- 1Centro Universitario del Sur, Universidad de Guadalajara, Guadalajara, Mexico
- 2Centro de Investigación en Alimentación y Desarrollo, A.C., (CIAD), Hermosillo, Sonora, Mexico
Industrial processing of citrus fruits generates an important amount of wastes that evoke environmental damage. Orange is the main citrus fruit consumed worldwide, and after its use, approximately more than a half of the fruit remains as by-products, which comprise important bioactive compounds useful for the development of promising nutraceuticals for the treatment of non-communicable diseases. This study aimed to gather scientific evidence about the biological effects of orange by-products using a systematic review. A total of 14 studies that were carried out in rodent models in the last 10 years were retrieved from PubMed and ScienceDirect databases. Studies that used another animal species, another type of citrus, or a combination of orange with other citrus were excluded. The risk of bias was assessed by using the SYRCLE RoB tool, and the results obtained are shown in an informative table, which showed that most of the studies used a pathological model of chronic diseases. We found that the peel is the most used agri-food by-product, and that it has the potential of reducing the levels of triglycerides, total cholesterol, glucose, and systolic blood pressure. However, to clinically assess these effects, these results need to be tested in future in humans. The included studies on the use of orange by-products strengthen the global sustainable food agenda. It is important to consider new research directions about the use of citrus fruit residues since it not only impacts the problem of its disposal but also provides solutions to eliminate the resulting contamination.
Introduction
Chronic non-communicable diseases (NCDs) are a group of conditions that represents long-term health consequences and treatments, and overall, these are the principal cause of death and disability worldwide (Pan American Health Organization, 2022). In 2022, NCDs such as cancer, diabetes, respiratory diseases, and cardiovascular diseases are the principal causes of death worldwide, representing 74% of cases (World Health Organization, 2022). These four NCDs are contribute to about 80% of premature deaths associated with these diseases (Pan American Health Organization, 2022). Metabolic risk factors of these conditions are overweight, obesity, hypertension, hyperglycemia, and hyperlipidemia, due to tobacco and alcohol use, lack of physical activity, and unhealthy diet (Pan American Health Organization, 2022; World Health Organization, 2022). The World Health Organization-recommended “best buys” provide evidence of cost-effective actions for the prevention and control of NCDs, where a change in eating behavior and the promotion of a healthy diet are considered (Pan American Health Organization, 2022). In addition to these actions, the consumption of functional foods can also reduce the risk for developing NCDs (Plasek et al., 2019). Recently, the interest in natural sources to prevent and treat non-communicable diseases has increased, in part due to the need for cost-effective treatments. Diverse fruits and their by-products are natural products and are considered safe, with less side effects and toxicity than drugs (Ahmed et al., 2017).
Among them, Citrus L. is a group of fruits that include several products, such as oranges, mandarins, lemons, grapefruits, citron, bergamots, fingered citron, limes, and others. The internal structure of this fruits consist of an endocarp, flavedo, and albedo (Garcia-Castello et al., 2022). Oranges (Citrus sinensis L.) are the most cultivated citrus (with over 40% of exportations), followed by tangerines, lemons, and grapefruits. Juices, oils, and citric acid are the main products extracted from citrus fruits. After the extraction of these products, the generated wastes are often discarded or used as animal feed (Food Agriculture Organization of the United Nations, 2022).
The global production of oranges in January 2021/22 was approximately over 48 million tons, with Brazil, China, the European Union, Mexico, and the United States being the main producers. In several countries, oranges are mainly used for extracting juice, representing 1.7 million tons of its production until January 2021/22 (United States Department of Agriculture, 2022). Some important compounds present in orange fruit are folic acid, thiamine, riboflavin, niacin, pantothenic acid, B6, potassium, phosphorus, calcium, iron, magnesium, sodium, ascorbic acid, amino acids, flavonoids, and phenolic compounds (U.S. Department of Agriculture, 2019).
After its use, more than a half of the fruit remains as a by-product. The orange peel represents the highest discarded waste (Olabinjo et al., 2017; Ayala et al., 2021), which is an important source of soluble sugar, pectin, ascorbic acid, fiber, and phenolic compounds (Parmar and Kar, 2008; Ezejiofor et al., 2011; Ahmed et al., 2017; Ashraf et al., 2017; Sathiyabama et al., 2018). Seeds are another most discarded waste, which contains an important quantity of oil, protein, potassium, sodium, calcium, phosphorous, iron, ascorbic acid, oxalate, alkaloids, fatty acids, phytosterols, tocopherols, and fiber (Okoye et al., 2011; El-safy et al., 2012; Malacrida et al., 2012; Zayed et al., 2018; Adubofuor et al., 2021). Orange pomace is yet another waste with a large number of bio-functional compounds; however, its use requires special attention because its large amount of moisture makes it the most perishable waste due to its high susceptibility to microbial spoilage (Afrin et al., 2022). The proximate composition of orange by-products (including peel, seed, albedo, and flavedo) includes ~60–70 of dietary fiber, 5.9–8.9 protein, and 1.8–4.5 g/100g dry matter of lipids, and 534 mg GAE/100 g of dry matter of phenolic compounds (Fernández-López et al., 2009; de Moraes Crizel et al., 2013; de Castro et al., 2020). These by-products can be revalorized as a natural source of vitamins, pectin, polyphenols, or essential oils with important health effects (Garcia-Castello et al., 2022). Therefore, this review aimed to gather scientific evidence about the biological effects of orange by-products, and to discuss the proposed mechanism of action.
Materials and methods
Studies retrieved from two databases were searched in this systematic review using the PRISMA 2020 statement (Page et al., 2021).
Search strategy and information sources
PubMed and ScienceDirect were the two databases used for the search of information of this review. The bibliographic search was carried out in English from March 2022 to May 2022.
The following combination of keywords were sought: “orange” OR “citrus sinensis” AND “peel” OR “seed” OR “waste” AND “triglycerides” OR “cholesterol” OR “glucose” OR “arterial pressure.” A total of 12 combinations of search terms were used. The filters applied to each database were as follows: articles published in the last 10 years (2012–2022) and “research articles.”
Eligibility criteria
This review included research studies that have evaluated the biological effects of orange (Citrus sinensis L.) by-products conducted in rodents. The inclusion criterion was studies written in English that evaluated the effect exerted by the administration of orange by-products on metabolic biomarkers. The exclusion criteria are as follows: articles with results other than those of interest or with incomplete results; articles written in languages other than English; studies used animal species other than rodents, studies used citrus other than orange, or studies used a combination of orange with other citrus.
