- 1CEB-Centre of Biological Engineering, University of Minho, Braga, Portugal
- 2LABBELS—Associate Laboratory in Biotechnology, Bioengineering and Microelectromechanical Systems, University of Minho, Braga, Portugal
The odor of four algae was investigated and compared to evaluate the potential of these algae to mimic shrimp aroma. Solid-phase microextraction followed by gas chromatography analysis coupled with sensory analysis was used for performance assessment. The volatile organic compounds were determined in non-processed, raw samples (r), and processed cooked (c) and cooking water (w) samples for two microalgae [Nannochoropsis oceanica (NO) and Tetraselmis chuii (TC)], two macroalgae [Ulva rígida (UR) and Saccharina latíssima (SL)], and shrimp Vannamei cong (SH). The results showed significant differences in the composition of volatile compounds between macroalgae and microalgae. The key odorants in macroalgae were octanal, 2-octenal, nonanal, and β-ionone, and in microalgae were 1,5-octadien-3-ol, hexanal, 2,4-decadienal, 2-octenal, octanal, nonanal, 3,5-octadien-2-one, and terpenes. The PCA analysis of GC-MS data showed odor similarities between the studied samples, which were divided into five main groups: (1) TC(c) and TC(w); (2) TC(r) and NO(c); (3) NO(r), NO(w), and SL(w); (4) SL(c), UR(r), UR(c), and UR(w); and (5) SL(r). The data from the sensory analysis show bigger similarities between the macroalgae and the shrimp odor. Overall, the data provided indicate that the cooking water and cooked samples are very similar in key components of odorants. These features allow the possibility to use algae and their processed resulting products as a shrimp flavor replacement in non-animal-based food formulations, thus decreasing the pressure on seafood crops and aquaculture-associated issues leading to more sustainable livestock. Furthermore, circularity and waste reduction may be further enabled by the use of otherwise wasted cooking water as an odorant agent.
Introduction
The Food and Agriculture Organization (FAO) claims that global meat production is expected to double by 2050, due to the increase in world population which is estimated to reach 10 billion people within the next 30 years. This fact makes the search for new alternative proteins an objective of the utmost importance (Dopelt et al., 2019). Due to the enormous increase in the world population, awareness of animal welfare, health, and ecological impact, the study and investment in plant-based alternatives for meat and dairy products have been rapidly increasing (van Vliet et al., 2020), yet fish and seafood plant-based alternatives have been neglected (Koyande et al., 2019). Aquatic animal food consumption, such as finfish, mollusks, crustaceans, and cephalopods, provides 17% of human dietary intake of animal protein, although large disparities are ostensible across regions (FAO, 2018). Yet, dietary guidelines recommend fish as part of sustainable healthy diets, up to 28 g/d (Willett et al., 2019). It is known that dietary fish consumption has been linked to human health outcomes associated with the prevention of stroke and cardiovascular disease, as well as fish consumption during pregnancy is linked to the neurodevelopment of offspring (Iannotti et al., 2021).
The replacement of meat and fish proteins for plant proteins is one promising strategy to reduce meat consumption, although most of the products are not well-accepted by meat consumers due to their low sensory appeal. Due to this issue, industries have been moving toward the development of plant-based products with meat-like sensory attributes, commonly referred to as meat analogs (Fiorentini et al., 2020).
Micro- and microalgae are gaining an increasing interest in the EU bioeconomy. Besides being ecological, vegan, and healthy products (Geada et al., 2021), they also have an interesting nutritional profile: microalgae protein can represent up to 70% of total algae biomass and in macroalgae up to 22% (Bleakley and Hayes, 2017). Although algae-based products are trendy and dozens of food products have been already launched into the market in the food sector, one of the major reported issues is their undesirable strong marine taste and odor (Koyande et al., 2019; Lafarga, 2019, 2020). It is proven that flavor can be the decisive factor in the choice of a particular product by the consumer, and algae's odor can act as a pro or con in decision-making.
The classic and characteristic fishy and seafood algae's odor comes from a complex mixture of different volatile organic compounds (VOCs) and their concentrations. These products are mainly composed of polyunsaturated fatty acids, aldehydes (e.g., hexanal, 2-heptenal, 2-octenal, and 2,6-nonadienal), alcohols (e.g., 3,5-octadien-2-ol and 1-octen-3-ol), and ketones (e.g., 1-octen-3-one and 3,5-octadien-2-one), and also sulfur compounds and nitrogen-containing compounds, such as trimethylamine, although there can be significant differences between the algal species (Varlet and Fernandez, 2010; Isleten Hosoglu, 2018; Coleman et al., 2022). VOCs are molecules with high vapor pressure, moderate hydrophilicity, and low molecular weight (Garicano Vilar et al., 2020), and are produced by algae depending on the species, process, culture, and environmental conditions. Besides these VOCs, the odor activity value (OAV) also plays a major role in identifying the key odorants because it estimates the importance of a flavor compound in food, based on the ratio of its concentration to its odor threshold concentration in that food. This means that only compounds which exceed the threshold level in food will impact the flavor and thus sensory perception. Therefore, the larger the OAV, the greater the referred compound will contribute to the overall odor (Garicano Vilar et al., 2020).
Although algae have been reported to be used as a flavor ingredient in plant-based seafood alternatives and some studies have found similar VOCs between algae and seafood (Coleman et al., 2022; Moran et al., 2022), to the best of the authors' knowledge, there are no studies on the VOCs present in algae in different matrices resulting from food processing, such as raw, cooked, and cooking water samples. Therefore, the objective of this study was to investigate the potential of micro- and macroalgae to be used as flavor ingredients in plant-based shrimp alternatives. The study focused on cooked algae and/or their cooking water to benchmark with shrimp-based foods, and their VOCs were compared with the freeze-dried matrix. Two microalgae [Nannochoropsis oceanica (NO) and Tetraselmis chui (TC)] and two macroalgae [Ulva rígida (UR) and Saccharina latíssima (SL)] were studied to determine the VOCs present in the processed and non-processed samples and their similarity to shrimp odor was analyzed using a combination of sensory evaluation and chemical profiling techniques by headspace solid-phase microextraction (HS-SPME) followed by gas chromatography coupled to mass spectrometry (GC-MS).
Materials and methods
Algae and shrimp processing
The raw biomass samples of microalgae TC and NO were obtained as a power, kindly provided by AllMicroAlgae, Portugal. The raw biomass samples of macroalgae UR and SL were also obtained as power from a local producer (AlgaPlus, Portugal) and milled before analyses. The shrimps (SH), species vannamei cong, were bought frozen at a local supermarket raw with head and carapace and used after thawing.
