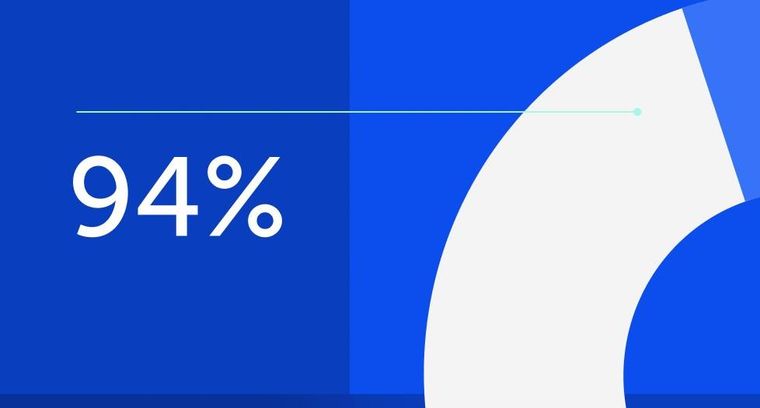
94% of researchers rate our articles as excellent or good
Learn more about the work of our research integrity team to safeguard the quality of each article we publish.
Find out more
ORIGINAL RESEARCH article
Front. Sustain. Food Syst., 04 October 2022
Sec. Agroecology and Ecosystem Services
Volume 6 - 2022 | https://doi.org/10.3389/fsufs.2022.1013470
This article is part of the Research TopicAdvances in Molecular Crop PathologyView all 9 articles
Rehmannia glutinosa is a top-grade traditional Chinese medicine, and also is an important planting medicinal material for Chinese poor farmers shaking off poverty. Rehmannia mosaic virus (ReMV) causes big economic loss of R. glutinosa in planting area. However, there is no effective methods for quick, accurate, and high-throughput detection for ReMV in Chinese production area. The preserved R. glutinosa samples carrying ReMV was taken for research material. The coat protein coding sequences (CPReMV) was cloned and sequenced. The target sequence was further placed into a prokaryotic expression vector to express the N-terminal-tagged recombinant CPReMV protein (His-CPReMV). Purified His-CPReMV was used as an antigen to immunize New Zealand white rabbits, and antiserum was obtained. The titers and sensitivities of the antisera were analyzed and evaluated. Polyclonal antibodies were purified from the antiserum, and the titers and sensitivity to the target His-CPReMV protein were evaluated. The results demonstrate that the obtained polyclonal antibodies against His-CPReMV could be successfully used for rapid, accurate, and high-throughput detection of ReMV from R. glutinosa planted in the wild. Our investigation established serological-based detection methods for ReMV for the first time, and provides a foundation for future exploration of the pathogenic mechanisms of ReMV in R. glutinosa.
Rehmannia glutinosa is a dicotyledonous plant named because its underground root tuber is yellowish-white. It is the source of one of the four Huaiyao drugs and is a common bulk medicinal material in China. It was first recorded in the Shennong herbal classic, being listed as a top-grade traditional Chinese medicine (Wang et al, 2004) and the main component of traditional Chinese medicines, such as Liuwei Rehmannia pills® and Tao Hong Siwu Decoction® (Song, 2021). It is planted in Henan, Shandong, Shanxi, and other areas, among which Henan has the largest Rehmannia planting and processing base in China. Studies have confirmed that iridoids, ionones, and other substances in R. glutinosa effectively protect heart and brain arteries, repair nerves, reduce blood glucose, and enhance human immunity (Li and Meng, 2015; Wang et al., 2015). The developmental history of R. glutinosa germplasm resources in China are also very long, and there are nearly 70 cultivated varieties (Wang et al., 2018).
Rehmannia mosaic virus (ReMV) was first reported in Henan Province, China. It was first isolated from R. glutinosa in 1962 by Professor Bo Tian and was identified as a member of the genus tobamovirus (Tian, 1962). In Hou et al. (2007) found that the ReMV particle is rod-shaped, and the virus also infects tomatoes, peppers, cucumbers, and other plants after mechanical inoculation (sap rubbing). Infected plants usually show symptoms, such as flowering leaves, rolling leaves, and wilting. The planting of R. glutinosa in the production area is relatively simple. Generally, nutritional reproduction through rhizomes is a common method for R. glutinosa (Kang, 2017), which allows easy accumulation of viruses, viroids, and bacterioplasts for a relatively longer time, resulting in a decline in the quality and yield of the plant. The diseases caused by these pathogens pose a great threat to the production safety and stability of R. glutinosa.
ReMV is a positive single-strand RNA virus with a genome of 6,395 nucleotides as described (Lei, 2006). ReMV contains four opening reading frames (ORFs), which encode a 126 kDa protein, a 183 kDa protein, a 30 kDa movement protein (MP) and a 17.5 kDa coat protein (CP). The 5′ and 3′ ends of the genome have non-coding regions of 71 and 204 nts, respectively (Lei, 2006) (Supplementary Figure S1). The ReMV Shanxi isolates (ReMV-SX) has a full-length sequence of 6,395 nts and encodes four proteins (Wang et al., 2021). ORF1 contains 1,116 amino acids (aa) (126 kDa), ORF1a contains 1,615 aas (180 kDa), ORF3 contains 267 aas (MP, 30 kDa), and ORF4 contains 159 aas (CP, 17.5 kDa). The 5′-UTR is 71 nts long, and the 3′ URT is 204 nts long (Wang et al., 2021). ReMV is widely distributed in Shanxi, Henan, Japan, and South Korea (Kubota et al., 2012; Lim et al., 2016).
