- 1Guangdong Provincial Key Laboratory of Utilization and Conservation of Food and Medicinal Resources in Northern Region, Shaoguan University, Shaoguan, China
- 2Henry Fok College of Biology and Agriculture, Shaoguan University, Shaoguan, China
- 3Shaoguan Aromatic Plant Engineering Research Center, Shaoguan, China
The browning on the cut surface is a big problem reducing the quality of fresh-cut taro (FCT), which causes severe postharvest losses and may raise environmental impacts. Citronellal (CA) is a natural compound in several aromatic plants. This study investigated the effects of CA treatments in different concentrations (0.2, 0.5, and 1 mL/L) on the browning of FCT under cold storage at 5°C. The results indicated that low-dose (0.2 mL/L) CA treatment showed best anti-browning effects, reflecting at the maintained L* values but the reduced a*, b* and browning index values by CA treatment during cold storage. A repeated experiment using 0.2 mL/L CA confirmed fine anti-browning effects again. Furthermore, CA (0.2 mL/L) treatment markedly reduced the contents of total phenolic compounds and soluble quinones, restrained the activities of phenylalanine ammonia lyase, peroxidase and polyphenol oxidase, down-regulated the gene expression of 18 browning-related enzymes in FCT. These results together strongly demonstrate the validity of CA on FCT browning prevention. Given that CA is a natural compound existing in plants, low concentration of CA possesses strong anti-browning effects on FCT and also inhibitory effects on pathogens, implying that its application has potentiality to preserve high quality of fresh-cut produce for processing and storage. Moreover, CA treatment significantly decreased malondialdehyde contents and lipoxygenase activity. Correlation analysis indicated that the lipid peroxidation of cell membrane was mostly correlated with FCT browning. The results suggest that membrane lipid peroxidation was a possible reason for FCT browning and CA treatment reduced browning, in part, through alleviating the lipid peroxidation of cell membrane. Overall, our results demonstrate that CA is a novel browning mitigator for FCT under cold storage condition.
Introduction
The food industry shows increased interests in the innovation of products bringing health and convenience benefits (Rodríguez-Arzuaga et al., 2021). Fresh-cut foods (known as minimally processed products) are ready-to-eat healthy foods with a lot of advantages such as high nutrition, fresh appearance, great convenience, low waste and free pollution (María et al., 2020). Therefore, the economic importance of fresh-cut food market is becoming more remarkable (Alves et al., 2017; Zhang et al., 2020b). Nevertheless, the cut surface browning is still a big problem restricting the shelf life of many fresh-cut products including taro (Colocasia esculenta) during postharvest storage and consumption (Xiao et al., 2021), even though the products are stored under low temperature conditions (Mendoza-Enano et al., 2019). Taro is a staple food and/or root vegetable in many developing countries in the South Pacific, the Caribbeans, Asia, Central Africa, and South/Central America, due to the existence of abundant starch and other nutrients in root tissues (Bellinger et al., 2020). In China, fresh taro corms are used to manufacture delicious snacks, soups, or dishes after peeling (Xiao et al., 2022).
It is well-established that the main causes resulted in color degradation of fresh-cut products are enzymatic activity (Capotorto et al., 2017; Giannakourou and Tsironi, 2021). Mechanical operations such as handling and cut processing cause physical damages on cell tissues of fresh-cut foods, and enable the immediate interactions between substrates and enzymes under the existence of oxygen molecules (Giannakourou and Tsironi, 2021). The key enzymes involved in the reaction of enzymatic browning in fresh-cut foods include polyphenol oxidase (PPO), peroxidase (POD), phenylalanine ammonia lyase (PAL) and lipoxygenase (LOX; Qiao et al., 2021; Xiao et al., 2022). PPO and POD are two major pro-oxidative enzymes in plant, they involved in fresh product browning through contributing to phenolic oxidation to form dark-colored compounds (Rouet-Mayer et al., 1990; Ma et al., 2015; Sikora and Swieca, 2018; Zhu et al., 2022). Apart from PPO and POD, PAL activity positively correlates with the browning of fresh-cut products, as the enhanced activity can accelerate the process of phenolic biosynthesis (Banerjee et al., 2015). Unsaturated fatty acids are the major constituents of cell membrane (Kalappurakkal et al., 2020), and the oxidation of unsaturated fatty acids caused by LOX might be an important mechanism resulting in the browning of fresh-cut foods (Gao et al., 2017; Kan et al., 2019; Qiao et al., 2021). Therefore, the inhibition of the activities of those browning-related enzymes via the application of some chemicals may be a feasible strategy for the browning inhibition of fresh-cut products.
Dipping the cut products into solutions of anti-browning chemicals is the widest method of browning control in the fresh-cut industry (Rojas-Graü et al., 2007). Several compounds have been proposed to control fresh-cut product browning. For example, hydrogen sulfide is effective in reducing the surface browning of fresh-cut Chinese water chestnuts (Dou et al., 2021), lotus (Sun et al., 2015), apple (Chen et al., 2017), pears (Hu et al., 2014), and fresh litchi fruit (Siddiqui et al., 2021). Chlorine-containing solutions are widely used, and the efficacy of such solutions on browning inhibition of fresh-cut foods was evaluated in several food species including asparagus lettuce (Chen et al., 2010), apple (Fu et al., 2007), lotus (Du et al., 2009), and longan fruit (Intarasit et al., 2018). Electrolyzed water and ozone are also suggested to have potential anti-browning effects on fresh-cut foods (Ali et al., 2018). However, as the above-mentioned, the most commonly used compounds are chemicals which might pose potential risks to human health considering the food safety (Fan and Wang, 2022). Therefore, it is an important priority to develop more effective and safe browning inhibitors for fresh-cut products.
Several studies have been reported that some plant essential oils possess preventive effects on fresh-cut food browning. For example, clove essential oil was showed to prevent the browning of fresh-cut lettuce and table grapes (Talab and Gholami, 2012; Chen et al., 2017) by inhibiting browning-related enzyme activities. Tragacanth gum coating thyme essential oil delayed button mushroom browning (Nasiri et al., 2017). The application of essential oil extracted from pine leaves to fresh-cut lettuce significantly prevented the browning (Kim et al., 2014). These studies suggest that plant essential oils could be a resource of browning inhibitors controlling enzymatic browning of fresh-cut foods. Citronellal (CA) is one of the major components of citronella aromatic oil (Sharma et al., 2019). CA does not present a concern for genetic, developmental and reproductive toxicities based on the current existing data and usage levels (Api et al., 2021). It has been fully confirmed in many studies that CA displays strong antifungal activities against various pathogens in food system (Wu et al., 2016; Sharma et al., 2019; OuYang et al., 2021; Zhang et al., 2022). However, few studies were conducted to determine whether CA application could prevent fresh-cut food browning.
The purpose of this study was to assess CA effects on the browning inhibition of fresh-cut taro (FCT) under cold condition. In addition, CA-treatment-induced changes in browning indicators such as activities of PAL, PPO, LOX and POD, and contents of malondialdehyde (MDA), total phenolic compound (TPC) and soluble quinone (SQ) were investigated in FCT. The results from this study would lay a foundation for probable applications of CA in the fresh-cut industry.
Materials and methods
Material selection, processing, storage condition, and sampling
Taro (Colocasia esculenta cv Binglang) were harvested at a commercially ripe stage in a vegetable garden in Shaoguan city, Guangdong Province, China, in November and December 2020. In this stage, the corm expansion comes to a complete stop, nutrients are fully accumulated, and the aboveground leaves begin to turn yellow and even some wither seriously. Trial taros were selected based on the standard of uniform sizes, free physical damages and disease symptoms. Before treatment, taros were washed with running water and peeled using a peeler. Then the peeled corms were cut into equal slice with 1 cm thickness.
For treatments, the FCT pieces were dipped into distilled water (DW) and different concentrations of CA solutions (0.2, 0.5, or 1 mL/L) for 30 min, respectively, and dried at room temperature (25°C) for 20 min. Three replications were applied for each treatment, and each replication contained 30 slices. After treatment, DW- and CA-treated FCTs were hermetically wrapped with plastic bags (0.02 mm thickness), and then were stored at 5°C and 90–95% relative humidity for 12 days for progressive assessments. Each bag contained 10 thin pieces. The whole FCT slices were used to sampling, and samples were collected every 3 days during storage. The collected samples from the same treatment were pooled and ground to a powder in liquid nitrogen and stored at −80°C for subsequent measurements.