To obtain the processed samples, 2 g of dried microalgae, macroalgae, or shrimp was placed in a 50 mL glass bottle with 20 mL of water and immersed in a water bath at 98°C for 5 min. Then the mixtures were centrifuged at 330 g for 15 min, and the two phases were separated (liquid and solid). The solid phase was considered as cooked samples (c) and the liquid phase as cooking water (w).
To obtain the non-processed samples, 0.5 g of powder microalgae and macroalgae or thawed shrimp was directly placed into a 20 mL vial and prepared for HS-SPME extraction.
Headspace solid-phase microextraction of volatile compounds
The VOCs of r, c, and resulting w were extracted using an HS-SPME method developed by Zhang and coworkers (Zhang et al., 2020) with slight modifications. This microextraction technique is solvent-free, cheap, easy to use, relatively fast, needs a low volume of sample, and is also sensitive enough for quality control purposes (Iranmanesh et al., 2018).
For extraction and absorption of compounds, 0.5 g of solid sample (r and c) or 0.5 mL of w samples was transferred to a 20 mL vial with a three-phase SPME fiber (divinylbenzene (DVB)/carboxen (CAR)/polydimethylsiloxane (PDMS), 50/30 μm) from Supelco (Bllefonte, PA, USA). Moreover, 5 μL of internal standard (IS), 6-methyl-5-hepteno-2-one (from Sigma-Aldrich, Missouri, USA), in n-hexane at concentrations of 0.1 ug/mL (for TC, NO, and SL) and 0.02 ug/mL (for SH and UR) were added to each sample prior to incubation. The vial was incubated in an oven at 60°C for 40 min. The absorption conditions of the compounds were selected taking into account the previous results (data not shown) and studies reported in the literature (Zhang et al., 2020). Before incubation, fiber was conditioned into the injector of a gas chromatograph for 20 min at 250°C and was used immediately to prevent contamination.
After incubation, the volatile compounds were analyzed in GC-MS. The analyses were done in triplicate.
Gas chromatography-mass spectrometry analysis
The samples were analyzed in a GC coupled with a mass detector (MS) equipped with an ion-trap analyzer (Shimadzu QP2020 NX, Kyoto, Japan). The volatile compounds were desorbed by inserting the fiber into the injection port, which remained in the injector for 5 min at a temperature of 250°C. The split injection of samples was set at a ratio of 1:10, while helium (99.999% purity) was used as a carrier gas at a flow rate of 1.2 mL/min. Compounds were separated by using a Stabilwax capillary column (30 m × 0.25 mm × 0.25 μm film thickness, Agilent). The column temperature was programmed as follows: it was held at 40°C for 3 min, and then increased to 100°C at a rate of 5°C/min, to 180°C at a rate of 2°C/min, to 250°C at a rate of 10°C/min, and then maintained at 250°C for 5 min (Zhang et al., 2020). The MS ionization energy (EI) was 70 eV, the ion source temperature was 230°C, and the interface detector temperature was 250°C. The mass scanning range (m/z) was 40–450 at a scanning rate of 1.8 scans/s as described previously (Zhang et al., 2020).
Identification and quantitative analysis of volatile compounds
The volatile compounds were identified based on mass spectral interpretation and comparison with the National Institute of Standards and Technology (NIST) and WILEY libraries installed in GC–MS (match quality > 80%).
A semi-quantitative determination of the volatiles was done by directly comparing them with IS (Gu et al., 2013). The area of the chromatographic peak of each identified volatile was divided by the area corresponding to the IS. To semi-quantitatively calculate the concentrations of each volatile, the obtained responses were multiplied by the concentration of IS in the sample, assuming that all of the response factors were equal to one (Coleman et al., 2022). Odor activity values (OAVs) were calculated as the ratio of their concentration to odor thresholds in water. Only aroma compounds exceeding their odor threshold concentration are suggested to contribute to the overall aroma of food. Odor thresholds in water were determined following a previously published protocol (van Gemert, 2011). Three replicate analyses were performed on each sample.
Sensory analysis
To evaluate the similarity of smell between algae and shrimp samples, a non-trained panel consisting of 50 assessors (17 men and 33 women, aged between 22 and 65 years) was used. A difference test (used to determine if a sensory difference, such as odor, taste, and texture, exists between samples) was performed using the shrimp sample as a control (Meilgaard et al., 2016).
Using only the olfactory tract, the panelist was asked to classify the algal samples from 1 (no similarity) to 7 (equal odor) by comparing the shrimp smell with raw, cooked, and water samples.
Statistical analysis
Principal component analysis (PCA) was carried out to visualize the differences between the concentration of different samples based on GC-MS and sensory analysis data using IBM SPSS statistics (version 27; Granato et al., 2018). In the case of the GC-MS results, the concentration of 42 volatile compounds detected by the GC-MS was taken into consideration when building the matrix data set. The mean of at least three trials was used, and the results were presented in ng/g. Furthermore, SPSS statistics used a correlation matrix during data processing with the direct oblimin rotation method with Kraiser normalization.
Results and discussion
In today's reality, the importance of fisheries cannot be underestimated, especially due to the huge demand for animal protein and the importance of fish nutrition. Aquaculture has been contributing to the growth of seafood and fish supply intended for the human supply, although the literature has highlighted some harmful results of aquaculture production and its environmental and ecological impacts. It is known that the rapid growth in shrimp farming is a key driver of mangrove forest degradation and the reduction of natural habitats and biodiversity. Also, aquaculture production may lead to a decrease in biodiversity and nutrition diversity, as it usually focuses on a few selected species (Sampantamit et al., 2020). Therefore, sustainable alternatives to seafood and seafood crops are of upcoming importance. However, for a meat/fish/seafood replacer to succeed in the market, it must be first accepted by the public in terms of overall liking, and the odor is the key in this aspect (Fiorentini et al., 2020).
The results comprising the identification of VOCs, their relative concentration, and OAVs of the different processed and non-processed microalgae, macroalgae, and shrimp, are presented in Tables 1–3, respectively. Figures 1A–C show the chromatograms of rNO, cNO, and wNO as an example.