Henan Province is located in the transitional zone of the north–south climate. Counties located in Henan Province were the main places for poverty alleviation. Planting R. glutinosa is the important grasp for government and farmers to get rid of poverty. Hence, to ensure steady development of the regional economy and maintain farmers' income, it is urgent to control the occurrence of ReMV in Henan Province under the current background of rural revitalization.
Quick, accurate, and high-throughput diagnostics of ReMV was the most essential tool for controlling of the virus disease in R. glutinosa cultivation. The high specific polymerase chain reaction (PCR), as well as the high-throughput sequencing technologies combined with PCR supplied accurate identification of viruses, multiple viruses' detection, virus quantification, and discovering of new emerging viruses (Rubio et al., 2020; Mehetre et al., 2021). The PCR detection methods can sometimes result in false-negative amplification as a consequences of nucleotide sequences variation in primer-binding genomic portions. Besides, the PCR inhibitors in analyzed plant samples can also cause wrong pairing of the primer with the corresponding position or PCR inhibition. Other methods, such as serological-based detections (western blot, dot-blot, and enzyme-linked immunosorbent assays) (2021-crop protection), CRISPR/Cas12a-based detection (Alon et al., 2021), and high-throughput sequencing (Villamor et al., 2019; Liefting et al., 2021), are often widely used in routine plant virus detection. For ReMV detection, the PCR based on specific primers was the main detection method so far. Serological methods utilizing the polyclonal antibody was not reported. Thus, accurate detection of ReMV based on serological methods is currently needed for R. glutinosa production areas.
In this study, R. glutinosa samples carrying ReMV were identified and analyzed. The CP gene sequence was successfully cloned from the ReMV Henan isolate by RT-PCR. A prokaryotic expression vector of CPReMV was constructed, and the N-terminal His-tagged recombinant His-CPReMV protein was purified. Using the purified protein as an antigen to immunize New Zealand white rabbits, we obtained antiserum with polyclonal antibodies. After a series evaluation, we determined that the polyclonal antibodies had high titer and sensitivity against the purified His-CPReMV and the ReMV-infected R. glutinosa. Serological detection methods, such as western blotting, dot blotting, and enzyme-linked immunosorbent assays (ELISA), for the efficient detection of ReMV were successfully established from R. glutinosa planted in the field. Our exploration provides an alternative and efficient detection of ReMV using polyclonal antibodies against its viral proteins, and lays a foundation for the identification and control of ReMV and to study its pathogenesis in the future.
We established serological methods using ReMV-infected and uninfected R. glutinosa samples as controls. Molecular assays were performed using RT-PCR assays of eight symptomatic or asymptomatic plants collected from a Chinese traditional and herbal drug park in Henan Province. R. glutinosa samples used in the validation of these assays were collected from a plantation in Jiaozuo City, Henan Province, China, in 2019. These R. glutinosa often exhibited yellowing, mosaicism, leaf curling, wilting, and other symptoms (Supplementary Figure S2).
According to the contigs that contains the coding sequences of ReMV CP gene from Jiaozuo City in Henan Province, we have designed the primers. The expected amplicons using the designed pair of primer are summarized in Table 1.
Total RNA was extracted from the tissues of ReMV-infected R. glutinosa. The Takara PrimeScript RT Reagent Kit was used to generate cDNA by reverse transcription (RT). The putative coding region of CPReMV gene (480 bp) was amplified from the template cDNA prepared previously by RT-PCR using the primer pair CPReMV-F-Eco RI and CPReMV-R-Xho I (Table 1). After double digestion with Eco R1 and Xho 1 for 5 h, the amplified product was cloned into pET-28a(+), generating the recombinant expression vector pET28a-CPReMV. The target vector was transformed into Escherichia coli DH5α cells. Colony PCR was performed to confirm the correct expression of the target plasmid using the putative CP-specific primers CPReMV-F and CPReMV-R (Table 1). Sequencing was performed using the T7 promoter sequencing primer (BBI Life Science, China), and sequence alignment was performed by comparison with the reported ReMV sequence (Accession No. NC_009041.1). The expected size of the recombinant CPReMV protein was predicted from the cloned sequence using DNAman software.
Two validated pET-28(a)-CPReMV recombinant clones were transformed into E. coli BL21 for small-scale expression testing of the putative His-CPReMV recombinant protein. Recombinant proteins expressed by each of the two clones were analyzed by sodium dodecyl sulfate-polyacrylamide gel electrophoresis (SDS-PAGE) and coomassie brilliant blue (CBB) staining. The clone with the highest expression level of recombinant His-CPReMV protein was selected for large-scale expression and purification, according to a previously described method (Zhang et al., 2022).
