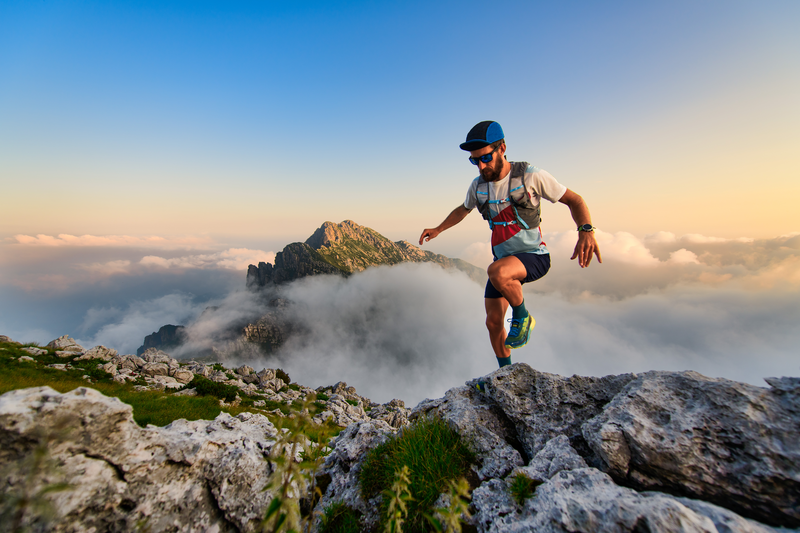
94% of researchers rate our articles as excellent or good
Learn more about the work of our research integrity team to safeguard the quality of each article we publish.
Find out more
ORIGINAL RESEARCH article
Front. Sustain. Food Syst. , 18 October 2022
Sec. Agroecology and Ecosystem Services
Volume 6 - 2022 | https://doi.org/10.3389/fsufs.2022.1003637
Conservation and organic agriculture are two alternative crop management strategies associated with environmental impact reduction, leading theoretically to more biodiversity and higher ecological functioning, underpinning better ecosystem service delivery. The combination of these two farming strategies is increasingly seen as an opportunity to mitigate shortcomings of each of them taken individually. However, combining organic and conservation agriculture is undeniably challenging, since it leaves no curative option (neither synthetic pesticides nor soil plowing) for pest control (phytophagous, pathogens, and weeds). Hence, the latter must be ensured by effective restoration of predatory communities. The present research investigated the potential of combining organic and conservation agriculture to support two major ground-dwelling natural enemy communities: carabids and spiders. We used pitfall traps to sample these two communities in paired adjacent cereal fields conducted under organic-conservation (OC) and conventional (CV) agriculture in Belgium. Community assemblages were significantly different across system types, both in terms of species diversity and functional trait diversity. OC parcels showed higher alpha and beta species diversities for both carabids and spiders and species composition differed between OC and CV parcels. OC systems were associated with higher variation of species assemblages among parcels than CV systems Functional traits also differed across system types for both groups. We found more herbivorous and granivore carabids in OC parcels, and more predator species in CV parcels. We found larger spiders, more hunting spiders and more wetland spiders in OC parcels, whereas we found more web weavers and more forest spiders in CV ones. Functional diversity was higher in OC parcels for carabids, but not for spiders. In brief, OC parcels supported more diverse communities, both taxonomically and functionally. These outcomes show that OC systems are promising systems to support these natural enemy communities. Further studies should assess whether combining organic and conservation agricultural enables sufficient pest control levels to achieve high levels of food production and farmers income.
The role of agriculture in biodiversity loss has been acknowledged for a long time (McLaughlin and Mineau, 1995), and remains a central issue in recent assessments (Dudley and Alexander, 2017; IPBES, 2019). Agriculture covers over a third of the world's ice-free land surface, i.e., most of the land suitable for food production (Foley, 2011). Its pressure on biodiversity is high and expected to increase in a business-as-usual scenario in order to feed an ever-growing human population (Tilman and Clark, 2014; Gordon et al., 2017). Therefore, it is urgent to develop a biodiversity-friendly and productive agriculture.
Among alternative agricultural systems claiming to offer such synergies, organic agriculture (absence of chemical inputs) and conservation agriculture (minimal soil disturbance, permanent soil cover, and diversified crop rotation) are among the most promising. Although these two agricultural systems are promoted by distinct professional communities (Fleury et al., 2014), they are increasingly considered conjointly since the early 2000s (Casagrande et al., 2016; Peigné et al., 2016). Indeed, organic farming does not always imply improved biodiversity (Tscharntke et al., 2021), soil quality or resilience (Darnhofer et al., 2010; Leifeld, 2012; Peigné et al., 2016). On the other hand, conservation agriculture mainly focuses on soil health but relies on chemicals to control pests and weeds (Kirkegaard et al., 2014). Hence, the combination of organic and conservation agriculture is increasingly seen as an opportunity to mitigate shortcomings of either approaches.
However, combining organic and conservation agriculture is challenging because it leaves no curative option (neither synthetic pesticides nor soil plowing) to control pests. “Pest” includes a broad spectrum of agents putting pressure on crops, either directly through consumption (e.g., slugs, aphids) or diseases (e.g., fungi pathogens), or indirectly through competition (e.g., weeds). In conventional agriculture, pests are controlled thanks to synthetic pesticides (e.g., insecticides and herbicides) or soil mechanical tillage for weeds. Both of these options have well known negative environmental (Tilman et al., 2011), health (Mostafalou and Abdollahi, 2017) and economic effects (Wilson and Tisdell, 2001). Maintaining high levels of food production without these curative options requires promoting biodiversity that effectively regulates pests, following the “agroecological crop protection approach” (Deguine et al., 2017). Such useful biodiversity is referred to as “functional agrobiodiversity” (ELN FAB, 2012). It provides multiple ecosystem services benefiting agriculture itself, rendering it more autonomous with regards to external chemical or mechanical interventions, hence more resilient to global (e.g., market or climatic) changes (Altieri, 1999; Hatt et al., 2016).
Among functional agrobiodiversity groups that potentially support pest regulation service are two major ground-dwelling natural enemy communities: Carabidae (carabids, ground beetles) and Araneae (spiders). Carabidae is a well-known insect Family as they are rather sensitive to habitat alteration and have thus regularly been studied as “bioindicators” (Rainio and Niemela, 2003; Kotze et al., 2011). Because of their high abundance and diverse feeding behaviors (carnivores, granivorous, and omnivorous), they are considered to play an important role in natural pest regulation processes (Kromp, 1999; Winder et al., 2005; Bohan et al., 2011; Kulkarni et al., 2015). Spiders are also abundant arthropods, with mostly insectivorous diet, and therefore considered as important predators in agroecosystems (Nyffeler and Sunderland, 2003; Michalko et al., 2019).
To date, knowledge on the effect of combining organic and conservation (OC) approaches on these natural enemies remains sparse. Indeed, most research has focused either on organic agriculture or reduced tillage practices separately. Organic agriculture is one of the most studied type of alternative agriculture, and its effects on biodiversity have been widely documented. Overall, organic agriculture has a positive effect on the density and diversity of carabids and spiders (Pfiffner and Niggli, 1995; Feber et al., 1998; Kromp, 1999; Bengtsson et al., 2005). Plowing seems to have a negative effect on these two groups, thus arguing in favor of reduced tillage practices recommended in conservation agriculture (Kromp, 1999; Sunderland and Samu, 2000; Tamburini et al., 2016). Rivers et al. (2017, 2018) explored the potential effects of OC systems on carabids by studying these communities in no-tillage parcels undergoing transition toward organic farming. They observed higher density and diversity in arthropod species over time, indicating an added value of OC agriculture with regards to conservation agriculture alone. To our knowledge, there is no other study analyzing the potential of OC systems to restore functional agrobiodiversity.