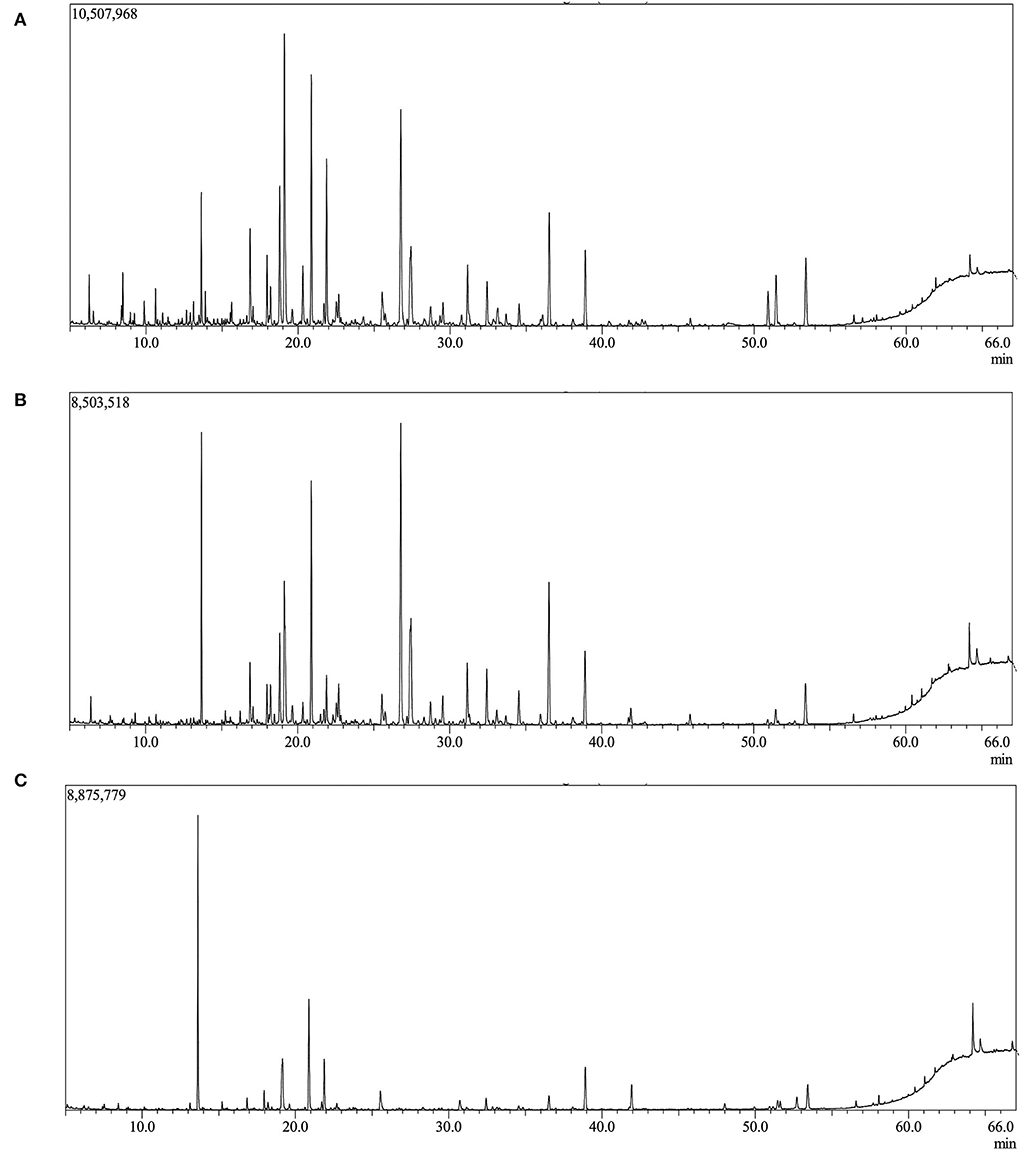
Figure 1. Chromatogram by GM-MS from (A) raw Nannochloropsis oceanica, (B) cooked Nannochloropsis oceanica, and (C) cooking water from Nannochloropsis oceania.
Aroma-active compounds in microalgae
Following the volatile analysis, a total of 103 compounds were primarily identified in microalgae NO and TC. Of these, only a total of 42 were considered for both microalgae due to the absence of an odor descriptor for the other compounds. Table 1 shows the sum of the concentration and OAVs of volatiles within a specific group to reveal similarities and differences between the aroma profiles of r, c, and w microalgae. These are composed of several classes of organic compounds: esters and hydrocarbons were the smallest groups with only one compound identified, followed by terpenes and acids (2), ketones (3), alcohols (3), and aldehydes were the largest group (13) (Table 1).
The origin of ketones is variable, but generally linear ketones are derived from lipid oxidation, while methyl ketones may result from the β-oxidation of the fatty acids and subsequent decarboxylation (Moran et al., 2022). In general, saturated ketones are related to sweet, floral, and fruity odor notes, while unsaturated ketones are responsible for green odor notes. Ketones of VOCs were low in samples and only one was found with OAV>1 (rNO, cNO, and rTC), namely, 3,5-octadien-2-one. Furthermore, 3,5-octadien-2-one concentration was three times higher in NO than in TC. These results agree with Coleman et al. (2022) who refer 3,5-octadien-2-one as one of the major compounds that contribute to the overall aroma of NO and TC and confer a mushroom/earthy smell. Moreover, previous studies related the seafood-like odor in microalgae to their high content of diketones, such as 3,5-octadien-2-one (Van Durme et al., 2013; Coleman et al., 2022; Moran et al., 2022). Similarly, our results also suggested that 3,5-octadien-2-one may have a major contribution to the aroma not only in the raw microalgae matrix but also in c and w NO samples, though in a milder way in the processed samples.
Most aldehydes have low threshold values and are important VOCs of aquatic products. In this study, only short-chain aldehydes (between 5 and 10 carbons) were found, probably due to the low extraction temperature used. Seven aldehydes were found to be present in rNO with a significant impact on the overall aroma, namely, hexanal, 2-octenal, benzaldehyde, 2,4-decadienal, octanal, trans-2-cis-6-nonadienal, and nonanal. In rTC, the main compounds are 2,4-decadienal, hexanal, octanal, and nonanal, but only the last one was present in the three types of samples (r, c, and w).
In microalgae, alcohols are mainly formed as a result of secondary decomposition of hydroperoxides of fatty acids, except for branched alcohols which come from carbohydrates via glycolysis or from amino acids through the Ehrlich pathway (Giri et al., 2010). In contrast to other studies (Coleman et al., 2022; Moran et al., 2022), 1-octen-3-ol does not seem to have an impact on the overall aroma (Van Durme et al., 2013), although the isomer trans-2-octen-1-ol shows an effect. In the present study, 1,5-octadien-3-ol has high OAV for NO and TC, especially in the raw form.
Previous studies conducted on lyophilized algae have ascribed the strong fishy/ grassy odors of the NO and TC to the presence of hexanal, 2,6-nonadienal, 2,4-decadienal, and 1-octen-3-ol. As aforementioned, in the current work, the results did not support the hypothesis that 2,6-nonadienal is a key odorant in TC due to the absence of the compound. Furthermore, due to their high odor threshold, 1-octen-3-ol also seems to not justify that strong fishy odor, as its OAV is < 1.
Furans can provide a burned, savory, sweet, and green aroma to foods (Starowicz and Zieliński, 2019). In rNO, cNO, and rTC samples, 2-penthylfuran, an oxidative derivative of n-6 PUFAs characterized by their green notes, was detected, which may significantly contribute to their aroma in the raw samples, especially in NO.