Selection process and data collection
The selected articles were systematically reviewed and analyzed by two authors individually and in an independent manner for the effect of orange by-products on metabolic biomarkers such as triglycerides, total cholesterol, HDL cholesterol, glucose levels, and arterial pressure. Duplicated articles were eliminated from the final review. The data extracted from each article were organized and are presented in tables. In addition to the by-product effect on metabolic biomarkers, this review included data on the characteristics of the experimental model and the sample of the by-product used.
The realization of the meta-analysis was not considered due to the significant heterogeneity between study designs and interventions.
Risk of bias assessment
The SYRCLE RoB tool was used to assess the risk of bias (RoB) of experimental animal studies. The tool contains 10 entries related to six types of bias: selection, performance, detection, attrition, reporting, and other bias (Hooijmans et al., 2014). Two independent reviewers made the judgment for each study. The answers were “yes” (low RoB), “no” (high RoB), and “unclear” (insufficient details have been reported to assess the RoB).
Results
Search for articles and data collection
Through the keyword search in PubMed and ScienceDirect databases, 21,039 records were identified. An additional three records were identified from the citation search. Duplicate studies, studies including different topics, studies that used another animal species, studies that used a combination of citrus or food were eliminated. Finally, a total of 14 studies fulfilled the eligibility criteria and were included in the review. Figure 1 depicts the PRISMA 2020 flow diagram of the selection process for the articles included in this systematic review.
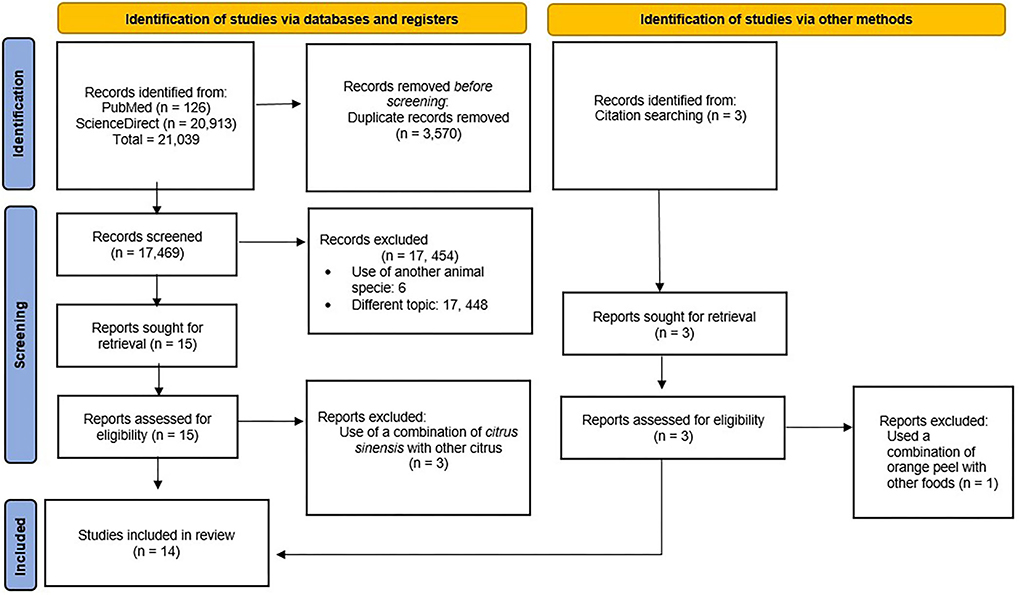
Figure 1. PRISMA 2020 flow diagram of the selection process for the articles included in the systematic review. From Page et al. (2021). For more information, visit: http://www.prisma-statement.org/.
Risk of bias assessment
In Figure 2, overall and individual RoBs were assessed for all the included studies. For selection bias, >90% of the studies did not specify the random allocation of animals. For those studies, we assumed a non-random application because for this type of research, the researchers included the subjects based on their preference for sex or animal strain, the result of a laboratory test, or even the availability of animals in the laboratory. Baseline characteristics (>90%) were frequently reported because these features were mostly considered the starting point for assigning experimental groups. Allocation concealment was not considered in these investigations, which resulted in a high RoB. Performance bias considered random housing (>90%), which resulted in a low RoB. However, blinding of investigators and caregivers was completely unclear. For detection bias, the random outcome assessment and blinding of outcome assessment items were rated as unclear RoBs. For attrition and reporting bias, incomplete outcome data and selective outcome reporting were mostly judged as a low RoB. Reporting of other biases was unclear as animal studies often do not report them. Overall, for the 10 entries of the RoB tool, 50% was ranked as “unclear” due to the lack of information from the studies, almost 40% was evaluated as a low RoB, and 10% as a high RoB.
