- 1Department of Horticulture, Washington State University, Northwestern Washington Research and Extension Center, Mount Vernon, WA, United States
- 2Department of Entomology and Nematology, University of Florida, Gainesville, FL, United States
- 3Department of Entomology, Michigan State University, East Lansing, MI, United States
- 4IMPACT Center, School of Economic Sciences, Washington State University, Pullman, WA, United States
- 5Department of Mathematics and Statistics, Washington State University, Pullman, WA, United States
- 6Department of Horticulture, Oregon State University, Corvallis, OR, United States
Highbush blueberry (Vaccinium spp.) is a globally important fruit crop that depends on insect-mediated pollination to produce quality fruit and commercially viable yields. Pollination success in blueberry is complex and impacted by multiple interacting factors including flower density, bee diversity and abundance, and weather conditions. Other factors, including floral traits, bee traits, and economics also contribute to pollination success at the farm level but are less well understood. As blueberry production continues to expand globally, decision-aid technologies are needed to optimize and enhance the sustainability of pollination strategies. The objective of this review is to highlight our current knowledge about blueberry pollination, where current research efforts are focused, and where future research should be directed to successfully implement a comprehensive blueberry pollination decision-making framework for modern production systems. Important knowledge gaps remain, including how to integrate wild and managed pollinators to optimize pollination, and how to provide predictable and stable crop pollination across variable environmental conditions. In addition, continued advances in pesticide stewardship are required to optimize pollinator health and crop outcomes. Integration of on- and off-farm data, statistical models, and software tools could distill complex scientific information into decision-aid systems that support sustainable, evidence-based pollination decisions at the farm level. Utility of these tools will require multi-disciplinary research and strategic deployment through effective extension and information-sharing networks of growers, beekeepers, and extension/crop advisors.
Introduction
Highbush blueberry is a domesticated fruit crop from eastern North America that has gained cosmopolitan status in recent years due to its attractive fruit with human health benefits (Eck and Childers, 1966; Retamales and Hancock, 2018; Kalt et al., 2020). Global blueberry production has more than doubled since 2005 and expanded to all continents except Antarctica (Brazelton et al., 2021). Production systems of northern highbush blueberry (Vaccinium corymbosum L.) and southern highbush blueberry (complex hybrids of V. corymbosum and low-chill Vaccinium species) have rapidly modernized for efficient production with new cultivars and research-driven recommendations for irrigation, fertilization, harvesting, postharvest management, pruning and training, and pest and disease management. Parallel advances in pollination management, however, are lagging. There is a need for investment in research that can support evidence-based decision support systems to optimize sustainable pollination and ensure economical production of this globally important and expanding crop.
Insect-mediated pollination is needed to produce blueberries of marketable size for commercial production (MacKenzie, 1997; Retamales and Hancock, 2018; Martin et al., 2021). Blueberry fields frequently suffer from pollen limitation across different production regions, indicating optimal or maximum levels of insect-mediated pollination are not always achieved (Button and Elle, 2014; De Groot et al., 2015; Nicholson and Ricketts, 2019; Reilly et al., 2020). Wild pollinators alone are not sufficient in large and intensive crop systems where they are less abundant (Isaacs and Kirk, 2010), causing many growers to rely on renting managed western honey bee (Apis mellifera L.) and bumble bee (Bombus spp.) colonies for pollination services (Arrington and DeVetter, 2018a; Bobiwash et al., 2018; Cavigliasso et al., 2020; Mallinger et al., 2021). The number of honey bee colonies has globally increased over the last seven decades (Osterman et al., 2021a) and the supply of this pollinator to blueberry farms in North America has not experienced the decrease observed in other crops (Burgett et al., 2004, 2010; Aizen and Harder, 2009; Breeze et al., 2014). However, reliance only on honey bees may not be a viable long-term strategy for sustainable crop pollination in some regions given the increased expansion of pollinator-dependent crops relative to the supply of honey bees (Aizen and Harder, 2009; Breeze et al., 2014) and the potential for honey bee foraging to be inhibited by cool weather. Reported levels of honey bee colony mortality have been high in North American (Osterman et al., 2021a), and the health of both wild and managed bees is affected by individual or synergistic effects of poor nutrition, pesticide exposure, abiotic stressors, and pests and diseases that threaten the stability of pollination (Potts et al., 2010; Goulson et al., 2015), requiring a focus on overall pollinator health to ensure effective crop pollination.
Given these factors affecting honey bee and wild bee supply and health, blueberry pollination strategies should encompass the Integrated Crop Pollination (ICP) concept to move toward more resilient pollination systems and success for growers. Introduced by Isaacs et al. (2017), ICP is “the use of managed pollinator species in combination with farm management practices that support, augment, and protect pollinator populations to provide reliable and economical pollination of crops.” Implementation of ICP for blueberries will require understanding and addressing knowledge gaps within the fundamental components of ICP such as the use of common and alternative managed pollinator species, wild pollinators, habitat enhancement, pesticide stewardship, and horticultural practices. Only by understanding these components can advances be made in evidence-based decision support systems to optimize pollination and yield. The objective of this review is to highlight what is known about blueberry pollination within the context of ICP, where current efforts are focused, and where future research should be directed to support sustainable pollination strategies for profitable modern blueberry production systems (Figure 1). Floral traits of different cultivars, environmental factors that influence pollination, economics, and the use of decision-aid technologies will also be considered as components to blend within the ICP framework with the aim of better understanding and improving pollination for this globally expanding crop.
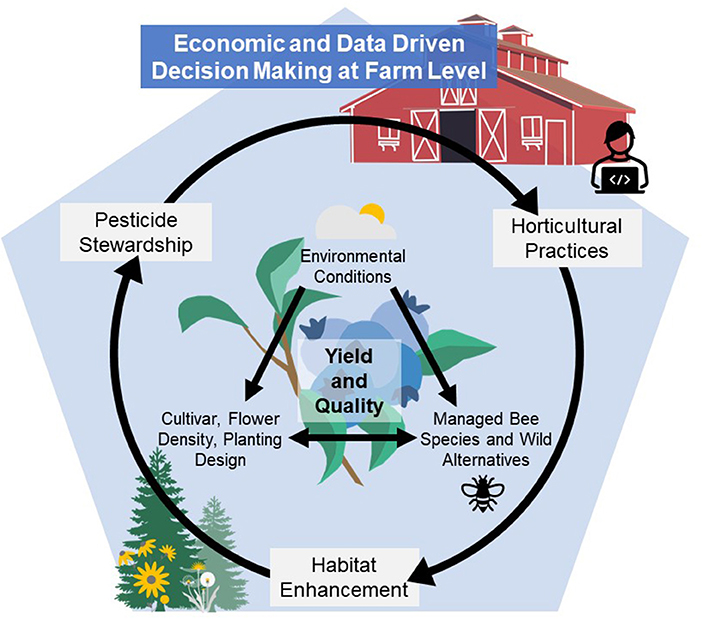
Figure 1. Highbush blueberry yield and quality depends on pollination success, which is affected by components within this blended Integrated Crop Pollination (ICP) framework that includes use of common and alternative managed pollinator species, wild pollinators, habitat enhancement, pesticide stewardship, and horticultural practices. Floral traits, environmental conditions, on-farm economics, and the potential use of decision-aid technologies all have the potential for unique impacts on blueberry pollination, thus requiring a deeper understanding of how they impact pollination success and contribute to economic and effective evidenced-based decisions at the farm level.
Pollination requirements
Highbush blueberry has the potential to set nearly 100% of its flowers into fruits when resources are not limiting (Ehlenfeldt, 2001; Kumarihami et al., 2021), but this may not be achieved if pollination is inadequate or disrupted. Cultivated highbush blueberries are highly dependent upon successful pollination, with individual flowers requiring deposition of about 30 to 100 pollen tetrads to maximize fruit set and fruit mass, and to minimize ripening time (Dogterom et al., 2000; Sun et al., 2021). While some parthenocarpy has been observed in some highbush blueberry genotypes, it yields generally small fruits and is insufficient at producing marketable yields for any cultivar (Harrison et al., 1993; MacKenzie, 1997; Kazokas, 2003; Ehlenfeldt and Vorsa, 2007; Ehlenfeldt, 2012). Insect pollinators are necessary due to low auto-fertility (sensu Rodger et al., 2021). Blueberry flowers excluded from insect pollinators have reduced fruit set, and any resulting berries are smaller than insect-pollinated flowers (Tuell and Isaacs, 2010a; Gibbs et al., 2016; Campbell et al., 2018). Low levels of auto-fertility in blueberry may be due to various aspects of floral development and morphology, including poricidal anthers that require sonication by insects to adequately release pollen (Cardinal et al., 2018; Cooley and Vallejo-Marín, 2021), and upside-down, urn-shaped flowers with a style sometimes shorter than the corolla that limits pollen deposition on the stigma either by autonomous self-pollination or by wind pollination (Sampson et al., 2013).
Modern highbush blueberry cultivars have a relatively high degree of self-fertility, i.e., a high ability for flowers to set fruits when pollinated by self-pollen, including pollen derived from the same flower, from flowers of the same plant, or from plants of the same cultivar (Dogterom et al., 2000; Ehlenfeldt, 2001; Taber and Olmstead, 2016; Kendall et al., 2020). That is why many fields of northern highbush blueberry are established as single-cultivar blocks. Southern highbush blueberry, in contrast, is sometimes planted in mixed-cultivar configurations with overlapping bloom periods to promote cross pollination (Mallinger and Phillips, 2022). Contrary to what many papers state due to a semantic confusion, the whole Vaccinium genus is self-compatible, meaning self-pollen can germinate in pollen tubes that can grow through the style and fertilize ovules to the same degree as cross-pollen (Garvey and Lyrene, 1987; Krebs and Hancock, 1988; Czesnik et al., 1989; Hokanson, 1995; Hokanson and Hancock, 2000; Schott, 2000; Brevis et al., 2006; Miller et al., 2011; Leposavić et al., 2021). But on the other hand, the Vaccinium genus is partially self-sterile (terminology proposed by Gibbs, 2014) as it is affected by an early-acting inbreeding depression (EID) whereby embryos resulting from self-pollination may be partly aborted due to genetic load (Krebs and Hancock, 1990, 1991; Guillaume and Jacquemart, 1999; Rasp et al., 2004; Nuortila et al., 2006).
Consequently, as it is known from the early 20th century (Coville, 1921), many highbush blueberry genotypes show improved fertility with cross-pollination compared to self-pollination. For northern highbush, 70% of evaluated genotypes tested in the literature showed improved fruit set with hand cross-pollination, at least under certain conditions, and 86% showed improved fruit size (Supplementary Table 1). Likewise, southern highbush genotypes showed 63 and 65% improvement in fruit set and fruit size with hand cross-pollination, respectively. The magnitude of fertility gains may depend on the degree of inbreeding for a given genotype (Hellman and Moore, 1983; Krebs and Hancock, 1988, 1990) and on the pollen donor (White and Clark, 1939; Cremins, 1952; Gupton, 1984, 1997; Czesnik et al., 1989; Gupton and Spiers, 1994; Huang et al., 1997; Ehlenfeldt, 2003; Martin K. et al., 2019; Nagasaka et al., 2022). Cross-pollination can also result in a decrease of ripening time (Supplementary Table 1) and an increase of anthocyanin content (Doi et al., 2021). Insect pollinators vary in their efficiency in cross pollination: high pollen loads on their bodies combined with foraging behavior across rows and cultivars can facilitate greater cross pollination by bumble bees (Miñarro and García, 2021), although in some cases honey bees have been observed to alternate between cultivars more than bumble bees (Estravis-Barcala et al., 2021b). Northern and southern highbush blueberry cultivars have similar levels of heterozygosity (Brevis et al., 2008) and are consequently similarly prone to EID. Domesticated blueberry cultivars have become increasingly inbred over time (Hancock and Siefker, 1982; Ehlenfeldt, 1994), which may increase their susceptibility to EID, indicating that the benefits of cross pollination will likely increase as new cultivars continue to be developed. Understanding the breeding history of modern cultivars and their levels of inbreeding will help to predict how well each cultivar will perform in single-cultivar plantings and which cultivars should be co-planted for effective cross-pollination.
Floral visitation
Floral visitation rates, driven by the diversity and abundance of the pollinator community and by the attractiveness of the crop flowers, are a major determinant of successful blueberry pollination. While considerable attention has been given to understanding the factors that influence pollinator abundance and diversity within blueberry fields (Isaacs and Kirk, 2010; Tuell and Isaacs, 2010a; Benjamin et al., 2014; Gibbs et al., 2016; Vieli et al., 2016; Graham et al., 2021a), neither diversity nor abundance of pollinators can positively influence pollination without successful recruitment (Gaines-Day and Gratton, 2016; Geslin et al., 2017a; Danka et al., 2019; Mallinger et al., 2021). Enhancing pollinator recruitment to crop flowers not only improves pollination but may also reduce the density of managed pollinators required, thereby reducing input costs.
Identifying and manipulating floral traits that maximize pollinator recruitment is key to optimizing pollination and tailoring pollination strategies to different blueberry cultivars. This is a promising avenue for crop breeding and is currently being explored in blueberry and other cropping systems (Ritzinger and Lyrene, 1999; Palmer et al., 2009; Bailes et al., 2015; Prasifka et al., 2018). Variation in floral rewards, including the quantity and quality of nectar and pollen, and in flower morphology and visual and olfactory cues can all influence pollinator attraction and subsequent pollination success (Prasifka et al., 2018; Cortés-Rivas et al., 2022 and references therein; Sampson et al., 2013; Vaudo et al., 2020). For example, honey bees showed greater visitation rates to blueberry flowers with wider floral tubes, specifically wider corolla opening diameters (Courcelles et al., 2013). Flower morphology can also interact with pollinator foraging behaviors: rates of nectar robbing or side working by carpenter bees (Xylocopa spp.) differed among blueberry cultivars and was associated with differences in flower morphology (Sampson et al., 2004; Courcelles et al., 2013; Tucker et al., 2019). Bees are more likely to rob nectar from long and narrow blueberry flowers and more likely to make legitimate visits to shorter and wider blueberry flowers (Courcelles et al., 2013). In another example, individual flower size, corolla width, anther and pistil lengths, stigma-anther separation, and stigma protrusion across Vaccinium species was positively correlated with percent fruit set and/or berry weight following visits by the blueberry bee, Osmia ribifloris Cockerell (Sampson et al., 2013). Blueberry flowers can also differ in the degree to which petals are fused, but how these morphological traits affect pollinator recruitment is unknown (Arrington and DeVetter, 2018b).
Quantity and quality of blueberry rewards can also drive floral visitation rates. Honey bees primarily collect nectar from blueberry flowers and may respond to differences in nectar volume and sugar concentration found across cultivars (Jablonski et al., 1985; Bozek, 2021; Estravis-Barcala et al., 2021b). Bumble bees more frequently collect blueberry pollen than honey bees (Bobiwash et al., 2018; Miñarro and García, 2021) and may be affected by pollen traits including pollen chemistry, which has been found to differ across wild and cultivated blueberries and to affect bumble bee foraging preferences in other systems (Vaudo et al., 2016; Egan et al., 2018). For example, higher nectar volume increased honey and bumble bee visits to onion (Allium cepa L.) and zucchini (Cucurbita pepo L.) cultivars, while higher quantities of floral volatiles increased wild solitary bee visits to strawberry (Fragaria x ananassa Duch.) cultivars (Prasifka et al., 2018). Floral volatiles can indicate the age and pollination status of a flower, in turn signaling reward quantity and quality (Stout and Goulson, 2001). In blueberry, pollinators showed greater attraction to unvisited flowers that emitted greater amounts of floral volatiles (Rodriguez-Saona et al., 2011).