High carotenoid concentrations are normally associated with a high concentration of ionones (terpenes) that are produced due to reactions with the unstable conjugated double-bound structure of carotenes. At the end of the degradation of carotenoids, where their long-chain compounds are mostly oxidized, large amounts of short-chain monooxygenated and deoxygenated compounds, such as β-cyclocitral, are formed (Van Durme et al., 2013). In NO, it seems that cNO contains a higher concentration of terpenes, particularly β-ionone, when compared with the raw and liquid samples. Yet for TC, the raw sample has an OAV almost three times higher than the cTC. Also, α-ionone is present in both cTC and cNO, although in smaller concentrations. Moreover, for both wNO and wTC samples, α-ionone was not detected.
The smell of either rNO, cNO, and rTC can be explained by the mixture of 1,5-octadien-3-ol, hexanal, 2,4-decadienal, 2-octenal, octanal, nonanal, 3,5-octadien-2-one, and terpenes. cNO shows the same key compounds as rNO, although in a smaller concentration, except for 2,4-decadienal, nonanal, and terpenes. wNO seems to present a softer smell when compared with the two other NO samples (rNO and cNO) due to the lack or low concentration of some of the key odorants. The odor of cTC and wTC seems to be contributed by nonanal and terpenes, and in cTC also by octanal and 2-octenal. wNO sample seems to retain 1,5-octadien-3-ol, 2-octenal, nonanal, 3,5-octadien-2-one, and terpenes.
Aroma-active compounds in macroalgae
Seventy-seven VOCs were primarily identified for SL and 79 for UR. Of these, only a total of 27 were considered due to the absence of an odor descriptor for the other compounds, as shown in Table 2.
These are composed of several classes of organic compounds: acids were the smallest group (2 compounds), followed by terpenes (2), hydrocarbons (3), alcohols (3), ketones (5), and aldehydes (11).
Only one alcohol was found to have an impact on the overall aroma of raw SL, that is, the trans-2-octen-1-ol. Alcohols in macroalgae derive from unsaturated acids by peroxidation reactions, from carbohydrates by glycolysis, or from amino acids (Sánchez-García et al., 2019). A study performed by Pina et al. (2014) found 1-octen-3-ol and other alcohols in dried red macroalgae, but only ethanol and 1-penten-3-ol in samples submitted to different culinary treatments. Branched-chain alcohols like 1-octen-3-ol are often associated with fishy and grassy aromas and seem to contribute significantly to the aromatic fraction in macroalgae if their low odor threshold values are considered, although in this study, only trans-2-octen-1-ol showed an OAV>1 (Sánchez-García et al., 2019).
Three aldehydes were present in both UR and SL: octanal, nonanal, and 2-octenal. In general, linear and branched chain aldehydes contribute to herbaceous and grassy aromas, while unsaturated aldehydes provide green and fishy odors (Sánchez-García et al., 2019). Some authors have reported that, in macroalgae, short- and middle-chain aldehydes are derived from fatty acids (Akakabe and Kajiwara, 2008). Nonanal was found in all studied algae, and in contrast to all other compounds, their concentration was higher in cooking water and in the cooked sample. On the other hand, 2-octenal was found only in raw and cooked matrices in both macroalgae. In contrast to other studies, hexanal was found in both macroalgae, although it does not seem to play a role in overall aroma (Sánchez-García et al., 2019).
Regarding hydrocarbons, only three were detected, but none seem to have an impact on the overall aroma.
The PCA was performed to explore further similarities of odor among processed and non-processed algal samples based on GC-MS data. The raw matrix data set is presented in Supplementary Table 1. Figure 2 shows the loading plots of VOC concentration with OAV > 1 from GC-MS of all processed and non-processed algae. The samples seem to be grouped into five groups: (1) cTC and wTC; (2) rTC and cNO; (3) rNO, wNO, and wSL (4) cSL, rUR, cUR, and wUR; and (5) rSL. Although groups 2, 3, and 4 are in the same quadrant, the distance and the deviation between them are different. The processed macroalgae tend to be more similar to each other due to the already identified VOCs, such as 1,5-octadien-3-ol, 2,4-decadienal, hexanal, nonanal, octanal, α-ionone, β-ionone, and 3,5-octadien-2-one. The similarity between rNO, cNO, wNO, rSL, and wSL may be due to the compounds nonanal, β-ionone, and 3-buten-2-one.
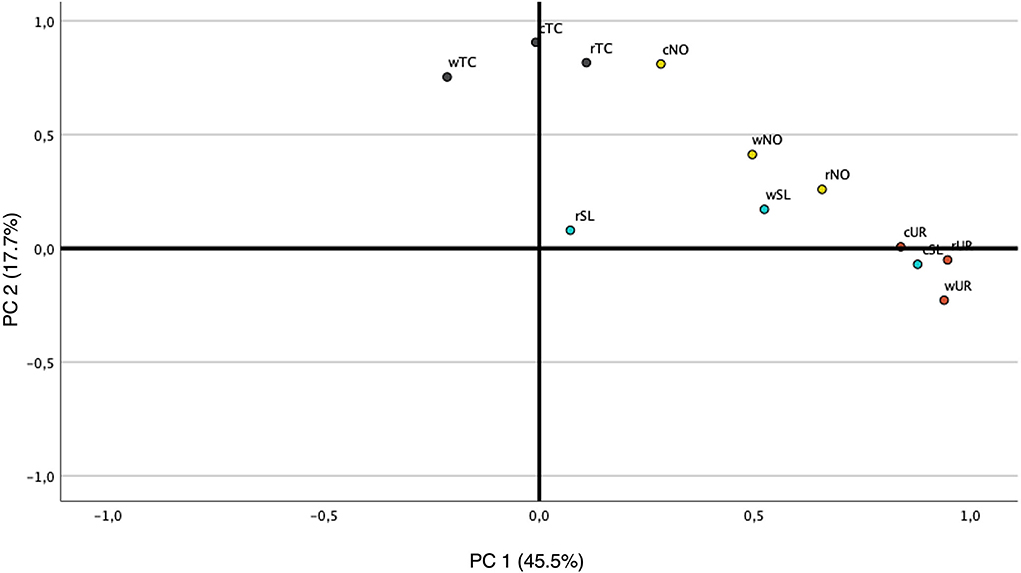
Figure 2. PCA correlation loadings plot for active aroma compound concentrations from GC-MS in the four processed and non-processed algae. rNO, raw Nannochoropsis oceanica; rTC, raw Tetraselmis chuii; rSL, raw Saccharina latissima; rUR, raw Ulva rígida; cNO, cooked Nannochoropsis oceanica; cTC, cooked Tetraselmis chuii; cSL, cooked Saccharina latissima; cUR, cooked Ulva rígida; wNO, water Nannochoropsis oceanica; wTC, water Tetraselmis chuii; wSL, water Saccharina latissima; wUR, water Ulva rígida.