The concentrated protein was sent to immunize New Zealand white rabbits. The total amount of antigen was 2 mg, with 0.5 mg being used in each immunization, and the interval between injections was 10 days. Following immunization, the 1 mL rabbit blood was drawn from the ear vein, and the antiserum was placed at 4°C overnight for titer evaluation. Total crude antisera against CPReMV were obtained after centrifugation at 12,000 × g for 15 min, as previously described (Zhang et al., 2021). Sodium sulfate solution (50, 33, and 30%) was used to precipitate immunoglobulin IgG. After a series of purification procedures (Zhang et al., 2022), the polyclonal antibody against CPReMV (PAb-CPReMV) with a relatively high degree of purity was obtained.
The PAb-CPReMV was used to establish the western blotting, immuno-dot blotting, and ELISA methods for ReMV detection form R. glutinosa. All performances followed standard protocols (Barker, 1998; Zhang et al., 2021).
For western blotting, a 0.1 g sample was frozen in liquid nitrogen and ground quickly. Total protein was extracted using protein isolation buffer as previously described (Zhang et al., 2022). In the first antibody incubation step, the antiserum against His-CPReMV was diluted 103-fold (Figure 2D), and serially diluted (103, 2 × 103, 5 × 103, 104, and 2 × 104; Figure 2D), whereas the PAb-CPReMV was diluted 103-fold and 2 × 104-fold (Figures 3C, 4, respectively). The secondary antibody used was alkaline phosphatase (AP)-conjugated anti-rabbit immunoglobulin (AP-A) (Sangon Biotech, CA).
For immuno-dot blotting, leaves were ground, and total protein was extracted and treated as previously described, with minor revisions (Zhang et al., 2022). In the first antibody incubation step, the antiserum was diluted for 101, 102, 103, 104, 105, and 106-fold in Figure 2C, whereas the dilution was 105-fold (Figures 4, 5A, respectively).
For ELISA, leaves (0.1 g) of suspected susceptible and healthy plants were frozen and ground to powder in liquid nitrogen, then 500 μ L protein extraction buffer was added and the mixture placed on ice for 10 min. Centrifugation was performed at 12,000 g for 10 min. The supernatant was collected, and ~100 μL was loaded into a 96-well ELISA plate (100 μL/well), covered with silver paper, then incubated at 37°C for 4 h, as previously described with minor revisions (Zhang et al., 2022). In the first antibody incubation step, the antiserum was diluted to 2.0 × 102, 103, 4 × 103, 1.6 × 104, 6.4 × 104, 2.56 × 105, and 1.024 × 106-fold (Figure 2B). The PAb-CPReMV was diluted for 2.0 × 104-fold.
Plant leaves were subjected to liquid nitrogen quick-freezing and then ground to a powder with a 4 cm diameter hole punch (LABGIC, China). Then, the plant tissue powder was placed into a pre-cooled 1.5 mL eppendorf tube without RNA enzyme, while adding 600 μL water saturation/chloroform/isoamyl alcohol (25:24:1) and 600 mL RNA extraction buffer (20 mM Tris-HCL, pH 7.8, 5 mM EDTA, 200 mM NaCl, and 1% SDS, simultaneously. The system was mixed gently by vortex oscillation (vortex-genie 2, USA). After placing on the ice for 5 min, the tube was centrifuged at 12,000 rpm for 20 min at 4 m°C. The supernatant (500 μL) was transferred to an RNase-free tube, and the same volume of 4 M LiCl solution was added to precipitate the RNA overnight at −20°C. RNA precipitate was collected by centrifugation at 12,000 rpm for 20 min at 4°C. Finally, the RNA was washed with 70 and 100% ethanol, and then dried at low temperature and vacuum conditions. The extracted RNAs were stored and reserved at −80°C or were dissolved in diethyl oxydiformate (DEPC)-treated deionized water.
The integrity of the isolated total RNAs was verified by staining ribosomal RNA with ethidium bromide (EB) by 1.2% agarose gel electrophoresis, and DNase 1 (Takara, Dalian) digestion was used to remove contaminant genomic DNA before RT-PCR. The HiScript 1st Strand cDNA Synthesis Kit (Vazyme Company, Nanjing, China) and CPReMV reverse primers were used to synthesize template cDNA, and PCR was performed using the primers CPReMV-F and CPReMV-R to amplify the CPReMV coding sequence. The PCR products were analyzed using 1.2% agarose gel electrophoresis and EB staining.