Color assessment on the surface of FCT
Color (CIELAB values) on the surface of FCT was quantitatively measured using a CR-400 Chroma meter (KONICA MINOLTA, Japan) calibrated by the standard white tile (Y = 84.4, x = 0.3205, and y = 0.3377; Xiao et al., 2022). Measurements were made at central area of the cut faces of each taro slice. Values of L*, a*, and b* were recorded in the Chroma meter, and the browning index (BI) was also evaluated according a formula previously established (Xiao et al., 2020).
Determination of total phenolic compound (TPC) and soluble quinone (SQ) and malondialdehyde (MDA) contents
TPC contents were measured basing on the Folin-Ciocalteu method (Xiao et al., 2022). Briefly, 1.0 g of taro samples were homogenized with a 5 mL of 1 % (v/v) HCl-methanol reagent and extracted at ambient temperature (25°C) for 3 h. Then, the extracts were centrifuged at 4°C (12,000 × g) for 10 min. The supernatant was collected as crush extracts for the determination of TPC content in taro samples. For determination, 0.5 mL of the supernatants, 1.5 mL of 1 M sodium carbonate, and 1.0 mL of Folin-Ciocalteu reagent were orderly added in a new tube and then were well-mixed. Then reaction mixture was reacted at 25°C for 30 min in the darkened environment. The absorbance of reaction solution was recorded at 765 nm with an UV-visible spectrophotometer (MAPADA UV-1800, China). A standard curve of gallic acid (GA) was established to quantify TPC contents as milligram GA equation per kilogram of fresh weight (mg kg−1 FW).
For the measurement of SQ concentrations, quinone extracts were prepared with 5.0 g of taro samples in a 10 mL of methanol at 25°C for 2 h. Then, the extract solutions were centrifuged at 12,000 × g for 10 min, and the collected supernatants were used to determine SQ contents. The absorbance of each supernatant at 437 nm was recorded using the above mentioned UV-visible spectrophotometer. The SQ concentrations of each sample were expressed as OD437 per gram of fresh weight (OD437 g−1 FW; Ali et al., 2021).
MDA contents in FCT were estimated with a commercial kit (D799761, Sangong Biotech, China) based on the manufacturer's specifications. The 0.3 g of taro powders from three different slices were added in 5 mL of trichloroacetic acid (5%) solution and then were fully mixed in ice bath. The mixtures were centrifuged (8,000 × g) at 4°C for 10 min and the supernatants were carefully collected before measurement. 0.2 mL of supernatant was mixed with 0.8 mL of thiobarbituric acid (1%) solution and boiled in a water bath for 60 min. Thereafter, boiled reactions were cooled to ambient temperature (25°C) and centrifuged at 10,000 × g. The absorbance of supernatant was noted at 600, 532, and 450 nm. The MDA content of taro samples was calculated using the following equation.
MDA content = [6.45 × (A532–A600) – 0.56 × A450] × Vt/(Vs × m) (Ali et al., 2021). Vs represents the extract volume required for measurement, while Vt represents the total volume of sample extract. The result of MDA concentration was expressed as μmol per kilogram of fresh weight (μmol kg−1 FW).
The activities of browning-related enzymes
Phenylalanine ammonia lyase (PAL) activity was determined according to a previous publication (Wang et al., 2022). Taro grinding liquid was composed of precooled borate buffer (pH 7.8, 0.2 M) and 2% (w/v) polyvinylpyrrolidone (PVP). The homogenates were centrifuged at 4°C (12,000 × g) for 10 min to collect the supernatants. For PAL activity measurement, 0.5 mL of the supernatants were admixed with 1.5 mL of 0.1 mM sodium borate (pH 8.8), 0.5 mL of 5 mM dithiothreitol and 0.5 mL of 0.02 M L-phenylalanine, and kept at 25°C for 2 h. The generation of trans-cinnamate was monitored at a wavelength of 290 nm using the above mentioned UV-visible spectrophotometer.
The crushed extracts of POD, PPO, and LOX were prepared before measurement with the method of Xiao et al. (2020). First, 1.0 g of taro samples were homogenized into a 5 mL of precooled sodium phosphate buffer (pH 7.0, 0.05 M) containing 0.1 g of PVP and centrifuged at 4°C (12,000 × g) for 15 min. After centrifugation, the supernatants were collected into a new centrifugal tube and used to determine enzyme activities.
For the POD activity assessment, a 3 mL of reaction mixture was composed of 0.1 mL of the supernatants, 2.7 mL of 0.1 M phosphate buffer (pH 7.0), 0.1 mL of 1% (v/v) guaiacol and 0.1 mL of 0.5% H2O2 (Xiao et al., 2022). The absorbance of mixture at 470 nm was continuously recorded every 1 min during the monitored 3 min.
For the PPO activity measurement, 0.2 mL of the supernatants and 0.1% (w/v) pyrocatechol were added in order and mixed well in a centrifugal tube. The absorbance of each mixture at 398 nm was recorded every 1 min and continuously detected for 3 min (Wang et al., 2022).
For LOX activity determination, 0.1 mL of the supernatants, 2.7 mL of 0.5 M borax-borate buffer (pH 7.0) and 0.2 mL of 5 mM linoleic acid solution were orderly added in a tube and mixed well. Taro LOX activity was measured by monitoring the formation of conjugated diene at 234 nm (Xiao et al., 2021).
The results of all enzyme activities were shown as units per kilogram of fresh weight (U kg−1 FW).
Gene expression analysis by real-time quantitative PCR (RT-qPCR)
Total RNA from taro slices was extracted using an RNA extraction kit (DP441, TianGen, China), and one strand of cDNA used for PCR amplification was synthesized using an cDNA synthesis kit (11123ES60, Yeasen, China) following the manufacturer's instructions (Xiao et al., 2022). RT-qPCR was used to analyze the expression of specific gene. RT-qPCR was performed through an iCyeler iQTM/Clooo system (Bio-Rad, USA) and a Hieff® qPCR SYBR Green Master Mix (No Rox) kit was employed (11201ES03, Yeasen, China). Taro Actin7 (GenBank: MQM19396.1) was used as the internal control to normalize relative expression level of each target gene. mRNA sequences of candidate genes for qRT-PCR analysis were isolated from the taro genome (https://www.ncbi.nlm.nih.gov/genome/12429) using BLAST searcher against published sequences. Primer information was listed in Supplementary Table 1.
Statistical analysis
All experiments in this study were randomly designed, and were repeated at least twice for each analysis under the same conditions. The results presented in this study was from one set of experiment. In all figures, data were presented as means and the vertical line above each column represents the standard error (SE) from nine or three replications. Statistical analysis on the data were performed by Duncan's multiple range test to determine significant differences between treatments (P < 0.05) in the SPSS software (version 25). Correlation analysis for control samples between browning-related indices was analyzed in the Microsoft Excel software (version 2016). Plots of correlation analysis was made by TBtool software (Chen et al., 2020) using the HeatMap lllustrator tool.
Results
Effects of citronellal treatments in different concentrations on FCT browning during cold storage
L*, a*, and b* values are suitable indicators for evaluating browning degree of fresh-cut products (Qiao et al., 2021). Higher L* but lower BI values mean that the product has less browning (Kim et al., 2014; Kan et al., 2019). In the present study, FCT browning was represented as the color values (L*, a*, b*, and BI) on cut-surfaces. To determine suitable CA treatment concentration reducing FCT browning, we first conducted a preliminary experiment using three concentrations (0.2, 0.5, and 1 mL/L).
The L* values of FCT tended to decline with increasing storage days (Figure 1A). Two CA treatments (0.5 and 1 mL/L) did not exhibit significant influences on L* values between the control (DW) (Figure 1A). However, the decline rate of L* values in 0.2 mL/L CA treatment were evidently lower than that in DW-treated samples (control). 0.2 mL/L CA-treated FCT showed significantly higher L* values than DW-treated taros after 3 days of cold storage (Figure 1A).