A promising new approach to enhance recruitment is feeding honey bee and bumble bee colonies with caffeine- or arginine-treated syrup flavored with crop flower scents just before bloom onset (Farina et al., 2020; Arnold et al., 2021; Estravis-Barcala et al., 2021a), training them to crop-specific rewards. Blueberry flower scents have been identified (Rodriguez-Saona et al., 2011; Forney et al., 2012; Huber, 2016) and could be used to aid bee recruitment. Brood, queen mandibular, and Nasonov pheromones are produced by honey bees for communication and manufactured versions of these pheromones are currently sold to growers to enhance honey bee recruitment (Free and Williams, 1970; Pankiw et al., 1994, 1998). Commercial bee attractants are also available on the market. The utility of these products when applied to blooming crops is questionable. Indeed, most studies have shown application of pheromones and attractants to be unreliable at increasing pollination and yield outcomes across various crops (Schultheis et al., 1994; Connell, 2000; Ellis and Delaplane, 2009; Williamson et al., 2018).
There are many opportunities to breed or manage the crop for more attractive flowers including accessible floral tubes to increase visitation rates and reduce nectar robbing, increased floral rewards (nectar and pollen) to enhance recruitment, and increased floral volatile emissions to signal attractive and rewarding flowers. These floral traits can be determined by genetic factors influenced by crop domestication (Egan et al., 2018) but recruitment can also be affected by environmental factors including temperature, water and nutrient availability, and interactions with herbivores and soil microorganisms (Lyrene, 1994; Cardel and Koptur, 2010; Halpern et al., 2010; Bruinsma et al., 2014; Brody et al., 2019; Rering et al., 2020). More studies are needed on the interaction of floral traits, rewards, and management to optimize floral visitation.
Horticultural factors
Horticultural factors such as field age, planting designs that include pollinizers, use of mulch, effective weed management, and conventional field management influence the probability of achieving higher yields (Retamales et al., 2015). Like most crops, soil conditions including plant nutrition and water availability directly affect blueberry productivity and need to be within an optimum range (Cameron et al., 1988; Bryla and Strik, 2015). Furthermore, soil conditions and pest and pathogen management may adjust allocation of resources to fruit and seed development independent of pollination so that pollination benefits may not be realized if soil conditions and pest and pathogen management are sub-optimal (Tamburini et al., 2019). Not only do these horticultural factors directly determine blueberry yield, but they also interact with pollination in complex ways that complicate our ability to quantify the benefits of insect-mediated pollination (Bos et al., 2007; Seppelt et al., 2011). For example, lack of pest control reduced yield of lowbush blueberry (V. angustifolium Ait.) independent of pollination and the highest yields were achieved when full, manual pollination was combined with the full input of recommended pesticides (Melathopoulos et al., 2014). Studies that attempt to disentangle the interaction between pollination and soil and pest and pathogen management in blueberry are lacking but needed given what has been shown in other cropping systems (Tamburini et al., 2019). Accounting for these other factors that contribute to yield will lend greater insight into the value of insect pollinators and build toward a more effective application of ICP.
Protected culture such as photo-selective nets and polytunnels can provide protection against environmental hazards such as hail, extremes in solar radiation, and vertebrate and arthropod pests (Wittwer and Castilla, 1995). Protected culture manipulates light quantity and quality, temperature, humidity, and airflow (Wittwer and Castilla, 1995; Shahak et al., 2008; Lobos et al., 2013) and can increase yields and advance harvest timing compared to open-field production (Retamal-Salgado et al., 2015). However, these protective methods can negatively impact pollination and pollinator health (Kendall et al., 2021). Modifications in light intensity and wavelength can negatively impact the ability of some pollinating insects to forage and return to their nests given that they rely on polarized light from the sun for navigation (Evangelista et al., 2014). Hall et al. (2020) showed honey bee pollination deficits in the middle of 100-m long polytunnels can reduce blueberry fruit weight and yield compared to the edges. High temperatures in polytunnel middles can have lethal or sublethal effects on honey bees and greater humidity may have an additive effect by reducing honey bee capability to thermoregulate (Free and Spencer-Booth, 1962). Bumble bees, leafcutter bees (Megachile spp.), and mason bees (Osmia spp.) are reported to forage more efficiently in polytunnels compared to honey bees (Goodwin, 2012; as reviewed by Kendall et al., 2021). Because the use of protected culture has increased as the blueberry industry expands into new production regions and tries to capitalize on lucrative market windows, it is important to have a better understanding of how protected culture affects pollinators, so that these methods can be modified to optimize both crop protection and pollination.
Plant growth regulators are also sometimes used to overcome poor pollination. Gibberellic acid (GA3) has received the most attention due to its role in increasing fruit set after a freeze event or physical injury (NeSmith et al., 1995). Controlled-environment studies have shown GA3 increases fruit set in non-pollinated rabbiteye blueberry (V. virgatum Ait.) flowers with a trade off in delayed fruit development and weight (Williamson et al., 1996). In northern highbush blueberry, effects of GA3 are subtle and inconsistent; its use may not always result in a yield response, and response to GA3 depends on cultivar, application timing, pollination, and weather conditions (Hanson and Kelsey, 2019).
Managed honey bees
Honey bees have long been used for highbush blueberry pollination because their large population sizes and ease of transport facilitate the high densities needed to meet pollination requirements (Tuell and Isaacs, 2010a; Estravis-Barcala et al., 2021b; Mallinger et al., 2021). Stocking recommendations and practices are highly variable: earlier guides recommended stocking rates ranging from 1.2 to 25 honey bee colonies per hectare (Delaplane et al., 2000; Rucker et al., 2012), while current practices can range from 0 to 40 colonies per hectare (Gibbs et al., 2016; Mallinger et al., 2021). Colony stocking rate is poorly correlated with honey bee visitation and thus with blueberry yield, although honey bee visitation rates have been positively correlated with yield (Supplementary Table 2). Honey bees can become diluted in the landscape when there are natural habitats or other blooming entomophilous crops, and they can also be attracted to some cultivars more than others, resulting in poor correlation between stocking rate and managed bee density in the field (Díaz et al., 2013; Gaines-Day and Gratton, 2016; Quinet et al., 2016; Estravis-Barcala et al., 2021b; Osterman et al., 2021b). Furthermore, there may be interactive effects between honey bees and wild bee densities that could affect pollination outcomes (Eaton and Nams, 2012).
Colony size and effective forager population can also vary considerably, ranging from 5,000 to 65,000 workers (Farrar, 1937; Schmickl and Crailsheim, 2007; Chabert et al., 2021). Larger honey bee colonies have higher foraging activity, increasing pollination within the same colony stocking rate (Geslin et al., 2017a; Cavigliasso et al., 2021). Farrar's (1937) study suggests that large colonies allocate an even higher proportion of their workers to the task of foraging, implying that large colonies would have a higher pollination performance than small colonies for the same number of workers provided in total. For some crops such as almond [Prunus amygdalus L., syn. P. dulcis (Mill.) DA Webb] or oilseed rape (Brassica napus L.) in hybrid seed production, growers request honey bee colonies with a minimum population size in their pollination contract, with increasing fees when colonies exceed the minimum request (Ovinge and Hoover, 2018; Goodrich, 2019; Goodrich and Goodhue, 2020). However, with the aim of promoting sustainable beekeeping for crop pollination, instead of absolutely meeting a standardized colony size that can be harmful for colonies (Durant, 2019), beekeepers could offset a low colony size by providing more colonies as long as the colonies are healthy with a laying queen alongside brood and enough empty combs (Sagili and Burgett, 2011). Combined, these functional differences based on colony size and forager population further complicate the utility of stocking density recommendations on a per colony basis. Assessing honey bee colony size can be done using the cluster size method (Nasr et al., 1990; Chabert et al., 2021) implemented with a beekeeper and/or an intermediary such as a bee broker (Goodrich, 2017), with thermographic imaging (Shaw et al., 2011; López-Fernández et al., 2018), or by assessing activity at hive entrances (Rodet and Henry, 2014; Ovinge and Hoover, 2018; Grant et al., 2021). When assessing honey bee colony sizes, it should be noted that the relationship between colony size and cluster size is exponential and not linear (Chabert et al., 2021).
In addition to colony stocking rates, Garibaldi et al. (2020) suggested identifying target pollinator densities to reach per flower in crops so that pollination is not limiting. These target densities can be assessed either empirically (Reilly et al., 2020; Chabert et al., 2022), or with a modular approach (Ne'eman et al., 2010; Garibaldi et al., 2020). With the modular approach, pistil receptivity lasts at least 4 days in highbush blueberry (Moore, 1964; Young and Sherman, 1978; Krebs and Hancock, 1990; Kirk and Isaacs, 2012), flowers require 5 to 15 bee visits to maximize fruit weight (Danka et al., 1993; Rogers et al., 2013; Kendall et al., 2020), and honey bees visit about 5 blueberry flowers per minute (Shaw et al., 1939; Estravis-Barcala et al., 2021b; Sun et al., 2021). Based on the assumption of 6 hours of daily foraging activity, one blueberry field would require about 0.07 to 0.2 honey bees per 100 flowers (i.e., 0.2 to 0.6 honey bee visits per flower per hour). Empirically, Reilly et al. (2020) found that “Bluecrop” fields would require 16.7–26.3 bees per 100 m row to maximize fruit weight. Pollinator density estimates should always be associated with a flower count in pollination empirical studies (Chabert et al., 2022), highlighting the importance of developing simple and fast flower monitoring approaches with new methods such as image analysis with deep learning (Farjon et al., 2020; Hicks et al., 2021). Flower monitoring approaches could also be used by growers and crop advisors to assess pollination and inform pollination management.
Current recommendations indicate hive placement should begin when blueberry flowers are between 5 and 25% bloom (Howell et al., 1972) because honey bees may disperse onto alternative resources and forage less in the crop when placed before the onset of bloom (Jay, 1986). Some also advocate for the sequential introduction of colonies to crops resulting in higher bee densities in the crop and more bee movement between cultivars (Mayer, 1994; Shafir, 2011 and references therein). Sequential introduction or colony rotation could be applied in blueberry, especially in fields with mixed cultivars benefitting from cross-pollination, but it is unclear if any increase in pollination would offset the increased labor and transport costs. Many authors recommend placing colonies as close as possible to the crop and distributed as evenly as possible along the field to ensure effective and even pollination (Jay, 1986; Cunningham et al., 2016; Cavigliasso et al., 2021; Li et al., 2022), though these practices result in increased labor and transport costs for beekeepers and increased risk of pesticide drift. More investigations are required for creating recommendations on hive placement and density, so that target bee densities for maximum pollination and yields are achieved.
Alternative managed bees
Supplementing honey bees with other bee species can result in higher crop pollination (Rogers et al., 2013, 2014; Mallinger et al., 2021). Several researchers have investigated alternative managed pollinators for blueberry pollination (Table 1; Supplementary Table 3). Among the 16 species investigated other than the honey bee, 14 can be used in open field production, including 10 that are already commercially available, and four which have potential but require further investigation.
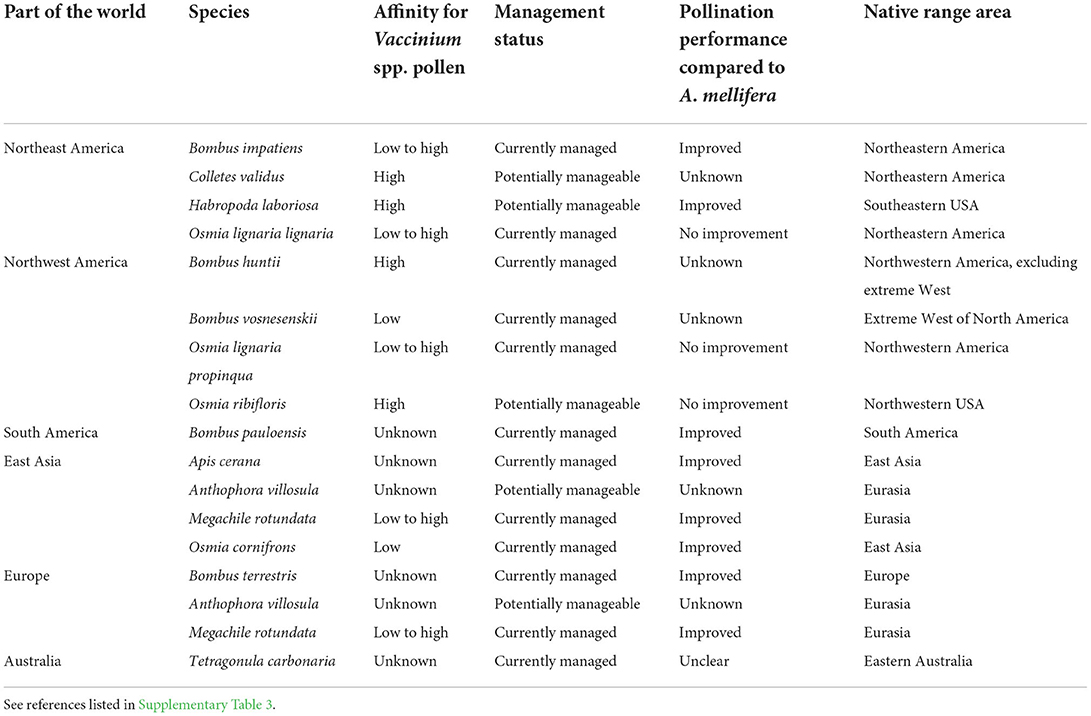
Table 1. Alternative managed pollinator species other than the western honey bee (Apis mellifera L.) for highbush blueberry (Vaccinium spp.) pollination.
Bumble bees are the most widely used alternative managed pollinators of blueberry due to their ability to sonicate (King and Buchmann, 2003; Cardinal et al., 2018), frequency of collecting blueberry pollen (Supplementary Table 3), ability to carry pollen on their bodies and deposit higher pollen loads on stigmas than honey bees (Dogterom, 1999; Rogers et al., 2013; Estravis-Barcala et al., 2021b; Sun et al., 2021), and ability to forage at lower air temperatures (Stubbs and Drummond, 2001; Tuell and Isaacs, 2010a). Usual stocking rates for bumble bee colonies range from 0.5 to 10 colonies per hectare (Tuell and Isaacs, 2010a; Estravis-Barcala et al., 2021b; Mallinger et al., 2021). While both bumble bees and the southeastern blueberry bee (Habropoda laboriosa Fabricius) can sonicate flowers (King and Buchmann, 2003; Cardinal et al., 2018), European leaf-cutting bees (M. rotundata Fabricius), orchard mason bees (O. lignaria Say), blueberry bees, and other bees are also able to collect pollen, deposit pollen on stigmas, and induce fertilization (Table 1; Supplementary Table 3). All the species also visit blueberry flowers for nectar (Stubbs et al., 1994; Dogterom, 1999; Javorek et al., 2002; Tuell and Isaacs, 2010a; Rogers et al., 2013; Estravis-Barcala et al., 2021b). Although nectar foragers are less efficient than pollen foragers for pollination (Javorek et al., 2002; Estravis-Barcala et al., 2021b), they can nevertheless carry pollen on their body (Dogterom and Winston, 1999; Hoffman et al., 2018; Estravis-Barcala et al., 2021b), contribute to blueberry pollination, and broaden the potential of alternative managed species that can augment blueberry pollination. While the nest design for managed bumble bee colonies has been optimized for some species to make them commercially available, recent studies have also identified the preferred nesting material, nest hole diameter and attractant sprays to increase mason bee nesting success (Eeraerts et al., 2022; Pinilla-Gallego et al., 2022). Further studies are required to optimize management and targeted application of solitary bees, especially ground nesting species (Cane, 1997; Mader et al., 2010; Leonard and Harmon-Threatt, 2019; Nelson et al., 2022), for blueberry pollination.