This study tackles this knowledge gap by assessing the effects of combining organic with conservation agriculture on carabids and spiders, two groups of ground dwelling natural enemies potentially playing important roles in pest regulation. We studied cereal OC farms located in the western part of the Hainaut Province in Belgium. We sampled carabids and spiders in parcels of these OC farms and in paired adjacent conventional (CV) parcels. We then tested the hypothesis that OC parcels support higher taxonomic and functional diversity in comparison with CV parcels. Indeed, without pesticides and plowing, OC parcels have the potential to offer more resources and support more diverse communities. For instance, reduced tillage is associated with higher amounts of residues on the soil surface, thereby increasing the abundance of saprophytic and detritus feeding species, upon which many predator arthropods feed (Holland and Reynolds, 2003) and it also enhances niche availability for non-cropped plants, thus favoring granivorous insects feeding on their seeds (Navntoft et al., 2009; Trichard et al., 2014; Petit et al., 2017). Moreover, we tested the hypothesis that OC parcels generate stronger variation of species assemblages than among CV parcels. Indeed, in OC parcels, the absence of strongly constraining practices allows greater expression of local conditions (soil, micro-climate, etc.) hence supporting more variety among communities to be expressed.
The study was conducted in the western part of the Hainaut province in Belgium (Figure 1). The climate is oceanic temperate, with annual rainfall around 800 mm/year and average temperatures around 10°C. We selected three farms that are pioneer agroecological farms in Belgium, that had been combining organic and conservation agriculture for 8 years at the time of sampling (2016). They are certified organic, meaning they make no use of phytosanitary products to control pests and only use organic fertilizers; and apply principles of conservation agriculture, meaning they reduce tillage or seed directly, grow cereals in intercropping (triticale, oats, rye, spelt, pea, and vetch) and/or implement winter covers.
Figure 1. Maps of the study site within Belgium (red dot), the three locations (A, B, C), conventional parcels (light gray) and organic-conservation parcels (black).
We studied all cereal parcels within these three farms. Each OC cereal parcel was then “paired” with a “reference” CV winter wheat parcel in the vicinity. Practices in CV parcels were representative of practices in conventional Walloon farms: mineral fertilizers and synthetic weed and pest controls applications as well as regular soil plowing. Phytosanitary products applied during sampling were mostly herbicides, fungicides, growth regulators and to a lesser extent (3 parcels out of 15) insecticides. In total, we studied 15 OC parcels and 15 CV parcels spread across three locations centered on each of the three OC farms (referred to as locations A, B, and C, Figure 1). This pairing approach avoids environmental parameters to bias results and interpretations, i.e., within a pair, the CV and OC parcel share similar pedo-ecological parameters. All farms were integrated crop-livestock systems, and all cereals were harvested for fodder purposes. Further details on OC and CV practices are provided in Tables 1, 2, respectively, in Boeraeve et al. (2020).
Within each parcel, we placed four pitfall traps on the ground to capture ground-dwelling arthropods. Pitfall traps (or Barber traps) consisted of two nested containers (dimensions: 400 mL, h = 100 mm, top/inf diameter = 85/72 mm), the outer container serving as support for the second one allowing to harvest containers limiting disturbances of the substrate around the trap. Each unit was filled with 200 mL of white vinegar, a conservative solution, not attractive to the insects and without surface tension, thus limiting the risk of individuals escaping. A 25 mm wire grid was placed above each trap to avoid capturing micro-vertebrates. The four traps were placed in the four corner of a 10 m-square and at least 20 m apart from field borders. Traps were left open during 7 days, and then recapped empty for 15 days before the next session. In total, three sessions were carried out between 28th of May and 17th of July 2016. All captured insects were stored in the refrigerator before sorting, after which collected individuals were stored in alcohol (96°) for further identification with official identification keys (Jeannel, 1941, 1942; Nentwig et al., 2022) and using Fauna Europaea (https://fauna-eu.org) as a taxonomy standard.
All analyses were computed in R version 4.1.2 (R Core Team, 2021). All multivariate analyses [namely Principal Component Analyses (PCA), Principal Coordinate Analyses (PCOA), distance matrices and Redundancy Analyses (RDA)], Constrained analysis of Principal Coordinates (CAP), were performed using the package “vegan” (Oksanen, 2018). Normality was checked and, when necessary, log- or square-based transformations were applied to meet the normality assumption.
First, species diversity was investigated through its alpha and beta components. We computed the following alpha diversity indices with the package “diverse” (Guevara et al., 2016) for each parcel at each sampling time: abundance, species richness (number of species), Shannon–entropy, Berger-Parker, Simpson and Rao Stirling. We then ran linear mixed-effect models using “lme4” (Bates and Maechler, 2018) and “car” (Fox et al., 2018) packages to determine whether system type (OC vs. CV) influenced these indices. System type was considered as fixed effect while the location (A, B, or C) and pairs (each OC parcel was paired to an analogous CV parcel) were considered as random effects. Pairs were nested within location, since pairs of parcels changed across locations.
Second, we analyzed beta species diversity between OC and CV parcels using Bray-Curtis dissimilarity matrices and PCOA to visualize these dissimilarities. We then computed turnover and nestedness distance matrices with the betapart package (Baselga and Orme, 2012) to assess the nestedness (i.e., species loss across parcels) and turnover (i.e., species replacement). We then constrained these distance matrices by system types by means of a CAP on which we carried out ANOVA to test for differences between systems.
Next, we identified species that were most strongly associated with each system type using IndVal values (Dufrêne and Legendre, 1997). We quantified the fidelity (i.e., probability of finding the species in sites belonging to the targeted system type) and the specificity (i.e., probability of being in the targeted system type when the species is found) of each species for each system type using “indicspecies” package (Caceres and Legendre, 2009). We only considered species for which the P-value of the associated permutation test was below 0.05.
Last, to assess how system type affects functional diversity, we extracted data of life history trait from the literature for carabids (Desender, 1986; BETSI, 2014; Homburg et al., 2014) and spiders (Uetz et al., 1999; Gloor et al., 2010; BETSI, 2014). For carabids, we considered body size, diets (granivore, omnivore, herbivore, predator) and wing system (di/polymorphic, brachypterous, macropterous). For spiders, we considered, body size, habitat preference (agricultural land, forests and semi-natural habitats, wetlands, caves, artificial lands) and foraging mode (web weaver or active hunter). We selected these traits because they are linked with pest regulation processes (Purtauf et al., 2005a; Venn, 2007; Rouabah et al., 2014; Rusch et al., 2015). We used data on these traits to compute the function “dbFD” within the package “FD” to compute multidimensional functional diversity indices (Laliberté et al., 2015). We computed functional richness (volume of the functional space occupied by the community), functional evenness (regularity of the distribution of abundance in this volume), functional divergence (divergence in the distribution of abundance in this volume), functional dispersion and the community weighted mean (CWM—the average of trait values in the community weighted by the relative abundance of the species carrying each value). For each trait CWM and each functional diversity index, we ran mixed linear models, using the same model structure as for species diversity indices, to determine whether there were significant differences between system types. To illustrate variations in trait CWM and functional diversity values across parcels and system types we conducted a PCA. To assess potential differences in diversity indices between system types, we conducted a RDA, followed by an ANOVA on scores along RDA axes.