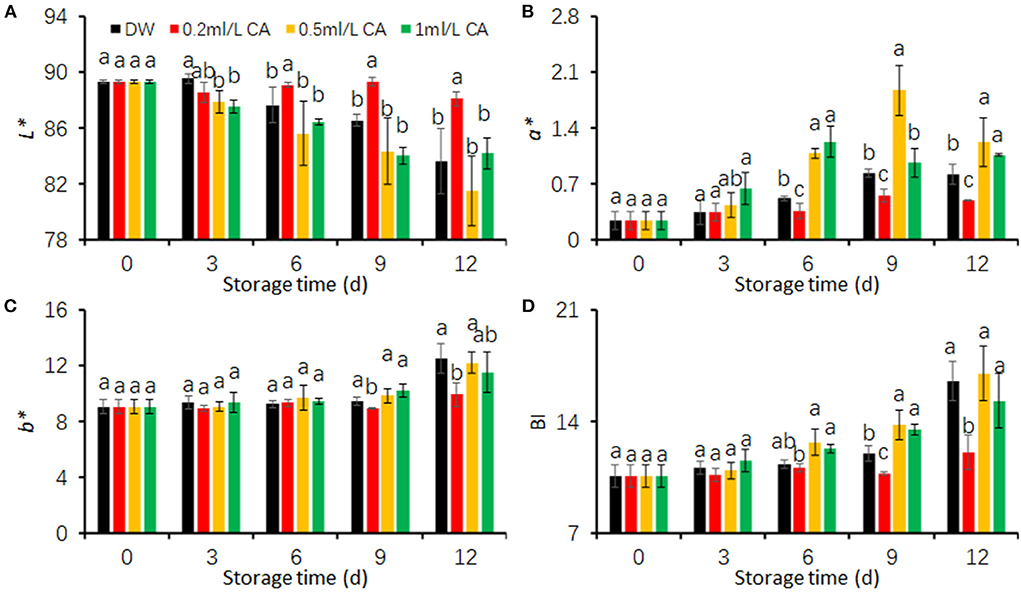
Figure 1. Effects of different concentrations (0.2, 0.5, and 1 mL/L) of citronellal (CA) treatments on the browning of fresh-cut taros during cold storage. (A) L* value; (B) a* value; (C) b* value; (D) browning index (BI). Fresh-cut taros were stored at 5°C during the entire storage. DW, distilled water. Each value is presented as the mean ± SE (n = 9). Statistical differences (p ≤ 0.05) are indicated using different letters above the bars.
a*, b*, and BI values of FCT in this study showed similar patterns during cold storage (Figures 1B–D). The values tended to raise with increasing storage duration. The raising rate of three browning indicators were significantly lower in the cut taros treated with 0.2 mL/L CA than in the other treatments (Figures 1B–D). The a* values were obviously higher in the slices treated with 0.5 and 1 mL/L CA during the last 6 days. These results together show that 0.2 mL/L CA treatment had a significant effect on maintaining fresh color of FCT. Therefore, CA concentration of 0.2 mL/L was used in the following experiment to investigate diverse effects of CA on FCT during cold storage.
To confirm the inhibitory effects of 0.2 mL/L CA treatment, a repeated experiment was conducted under the same conditions in accordance with those of the above experiment. As compared with the control, CA treatment at 0.2 mL/L concentration significantly reduced FCT browning, reflecting at higher L* values but lower a* and b* and BI values in CA-treated taros (Figures 2A–D). Microbial development was observed on the cut-surface of control slices (Figure 2E), implying that CA treatment also had adverse effects on pathogen growth especially mold. In the control, visual browning symptoms far from disease spot on the surface of taro disks were occurred at day 6 of storage at 5°C, but progressively aggravated during the remaining storage period. However, taro slices treated with 0.2 mL/L CA had a bright appearance throughout the whole storage period (Figure 2E). These results strongly demonstrate that 0.2 mL/L CA is effective in preventing FCT browning.
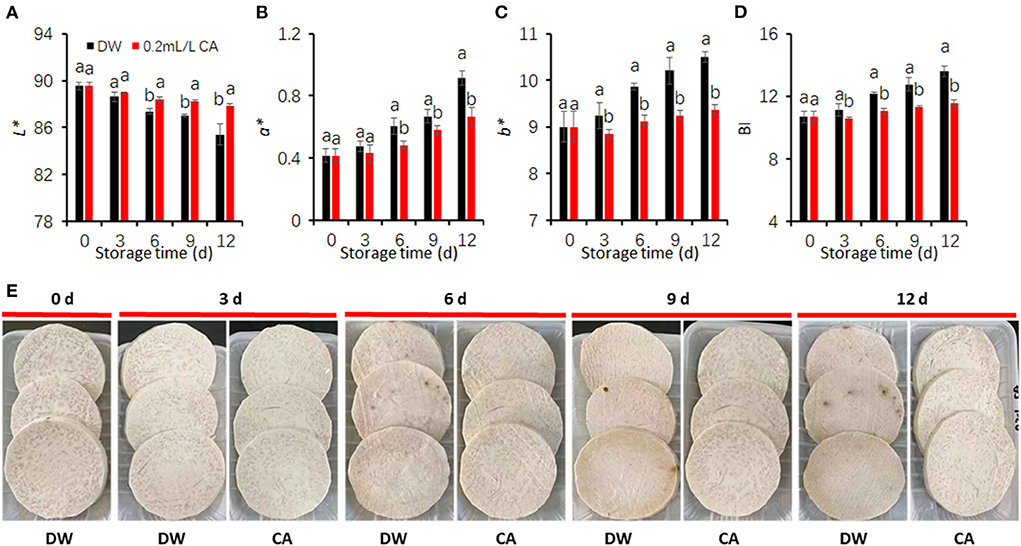
Figure 2. Inhibitory effects of 0.2 mL/L citronellal (CA) treatment on the browning of fresh-cut taros. (A) L* value; (B) a* value; (C) b* value; (D) browning index (BI); (E) representative pictures from DW and CA treated taros during cold storage. DW, distilled water. Each value is presented as the mean ± SE (n = 9). Statistical differences (p ≤ 0.05) are indicated as different letters above the bars.
Effects of citronellal treatment on phenolic oxidation in taro slices
In the control, TPC contents increased after peeling, from the initial value of 203.58–215.33 mg/kg at 12 d. However, CA (0.2 mL/L) treatment strongly inhibited the increase in TPC contents (Figure 3A). SQ contents in FCT tended to increase with increasing storage period. SQ contents in the control quickly increased during cold storage, whereas that in CA treatment increased slowly (Figure 3B). CA treatment (0.2 mL/L) significantly reduced SQ contents in FCT comparing with the control.
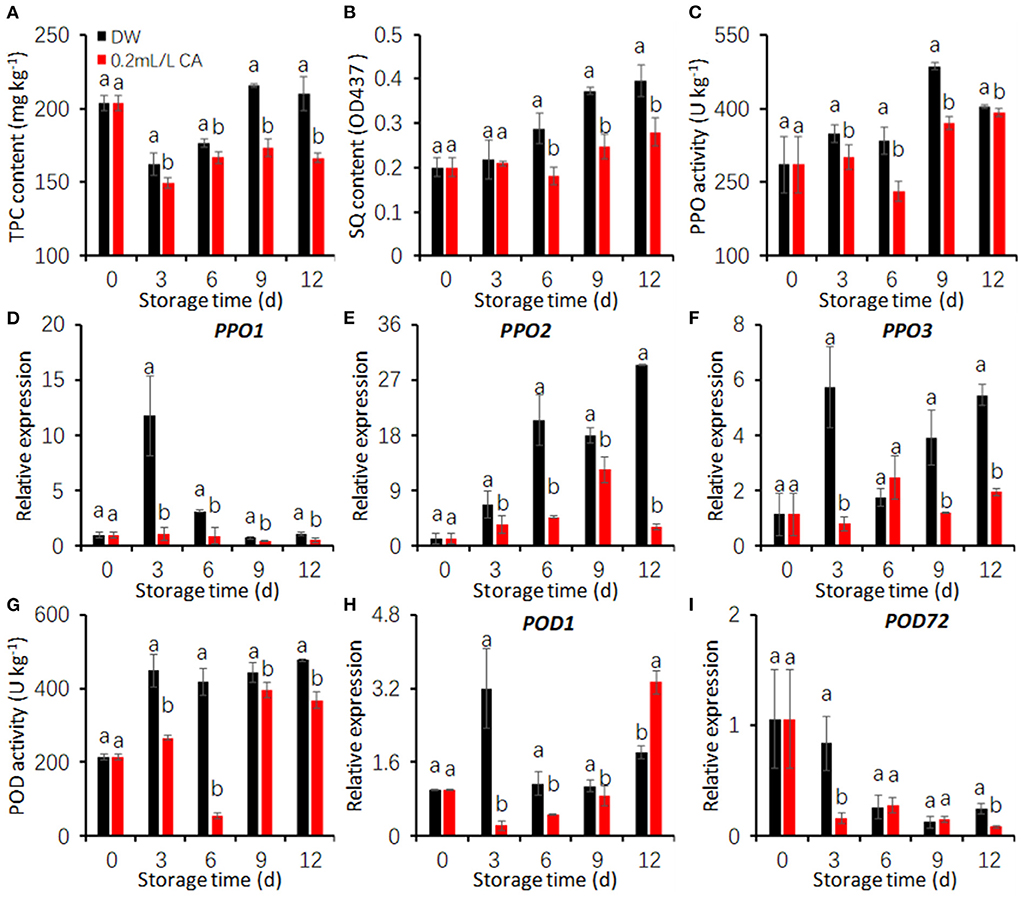
Figure 3. Effects of 0.2 mL/L citronellal (CA) treatment on the oxidation of phenolics in fresh-cut taros. (A) Total phenolic compound (TPC) content; (B) soluble quinone (SQ) content; (C–F) PPO activity and gene expression; (G–I) POD activity and gene expression. DW, distilled water. The expression levels were all normalized to 100% (1.0) at 0 d (before treatment). Each value is presented as the mean ± SE (n = 3). Statistical differences (p ≤ 0.05) are indicated as different letters above the bars.
