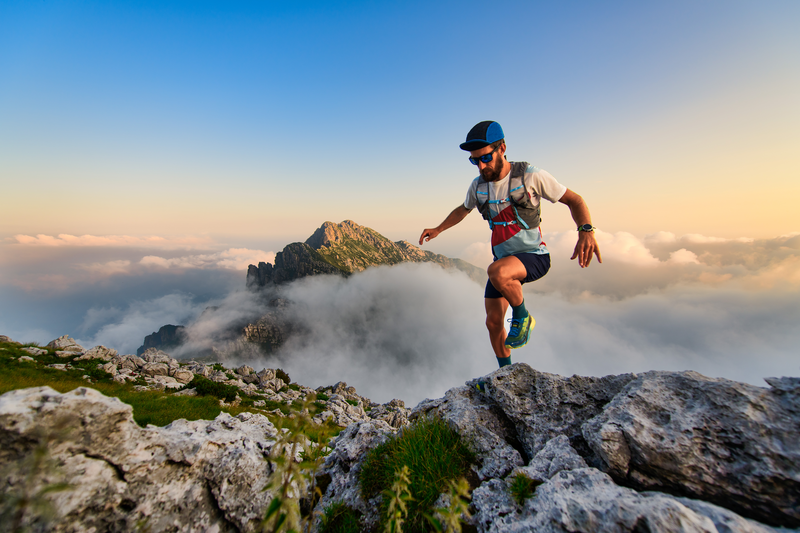
94% of researchers rate our articles as excellent or good
Learn more about the work of our research integrity team to safeguard the quality of each article we publish.
Find out more
ORIGINAL RESEARCH article
Front. Sustain. Food Syst. , 28 January 2022
Sec. Agro-Food Safety
Volume 5 - 2021 | https://doi.org/10.3389/fsufs.2021.808327
This article is part of the Research Topic Recent Advances and Approaches in the Application of Elicitors to Enhance Resistance Mechanisms in Fresh Produce View all 5 articles
Within this study, influences of abiotic factors inducing biomass and synthesis of secondary metabolites in Japanese mint (Mentha arvensis) were studied. Plants were cultivated in the evaporate greenhouse system under 50% shading, at temperatures of 25–28°C and with relative humidity of 60–65%. After 12 weeks of planting, the results showed that water management at 75% and 100% evaporate transpiration crops (ETc) gave the highest growth measures for plant height (22.96 cm), number of stems (7.75), stem thickness (3.69 mm), diameter of canopy (28.03 cm), number of leaves per plant (151.28), fresh weight (108.07 g), and dry weight (22.29 g), while leaf area value (1,104.10 cm2) was the highest at 75% water and the highest SPAD value was at 25% (41.13 SPAD unit). Levels of secondary metabolite as described by DPPH and ABTS antioxidant potential remained stable; however, total flavonoid was higher in ETc 25% and 50% as compared to other treatments (0.41 mg GAE/g DW). The content of menthol corresponded well with biomass yield. In the second experiment, nitrogen levels at 210 and 310 mg/l gave the highest in growth measures for plant height (21.41 cm) and SPAD unit (25.54 SPAD unit). In addition, the nitrogen level at 310 mg/l gave the highest growth measures, viz., number of stem (9.00 stems), stem thickness (3.28 mm), diameter of canopy (27.55 cm), number of leaves per plant (162.57), leaf area (568.38 cm2), fresh weight (80.79 g), and dry weight (17.09 g). At 12 weeks of cultivation, the highest levels of total phenolic and flavonoid and menthol were achieved when a higher rate of nitrogen was applied. The results advised that Japanese mints adjusted well to the stresses in the controlled atmospheric humidity of the greenhouse, and nitrogen was a key element for increasing the productivity.
Graphical Abstract. Schematic diagram of physiological and biochemical responses of Japanese mint grown under the limit supplies of water and nitrogen fertilizer.