Taking advantage of the cloned ReMV CP sequence from Jiaozuo city of Henan Province and the CP sequences deposited in NCBI, we constructed the phylogenetic tree, which indicating that the nucleotide of the CP was highly conserved (Supplementary Figure S3). Hence, to obtain the recombinant CP protein was essential and general for preparation of the antiserum against the ReMV. The CPReMV coding sequence (480 bp) was successfully cloned into pET28- CPReMV, and then the correct plasmid was amplified using E. coli DH5α. After confirming the correct reading frame and sequence, the resultant pET28- CPReMV was transformed into E. coli BL21 strain. Small-scale expression experiments were performed, and the results show that the two clones expressed a 21 kDa protein in the presence of IPTG induction (Figure 1A), which is consistent with the predicted size of the recombinant His-CPReMV protein. Because of the high amount of target protein produced in the presence of IPTG, clone 2 was selected for subsequent large-scale production of the recombinant protein. SDS-PAGE analysis of the eluents of step-wise imidazole elution showed that the resin-binding recombinant protein was more efficiently eluted by the 200 mM imidazole solution than that by other concentrations of imidazole (Figure 1B), and there were barely visible background contaminant proteins in all elutes (Figure 1B). These results demonstrate that a significant amount of the recombinant protein remained in the supernatant after bacterial lysis (Figure 1B). All supernatants were further concentrated and purified using a centrifugal filter unit (Millipore Amicon Ultra-0.5, Sigma-Aldrich, USA) and dialysis tubing (SnakeSkin Dialysis TubingTM, ThermoFisher, USA), respectively. In summary, the purity and concentration of the purified His-CPReMV were suitable for the production of polyclonal antibodies.
Figure 1. Expression and purification of the recombinant His-CPReMV protein in E. coli BL21 by affinity chromatography. (A) SDS-PAGE (12.5%) analysis the expression of His-CPReMV protein in two independent E. coli BL21 transformants (1#, 2#), which showing the expression levels of target protein with (+) or without (–) IPTG induction. (B) SDS-PAGE (12.5%) analysis of eluents from affinity chromatography purification of the prokaryotically expressed target protein. Both the supernatant, the precipitate and the column pass-through fluid obtained after centrifugation and column-affinity binding of the bacterial cell extraction. Protein profiles of the eluents obtained after elution of the Ni-NTA resin column with the indicated concentrations of imidazole (60–400 mM) are shown. M, protein markers; Black triangle, the bands of target protein.
The affinity column-purified and dialyzed His-CPReMV preparation was subjected to SDS-PAGE analysis, and the results show that the concentration (1.25 mg/mL) and purity of the target protein were of high quality (Figure 2A). The titer of the obtained antiserum was determined by enzyme linked immunosorbent assay (ELISA), and the results show that the OD450 was still above 0.6, even when the antiserum was diluted 64,000 times (Figure 2B, red Line). The purified His-CPReMV could be clearly detected, and the membrane showed strong signals at the expected positions in western blotting using the diluted antisera that were diluted at a concentration gradient ratio of 1:1,000, 1:2,000, 1:5,000, 1:10,000, and 1:20,000 (Figure 2D). These western blotting results show that the specific bands could still be seen even when the dilution ratio of the antiserum was 1:20,000 (Figure 2D), indicating that the prepared antisera had high titer and quality. Further, the antisera of CPReMV were diluted 1:10−1, 1:10−2, 1:10−3, 1:10−4, 1:10−5, and 1:10−6 for immuno-dot blotting, showing that there was still a visible color reaction even when the dilution was 10−5-fold (Figure 2C). The ELISA, western blotting, and dot blotting results demonstrate that the prepared polyclonal antiserum against CPReMV had high titer, sensitive, and therefore suitable for immunological detection.
Figure 2. Evaluation of the quality of the prepared antiserum against the His-CPReMV protein using three serological methods. (A) SDS-PAGE analysis of the dialyzed His-CPReMV protein purified by affinity column. (B) The titer of the crude antiserum was determined by enzyme linked immunosorbent assay (ELISA), using commercial horseradish peroxidase (HRP)-labeled as secondary antibody. The X-axis shows the antiserum dilutions folds that using phosphate buffer solution (PBS). Y-axis shows the absorbance values measured at 450 nm. M stands for the protein markers. (C) The crude antiserum was evaluated by Immuno-dot-blot assay by diluted for different folds by PBS. The purified His-CPReMV was taken as antigen. (D) Evaluation of the crude antiserum by western blot assays. The crude antiserum was diluted for different folds by PBS, and purified His-CPReMV was taken as antigen.
The eight R. glutinosa samples collected from Jiaozuo City in Henan Province were subjected to RT-PCR assays using CP gene-specific primers (CPReMV-F and CPReMV-R) (Table 1). The results show that an amplicon with expected size (approximately 480 bp) was observed, which indicated that samples 2#, 3#, 6#, 7#, and 8# were ReMV-infected (Figure 3A). Samples 1#, 2#, 5#, and 7# were further validated by RT-PCR using primers (ReMV-F and ReMV-R) for amplification of the full-length ReMV cDNA (Table 1), and the results confirmed that samples 2# and 7# were ReMV-infected and samples 1# and 5# were not (Figure 3B). Further, western blotting was performed to detect ReMV from R. glutinosa samples 1#, 2#, 5#, and 7# using the obtained CPReMV antisera. The results show that only samples 2# and 7# had corresponding putative 21 KDa bands on the membrane, indicating that samples 2# and 7# were ReMV-infected, whereas samples 1# and 5# were not (Figure 3C). We selected two ReMV-infected positive samples (2# and 7#) and two negative samples (1# and 5#) for further establishment of serological detection methods based on polyclonal antibodies against CPReMV (PAb-CPReMV).