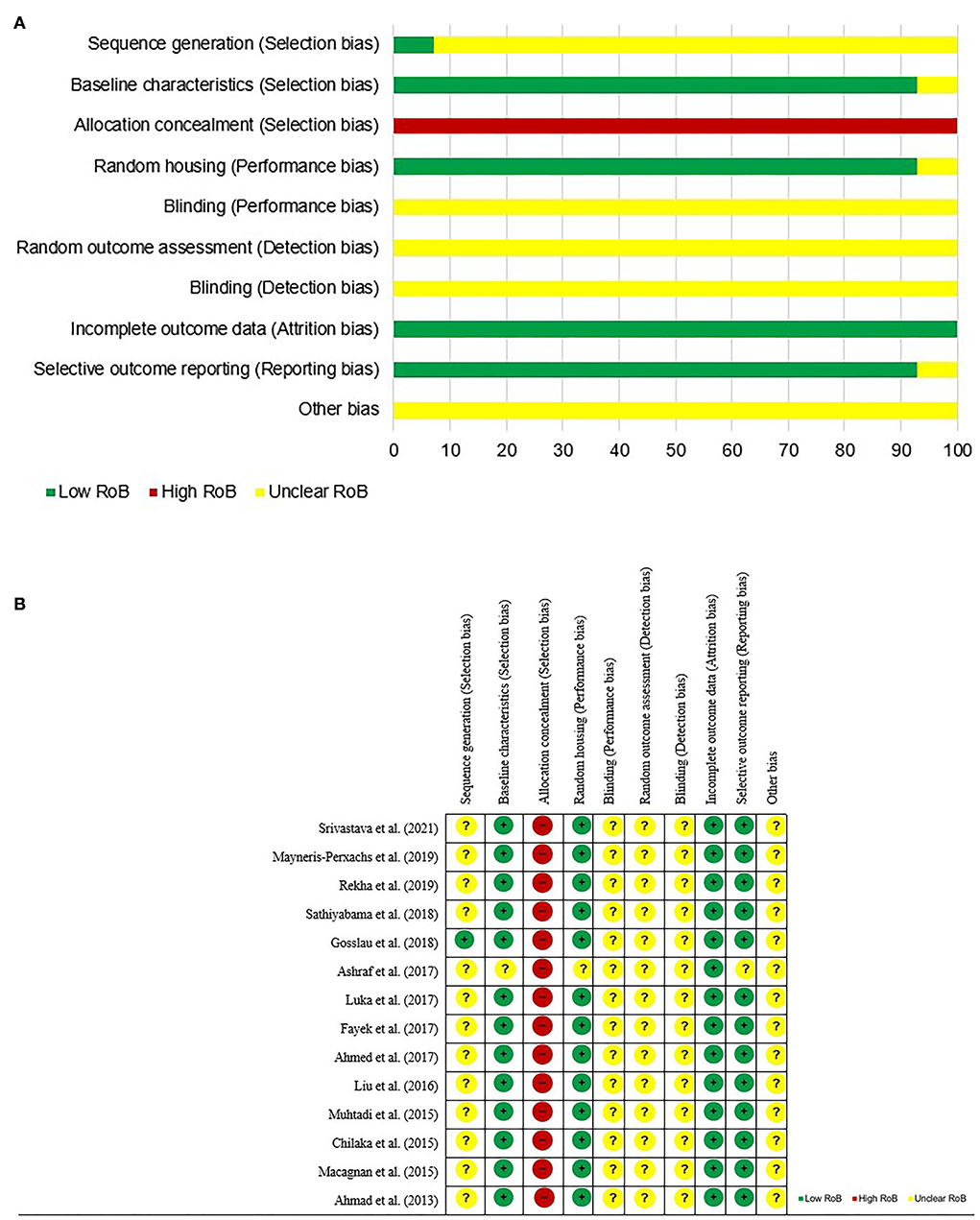
Figure 2. SYRCLE's RoB tool to assess the Risk of Bias (RoB). (A) Percentage of overall RoB for all included studies. (B) Individual RoB for each item of the tool.
Characteristics of the studies
From the 14 articles, 28.6% of the articles were published in 2017, 21.42% in 2015, 14.28% between 2018 and 2019, and 7.14% in 2013, 2016, and 2021.
Table 1 shows the articles that used in vivo rodent models to evaluate the effect of by-products. The data only included the information of the experimental groups exposed to an orange by-product and their comparative results with the negative control group or the healthy control group, as appropriate. The rest of groups that served as controls or were not exposed to the by-product were not included in the review.
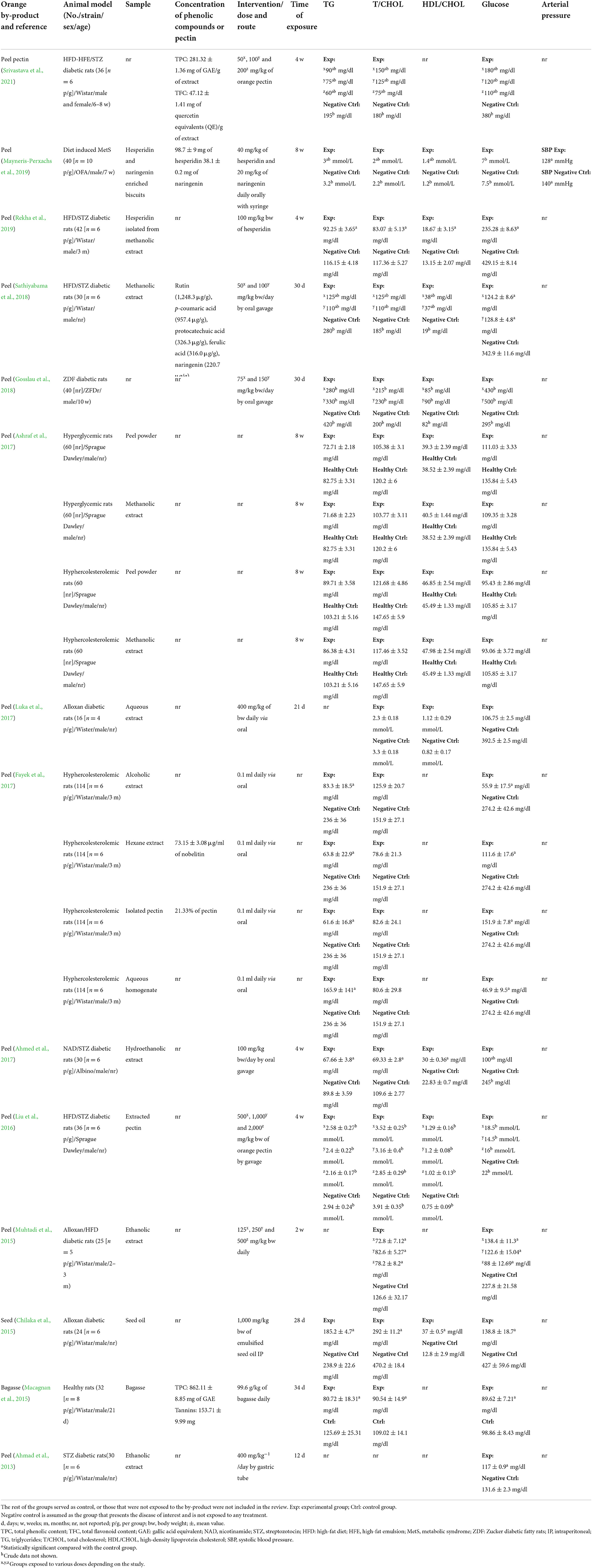
Table 1. Characteristics of the in vivo rodent studies and the effect of orange (Citrus sinensis) by-products.