Precautions must be taken when considering honey bees and alternative managed bees outside of their native ranges, as they can become invasive and detrimental to native flora and/or entomofauna, or even for crop pollination (Goulson, 2003; Russo, 2016; Geslin et al., 2017b; Morales et al., 2017; Aizen et al., 2019, 2020; LeCroy et al., 2020; Russo et al., 2021). Managing bees within their native range can also be detrimental to wild entomofauna by competing for floral resources or nesting habitats, spreading pests and pathogens, eroding genetic diversity, and disrupting local adaptations by hybridization between native and commercial lines (Fürst et al., 2014; Lindström et al., 2016; Mallinger et al., 2017; Seabra et al., 2019; Bartomeus et al., 2020; Russo et al., 2021).
Wild pollinators and habitat enhancement
The contribution of wild bees and non-Apis insect pollinators depends on their pollination performance and abundance (Ne'eman et al., 2010). More specifically, this contribution can vary considerable from field to field, ranging from 12 to 82% (Isaacs and Kirk, 2010; see also Benjamin and Winfree, 2014; De Groot et al., 2015). A great diversity of wild bees has been found to visit blueberry flowers worldwide (De Groot et al., 2015; Martins et al., 2018; Graham et al., 2021a; Cortés-Rivas et al., 2022), and the pollination efficiency has been found to vary widely among bee species (Rogers et al., 2013, 2014; Sun et al., 2021; Cortés-Rivas et al., 2022) and blueberry cultivars (Cortés-Rivas et al., 2022). Differences in foraging behavior across pollinator taxa have been found in highbush blueberry cultivars as well (Courcelles et al., 2013; Estravis-Barcala et al., 2021b). Bumble bees and some solitary bees can sonicate and hence can release more pollen than honey bees and other insect pollinators who cannot sonicate (Buchmann, 1983; King and Buchmann, 2003; Cardinal et al., 2018; Cortés-Rivas et al., 2022). On the other hand, bumble bees and carpenter bees can sometimes steal nectar from blueberry flowers by using their mandibles to make a hole in the corolla and this foraging behavior reduces these species' pollination contribution (Sampson et al., 2004; Rogers et al., 2013), and can induce other bees to rob nectar (Dedej and Delaplane, 2004). Differences in pollination efficiency, foraging behavior, and abundance across different pollinator taxa can provide functional complementarity and stability for crop pollination when different taxa are present during the blueberry boom period (Blüthgen and Klein, 2011).
Although wild pollinators can contribute substantially to blueberry pollination, their abundance is often too low to fulfill commercial demands for pollination, especially in large fields (Isaacs and Kirk, 2010; Benjamin and Winfree, 2014; Gibbs et al., 2016). Variation in the abundance of wild bees visiting highbush blueberry flowers depends on many factors including production region (Gibbs et al., 2016), farm size (Isaacs and Kirk, 2010), farming practices (Nicholson et al., 2017; Mallinger et al., 2021), and the amount of semi-natural habitat (SNH) in the landscape around the farm (Gibbs et al., 2016; Nicholson et al., 2017). Increasing cover of intensive agriculture has a negative effect on wild bee visitation in blueberry fields (Benjamin et al., 2014; Nicholson et al., 2017), as agricultural intensification reduces species richness of pollinator assemblages and wild insect visitation (Klein et al., 2007; Garibaldi et al., 2011). In general, the amount of SNH enhances floral visitation by wild bees (Gibbs et al., 2016; Nicholson et al., 2017; Mallinger et al., 2021), but the landscape scale by which wild bees benefit from SNH depends on their body size. Smaller bees respond over shorter distances to local field conditions than those with larger body sizes that can utilize resources across the larger landscape-scale (Benjamin et al., 2014). Conservation of SNH and implementing habitat enhancements near blueberry fields is therefore advised to provide nutritional and nesting resources for wild bees and boosting their local populations.
It has been recommended that agricultural landscapes should have at least 20% SNH to support agricultural productivity by improving pollination and other ecosystem services (e.g., as well as enhancing soil nutrition, pest control, and/or preventing floods and regulating climate; Garibaldi et al., 2021). Beyond conservation of SNH, pollinators and pollination also benefit from increasing landscape diversity (Martin E. A. et al., 2019), which has been found to support wild bee communities and their corresponding pollination services in blueberry. Reducing blueberry field size (Isaacs and Kirk, 2010), providing mass-flowering crops with complementary phenologies (Martins et al., 2018; Eeraerts, 2022), and reducing agricultural management intensity (e.g., organic management; Nicholson et al., 2017; Mallinger et al., 2021) are additional ways to promote landscape diversity and pollination by wild bees. Honey bee health will also benefit from the alternative forage provided by SNH and habitat enhancement efforts (Couvillon et al., 2014; Danner et al., 2017).
Creating SNH at larger scales may not be a plausible option for a single farmer given a lack of space as well as financial and logistical constraints (Osterman et al., 2021c). Decreasing agricultural intensity for pollination may also not be a realistic option for commercial blueberry growers. However, incorporation of small-scale habitat elements is one promising way to encourage wild bees. For example, hedgerows composed of linearly shaped plantings with flowering woody and herbaceous plants as well as flower strips of herbaceous plants placed at field margins do not take much land area and can be of high value to native pollinators, particularly if plant species are locally adapted (Garibaldi et al., 2014; Albrecht et al., 2020). The contribution of flower strips to blueberry pollination and yield has been investigated, with Blaauw and Isaacs (2014) concluding that these plantings can support higher crop yields 3 years after seeding, and are economical, even when considering the costs of establishment and maintenance. Careful selection of plant species is essential and should be done to support key pollinators of blueberry (Eeraerts et al., 2021; Windsor et al., 2021) while minimizing their potential to serve as alternative hosts to blueberry pests and diseases. An evaluation of hedgerows has not been carried out to date for blueberry systems and is challenging experimentally because it takes a considerable time for establishment and to observe the effects on pollinators and pollination (e.g., Morandin and Kremen, 2013). Nevertheless, the impacts of hedgerows and other perennial plantings on pollinators and pollination in blueberry should be studied as these elements can provide floral resources throughout the year (Timberlake et al., 2019), nesting habitat for wild bees (Eeraerts et al., 2021), and have great potential to enhance pollination without requiring significant land area and changes to in-field production practices.
Wild bees, as well as alternative managed bees, are an important component of ICP and can complement honey bees as some of these non-Apis bees forage in suboptimal weather conditions, or early morning and late afternoon, when honey bees are less likely to forage (Tuell and Isaacs, 2010a; Rogers et al., 2013, 2014). Furthermore, wild bees may forage in different parts of the plant canopy and may also improve the foraging behavior and pollination efficiency of honey bees in some crop systems requiring cross-pollination (Tuell and Isaacs, 2009; Brittain et al., 2013; Eeraerts et al., 2020). A mechanistic explanation regarding the positive interactive effect of non-Apis bees on honey bees is not entirely clear, but likely includes direct interactions between different kinds of bees during flower visitation (Greenleaf and Kremen, 2006; Carvalheiro et al., 2011) and indirect interactions between bees due to scent marks deposited on flowers after they have been visited (Stout and Goulson, 2001). This functional complementary of foraging behavior contributes to the benefits of combining honey bees and non-Apis bees and their corresponding pollination contribution (Blüthgen and Klein, 2011). So far, these interactions between honey bees and non-Apis bees have not been investigated in blueberry but are expected to be similar.
Environmental factors
Temperature is a key driver of plant processes related to pollination such as bloom timing, bloom duration, pollen germination, pollen tube growth, ovule viability, flower longevity, and duration of flower receptivity. Studies investigating the effects of temperature on blueberry physiology are limited, especially as it relates to flower biology and reproduction, but are needed to inform adaptation strategies as the climate changes. Kirk and Isaacs (2012) compared blueberry bloom phenology in field-planted and greenhouse-potted bushes and found that flowering of potted bushes was delayed by ~100 growth degree days (GDD), which was likely attributed to potted bushes receiving a lower number of chilling hours than those assessed in the field. As climate change intensifies, the number of chilling hours experienced by blueberry plants will decrease in many key production regions and will likely impact bloom timing and characteristics. Variation in blueberry bloom timing may lead to complications with pollination such as phenological mismatch with the emergence of wild spring pollinators, and difficulties in predicting timing of honey bee hive placement.
Among the stages of plant reproductive development, sensitivity to heat extremes has been observed during pollen development, pollen germination, and pollen tube elongation for various crop plants (Mesihovic et al., 2016). However, pollen performance in blueberry is not well characterized (Yang et al., 2019; Gan et al., 2020). Gan et al. (2020) explored pollen germination and pollen tube length for four commercially relevant northern highbush blueberry cultivars at 2, 7, 13, 18, and 24°C and found that germination generally increased from 2 to 24°C across all cultivars (besides “Liberty”, which peaked at 13 and 18°C). Pollen tube growth increased linearly across all cultivars from 2 to 24°C. Yang et al. (2019) assessed pollen germination and tube growth of “Gardenblue” rabbiteye blueberry in “Brightwell” pistils in situ and found that after 24 h over 90% of pollen on styles had germinated at 15, 20 and 25°C, while at 10, 30, and 35°C the percentage of the style with germinating pollen was 72, 63, and 57%, respectively. This study also reported that pollen failed to fully transverse the style at 35°C. Further limiting fertilization success, the same study reported that hot conditions at 30 and 35°C accelerated “Brightwell” ovule degradation after only 4 h and 74–100% after 120 h of exposure to these temperatures. Cooler temperatures (0 and 5°C) had less impact on “Brightwell” ovule degradation. Further investigations should explore thermotolerance capacities of blueberry pollen and ovaries for commercially important cultivars and develop an understanding of how environmental conditions can be incorporated into ICP programs for this crop.
Pesticide stewardship
During bloom, blueberry plants are susceptible to various fungal diseases such as mummy berry [Monilinia vaccinii-corymbosi (Reade) Honey], anthracnose (Colletotrichum spp.), and botrytis (Botrytis cinerea Pers.), and they can also become infected by viruses (Polashock et al., 2017). Blueberry fields may also need protection from insects including aphids that transmit viruses, fruitworm larvae that bore into developing berries, and various moth larvae that can defoliate bushes at this time of the season. Integrated pest management programs that incorporate monitoring, predictive models, resistant cultivars, and cultural controls have been developed to reduce the risk of economic injury from these pests (Rodriguez-Saona et al., 2019), but pesticide applications remain a common and frequent input in most blueberry production regions.
Bees can be exposed to pesticides via direct exposure during application, from landing on treated surfaces, through contaminated nectar and guttation fluid, and by residues collected in pollen and nectar. Pesticide residues are common in commercial honey bee colonies used for pollination (Mullin et al., 2010). In a recent study in Michigan blueberry farms, 22 pesticides were detected on average in pollen samples collected from bumble bee and honey bee colonies during bloom, even at sites that were not being sprayed (Graham et al., 2021b). As expected, the highest concentrations detected were pesticides applied during bloom, but the 80 identified active ingredients also included various other pesticides likely collected from outside of blueberry farms. The chemical residues and their high concentrations highlight the need for development and adoption of practices that minimize the need for pesticide applications during crop flowering, combined with widespread awareness of exposure from neighboring lands.
Given their direct toxicity (Johnson et al., 2010) and potential to contribute to the stresses experienced by wild and managed bees (Goulson et al., 2015; Sánchez-Bayo et al., 2016), application of the most toxic insecticides is restricted during bloom. These limits, however, do not completely prevent risk of harm to bees and other pollinators. First, many wild pollinators have flight periods that extend before and after blueberry bloom, so they may not be protected by restrictions limited to bloom time. Label restrictions are also only for the few pesticides known to be acutely toxic, and not for the multiple other agrichemicals that can cause sublethal effects. Finally, pesticides applied elsewhere provide additional exposure routes for bees foraging outside the blueberry farms.
While it has been shown that wild bee communities in blueberry fields are less diverse in farms with higher pesticide toxicity (Tuell and Isaacs, 2010b), more research is needed to understand the effects of pesticide use on pollination outcomes. Many aspects of pesticide risk are profoundly understudied (Cullen et al., 2019), including combination exposures, synergistic effects (Siviter et al., 2021), effects of adjuvants and inactive ingredients (Straw et al., 2022; Wernecke et al., 2022), effects on developing bees (Claus et al., 2021), and effects on wild bees. Pollinator health risks from fungicides should be especially examined because exposure rates are so high (Graham et al., 2021b), and new research shows harm from fungicides to both bumble bees (for example Bernauer et al., 2015) and honey bees (Fisher et al., 2021). The Integrated Pest and Pollinator Management framework (Biddinger and Rajotte, 2015) can help balance the economic implications of spending money on pest control inputs vs. conserving wild pollinators and protecting honey bees. This approach can be tailored to blueberry settings using the regionally relevant community of insect and disease pests combined with the economics of pesticide and pollinator inputs (Egan et al., 2020).
Economic value of Integrated Crop Pollination
Successful application of integrated blueberry pollination strategies at the farm level will need to encompass economics to ensure farms remain profitable. The benefits of pollination in agricultural settings are typically measured in terms of crop yield and quality. For example, Rollin and Garibaldi (2019) conducted a meta-analysis of 22 studies, involving various crops grown in different countries, to examine the impact of honey bee densities on crop productivity variables such as fruit set, seed set, fruit weight, and yield. They found that increased colony density and visitation rates augmented all productivity variables to a certain optimum, after which more honey bees do not increase pollination and yield any further. Several other studies documented positive linkages of pollination and crop productivity (see previous sections above). However, there are costs associated with implementing any pollination strategy and it is critical that benefits exceed costs to ensure a return on investment.
In the U.S., Hellerstein et al. (2017) conducted a national survey in 2015 and showed blueberry ranked third in terms of highest demand for honey bee pollination with rankings based on the total pollination fees paid for each crop determined by the total number of colonies used, price per colony, and total acreage pollinated. Only almond and apple (Malus domestica Borkh.) preceded blueberry, highlighting the significance of blueberry pollination. The insect pollination economic value (IPEV) of honey bee is known to be high (Morse and Calderone, 2000; Reilly et al., 2020; Jordan et al., 2021). Reilly et al. (2020) estimated the IPEV of seven crops [almond, apple, blueberry, pumpkin (C. pepo L.), sweet cherry (Prunus avium L.), tart cherry (P. cerasus L.), and watermelon (Citrullus lanatus (Thunb.) Matsum. & Nakai)] in major producing areas in the U.S. and placed it at ~$6.4 billion by managed honey bees and $1.5 billion by wild bees, with wild bees contributing $50 million in blueberry. Jordan et al. (2021) considered all insect pollinators and estimated an average IPEV of $34 billion for 75 crops across the U.S.