Figure 3. Screening for the ReMV-infected and non-infected R. glutinosa plants. (A) Agarose-gel analysis of the RT-PCR products from eight R. glutinosa plants collected from the field. The amplicon position of the CP coding region sequence was indicated by a black arrowhead. (B) Agarose-gel analysis of the selected R. glutinosa samples 1#, 2#, 5#, and 7# by RT-PCR using the primers corresponding to full-length cDNA of ReMV genomic RNA. (C) Western blot analysis of the R. glutinosa samples 1#, 2#, 5#, and 7# using the crude antiserum against CP of ReMV (diluted 20,000-fold). M, DNA or protein markers.
To improve the accuracy of ReMV detection based on the obtained antisera, we explored the application of purified PAb-CPReMV for the detection of ReMV in R. glutinosa. We selected ReMV-infected, R. glutinosa samples 2# and 7# as tested materials, and purified recombinant His-CPReMV and His-GFP were used as positive and negative controls, respectively. We extracted total proteins from these samples and performed western blotting assays using 10−4-fold diluted PAb-CPReMV. The blotting membrane showed a strong and clear band with an expected size of 21 KDa (Figure 4, upper panel), demonstrating that the purified PAb-CPReMV had relatively high purity and titer. Immuno-dot blotting was performed to further evaluate the quality of the PAb-CPReMV. The blotting stripe showed clear red dots even when purified PAb-CPReMV was diluted 10−6-fold (Figure 4, middle panel), which also indicated that the purified PAb-CPReMV had high titer and sensitivity. RT-PCR confirmed that samples 2# and 7# were ReMV-infected, positive R. glutinosa, which was similar to the positive control (Figure 4, bottom panel). Taken together, our serological analyses demonstrate that the purified PAb-CPReMV had a high titer and degree of purity.
Figure 4. Establishments of the serological detections using the selected samples and purified polyclonal antibodies against CP of ReMV (PAb-CPReMV) from R. glutinosa plants in filed. The R. glutinosa samples 2# and 7# were detected by western blot analysis using the PAb-CPReMV (diluted 10,000-fold) (upper panel). The R. glutinosa samples 2# and 7# were detected by immuno-dot-blot assay using PAb-CPReMV (diluted 1,000,000-fold) (middle panel). Agarose-gel analysis of RT-PCR products of R. glutinosa samples 2# and 7# using the corresponding CP primers (bottom panel). M, DNA or protein markers; the P stands for purified His-CPReMV (positive control); and the N stands for ReMV uninfected R. glutinosa samples (negative control, 1#).
To extend the application of PAb-CPReMV in ReMV detection on R. glutinosa, we explored the possibility of performing high-throughput ELISA and immuno-dot blotting using PAb-CPReMV. We collected 40 R. glutinosa samples from a Chinese herbal medicine plantation in Henan Province. Total proteins were extracted, and immuno-dot blotting assays were performed. We observed intense color reactions on the membrane when the PAb-CPReMV was diluted 10−6-fold as described above (Figure 5A). The results suggest that 12 samples were ReMV-infected, and the ReMV incidence was 28.95% in this garden. Furthermore, we performed ELISA using PAb-CPReMV (Figures 5B,C). The results are consistent with those of the immuno-dot blotting assays, as well as the readings at OD405, which demonstrates that ELISA could be used for ReMV detection using PAb-CPReMV. In summary, our results show that ELISA and immuno-dot blotting could be used for high-throughput detection of ReMV in R. glutinosa form fields.
Figure 5. Establishments of the high-throughput immuno-dot-blot assay and ELISA detections of ReMV for R. glutinosa field samples. (A) Immuno-dot-blot assay results of the 38 field samples that collected form Henan Province. The A1 represents the negative control, and the A2 stands for positive control. Samples that from A3–A8, B1–B8, C1–C8, D1–D8, and E1–E8 comprised the 38 R. glutinosa samples from Henan. (B) Pictures of the ELISA blank that show the same 38 samples from Henan. (C) ELISA absorbance (OD405) readings of the 38 samples from (B) measured at a 405 nm, and the histogram was mapped.
As one of the important bulk medicinal materials, R. glutinosa is cultivated in many countries around the world. With the continuous development and utilization of the effective components of R. glutinosa, demand is also increasing. Currently, because of different farming systems and geographical environments, R. glutinosa is affected by a variety of viruses in different planting areas. Presently, the main viruses infecting R. glutinosa include ReMV, tobacco mosaic virus (TMV), tomato mosaic virus (ToMV), broad bean wilt virus-2 (BBWV-2), cucumber mosaic virus (CMV), potato virus X (PVX), and carnation ringspot virus (CIRV) (Hou et al., 2007; Gao et al., 2009; Zhou et al., 2010; Du et al., 2013).