PPO activities of FCT exhibited an overall increased trend during the entire storage (Figure 3C). Nevertheless, CA treatment significantly prevented the increase of PPO activity during the entire cold storage when compared with the control. The effects of CA treatment on the expression of three PPO genes were also assessed. CA treatment effectively repressed PPO1 and PPO2 expression during the entire storage, PPO3 expression during cold storage excepting at 6 d (Figures 3D–F).
In the control, POD activities drastically increased during the first 3 days and maintained at high levels during the later storage (Figure 3G). In CA treatment, POD activity mildly increased at 3 d, then suddenly dropped into very low levels at 6 d and sharply increased at 9 d. POD activity in CA treatment was significantly lower than that in the control group during the entire storage duration (Figure 3G). CA treatment markedly repressed POD1 expression in the first 9 days (Figure 3H), POD72 expression at 3 d and 12 d (Figure 3I).
Effects of citronellal treatment on the phenolic biosynthesis in FCT
Our previous studies suggested that taro browning may be associated with phenolic biosynthesis induced by cutting operations (Xiao et al., 2022). The phenylpropanoid pathway is the major approach to the synthesis of various phenols (Yao et al., 2021). To examine whether CA mitigator reduced taro browning by suppressing the phenolic biosynthesis, the effects of CA treatment on the activity and gene expression of key enzymes in the phenylpropanoid pathway were investigated.
PAL activities in both the control and CA treatment declined during the first 6 days and increased thereafter. PAL activities of CA treatment were significantly lower than that of the control during the last 9 days (Figure 4A). Additionally, CA treatment also restricted the expression of two PAL genes during storage excepting at 9 d (Figures 4B,C).
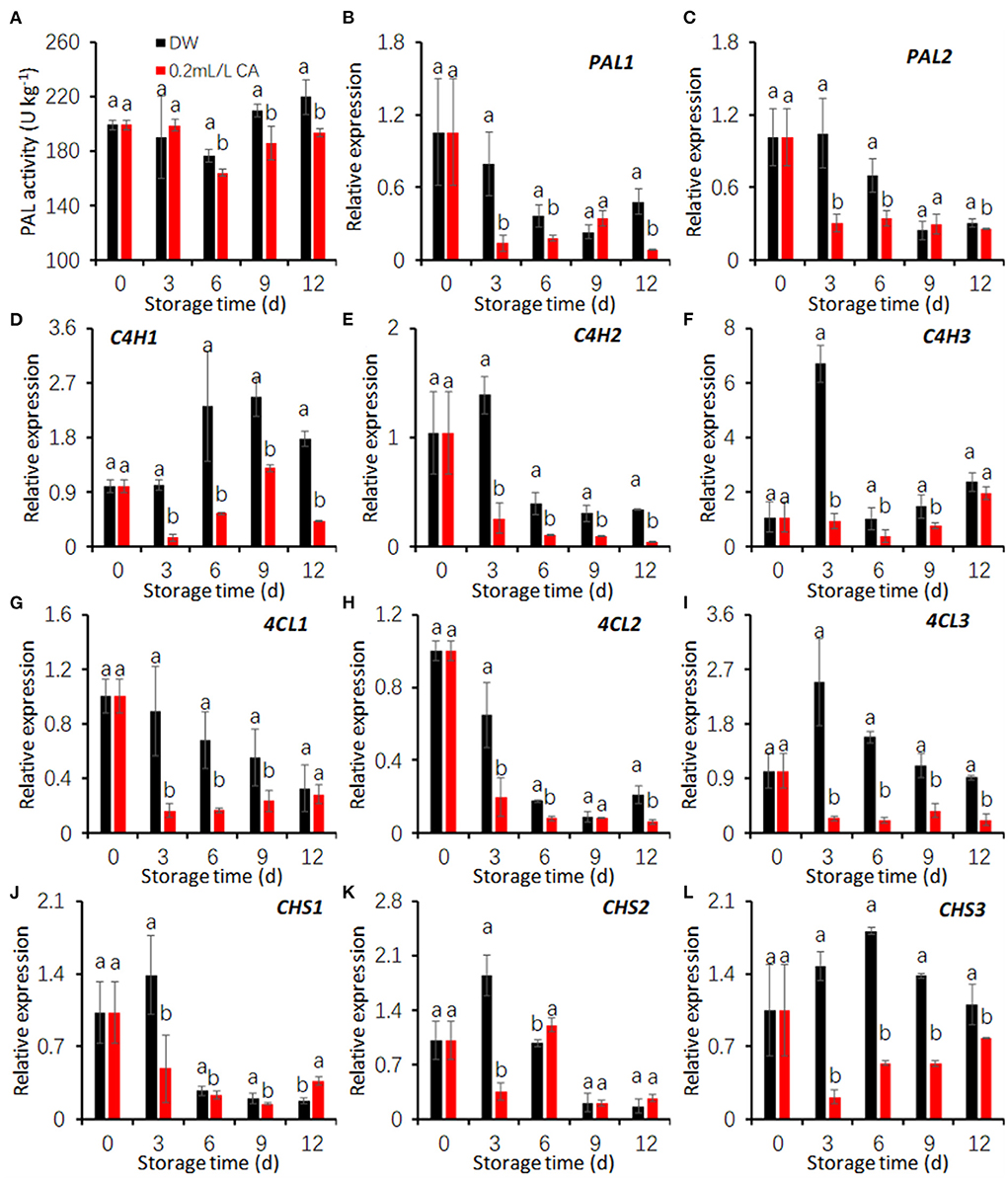
Figure 4. Effects of 0.2 mL/L citronellal (CA) treatment on the phenolic biosynthesis in fresh-cut taros. (A–C) PAL activity and gene expression; (D–F) relative expression of C4H genes; (G–I) relative expression of 4CL genes; (J–L) relative expression of CHI genes. DW, distilled water. The expression levels were all normalized to 100% (1.0) at 0 d (before treatment). Each value is presented as the mean ± SE (n = 3). Statistical differences (p ≤ 0.05) are indicated as different letters above the bars.
In the control group, the expression of three genes coding cinnamic acid-4-hydroxylase (C4H) were increased after cutting (Figures 4D–F). CA treatment markedly restricted the expression induction of three C4H genes during cold storage (Figures 4D–F) comparing with the control.
In both the control and CA groups, the expression of two genes encoding 4-coumarate: CoA ligase (C4L), C4L1, and C4L2, decreased with prolonging storage period (Figures 4G,H). The expression levels of C4L3 in the control first peaked at 3 d and fell hereafter, while that in CA treatment was decreased throughout storage (Figure 4I). Compared with the control, CA treatment significantly reduced the expression levels of three taro C4L genes (Figures 4G–I).
In the control group, the expression of three chalcone synthase (CHS) genes was induced by cutting at 3 d of storage at 5°C (Figures 4J–L). CA treatment significantly downregulated CHS1 during the first 9 days, and CHS2 expression at 3 d, and CHS3 expression during the whole storage (Figures 4J–L). The results suggest that CA treatment reduced the activities of key enzymes in the phenylpropanoid pathway by down-regulating the expression of coding genes.
Effects of citronellal treatment on MDA concentration and LOX activity
In two treatments, MDA concentrations continuously increased with storage time progressed (Figure 5A). However, CA treatment effectively prevented the increase in MDA concentrations during storage. Consequently, MDA contents in CA-treated samples were markedly lower than that in DW-treated samples (Figure 5A). Taro slices subjected to CA solutions showed 26.88% less MDA contents at day 12 when compared to the control.