Our study aims at investigating how combining organic and conservation agriculture can restore species and functional diversity among two groups of ground dwelling natural enemies: carabids and spiders. Based on field sampling of these two communities in OC and CV parcels, this section first presents how system type affects species diversity by investigating alpha (Section How does OC farming impact alpha diversity?) and beta (Section How does OC farming impact Beta diversity?) diversities as well as indicator species (Section Which species are associated with each system?). In a second step, functional diversity is examined (Section How does OC farming impact traits distribution?) by analyzing how traits and their diversities differ between system types. Data on the mean and standard deviation of each index is provided in Supplementary material 1.
Our field sampling gathered a total of 9,339 individuals carabids and 74 species. Most abundant species were Loricera pilicornis Fabricius, 1775 (2,449 individuals), Bembidion lampros/properans (1,543) and Pterostichus melanarius Illiger, 1798 (1,052). For spiders, 46 species were trapped representing 2,483 individuals. Most abundant spider species were Erigone atra Oedothorax sp. Bertkau, 1883 (602) and Pardosa proxima L. Koch, 1882 (222).
Although we detected no difference in abundance and species richness between system types, both carabids and spiders communities differed in terms of other diversity indices (Figure 2). OC parcels hosted more equitable distributions across species (in terms of Simpson, Berger-Parker and Rao-Stirling indices for spiders and in terms of Shannon and Simpson indices for carabids). Higher Shannon or smaller Simpson and Berger-Parker indicate higher diversity because more equitable distributions of abundances across species, with Shannon giving more weight to rare species and Simpson giving more weight to abundant ones.
Figure 2. Multiple boxplots for six diversity indices: abundance, species richness, Shannon, Simpson, Berger-Parker and Rao-Stirling indices for carabids and spiders in conventional (CV) and organic-conservation (OC) parcels. Results of χ2 tests carried out on the mixed linear models (χ2 and P-values) of testing each diversity indicator between system types are indicated on the top of each boxplot. Asterisks illustrate the power of the levels of significance of the statistical test: “*” ≤ 0.05; “**” ≤ 0.01; “***” ≤ 0.001.
The PCOA carried out on Bray-Curtis dissimilarity matrices showed a clear difference in species assemblages between OC and CV parcels (Figure 3). For carabids (Figure 3- left), this difference was visible along the second component. The first component rather segregated the three locations, with location A on the left of the axis and location B and C covering a wider gradient along the first component. This visual difference across system types was confirmed by the ANOVA carried out on the CAP (F = 8.95, P < 0.001). PCOA of the Bray-Curtis dissimilarity matrix of spiders (Figure 3- right) showed a gradient along the first axis with a decreasing amount of OC parcels and an increasing amount of CV parcels from left to right. Again, this visual difference was confirmed statistically by the ANOVA carried out on the CAP attesting of a significant different species assemblage across the two system types (F = 5.60, P < 0.001).
Figure 3. Principal Coordinates Analyses (PCOA) represented under their two principal components for carabids (left) and spiders (right). System types are represented in colors with black for organic-conservation (OC) parcels and gray for conventional (CV) ones. Shapes represent locations. Each system type is surrounded to help visualization.
This difference in species assemblages between OC and CV parcels was further described using turnover and nestedness (Baselga, 2010) (Table 1). For both carabids and spiders, OC parcels were characterized by a higher turnover than in CV parcels. This means OC systems are associated with a higher variation of species assemblages among parcels than CV systems. On the other hand, nestedness did not significantly differ between OC and CV parcels.
Table 1. Results of statistical tests (Anova, P < 0.05) carried out on the constrained analysis of principal coordinates (CAP) which constrained distance matrices of carabids and spiders with system types, i.e., significant P-values indicate a significant differences between system types for each group across both components of beta-diversity (turnover and nestedness) and their total.
For both carabids and spiders, OC parcels had higher number of indicator species. These are depicted by IndVal values for which the combination of specificity and fidelity showed a significant relationship between the species and the targeted system type (Tables 2, 3).
Table 2. Specificity and fidelity components of value index (indval) for carabids in organic conservation parcels and conventional ones, last column indicates the significance of the relationship between the species and the targeted system type.
Table 3. Specificity and fidelity components of value index (indval) for spiders in organic conservation parcels and conventional ones, last column indicates the significance of the relationship between the species and the targeted system type.
For carabids (Table 2), eleven species can be considered specific to OC parcels due to their high fidelity and specificity to this system type. Among these, four [Notiophilus palustris (Duftschmid, 1812), Parophonus maculicornis (Duftschmid, 1812), Stenolophus teutonus (Schrank, 1781) and Anisodactylus binotatus (Fabricius, 1787)] were only found in OC parcels (specificity = 1). On the other hand, four species can be considered specific to CV parcels due to their high specificity and fidelity, among which one was entirely specific to this farming type [Demetrias atricapillus (Linnaeus, 1758)].
For spiders (Table 3), five species showed high specificity and fidelity to OC parcels while only two species did for CV parcels. Among the five species representative of OC parcels, one [Pardosa pullata (Clerck, 1757)] was only found in OC parcels. On the other hand, CV parcels did not show any species which was exclusively specific to their system type.
PCA biplots represented a high percentage of variation in the distribution of traits (expressed as CMW) and functional diversity (60% for carabids and 63% for spiders; Figures 4, 5, respectively). These figures show that OC and CV parcels are segregated along the second component. For both carabids and spiders, most functional trait information point toward OC parcels. Constraining these trait datasets by system types by means of RDA showed that traits and functional diversity indices were significantly correlated with system types for both carabids and spiders (F = 15.02, P < 0.001; F = 24.82, P ≤ 0.001, respectively).
Figure 4. Principal Component Analysis of CWM-traits attributes and functional diversity indices of carabids community in organic-conservation (black) and conventional (gray) parcels. Shapes indicate the three sampling locations as detailed in legend box. CWM- traits included are: regime (herbivore, granivore, omnivore, predator); wing system type (di- and poly-morphic, macropterous, brachypterous); mean body size. The black arrow depicts the system variable, allowing interpretation between trait attributes and farming system type. Each system type is surrounded to help visualization.
Figure 5. Principal Component Analysis of CWM-traits attributes and functional diversity indices of spider community in organic-conservation (black) and conventional (gray) parcels. Shapes indicate the three sampling locations as detailed in legend box. CWM-traits included are: habitat type (agriculture, wetlands, forests and semi natural habitats, caves); mean body size; foraging mode (web weaver or run and kill). The black arrow depicts the system variable, allowing interpretation between trait attributes and farming system type. Each system type is surrounded to help visualization.
Regarding carabids, OC tended to host more herbivorous and granivorous, while CV presented more predator species. No significant differences could be observed for wing morphology and size (Table 4). In terms of functional diversity, OC parcels showed higher functional divergence, dispersion and evenness compared to CV parcels, but there was no significant difference in terms of functional richness between parcel types (Table 5).
Table 4. Summary tables of χ2 tests applied on linear mixed models testing the impact of system type (OC and CV) on each trait expressed as community weighted mean (cwm) for carabids (A) and spiders (B).