Characteristics of animal models
Most of the studies used a pathological model of chronic diseases. Overall, 35.7% of the studies used genetically and chemically induced diabetic rats (Ahmad et al., 2013; Chilaka et al., 2015; Ahmed et al., 2017; Luka et al., 2017; Gosslau et al., 2018); another 35.7% of studies used rat models induced by ingestion of high-fat diets with chemical induction by streptozotocin or alloxan (Muhtadi et al., 2015; Liu et al., 2016; Sathiyabama et al., 2018; Srivastava, 2018; Rekha et al., 2019); one study used rats with diet-induced metabolic syndrome (Mayneris-Perxachs et al., 2019); two studies used hyperglycemic and hypercholesterolemic rodents (Ashraf et al., 2017; Fayek et al., 2017); and one study evaluated the effect of orange bagasse in healthy rats (Macagnan et al., 2015).
Experimental groups with 4–10 rats per group were integrated; 57% of the studies had groups composed of six animals. Wistar rats were used in most of the studies (Ahmad et al., 2013; Chilaka et al., 2015; Macagnan et al., 2015; Muhtadi et al., 2015; Ahmed et al., 2017; Fayek et al., 2017; Luka et al., 2017; Sathiyabama et al., 2018; Srivastava, 2018; Rekha et al., 2019), followed by Sprague Dawley (Liu et al., 2016; Ashraf et al., 2017), OFA (Mayneris-Perxachs et al., 2019), and Zucker diabetic fatty rats (Gosslau et al., 2018). Male rodents were used in 92.85% of the studies, and one study used rats of both sexes (Srivastava, 2018). The age of the rats ranged between 3 and 12 weeks (Macagnan et al., 2015; Muhtadi et al., 2015; Fayek et al., 2017; Gosslau et al., 2018; Srivastava, 2018; Mayneris-Perxachs et al., 2019; Rekha et al., 2019), whereas 50% of studies did not report the age of experimental animals.
Orange by-product intervention
Peel was the most used by-product (Ahmad et al., 2013; Muhtadi et al., 2015; Liu et al., 2016; Ahmed et al., 2017; Ashraf et al., 2017; Fayek et al., 2017; Luka et al., 2017; Gosslau et al., 2018; Sathiyabama et al., 2018; Srivastava, 2018; Mayneris-Perxachs et al., 2019; Rekha et al., 2019), and some studies also used seed (Chilaka et al., 2015) and bagasse (Macagnan et al., 2015). In most research studies, an extract from the by-product was used, including methanolic, alcoholic, hexane, hydroethanolic, and aqueous extracts, whereas in other studies, a part of the by-product was obtained, for example, pectin from orange peel.
Some studies determined and quantified phenolic compounds and pectin from the by-products (Macagnan et al., 2015; Fayek et al., 2017; Sathiyabama et al., 2018; Srivastava, 2018; Mayneris-Perxachs et al., 2019). The preferred route of administration was orally by gavage or syringe, whereas seed oil was administered intraperitoneally (Chilaka et al., 2015). Various doses were evaluated on the basis of milligrams per kilogram body weight. The effect of low, medium, and high doses was compared (Gosslau et al., 2018; Sathiyabama et al., 2018; Srivastava, 2018). The time of exposure varied between 12 days and 8 weeks; exposure for 4 weeks was the most frequently used (Chilaka et al., 2015; Liu et al., 2016; Ahmed et al., 2017; Srivastava, 2018; Rekha et al., 2019).
Effect on metabolic biomarkers
Overall, 78.6% of the articles reported levels of triglycerides, total cholesterol, and glucose levels, while arterial pressure was reported in one article (Mayneris-Perxachs et al., 2019). Regardless of the control group with which it was compared, the effect of orange by-products was always reported to be lower in the group exposed to orange.
Discussion
Orange represents 58% of global citrus production. After its industrial processing, around a half of its weight is discarded as waste, contributing to environmental pollution (Pacheco et al., 2019). This represents a great challenge for agroindustry; therefore, current research efforts focus on considering these wastes as potential products to obtain cost-efficient and promising nutraceuticals.
Derived from these arguments, this research focused on collecting scientific evidence of the assessment of the potential biological effect reported by the studies on orange by-products through a systematic review.
The results indicated that studies on orange residues are scarce. This review collected only 14 studies that met the eligibility criteria. Another aspect to consider is that this research was interested in experiments conducted in rodents as models as rats are the most widely used animal model in medical research since their pathophysiology is close to that of humans as well as since their genome is similar to the human genome (Iannaccone and Jacob, 2009; Blais et al., 2017). Overall, 92.8% of studies included in this review used models that mimic conditions of human chronic diseases.
Undoubtedly, animal experimentation provides various advantages; however, transferring the results to clinical practice in humans has been widely debated. Systematic reviews help collect the information needed for making evidenced-based decisions. Tools such as SYRCLE RoB allows for assessing the methodological quality of studies. When we applied this tool for the review, we noticed that the lack of information in the studies made the assessment of their quality unclear and that randomization bias represented a high RoB for the results, which prohibits most of animal studies from translating their results to human trials (van der Worp et al., 2010).
In addition, it is important to highlight that half of the studies reviewed did not indicate the age of their experimental subjects. Data on the age of the animal is essential since the attempt to transfer the results to human beings requires identifying the stage of animal life in which the results were obtained and it has also been shown that the age of the animal plays an important role in the modeling of human diseases (Ghasemi et al., 2021). It has been calculated that in adulthood, 1 day in the life of the rat is equivalent to 34.8 human days (Sengupta, 2013).
On the other hand, the bio-functionality of orange by-products requires evidence of its biosafety. In relation to this, the toxicity of Citrus sinensis has been evaluated. Li et al. determined the safety of hesperidin isolated from the methanolic extract of dried peel. They concluded that hesperidin is lethal at a median dose of 4,837.5 mg/kg and showed a low adverse effect at 1,000 mg/kg in the studied rats (Li et al., 2019), while Unanma et al. showed using the test of acute toxicity of the citrus methanol peel extract that a dose of 5,000 mg/kg was tolerable (Unanma et al., 2021).
The peel was extensively reported in the studies probably because this derivative is the major by-product, representing 50%−65% of the total weight of the citrus (Pacheco et al., 2019; Ricci et al., 2019). The citrus fruit peel contains more antioxidant compounds than pulp and seeds (Zayed et al., 2021). Also, the peel has been used to extract essential oils, carotenoids, pectin, and flavonoids. Some of the compounds that have been identified in orange peel are nobiletin, aglycone quercetin, diosmin, naringenin, rutin, hesperidin, naringin, among others, with naringin and hesperidin being the two major flavonoid glycosides present in citrus (El-Kersh et al., 2021; Saini et al., 2022).