In recent years, there have been few studies on the biological characteristics of ReMV, and fewer on serological and molecular detection methods of the virus. In this study, total RNA was extracted from several virus-infected R. glutinosa samples collected from the city of Jiaozuo located in Henan Province at the summer of 2019. We obtained the CPReMV coding sequence of ReMV isolated form city of Jiaozuo in Henan Province by RT-PCR. Taking advantage of the existing ReMV CP sequences deposited in NCBI, we constructed a phylogenetic evolution tree of different regional isolates based on the CP gene of ReMV (Supplementary Figure S3). Sequence alignment analyses show that there was a relatively high similarity between ReMV and TMV and Oilseed rape mosaic virus (ORMV), but no serological detection methods for the sensitive and accurate identification of ReMV in R. glutinosa production areas were available. Hence, preparation of an antiserum against the CP of ReMV and development of serological-based detection methods are necessary for quick diagnosis of the disease in R. glutinosa production fields.
To investigate the molecular variation and genetic differentiation of ReMV in Jiaozuo City, Henan Province, we cloned the CP gene sequence of the ReMV isolate reported in Shanxi and Zhengzhou, Henan Province, as well as in South Korea, Japan, and the United States. The results show that the CP genes of ReMV isolates from different regions are generally close to each other in genetic relationships, but there is also a certain genetic variation (Supplementary Figure S3). This may result from the comprehensive effects of the farming system, climate, environment, and other factors in different regions, which accelerated the variation of the viral genomic RNA. In addition, to ensure stable production of healthy and high-quality R. glutinosa, quick diagnosis and cure strategies for viral diseases are essential for farmers. In summary, there is an urgent need to establish serological detection methods for accurate and sensitive detection of ReMV in R. glutinosa production areas. We used the CP coding sequence of ReMV for the prokaryotic expression of recombinant His-CPReMV from Jiaozuo County, Henan Province. The purified His-CPReMV protein was used as an antigen to immunize New Zealand white rabbits, and high-quality antiserum against His-CPReMV was obtained. Using the specific polyclonal antiserum against His-CPReMV, we established an accurate and sensitive serological method for detecting ReMV in R. glutinosa (Figure 2). Furthermore, we purified IgG antibodies from the polyclonal antiserum and obtained PAb-CPReMV. Using the ReMV-infected and uninfected R. glutinosa samples (1#, 5#, 2#, and 7#), we established high-throughput, accurate, and sensitive immuno-dot blotting and ELISA detection methods for ReMV from field plants (Figures 3–5). Recent studies have shown that an antiserum for ReMV is still lacking, especially for serological detection of ReMV in the field (Kubota et al., 2012; Hamada et al., 2019; Li et al., 2021). Our study generated polyclonal antiserum against His-CPReMV and PAb-CPReMV with high purity, sensitivity, and specificity. Using the PAb-CPReMV, we established high-throughput, accurate, and sensitive detection methods for farmers in R. glutinosa stable production and provided a quick and accurate detection method for preventing and controlling Rehmannia virus disease.
In addition, we obtained CP sequences from the ReMV in Jiaozuo County, Henan Province. The sequence has been uploaded to GenBank (Accession # OM964874). Then, the target sequence was aligned to the NCBI database using the online BLAST tool, and we found that the coding region of the CPTMV gene in some regions was highly like to the obtained target sequence, which implied that the obtained polyclonal antiserum or the PAb-CPReMV against the ReMV may have cross-reactivity with TMV in serological detections, which requires further investigation.
The target CPReMV gene sequence was compared to those previously deposited in the NCBI database, and these CP genes were from different regions in China. The results show that the CP gene sequence of the ReMV Henan Jiaozuo isolate had the lowest homology (94.1%) with that of the American ReMV isolate (MF348202.1) (Supplementary Figure S3), whereas the identities of CP genes (MG418836.1, KU133476.1, JX575184.1, JQ285996.1, LC571586.1, EF375551.1, and NC009041.1) of ReMV isolates from Korea, Shanxi, Japan, and Henan were 97.9% (Supplementary Figures S4A–H). Taken together, these results show that the ReMV CP sequence was relatively conserved, and there were no remarkable genetic differences. In this study, we analyzed only the CP gene of ReMV isolated from Jiaozuo City, Henan Province, which has certain limitations. In the follow-up, it is necessary to obtain samples from additional regions to further analyze ReMV isolates from different hosts and regions. However, we successfully cloned the coding sequence of CPReMV and expressed the recombinant His-CPReMV protein. Furthermore, we obtained polyclonal antiserum and PAb- CPReMV against ReMV, and established a serology-based, sensitive, accurate, and high-throughput immuno-dot blotting and ELISA for ReMV from R. glutinosa planted in plant fields. Our proposed methods filled the gap of serological-based detection of ReMV from R. glutinosa in the field and laid a foundation for further research on the pathogenesis of ReMV in R. glutinosa.
The datasets presented in this study can be found in online repositories. The names of the repository/repositories and accession number(s) can be found in the article/Supplementary material.
CF, JC, XG, and TG carried out the experiments. JC wrote the manuscript with support from KZ and JC helped supervise the project. CF conceived the original idea. All authors contributed to the article and approved the submitted version.
This work was supported by the National Natural Science Foundation of China (81903764, 31801699), the Excellent Youth Fund of Jiangsu Natural Science Foundation (BK20220116), and the Foundation of Guangdong Provincial Key Laboratory of High Technology for Plant Protection (zhizhong2021-07), and the Yangzhou Modern Agriculture Key R&D Project (YZ2021032).