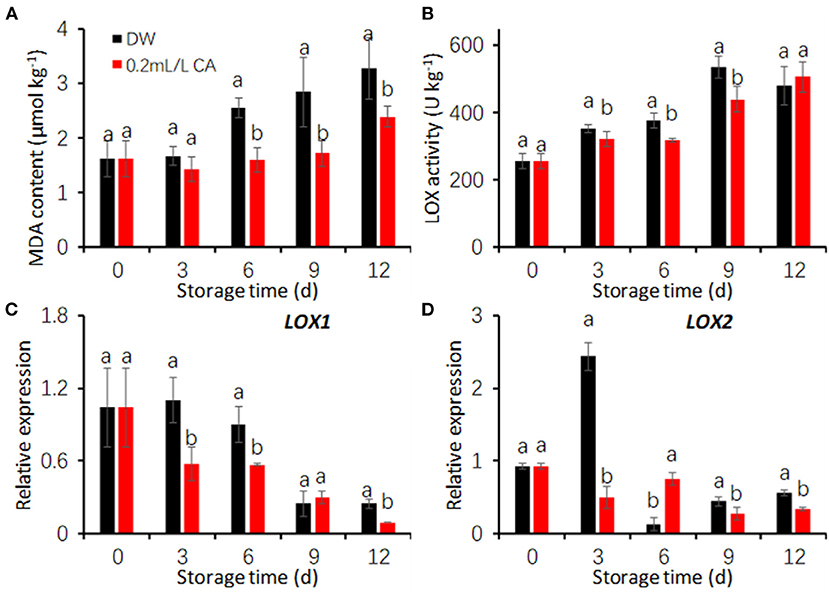
Figure 5. Effects of citronellal (CA) treatment on malondialdehyde (MDA) content (A) and LOX activity (B) and gene expression (C,D) in fresh-cut taros. DW, distilled water. The expression levels were all normalized to 100% (1.0) at 0 d (before treatment). Each value is presented as the mean ± SE (n = 3). Statistical differences (p ≤ 0.05) are indicated as different letters above the bars.
Similar to MDA content, LOX activity progressively increased with prolonging storage time in two treatments (Figure 5B). CA treatment significantly decreased LOX activities during the first 9 days comparing with the control (Figure 5B). In addition, the expression of two LOX genes, LOX1 and LOX2, were also repressed by CA treatment during cold storage (Figures 5C,D). The time-course trends of LOX activity and gene expression are opposite, suggesting that other member of LOX family genes could complement this and contribute to the higher enzyme activity during the late storage, as LOX is encoded by a multigene family (Porta and Rocha-Sosa, 2002).
Correlation analysis of browning-related indicators
Browning-related indices including L*, a*, b* and BI values, TPC and SQ and MDA content, and PPO, POD, PAL, and LOX activity from the control samples were employed to perform correlation analysis. As shown in Figure 6, the BI, a characteristic index of FCT browning, was positively correlated with the parameters of a* and b* values, MDA and SQ and TPC contents, and POD and LOX and PPO and PAL activities, but negatively correlated with L* value. BI had the highest Pearson correlations with b* value (r = 1.00) and L* (r = −0.99) and MDA content (r = 0.99) in the FCT, followed by a* value and SQ content (r = 0.98; Figure 6). The correlation coefficients between BI and LOX activity, POD activity, PPO activity, and PAL activity were 0.87, 0.72, 0.72, and 0.55, respectively (Figure 6). These results imply that the key enzymes involving in FCT browning were LOX, followed by PPO and POD, and PAL was the secondary factor affecting taro browning.
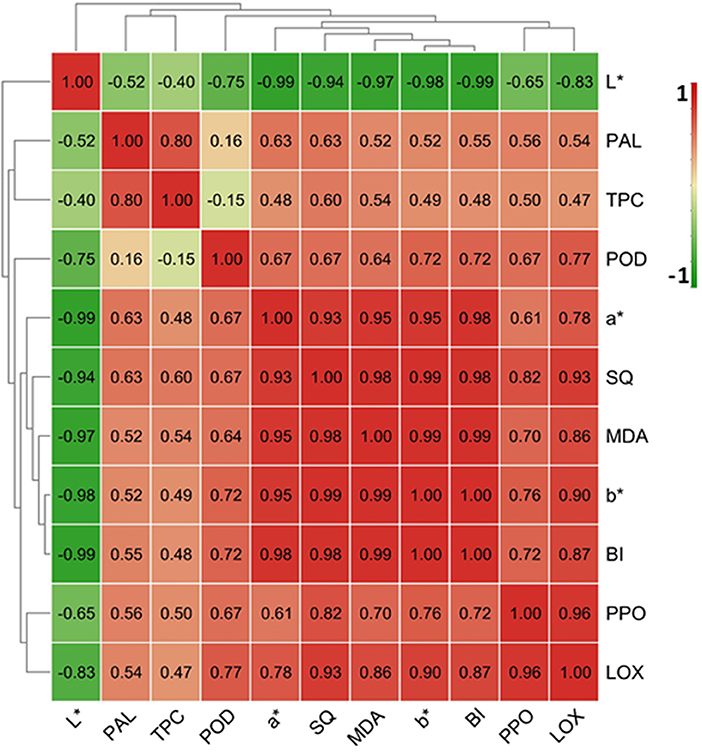
Figure 6. Correlation analysis of browning-related indicators in fresh-cut taro during cold storage. The map was made by TBtool software (Chen et al., 2020). The correlation coefficient between two browning-related indices was indicated in the map. The color code depicted at the right side of the image ranged from −1.0 (green) to 1.0 (red).
Discussion
Taro is the fifth root crop and the 14th vegetable in the world (Bellinger et al., 2020). However, the cut surface browning is one of the major causes for the deterioration of FCT roots during processing and storage (Xiao et al., 2022). Here, the inhibitory effects of different concentrations of CA treatments on taro browning were investigated during cold storage. The results presented in this study showed that 0.2 mL/L CA significantly prevented the development of enzymatic browning of taro corm slices (Figures 1, 2), suggesting that this concentration might be suitable for the browning prevention. More investigations are needed to determine whether lower CA concentration is effective. It also noted that CA treatments in 0.5 and 1 mL/L concentration displayed slightly aggravated browning (Figure 1). We guessed that high concentration of CA solution treatments might cause chemical injuries on FCT tissues, as CA could impair the integrity of plasma membrane of living organisms (Wu et al., 2016) and cause a severe phytotoxicity on some plants (Singh et al., 2006) at a high concentration.
The growth and development of pathogens, mainly bacteria and mold, on the surface of fresh-cut produce negatively affect the microbial safety (Fan and Wang, 2022), as many pathogens in food are capable of secreting a wide range of exotoxins which severely threaten consumers' health (Liu et al., 2022). Numerous studies have demonstrated that CA possesses strong antifungal activities against many pathogens in food (Sharma et al., 2019). The mechanisms of CA inhibiting pathogen development are probably that it causes plasma membrane damage (Wu et al., 2016) and inhibits the biosynthesis of ergosterol, an integral component of the cell membrane (OuYang et al., 2021), and inhibits hemolytic activities of pathogens (Zhang et al., 2022). In this study, we observed that 0.2 mL/L CA treatment seemed to restrict some pathogens development on the FCT slices (Figure 2E), suggesting that low-dose CA treatment improved the microbial safety of FCT under cold storage condition by directly inhibiting pathogen development. However, how CA treatment restrains pathogen development on the surface of FCT and it restricts which kind of specific pathogen should be investigated in the future.
The browning on cut-surface in most fresh-cut products is generally attributed to the oxidation of phenolic compounds by two oxidases, PPO and POD (Altunkaya and Gokmen, 2010; Kasnak, 2020). In the current study, 0.2 mL/L CA treatment reduced POD and PPO activities and gene expression and SQ contents in FCT (Figure 3), suggesting that CA treatment restricted phenolic oxidation to form black-dark quinones by inactivating PPO and POD activity. Correlation analysis for control samples in this study showed positive correlations between BI and both SQ contents (r = 0.98) and PPO (r = 0.72) and POD activities (r = 0.72; Figure 6). The application of 4-hexylresorcinol completely inhibited PPO activity and the browning of fresh-cut pears (Oms-Oliu et al., 2010). Ascorbic acid treatment prevented the oxidation of phenolics and flavonoids in fresh-cut apples by inhibiting PPO and POD activities (Wen et al., 2021). These investigations concur with our study and suggest that CA treatment prevented FCT browning by retarding the oxidation of phenolic compounds.