The fiber and phenolic compounds present in the fruit contribute to biological effects obtained from orange by-products. Anti-diabetic effects have been associated with flavonoids, which are widely found in citrus peels (Ahmed et al., 2017). Soluble fiber and pectin extracted from orange peel have been associated with glucose improvement and lower plasma lipid levels (Srivastava et al., 2021). Ahmed et al. (2017) demonstrated the anti-hyperglycemic, anti-hyperlipidemic, and antioxidant effects in diabetic rats due to the content of naringin and naringenin present in the orange peel extract. Some of the mechanisms of action are the improvement in excretion and tissue sensitivity of insulin, which could be due to improved expression of insulin, adiponectin, and GLUT4 receptors (Ahmed et al., 2017). The same compounds extracted from lemon peel exerted an inhibitory effect on lipase, α-amylase, and α-glucosidase (Gavahian et al., 2022), while hesperidin showed a protective effect against oxidative stress in embryos of diabetic pregnant rats and an inhibitory effect in the Cu2+-induced oxidation of low-density lipoprotein in an in vitro study. Naringin also demonstrated an antioxidant effect and led to an increase in the effect of enzymes like catalase and superoxide dismutase in case of tissue injuries (Zhou et al., 2015). Sweet orange peel extract has been shown to lower the lipid profile by inhibiting the activity of the NPC1L1 gene in the intestinal wall, which can be attributed to the flavonoid content, especially hesperidin (Samsudin et al., 2017). Similarly, Sathiyabama et al. (2018) reported an antilipidemic effect of orange peel extract and also a protective effect on the pancreatic β-cell structure and function (Sathiyabama et al., 2018). Seeds of fruits are an important source of compounds such as tocopherols (α-tocopherol), phytosterols (β-sitosterol, campesterol, and stigmasterol), and carotenoids (α and β carotenes). The antioxidant activity of oils derived from different varieties of orange seeds was correlated with the α-tocopherol content (Jorge et al., 2016).
Overall, total phenolic compounds, total flavonoid content, and compounds hesperidin, eriocitrin, narirutin, didymin, tangeretin, sinensetin, nobiletin, limonene, myrcene, β-pinene, β-ocimene, and sabinene from Citrus sinensis juice, pulp, seed, peel, and leaves have been associated with antioxidant activity, inhibition of peroxidation, and inhibition of α-amylase and β-glucosidase (Leporini et al., 2021). These properties have advantage in the treatment of non-communicable diseases.
Of the studies reviewed, positive effects were observed in the physiological parameters evaluated since the experimental groups exposed to the orange by-product presented a reduction in the levels of triglycerides, total cholesterol, glucose, and systolic blood pressure, while HDL cholesterol increased (see Table 1). These findings were observed both in the pathological models of rats and in the healthy experimental groups, so we suggest orange by-products could be considered not only for the treatment of chronic diseases but also as a preventive approach both in healthy people and those with risk factors.
The proposed mechanisms of actions related to the biological effects of orange are diverse. The by-products have demonstrated to improve glucose levels via insulinotropic effects by enhancing the GLUT4 insulin receptor and adiponectin expression in adipose tissue (Ahmed et al., 2017), reduce the formation of advanced glycation end products (AGEs) via their capacity to inhibit the glycation activity (Deve et al., 2014), and delay the absorption and digestion of carbohydrates by soluble fibers that increase the viscosity of the food and disturb the diffusion of glucose, and, on the other hand, demonstrated the possibility of the fiber to inhibit α-amylase enzyme activity (Macagnan et al., 2015; Huang et al., 2019). Fruit fiber is related to the reduction of triglycerides through the secondary increase in insulin derived from absorption of glucose and the increase in lipoprotein lipase activity (Macagnan et al., 2015). These positive effects are also attributed to the mediation of lipid and cholesterol homeostasis by altering liver lipid metabolites and even regulating genes (SREBP-1c, ACC, and FAS) related to lipid metabolism (Feng et al., 2020).
It is important to mention that Citrus sinensis exerted similar results obtained by common drugs in the treatment of metabolic diseases (Chilaka et al., 2015; Sathiyabama et al., 2018). The results obtained from experimental animals increase the knowledge of the disease and the mechanism of action of treatments, including those from natural sources. Nevertheless, the disparity between the results in animals and those in clinical trials remains controversial due to various aspects in both types of studies (van der Worp et al., 2010). Therefore, to predict the results of clinical trials, these results should be tested in human beings in future.
Finally, the studies collected on the use of orange by-products, either through the recovery of bioactive compounds or through the incorporation of these elements in functional foods, strengthen the global sustainable food agenda. Researchers are currently working on incorporating new and more efficient innovative methods to use fruit waste in ecological and sustainable food chains. The purpose of recovering bioactive compounds from waste should follow processes such as extraction, isolation, and characterization of bioactive components. To properly harness its biological potential, appropriate steps must be taken to ensure that bioactive components are not destroyed, lost, or altered during the extraction process. Thus, in addition to studies of the health effects of these wastes, lines of biotechnological research should be strengthened to identify new “green” extraction techniques for the recovery of specific bioactive compounds and in order to improve its performance through time- and resource-efficient methods. In this context, an interdisciplinary study is appreciated for the use of resources, food issues, and their pathologies, as well as biosafety and consumption limits. It is important to consider new research directions for the use of citrus fruit residues since it not only impacts the problem of its disposal but also provides solutions to eliminate the resulting contamination. The final goal should be closer to a responsible use of fruit waste that leads to zero waste.
Conclusion
Although there are only few studies that have evaluated the functional properties of orange by-products, based on this systematic review of the biological effects of by-products, the peel was found to be the most used derivative, and positive effects on the lipid profile and glucose levels have been reported in the intervention with animal models. These properties have advantage in the treatment of non-communicable diseases. Despite the fact that the results have been favorable in terms of the functional effect of orange by-products, methodological quality assessed using the SYRCLE RoB revealed the lack of information from studies, which represents a high risk of bias for translational results. It would be important for researchers to note this tool when planning animal interventions.
Data availability statement
The original contributions presented in the study are included in the article/supplementary material, further inquiries can be directed to the corresponding author.
Author contributions
JP-L, VF-B, AM-M, and CV-C: conceptualization, investigation, writing—original draft, writing—review and editing, and project administration. JP-L and CV-C: methodology and visualization. VF-B and CV-C: formal analysis. JP-L and AM-M: resources. VF-B and AM-M: supervision. All authors have read and agreed to the published version of the manuscript.