The key odorants present in microalgae belong essentially to alcohols (1,5-octadien-3-ol and trans-2-octen-1-ol), aldehydes (2,4-decadienal, hexanal, octanal, 2-octenal, trans-2,cis-6-nonadienal, and nonanal), terpenes (α-ionone and β-ionone), and ketone (3,5-octadien-2-one) groups. Macroalgae odor seems to be characterized fundamentally by nonanal, terpenes, and 2-octenal. Compared to microalgae, the amount and concentration of key odorants found in macroalgae are much lower, and it seems that their smell is more green than fishy. As found in microalgae, terpenes play a major role as VOCs, especially β-ionone. Also, between processed and non-processed algae, some compounds are different; for example, hydrocarbon was not found in processed algae. Cooking waters presented a lower number of compounds and, in general, a low concentration of the presented compounds compared with those observed in raw and cooked algae.
Comparison of aroma-active compounds in shrimp and algae
For shrimp volatile analysis, a total of 21 compounds were primarily identified. Of these, only six were considered due to the absence of an odor descriptor for the others (Table 3).
In this work, the major odorant in SH was found to be nonanal. The results obtained for cSH were not similar to those reported in the literature. Zhang et al. (2020) have described that shrimp's key odorants are mainly pyrazine, such as 3-ethyl-2,5-dimethylpyrazine and 2,5-dimethyl pyrazide, trimethylamine, and 3-(methyltio)propionaldehyde. On the other hand, Mall and Schieberle (2017) have reported that 3,5-octadien-2-one and 2-acetyl-1-pyrroline are the main odorants in the studied shrimps. This divergence between the studies could be due to different shrimp samples (Penaeus vannamei and Litopenaeus vannamei), shrimp conservation method (fresh vs. frozen), shrimp processing method (e.g., raw, cooked, hot air dry, and fried), different extraction protocol (e.g., HS-SPME vs. liquid extraction), or different GC columns (such as HP-5MS, Agilent, or Stabilwax), among others. Furthermore, these works only consider the odorant concentration and not OAVs.
In the present study, although 3,5-octadien-2-one was detected, their OAV does not support it as a key odorant. Yet, similar to algae, nonanal (OAV = 16.83; 29.84; 50.04 for raw, cooked, and boiled water, respectively) seems to play a major role in shrimp's aromatic profile. When looking at the PCA which correlates the VOC concentrations of the algae and shrimp samples by GC-MS (Figure 3), it appears that wTC is most similar to the odor of rSH and cSH, probably due to the high concentration of nonanal, 2-ethyl-1-hexanol, and butyl acetate, although neither 2-ethyl-1-hexanol nor butyl acetate seems to have an impact on the overall aroma (OAV < 1). In addition, four main groups were detected: (1) rSH, cSH and wTC; (2) cTC, rTC, cNO, and wSL; (3) wNO, rNO, rUR, cUR, wUR, and cSL; (4) wSH and rSL. Based on this information, it seems that microalgae, especially TC (processed and non-processed), have the potential to mimic fish odor although their concentration needs to be tuned. Macroalgae, except for wSL and wSL, seem to have a different odor from that of SH in all the samples studied.
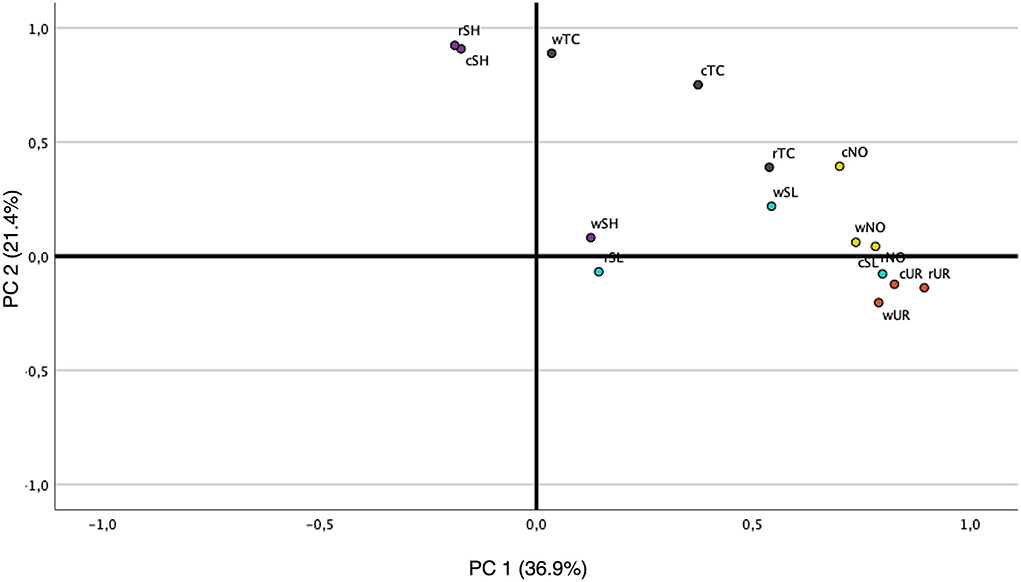
Figure 3. PCA correlation loadings plot for volatile organic compound concentrations from GC-MS in the four processed and non-processed algae and shrimp samples. rNO, raw Nannochoropsis oceanica; rTC, raw Tetraselmis chuii; rSL, raw Saccharina latissima; rUR, raw Ulva rígida; cNO, cooked Nannochoropsis oceanica; cTC, cooked Tetraselmis chuii; cSL, cooked Saccharina latissima; cUR, cooked Ulva rígida; wNO, water Nannochoropsis oceanica; wTC, water Tetraselmis chuii; wSL, water Saccharina latissima; wUR, water Ulva rígida.