Table 5. Summary table of χ2 tests applied on linear mixed models testing the impact of system type (OC and CV) on each functional diversity indicator for both carabids and spiders.
As for spiders, OC tended to host larger individuals and more hunting foraging strategies while CV parcels hosted smaller individuals and more web weavers (Table 4). In terms of habitat preferences, we found more species linked to wetlands in OC parcels and more species related to forests in CV ones. As for functional diversity indices, there was no significant difference between the two system types (Table 5).
Our sampling of carabids and spiders communities across OC and CV parcels showed that combining organic and conservation practices promotes these two ground-dwelling communities in terms of taxonomic and functional diversities. These results are consistent with the hypothesis that OC systems, by removing both synthetic inputs and deep soil plowing, can provide more resources and host more diverse communities. This was also illustrated by a general higher variability among OC parcels than CV ones (e.g., higher turnover beta diversity indices, higher variability among diversity indices or traits-cwm). Hence our two introduced hypotheses were supported by our results.
Despite higher diversities in OC compared to CV parcels, our results do not show significant difference in terms of abundance. This corroborates several studies that found lower or equal abundances of carabids either in parcels with reduced tillage (Baguette and Hance, 1997; Hatten et al., 2007) or in organic parcels compared to convention ones (Bengtsson et al., 2005; Hole et al., 2005; Purtauf et al., 2005b). Such abundance—diversity discrepancies can find several explanations. Firstly, intensive soil tillage mostly affects arthropods whose life cycles take place in soil (Rowen et al., 2020), thus, looking at community-level abundance may potentially hide this effect. Moreover, CV systems often favor a few generalist species, increasing the overall abundance without necessarily increasing diversity indices (Baguette and Hance, 1997). Additionally, pitfall traps may lead to bias sampling. Indeed, disturbances associated with farming practices (e.g. soil plowing) or the presence of bare soil (without residues or cover crop) may increase arthropod activity and increase the probability of capture in pitfall traps. This may result in the misleading conclusion that there are more individuals while they are only more active (Lang, 2000; Holland and Reynolds, 2003; Koivula et al., 2003; Lundgren et al., 2006).
Recently, several authors have claimed that functional trait approaches provide deeper understanding of processes shaping ecosystem functioning (Rusch et al., 2015; Wood et al., 2015; Brousseau et al., 2018). Our results showed that carabid and spider functional traits differ between OC and CV systems. OC parcels hosted more herbivorous and granivorous carabids, whereas CV parcels hosted more predator carabids. This increase in granivorous is consistent with our hypothesis, since OC systems enhance niche availability for non-cropped plants, thus providing resources for species feeding on their seeds (Navntoft et al., 2009; Trichard et al., 2014; Petit et al., 2017). Brachypterous carabids (i.e., with small wings) were more abundant in OC parcels. As long winged communities have shown to represent a response to disturbed environment by investing in flight ability (Venn, 2007), our finding suggests that OC systems are likely more stable environments. Surprisingly, we found no difference in carabid size between systems, and we found larger spiders in OC. This finding is in contradiction with our predictions. Indeed, OC systems are associated with higher vegetation cover density and heterogeneity (because OC parcels were sown with intercropping mixes of six different species), which is expected to hamper the activity of larger specimen (Rouabah et al., 2015; Fischer et al., 2020). Previous research has for instance shown that parcels with reduced tillage are associated with larger beetle species (Hatten et al., 2007). Spiders' hunting strategy also differed across system types with more web weavers in CV parcels and more active hunters in OC parcels. Spiders' habitat preferences also showed distinguished trends between the two systems, with OC parcels showing more species specific to wetlands and agriculture and CV parcels hosting more species specific of forests. This illustrate once more how changing practices really shapes habitats for biodiversity.
Higher species and functional diversities is expected to be correlated with increased agroecosystem resilience, i.e., an increased ability to maintain ecosystem functions under disturbances (e.g., extreme climate events such as droughts; Peterson et al., 1998; Mijatović et al., 2013; Altieri et al., 2015). In the light of our results, it is fair to conclude that OC systems tend to support higher levels of biodiversity (taxonomic), and that thanks to their higher functional diversity and more even distribution, they are likely to provide more stable functions and represent more resilient farming systems to climate change.
However, the relationship between species diversity and pest regulation is controversial. For instance, a site with low diversity can have a few generalist species having important roles as pest regulator (e.g., the very common carabid species Poecilus cupreus is a significant weed seed predator). On the other hand, a high diversity can be composed of many rare species not able to play such role. Moreover, the actual pest regulating service is likely to be determined by a wide and interacting network of communities including other ground dwelling groups (e.g., springtails, mites), flying arthopods (e.g., hoverflies, lady bugs) and even below ground communities (e.g., nematods). Our results show that our two studied communities respond differently to farming practices change, with carabids being sensitive in terms of functional diversities and spiders in terms of species diversities. This result corroborates previous studies showing that different communities respond differently to each farming practice (Thorbek and Bilde, 2004; Puech et al., 2014; Muneret et al., 2019a; Rowen et al., 2020). Hence, further research, including groups other than carabids and spiders, is warranted in order to have a comprehensive overview of the effect of OC on pest regulating communities.
Several recent studies have argued that functional diversity better predicts pest regulation (Rusch et al., 2015; Jacobsen et al., 2022). The hypothesis laying behind is that when the diversity of predator traits is high, niche overlap and thus competition among predators is reduced and a broader range of prey species is predated (Cadotte et al., 2011). Rusch et al. (2015) found that smaller spider sizes and spiders with a preference for arable land were positively related to aphids predation rates. In our study, while we found larger spiders in OC parcels, OC parcels showed higher proportions of arable spider species, thus potentially supporting higher predation potential. Rusch et al. (2015) also found that functional diversity explained a great part of variation in predation rates. In our study, carabids' functional diversities were significantly favored in OC parcels, while no significant difference were found for spiders.
In brief, our study shows that pest control is potentially restored in OC parcels based on higher taxonomic and functional diversities. Further research is warranted to assess the effect of OC practices on both predatory community measurements and pest community measurements in order to provide actual measurement of the pest regulation service. We had planned to study aphid communities and aphid predation rates in all 15 fields sampled. Unfortunately, 2016 was characterized by an extremely dry spring and no aphids could be found in any of the fields. Nevertheless, aphid counting and predation assessment carried out in 2015 and 2017 in the same parcels showed higher aphid densities in CV parcels and no difference in terms of aphid predation rates (see Boeraeve et al., 2020 for protocols and published data). This data suggests that aphids were effectively regulated in OC parcels, though not through higher predation, thus confirming the relevance of conducting further research on pest regulation in OC systems.
Although our study showed that OC systems support biodiversity, it did not assess the benefit of combining organic and conservation agriculture compared to the application of either approach. While organic agriculture usually shows higher potential of pest regulation than conservation agriculture, it is also often associated with lower yields (Chabert and Sarthou, 2020; Wittwer et al., 2021). Our study is encouraging with regards to the effect of combining organic and conservation agriculture on biodiversity, and corroborates previous work of Rivers et al. (2017, 2018) who showed that conservation system undergoing organic transition positively influenced carabids activity, diversity and trophic groups throughout the transition. Recent studies showed that combing both approaches also enhanced other ecosystem service delivery (o.a. soil fertility, carbon sequestration, etc.), though such systems still lack behind in terms of productivity (Boeraeve et al., 2020; Wittwer et al., 2021). Since transitions to OC systems are often relatively recent, a time lag is likely to take place between the restoration of ecosystem services, and the achievement of high yields, as yield in OC systems mainly depend on ecosystem services (such as soil fertility, pest regulation, etc.). Thus, these first attempts to evaluate OC systems are encouraging in terms of biodiversity support and multifunctionality, but more work and time are required to understand and alleviate this remaining productivity gap.