Insect pollination economic value estimates pertain to direct benefits of pollination services. However, there are limitations to the IPEV, such as the assumption of static pollination densities and constant dependency coefficients despite the crops being planted in different fields, production regions, and years (Melathopoulos et al., 2015). Also, there are indirect benefits to the environment (spillover pollination from surrounding landscapes), consumers (nutrition), and downstream industry sectors (e.g., livestock feed, food processing, consumer products) that must be considered to capture a full accounting of the economic benefits of crop pollination. Hence, values provided by the above studies can be considered conservative estimates but are useful to start framing the economic importance of pollinators. More holistic economic assessments that implement all components of ICP are also lacking. Consequently, comparing the costs of implementing an ICP framework to the contribution of improved pollination on the productivity of the cropping system are needed to determine its value for farmers.
Despite the economic value of wild bees in blueberry systems (Reilly et al., 2020), pollinator habitat establishment costs are perceived to be high and this in turn can be a barrier to implementing habitat elements that would benefit wild pollinators on farms (Garbach and Morgan, 2017). However, a multi-year study showed yield and profitability of blueberry fields adjacent to native wildflower plantings were greater than fields without wildflowers and wildflower establishment costs could be recovered within 3–4 years (Blaauw and Isaacs, 2014). Landscape and management can influence the costs and benefits of maintaining or converting land into natural habitat that could benefit wild pollinators and their services (Magrach et al., 2019), underscoring the need for a robust understanding of these systems to develop accurate estimates for economic valuation. Of note is that blueberry is a perennial crop managed intensively for pest control. Pollinator habitat is typically located in non-arable land outside the crop fields. In some instances the extent of non-arable land can be considerable, but elsewhere, it may be limited. In these situations there may be direct and indirect opportunity costs that provide only small, private benefits to the grower making the investment (Lonsdorf et al., 2020). Few studies, in fact, have calculated the opportunity costs associated with converting land to other valuable uses. Some scenarios show the opportunity costs may outweigh the benefits accrued from additional wild pollination services (Magrach et al., 2019). More research should be dedicated to estimate opportunity costs against wild pollination benefits across different blueberry growing regions.
Decision aid systems
Effective implementation of improved blueberry pollination strategies that maximize profit may be aided by advances in and implementation of technologies like Big Data, Remote Sensing, Artificial Intelligence, and Cloud Computing which support “Agriculture 4.0.” This is poised to enable growers to be more efficient and economical in their production of quality crops while minimizing potential harms to agroecosystems through informed decisions when it comes to implementation of various agricultural practices. Decision aid or decision support systems (DAS or DSS, respectively) are tools that encompass quantitative models inside interactive software applications and can assist growers in making complex, evidence-based, and precise decisions (Jones et al., 1998). Effective utilization of DAS depends on upstream efforts to formulate models that provide predictions with sufficient accuracy and deliver resultant information in user-friendly platforms. The careful selection of model inputs, both decision variables and predictors, is an essential component of constructing a DAS that can effectively compare different courses of action. Model inputs may include historically collected data, routinely updated data streams, user input, and should be chosen to balance model accuracy with the cost efficiency and reliability of inputs (Zhai et al., 2020).
For optimization of pollination and yield in blueberry, a DAS should predict changes in crop pollination and yield components based on changes in managed and wild bee populations. In its simplest form, this model might be characterized by estimating the effect of pollinator density on pollinator visits, the effect of pollinator visits on fruit set, seed set, and berry size, and the impact of these three quantities on yield. The impact of the number of honey bee hives on blueberry yield can depend on the densities of other bees in the surrounding area (Eaton and Nams, 2012). Thus, a DAS would likely benefit from including user inputs or data that characterizes wild pollinator populations in the surrounding area and components of ICP.
If a DAS is to be used to time the placement of honey bee colonies, it may be advantageous to model the timing of blueberry anthesis (Kirk and Isaacs, 2012). Phenology timing may be modeled based in part on temperature and heat accumulation to capture phenological effects on pollinator behavior as well as limit pollinator effects to the bloom window. Furthermore, as pollination is heavily weather dependent, the effect of meteorological variables on pollinator foraging activity and crop reproduction can be integrated too (Tuell and Isaacs, 2010a). Cultivar effects should also be accounted for given floral morphological traits and subsequent visitation rates by bees varies by cultivar (Courcelles et al., 2013).
The DAS approach has been developed in some cropping systems for pest management and irrigation to improve water use efficiency (Samietz et al., 2007; Jones et al., 2010; Bonfante et al., 2019). DAS is starting to be used to inform pollination-related decisions and could be a valuable tool for ICP. BEE-STEWARD is one research and decision-support software tool that can show how different environmental variables impact wild bumble bees in the landscape (Twiston-Davies et al., 2021). Climate-induced declines in fruit set in bog blueberry (Vaccinium uliginosum L.) has also been predicted to be mitigated with increases in honey bee and bumble bee densities using predictive modeling approaches based on the transfer learning concept to solve for data deficiency problems (Qu et al., 2021). More recently, an agent-based model was used in kiwi [Actinidia chinensis var. deliciosa (A. Chev)] to track and predict pollination outcomes in a dioecious crop under a range of management scenarios (Broussard et al., 2022). Similar approaches and platforms may be used to aid growers in making decisions integral to successful pollination. However, issues of interoperability, scalability, accessibility, and usability need to be addressed (Zhai et al., 2020). Other relevant challenges identified by Zhai et al. (2020) include adapting to uncertainty and dynamic factors to provide accurate decision support, incorporating knowledge from experts, enabling prediction and forecasts to enhance farm planning, and using historical information. Furthermore, release of DAS tools to growers needs to be synergized with extension education programs and training to ensure successful utilization.
Conclusion
Development of an integrated approach to sustainable blueberry pollination will require a thorough understanding of cultivar-specific pollination requirements within traditional and new production regions. Coordinated use of managed honey bees, wild pollinators, and alternative managed bees where their use provides a return on investment will ensure resilience in blueberry pollination systems. However, this coordination should be integrated with an awareness of how horticultural factors, habitat enhancement, and pesticide stewardship also influence pollination and yield. At the same time, adoption of improved blueberry production systems will be most likely if their profitability can be documented, so there is a need to measure the costs of inputs and the value of returns to determine the economic viability of ICP approaches. Multidisciplinary research that includes the perspectives and experiences shared by growers, beekeepers, and extension/crop advisors will be needed to address knowledge gaps and translate information gained into practice at the farm level. Key gaps requiring research focus in honey bee dominated systems are the integration of wild and alternative managed pollinators to benefit from their pollination contribution, particularly when weather is suboptimal for honey bees. At the same time, honey bees are integral pollinators and research on efficacious honey bee deployment as a function of colony size, hive density, crop demand, and landscape structure are needed while encouraging pollinator recruitment and minimizing the potential negative effects on native insect populations. Research on habitat enhancement and landscape modification will also be needed to support all pollinators and attention should particularly be focused on how to realistically and economically achieve this at commercial scales. While pesticide stewardship is a relatively well-defined component of ICP, the role of horticultural practices remains ambiguous and will be an important area of future research. Many plant and soil factors seemingly independent of pollination may influence yield and crop quality outcomes in blueberry. Understanding and optimizing these factors within the ICP framework is expected to promote long-term crop yield and quality while enhancing growers' returns on their investments. With increasing availability of on- and off-farm data, statistical models, and software tools, there is also potential that the complexity of applying ICP on commercial blueberry farms could be simplified and made more accessible to growers and consultants. This could be accomplished through effective use of DAS that leverages existing and emerging information, adding new layers, and improved function as new research is completed. This must be blended with education and extension programs that encourage adoption, make adjustments when needed, and foster implementation to provide for more evidence-based blueberry pollination systems.
Author contributions
LWD, RI, REM, SG, and AM procured funding. LWD, ME, and RI created the framework of the article. SC created tables (including Supplementary Tables) and KB created the figure and graphical abstract. All authors contributed to review of the literature, writing, critical review, and gave final approval for publication.
Funding
This article is based upon work that is supported by the National Institute of Food and Agriculture (NIFA), U.S. Department of Agriculture, under award number 2020-51181-32155 from the Specialty Crops Research Initiative, and NIFA Hatch project 1014919. ME was supported as a Belgian American Educational Foundation postdoctoral fellow. Any opinions, findings, conclusions, or recommendations expressed in this article are those of the authors and do not necessarily reflect the view of the U.S. Department of Agriculture.
Conflict of interest
The authors declare that the research was conducted in the absence of any commercial or financial relationships that could be construed as a potential conflict of interest.
Publisher's note
All claims expressed in this article are solely those of the authors and do not necessarily represent those of their affiliated organizations, or those of the publisher, the editors and the reviewers. Any product that may be evaluated in this article, or claim that may be made by its manufacturer, is not guaranteed or endorsed by the publisher.
Supplementary material
The Supplementary Material for this article can be found online at: https://www.frontiersin.org/articles/10.3389/fsufs.2022.1006201/full#supplementary-material
References
Aizen, M. A., Arbetman, M. P., Chacoff, N. P., Chalcoff, V. R., Feinsinger, P., Garibaldi, L. A., et al. (2020). Invasive bees and their impact on agriculture. Adv. Ecol. Res. 63, 49–92. doi: 10.1016/bs.aecr.2020.08.001
Aizen, M. A., and Harder, L. D. (2009). The global stock of domesticated honey bees is growing slower than agricultural demand for pollination. Curr. Biol. 19, 915–918. doi: 10.1016/j.cub.2009.03.071
Aizen, M. A., Smith-Ramírez, C., Morales, C. L., Vieli, L., Sáez, A., Barahona-Segovia, R. M., et al. (2019). Coordinated species importation policies are needed to reduce serious invasions globally: the case of alien bumblebees in South America. J. Appl. Ecol. 56, 100–106. doi: 10.1111/1365-2664.13121
Albrecht, M., Kleijn, D., Williams, N. M., Tschumi, M., Blaauw, B. R., Bommarco, R., et al. (2020). The effectiveness of flower strips and hedgerows on pest control, pollination services and crop yield: a quantitative synthesis. Ecol. Lett. 23, pp.1488–1498. doi: 10.1111/ele.13576
Arnold, S. E., Dudenhöffer, J. H., Fountain, M. T., James, K. L., Hall, D. R., Farman, D. I., et al. (2021). Bumble bees show an induced preference for flowers when primed with caffeinated nectar and a target floral odor. Curr. Biol. 31, 4127–4131. doi: 10.1016/j.cub.2021.06.068
Arrington, M., and DeVetter, L. W. (2018a). Increasing honey bee hive densities promotes pollination and yield components of highbush blueberry in Western Washington. HortScience 53, 191–194. doi: 10.21273/HORTSCI12644-17
Arrington, M., and DeVetter, L. W. (2018b). Floral morphology differs among new northern highbush blueberry cultivars. J. Hortic. 5, 1–4. doi: 10.4172/2376-0354.1000223
Bailes, E. J., Ollerton, J., Pattrick, J. G., and Glover, B. J. (2015). How can an understanding of plant–pollinator interactions contribute to global food security? Curr. Opin. Plant Biol. 26, 72–79. doi: 10.1016/j.pbi.2015.06.002
Bartomeus, I., Molina, F. P., Hidalgo-Galiana, and A., Ortego, J. (2020). Safeguarding the genetic integrity of native pollinators requires stronger regulations on commercial lines. Ecol. Solut. Evid. 1, e12012. doi: 10.1002/2688-8319.12012
Benjamin, F. E., Reilly, J. R., and Winfree, R. (2014). Pollinator body size mediates the scale at which land use drives crop pollination services. J. Appl. Ecol. 51, 440–449. doi: 10.1111/1365-2664.12198
Benjamin, F. E., and Winfree, R. (2014). Lack of pollinators limits fruit production in commercial blueberry (Vaccinium corymbosum). Environ. Entomol. 43, 1574–1583. doi: 10.1603/EN13314
Bernauer, O. M., Gaines-Day, H. R., and Steffan, S. A. (2015). Colonies of bumble bees (Bombus impatiens) produce fewer workers, less bee biomass, and have smaller mother queens following fungicide exposure. Insects 6, 478–488. doi: 10.3390/insects6020478
Biddinger, D. J., and Rajotte, E. G. (2015). Integrated pest and pollinator management—adding a new dimension to an accepted paradigm. Curr. Opin. Insect Sci. 10, 204–209. doi: 10.1016/j.cois.2015.05.012
Blaauw, B. R., and Isaacs, R. (2014). Flower plantings increase wild bee abundance and the pollination services provided to a pollination-dependent crop. J. Appl. Ecol. 51, 890–898. doi: 10.1111/1365-2664.12257
Blüthgen, N., and Klein, A. (2011). Functional complementarity and specialisation: the role of biodiversity in plant-pollinator interactions. Basic Appl. Ecol. 12, 282–291. doi: 10.1016/j.baae.2010.11.001
Bobiwash, K., Uriel, Y., and Elle, E. (2018). Pollen foraging differences among three managed pollinators in the highbush blueberry (Vaccinium corymbosum) agroecosystem. J. Econom. Entomol. 111, 26–32. doi: 10.1093/jee/tox344
Bonfante, A., Monaco, E., Manna, P., De Mascellis, R., Basile, A., Buonanno, M., et al. (2019). LCIS DSS—an irrigation supporting system for water use efficiency improvement in precision agriculture: a maize case study. Agric. Syst. 176, 102646. doi: 10.1016/j.agsy.2019.102646
Bos, M. M., Veddeler, D., Bogdanski, A. K., Klein, A. M., Tscharntke, T., Steffan-Dewenter, I., et al. (2007). Caveats to quantifying ecosystem services: fruit abortion blurs benefits from crop pollination. Ecol. Appl. 17, 1841–1849. doi: 10.1890/06-1763.1
Bozek, M. (2021). Nectar production and spectrum of insect visitors in six varieties of highbush blueberry (Vaccinium corymbosum L.) in SE Poland. Acta Agrobot. 74, 7410. doi: 10.5586/aa.7410
Brazelton, C., Fain, C., Ogg, M., Riquelme, C., Rodriguez, V., and Mulvey, D. (2021). Global state of the blueberry industry report-−2021. Int. Blueberry Org. 1–167.
Breeze, T. D., Vaissière, B. E., Bommarco, R., Petanidou, T., Seraphides, N., Kozak, L., et al. (2014). Agricultural policies exacerbate honeybee pollination service supply-demand mismatches across Europe. PloS ONE 9, e82996. doi: 10.1371/journal.pone.0082996
Brevis, P. A., Bassil, N. V., Ballington, J. R., and Hancock, J. F. (2008). Impact of wide hybridization on highbush blueberry breeding. J. Am. Soc. Hortic. Sci. 133, 427–437. doi: 10.21273/JASHS.133.3.427
Brevis, P. A., NeSmith, D. S., and Wetzstein, H. Y. (2006). Flower age affects fruit set and stigmatic receptivity in rabbiteye blueberry. HortScience 41, 1537–1540. doi: 10.21273/HORTSCI.41.7.1537
Brittain, C., Williams, N., Kremen, C., and Klein, A. (2013). Synergistic effects of non-Apis bees and honey bees for pollination services. Proc. Royal Soc. B 280, 20122767. doi: 10.1098/rspb.2012.2767
Brody, A. K., Waterman, B., Ricketts, T. H., Degrassi, A. L., González, J. B., Harris, J. M., et al. (2019). Genotype-specific effects of ericoid mycorrhizae on floral traits and reproduction in Vaccinium corymbosum. Am. J. Bot. 106, 1412–1422. doi: 10.1002/ajb2.1372
Broussard, M. A., Jochym, M., Tomer, N., Jesson, L., Shaw, A. K., Crowder, D. W., et al. (2022). Using agent-based models to predict pollen deposition in a dioecious crop. bioRxiv. In press. doi: 10.1101/2022.07.28.501917
Bruinsma, M., Lucas-Barbosa, D., ten Broeke, C. J., van Dam, N. M., van Beek, T. A., Dicke, M., et al. (2014). Folivory affects composition of nectar, floral odor and modifies pollinator behavior. J. Chem. Eco. 40, 39–49. doi: 10.1007/s10886-013-0369-x
Bryla, D. R., and Strik, B. C. (2015). Nutrient requirements, leaf tissue standards, and new options for fertigation of northern highbush blueberry. HortTechnology 25, 464–470. doi: 10.21273/HORTTECH.25.4.464
Buchmann, S. L. (1983). “Buzz pollination in angiosperms,” in: Handbook of Experimental Pollination Biology, eds C. E. Jones, R. J. Little (New York: Scientific and Academic Editions). p. 73–113.