The de novo biosynthesis of phenlics induced by cutting and peeling through the phenylpropanoid pathway was suggested to be related to the browning of several fresh-cut foods (Song et al., 2019) including taros (Xiao et al., 2022). In plant, PAL, 4CL, C4H, and CHS are the four key enzymes responsible for phenolic biosynthesis through the phenylpropanoid pathway (Dong and Lin, 2021). Briefly, PAL catalyzes the deamination of L-Phenylalanine, and resulting in the formation of cinnamic acid (Medda et al., 2020). Cinnamic acid is subsequently hydroxylated by C4H to form p-coumaric acid (Zhang et al., 2020a). 4CL catalyzes the formation of CoA esters for some phenolics including ferulic acid, 4-coumaric acid, benzoic acid and caffeic acid (Klempien et al., 2012). CHS can use 4-coumaroyl-CoA to produce naringenin-chalcone, which can spontaneously isomerize into naringenin (Liu et al., 2019). In this study, CA treatment significantly reduced total phenolic contents (Figure 3A), PAL activity and the expression of nine C4H, 4CL, and CHS genes in FCT (Figure 4), suggesting CA treatment reduced cutting-induced phenolic biosynthesis by suppressing the activities of key enzymes in the phenylpropanoid pathway. The repressed phenolic biosynthesis by CA treatment might contribute to a delay in FCT browning.
The stability and integrity of the cell membrane has been suggested to be closely associated with the browning of fresh-cut foods (Li et al., 2017; Worarad et al., 2021). LOX is a lipid peroxidizing enzyme involving in the oxygenation of polyunsaturated fatty acids into lipid hydroperoxides, and locates in the cell membrane (Shewfelt and del Rosario, 2000; Lv et al., 2021). Fresh-cut operations usually induce LOX activity, and the enhanced LOX activity accelerates membrane lipid peroxidation (Gao et al., 2017; Zhao et al., 2021). MDA is generally supposed to be an indicator of membrane lipid peroxidation in plant (Dhindsa et al., 1981). LOX is proposed to promote the browning through inducing oxidative damages to cell membrane as seen in fresh-cut pears (Hu et al., 2014; Li et al., 2017), lotus roots (Gao et al., 2017), lily bulbs (Kan et al., 2019), as well as taros (Xiao et al., 2021). In the current study, CA treatment significantly reduced MDA contents and LOX activities and gene expression in FCT under cold condition (Figure 5). These results suggest that CA treatment reduced the membrane lipid peroxidation of FCT by restricting LOX activity, and thus maintaining an intact compartmentation for enzymes and substrates resulting in taro browning. In addition, correlation analysis indicated that there were positive correlations between BI and both MDA content (r = 0.99) and LOX activity (r = 0.87; Figure 6). The results suggest that MDA content and LOX activity could be browning indicators in FCT.
Now the question is: how can CA reduce the activities of browning-related enzymes? The answer may hide behind its chemical attributes. CA is a monoterpene aldehyde which has the ability to react with amino group (Han et al., 2017). The aldehyde compounds such as citral from citrus essential oil can directly react with the amino group in mushroom PPO to form a Schiff base (Lante and Tinello, 2015). A comparison of inhibitory activity for PPO by several aldehydes indicated that the stability of the Schiff base formed between PPO and one aldehyde was correlated with PPO activity (Kubo and Kinst-Hori, 1999). These results suggest that, at protein level, CA agent could directly inactivate the activities of browning-related enzymes by forming a Schiff base with amino group inner enzymes. Apart from regulations at protein level, CA treatment also suppressed the expression of 18 genes coding browning-related enzymes (Figures 3–5). The expression levels of all the 13 ergosterol biosynthesis genes were repressed after citronellal treatment (OuYang et al., 2021). These results suggest that CA treatment regulates gene expression via an as yet unknown mechanism. In all, the results suggest that CA treatment regulates the activities of browning-related enzymes at both protein and gene levels.
In conclusion, CA treatment in 0.2 mL/L showed a strong anti-browning activity against FCT browning. It could restrict the activity and gene expression of browning-related enzymes including PPO, POD, PAL and LOX, reduce TPC, SQ, and MDA contents in FCT during cold storage. The results of this study demonstrated that CA was a novel anti-browning agent at preserving high quality of FCT for processing. Further studies are required to investigate the detailed mechanisms behind the effects of CA on the browning reduction of FCT. Additionally, whether CA treatment could affect the aroma quality of FCT and consumers' acceptability should be thoroughly evaluated in future studies. Moreover, how CA treatment influences the microbial attributes of fresh-cut produce and which microbe is restrained need in-depth investigations.
Data availability statement
The datasets presented in this study can be found in online repositories. The names of the repository/repositories and accession number(s) can be found at: https://www.ncbi.nlm.nih.gov/protein/MQM19396.1.
Author contributions
BW: writing—original draft, data curation, investigation, resources, and methodology. YW: writing—original draft and data curation. YH: investigation and methodology. YJ: writing—original draft. JH: conceptualization, funding acquisition, and writing—review and editing. YX: writing—review and editing. All authors contributed to the article and approved the submitted version.
Funding
This study was supported by the Key Research Project of Shaoguan University (No. SZ2018KJ02).
Conflict of interest
The authors declare that the research was conducted in the absence of any commercial or financial relationships that could be construed as a potential conflict of interest.
Publisher's note
All claims expressed in this article are solely those of the authors and do not necessarily represent those of their affiliated organizations, or those of the publisher, the editors and the reviewers. Any product that may be evaluated in this article, or claim that may be made by its manufacturer, is not guaranteed or endorsed by the publisher.
Supplementary material
The Supplementary Material for this article can be found online at: https://www.frontiersin.org/articles/10.3389/fsufs.2022.1001362/full#supplementary-material
References
Ali, A., Yeoh, W. K., Forney, C., and Siddiqui, M. W. (2018). Advances in postharvest technologies to extend the storage life of minimally processed fruits and vegetables. Crit. Rev. Food Sci. 58, 2632–2649. doi: 10.1080/10408398.2017.1339180
Ali, S., Sattar Khan, A., Ullah Malik, A., Anwar, R., Akbar Anjum, M., Nawaz, A., et al. (2021). Combined application of ascorbic and oxalic acids delays postharvest browning of litchi fruits under controlled atmosphere conditions. Food Chem. 350, 129277. doi: 10.1016/j.foodchem.2021.129277
Altunkaya, A., and Gokmen, V. (2010). Purification and characterization of polyphenol oxidase, peroxidase and lipoxygenase from freshly cut lettuce (L. sativa). Food Technol. Biotech. 49, 249–256. doi: 10.1016/j.foodchem.2010.09.099
Alves, M. M., Gonalves, M. P., and Rocha, C. M. R. (2017). Effect of ferulic acid on the performance of soy protein isolate-based edible coatings applied to fresh-cut apples. LWT Food Sci. Technol. 80, 409–415. doi: 10.1016/j.lwt.2017.03.013
Api, A. M., Belsito, D., Biserta, S., Botelho, D., Bruze, M., Burton, G. A., et al. (2021). Rifm fragrance ingredient safety assessment, citronellal, cas registry number 106-23-0. Food Chem. Toxicol. 1, 111991. doi: 10.1016/j.fct.2021.111991