Responses of biological communities to agricultural practices depends on local soil-ecological conditions, on the biological diversity present in the plots before the transition and on the biological diversity and practices in the surrounding landscape. It has been shown, for example, that the impact of organic farming (Tscharntke et al., 2005) or reduced tillage practices (Petit et al., 2017) within more heterogeneous landscapes can have less or detrimental effect on some biological groups; or that landscape parameters can have a stronger influence than that of agricultural practices (Purtauf et al., 2005b; Rusch et al., 2016). Landscape hospitability is not only defined by its heterogeneity, but also by the practices applied within its agricultural matrix. For instance, the proportion of organic farming in the landscape can be a stronger driver of species abundance than the proportion of semi-natural habitats (Muneret et al., 2019a), but again, this landscape effect is highly taxonomic group—dependent (Muneret et al., 2019b). Variability of responses may also be due to the variability of farming practices within each farming category. Indeed, a diversity of practices exist within each farming category and a gradient of practices intensity is present, with farming categories overlapping, rather than unequivocal and distinct farming groups (Puech et al., 2014; Chabert and Sarthou, 2017). Within our study, landscape components were controlled by pairing OC and CV adjacent parcels, thus comparisons are carried out within similar pedo-ecological contexts. Hence, the variation encountered across parcels and within system types in our study is more likely to be due to the diversity of agricultural practices within each system category (OC—CV; see Boeraeve et al., 2020 for more detailed description of the set of practices applied in each category). Further studies should thus detail technical itineraries for each parcel and take these into account in their analyses.
In view of this high complexity, the major challenge is to keep on documenting these interrelationships in different contexts, in other to gradually build fundamental knowledge on the conditions required to restore agricultural biodiversity, pest-predator networks and resilient agroecosystems. While an increasing amount of farmers are seeking to combine organic and conservation farming strategies (Mäder and Berner, 2012; Casagrande et al., 2016; Peigné et al., 2016), and while this generating growing interest among the scientific community, these systems remain poorly understood (Carr et al., 2012). Yet, organic and conservation agricultures share common principles, like enhancing biological activity to increase resilience and autonomy. As conservation agriculture does not specifically address pest management, it could benefit from strategies and knowledge developed in organic agriculture on pest and predator life cycles and their interactions with the environment to manage these without synthetic nor mechanical inputs (Nawaz and Jam Nazeer, 2015). Since no curative tools remain to control pests under OC farming strategy, a greater understanding of ecological functions underpinning this ecosystem service is required. We thus encourage further research to go beyond classical typologies of farming strategies (organic vs. conventional) and to consider innovative and promising systems like OC.
The present study showed that combining organic and conservation agriculture leads to higher taxonomic and functional diversities and higher variability of responses for two major ground dwelling predatory communities, carabids and spiders. It also showed that OC systems are associated with a higher variation of species assemblages among parcels than CV systems. Whether these diversities allow increased pest regulation remains to be explored. The present research represents one of the first attempt to assess the effect of combining organic and conservation agriculture to restore functional agrobiodiversity and, while these first results are encouraging, further research is required to better disentangle the complex and multi-faceted interrelationships between functional agrobiodiversity and ecosystem service delivery. OC agriculture would pose the least possible risk to human health and the environment, but remains to be further studied to ensure economic viability through an efficient restoration of ecosystem services.
The original contributions presented in the study are included in the article/Supplementary materials, further inquiries can be directed to the corresponding author.
MD and FB: conceptualization of research experiment and funding acquisition. FB: formal analysis and investigation. JT and GC: arthropod taxonomic identification. MD: supervision. FB: writing—original draft. All authors: writing—review and editing. All authors have read and agreed to the published version of the manuscript.
This research was funded by the National Research Fund for Research of Belgium (Fond National de la Recherche Scientifique—FNRS).
We thank all farmers who have given access to their parcels for the sampling of carabids and spiders individuals. We thank the National Fund for Scientific Research (FNRS) for providing a Ph.D. scholarship to FB and a Research Credit awarded to MD. We thank both reviewers for their insightful and careful reviews, as well as the editor for the effective handling of the review and publication processes.
The authors declare that the research was conducted in the absence of any commercial or financial relationships that could be construed as a potential conflict of interest.
All claims expressed in this article are solely those of the authors and do not necessarily represent those of their affiliated organizations, or those of the publisher, the editors and the reviewers. Any product that may be evaluated in this article, or claim that may be made by its manufacturer, is not guaranteed or endorsed by the publisher.
The Supplementary Material for this article can be found online at: https://www.frontiersin.org/articles/10.3389/fsufs.2022.1003637/full#supplementary-material
Altieri, M. A. (1999). The ecological role of biodiversity in agroecosystems. Agric. Ecosyst. Environ. 74, 19–31. doi: 10.1016/S0167-8809(99)00028-6
Altieri, M. A., Nicholls, C. I., Henao, A., and Lana, M. A. (2015). Agroecology and the design of climate change-resilient farming systems. Agron. Sustain. Dev. 35, 869–890. doi: 10.1007/s13593-015-0285-2
Baguette, M., and Hance, T. (1997). Carabid beetles and agricultural practices: influence of soil ploughing. Biol. Agric. Amp Hortic. 15, 185–190. doi: 10.1080/01448765.1997.9755193
Baselga, A. (2010). Partitioning the turnover and nestedness components of beta diversity. Glob. Ecol. Biogeogr. 19, 134–143. doi: 10.1111/j.1466-8238.2009.00490.x
Baselga, A., and Orme, C. D. L. (2012). betapart : an R package for the study of beta diversity. Methods Ecol. Evol. 3, 808–812. doi: 10.1111/j.2041-210X.2012.00224.x
Bates, D. M., and Maechler, M. (2018). Package “lme4”: Linear Mixed-Effects Models using “Eigen” and S4. Available online at: https://cran.r-project.org/web/packages/lme4/lme4.pdf (accessed June 14, 2018).
Bengtsson, J., Ahnström, J., and Weibull, A.-C. (2005). The effects of organic agriculture on biodiversity and abundance: a meta-analysis. J. Appl. Ecol. 42, 261–269. doi: 10.1111/j.1365-2664.2005.01005.x
BETSI (2014). BETSIdatabase: A database for soil invertebrate biological and ecological traits. Database Soil Invertebr. Biol. Ecol. Traits. Available online at: http://betsi.cesab.org/ (accessed October 10, 2022).
Boeraeve, F., Dendoncker, N., Degrune, F., Cornelis, J.-T., and Dufrêne, M. (2020). Contribution of agroecological farming systems to the delivery of ecosystem services. J. Environ. Manage. 260, 109576. doi: 10.1016/j.jenvman.2019.109576
Bohan, D. A., Boursault, A., Brooks, D. R., and Petit, S. (2011). National-scale regulation of the weed seedbank by carabid predators. J. Appl. Ecol. 48, 888–898. doi: 10.1111/j.1365-2664.2011.02008.x
Brousseau, P.-M., Gravel, D., and Handa, I. T. (2018). Trait matching and phylogeny as predictors of predator–prey interactions involving ground beetles. Funct. Ecol. 32, 192–202. doi: 10.1111/1365-2435.12943
Caceres, M. D., and Legendre, P. (2009). Indicspecies Package - Associations Between Species and Groups of Sites: Indices and Statistical Inference. Available online at: http://sites.google.com/site/miqueldecaceres/ (accessed January 1, 2022).