Menthol mint (Mentha arvensis), a perennial herb of the Lamiaceae family, is a well-known essential oil crop of economic value in food, perfumery, and pharmaceutical industries (Pandey et al., 2003). Mint oil comprises L-menthol, L-menthone, and carvone as major volatile constituents (Taneja and Chandra, 2012) and is the most important intermediate raw material for menthol crystallization (Berger, 2007; Kamatou et al., 2013; Skalicka-Wozniak and Walasek, 2014). This has led to an annual demand of the herbage volume of ~32,000 metric tons (Lawrence, 2006). The advantage of growing plants in a greenhouse is the agronomic protection from environmental threats including temperature, light intensity, humidity, and pests (Mekonnen et al., 2015; Treadwell et al., 2020). There is also an increasing demand of subtropical cultivation of herbs like mint in the greenhouse due to low operating cost and simple management. The evaporative cooling relies on the conversion of sensible into latent heat through the evaporation of circling water, along with the ventilation system. This technique decreases the cultivating temperature to about 3–6°C, lower than that of the atmospheric temperature depending on the dryness of the external air and humidity. To this end, agronomical management to simulate the optimal growth of subclimate species can be achieved by greenhouse evapotranspiration (Albaho et al., 2008; Villarreal-Guerrero et al., 2012; Zhang et al., 2017).
Triggers, either biotic or abiotic, along with genetics are drivers affecting the biosynthesis of plant secondary metabolites (Verma and Shukla, 2015). Plants respond to environmental stresses differently as the influences of their diverse phenological and physiological processes, the climate conditions, and agronomic practices (Alinejad et al., 2020). Plant secondary metabolites are generally thought to be a result of plant interactions with the environment, although they are not essential during growth and development (Chen et al., 2009). The volatile metabolite in particular possesses a number of functions including indirect defense and direct defense against herbivores, resistance to abiotic stress, and wound-activated signaling (Pichersky et al., 2006). Like other plants, Mentha spices produce volatile metabolites that may render unique fitness benefits to the host plants grown in a specific stress environment, although detailed study is still needed. Among the environmental traits, water is the most important agronomic factor and the deficit could adversely affect the productivity of the produce. Drought stress induces closure of stomata that leads to decreased rates of photosynthesis and consequently exhibits a C/N ratio which alternates biosynthesis of the metabolites (Alinejad et al., 2020). In herbaceous plants such as sweet basil and American basil, water stress increases essential oil yield and proline, and total carbohydrate content only at the irrigation of 75% field capacity was sufficient to maximize crop production and the oil yield (Khalid, 2006). In Mentha spices, however, full irrigation and fertilization were necessary to achieve maximum yield and high quantity of secondary metabolites (Lawrence, 2006). Plants require a different fertigation with adequate nutrients for their growth and development as well as during the secondary mechanism (Marschner, 2012). Mints (spearmint and peppermint) require ~280 kg/ha of nitrogen to reach their optimum plant biomass and oil yields (Brown et al., 2003). Shormin et al. (2009) reported that supplying Japanese mints with 240 kg N/ha, the maximum fresh weight yield can be obtained Alsafar and Al-Hassan (2009), however, reported that 70 kg N/ha supply could increase the highest total dry matter and essential oil yield of wild mint (M. longifolia L.). Although Japanese mint is not native to Thailand, the plant has been cultivated and adapted well mostly in the highland area (rainfall in the range of 95–105 cm, altitude of 250–400 m) (Bhattacharya, 2016). Mint has wide adaptability to different environments and high resistance to stresses. Mekonnen et al. (2015) and Lawrence (2006) suggested that the optimum temperature to grow Japanese mint is 15–30°C with high light intensity, although it also succeeds in partial shade.
At one end, information on water and plant nutrient management of Japanese mint in the evaporated greenhouse system is lacking. Additionally, contrasting effects of drought and nitrogen supplies on the alteration of the secondary metabolites of Japanese mint have not yet been reported. This research therefore aims to evaluate the effect of nitrogen and water supplies on growth, essential oil, and secondary metabolite quantity in Japanese mint grown in the imitative sub-temperature condition. The contribution of this research is toward a deep understanding of the mechanism of plant stress response and also to encourage a sustainably alternative production of this subtropical herb in the changing climate.
Four-week-old cuttings of M. arvensis grown in peat moss and coconut dust were obtained from Khun Pae Royal Project, Chom Thong, Chiang Mai, Thailand. They were transferred to a 2-l pot, containing a perlite and vermiculite ratio of 1:1. The experiments were conducted in an evaporative cooling greenhouse system with a temperature controlled between 25 and 30°C and relative humidity ranging 60–70% at Mae-Hia Agricultural Research, Demonstrative and Training Centre, Faculty of Agriculture, Chiang Mai University. Nitrogen treatments (4 levels) including nitrogen 0, 105, 210, and 315 ppm were incorporated in the Hoagland and Arnon solution at pH 6.5–7.0 with electrical conductivity (EC) at 1,900–2,000 μS/cm (Bhattacharya, 2016). Plants were fertilized twice a day and watered every day. In a water deficiency experiment, 4 treatments were considered including water regimes of control (100) and 75, 50, and 25% evaporate transpiration crops (ETc). The treatments were arranged in a randomized complete block design (RCBD) in three replicates.
