmers in Kenya. Aquacul. Int. 18, 393–402. doi: 10.1007/s10499-009-9252-8
R Development Core Team (2020). R - A Language and Environment for Statistical Computing v. 4.0.2. Vienna: R Foundation for Statistical Computing. Available online at: https://www.r-project.org/ (accessed May 15, 2022).
Radwan, M. (2021). Vital economic threat of predatory birds and parasites to cultivated fishes in Egypt. Aquacul. 548, 737666. doi: 10.1016/j.aquaculture.2021.737666
Sebasti'an-Gonz'alez, E., and Green, A. J. (2013). Habitat use by waterbirds in relation to pond size, water depth, and isolation: lessons from a restoration in southern Spain. Restor. Ecol. 22, 311–318. doi: 10.1111/rec.12078
Smith, M. W. (1968). Fertilization and predator control to increase growth rate and yield of trout in a natural lake. J.Fish. Resour. Board Canad. 25, 2011–2036. doi: 10.1139/f68-181
Sutherland, W. J., (ed.) (2006). Ecological Census Techniques: A Handbook. Cambridge: Cambridge University Press.
Ugland, K. I., Gray, J. S., and Ellingsen, K. E. (2003). The species-accumulation curve and estimation of species richness. J. Anim. l Ecol. 72, 888–897. doi: 10.1046/j.1365-2656.2003.00748.x
USDA (1994). Bird Predation and its Control at Aquaculture Facilities in the Northeastern United States. USDA-APHIS Fact Sheets on Wildlife Damage Management Paper 33. Available online at: http://digitalcommons.unl.edu/usdaaphisfactsheets/33 (accessed June 30, 2022).
Ver Hoeff, J. M., and Boveng, P. L. (2007). Quasi-Poisson vs. negative binomial regression: how should we model over-dispersed count data? Ecology 88, 2766–2772. doi: 10.1890/07-0043.1
Vilches, A., Arizaga, J., Salvo, I., and Miranda, R. (2013). An experimental evaluation of the influence of water depth and bottom color on the Common kingfisher's foraging performance. Behaviour. Proc. 98, 25–30. doi: 10.1016/j.beproc.2013.04.012
Walakira, J., Akoll, P., Engole, M., Sserwadda, M., and Majalija, S. (2014). Common fish diseases and parasites affecting wild and farmed Tilapia and catfish in Central and Western Uganda. Ug. J. Agric. Sci. 15, 113–125. Available online at: https://www.ajol.info/index.php/ujas/article/view/126198
Were, E. O., Ngugi, C., and Veverica, K. (2006). Yields and economic benefits of tilapia and catfish polyculture in ponds using locally available feeds. J. East Afr. Natur. Resour. Manag. 1, 1–10. Available online at: https://aquafishcrsp.oregonstate.edu/biblio/yields-and-economic-benefits-tilapia-oreochromis-niloticus-and-catfish-clarias-gariepinus
Werner, S., Harrel, J., and Wooten, D. E. (2007). Foraging behavior and monetary impact of Wading birds at Arkansas Baitfish Farms. J. World Aquacul. Soc. 36, 356–364. doi: 10.1111/j.1749-7345.2005.tb00339.x
Keywords: piscivorous waterbirds, tilapia predation, fish-pond, barrier efficacy, food security, pond fertilization, rural nutrition
Citation: Otieno NE and Shidavi E (2022) Effectiveness of physical barriers and enhanced fertilization in controlling predation on tilapia and catfish aquaculture systems by four piscivorous water bird families. Front. Sustain. Food Syst. 6:1018064. doi: 10.3389/fsufs.2022.1018064
Received: 12 August 2022; Accepted: 28 September 2022;
Published: 14 October 2022.
Edited by:
Erick Ochieng Ogello, Maseno University, KenyaReviewed by:
Emmanuel Amagu Echiegu, University of Nigeria, Nsukka, NigeriaAbdullah-Al Mamun, Noakhali Science and Technology University, Bangladesh
Nicholas Outa, Maseno University, Kenya
Copyright © 2022 Otieno and Shidavi. This is an open-access article distributed under the terms of the Creative Commons Attribution License (CC BY). The use, distribution or reproduction in other forums is permitted, provided the original author(s) and the copyright owner(s) are credited and that the original publication in this journal is cited, in accordance with accepted academic practice. No use, distribution or reproduction is permitted which does not comply with these terms.
*Correspondence: Nickson Erick Otieno, otieno_nickson@yahoo.com