Acknowledgments
The authors thank to the Departamento de Promoción, Preservación y Desarrollo de la Salud, Departamento de Ciencias Exactas y Metodologías and Departamento de Ciencias Sociales of the Centro Universitario del Sur de la Universidad de Guadalajara, Mexico.
Conflict of interest
The authors declare that the research was conducted in the absence of any commercial or financial relationships that could be construed as a potential conflict of interest.
Publisher's note
All claims expressed in this article are solely those of the authors and do not necessarily represent those of their affiliated organizations, or those of the publisher, the editors and the reviewers. Any product that may be evaluated in this article, or claim that may be made by its manufacturer, is not guaranteed or endorsed by the publisher.
References
Adubofuor, J., Akyereko, Y. G., Batsa, V., Apeku, O.-J. D., Amoah, I., Diako, C., et al. (2021). nutrient composition and physical properties of two orange seed varieties. Int. J. Food Sci. 2021, 1–11. doi: 10.1155/2021/6415620
Afrin, S. M., Acharjee, A., and Sit, N. (2022). Convective drying of orange pomace at different temperatures and characterization of the obtained powders. J. Food Sci. Technol. 59, 1040–1052. doi: 10.1007/s13197-021-05108-2
Ahmad, M., Ansari, M. N., Alam, A., and Khan, T. H. (2013). Oral dose of citrus peel extracts promotes wound repair in diabetic rats. Pak. J. Biol. Sci. 16, 1086–1094. doi: 10.3923/pjbs.2013.1086.1094
Ahmed, O. M., Hassan, M. A., Abdel-Twab, S. M., and Abdel Azeem, M. N. (2017). Navel orange peel hydroethanolic extract, naringin and naringenin have anti-diabetic potentials in type 2 diabetic rats. Biomed. Pharmacother. 94, 197–205. doi: 10.1016/j.biopha.2017.07.094
Ashraf, H., Butt, M. S., Iqbal, M. J., and Suleria, H. A. R. (2017). Citrus peel extract and powder attenuate hypercholesterolemia and hyperglycemia using rodent experimental modeling. Asian Pac. J. Trop. Biomed. 7, 870–880. doi: 10.1016/j.apjtb.2017.09.012
Ayala, J. R., Montero, G., Coronado, M. A., García, C., Curiel-Alvarez, M. A., León, J. A., et al. (2021). Characterization of orange peel waste and valorization to obtain reducing sugars. Molecules 26, 1348. doi: 10.3390/molecules26051348
Blais, E. M., Rawls, K. D., Dougherty, B. V., Li, Z. I., Kolling, G. L., Ye, P., et al. (2017). Reconciled rat and human metabolic networks for comparative toxicogenomics and biomarker predictions. Nat. Commun. 8, 14250. doi: 10.1038/ncomms14250
Chilaka, K. C., Ifediba, E. C., and Ogamba, J. O. (2015). Evaluation of the effects of Citrus sinensis seed oil on blood glucose, lipid profile and liver enzymes in rats injected with alloxan monohydrate. J. Acute Dis. 4, 129–134. doi: 10.1016/S2221-6189(15)30022-6
de Castro, L. A., Lizi, J. M., das Chagas, E. G. L., de Carvalho, R. A., and Vanin, F. M. (2020). From orange juice by-product in the food industry to a functional ingredient: application in the circular economy. Foods 9, 593. doi: 10.3390/foods9050593
de Moraes Crizel, T., Jablonski, A., de Oliveira Rios, A., Rech, R., and Flôres, S. H. (2013). Dietary fiber from orange byproducts as a potential fat replacer. LWT - Food Sci. Technol. 53, 9–14. doi: 10.1016/j.lwt.2013.02.002
Deve, A. S., Kumar, T. S., Kumaresan, K., and Rapheal, V. S. (2014). Extraction process optimization of polyphenols from Indian Citrus sinensis- as novel antiglycative agents in the management of diabetes mellitus. J. Diabetes Metab. Disord. 13. doi: 10.1186/2251-6581-13-11
El-Kersh, D. M., Ezzat, S. M., Salama, M. M., Mahrous, E. A., Attia, Y. M., Ahmed, M. S., et al. (2021). Anti-estrogenic and anti-aromatase activities of citrus peels major compounds in breast cancer. Sci. Rep. 11, 7121. doi: 10.1038/s41598-021-86599-z
El-safy, S., Salem, R., and Abd El-Ghany, M. (2012). Chemical and nutritional evaluation of different seed flours as novel sources of protein. World J. Dairy Food. Sci. 7, 59–65. doi: 10.5829/idosi.wjdfs.2012.7.1.61215
Ezejiofor, T. L. N., Eke, N. V., Okechukwu, R. I., Nwoguikpe, R. N., and Duru, C. M. (2011). Waste to wealth: industrial raw materials potential of peels of Nigerian sweet orange (Citrus sinensis). Afr. J. Biotechnol. 10, 6257–6264. doi: 10.5897/AJB10.1931
Fayek, N. M., El-Shazly, A. H., Abdel-Monem, A. R., Moussa, M. Y., Abd-Elwahab, S. M., El-Tanbouly, N. D., et al. (2017). Comparative study of the hypocholesterolemic, antidiabetic effects of four agro-waste citrus peels cultivars and their HPLC standardization. Rev. Bras. Farmacogn. 27, 488–494. doi: 10.1016/j.bjp.2017.01.010
Feng, K., Zhu, X., Liu, G., Kan, Q., Chen, T., Chen, Y., et al. (2020). Dietary citrus peel essential oil ameliorates hypercholesterolemia and hepatic steatosis by modulating lipid and cholesterol homeostasis. Food Funct. 11, 7217–7230. doi: 10.1039/D0FO00810A
Fernández-López, J., Sendra-Nadal, E., Navarro, C., Sayas, E., Viuda-Martos, M., Alvarez, J. A. P., et al. (2009). Storage stability of a high dietary fibre powder from orange by-products. Int. J. Food Sci. Technol. 44, 748–756. doi: 10.1111/j.1365-2621.2008.01892.x
Food Agriculture Organization of the United Nations. (2022). Citrus. Markets and Trade. Available online at: https://www.fao.org/markets-and-trade/commodities/citrus/en/ (accessed June 6, 2022).