Banerjee, A., Penna, S., and Variyar, P. (2015). Allyl isothiocyanate enhances shelf life of minimally processed shredded cabbage. Food Chem. 183, 265–272. doi: 10.1016/j.foodchem.2015.03.063
Bellinger, M. R., Paudel, R., Starnes, S., Kambic, L., Kantar, M. B., Wolfgruber, T., et al. (2020). Taro genome assembly and linkage map reveal QTLs for resistance to taro leaf blight. G3 8, 2763–2775. doi: 10.1534/g3.120.401367
Capotorto, I., Amodio, M. L., Diaz, M. T. B., de Chiara, M. L. V., and Colelli, G. (2017). Effect of anti-browning solutions on quality of fresh-cut fennel during storage. Postharvest. Biol. Tec. 137, 21–30. doi: 10.1016/j.postharvbio.2017.10.014
Chen, C. J., Chen, H., Zhang, Y., Thomas, H. R., Frank, M. H., He, Y. H., et al. (2020). TBtools-an integrative toolkit developed for interactive analyses of big biological data. Mol. Plant 13, 1194–1202. doi: 10.1016/j.molp.2020.06.009
Chen, X., Ren, L., Li, M., Qian, J., Fan, J., and Du, B. (2017). Effects of clove essential oil and eugenol on quality and browning control of fresh-cut lettuce. Food Chem. 214, 432–439. doi: 10.1016/j.foodchem.2016.07.101
Chen, Z., Zhu, C. H., Zhang, Y., Niu, D. B., and Du, J. H. (2010). Effects of aqueous chlorine dioxide treatment on enzymatic browning and shelf-life of fresh-cut asparagus lettuce (Lactuca sativa L.). Postharvest Biol. Tec. 58, 232–238. doi: 10.1016/j.postharvbio.2010.06.004
Dhindsa, R. S., Pulmb-Dhindsa, P., and Thorpe, T. A. (1981). Leaf senescence correlated with increased levels of membrane permeability and lipid peroxidation and decreased levels of superoxide dismutase and catalase. J. Exp. Bot. 32, 93–101. doi: 10.1093/jxb/32.1.93
Dong, N. Q., and Lin, H. X. (2021). Contribution of phenylpropanoid metabolism to plant development and plant-environment interactions. J. Integr. Plant Biol. 63, 180–209. doi: 10.1111/jipb.13054
Dou, Y., Chang, C., Wang, J., Cai, Z., Zhang, W., Du, H., et al. (2021). Hydrogen sulfide inhibits enzymatic browning of fresh-cut Chinese water chestnuts. Front. Nutr. 8, 652984. doi: 10.3389/fnut.2021.652984
Du, J., Fu, Y., and Wang, N. (2009). Effects of aqueous chlorine dioxide treatment on browning of fresh-cut lotus root. LWT Food Sci. Technol. 42, 654–659. doi: 10.1016/j.lwt.2008.08.007
Fan, X., and Wang, W. (2022). Quality of fresh and fresh-cut produce impacted by nonthermal physical technologies intended to enhance microbial safety. Crit. Rev. Food Sci. 62, 362–382. doi: 10.1080/10408398.2020.1816892
Fu, Y., Zhang, K., Wang, N., and Du, J. (2007). Effects of aqueous chlorine dioxide treatment on polyphenol oxidases from golden delicious apple. LWT Food Sci. Technol. 40, 1362–1368. doi: 10.1016/j.lwt.2006.11.001
Gao, H., Chai, H. K., Cheng, N., and Cao, W. (2017). Effects of 24-epibrassinolide on enzymatic browning and antioxidant activity of fresh-cut lotus root slices. Food Chem. 217, 45–51. doi: 10.1016/j.foodchem.2016.08.063
Giannakourou, M. C., and Tsironi, T. N. (2021). Application of processing and packaging hurdles for fresh-cut fruits and vegetables preservation. Foods 10, 830. doi: 10.3390/foods10040830
Han, J. H., Lee, S. M., and Kim, Y. S. (2017). Effects of schiff base formation and aldol condensation on the determination of aldehydes in rice wine using GC-MS. Molecules 22, 618. doi: 10.3390/molecules22040618
Hu, K. D., Wang, Q., Hu, L. Y., Gao, S. P., Wu, J., Li, Y. H., et al. (2014). Hydrogen sulfide prolongs postharvest storage of fresh-cut pears (Pyrus pyrifolia) by alleviation of oxidation of oxidative damage and inhibition of fungal growth. PLoS ONE 9, e85524. doi: 10.1371/journal.pone.0085524
Intarasit, S., Chotikakham, S., Chumyam, A., Uthaibutra, J., and Saengnil, K. (2018). Protective effects of chlorine dioxide solution on postharvest pericarp browning and oxidative damage of longan fruit. Acta hort. 1210, 177–184. doi: 10.17660/ActaHortic.2018.1210.25
Kalappurakkal, J. M., Sil, P., and Mayor, S. (2020). Toward a new picture of the living plasma membrane. Protein Sci. 29, 1355–1365. doi: 10.1002/pro.3874
Kan, J., Xie, W., Wan, B., Huo, T. B., Lin, X. P., Liu, J., et al. (2019). Heat-induced tolerance to browning of fresh-cut lily bulbs (Lilium lancifolium Thunb.) under cold storage. J. Food Biochem. 43, e12816. doi: 10.1111/jfbc.12816
Kasnak, C. (2020). Effects of anti-browning treatments on the polyphenol oxidase and antioxidant activity of fresh-cut potatoes by using response surface methodology. Potato Res. 63, 417–430. doi: 10.1007/s11540-019-09448-7
Kim, D. H., Kim, H. B., Chung, H. S., and Moon, K. D. (2014). Browning control of fresh-cut lettuce by phytoncide treatment. Food Chem. 159, 188–192. doi: 10.1016/j.foodchem.2014.03.040
Klempien, A., Kaminaga, Y., Qualley, A., Nagegowda, D. A., Widhalm, J. R., Orlova, I., et al. (2012). Contribution of CoA ligases to benzenoid biosynthesis in petunia flowers. Plant Cell 24, 2015–2030. doi: 10.1105/tpc.112.097519
Kubo, I., and Kinst-Hori, I. (1999). Tyrosinase inhibitory activity of the olive oil flavor compounds. J. Agr. Food Chem. 47, 4574–4578. doi: 10.1021/jf990165v
Lante, A., and Tinello, F. (2015). Citrus hydrosols as useful by-products for tyrosinase inhibition. Innov. Food Sci. Emerg. 27, 154–159. doi: 10.1016/j.ifset.2014.11.001
Li, Z., Zhang, Y., and Ge, H. (2017). The membrane may be an important factor in browning of fresh-cut pear. Food Chem. 230, 265–270. doi: 10.1016/j.foodchem.2017.03.044
Liu, C. M., Shen, Y., Yang, M., Chi, K. M., and Guo, N. (2022). Hazard of staphylococcal enterotoxins in food and promising strategies for natural products against virulence. J. Agric. Food Chem. 70, 2450–2465. doi: 10.1021/acs.jafc.1c06773
Liu, Y., Jing, S. X., Luo, S. H., and Li, S. H. (2019). Non-volatile natural products in plant glandular trichomes: chemistry, biological activities and biosynthesis. Nat. Prod. Rep. 36,626–665. doi: 10.1039/C8NP00077H
Lv, G., Tian, Q., Zhang, F., Chen, J., Niaz, M., Liu, C., et al. (2021). Reduced expression of lipoxygenase genes improves flour processing quality in soft wheat. J. Exp. Bot. 72, 6247–6259. doi: 10.1093/jxb/erab264
Ma, Y., Lu, X., Nock, J., and Watkins, C. (2015). Peroxidase and polyphenoloxidase activities in relation to flesh browning of stem-end and calyx-end tissues of 'Empire' apples during controlled atmosphere storage. Postharvest. Biol. Tec. 108, 1–7. doi: 10.1016/j.postharvbio.2015.05.002
María, V. F., María, V. A., and Jagus, R. J. (2020). Effect of packaging material on the quality of fresh-cut beet leaves packed in modified atmospheres. J. Food Process. Pres. 44, e14552. doi: 10.1111/jfpp.14552
Medda, S., Dessena, L., and Mulas, M. (2020). Monitoring of the PAL enzymatic activity and polyphenolic compounds in leaves and fruits of two myrtle cultivars during maturation. Agriculture 10, 389. doi: 10.3390/agriculture10090389
Mendoza-Enano, M. L., Stanley, R., and Frank, D. (2019). Linking consumer sensory acceptability to volatile composition for improved shelf-life: a case study of fresh-cut watermelon (Citrullus lanatus). Postharvest Biol. Tec. 154, 137–147. doi: 10.1016/j.postharvbio.2019.03.018
Nasiri, M., Barzegar, M., Sahari, M. A., and Niakousari, M. (2017). Tragacanth gum containing zataria multiflora boiss. Essential oil as a natural preservative for storage of button mushrooms (Agaricus bisporus). Food Hydrocolloid 72, 202–209. doi: 10.1016/j.foodhyd.2017.05.045
Oms-Oliu, G., Aguiló-Aguayo, I., and Martín-Belloso, O. (2010). Inhibition of browning on fresh-cut pear wedges by natural compounds. J. Food Sci. 71, S216–S224. doi: 10.1111/j.1365-2621.2006.tb15644.x