Plants were sampled for growth determination including plant height, leaf area, number of leaves, number of branches, SPAD value, diameter of canopy, and fresh and dry weights of aerial part and root part at week 4 representing a mature stage and week 12 representing the commercial harvesting stage (Garcia-Mata, 2001; Doymaz, 2006; Lawrence, 2006). The vegetative part was cut and dried at 55°C in a hot air oven, until the constant weight was reached (Doymaz, 2006). Dried leaves were ground to powder using a handheld grinder (Philips, Amsterdam, the Netherlands) at low speed, for substantial phytochemical extractions.
From each treatment, clean leaf discs (1.0 cm2) were obtained from the young fully expanded leaves (3rd−4th pairs form the shoot). These were submerged into 10 ml of deionised water in a test tube and maintained at room temperature for 2 h. Thereafter, the initial conductivity (EC1) in the solution was measured using a Eutech CON 700 conductivity meter (Eutech Instruments Pte Ltd., Singapur, Singapore). The same test tube was then incubated in a temperature-controlled water bath at 80°C for 2 h, and the total ions present in leaf discs (EC2) at room temperature. The electrolyte leakage is presented as percentage of total ions released which can be calculated as the following (Garcia-Mata, 2001):
The dried leaf powder (0.05 g) was extracted with 500 μl of 95% (v/v) methanol twice, and the supernatants were combined after centrifugation at 6,000 × g. The extractions were of three separate lots of samples. The 2,2-diphenyl-1-picrylhydrazyl (DPPH) radical scavenging activity was measured using the method of Sommano (Sommano, 2015). The methanol extract (25 μl) was mixed with 0.2 mM DPPH–methanol solution (250 μl), then the reaction was allowed to react in the darkness at room temperature for 30 min. The absorbance was measured at 517 nm. The percentage of the DPPH radical scavenging activity was calculated using the following equation:
where Acontrol is the absorbance of control [using 95% (v/v) methanol instead of the sample], Asample is the absorbance of the mixture of the sample extract and 0.2 mM DPPH–methanol solution.
The 2,2′-azino-bis-(3-ethylbenzothiazoline-6-sulfonate) (ABTS) radical cation scavenging activity was measured by incubating 200 μl ABTS working solution with 10 μl of the methanol extract (Goudarzian et al., 2020). The mixed solution was incubated at room temperature in the darkness for 30 min. The absorbance readings were recorded at 734 nm. The percentage of the ABTS radical scavenging activity was calculated using the following equation:
where Acontrol is the absorbance of the ABTS working standard, Asample is the absorbance of the mixture of the sample extract and ABTS working solution.
The supernatant was then used for the analyses of total phenolic and total flavonoid contents and radical scavenging activities. The total phenolic content was determined spectrophotometrically according to the Folin-Ciocalteu colorimetric method against the gallic acid standard, and the total flavonoid content was the aluminum chloride colorimetric method with standard catechin (CE) after the methods of Sommano et al. (2017) and Janpen et al. (2019b). The results were expressed as mg of standard equivalent per g DW of sample.
Monoterpene recovery was from the extraction of sample powder (0.4 g) with 2 ml dichloromethane consisting of 215 mg menthol standard (CAS 2216-51-5, Merck, Singapore, Singapore). The sample was then ultrasonicated for 5 min prior to shaking on an automatic shaker for 55 min at 150 rpm and 22°C (Janpen et al., 2019a). After centrifugation, the supernatant was filtered using a 0.45-μm nylon filter and then injected into a gas chromatography system equipped with mass spectrophotometry. The monoterpene fraction was determined by GC equipped with a flame ionization detector (Ciriminna et al., 2017) using an Rxi®-5Sil MS (Restek, Centre County, PA, USA) capillary column (30 m × 0.25 mm i.d., film thickness 0.25 m). Helium gas was used as a carrier gas in a constant linear velocity mode at a flow rate of 1 ml/min. The split ratio was 1:100 (v/v). The injector temperature was 250°C. The column temperature programming was 35–180°C at 4°C/min then 180–280°C at 17°C/min, and at 280°C for 10 min. An extracted sample (1 μl) solution was injected into the GC system. The concentration of menthol was determined based on automatically integrated peak areas of the GC-FID signal (Goudarzian et al., 2020).