Burgett, M., Daberkow, S., Rucker, R., and Thurman, W. N. (2010). U.S. pollination markets: recent changes and historical perspective. Am. Bee J. 150, 35–40.
Burgett, M., Rucker, R. R., and Thurnman, W. N. (2004). Economics and honey bee pollination markets. Am. Bee J. 144, 269–271.
Button, L., and Elle, E. (2014). Wild bumble bees reduce pollination deficits in a crop mostly visited by managed honey bees. Agric. Ecosyst. Environ. 197, 255–263. doi: 10.1016/j.agee.2014.08.004
Cameron, J. S., Brun, C. A., and Hartley, August, C. A. (1988). The influence of soil moisture stress on the growth and gas exchange characteristics of young highbush blueberry plants (Vaccinium corymbosum L.). Acta Hortic. 241, 254–259. doi: 10.17660/ActaHortic.1989.241.41
Campbell, J. W., Kimmel, C. B., Bammer, M., Stanley-Stahr, C., Daniels, J. C., and Ellis, J. D. (2018). Managed and wild bee flower visitors and their potential contribution to pollination services of low-chill highbush blueberry (Vaccinium corymbosum L.; Ericales: Ericaceae). J. Econom. Entomol. 111, 2011–2016. doi: 10.1093/jee/toy215
Cane, J. H. (1997). Ground-nesting bees: the neglected pollinator resource for agriculture. VII Int. Symp. Pollinat. 437, 309–324. doi: 10.17660/ActaHortic.1997.437.38
Cardel, Y. J., and Koptur, S. (2010). Effects of florivory on the pollination of flowers: an experimental field study with a perennial plant. In. J. Plant Sci. 171, 283–292. doi: 10.1086/650154
Cardinal, S., Buchmann, S. L., and Russell, A. L. (2018). The evolution of floral sonication, a pollen foraging behavior used by bees (Anthophila). Evolution 72, 590–600. doi: 10.1111/evo.13446
Carvalheiro, L. G., Veldtman, R., Shenkute, A. G., Tesfay, G. B., Pirk, C. W. W., Donaldson, J. S., et al. (2011). Natural and within-farmland biodiversity enhances crop productivity. Ecol. Lett. 14, 251–259. doi: 10.1111/j.1461-0248.2010.01579.x
Cavigliasso, P., Bello, F., Rivadeneira, M. F., Monzon, N. O., Gennari, G. B., and Basualdo, M. (2020). Pollination efficiency of managed bee species (Apis mellifera and Bombus pauloensis) in highbush blueberry (Vaccinium corymbosum) productivity. J. Hortic. Res. 28, 57–64. doi: 10.2478/johr-2020-0003
Cavigliasso, P., Negri, P., Viel, M., Graziani, M. M., Challiol, C., Bello, F., et al. (2021). Precision management of pollination services to blueberry crops. Sci. Rep. 11, 20453. doi: 10.1038/s41598-021-00068-1
Chabert, S., Mallinger, R. E., Sénéchal, C., Fougeroux, A., Geist, O., Guillemard, V., et al. (2022). Importance of maternal resources in pollen limitation studies with pollinator gradients: a case study with sunflower. Agric. Ecosyst. Environ. 330, 107887. doi: 10.1016/j.agee.2022.107887
Chabert, S., Requier, F., Chadoeuf, J., Guilbaud, L., Morison, N., and Vaissiere, B. E. (2021). Rapid measurement of the adult worker population size in honey bees. Ecol. Indic. 122, 107313. doi: 10.1016/j.ecolind.2020.107313
Claus, G., Pisman, M., Spanoghe, P., Smagghe, G., and Eeraerts, M. (2021). Larval oral exposure to thiacloprid: Dose-response toxicity testing in solitary bees, Osmia spp. (Hymenoptera: Megachilidae). Ecotox. Environ. Saf. 215, 112143. doi: 10.1016/j.ecoenv.2021.112143
Connell, J. H. (2000). Pollination of almonds: practices and problems. HortTechnology 10, 116–119. doi: 10.21273/HORTTECH.10.1.116
Cooley, H., and Vallejo-Marín, M. (2021). Buzz-pollinated crops: a global review and meta-analysis of the effects of supplemental bee pollination in tomato. J. Econom. Entomol. 114, 505–519. doi: 10.1093/jee/toab009
Cortés-Rivas, B., Smith-Ramirez, C., Monzón, V. H., and Mesquita-Neto, J. N. (2022). Native bees with floral sonication behaviour can achieve high-performance pollination of highbush blueberry in Chile. Agric. Forest Entomol. 1–12. doi: 10.1111/afe.12533
Courcelles, D. M. M., Button, L., and Elle, E. (2013). Bee visit rates vary with floral morphology among highbush blueberry cultivars (Vaccinium corymbosum L.). J. Appl. Entomol. 137, 693–701. doi: 10.1111/jen.12059
Couvillon, M. J., Schürch, R., and Ratnieks, F. L. W. (2014). Waggle dance distances as integrative indicators of seasonal foraging challenges. PLoS ONE 9, 1–7. doi: 10.1371/journal.pone.0093495
Coville, F. V. (1921). Directions for blueberry culture. U.S.D.A. Bull. 974. doi: 10.5962/bhl.title.108248
Cremins, W. J. (1952). Effects of certain pollinators on fruit and seed development in the highbush blueberry (MSc thesis). Oregon State College, Oregon, USA.
Cullen, M. G., Thompson, L. J., Carolan, J. C., Stout, J. C., and Stanley, D. A. (2019). Fungicides, herbicides and bees: a systematic review of existing research and methods. PLoS ONE 14, e0225743. doi: 10.1371/journal.pone.0225743
Cunningham, S. A., Fournier, A., Neave, M. J., and Le Feuvre, D. (2016). Improving spatial arrangement of honeybee colonies to avoid pollination shortfall and depressed fruit set. J. Appl. Ecol. 53, 350–359. doi: 10.1111/1365-2664.12573
Czesnik, E., Bounous, G., and Gioffr,è, D. (1989). A survey of self-incompatibility in highbush blueberry (Vaccinium corymbosum L.). Acta Hortic. 241, 56–63. doi: 10.17660/ActaHortic.1989.241.6
Danka, R. G., Lang, G. A., and Gupton, C. L. (1993). Honey bee (Hymenoptera: Apidae) visits and pollen source effects on fruiting of ‘Gulfcoast' southern highbush blueberry. J. Econom. Entomol. 86, 131–136. doi: 10.1093/jee/86.1.131
Danka, R. G., Sampson, B. J., and Villa, J. D. (2019). Association between density of foraging bees and fruit set in commercial fields of rabbiteye blueberries (Ericales: Ericaceae) in Louisiana and Mississippi. J. Econom. Entomol. 112, 1322–1326. doi: 10.1093/jee/toz005
Danner, N., Keller, A., Haller, S., and Steffan-Dewenter, I. (2017). Honey bee foraging ecology: season but not landscape diversity shapes the amount and diversity of collected pollen. PLoS ONE 12, e0183716. doi: 10.1371/journal.pone.0183716
De Groot, G. A., Van, K.ats, Reemer, R., van der Sterren, D., Biesmeijer, J. C., and Kleijn, D. (2015). De bijdrage van (wilde) Bestuivers aan de Opbrengst Van Appels en Blauwe Bessen; Kwantificering Van Ecosysteemdiensten in Nederland. Wageningen, Alterra Wageningen UR: University and Research centre, Alterra-rapport. p. 2636.
Dedej, S., and Delaplane, K. S. (2004). Nectar-robbing carpenter bees reduce seed-setting capability of honey bees (Hymenoptera: Apidae) in rabbiteye blueberry, Vaccinium ashei, “Climax.” Environ. Entomol. 33, 100–106. doi: 10.1603/0046-225X-33.1.100
Delaplane, K. S., Mayer, D. R., and Mayer, D. F. (2000). Crop Pollination by Bees. New York, USA: CABI Publishing. doi: 10.1079/9780851994482.0000
Díaz, P. C., Arenas, A., Fernández, V. M., Susic Martin, C., Basilio, A. M., and Farina, W. M. (2013). Honeybee cognitive ecology in a fluctuating agricultural setting of apple and pear trees. Behav. Ecol. 24, 1058–1067. doi: 10.1093/beheco/art026
Dogterom, M. H. (1999). “Comparison of four bee species as pollinators of highbush blueberry Vaccinium corymbosum L. (Ericaceae) ‘Bluecrop',” in Pollination by Four Species of Bees on Highbush Blueberry. PhD thesis (British Columbia, Canada: Simon Fraser University). p. 23–111.
Dogterom, M. H., and Winston, M. L. (1999). Pollen storage and foraging by honey bees (Hymenoptera: Apidae) in highbush blueberries (Ericaceae), cultivar Bluecrop. Can. Entomol. 131, 757–768. doi: 10.4039/Ent131757-6
Dogterom, M. H., Winston, M. L., and Mukai, A. (2000). Effect of pollen load size and source (self, outcross) on seed and fruit production in highbush blueberry cv. “Bluecrop” (Vaccinium corymbosum; Ericaceae). Am. J. Bot. 87, 1584–1591. doi: 10.2307/2656734
Doi, K., Inoue, R., and Iwasaki, N. (2021). Seed weight mediates effects of pollen on berry weight, ripening, and anthocyanin content in highbush blueberry. Sci. Hortic. 288, 110313. doi: 10.1016/j.scienta.2021.110313
Durant, J. (2019). “Chapter three: feedlot bees/unruly bees—the industrialization of honey bees for almond pollination,” in: Bitter Honey: A Political Ecology of Honey Bee Declines in California. PhD thesis. (Berkeley, California, USA: University of California). p. 82–110.
Eaton, L. J., and Nams, V. O. (2012). Honey bee stocking numbers and wild blueberry production in Nova Scotia. Can. J. Plant Sci. 92, 1305–1310. doi: 10.4141/cjps2012-045
Eck, P., and Childers, N. F. (1966). Blueberry Culture. New Brunswick, NJ: Rutgers University Press.
Eeraerts, M. (2022). Increasing wild bee richness and abundance on sequentially flowering cultivars of a pollinator-dependent crop. Agric Ecosyst Environ. 325, 107745. doi: 10.1016/j.agee.2021.107745
Eeraerts, M., Clymans, R., Van Kerckvoorde, V., and Beliën, T. (2022). Nesting material, phenology and landscape complexity influence nesting success and parasite infestation of a trap nesting bee. Agric. Ecosys. Environ. 332, 107951. doi: 10.1016/j.agee.2022.107951
Eeraerts, M., Smagghe, G., and Meeus, I. (2020). Bumble bee abundance and richness improves honey bee pollination behaviour in sweet cherry. Basic Appl. Ecol. 43, 27–33. doi: 10.1016/j.baae.2019.11.004
Eeraerts, M., Van Den Berge, S., Proesmans, W., Verheyen, K., Smagghe, G., and Meeus, I. (2021). Fruit orchards and woody semi-natural habitat provide complementary resources for pollinators in agricultural landscapes. Landsc. Ecol. 36, 1377–1390. doi: 10.1007/s10980-021-01220-y
Egan, P. A., Adler, L. S., Irwin, R. E., Farrell, I. W., Palmer-Young, E. C., and Stevenson, P. C. (2018). Crop domestication alters floral reward chemistry with potential consequences for pollinator health. Front. Plant Sci. 9, 1357. doi: 10.3389/fpls.2018.01357
Egan, P. A., Dicks, L. V., Hokkanen, H. M. T., and Stenberg, J. A. (2020). Delivering integrated pest and pollinator management (IPPM). Trends Plant Sci. 25, 577–589. doi: 10.1016/j.tplants.2020.01.006
Ehlenfeldt, M. K. (1994). The genetic composition and tetrasomic inbreeding coefficients of highbush blueberry cultivars. HortScience 29, 1342–1345. doi: 10.21273/HORTSCI.29.11.1342
Ehlenfeldt, M. K. (2001). Self- and cross-fertility in recently released highbush blueberry cultivars. HortScience 36, 133–135. doi: 10.21273/HORTSCI.36.1.133
Ehlenfeldt, M. K. (2003). Investigations of metaxenia in northern highbush blueberry (Vaccinium corymbosum L.) cultivars. J. Am. Pomol. Soc. 57, 26–31.
Ehlenfeldt, M. K. (2012). Breeding for parthenocarpic fruit development in blueberry. Int. J. Fruit Sci. 12, 261–268. doi: 10.1080/15538362.2011.623082
Ehlenfeldt, M. K., and Vorsa, N. (2007). Inheritance patterns of parthenocarpic fruit development in highbush blueberry (Vaccinium corymbosum L.). HortScience 42, 1127–1130. doi: 10.21273/HORTSCI.42.5.1127
Ellis, A., and Delaplane, K. S. (2009). An evaluation of Fruit-Boost™ as an aid for honey bee pollination under conditions of competing bloom. J. Apic. Res. 48, 15–18. doi: 10.3896/IBRA.1.48.1.04
Estravis-Barcala, M. C., Palottini, F., and Farina, W. M. (2021a). Learning of a mimic odor combined with nectar nonsugar compounds enhances honeybee pollination of a commercial crop. Sci. Rep. 11, 1–8. doi: 10.1038/s41598-021-03305-9
Estravis-Barcala, M. C., Palottini, F., Macri, I., Nery, D., and Farina, W. M. (2021b). Managed honeybees and South American bumblebees exhibit complementary foraging patterns in highbush blueberry. Sci. Rep. 11, 8187. doi: 10.1038/s41598-021-87729-3
Evangelista, C., Kraft, P., Dacke, M., Labhart, T., and Srinivasan, M. V. (2014). Honeybee navigation: Critically examining the role of the polarization compass. Philos. Trans. R. Soc., B, Biol. Sci. 369, 20130037. doi: 10.1098/rstb.2013.0037
Farina, W. M., Arenas, A., Díaz, P. C., Martin, C. S., and Barcala, M. C. E. (2020). Learning of a mimic odor within beehives improves pollination service efficiency in a commercial crop. Curr. Biol. 30, 4284–4290. doi: 10.1016/j.cub.2020.08.018
Farjon, G., Krikeb, O., Hillel, A. B., and Alchanatis, V. (2020). Detection and counting of flowers on apple trees for better chemical thinning decisions. Precis. Agric. 21, 503–521. doi: 10.1007/s11119-019-09679-1
Farrar, C. L. (1937). The influence of colony populations on honey production. J. Agric. Res. 54, 945–954.