Sensorial evaluation of samples
Sensory analysis reveals that odor is an important part of overall appeal and a major factor in the selection of a particular product by a consumer (Fiorentini et al., 2020). The overall sensory perception is a dynamic process and occurs from the start of smell/taste to the end of oral processing. Table 4, which presents the mean (M) and standard deviation (SD) of the panelists' scores based on the similarity of the algae compared to shrimp, indicates that within the raw samples, UR is the most similar one to shrimp (4.00 ± 2.03) followed by SL (3.00 ± 2.01). These results are supported by Figure 4 which indicates the sum of the scores provided by the panelist (rUL = 200, rSL = 178, rTC = 155, and rNO = 150 out of 350), in which rUL is given the best score in terms of resemblance to rSH odor. In the processed algae, SL seems to be the best-ranked sample (5.00 ± 1.94) followed by UR (4.00 ± 1.91), and again Figure 4 supports this statement (cUL = 205, cSL = 210, cTC = 130, and cNO = 141 out of 350). Finally, in the cooking water, macroalgae were again indicated as the most similar (SL = 4.00 ± 1.70 and UR = 4.00 ± 1.50), and the sum of the scores was 213 for wSL, 107 for wTC, 144 for wNO, and 180 for wUL. In other words, sensory evaluation demonstrates that the smell of macroalgae is more similar to the smell of shrimp than microalgae. The reason for this contradiction between GC analysis data and the sensory panel may be justified by the intensity of the odor. Even though the same amount of processed and non-processed algae was used during the sensory analysis (0.5 g or 0.5 mL), their odor intensity (OAV) was not the same, as verified by the results of VOCs presented in Tables 1–3. Given that the desired sensory evaluation analysis compared the samples' odor with that of shrimp and that the microalgae have a more intense smell than both macroalgae and shrimp, this factor may have interfered with the test, leading to a choice toward softer odors. Also, this could be the reason why SD values are so high. The members of the panel have commonly referred that although microalgae have a more marine odor, their odor intensity was very different from that of shrimp, and the softer smell of macroalgae could be a factor to rank them more likely to shrimp odor. Also, a non-trained panelist was used in this study, so this duality when scoring leading to a higher SD could be justified by that. The interesting fact provided in Figures 2, 3 is the similarity of VOCs between the matrices containing algae (raw or cooked) and the cooking water. The data provided by GC-MS (Figure 2) seem to indicate that the cooking water of TC, NO, and UR are extremely similar to their processed matrix, differing in the concentration of compounds and consequently in the intensity of the odor. Furthermore, the evidence presented in Figure 3 suggests that the wTC odor resembles the rSH and cSH. The raw matrix data set for the PCA of the sensorial analysis results is presented in Supplementary Table 2. Moreover, the input from sensory analysis seems to indicate that all cooking waters are identical to their cooked matrix. This is a highly interesting indicator and has immense potential in terms of sustainability (biorefinery), for food production and consumers' perception of foods. A shortcoming could be consumer acceptance, although it seems that people who are concerned about food sustainability and animal welfare consider meat/fish analog as an alternative option. However, consumer studies showed that most countries are not yet ready to accept analogs in their daily diet. Yet, literature shows that several studies have used sensory evaluation to analyze meat analogs in terms of odor, appearance, taste, and texture of products with great consumer perception (Los Ricardo et al., 2021; Fu et al., 2022; Silva et al., 2022). As mentioned earlier, algae are rich not only in protein (most relevant in microalgae), but also in vitamins and minerals (Bleakley and Hayes, 2017), and for this reason, products have been developed for human consumption based on algae or using algae in their composition. However, one of the disadvantages to its application during food formulation is its fishy flavor, which in some foods can be a disadvantage, but in others, it brings an enormity of benefits (Koyande et al., 2019; Lafarga, 2019, 2020).
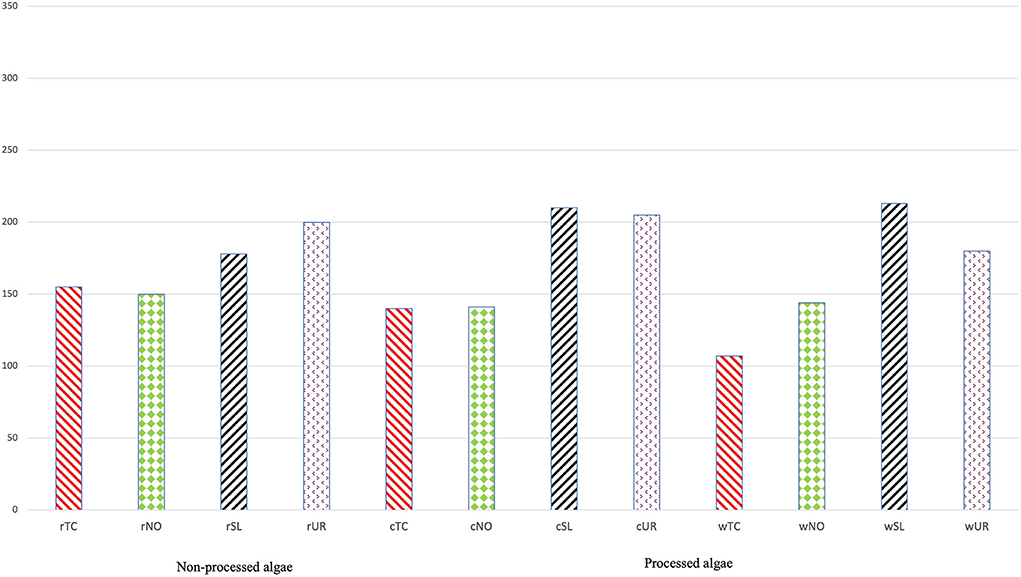
Figure 4. Evaluation sum of panelists' scores. rNO, raw Nannochoropsis oceanica; rTC, raw Tetraselmis chuii; rSL, raw Saccharina latissima; rUR, raw Ulva rígida; cNO, cooked Nannochoropsis oceanica; cTC, cooked Tetraselmis chuii; cSL, cooked Saccharina latissima; cUR, cooked Ulva rígida; wNO, water Nannochoropsis oceanica; wTC, water Tetraselmis chuii; wSL, water Saccharina latissima; wUR, water Ulva rígida.
The market for meat substitutes has been growing exponentially in the past few years (van Vliet et al., 2020). However, very little attention has been paid to fish and seafood analogs (Koyande et al., 2019). This paper has shown the potential of NO, TC, SL, and UR to contribute to the mimicking of the odoriferous power of shrimps and also of their cooking waters. The use of cooking water not only has an economic impact by reducing the costs associated with the development of these products, but also has an environmental impact by helping not only in the reuse of water but also in the recycling of algal products. Pre-washing seaweed with water is a standard procedure when using it for protein or carbohydrate extraction. In the same way, the reuse of the soaking water in order to utilize the VOCs in it would reduce waste and contribute to the sustainability of the process (Ferreira-Santos et al., 2020).
Thus, this by-product can be used as a shrimp smell mimic and has the advantage of having a lower smell intensity when compared to raw or cooked macroalgae. From the food industry perspective, the present work boosted the application of NO, TC, SL, and UR cooking waters as possible flavorings of the shrimp odor, thus reducing shrimp farming, water waste, and promoting a circular economy.
Conclusion
Assessing the VOCs of micro- and macroalgae is important when targeting food applications, as the marine flavor and odor attributed by many algae could be used as a strategy to develop innovative and novel foods. Based on the GC-MS results, the microalgae showed the most promising potential to mimic the odor of seafood, more specifically shrimp, due to the presence of 1,5-octadien-3-ol, trans-2-octen-1-ol, 2,4-decadienal, hexanal, octanal, 2-octenal, nonanal, α-ionone, β-ionone, and 3,5-octadien-2-one, identified as key odorants. From the sensory analysis data, macroalgae showed the best results when questioning the similarity between algae and shrimp odor. The panelist scores were higher for the processed algae, and this can be justified by an increase in the power smell in the shrimp standard, increasing the resemblance between algae and shrimp samples. For both macro- and microalgae, non-processed and cooked algae showed a more intense odor when compared to the cooking water.