The data were subjected to analysis of variance (ANOVA), and the comparison between the mean values of treatment was confirmed by Tukey's honest significant difference (HSD) test at the 5% level of significance. All statistical analyses were performed using the IBM SPSS program v. 23.0 (Armonk, New York, NY, USA). The correlation between physical properties and biochemical data and the treatments were analyzed using principal component analysis (PCA) by XLSTAT v. 2020 (Addinsoft, New York, NY, USA).
Table 1 illustrates the growth parameters of Japanese mints grown in the evaporative cooling greenhouse system with different water regimes. In the first 4 weeks of the water treatments at 25, 50, 75, and 100% ETc, results showed that there was no significant difference in plant height (14.57–17.88 cm), number of stem (1.11–1.33 stem/pot), stem thickness (2.21–2.37 mm), SPAD value (25.61–28.11 unit), and fresh (6.84–10.15 g) and dried weight (1.19–1.94 g). At this same period, the high level of water treatments (ETc ≥ 75%) gave a higher number of leaves and leaf area (58.60–61.60 leaves/pot and 70.08–76.11 cm2), while limited water treatment showed lower values of these parameters (35.40 leaves/pot and 43.55 cm2, respectively). At 12 weeks of the growing period, the higher levels of water treatments gave the highest plant height range (22.14–23.77 cm), number of stem (7.50–8.00 stems/pot), stem thickness (3.67–3.71 mm), canopy diameter (27.04–29.01 cm), leaf number (143.78–153.78 leaves/pot), and fresh (106.23–109.91 g) and dry weight (21.75–22.81 g). Plants gave the highest leaf area when they were given 75% ETc water treatment, while SPAD was the highest with 25% ETc treatment (41.13 units). The overall growth and development at 12 weeks of cultivation are shown in Figure 1A. It is well perceived that water potential has a strong relation to plant water uptake and carbon fixation which influence the growth and development of plants (Cowan, 1982; Sarker and Oba, 2019). Abiotic stresses like drought or salinity create osmotic stress (Sarker and Oba, 2020a) that lead to several physiological changes, including a decrease in photosynthetic activities (Sarker and Oba, 2018), DNA, protein and membrane damages, and nutritional imbalance in plants (Sarker and Oba, 2020b). Additionally, when the water uptake decreases, the transpiration rate also decreases after the initial increase which inhibits nutrient assimilation (Erlandsson, 1975). In menthol mint, water scarcity resulted in the significant losses in CO2 exchange rate, total assimilatory area, plant nutrient level, and the total biomass which was empirical for essential oil production (Misra and Srivastava, 2000; Brar et al., 2021). It is estimated that this plant required more water (>100% ETc) in the arid environment to achieve maximum productivity (Behera et al., 2014). The benefit of the evaporative cooling greenhouse system is the adjustable growing environment in which, in this case, the internal condition was maintained at the optimum growing condition at 20°C and 70% relative humidity (Fahmy et al., 2012). The results supported that the water regimes between 75% and 100% ETc were adequate in the greenhouse system. However, the chlorophyll content was higher in the limit water regime (25% ETc) which was contradictory to the report of Brar et al. (2021). The decrease in the chlorophyll content under drought stress is known as a non-stomatal limit factor. The increase, nonetheless, indicates the resistance of the plant to drought stress (Rahimi et al., 2010). It seems that by maintaining the environmental humidity under the evaporative system, the stomatal conductance and bulk leaf water potential balance is retained which consequently influences the transpiration and rate of CO2 and H2O exchange (Erlandsson, 1975; El-Sharkaway and Cock, 1984). In addition, when the water potential is relatively low, i.e., from drought stress, plants require a higher water uptake and thereby increase the rate of photosynthesis, resulting in a higher SPAD value.
Table 1. Physiological changes of potted Mentha arvensis grown in greenhouse with different water regimes.
Figure 1. The morphology of potted Mentha arvensis grown in the evaporative greenhouse system with different water regimes (A) and different levels of nitrogen fertigation (B).