OuYang, Q., Liu, Y., Oketch, O. R., Zhang, M., Shao, X., and Tao, N. (2021). Citronellal exerts its antifungal activity by targeting ergosterol biosynthesis in Penicillium digitatum. J. Fungi. 7, 432. doi: 10.3390/jof7060432
Porta, H., and Rocha-Sosa, M. (2002). Plant lipoxygenases. Physiological and molecular features. Plant Physiol. 130, 15–21. doi: 10.1104/pp.010787
Qiao, L. O., Gao, M., Zheng, J. X., Zhang, J. Y., Lu, L. F., and Liu, X. (2021). Novel browning alleviation technology for fresh-cut products: preservation effect of the combination of Sonchus oleraceus L. extract and ultrasound in fresh-cut potatoes. Food Chem. 348, 129132. doi: 10.1016/j.foodchem.2021.129132
Rodríguez-Arzuaga, M., Salsi, M. S., and Piagentini, A. M. (2021). Storage quality of fresh-cut apples treated with yerba mate (Ilex paraguariensis). J. Food Sci. Tech. 58, 186–196. doi: 10.1007/s13197-020-04528-w
Rojas-Graü, M. A., Grasa-Guillem, R., and Martín-Belloso, O. (2007). Quality changes in fresh-cut Fuji apple as affected by ripeness stage, antibrowning agents, and storage atmosphere. J. Food Sci. 72, S36–S43. doi: 10.1111/j.1750-3841.2006.00232.x
Rouet-Mayer, M. A., Ralambosoa, J., and Philippon, J. (1990). Roles of o-quinones and their polymers in the enzymic browning of apples. Phytochemistry 29, 435–440. doi: 10.1016/0031-9422(90)85092-T
Sharma, R., Rao, R., Kumar, S., Mahant, S., and Khatkar, S. (2019). Therapeutic potential of citronella essential oil: a review. Curr. Drug Discov. Technol. 16, 330–339. doi: 10.2174/1570163815666180718095041
Shewfelt, R. L., and del Rosario, B. A. (2000). The role of lipid peroxidation in storage disorders of fresh fruits and vegetables. HortScience 35, 575–579. doi: 10.21273/HORTSCI.35.4.575
Siddiqui, M. W., Deshi, V., Homa, F., Aftab, M. A., and Aftab, T. (2021). Inhibitory effects of hydrogen sulfide on oxidative damage and pericarp browning in harvested litchi. J. Plant Growth Regul. 40, 2560–2569 doi: 10.1007/s00344-021-10300-x
Sikora, M., and Swieca, M. (2018). Effect of ascorbic acid postharvest treatment on enzymatic browning, phenolics and antioxidant capacity of stored mung bean sprouts. Food Chem. 239, 1160–1166. doi: 10.1016/j.foodchem.2017.07.067
Singh, H. P., Batish, D. R., Kaur, S., Kohli, R. K., and Arora, K. (2006). Phytotoxicity of the volatile monoterpene citronellal against some weeds. Zeitschrift fur Naturforschung. C. J. Biosci. 61, 334–340. doi: 10.1515/znc-2006-5-606
Song, M. B., Wu, S. J., Shuai, L., Duan, Z. H., Chen, Z. L., Shang, F. F., et al. (2019). Effects of exogenous ascorbic acid and ferulic acid on the yellowing of fresh-cut chinese water chestnut. Postharvest. Biol. Tec. 148, 15–21. doi: 10.1016/j.postharvbio.2018.10.005
Sun, Y., Zhang, W., Zeng, T., Nie, Q. X., Zhang, F. Y., and Zhu, L. Q. (2015). Hydrogen sulfide inhibits enzymatic browning of fresh-cut lotus root slices by regulating phenolic metabolism. Food Chem. 177, 376–381. doi: 10.1016/j.foodchem.2015.01.065
Talab, Z. V., and Gholami, M. (2012). The effect of essential oil and extract of clove buds (Eugenia Caryophyllata) on some quality characteristics of table grapes during storage. Iran. J. Hortic. Sci. 43, 255–265. doi: 10.22059/IJHS.2012.25116
Wang, B., Huang, Y. Y., Zhang, Z. M., Xiao, Y. H., and Xie, J. (2022). Ferulic acid treatment maintains the quality of fresh-cut taro (Colocasia esculenta) during cold storage. Front. Nut. 9, 884844. doi: 10.3389/fnut.2022.884844
Wen, Y. T., Liang, Y. Q., Chai, W. M., Wei, Q. M., Yu, Z. Y., and Wang, L. J. (2021). Effect of ascorbic acid on tyrosinase and its anti-browning activity in fresh-cut Fuji apple. J. Food Biochem. 45, e13995. doi: 10.1111/jfbc.13995
Worarad, K., Suzuki, T., Norii, H., Mochizuki, Y., Ishii, T., Shinohara, K., et al. (2021). Transcriptome profiling for pericarp browning during long-term storage of intact lotus root (Nelumbo nucifera). Plant Growth Regul. 95, 1–15. doi: 10.1007/s10725-021-00736-2
Wu, Y., Ouyang, Q., and Tao, N. (2016). Plasma membrane damage contributes to antifungal activity of citronellal against Penicillium digitatum. J. Food Sci. Technol. 53, 3853–3858. doi: 10.1007/s13197-016-2358-x
Xiao, Y. H., He, J. M., Zeng, J., Yuan, X., Zhang, Z. M., and Wang, B. (2020). Application of citronella and rose hydrosols reduced enzymatic browning of fresh-cut taro. J. Food Biochem. 44, e13283. doi: 10.1111/jfbc.13283
Xiao, Y. H., Xie, J., Wu, C. S., He, J. M., and Wang, B. (2021). Effects of melatonin treatment on browning alleviation of fresh-cut foods. J. Food Biochem. 45, e13798. doi: 10.1111/jfbc.13798
Xiao, Y. H., Zhang, J. L., Jiang, Y. Y., Yuan, Y., Xie, J., He, J. M., et al. (2022). Cinnamic acid treatment reduces the surface browning of fresh-cut taro. Scientia Hortic. 291, 110613. doi: 10.1016/j.scienta.2021.110613
Yao, T., Feng, K., Xie, M., Barros, J., Tschaplinski, T. J., Tuskan, G. A., et al. (2021). Phylogenetic occurrence of the phenylpropanoid pathway and lignin biosynthesis in plants. Front. Plant Sci. 12, 704697. doi: 10.3389/fpls.2021.704697
Zhang, B., Lewis, K. M., Abril, A., Davydov, D. R., Vermerris, W., Sattler, S. E., et al. (2020a). Structure and function of the cytochrome P450 monooxygenase cinnamate 4-hydroxylase from Sorghum bicolor. Plant Physiol. 183, 957–973. doi: 10.1104/pp.20.00406
Zhang, M., Li, H., Agyekumwaa, A. K., Yu, Y., and Xiao, X. (2022). Effects of citronellal on growth and enterotoxins production in Staphylococcus aureus ATCC 29213. Toxicon 213, 92–98. doi: 10.1016/j.toxicon.2022.04.016
Zhang, Y. M., Peng, Y., Jia, R. Y., Wang, Q. G., Lou, X. Q., et al. (2020b). Sodium chloride combined with polypropylene film can maintain the quality of fresh-cut ginger. Food Packaging Shelf 25, 100541. doi: 10.1016/j.fpsl.2020.100541
Zhao, H. N., Fan, Z. T., Wu, J. H., and Zhu, S. H. (2021). Effects of pre-treatment with S-nitrosoglutathione-chitosan nanoparticles on quality and antioxidant systems of fresh-cut apple slices. LWT Food Sci. Technol. 139, 110565. doi: 10.1016/j.lwt.2020.110565
Zhu, L. J., Hu, W. F., Murtaza, A., Iqbal, A., Li, J. X., Zhang, J., et al. (2022). Eugenol treatment delays the flesh browning of fresh-cut water chestnut (Eleocharis tuberosa) through regulating the metabolisms of phenolics and reactive oxygen species. Food Chem. 14, 100307. doi: 10.1016/j.fochx.2022.100307
Keywords: fresh-cut taro, enzymatic browning, browning mitigator, natural extract, citronellal
Citation: Wang B, Wang Y, Huang Y, Jiang Y, He J and Xiao Y (2022) Anti-browning effects of citronellal on fresh-cut taro (Colocasia esculenta) slices under cold storage condition. Front. Sustain. Food Syst. 6:1001362. doi: 10.3389/fsufs.2022.1001362
Received: 23 July 2022; Accepted: 12 August 2022;
Published: 07 September 2022.
Edited by:
Philippa Chinyere Ojimelukwe, Michael Okpara University of Agriculture, NigeriaReviewed by:
Sajid Ali, Bahauddin Zakariya University, PakistanChanthima Phungamngoen, Khon Kaen University, Thailand
Copyright © 2022 Wang, Wang, Huang, Jiang, He and Xiao. This is an open-access article distributed under the terms of the Creative Commons Attribution License (CC BY). The use, distribution or reproduction in other forums is permitted, provided the original author(s) and the copyright owner(s) are credited and that the original publication in this journal is cited, in accordance with accepted academic practice. No use, distribution or reproduction is permitted which does not comply with these terms.
*Correspondence: Jinming He, aGptQHNndS5lZHUuY24=; Yanhui Xiao, eWh4aWFvQHNndS5lZHUuY24=
†ORCID: Bin Wang orcid.org/0000-0002-6812-235X