We thank all the other members of Plant Virology Laboratory at Yangzhou University for their helpful suggestions and constructive criticism.
The authors declare that the research was conducted in the absence of any commercial or financial relationships that could be construed as a potential conflict of interest.
All claims expressed in this article are solely those of the authors and do not necessarily represent those of their affiliated organizations, or those of the publisher, the editors and the reviewers. Any product that may be evaluated in this article, or claim that may be made by its manufacturer, is not guaranteed or endorsed by the publisher.
The Supplementary Material for this article can be found online at: https://www.frontiersin.org/articles/10.3389/fsufs.2022.1013470/full#supplementary-material
Supplementary Figure S1. The genome organization of the rehmannia mosaic virus (ReMV). ReMV contains a single strand positive genomic RNA, and encodes fours proteins. ORF1 is the small subunit of the RNA-dependent RNA polymerase (RdRp), ORF2 is the readthrough proteins of ORF1, and encodes the big subunit of RdRp. These two subunit forms complexes and play essential roles in viral genomic RNA replications. ORF3 is the viral movement protein (MP), which is responsible for virus cellular and systemic movement. ORF4 is the viral coat protein (CP), which functions in virion assembly.
Supplementary Figure S2. Phylogenetic tree of obtained CP coding sequences of ReMV and other tobamoviruses' coat protein coding sequences deposited in NCBI. Numbers represent the percentage of for repeated for 1,000 times. The bar indicates the relative genetic distance. The bold letters indicate the CP coding sequence we submitted.
Supplementary Figure S3. The disease caused by the ReMV infection on R. glutinosa. The mosaic (A), necrosis (B), stunt (C), and yellowing (D) appeared in the Chinese traditional and herbal drug park in Henan Province.
Supplementary Figure S4. Sequences alignment of the CP sequences to the other sequences deposited in NCBI. (A–H) Two CP sequences alignment, one is the CP we submitted, other CP is the sequence deposited in NCBI.
Alon, D. M., Hak, H., Bornstein, M., Pines, G., and Spiegelman, Z. (2021). Differential Detection of the Tobamoviruses tomato mosaic virus (ToMV) and Tomato brown rugose fruit virus (ToBRFV) using CRISPR-Cas12a. Plants 10:1256. doi: 10.3390/plants10061256
Barker, K. (1998). At the Bench: A Laboratory Navigator. New York, NY: Cold Spring Harbor Laboratory Press.
Du, L., Xiang, J. L., Fan, J. L., Li, X., and Luo, L. (2013). Detection of tobacco mosaic virus (TMV) in Rehmannia glutinosa f. hueichingensis by IC-RT-PCR. China J. Chin. Mat. Med. 38, 2092–2095. doi: 10.4268/cjcmm20131311
Gao, Y. H., Li, M. F., Liu, D. M., Li, G. F., Wang, M., Wang, J. Z., et al. (2009). Identification and detection of a viral pathogen of Rehmannia glutinosa disease. J. Beijing Univ. Agric. 24, 31–34. doi: 10.13473/j.cnki.issn.1002-3186.2009.02.006
Hamada, T., Mise, K., Kiba, A., and Hikichi, Y. (2019). Systemic necrosis in tomato induced by a Japanese isolate of Rehmannia mosaic virus in a temperature-sensitive manner. Plant Pathol. 68, 1025–1032. doi: 10.1111/ppa.13006
Hou, H. Q., Zhang, Z. C., Lei, C. Y., Chen, R., and Zhang, D. S. (2007). Biological characteristics of rehmannia mosaic virus (ReMV). Chin. Agric. Sci.Bull. 23, 406–408. doi: 10.3969/j.issn.1000-6850.2007.09.087
Kang, C. X. (2017). Microorganism Mechanism of Continuous Cropping Obstacles of Rehmannia glutinosa and Development of Beneficial Microbial Inoculant. Henan Normal University, Henan.
Kubota, K., Usugi, T., Tomitaka, Y., Matsushita, Y., Higashiyama, M., Kosaka, Y., et al. (2012). Characterization of Rehmannia mosaic virus isolated from chili pepper (Capsicum annuum) in Japan. J. Gen. Plant Pathol. 78, 43–48. doi: 10.1007/s10327-011-0345-7
Lei, C. Y. (2006). Studies on the Genome Structure and Biologic Characteristics of Rehmannia Mosaic Virus. Henan Agricultural University, Henan.