Fisher, I. I. A., Cogley, T., Ozturk, C., DeGrandi-Hoffman, G., Smith, B. H., Kaftanoglu, O., et al. (2021). The active ingredients of a mitotoxic fungicide negatively affect pollen consumption and worker survival in laboratory-reared honey bees (Apis mellifera). Ecotoxicol. Environ. Saf. 226: 112841. doi: 10.1016/j.ecoenv.2021.112841
Forney, C. F., Javorek, S. K., Jordan, M. A., and Vander Kloet, S. P. (2012). Floral volatile composition of four species of Vaccinium. Botany 90, 365–371. doi: 10.1139/b2012-008
Free, J. B., and Spencer-Booth, Y. (1962). The upper lethal temperatures of honeybees. Entomol. Exp. Appl. 5, 249–254. doi: 10.1111/j.1570-7458.1962.tb00588.x
Free, J. B., and Williams, I. H. (1970). Exposure of the Nasonov gland by honeybees (Apis mellifera) collecting water. Behaviour 37, 286–290. doi: 10.1163/156853970X00385
Fürst, M. A., McMahon, D. P., Osborne, J. L., Paxton, R. J., and Brown, M. J. F. (2014). Disease associations between honeybees and bumblebees as a threat to wild pollinators. Nature 506, 364–366. doi: 10.1038/nature12977
Gaines-Day, H. R., and Gratton, C. (2016). Crop yield is correlated with honey bee hive density but not in high-woodland landscapes. Agric. Ecosyst. Environ. 218, 53–57. doi: 10.1016/j.agee.2015.11.001
Gan, W., Zhang, H., Bostan, N., and DeVetter, L. (2020). Pollen performance differs among cultivars of northern highbush blueberry (Vaccinium corymbosum L.). J. Am. Pomol. Soc. 72, 66–75.
Garbach, K., and Morgan, G. P. (2017). Grower networks support adoption of innovations in pollination management: The roles of social learning, technical learning, and personal experience. J. Environ. Manage. 204, 39–49. doi: 10.1016/j.jenvman.2017.07.077
Garibaldi, L. A., Carvalheiro, L. G., Leonhardt, S. D., Aizen, M. A., Blaauw, B. R., Isaacs, R., et al. (2014). From research to action: Enhancing crop yield through wild pollinators. Front. Ecol. Environ. 12, 439–447. doi: 10.1890/130330
Garibaldi, L. A., Sáez, A., Aizen, M. A., Fijen, T., and Bartomeus, I. (2020). Crop pollination management needs flower-visitor monitoring and target values. J. Appl. Ecol. 57, 664–670. doi: 10.1111/1365-2664.13574
Garibaldi, L. A., Steffan-Dewenter, I., Kremen, C., Morales, J. M., Bommarco, R., Cunningham, S. A., et al. (2011). Stability of pollination services decreases with isolation from natural areas despite honey bee visits. Ecol. Lett. 14,1062–1072. doi: 10.1111/j.1461-0248.2011.01669.x
Garibaldi, L. W., Oddi, F. J., Miguez, F. E., Bartomeus, I., Orr, M. C., Jobbágy, E. G., et al. (2021). Working landscapes need at least 20% native habitat. Conserv. Lett. 14, e12773. doi: 10.1111/conl.12773
Garvey, E. J., and Lyrene, P. M. (1987). Self incompatibility in 19 native blueberry selections. J. Am. Soc. Hortic. Sci. 112, 856–858. doi: 10.21273/JASHS.112.5.856
Geslin, B., Aizen, M. A., Garcia, N., Pereira, A. J., Vaissière, B. E., and Garibaldi, L. A. (2017a). The impact of honey bee colony quality on crop yield and farmers' profit in apples and pears. Agric., Ecosyst. Environ 248, 153–161. doi: 10.1016/j.agee.2017.07.035
Geslin, B., Gauzens, B., Baude, M., Dajoz, I., Fontaine, C., Henry, M., et al. (2017b). Massively introduced managed species and their consequences for plant–pollinator interactions. Adv. Ecol. Res. 57, 147–199. doi: 10.1016/bs.aecr.2016.10.007
Gibbs, J., Elle, E., Bobiwash, K., Haapalainen, T., and Isaacs, R. (2016). Contrasting pollinators and pollination in native and non-native regions of highbush blueberry production. PloS ONE 11, e0158937. doi: 10.1371/journal.pone.0158937
Gibbs, P. E. (2014). Late-acting self-incompatibility—the pariah breeding system in flowering plants. New Phytol. 203, 717–734. doi: 10.1111/nph.12874
Goodrich, B. K. (2017.). The California almond pollination market: contracts and honey bee colony health. (Doctoral dissertation) University of California, Davis (CA), USA.
Goodrich, B. K. (2019). Do more bees imply higher fees? Honey bee colony strength as a determinant of almond pollination fees. Food Policy 83, 150–160. doi: 10.1016/j.foodpol.2018.12.008
Goodrich, B. K., and Goodhue, R. E. (2020). Are all colonies created equal? The role of honey bee colony strength in almond pollination contracts. Ecol. Econom. 177, 106744. doi: 10.1016/j.ecolecon.2020.106744
Goodwin, M. (2012). Pollination of crops in Australia and New Zealand. Australia: Rural Industries Research and Development Corporation.
Goulson, D. (2003). Effects of introduced bees on native ecosystems. Ann. Rev. Ecol. Evol. Syst. 34, 1–26. doi: 10.1146/annurev.ecolsys.34.011802.132355
Goulson, D., Nicholls, E., Botías, C., and Rotheray, E. L. (2015). Bee declines driven by combined stress from parasites, pesticides, and lack of flowers. Science 347, 1255957. doi: 10.1126/science.1255957
Graham, K. K., Gibbs, J., Wilson, J., May, E., and Isaacs, R. (2021a). Resampling of wild bees across fifteen years reveals variable species declines and recoveries after extreme weather. Agric. Ecosyst. Environ. 317, 107470. doi: 10.1016/j.agee.2021.107470
Graham, K. K., Milbrath, M. O., Zhang, Y., et al. (2021b). Identities, concentrations, and sources of pesticide exposure in pollen collected by managed bees during blueberry pollination. Sci. Rep. 11, 16857. doi: 10.1038/s41598-021-96249-z
Grant, K. J., DeVetter, L., and Melathopoulos, A. (2021). Honey bee (Apis mellifera) colony strength and its effects on pollination and yield in highbush blueberries (Vaccinium corymbosum). PeerJ 9, e11634. doi: 10.7717/peerj.11634
Greenleaf, S. S., and Kremen, C. (2006). Wild bees enhance honey bees' pollination of hybrid sunflower. Proc. Natl. Acad. Sci. U.S.A. 103, 13890–13895. doi: 10.1073/pnas.0600929103
Guillaume, P., and Jacquemart, A. L. (1999). Early-inbreeding depression in Vaccinium myrtillus and V. vitis-idaea. Protoplasma 208, 107–114. doi: 10.1007/BF01279080
Gupton, C. L. (1984). Effect of pollen source on fruit characteristics of low-chilling highbush type blueberries. HortScience 19, 531–532. doi: 10.21273/HORTSCI.19.4.531
Gupton, C. L. (1997). Evidence of xenia in blueberry. Acta Hortic. 446, 119–123. doi: 10.17660/ActaHortic.1997.446.16
Gupton, C. L., and Spiers, J. M. (1994). Interspecific and intraspecific pollination effects in rabbiteye and southern highbush blueberry. HortScience 29, 324–326. doi: 10.21273/HORTSCI.29.4.324
Hall, M. A., Jones, J., Rocchetti, M., Wright, D., and Rader, R. (2020). Bee visitation and fruit quality in berries under protected cropping vary along the length of polytunnels. J. Econom. Entomol. 113, 1337–1346. doi: 10.1093/jee/toaa037
Halpern, S. L., Adler, L. S., and Wink, M. (2010). Leaf herbivory and drought stress affect floral attractive and defensive traits in Nicotiana quadrivalvis. Oecologia 163, 961–971. doi: 10.1007/s00442-010-1651-z
Hancock, J. F., and Siefker, J. H. (1982). Levels of inbreeding in highbush blueberry cultivars. HortScience 17, 363–366. doi: 10.21273/HORTSCI.17.3.363
Hanson, E., and Kelsey, L. (2019). Gibberellin use for fruit set on blueberries. Michigan State University Extension. Available online at: https://www.canr.msu.edu/news/gibberellin-use-for-fruit-set-on-blueberries.
Harrison, R. E., Luby, J. J., and Ascher, P. D. (1993). Pollen source affects yield components and reproductive fertility of four half-high blueberry cultivars. J. Am. Soc. Hortic. Sci. 119, 84–89. doi: 10.21273/JASHS.119.1.84
Hellerstein, D., Hitaj, C., Smith, D., and Davis, A. (2017). Land use, land cover, and pollinator health: a review and trend analysis. U.S. Department of Agriculture, Economic Research Service Report Number 232. Available online at: https://www.ers.usda.gov/webdocs/publications/84035/err-232.pdf?v=9807.5.
Hellman, E. W., and Moore, J. N. (1983). Effect of genetic relationship to pollinizer on fruit, seed, and seedling parameters in highbush and rabbiteye blueberries. J. Am. Soc. Hortic. Sci. 108, 401–405. doi: 10.21273/JASHS.108.3.401
Hicks, D., Baude, M., Kratz, C., Ouvrard, P., and Stone, G. (2021). Deep learning object detection to estimate the nectar sugar mass of flowering vegetation. Ecol. Solut. Evid. 2, e12099. doi: 10.1002/2688-8319.12099
Hoffman, G. D., Lande, C., and Rao, S. (2018). A novel pollen transfer mechanism by honey bee foragers on highbush blueberry (Ericales: Ericaceae). Environ. Entomol. 47, 1465–1470. doi: 10.1093/ee/nvy162
Hokanson, K., and Hancock, J. (2000). Early-acting inbreeding depression in three species of Vaccinium (Ericaceae). Sex. Plant Reprod. 13, 145–150. doi: 10.1007/s004970000046
Hokanson, K. E. (1995). The consequences of polyploidy on inbreeding depression in Vaccinium (blueberry) species. PhD thesis (Michigan, USA: Michigan State University).
Howell, G. S., Kilby, M. W., and Nelson, J. W. (1972). Influence of timing of hive introduction on production of highbush blueberries. HortScience 7, 129–131. doi: 10.21273/HORTSCI.7.2.129
Huang, Y. H., Johnson, C. E., Lang, G. A., and Sundberg, M. D. (1997). Pollen sources influence early fruit growth of southern highbush blueberry. J. Am. Soc. Hortic. Sci. 122, 625–629. doi: 10.21273/JASHS.122.5.625
Huber, G. (2016). An investigation of highbush blueberry floral biology and reproductive success in British Columbia. Doctoral dissertation, University of British Columbia, Vancouver, Canada.
Isaacs, R., and Kirk, A. K. (2010). Pollination services provided to small and large highbush blueberry fields by wild and managed bees. J. Appl. Ecol. 47, 841–849. doi: 10.1111/j.1365-2664.2010.01823.x
Isaacs, R., Williams, N., Ellis, J., Pitts-Singer, T. L., Bommarco, R., and Vaughan, M. (2017). Integrated crop pollination: combining strategies to ensure stable and sustainable yields of pollination-dependent crops. Basic Appl. Ecol. 22, 44–60. doi: 10.1016/j.baae.2017.07.003
Jablonski, B., Król, S., Pliszka, K., and Zurowska, Z. (1985). Nectar secretion and pollination of the blueberry (Vaccinium corymbosum L.). Acta Hortic. 165, 133–144. doi: 10.17660/ActaHortic.1985.165.17
Javorek, S. K., Mackenzie, K. E., and Vander Kloet, S. P. (2002). Comparative pollination effectiveness among bees (Hymenoptera: Apoidea) on lowbush blueberry (Ericaceae: Vaccinium angustifolium). Ann. Entomol. Soc. Am. 95, 345–351. doi: 10.1603/0013-8746(2002)095[0345:CPEABH]2.0.CO;2
Jay, S. C. (1986). Spatial management of honey bees on crops. Ann. Rev. Entomol. 31, 49–65. doi: 10.1146/annurev.en.31.010186.000405
Johnson, R. M., Ellis, M. D., Mullin, C. A., and Frazier, M. (2010). Pesticides and honey bee toxicity–USA. Apidologie 41, 312–331. doi: 10.1051/apido/2010018
Jones, J. W., Tsuji, G. Y., Hoogenboom, G., Hunt, L. A., Thornton, P. K., Wilkens, P. W., et al. (1998). “Decision support system for agrotechnology transfer: DSSAT v3,” in Understanding Options for Agricultural Production, eds G. Y. Tsuji, G. Hoogenboom, P. K. Thornton (Springer Netherlands, Dordrecht). p. 157–177. doi: 10.1007/978-94-017-3624-4_8
Jones, V. P., Brunner, J. F., Grove, G. G., Petit, B., Tangren, G. V., and Jones, W. E. (2010). A web-based decision support system to enhance IPM programs in Washington tree fruit. Pest Manag. Sci. 66, 587–595. doi: 10.1002/ps.1913
Jordan, A., Patch, H. M., Grozinger, C. M., and Khanna, V. (2021). Economic dependence and vulnerability of United States agricultural sector on insect-mediated pollination service. Environ. Sci. Technol. 55, 2243–2253. doi: 10.1021/acs.est.0c04786
Kalt, W., Cassidy, A., Howard, L. R., Krikorian, R., Stull, A. J., Tremblay, F., et al. (2020). Recent research on the health benefits of blueberries and their anthocyanins. Adv. Nutr. 11, 224–236. doi: 10.1093/advances/nmz065
Kazokas, W. C. (2003). Self-fruitfulness and parthenocarpy in southern highbush blueberry (Vaccinium corymbosum L. hybrids). PhD thesis (Florida, USA: University of Florida).
Kendall, L. K., Evans, L. J., Gee, M., Smith, T. J., Gagic, V., Lobaton, J. D., et al. (2021). The effect of protective covers on pollinator health and pollination service delivery. Agric. Ecosyst. Environ. 319, 107556. doi: 10.1016/j.agee.2021.107556
Kendall, L. K., Gagic, V., Evans, L. J., Cutting, B. T., Scalzo, J., Hanusch, Y., et al. (2020). Self-compatible blueberry cultivars require fewer floral visits to maximize fruit production than a partially self-incompatible cultivar. J. Appl. Ecol. 57, 2454–2462. doi: 10.1111/1365-2664.13751
King, M. J., and Buchmann, S. L. (2003). Floral sonication by bees: Mesosomal vibration by Bombus and Xylocopa, but not Apis (Hymenoptera: Apidae), ejects pollen from poricidal anthers. J. Kansas Entomol. Soc. 76, 295–305.