The growth parameters of Japanese mints grown in the evaporative cooling greenhouse system with different nitrogen supplies are shown in Table 2. After 4 weeks of cultivation, plant height (14.64–17.48 cm), number of plants/pot (1.00–1.12), and stem thickness (1.77–1.97 mm) were not different among the treatments. The N315 treatment gave plants with the highest canopy diameter (7.71 cm), number of leaf (111.67), leaf area (99.62 cm2), total fresh and dry weight (13.63 and 1.77 g, respectively), and SPAD value (28.49 SPAD unit). At 12 weeks of cultivation, plants were 21.18–21.64 cm in N210 and N315 treatments and the highest nitrogen supply gave the highest number of plants/pot (9), stem thickness (3.28 mm), canopy size (27.55 cm), leaf number (162.57), leaf area (568.38 cm2), SPAD value (27.35 SPAD unit), and fresh and dry weight at 80.79 and 17.09 g, respectively. The overall growth and development at 12 weeks of cultivation in different nitrogen supplies are shown in Figure 1B. Nitrogen is the key nutrient element affecting the quantity and quality of essential oil crops (Azizi et al., 2009; Król et al., 2020). In Japanese mint, nitrogen enhances herbage yield (through cell enlargement, multiplication) and increases the rate of photosynthesis and oil yield (Behera et al., 2014, 2015). Its shallow root and high water demanding nature make it respond well with nitrogen (180 kg N/ha) and show good irrigation in the field production (Lakra and Verma, 2021). Methods of fertigation also influences the rate of nitrogen uptake, and the drip system is found to be more effective than surface application (Behera et al., 2015). The study on nutrient requirement of the menthol mint on the evaporative greenhouse system is limited. However, it has been also evident that high air humidity stimulates leaf morphology and leaf epidermal cells and lower stomatal frequency via nutrient uptake as an influence of a small vapor pressure deficit due to transpiration and the deficit on photosynthetic apparatus (Leuschner, 2002).
Table 2. Physiological changes of potted Mentha arvensis grown in the evaporative greenhouse system with different nitrogen fertigation.
The stress responses of the potted Japanese mints to the treatments are illustrated in Figure 2. The electrolyte leakage of the plant treated with different water regimes increased with time and was highest at 12 weeks (10.36–11.06%) in all treatments except for that of the ETc 50% which was 8.55%. The DPPH activity was overall maintained throughout until 12 weeks that the increase was noticed to 90% at higher rates of the water regimes. The ABTS potentials however declined to ~60% in all treatments in weeks 4 and 12 of planting. Similar with the nitrogen treatments, the electrolyte leakage values of all treatments increased with times from 6.00 to 12.00% and were not significantly different among the treatments. The antioxidant potential either by DPPH or ABTS, however, dropped significantly from week 4 to week 12. These values were significantly higher in the N0 treatment at week 12.
Figure 2. Plant stress response as determined by electrolyte leakage (A,D), DPPH antioxidant potential (B,E), and ABTS antioxidant potential (C,F) of Japanese mints grown in the evaporative greenhouse system. The figures on the left are for Mentha arvensis grown in the evaporative greenhouse with different water regimes, and figures on the right are for Mentha arvensis grown in the evaporative greenhouse different level of nitrogen fertigation. *Represents significant difference at a 95 percent confidence.
Oxidative damage induced during stress can cause the inhibition of overall growth in plants (Halliwell, 2006). Reactive oxygen species (ROS) accumulation triggers lipid peroxidation, protein degradation, cell membrane decompartmentation, and genetic damage (Men et al., 2018). To downregulate the ROS response, plants switch on the antioxidative mechanism, thereby maintaining cell homeostasis (Sommano, 2015). Electrolyte leakage has been used as the index measure of plant stress (Sommano, 2015; Janpen et al., 2019b). It is the response of K+ efflux from plant cells, which is mediated by plasma membrane cation conductance and ROS generation. The efflux of positive charge ions is basically as to balance out the counterions (Cl−, , NO3−, citrate3−, malate2−) (Demidchik et al., 2014). It was therefore possible that the increase in electrolyte leakage in our experiment was possibly due to the increase in macronutrient uptake that was regulated especially in the high-humidity condition. This assumption, however, has nothing to do with the stress mechanism. Plant stress can be determined by the status of antioxidative potentials. It was apparent that the Japanese mints maintained their antioxidant status at least until 4 weeks of cultivation in the greenhouse system then dropped significantly, which indicated that the status was rather static than induced (Hasanuzzaman et al., 2012). Consequently, the overall finding can be suggested that under these growing conditions, plants may not experience stress.