Cadotte, M. W., Carscadden, K., and Mirotchnick, N. (2011). Beyond species: functional diversity and the maintenance of ecological processes and services. J. Appl. Ecol. 48, 1079–1087. doi: 10.1111/j.1365-2664.2011.02048.x
Carr, P. M., Mäder, P., Creamer, N. G., and Beeby, J. S. (2012). Editorial: overview and comparison of conservation tillage practices and organic farming in Europe and North America. Renew. Agric. Food Syst. 27, 2–6. doi: 10.1017/S1742170511000536
Casagrande, M., Peign,é, J., Payet, V., Mäder, P., Sans, F. X., Blanco-Moreno, J. M., et al. (2016). Organic farmers' motivations and challenges for adopting conservation agriculture in Europe. Org. Agric. 6, 281–295. doi: 10.1007/s13165-015-0136-0
Chabert, A., and Sarthou, J.-P. (2017). Practices of conservation agriculture prevail over cropping systems and landscape heterogeneity in understanding the ecosystem service of aphid biocontrol. Agric. Ecosyst. Environ. 249, 70–79. doi: 10.1016/j.agee.2017.08.005
Chabert, A., and Sarthou, J. P. (2020). Conservation agriculture as a promising trade-off between conventional and organic agriculture in bundling ecosystem services | Elsevier Enhanced Reader. Agric. Ecosyst. Environ. 292, 106815. doi: 10.1016/j.agee.2019.106815
Darnhofer, I., Lindenthal, T., Bartel-Kratochvil, R., and Zollitsch, W. (2010). Conventionalisation of organic farming practices: from structural criteria towards an assessment based on organic principles. A review. Agron. Sustain. Dev. 30, 67–81. doi: 10.1051/agro/2009011
Deguine, J.-P., Gloanec, C., Laurent, P., Ratnadass, A., and Aubertot, J.-N. (2017). Agroecological Crop Protection. Dordrecht: Springer Netherlands.
Desender, K. (1986). Distribution and ecology of carabid beetles in Belgium (Coleoptera, Carabidae). Part 3. Rijksuniv. Gent. 30, 153–217.
Dudley, N., and Alexander, S. (2017). Agriculture and biodiversity: a review. Biodiversity 18, 45–49. doi: 10.1080/14888386.2017.1351892
Dufrêne, M., and Legendre, P. (1997). Species assemblages and indicator species: the need for a flexible asymmetrical approach. Ecol. Monogr. 67, 345–366. doi: 10.2307/2963459
ELN FAB (2012). Functional Agrobiodiversity - Nature Serving Europe's Farmers. Tilburg, The Netherlands: ECNC-European Centre for Nature Conservation. Available online at: https://ec.europa.eu/environment/nature/natura2000/platform/documents/functional_agrobiodiversity_eln-fab_publication_en.pdf (accessed November 26, 2021).
Feber, R. E., Bell, J., Johnson, P. J., Firbank, L. G., and MacDonald, D. W. (1998). The effects of organic farming on surface-active spider (Araneae) assemblages in wheat in southern England, UK. J. Arachnol. 26, 190–202.
Fischer, C., Riesch, F., Tscharntke, T., and Batáry, P. (2020). Large carabids enhance weed seed removal in organic fields and in large-scale, but not small-scale agriculture. Landsc. Ecol. 36, 427–438. doi: 10.1007/s10980-020-01157-8
Fleury, P., Chazoule, C., and Peign,é, J. (2014). Ruptures et transversalités entre agriculture biologique et agriculture de conservation. Économie Rurale Agric. Aliment. Territ. 339–340, 95–112. doi: 10.4000/economierurale.4247
Foley, J. A. (2011). Can we feed the world & sustain the planet? Sci. Am. 305, 60–65. doi: 10.1038/scientificamerican1111-60
Fox, J., Weisberg, S., and Price, B. (2018). Package “car”: companion to Applied Regression. Available online at: https://cran.r-project.org/web/packages/car/car.pdf (accessed June 14, 2018).
Gloor, D., Blick, T., Nentwig, W., Kropf, C., and Hänggi, A. (2010). DataBase: Spiders of Europe. Available online at: https://araneae.nmbe.ch/ (accessed February 11, 2022).
Gordon, L. J., Bignet, V., Crona, B., Henriksson, P. J. G., Van Holt, T., Jonell, M., et al. (2017). Rewiring food systems to enhance human health and biosphere stewardship. Environ. Res. Lett. 12, 100201. doi: 10.1088/1748-9326/aa81dc
Guevara, M. R., Hartmann, D., and Mendoza, M. (2016). diverse: an R package to analyze diversity in complex systems. R J. 8, 60. doi: 10.32614/RJ-2016-033
Hatt, S., Artru, S., Brédart, D., Lassois, L., Francis, F., Haubruge, É., et al. (2016). Towards sustainable food systems: the concept of agroecology and how it questions current research practices. A review. Biotechnol. Agron. Soc. Environ. 20, 215–224. doi: 10.25518/1780-4507.12997
Hatten, T. D., Bosque-Pérez, N. A., Labonte, J. R., Guy, S. O., and Eigenbrode, S. D. (2007). Effects of tillage on the activity density and biological diversity of carabid beetles in spring and winter crops. Environ. Entomol. 36, 356–368. doi: 10.1093/ee/36.2.356
Hole, D. G., Perkins, A. J., Wilson, J. D., Alexander, I. H., Grice, P. V., and Evans, A. D. (2005). Does organic farming benefit biodiversity? Biol. Conserv. 122, 113–130. doi: 10.1016/j.biocon.2004.07.018
Holland, J. M., and Reynolds, C. J. (2003). The impact of soil cultivation on arthropod (Coleoptera and Araneae) emergence on arable land. Pedobiologia 47, 181–191. doi: 10.1078/0031-4056-00181
Homburg, K., Homburg, N., Schäfer, F., Schuldt, A., and Assmann, T. (2014). Carabids.org - a dynamic online database of ground beetle species traits (Coleoptera, Carabidae). Insect Conserv. Divers. 7, 195–205. doi: 10.1111/icad.12045
IPBES, Watson, R. T., Baste, I. A., Larigauderie, A., Leadley, P., Pascual, U., et al. (2019). The Global Assessment Report on Biodiversity and Ecosystem Services, Bonn, Germany: IPBES secretariat, 1148.
Jacobsen, S. K., Sigsgaard, L., Johansen, A. B., Thorup-Kristensen, K., and Jensen, P. M. (2022). The impact of reduced tillage and distance to field margin on predator functional diversity. J. Insect Conserv. 26, 491–501. doi: 10.1007/s10841-022-00370-x
Jeannel, R. (1941). Faune de France: Coléoptères Carabiques - Tome II. Paul Lechevalier&Fils. Paris. Available online at: http://www.faunedefrance.org/bibliotheque/docs/R.%20JEANNEL(FdeFr39)%20Carab%20vol%201.pdf (accessed June 11, 2018).