Kirk, A. K., and Isaacs, R. (2012). Predicting flower phenology and viability of highbush blueberry. HortScience 47, 1291–1296. doi: 10.21273/HORTSCI.47.9.1291
Klein, A. M., Vaissière, B. E., Cane, J. H., Steffan-Dewenter, I., Cunningham, S. A., Kremen, C., et al. (2007). Importance of pollinators in changing landscapes for world crops. Proc. Royal Soc. B 274, 303–313. doi: 10.1098/rspb.2006.3721
Krebs, S. L., and Hancock, J. F. (1988). The consequences of inbreeding on fertility in Vaccinium corymbosum L. J. Am. Soc. Hortic. Sci. 113, 914–918. doi: 10.21273/JASHS.113.6.914
Krebs, S. L., and Hancock, J. F. (1990). Early-acting inbreeding depression and reproductive success in the highbush blueberry, Vaccinium corymbosum L. Theor. Appl. Genet. 79, 825–832. doi: 10.1007/BF00224252
Krebs, S. L., and Hancock, J. F. (1991). Embryonic genetic load in the highbush blueberry, Vaccinium corymbosum (Ericaceae). Am. J. Bot. 78, 1427–1437. doi: 10.1002/j.1537-2197.1991.tb12609.x
Kumarihami, H. M. P. C., Park, H. G., Kim, S. M., Park, J. I., Lee, E. J., Kim, H. L., et al. (2021). Flower and leaf bud density manipulation affects fruit set, leaf-to-fruit ratio, and yield in southern highbush “Misty” blueberry. Sci. Hortic. 290, 110530. doi: 10.1016/j.scienta.2021.110530
LeCroy, K. A., Savoy-Burke, G., Carr, D. E., Delaney, D. A., and T'ai, H. R. (2020). Decline of six native mason bee species following the arrival of an exotic congener. Sci. Rep. 10, 1–9. doi: 10.1038/s41598-020-75566-9
Leonard, R. J., and Harmon-Threatt, A. N. (2019). Methods for rearing ground-nesting bees under laboratory conditions. Apidologie 50, 689–703. doi: 10.1007/s13592-019-00679-8
Leposavić, A., Đorđević, M., Cerović, R., Radičević, S., Vujović, T., and Đurović, D. (2021). Fertilization biology of ‘Reka' highbush blueberry. Acta Hortic. 1308, 279–284. doi: 10.17660/ActaHortic.2021.1308.39
Li, J., Broussard, M., Tomer, N., Jochym, M., Fonseka, D., Peace, A., et al. (2022). Honey bee (Apis mellifera) hive placement is more influential than orchard layout on the fruit set of a dioecious crop. Ecol. Model. 472, 110074. doi: 10.1016/j.ecolmodel.2022.110074
Lindström, S. A., Herbertsson, L., Rundlöf, M., Bommarco, R., and Smith, H. G. (2016). Experimental evidence that honeybees depress wild insect densities in a flowering crop. Proc. Royal Soc. B 283, 20161641. doi: 10.1098/rspb.2016.1641
Lobos, G. A., Retamales, J. B., Hancock, J. F., Flore, J. A., Romero-Bravo, S., and Del Pozo, A. (2013). Productivity and fruit quality of Vaccinium corymbosum cv. Elliott under photo-selective shading nets. Sci. Hortic. 153, 143–149. doi: 10.1016/j.scienta.2013.02.012
Lonsdorf, E. V., Koh, I., and Ricketts, T. (2020). Partitioning private and external benefits of crop pollination services. People and Nature 2, 811–820. doi: 10.1002/pan3.10138
López-Fernández, L., Lagüela, S., Rodríguez-Gonzálvez, P., Martín-Jiménez, J. A., and González-Aguilera, D. (2018). Close-range photogrammetry and infrared imaging for non-invasive honeybee hive population assessment. ISPRS Int. J. Geo-Inf. 7, 350. doi: 10.3390/ijgi7090350
Lyrene, P. M. (1994). Environmental effects on blueberry flower size and shape are minor. J. Am. Soc. Hortic. Sci. 119, 1043–1045. doi: 10.21273/JASHS.119.5.1043
MacKenzie, K. E. (1997). Pollination requirements of three highbush blueberry (Vaccinium corymbosum L.) cultivars. J. Am. Soc. Hortic. Sci. 122, 891–896. doi: 10.21273/JASHS.122.6.891
Mader, E., Spivak, M., and Evans, E. (2010). Managing Alternative Pollinators. A Handbook for Beekeepers, Growers, and Conservationists. Ithaca, New York: Natural Resource, Agriculture, and Engineering Service (NRAES).
Magrach, A., Champetier, A., Krishnan, S., Boreux, V., and Ghazoul, J. (2019). Uncertainties in the value and opportunity costs of pollination services. J. Appl. Ecol. 56,1549–1559. doi: 10.1111/1365-2664.13399
Mallinger, R., Ternest, J. J., and Naranjo, S. M. (2021). Blueberry yields increase with bee visitation rates, but bee visitation rates are not consistently predicted by colony stocking densities. J. Econom. Entomol. 114, 1441–1451. doi: 10.1093/jee/toab111
Mallinger, R. E., Gaines-Day, H. R., and Gratton, C. (2017). Do managed bees have negative effects on wild bees? A systematic review of the literature. PloS ONE 12, e0189268. doi: 10.1371/journal.pone.0189268
Mallinger, R. E., and Phillips, D. A. (2022). Pollination Best Practices in Southern Highbush Blueberry in Florida. Florida: University of Florida EDIS. p. ENY-172.
Martin, E. A., Dainese, M., Clough, Y., Báldi, A., Bommarco, R., Gagic, V., et al. (2019). The interplay of landscape composition and configuration: new pathways to manage functional biodiversity and agroecosystem services across Europe. Ecol. Lett. 22, 1083–1094. doi: 10.1111/ele.13265
Martin, K., Anderson, B., Minnaar, C., and de Jager, M. (2021). Honey bees are important pollinators of South African blueberries despite their inability to sonicate. S. Afr. J. Bot. 137, 46–51. doi: 10.1016/j.sajb.2020.09.030
Martin, K., Minnaar, C., de Jager, M., and Anderson, B. (2019). Cross-compatibility of five highbush blueberry varieties and ideal crossing combinations. BioRxiv (preprint) 742114. doi: 10.1101/742114
Martins, K. T., Albert, C. H., Lechowicz, M. J., and Gonzalez, A. (2018). Complementary crops and landscape features sustain wild bee communities. Ecol. Appl. 28, 1093–1105. doi: 10.1002/eap.1713
Mayer, D. F. (1994). Sequential introduction of honey bee colonies for pear pollination. Acta Hortic. 367, 267–269. doi: 10.17660/ActaHortic.1994.367.35
Melathopoulos, A. P., Cutler, G. C., and Tyedmers, P. (2015). Where is the value in valuing pollination ecosystem services to agriculture? Ecol. Econom. 109, 59–70. doi: 10.1016/j.ecolecon.2014.11.007
Melathopoulos, A. P., Tyedmers, P., and Cutler, G. C. (2014). Contextualising pollination benefits: effect of insecticide and fungicide use on fruit set and weight from bee pollination in lowbush blueberry. Ann. Appl. Biol. 165, 387–394. doi: 10.1111/aab.12143
Mesihovic, A., Iannacone, R., Firon, N., and Fragkostefanakis, S. (2016). Heat stress regimes for the investigation of pollen thermotolerance in crop plants. Plant Reprod. 26, 93–105. doi: 10.1007/s00497-016-0281-y
Miller, S., Alspach, P., Scalzo, J., and Meekings, J. (2011). Pollination of ‘Hortblue Petite' blueberry: evidence of metaxenia in a new ornamental home-garden cultivar. HortScience 46, 1468–1471. doi: 10.21273/HORTSCI.46.11.1468
Miñarro, M., and García, D. (2021). Complementary contribution of wild bumblebees and managed honeybee to the pollination niche of an introduced blueberry crop. Insects 12, 595. doi: 10.3390/insects12070595
Moore, J. N. (1964). Duration of receptivity to pollination of flowers of the highbush blueberry and the cultivated strawberry. Proc. Am. Soc. Hortic. Sci. 85, 295–301.
Morales, C. L., Sáez, A., Garibaldi, L. A., and Aizen, M. A. (2017). “Disruption of pollination services by invasive pollinator species,” in Impact of Biological Invasions on Ecosystem Services. Invading Nature, eds M. Vilà, P. E. Hulme (Cham, Switzerland: Springer Series in Invasion Ecology). p. 203–220. doi: 10.1007/978-3-319-45121-3_13
Morandin, L. A., and Kremen, C. (2013). Hedgerow restoration promotes pollinator populations and exports native bees to adjacent fields. Ecol. Appl. 23, 829–839. doi: 10.1890/12-1051.1
Morse, R. A., and Calderone, N. W. (2000). The Value of Honeybees as Pollinators of U.S. Crops in 2000. Bee Culture. Medina, OH: The Magazine of American Beekeeping.
Mullin, C. A., Frazier, M., Frazier, J. L., Ashcraft, A., Simonds, R., VanEngelsdorp, D., et al. (2010). High levels of miticides and agrochemicals in North American apiaries: implications for honey bee health. PloS ONE 5, e9754. doi: 10.1371/journal.pone.0009754
Nagasaka, K., Yamane, H., Nishiyama, S., Ebihara, S., Matsuzaki, R., Shoji, M., et al. (2022). Insights into the physiological and molecular mechanisms underlying highbush blueberry fruit growth affected by the pollen source. Hortic. J. 91, 140–151. doi: 10.2503/hortj.UTD-332
Nasr, M. E., Thorp, R. W., Tyler, T. L., and Briggs, D. L. (1990). Estimating honey bee (Hymenoptera: Apidae) colony strength by a simple method: measuring cluster size. J. Econom. Entomol. 83, 748–754. doi: 10.1093/jee/83.3.748
Ne'eman, G., Jürgens, A., Newstrom-Lloyd, L., Potts, S. G., and Dafni, A. (2010). A framework for comparing pollinator performance: Effectiveness and efficiency. Biol. Rev. 85, 435–451. doi: 10.1111/j.1469-185X.2009.00108.x
Nelson, W., Evans, L., Donovan, B., and Howlett, B. (2022). Lasioglossum bees–the forgotten pollinators. J. Apic. Res. 1–8. doi: 10.1080/00218839.2022.2028966
NeSmith, D. S., Krewer, G., Rieger, M., and Mullinix, B. (1995). Gibberellic acid-induced fruit set of rabbiteye blueberry following freeze and physical injury. HortScience 30, 1241–1243. doi: 10.21273/HORTSCI.30.6.1241
Nicholson, C. C., Koh, I., Richardson, L. L., Beauchemin, A., and Ricketts, T. H. (2017). Farm and landscape factors interact to affect the supply of pollination services. Agric. Ecosyst. Environ. 250, 113–122. doi: 10.1016/j.agee.2017.08.030
Nicholson, C. C., and Ricketts, T. H. (2019). Wild pollinators improve production, uniformity, and timing of blueberry crops. Agric. Ecosyst. Environ. 272, 29–37. doi: 10.1016/j.agee.2018.10.018
Nuortila, C., Tuomi, J., Aspi, J., and Laine, K. (2006). Early-acting inbreeding depression in a clonal dwarf shrub, Vaccinium myrtillus, in a northern boreal forest. Ann. Bot. Fennici 43, 36–48.
Osterman, J., Aizen, M. A., Biesmeijer, J. C., Bosch, J., Howlett, B. G., Inouye, D. W., et al. (2021a). Global trends in the number and diversity of managed pollinator species. Agric. Ecosyst. Environ. 322, 107653. doi: 10.1016/j.agee.2021.107653
Osterman, J., Landaverde-González, P., Garratt, M. P. D., Gee, M., Mandelik, Y., Langowska, A., et al. (2021c). On-farm experiences shape farmer knowledge, perceptions of pollinators, and management practices. Glob. Ecol. Conserv. 32, e01949. doi: 10.1016/j.gecco.2021.e01949
Osterman, J., Theodorou, P., Radzevičiut,e, R., Schnitker, P., and Paxton, R. J. (2021b). Apple pollination is ensured by wild bees when honey bees are drawn away from orchards by a mass co-flowering crop, oilseed rape. Agric. Ecosyst. Environ. 315, 107383. doi: 10.1016/j.agee.2021.107383
Ovinge, L. P., and Hoover, S. E. (2018). Comparison of honey bee (Hymenoptera: Apidae) colony units of different sizes as pollinators of hybrid seed canola. J. Econom. Entomol. 111, 1535–1541. doi: 10.1093/jee/toy155
Palmer, R. G., Perez, P. T., Ortiz-Perez, E., Maalouf, F., and Suso, M. J. (2009). The role of crop-pollinator relationships in breeding for pollinator-friendly legumes: from a breeding perspective. Euphytica 170, 35–52. doi: 10.1007/s10681-009-9953-0
Pankiw, T., Page Jr, R. E., and Fondrk, M. K. (1998). Brood pheromone stimulates pollen foraging in honey bees (Apis mellifera). Behav. Ecol. Sociobiol. 44, 193–198. doi: 10.1007/s002650050531
Pankiw, T., Winston, M. L., and Slessor, K. N. (1994). Variation in worker response to honey bee (Apis mellifera L.) queen mandibular pheromone (Hymenoptera: Apidae). J. Insect Behav. 7, 1–15. doi: 10.1007/BF01989823
Pinilla-Gallego, M. S., Rowe, L. M., Gibbs, J., Pitts-Singer, T. L., and Isaacs, R. (2022). Improving Osmia lignaria and O. cornifrons (Hymenoptera: Megachilidae) retention with preferred nest materials and attractant spray. J. Appl. Entomol. 146, 743–752. doi: 10.1111/jen.13001
Polashock, J. J., Caruso, F. L., Averill, A. L., Schilder, A., and Press, A. P. S. (2017). Compendium of Blueberry, Cranberry, and Lingonberry Diseases and Pests. St. Paul, MN: American Phytopathological Society. doi: 10.1094/9780890545386
Potts, S. G., Biesmeijer, J. C., Kremen, C., Neumann, P., Schweiger, O., and Kunin, W. E. (2010). Global pollinator declines: trends, impacts and drivers. Trends Ecol. Evol. 25, 345–353. doi: 10.1016/j.tree.2010.01.007
Prasifka, J. R., Mallinger, R. E., Portlas, Z. M., Hulke, B. S., Fugate, K. K., Paradis, T., et al. (2018). Using nectar-related traits to enhance crop-pollinator interactions. Front. Plant Sci. 9, 812. doi: 10.3389/fpls.2018.00812
Qu, H., Xiang, R., Obsie, E. Y., Wei, D., and Drummond, F. (2021). Parameterization and calibration of wild blueberry machine learning models to predict fruit-set in the northeast China bog blueberry agroecosystem. Agronomy 11, 1736. doi: 10.3390/agronomy11091736
Quinet, M., Warzée, M., Vanderplanck, M., Michez, D., Lognay, G., and Jacquemart, A. L. (2016). Do floral resources influence pollination rates and subsequent fruit set in pear (Pyrus communis L.) and apple (Malus x domestica Borkh) cultivars?. Eur. J. Agron. 77, 59–69. doi: 10.1016/j.eja.2016.04.001
Rasp,é, O., Guillaume, P., and Jacquemart, A. L. (2004). Inbreeding depression and biased paternity after mixed-pollination in Vaccinium myrtillus L. (Ericaceae). Int. J. Plant Sci. 165, 765–771. doi: 10.1086/422045
Reilly, J. R., Artz, D. R., Biddinger, D., Bobiwash, K., Boyle, N. K., Brittain, C., et al. (2020). Crop production in the USA is frequently limited by a lack of pollinators. Proc. Royal Soc. B. 287, 20200922. doi: 10.1098/rspb.2020.0922
Rering, C. C., Franco, J. G., Yeater, K. M., and Mallinger, R. E. (2020). Drought stress alters floral volatiles and reduces floral rewards, pollinator activity, and seed set in a global plant. Ecosphere 11, e03254. doi: 10.1002/ecs2.3254
Retamales, J. B., Mena, C., Lobos, G., and Morales, Y. (2015). A regression analysis on factors affecting yield of highbush blueberries. Sci. Hortic. 186, 7–14. doi: 10.1016/j.scienta.2015.02.003
Retamales, R., and Hancock, J. F. (2018). Blueberries, 2nd Edition, Vol 27. CABI: Crop Production Science in Horticulture. p. 423.