Li, H. W., and Meng, X. L. (2015). Research progress on chemical constituents and pharmacological activities of Rehmannia glutinosa. Drug Eval. Res. 38, 218–228. doi: 10.7501/j.issn.1674-6376.2015.02.022
Li, X. R., Zuo, X., Li, M. M., Yang, X., Zhi, J. Y., Sun, H., et al. (2021). Efficient CRISPR/Cas9-mediated genome editing in Rehmannia glutinosa. Plant Cell Rep. 40, 1695–1707. doi: 10.1007/s00299-021-02723-3
Liefting, L. W., Waite, D. W., and Thompson, J. R. (2021). Application of oxford nanopore technology to plant virus detection. Viruses 13:1424. doi: 10.3390/v13081424
Lim, S., Zhao, F. M., Y, R.H., Davaajargal, L., Jeong, J. C., Lee, H., et al. (2016). Complete genome sequence of Rehmannia mosaic virus infecting Rehmannia glutinosa in South Korea. Genome Announc. 4, e01595–e01515. doi: 10.1128/genomeA.01595-15
Mehetre, G. T., Leo, V. V., Singh, G., Sorokan, A., Maksimov, I., Yadav, M. K., et al. (2021). Current developments and challenges in plant viral diagnostics: a systematic review. Viruses 13:412. doi: 10.3390/v13030412
Rubio, L., Galipienso, L., and Ferriol, I. (2020). Detection of plant viruses and disease management: relevance of genetic diversity and evolution. Front. Plant Sci. 11:1092. doi: 10.3389/fpls.2020.01092
Song, M. Y. (2021). Study on the material benchmarking of the classical formula peach red siwu soup. Heilongjiang University of Traditional Chinese Medicine, Heilongjiang, China.
Tian, B. (1962). About a virus isolated from Rehmannia glutinosa in Henan. J. Microbiol. 8, 418–419.
Villamor, D. E. V., Ho, T., Al Rwahnih, M., Martin, R. R., and Tzanetakis, I. E. (2019). High throughput sequencing for plant virus detection and discovery. Phytopathology® 109, 716–725. doi: 10.1094/PHYTO-07-18-0257-RVW
Wang, D. F., Zhang, X. M., Guo, S., Shen, S. F., Long, D. D., LI, L.Y., et al. (2021). Complete genome sequencing and analysis of Rehmannia mosaic virus isolate from Shanxi province. Vol. 28, p. 58–65.
Wang, F. Q., Xie, C. X., Sun, R. B., and Zhang, Z. Y. (2018). Progress on germplasm enhancement and breeding of Rehmannia glutinosa. China J. Chin. Mat. Med. 43, 4203–4209. doi: 10.19540/j.cnki.cjcmm.20180820.012
Wang, T. X., Li, J. Y., and Hu, Z. H. (2004). Advances in studies on morphology and main chemical constituents of Rehmannia glutinosa. Chin. Trad. Herb. Drug. 35, 585–587.
Wang, Z. J., Wei, G. D., and Ma, S. T. (2015). Chemical and pharmacological effects of Rehmanniae radix polysaccharides. Chin. J. Exp. Trad. Med. Formulae 21. doi: 10.13422/j.cnki.syfjx.2015160231
Zhang, K., Zhuang, X. J., Xu, H. M., Gan, H. F., He, Z., and Chen, J. H. (2022). Development of polyclonal antibodies-based serological methods and a DIG-labelled DNA probe-based molecular method for detection of the vicia cryptic virus-M in field plants. J. Virol. Methods 299:114331. doi: 10.1016/j.jviromet.2021.114331
Zhang, K., Zhuang, X. J., Xu, H. M., Guo, X., He, Z., Xu, K., et al. (2021). Sensitive and high-throughput polyclonal antibody-based serological methods for rice stripe virus detection in both rice and small brown planthopper. Crop Prot. 144:105599. doi: 10.1016/j.cropro.2021.105599
Keywords: rehmannia mosaic virus, antiserum, polyclonal antibodies, detection, application
Citation: Chen J, Feng C, Guo X, Zhou Y, Gu T, Zhuang X, Cheng L and Zhang K (2022) Development of polyclonal antibodies-based serological methods for detection of the rehmannia mosaic virus in field plants. Front. Sustain. Food Syst. 6:1013470. doi: 10.3389/fsufs.2022.1013470
Received: 07 August 2022; Accepted: 16 September 2022;
Published: 04 October 2022.
Edited by:
Lei Jiang, Anhui Agricultural University, ChinaReviewed by:
Hongguang Cui, Hainan University, ChinaCopyright © 2022 Chen, Feng, Guo, Zhou, Gu, Zhuang, Cheng and Zhang. This is an open-access article distributed under the terms of the Creative Commons Attribution License (CC BY). The use, distribution or reproduction in other forums is permitted, provided the original author(s) and the copyright owner(s) are credited and that the original publication in this journal is cited, in accordance with accepted academic practice. No use, distribution or reproduction is permitted which does not comply with these terms.
*Correspondence: Lei Cheng, Y2xwaDI5OUAxNjMuY29t; Kun Zhang, emtAeXp1LmVkdS5jbg==; emhhbmdrdW44ODAzMjRAY2F1LmVkdS5jbg==
†These authors have contributed equally to this work
Disclaimer: All claims expressed in this article are solely those of the authors and do not necessarily represent those of their affiliated organizations, or those of the publisher, the editors and the reviewers. Any product that may be evaluated in this article or claim that may be made by its manufacturer is not guaranteed or endorsed by the publisher.
Research integrity at Frontiers
Learn more about the work of our research integrity team to safeguard the quality of each article we publish.