Jeannel, R. (1942). Faune de France: Coléoptères Carabiques - Tome I. Paul Lechevalier&Fils. Paris. Available online at: http://www.faunedefrance.org/bibliotheque/docs/R.%20JEANNEL(FdeFr40)%20Carab%20vol%202%20.pdf (accessed June 11, 2018).
Kirkegaard, J. A., Conyers, M. K., Hunt, J. R., Kirkby, C. A., Watt, M., and Rebetzke, G. J. (2014). Sense and nonsense in conservation agriculture: Principles, pragmatism and productivity in Australian mixed farming systems. Agric. Ecosyst. Environ. 187, 133–145. doi: 10.1016/j.agee.2013.08.011
Koivula, M., Kotze, D. J., Hiisivuori, L., and Rita, H. (2003). Pitfall trap efficiency: do trap size, collecting fluid and vegetation structure matter? Entomol. Fenn. 14, 1–14. doi: 10.33338/ef.84167
Kotze, D. J., Brandmayr, P., Casale, A., Dauffy-Richard, E., Dekoninck, W., Koivula, M. J., et al. (2011). Forty years of carabid beetle research in Europe – from taxonomy, biology, ecology and population studies to bioindication, habitat assessment and conservation. ZooKeys 100, 55–148. doi: 10.3897/zookeys.100.1523
Kromp, B. (1999). Carabid beetles in sustainable agriculture: a review on pest control efficacy, cultivation impacts and enhancement. Agric. Ecosyst. Environ. 74, 187–228. doi: 10.1016/S0167-8809(99)00037-7
Kulkarni, S. S., Dosdall, L. M., and Willenborg, C. J. (2015). The role of ground beetles (Coleoptera: Carabidae) in weed seed consumption: a review. Weed Sci. 63, 335–376. doi: 10.1614/WS-D-14-00067.1
Laliberté, E., Legendre, P., and Shipley, B. (2015). Package FD: “Measuring Functional Diversity (FD) From Multiple Traits, and Other Tools for Functional Ecology.” Available online at: https://cran.r-project.org/web/packages/FD/FD.pdf (accessed April 1, 2022).
Lang, A. (2000). The pitfalls of pitfalls: a comparison of pitfall trap catches and absolute density estimates of epigeal invertebrate predators in Arable Land. Anz. Für Schädlingskunde J. Pest Sci. 73, 99–106. doi: 10.1007/BF02956438
Leifeld, J. (2012). How sustainable is organic farming? Agric. Ecosyst. Environ. 150, 121–122. doi: 10.1016/j.agee.2012.01.020
Lundgren, J. G., Shaw, J. T., Zaborski, E. R., and Eastman, C. E. (2006). The influence of organic transition systems on beneficial ground-dwelling arthropods and predation of insects and weed seeds. Renew. Agric. Food Syst. 21, 227–237. doi: 10.1079/RAF2006152
Mäder, P., and Berner, A. (2012). Development of reduced tillage systems in organic farming in Europe. Renew. Agric. Food Syst. 27, 7–11. doi: 10.1017/S1742170511000470
McLaughlin, A., and Mineau, P. (1995). The impact of agricultural practices on biodiversity. Agric. Ecosyst. Environ. 55, 201–212. doi: 10.1016/0167-8809(95)00609-V
Michalko, R., Pekár, S., and Entling, M. H. (2019). An updated perspective on spiders as generalist predators in biological control. Oecologia 189, 21–36. doi: 10.1007/s00442-018-4313-1
Mijatović, D., Van Oudenhoven, F., Eyzaguirre, P., and Hodgkin, T. (2013). The role of agricultural biodiversity in strengthening resilience to climate change: towards an analytical framework. Int. J. Agric. Sustain. 11, 95–107. doi: 10.1080/14735903.2012.691221
Mostafalou, S., and Abdollahi, M. (2017). Pesticides: an update of human exposure and toxicity. Arch. Toxicol. 91, 549–599. doi: 10.1007/s00204-016-1849-x
Muneret, L., Auriol, A., Bonnard, O., Richart-Cervera, S., Thiéry, D., and Rusch, A. (2019a). Organic farming expansion drives natural enemy abundance but not diversity in vineyard-dominated landscapes. Ecol. Evol. 9, 13532–13542. doi: 10.1002/ece3.5810
Muneret, L., Auriol, A., Thiéry, D., and Rusch, A. (2019b). Organic farming at local and landscape scales fosters biological pest control in vineyards. Ecol. Appl. 29, e01818. doi: 10.1002/eap.1878
Navntoft, S., Wratten, S. D., Kristensen, K., and Esbjerg, P. (2009). Weed seed predation in organic and conventional fields. Biol. Control 49, 11–16. doi: 10.1016/j.biocontrol.2008.12.003
Nawaz, A., and Jam Nazeer, A. (2015). “Chapter 6 - Insect pest management in conservation agriculture,” in Conservation Agriculture, eds M. Farooq and K. H. M. Siddique (Switzerland: Springer International Publishing).
Nentwig, W., Blick, T., Bosmans, R., Gloor, D., Hänggi, A., and Kropf, C. (2022). Spiders of Europe. University of Bern. Available online at: https://araneae.nmbe.ch/ (accessed March 22, 2022).
Nyffeler, M., and Sunderland, K. D. (2003). Composition, abundance and pest control potential of spider communities in agroecosystems: a comparison of European and US studies. Agric. Ecosyst. Environ. 95, 579–612. doi: 10.1016/S0167-8809(02)00181-0
Peigné, J., Casagrande, M., Payet, V., David, C., Sans, F. X., Blanco-Moreno, J. M., et al. (2016). How organic farmers practice conservation agriculture in Europe. Renew. Agric. Food Syst. 31, 72–85. doi: 10.1017/S1742170514000477
Peterson, G., Allen, C. R., and Holling, C. S. (1998). Ecological resilience, biodiversity, and scale. Ecosystems 1, 6–18. doi: 10.1007/s100219900002
Petit, S., Trichard, A., Biju-Duval, L., McLaughlin, Ó. B., and Bohan, D. A. (2017). Interactions between conservation agricultural practice and landscape composition promote weed seed predation by invertebrates. Agric. Ecosyst. Environ. 240, 45–53. doi: 10.1016/j.agee.2017.02.014
Pfiffner, L., and Niggli, U. (1995). Effects of bio-dynamic, organic and conventional farming on ground beetles (Col. Carabidae) and other epigaeic arthropods in winter wheat. Biol. Agric. Hortic. Available online at: https://www.tandfonline.com/doi/abs/ doi: 10.1080/01448765.1996.9754758 (accessed December 6, 2021).
Puech, C., Baudry, J., Joannon, A., Poggi, S., and Aviron, S. (2014). Organic vs. conventional farming dichotomy: does it make sense for natural enemies? Agric. Ecosyst. Environ. 194, 48–57. doi: 10.1016/j.agee.2014.05.002
Purtauf, T., Dauber, J., and Wolters, V. (2005a). The response of carabids to landscape simplification differs between trophic groups. Oecologia 142, 458–464. doi: 10.1007/s00442-004-1740-y
Purtauf, T., Roschewitz, I., Dauber, J., Thies, C., Tscharntke, T., and Wolters, V. (2005b). Landscape context of organic and conventional farms: influences on carabid beetle diversity. Agric. Ecosyst. Environ. 108, 165–174. doi: 10.1016/j.agee.2005.01.005
R Core Team (2021). R Software : A Language and Environment for Statistical Computing. Available online at: https://www.R-project.org/ (accessed June 14, 2018).