The results showed the potential of algae to mimic seafood odor, being clear that the amounts to be used should be tuned to match the desired result. Furthermore, the use of their cooking waters may be beneficial in achieving this aim. The use of algae and their cooking water as seafood flavor replacement in food formulations enables the development of seafood substitutes, thus decreasing the pressure on seafood crops and aquaculture-associated issues, thereby leading to more sustainable livestock. Moreover, the use of otherwise wasted cooking water as an odorant allows an extra step toward zero waste, thus potentially increasing the circularity and sustainability of the process.
The presented results are the first step toward the use of algae to mimic seafood odor, but it is clear that more research is needed to relate the algae volatile profile to seafood odor, and the use of a trained panel is advised.
Data availability statement
The raw data supporting the conclusions of this article will be made available by the authors, without undue reservation.
Author contributions
CM: conceptualization, formal analysis, investigation, software, methodology, visualization, data processing, and writing—original draft. PF-S: conceptualization, investigation, methodology, validation, supervision, reviewing, editing, and formal analysis. JT: validation, reviewing, editing, and formal analysis. CR: conceptualization, methodology, supervision, reviewing, editing, and formal analysis. All authors contributed to the article and approved the submitted version.
Funding
This study was supported by the Portuguese Foundation for Science and Technology (FCT) under the scope of the strategic funding of UIDB/04469/2020 unit and by LABBELS—Associate Laboratory in Biotechnology, Bioengineering and Microelectromechanical Systems, LA/P/0029/2020. This study was also funded by the ESIF—European Structural and Investment Funds under the scope of the projects ALGAVALOR—Microalgae integrated production and valorization of its various applications (ref. POCI-01-0247-FEDER-035234; LISBOA-01-0247-FEDER-035234; ALG-01-0247-FEDER-035234; through Portugal 2020), and BioEcoNorte project (ref. NORTE-01-0145-FEDER-000070, through Portugal 2020 and Norte2020). CM is recipient of a Ph.D. scholarship supported by FCT (Ref. 2021.05734.BD).
Acknowledgments
The authors would like to thank Joana Dias and Bianca Marques for their assistance in organizing the sensory evaluations and to Zlatina Genisheva for providing support in data analysis.
Conflict of interest
The authors declare that the research was conducted in the absence of any commercial or financial relationships that could be construed as a potential conflict of interest.
Publisher's note
All claims expressed in this article are solely those of the authors and do not necessarily represent those of their affiliated organizations, or those of the publisher, the editors and the reviewers. Any product that may be evaluated in this article, or claim that may be made by its manufacturer, is not guaranteed or endorsed by the publisher.
Supplementary material
The Supplementary Material for this article can be found online at: https://www.frontiersin.org/articles/10.3389/fsufs.2022.1011020/full#supplementary-material
References
Akakabe, Y., and Kajiwara, T. (2008). Bioactive volatile compounds from marine algae: feeding attractants. J. Appl. Phycol. 20, 661–664. doi: 10.1007/s10811-007-9309-x
Bleakley, S., and Hayes, M. (2017). Algal proteins: extraction, application, and challenges concerning production. Foods 6, 33. doi: 10.3390/foods6050033
Coleman, B., Van Poucke, C., Dewitte, B., Ruttens, A., Moerdijk-Poortvliet, T., Latsos, C., et al. (2022). Potential of microalgae as flavoring agents for plant-based seafood alternatives. Future Foods 5, 100139. doi: 10.1016/j.fufo.2022.100139
Dopelt, K., Radon, P., and Davidovitch, N. (2019). Environmental effects of the livestock industry: the relationship between knowledge, attitudes, and behavior among students in Israel. Int. J. Environ. Res. Public Health 16, 81359. doi: 10.3390/ijerph16081359
FAO. (2018). The State of World Fisheries and Aquaculture 2018 - Meeting the Sustainable Development Goals. Rome: FAO.
Ferreira-Santos, P., Nunes, R., De Biasio, F., Spigno, G., Gorgoglione, D., Teixeira, J. A., et al. (2020). Influence of thermal and electrical effects of ohmic heating on C-phycocyanin properties and biocompounds recovery from Spirulina platensis. LWT 128, 109491. doi: 10.1016/j.lwt.2020.109491
Fiorentini, M., Kinchla, A. J., and Nolden, A. A. (2020). Role of sensory evaluation in consumer acceptance of plant-based meat analogs and meat extenders: a scoping review. Foods. 9, 1334. doi: 10.3390/foods9091334
Fu, J., Sun, C., Chang, Y., Li, S., Zhang, Y., and Fang, Y. (2022). Structure analysis and quality evaluation of plant-based meat analogs. J. Text. Stud. 2022, 12705. doi: 10.1111/jtxs.12705
Garicano Vilar, E., O'Sullivan, M. G., Kerry, J. P., and Kilcawley, K. N. (2020). Volatile compounds of six species of edible seaweed: a review. Algal Res. 45, 101740. doi: 10.1016/j.algal.2019.101740
Geada, P., Moreira, C., Silva, M., Nunes, R., Madureira, L., Rocha, C. M. R., et al. (2021). Algal proteins: production strategies and nutritional and functional properties. Bioresour. Technol. 332, 125125. doi: 10.1016/j.biortech.2021.125125
Giri, A., Osako, K., Okamoto, A., and Ohshima, T. (2010). Olfactometric characterization of aroma active compounds in fermented fish paste in comparison with fish sauce, fermented soy paste and sauce products. Food Res. Int. 43, 1027–1040. doi: 10.1016/j.foodres.2010.01.012
Granato, D., Putnik, P., Kovačević, D. B., Santos, J. S., Calado, V., Rocha, R. S., et al. (2018). Trends in chemometrics: food authentication, microbiology, and effects of processing. Comprehens. Rev. Food Sci. Food Saf. 17, 663–677. doi: 10.1111/1541-4337.12341
Gu, S., Wang, X., Tao, N., and Wu, N. (2013). Characterization of volatile compounds in different edible parts of steamed Chinese mitten crab (Eriocheir sinensis). Food Res. Int. 54, 81–92. doi: 10.1016/j.foodres.2013.05.018