Retamal-Salgado, J., Bastías, R. M., Wilckens, R., and Paulino, L. (2015). Influence of microclimatic conditions under high tunnels on the physiological and productive responses in blueberry'O'Neal'. Chil. J. Agric. Res. 75, 291–297. doi: 10.4067/S0718-58392015000400004
Ritzinger, R., and Lyrene, P. M. (1999). Flower morphology in blueberry species and hybrids. HortScience 34, 130–131. doi: 10.21273/HORTSCI.34.1.130
Rodet, G., and Henry, M. (2014). Analytic partitioning of honeybee (Apis mellifera L.) flight activity at nest entrance: adaptation and behavioural inertia in a changing environment. Ecol. Res. 29, 1043–1051. doi: 10.1007/s11284-014-1191-9
Rodger, J. G., Bennett, J. M., Razanajatovo, M., Knight, T. M., van Kleunen, M., Ashman, T. L., et al. (2021). Widespread vulnerability of flowering plant seed production to pollinator declines. Sci. Adv. 7, eabd3524. doi: 10.1126/sciadv.abd3524
Rodriguez-Saona, C., Parra, L., Quiroz, A., and Isaacs, R. (2011). Variation in highbush blueberry floral volatile profiles as a function of pollination status, cultivar, time of day and flower part: implications for flower visitation by bees. Ann. Bot. 107, 1377–1390. doi: 10.1093/aob/mcr077
Rodriguez-Saona, C., Vincent, C., and Isaacs, R. (2019). Blueberry IPM: past successes and future challenges. Ann. Rev. Entomol. 64, 95–114. doi: 10.1146/annurev-ento-011118-112147
Rogers, S. R., Tarpy, D. R., and Burrack, H. J. (2013). Multiple criteria for evaluating pollinator performance in highbush blueberry (Ericales: Ericaceae) agroecosystems. Environ. Entomol. 42, 1201–1209. doi: 10.1603/EN12303
Rogers, S. R., Tarpy, D. R., and Burrack, H. J. (2014). Bee species diversity enhances productivity and stability in a perennial crop. PloS ONE 9, e97307. doi: 10.1371/journal.pone.0097307
Rollin, O., and Garibaldi, L. A. (2019). Impacts of honeybee density on crop yield: a meta-analysis. J. App. Ecol. 56, 1152–1163. doi: 10.1111/1365-2664.13355
Rucker, R. R., Thurman, W. N., and Burgett, M. (2012). Honey bee pollination markets and the internalization of reciprocal benefits. Am. J. Agric. Econom. 94, 956–977. doi: 10.1093/ajae/aas031
Russo, L. (2016). Positive and negative impacts of non-native bee species around the world. Insects 7, 69. doi: 10.3390/insects7040069
Russo, L., de Keyzer, C. W., Harmon-Threatt, A. N., LeCroy, K. A., and MacIvor, J. S. (2021). The managed-to-invasive species continuum in social and solitary bees and impacts on native bee conservation. Curr. Opin. Insect Sci. 46, 43–49. doi: 10.1016/j.cois.2021.01.001
Sagili, R. R., and Burgett, D. M. (2011). “Evaluating honey bee colonies for pollination: a guide for commercial growers and beekeepers,” in: Pacific Northwest Extension publication PNW 623 (Corvallis (OR), USA: Oregon State University).
Samietz, J., Graf, B., Höhn, H., Schaub, L., and Höpli, H. U. (2007). Phenology modelling of major insect pests in fruit orchards from biological basics to decision support: the forecasting tool SOPRA. EPPO Bull. 37, 255–260. doi: 10.1111/j.1365-2338.2007.01121.x
Sampson, B. J., Danka, R. G., and Stringer, S. J. (2004). Nectar robbery by bees Xylocopa virginica and Apis mellifera contributes to the pollination of rabbiteye blueberry. J. Econ. Entomol. 97, 735–740. doi: 10.1603/0022-0493(2004)097[0735:NRBBXV]2.0.CO;2
Sampson, B. J., Stringer, S. J., and Marshall, D. A. (2013). Blueberry floral attributes and their effect on the pollination efficiency of an oligolectic bee, Osmia ribifloris Cockerell (Megachilidae: Apoidea). HortScience 48, 136–142. doi: 10.21273/HORTSCI.48.2.136
Sánchez-Bayo, F., Goulson, D., Pennacchio, F., Nazzi, F., Goka, K., and Desneux, N. (2016). Are bee diseases linked to pesticides?—a brief review. Environ. Int. 89, 7–11. doi: 10.1016/j.envint.2016.01.009
Schmickl, T., and Crailsheim, K. (2007). HoPoMo: A model of honeybee intracolonial population dynamics and resource management. Ecol. Modell. 204, 219–245. doi: 10.1016/j.ecolmodel.2007.01.001
Schott, G. W. (2000). The nature of infertility and response to inbreeding in Vaccinium (blueberry) species (Philosophical Dissertation). Michigan State University.
Schultheis, J. R., Ambrose, J. T., Bambara, S. B., and Mangum, W. A. (1994). Selective bee attractants did not improve cucumber and watermelon yield. HortScience 29, 155–158. doi: 10.21273/HORTSCI.29.3.155
Seabra, S. G., Silva, S. E., Nunes, V. L., Sousa, V. C., Martins, J., Marabuto, E., et al. (2019). Genomic signatures of introgression between commercial and native bumblebees, Bombus terrestris, in western Iberian Peninsula—implications for conservation and trade regulation. Evol. Appl. 12, 679–691. doi: 10.1111/eva.12732
Seppelt, R., Dormann, C. F., Eppink, F. V., Lautenbach, S., and Schmidt, S. (2011). A quantitative review of ecosystem service studies: approaches, shortcomings and the road ahead. J. Appl. Ecol. 48, 630–636. doi: 10.1111/j.1365-2664.2010.01952.x
Shafir, S. (2011). “Bee cognition and crop pollination: proven and potential applications,” in: All Flesh Is Grass, eds J. Seckbach, Z. Dubinsky (Dordrecht: Springer). p. 183–198. doi: 10.1007/978-90-481-9316-5_8
Shahak, Y., Ratner, K., Zur, N., Offir, Y., Matan, E., Yehezkel, H., et al. (2008). “Photoselective netting: an emerging approach in protected agriculture,” in International Symposium on Strategies Towards Sustainability of Protected Cultivation in Mild Winter Climate 807. p. 79–84. doi: 10.17660/ActaHortic.2009.807.7
Shaw, F. R., Bailey, J. S., and Bourne, A. I. (1939). The comparative value of honeybees in the pollination of cultivated blueberries. J. Econom. Entomol. 32, 872–874. doi: 10.1093/jee/32.6.872
Shaw, J. A., Nugent, P. W., Johnson, J., Bromenshenk, J. J., Henderson, C. B., and Debnam, S. (2011). Long-wave infrared imaging for non-invasive beehive population assessment. Opt. Express 19, 399–408. doi: 10.1364/OE.19.000399
Siviter, H., Bailes, E. J., Martin, C. D., Oliver, T. R., Koricheva, J., Leadbeater, E., et al. (2021). Agrochemicals interact synergistically to increase bee mortality. Nature 596, 389–392. doi: 10.1038/s41586-021-03787-7
Stout, J. C., and Goulson, D. (2001). The use of conspecific and interspecific scent marks by foraging bumblebees and honeybees. Anim. Behav. 62, 183–189. doi: 10.1006/anbe.2001.1729
Straw, E. A., Thompson, L. J., Leadbeater, E., and Brown, M. J. (2022). ‘Inert' ingredients are understudied, potentially dangerous to bees and deserve more research attention. Proc. Royal Soc. B 289, p. 20212353. doi: 10.1098/rspb.2021.2353
Stubbs, C. S., and Drummond, F. A. (2001). Bombus impatiens (Hymenoptera: Apidae): An alternative to Apis mellifera (Hymenoptera: Apidae) for lowbush blueberry pollination. J. Econom. Entomol. 94, 609–616. doi: 10.1603/0022-0493-94.3.609
Stubbs, C. S., Drummond, F. A., and Osgood, E. A. (1994). Osmia ribifloris biedermannii and Megachile rotundata (Hymenoptera: Megachilidae) introduced into the lowbush blueberry agroecosystem in Maine. J. Kansas Entomol. Soc. 67, 173–185.
Sun, Q., Zhao, X., Wu, L., Zhao, J., Yang, Y., and Zhang, Y. (2021). Differences in pollination efficiency among three bee species in a greenhouse and their effects on yield and fruit quality of northern highbush “Bluecrop” blueberry. HortScience 56, 603–607. doi: 10.21273/HORTSCI15714-21
Taber, S. K., and Olmstead, J. W. (2016). Impact of cross-and self-pollination on fruit set, fruit size, seed number, and harvest timing among 13 southern highbush blueberry cultivars. HortTechnology 26, 213–219. doi: 10.21273/HORTTECH.26.2.213
Tamburini, G., Bommarco, R., Kleijn, D., van der Putten, W. H., and Marini, L. (2019). Pollination contribution to crop yield is often context-dependent: a review of experimental evidence. Agric. Ecosyst. Environ. 280, 16–23. doi: 10.1016/j.agee.2019.04.022
Timberlake, T. P., Vaughan, I. P., and Memmott, J. (2019). Phenology of farmland floral resources reveals seasonal gaps in nectar availability for bumblebees. J. Appl. Ecol. 56, 1585–1596. doi: 10.1111/1365-2664.13403
Tucker, S. K., Ginsberg, H. S., and Alm, S. R. (2019). Effect of corolla slitting and nectar robbery by the eastern carpenter bee (Hymenoptera: Apidae) on fruit quality of Vaccinium corymbosum L. (Ericales: Ericaceae). Environ. Entomol. 48, 718–726. doi: 10.1093/ee/nvz055
Tuell, J. K., and Isaacs, R. (2009). Elevated pan traps to monitor bees in flowering crop canopies. Entomol. Exp. Appl. 131, 93–98. doi: 10.1111/j.1570-7458.2009.00826.x
Tuell, J. K., and Isaacs, R. (2010a). Weather during bloom affects pollination and yield of highbush blueberry. J. Econom. Entomol. 103, 557–562. doi: 10.1603/EC09387
Tuell, J. K., and Isaacs, R. (2010b). Community and species-specific responses of wild bees to insect pest control programs applied to a pollinator-dependent crop. J. Econom. Entomol. 103, 668–675. doi: 10.1603/EC09314
Twiston-Davies, G., Becher, M. A., and Osborne, J. L. (2021). BEE-STEWARD: a research and decision-support software for effective land management to promote bumblebee populations. Methods Ecol. Evol. 12, 1809–1815. doi: 10.1111/2041-210X.13673
Vaudo, A. D., Patch, H. M., Mortensen, D. A., Tooker, J. F., and Grozinger, C. M. (2016). Macronutrient ratios in pollen shape bumble bee (Bombus impatiens) foraging strategies and floral preferences. Proc. Natl. Acad. Sci. U.S.A. 113, E4035–E4042. doi: 10.1073/pnas.1606101113
Vaudo, A. D., Tooker, J. F., Patch, H. M., Biddinger, D. J., Coccia, M., Crone, M. K., et al. (2020). Pollen protein: lipid macronutrient ratios may guide broad patterns of bee species floral preferences. Insects 11, 132. doi: 10.3390/insects11020132
Vieli, L., Davis, F. W., Kendall, B. E., and Altieri, M. (2016). Landscape effects on wild Bombus terrestris (Hymenoptera: Apidae) queens visiting highbush blueberry fields in south-central Chile. Apidologie 47, 711–716. doi: 10.1007/s13592-015-0422-6
Wernecke, A., Eckert, J. H., Forster, R., Kurlemann, N., and Odemer, R. (2022). Inert agricultural spray adjuvants may increase the adverse effects of selected insecticides on honey bees (Apis mellifera L.) under laboratory conditions. J. Plant Dis. Prot. 129, 93–105. doi: 10.1007/s41348-021-00541-z
White, E., and Clark, J. H. (1939). Some results of self-pollination of the highbush blueberry at Whitesbog, New Jersey. Proc. Am. Soc. Hortic. Sci. 36, 305–309.
Williamson, J., Adams, C. G., Isaacs, R., and Gut, L. J. (2018). Evaluation of Nasonov pheromone dispensers for pollinator attraction in apple, blueberry, and cherry. J. Econom. Entomol. 111, 1658–1663. doi: 10.1093/jee/toy107
Williamson, J. G., Darnell, R. L., Krewer, G., Vanenvegen, J., and NeSmith, S. (1996). Gibberellic acid: a management tool for increasing yield of rabbiteye blueberry. J. Small Fruit Vitic. 3, 203–218. doi: 10.1300/J065v03n04_05
Windsor, F. M., Tavella, J., Rother, D. C., Raimundo, R. L. G., Devoto, M., Guimarães, P. R., et al. (2021). Identifying plant mixes for multiple ecosystem service provision in agricultural systems using ecological networks. J. Appl. Ecol. 1–13. doi: 10.1111/1365-2664.14007
Wittwer, S. H., and Castilla, N. (1995). Protected cultivation of horticultural crops worldwide. HortTechnology 5, 6–23. doi: 10.21273/HORTTECH.5.1.6
Yang, Q., Liu, E., Fu, Y., Yuan, F., Zhang, T., and Peng, S. (2019). High temperatures during flowering reduce fruit set in Rabbiteye blueberry. J. Am. Soc. Hortic. Sci. 144, 339–351. doi: 10.21273/JASHS04650-19
Young, M. J., and Sherman, W. B. (1978). Duration of pistil receptivity, fruit set, and seed production in rabbiteye and tetraploid blueberries. HortScience 13, 278–279. doi: 10.21273/HORTSCI.13.3.278
Keywords: Vaccinium, Integrated Crop Pollination, bees, sustainability, pollinator, crop yield, Apis mellifera, ecosystem service
Citation: DeVetter LW, Chabert S, Milbrath MO, Mallinger RE, Walters J, Isaacs R, Galinato SP, Kogan C, Brouwer K, Melathopoulos A and Eeraerts M (2022) Toward evidence-based decision support systems to optimize pollination and yields in highbush blueberry. Front. Sustain. Food Syst. 6:1006201. doi: 10.3389/fsufs.2022.1006201
Received: 29 July 2022; Accepted: 03 October 2022;
Published: 21 October 2022.
Edited by:
Giuseppina Rea, National Research Council (CNR), ItalyReviewed by:
Cesar Rodriguez-Saona, Rutgers, The State University of New Jersey, United StatesAlistair John Campbell, Embrapa Amazônia Oriental, Brazil
Copyright © 2022 DeVetter, Chabert, Milbrath, Mallinger, Walters, Isaacs, Galinato, Kogan, Brouwer, Melathopoulos and Eeraerts. This is an open-access article distributed under the terms of the Creative Commons Attribution License (CC BY). The use, distribution or reproduction in other forums is permitted, provided the original author(s) and the copyright owner(s) are credited and that the original publication in this journal is cited, in accordance with accepted academic practice. No use, distribution or reproduction is permitted which does not comply with these terms.
*Correspondence: Lisa W. DeVetter, bGlzYS5kZXZldHRlciYjeDAwMDQwO3dzdS5lZHU=; Meghan O. Milbrath, bWlsYnJhdDImI3gwMDA0MDttc3UuZWR1