Rainio, J., and Niemela, J. (2003). Ground beetles (Coleoptera: Carabidae) as bioindicators. Biodivers. Conserv. 12, 487–503. doi: 10.1023/A:1022412617568
Rivers, A., Mullen, C., Wallace, J., and Barbercheck, M. (2017). Cover crop-based reduced tillage system influences Carabidae (Coleoptera) activity, diversity and trophic group during transition to organic production. Renew. Agric. Food Syst. 32, 538–551. doi: 10.1017/S1742170516000466
Rivers, A. N., Mullen, C. A., and Barbercheck, M. E. (2018). Cover crop species and management influence predatory arthropods and predation in an organically managed, reduced-tillage cropping system. Environ. Entomol. 47, 340–355. doi: 10.1093/ee/nvx149
Rouabah, A., Lasserre-Joulin, F., Amiaud, B., and Plantureux, S. (2014). Emergent effects of ground beetles size diversity on the strength of prey suppression: ground beetle size diversity and pest control. Ecol. Entomol. 39, 47–57. doi: 10.1111/een.12064
Rouabah, A., Villerd, J., Amiaud, B., Plantureux, S., and Lasserre-Joulin, F. (2015). Response of carabid beetles diversity and size distribution to the vegetation structure within differently managed field margins. Agric. Ecosyst. Environ. 200, 21–32. doi: 10.1016/j.agee.2014.10.011
Rowen, E. K., Regan, K. H., Barbercheck, M. E., and Tooker, J. F. (2020). Is tillage beneficial or detrimental for insect and slug management? A meta-analysis. Agric. Ecosyst. Environ. 294, 106849. doi: 10.1016/j.agee.2020.106849
Rusch, A., Birkhofer, K., Bommarco, R., Smith, H. G., and Ekbom, B. (2015). Predator body sizes and habitat preferences predict predation rates in an agroecosystem. Basic Appl. Ecol. 16, 250–259. doi: 10.1016/j.baae.2015.02.003
Rusch, A., Chaplin-Kramer, R., Gardiner, M. M., Hawro, V., Holland, J., Landis, D., et al. (2016). Agricultural landscape simplification reduces natural pest control: A quantitative synthesis. Agric. Ecosyst. Environ. 221, 198–204. doi: 10.1016/j.agee.2016.01.039
Sunderland, K., and Samu, F. (2000). Effects of agricultural diversification on the abundance, distribution, and pest control potential of spiders: a review. Entomol. Exp. Appl. 95, 1–13. doi: 10.1046/j.1570-7458.2000.00635.x
Tamburini, G., De Simone, S., Sigura, M., Boscutti, F., and Marini, L. (2016). Conservation tillage mitigates the negative effect of landscape simplification on biological control. J. Appl. Ecol. 53, 233–241. doi: 10.1111/1365-2664.12544
Thorbek, P., and Bilde, T. (2004). Reduced numbers of generalist arthropod predators after crop management. J. Appl. Ecol. 41, 526–538. doi: 10.1111/j.0021-8901.2004.00913.x
Tilman, D., Balzer, C., Hill, J., and Befort, B. L. (2011). Global food demand and the sustainable intensification of agriculture. Proc. Natl. Acad. Sci. 108, 20260–20264. doi: 10.1073/pnas.1116437108
Tilman, D., and Clark, M. (2014). Global diets link environmental sustainability and human health. Nature 515, 518–522. doi: 10.1038/nature13959
Trichard, A., Ricci, B., Ducourtieux, C., and Petit, S. (2014). The spatio-temporal distribution of weed seed predation differs between conservation agriculture and conventional tillage. Agric. Ecosyst. Environ. 188, 40–47. doi: 10.1016/j.agee.2014.01.031
Tscharntke, T., Grass, I., Wanger, T. C., Westphal, C., and Batáry, P. (2021). Beyond organic farming – harnessing biodiversity-friendly landscapes. Trends Ecol. Evol. 36, 919–930. doi: 10.1016/j.tree.2021.06.010
Tscharntke, T., Klein, A. M., Kruess, A., Steffan-Dewenter, I., and Thies, C. (2005). Landscape perspectives on agricultural intensification and biodiversity - ecosystem service management. Ecol. Lett. 8, 857–874. doi: 10.1111/j.1461-0248.2005.00782.x
Uetz, G. W., Halaj, J., and Cady, A. (1999). Guild structure of spiders in major crops. J. Arachnol. 27, 270–280.
Venn, S. (2007). Morphological responses to disturbance in wing-polymorphic carabid species (Coleoptera: Carabidae) of managed urban grasslands. Balt. J Coleopt. 7, 51–59. Available online at: http://www.bjc.sggw.pl/viewart.php?artid=196
Wilson, C., and Tisdell, C. (2001). Why farmers continue to use pesticides despite environmental, health and sustainability costs. Ecol. Econ. 39, 449–462. doi: 10.1016/S0921-8009(01)00238-5
Winder, L., Alexander, C. J., Holland, J. M., Symondson, W. O. C., Perry, J. N., and Woolley, C. (2005). Predatory activity and spatial pattern: the response of generalist carabids to their aphid prey. J. Anim. Ecol. 74, 443–454. doi: 10.1111/j.1365-2656.2005.00939.x
Wittwer, R. A., Bender, S. F., Hartman, K., Hydbom, S., Lima, R. A. A., Loaiza, V., et al. (2021). Organic and conservation agriculture promote ecosystem multifunctionality. Sci. Adv. 7, eabg6995. doi: 10.1126/sciadv.abg6995
Keywords: agroecology, ecosystem services, functional biodiversity, biological control, resilience, sustainable agriculture, ecological intensive
Citation: Boeraeve F, Vialatte A, Sirami C, Caro G, Thenard J, Francis F and Dufrêne M (2022) Combining organic and conservation agriculture to restore biodiversity? Insights from innovative farms in Belgium and their impacts on carabids and spiders. Front. Sustain. Food Syst. 6:1003637. doi: 10.3389/fsufs.2022.1003637
Received: 26 July 2022; Accepted: 05 October 2022;
Published: 18 October 2022.
Edited by:
Inge Armbrecht, University of Valle, ColombiaReviewed by:
Victor Andres Galindo Canabal, University of Valle, ColombiaCopyright © 2022 Boeraeve, Vialatte, Sirami, Caro, Thenard, Francis and Dufrêne. This is an open-access article distributed under the terms of the Creative Commons Attribution License (CC BY). The use, distribution or reproduction in other forums is permitted, provided the original author(s) and the copyright owner(s) are credited and that the original publication in this journal is cited, in accordance with accepted academic practice. No use, distribution or reproduction is permitted which does not comply with these terms.
*Correspondence: Fanny Boeraeve, ZmFubnkuYm9lcmFldmVAZ21haWwuY29t
Disclaimer: All claims expressed in this article are solely those of the authors and do not necessarily represent those of their affiliated organizations, or those of the publisher, the editors and the reviewers. Any product that may be evaluated in this article or claim that may be made by its manufacturer is not guaranteed or endorsed by the publisher.
Research integrity at Frontiers
Learn more about the work of our research integrity team to safeguard the quality of each article we publish.