Garcia-Castello, E. M., Rodriguez-Lopez, A. D., Conidi, C., and Cassano, A. (2022). “Valorization of citrus by-products by membrane processes,” in Membrane Engineering in the Circular Economy, eds A. Lulianelli, C. Conidi, A. Cassano, and K. Petrotos (Amsterdam: Elsevier), 413–436. doi: 10.1016/B978-0-323-85253-1.00009-5
Gavahian, M., Yang, Y.-H., and Tsai, P.-J. (2022). Power ultrasound for valorization of Citrus limon (cv. Eureka) waste: effects of maturity stage and drying method on bioactive compounds, antioxidant, and anti-diabetic activity. Innov. Food Sci. Emerg. Technol. 79, 103052. doi: 10.1016/j.ifset.2022.103052
Ghasemi, A., Jeddi, S., and Kashfi, K. (2021). The laboratory rat: age and body weight matter. EXCLI J. 20, Doc1431; ISSN 1611–2156. doi: 10.17179/excli2021-4072
Gosslau, A., Zachariah, E., Li, S., and Ho, C. T. (2018). Effects of a flavonoid-enriched orange peel extract against type 2 diabetes in the obese ZDF rat model. Food Sci. Hum. Wellness 7, 244–251. doi: 10.1016/j.fshw.2018.10.001
Hooijmans, C. R., Rovers, M. M., de Vries, R. B., Leenaars, M., Ritskes-Hoitinga, M., Langendam, M. W., et al. (2014). SYRCLE's risk of bias tool for animal studies. BMC Med. Res. Methodol. 14, 43. doi: 10.1186/1471-2288-14-43
Huang, Y. L., Ma, Y. S., Tsai, Y. H., and Chang, S. K. C. (2019). In vitro hypoglycemic, cholesterol-lowering and fermentation capacities of fiber-rich orange pomace as affected by extrusion. Int. J. Biol. Macromol. 124, 796–801. doi: 10.1016/j.ijbiomac.2018.11.249
Iannaccone, P. M., and Jacob, H. J. (2009). Rats! Dis. Model. Mech. 2, 206–210. doi: 10.1242/dmm.002733
Jorge, N., da Silva, A. C., and Aranha, C. P. M. (2016). Antioxidant activity of oils extracted from orange (Citrus sinensis) seeds. An. Acad. Bras. Ciênc. 88, 951–958. doi: 10.1590/0001-3765201620140562
Leporini, M., Tundis, R., Sicari, V., and Loizzo, M. R. (2021). Citrus species: modern functional food and nutraceutical-based product ingredient. Ital. J. Food Sci. 33, 63–107. doi: 10.15586/ijfs.v33i2.2009
Li, Y., Kandhare, A. D., Mukherjee, A. A., and Bodhankar, S. L. (2019). Acute and sub-chronic oral toxicity studies of hesperidin isolated from orange peel extract in Sprague Dawley rats. Regul. Toxicol. Pharmacol. 105, 77–85. doi: 10.1016/j.yrtph.2019.04.001
Liu, Y., Dong, M., Yang, Z., and Pan, S. (2016). Anti-diabetic effect of citrus pectin in diabetic rats and potential mechanism via PI3K/Akt signaling pathway. Int. J. Biol. Macromol. 89, 484–488. doi: 10.1016/j.ijbiomac.2016.05.015
Luka, C., Istifanus, G., George, M., and Philip, C. (2017). The effect of aqueous extract of Citrus sinensis peel on some biochemical parameters in normal and alloxan-induced diabetic wister rats. Am. J. Phytomed. Clin. Ther. 5, 17. doi: 10.21767/2321-2748.100330
Macagnan, F. T., Santos, L. R. D., Roberto, B. S., Moura, F. A. D., Bizzani, M., Silva, L. P. D., et al. (2015). Biological properties of apple pomace, orange bagasse and passion fruit peel as alternative sources of dietary fibre. Bioact. Carbohydr. Diet. Fibre 6, 1–6. doi: 10.1016/j.bcdf.2015.04.001
Malacrida, C. R., Kimura, M., and Jorge, N. (2012). Phytochemicals and antioxidant activity of citrus seed oils. Food Sci. Technol. Res. 18, 399–404. doi: 10.3136/fstr.18.399
Mayneris-Perxachs, J., Alcaide-Hidalgo, J. M., de la Hera, E., del Bas, J. M., Arola, L., and Caimari, A. (2019). Supplementation with biscuits enriched with hesperidin and naringenin is associated with an improvement of the metabolic syndrome induced by a cafeteria diet in rats. J. Funct. Foods 61, 103504. doi: 10.1016/j.jff.2019.103504
Muhtadi, M., Haryoto, H., Azizah, T., Suhendi, A., and Yen, K. (2015). Antidiabetic and antihypercholesterolemic activities of Citrus sinensis peel: in vivo study. Natl. J. Physiol. Pharm. Pharmacol. 5, 382. doi: 10.5455/njppp.2015.5.2807201561
Okoye, C. O. B., Ibeto, C. N., and Ihedioha, J. N. (2011). Preliminary studies on the characterization of orange seed and pawpaw seed oils. Am. J. Food Technol. 6, 422–426. doi: 10.3923/ajft.2011.422.426
Olabinjo, O. O., Ogunlowo, A. S., Ajayi, O. O., and Olalusi, A. P. (2017). Analysis of physical and chemical composition of sweet orange (Citrus sinensis) peels. Int. J. Environ. Agric. Biotechnol. 2, 2201–2206. doi: 10.22161/ijeab/2.4.80
Pacheco, M. T., Moreno, F. J., and Villamiel, M. (2019). Chemical and physicochemical characterization of orange by-products derived from industry. J. Sci. Food Agric. 99, 868–876. doi: 10.1002/jsfa.9257
Page, M. J., McKenzie, J. E., Bossuyt, P. M., Boutron, I., Hoffmann, T. C., Mulrow, C. D., et al. (2021). The PRISMA 2020 statement: an updated guideline for reporting systematic reviews. BMJ. 372, n71. doi: 10.1136/bmj.n71
Pan American Health Organization. (2022). Noncommunicable Diseases. Noncommunicable Diseases. Avaialbe online at: https://www.paho.org/en/topics/noncommunicable-diseases (accessed June 07, 2022).