Iannotti, L. L., Blackmore, I., Cohn, R., Chen, F., Gyimah, E. A., Chapnick, M., et al. (2021). Aquatic animal foods for nutrition security and child health. Food Nutr. Bullet. 43, 127–147. doi: 10.1177/03795721211061924
Iranmanesh, M., Ezzatpanah, H., Akbari-Adergani, B., and Karimi Torshizi, M. A. (2018). SPME/GC-MS characterization of volatile compounds of Iranian traditional dried Kashk. Int. J. Food Properties 21, 1067–1079. doi: 10.1080/10942912.2018.1466323
Isleten Hosoglu, M. (2018). Aroma characterization of five microalgae species using solid-phase microextraction and gas chromatography–mass spectrometry/olfactometry. Food Chem. 240, 1210–1218. doi: 10.1016/j.foodchem.2017.08.052
Koyande, A. K., Chew, K. W., Rambabu, K., Tao, Y., Chu, D. T., and Show, P. L. (2019). Microalgae: a potential alternative to health supplementation for humans. Food Sci. Hum. Wellness 8, 16–24. doi: 10.1016/j.fshw.2019.03.001
Lafarga, T. (2019). Effect of microalgal biomass incorporation into foods: nutritional and sensorial attributes of the end products. Algal Res. 41, 101566. doi: 10.1016/j.algal.2019.101566
Lafarga, T. (2020). Cultured microalgae and compounds derived thereof for food applications: strain selection and cultivation, drying, and processing strategies. Food Rev. Int. 36, 559–583. doi: 10.1080/87559129.2019.1655572
Los Ricardo, P., Simões, D. R. S., Benvenutti, L., Zielinski, A. A. F., Alberti, A., and Nogueira, A. (2021). Combining chemical analysis, sensory profile, CATA, preference mapping and chemometrics to establish the consumer quality standard of Camembert-type cheeses. Int. J. Dairy Technol. 74, 371–382. doi: 10.1111/1471-0307.12753
Mall, V., and Schieberle, P. (2017). Evaluation of key aroma compounds in processed prawns (whiteleg shrimp) by quantitation and aroma recombination experiments. J. Agri. Food Chem. 65, 2776–2783. doi: 10.1021/acs.jafc.7b00636
Meilgaard, M. C., Civille, G. V., and Carr, B. T. (2016). Sensory Evaluation Techniques. Vol 1, 5th Edn. CRC Press. doi: 10.1111/j.1471-0307.2007.00330.x
Moran, L., Bou, G., Aldai, N., Ciardi, M., Morillas-España, A., Sánchez-Zurano, A., et al. (2022). Characterisation of the volatile profile of microalgae and cyanobacteria using solid-phase microextraction followed by gas chromatography coupled to mass spectrometry. Sci. Rep. 12, 1–14. doi: 10.1038/s41598-022-07677-4
Pina, A. L., Costa, A. R., Lage-Yusty, M. A., and López-Hernández, J. (2014). An evaluation of edible red seaweed (Chondrus crispus) components and their modification during the cooking process. LWT Food Sci. Technol. 56, 175–180. doi: 10.1016/j.lwt.2013.08.006
Sampantamit, T., Ho, L., Lachat, C., Sutummawong, N., Sorgeloos, P., and Goethals, P. (2020). Aquaculture production and its environmental sustainability in thailand: challenges and potential solutions. Sustainability 12, 2010. doi: 10.3390/su12052010
Sánchez-García, F., Mirzayeva, A., Roldán, A., Castro, R., Palacios, V., García-Barroso, C., et al. (2019). Evolution of volatile compounds and sensory characteristics of edible green seaweed (Ulva rigida) during storage at different temperatures. J. Sci. Food Agri. 99, 5475–5482. doi: 10.1002/jsfa.9808
Silva, E. de S., Dos Santos Junior, H. B., Guedes, T. J. F. L., Sandes, R. D. D., Rajan, M., Leite Neta, M. T. S., et al. (2022). Comparative analysis of fresh and processed mango (Mangifera indica L, cv. “Maria”) pulps: influence of processing on the volatiles, bioactive compounds and antioxidant activity. Food Sci. Technol. 42, 1–10. doi: 10.1590/fst.54020
Starowicz, M., and Zieliński, H. (2019). How maillard reaction influences sensorial properties (color, flavor and texture) of food products? Food Rev. Int. 35, 707–725. doi: 10.1080/87559129.2019.1600538
Van Durme, J., Goiris, K., De Winne, A., De Cooman, L., and Muylaert, K. (2013). Evaluation of the volatile composition and sensory properties of five species of microalgae. J. Agri. Food Chem. 61, 10881–10890. doi: 10.1021/jf403112k
van Gemert, L. J. (2011). Odour Thresholds : Compilations of Odour Threshold Values in Air, Water and Other Media. Utrecht: Oliemans; Punter & Partners B.V.
van Vliet, S., Kronberg, S. L., and Provenza, F. D. (2020). Plant-based meats, human health, and climate change. Front. Sustain. Food Syst. 4, 128. doi: 10.3389/fsufs.2020.00128
Varlet, V., and Fernandez, X. (2010). Review. Sulfur-containing volatile compounds in seafood: occurrence, odorant properties and mechanisms of formation. Food Sci. Technol. Int. 16, 463–503. doi: 10.1177/1082013210379688
Willett, W., Rockström, J., Brent, L., Springmann, M., Lang, T., Vermeulen, S., et al. (2019). Food in the anthropocene: the EAT–Lancet Commission on healthy diets from sustainable food systems. Lancet 393, 447–492. doi: 10.1016/S0140-6736(18)31788-4
Keywords: algae, GC-MS, odorants, solid-phase microextraction, volatile compounds, seafood processing, sensory evaluation, sustainability
Citation: Moreira C, Ferreira-Santos P, Teixeira JA and Rocha CMR (2022) Active aroma compounds assessment of processed and non-processed micro- and macroalgae by solid-phase microextraction and gas chromatography/mass spectrometry targeting seafood analogs. Front. Sustain. Food Syst. 6:1011020. doi: 10.3389/fsufs.2022.1011020
Received: 03 August 2022; Accepted: 15 September 2022;
Published: 26 October 2022.
Edited by:
Guadalupe Virginia Nevárez-Moorillón, Autonomous University of Chihuahua, MexicoReviewed by:
Raul Avila-Sosa, Meritorious Autonomous University of Puebla, MexicoAdriano Gomes Cruz, Adriano Gomes da Cruz, Brazil
Copyright © 2022 Moreira, Ferreira-Santos, Teixeira and Rocha. This is an open-access article distributed under the terms of the Creative Commons Attribution License (CC BY). The use, distribution or reproduction in other forums is permitted, provided the original author(s) and the copyright owner(s) are credited and that the original publication in this journal is cited, in accordance with accepted academic practice. No use, distribution or reproduction is permitted which does not comply with these terms.
*Correspondence: Cristina M. R. Rocha, Y21yb2NoYUBjZWIudW1pbmhvLnB0