Parmar, H. S., and Kar, A. (2008). Antiperoxidative, antithyroidal, antihyperglycemic and cardioprotective role of Citrus sinensis peel extract in male mice. Phytother. Res. 22, 791–795. doi: 10.1002/ptr.2367
Plasek, B., Lakner, Z., Kasza, G., and Temesi, Á. (2019). Consumer evaluation of the role of functional food products in disease prevention and the characteristics of target groups. Nutrients 12, 69. doi: 10.3390/nu12010069
Rekha, S. S., Pradeepkiran, J. A., and Bhaskar, M. (2019). Bioflavonoid hesperidin possesses the anti-hyperglycemic and hypolipidemic property in STZ induced diabetic myocardial infarction (DMI) in male Wister rats. J. Nutr. Intermed. Metab. 15, 58–64. doi: 10.1016/j.jnim.2018.12.004
Ricci, A., Diaz, A. B., Caro, I., Bernini, V., Galaverna, G., Lazzi, C., et al. (2019). Orange peels: from by-product to resource through lactic acid fermentation. J. Sci. Food Agric. 99, 6761–6767. doi: 10.1002/jsfa.9958
Saini, R. K., Ranjit, A., Sharma, K., Prasad, P., Shang, X., Gowda, K. G. M., et al. (2022). Bioactive compounds of citrus fruits: a review of composition and health benefits of carotenoids, flavonoids, limonoids, and terpenes. Antioxidants 11, 239. doi: 10.3390/antiox11020239
Samsudin, R. R., Kunsah, B., and Widyastuti, R. (2017). The effect of pacitan's sweet orange's (Citrus sinensis (L.) osbeck) peel powder on the lipid profile of male dyslipidemia rats (Rattus novergicus). Bali. Med. J. 6, 51. doi: 10.15562/bmj.v6i3.720
Sathiyabama, R. G., Gandhi, G. R., Denadai, M., Sridharan, G., Jothi, G., Sasikumar, P., et al. (2018). Evidence of insulin-dependent signalling mechanisms produced by Citrus sinensis (L.) Osbeck fruit peel in an insulin resistant diabetic animal model. Food Chem. Toxicol. 116, 86–99. doi: 10.1016/j.fct.2018.03.050
Sengupta, P. (2013). The laboratory rat: relating its age with human's. Int. J. Prev. Med. 4, 624–630.
Srivastava, R., Tripathi, L., Swain, S. R., and Singh, J. (2021). Neuroprotective validation of pectin in T2DM-induced allodynia and hyperalgesia in diabetic peripheral neuropathic pain. Arch. Physiol. Biochem. 23, 1–12. doi: 10.1080/13813455.2021.1884725
Srivastava, R. K. (2018). Need of nutraceuticals/functional food products for health benefits to world-wide people. J. Biotechnol. Biomed. Sci. 1, 1–13. doi: 10.14302/issn.2576-6694.jbbs-18-2408
U.S. Department of Agriculture. (2019). Oranges, raw, navels. Available online at: https://fdc.nal.usda.gov/fdc-app.html#/food-details/746771/nutrients (accessed April 14, 2022).
Unanma, H. C., Anaduaka, E. G., Uchendu, N. O., Ononiwu, C. P., and Ogugua, V. N. (2021). Ananas comosus and Citrus sinensis peels ameliorate CCl4-induced liver injury in Wistar rats. Sci Afr. 14:e01026. doi: 10.1016/j.sciaf.2021.e01026
United States Department of Agriculture. (2022). Citrus: World Markets and Trade. Available online at: https://usda.library.cornell.edu/concern/publications/w66343603?locale=en (accessed June 7, 2022).
van der Worp, H. B., Howells, D. W., Sena, E. S., Porritt, M. J., Rewell, S., O'Collins, V., et al. (2010). Can animal models of disease reliably inform human studies? PLoS Med. 7, e1000245. doi: 10.1371/journal.pmed.1000245
World Health Organization. (2022). Noncommunicable diseases: progress monitor 2022. Geneva: World Health Organization.Available online at: https://apps.who.int/iris/handle/10665/353048 (accessed September 4, 2022).
Zayed, A., Badawy, M. T., and Farag, M. A. (2021). Valorization and extraction optimization of citrus seeds for food and functional food applications. Food Chem. 355, 129609. doi: 10.1016/j.foodchem.2021.129609
Zayed, E. A., AinShoka, A. A., El Shazly, K. A., and Abd El Latif, H. A. (2018). Improvement of insulin resistance via increase of GLUT4 and PPARγ in metabolic syndrome-induced rats treated with omega-3 fatty acid or L-carnitine. J. Biochem. Mol. Toxicol. 32, e22218. doi: 10.1002/jbt.22218
Zhou, L., Pan, Y., Chonan, R., Batey, R., Rong, X., Yamahara, J., et al. (2015). Mitigation of insulin resistance by mangiferin in a rat model of fructose-induced metabolic syndrome is associated with modulation of CD36 redistribution in the skeletal muscle. J. Pharmacol. Exp. Ther. 356, 74–84. doi: 10.1124/jpet.115.229005
Keywords: Citrus sinensis, agri-food by-product, agri-food waste, phytochemicals, health effects, triglycerides, cholesterol, glucose
Citation: Pineda-Lozano JE, Fonseca-Bustos V, Martinez-Moreno AG and Virgen-Carrillo CA (2022) The biological effect of orange (Citrus sinensis L.) by-products on metabolic biomarkers: A systematic review. Front. Sustain. Food Syst. 6:1003144. doi: 10.3389/fsufs.2022.1003144
Received: 25 July 2022; Accepted: 15 September 2022;
Published: 02 November 2022.
Edited by:
Airton Kunz, EMBRAPA Swine and Poultry, BrazilReviewed by:
Pedro Ferreira Santos, University of Minho, PortugalSuzy Munir Salama, University of Malaya, Malaysia
Copyright © 2022 Pineda-Lozano, Fonseca-Bustos, Martinez-Moreno and Virgen-Carrillo. This is an open-access article distributed under the terms of the Creative Commons Attribution License (CC BY). The use, distribution or reproduction in other forums is permitted, provided the original author(s) and the copyright owner(s) are credited and that the original publication in this journal is cited, in accordance with accepted academic practice. No use, distribution or reproduction is permitted which does not comply with these terms.
*Correspondence: Carmen Alejandrina Virgen-Carrillo, Y2FybWVuLnZpcmdlbkBhY2FkZW1pY29zLnVkZy5teA==