At 4 weeks of cultivation, the phenolic content of plants grown with ETc = 25, 50, 75, and 100% treatments had 0.05 mg GAE/g DW. It seems also that the level of phenolic content increased with times and at the 12 weeks of cultivation; plants gave the maximum overall phenolic content with the 75 and 100% ETc treatments at 0.15 mg GAE/g DW. The total flavonoid content remained subtle until 4 weeks of the cultivation and increased when reaching 12 weeks. In contradictory to the phenolics, the flavonoids of the lower percentage of water regimes were higher at 0.40 mg CAE/g DW at the final harvest. The menthol content increased toward the end of the cultivation period, and the level was the highest (20.00 mg/g sample) in the 75% ETc treatment. Similar patterns were also found in the nitrogen fertigation experiment. The total phenolic and flavonoid contents increased with the increasing N supplies, and the level was the highest at 12 weeks of cultivation in the N315 treatment (0.12 mg GAE/g DW and 0.20 CAE/g DW, respectively). The menthol content also reached its maximum high at 18.00 mg/g sample at this level of nitrogen. The bioactive compound status of Japanese mints grown in the evaporative greenhouse system with different stress treatments is illustrated in Figure 3.
Figure 3. Bioactive compound status as determined by total phenolic content (A,D), total flavonoid content (B,E), and menthol (C,F) of Japanese mints grown in the evaporative greenhouse system. The figures on the left are for Mentha arvensis grown in the evaporative greenhouse with different water regimes, and figures on the right are for Mentha arvensis grown in the evaporative greenhouse different level of nitrogen fertigation.
Among diverse varieties, plants of the Mentha species are usually the phenolic and flavonoid's richest sources within the Lamiaceae family (Tepe, 2008; Turkoglu, 2015). Rosmarinic acid and sinapic acid, hesperidin, and o-coumaric acid are the most abundant phenolic components, and epicatechin, rutin, naringenin, and catechin are among the most common flavonoids found in Japanese mint and related species (Dhifi et al., 2013; Bahadori et al., 2018). The essential oil consists of primarily menthol which takes up >50% of the total composition followed by limonene and trans-dihydrocarvone and caryophyllene (Arjun et al., 2017). This being said, the increased level of the antioxidant may contribute to the accumulation of bioactive ingredients during the cellular defense system against ROS.
Sarker et al. (2018) also added that for stress homeostasis, stress-induced plants have evolved mechanisms to enhance the concentration of these metabolites and detoxify the ROS. It is also suggested that during stress, plants accumulate phytochemicals such as sugars, polyols, and amino acids to lower the osmotic potential in the cells to facilitate water potential to be able to survive (Arve et al., 2011). The total phenolic and flavonoid contents of M. piperita L. increased with the increasing stress levels and in harsh stress conditions such as drought; it may experience a significant drop in total oil percentage closely related to the reduction of herbage yield and damage to the chlorophyll apparatus (Jahani et al., 2021). Misra and Srivastava (2000) also confirmed that in M. arvensis water stress restricted the CO2 exchange rate and total assimilatory area, thereby inhibiting the overall plant growth and development and essential oil yield and shifted the volatile profile compositions. The previous work highlights the importance of nutrients during the biosynthesis of natural products especially the antioxidants (Janpen et al., 2019b). Apart from biomass escalation, nitrogen increases the level of essential oil and oil components (Singh et al., 1989; Shormin et al., 2009; Hamed and Seyed Ali Mohammad, 2018).
To see the overall landscape of plant response in these growing conditions, we used the multivariate PCA for biomass and biochemical data. Figures 4A,C illustrate the responses of plants with water regimes in the evaporated greenhouse system. Both plots were accounted for >80% of the variables across PC1 and 2 (F1 and 2). It has been depicted from the plots that the biomass and the bioactive compounds as determined by phenolic, menthol, and antioxidant potentials are highly correlated with high irrigation regimes (ETc 75 and 100%). However, the SPAD value and flavonoid content were highly associated with low levels of irrigation. A variable response of chlorophyll content to a biotic stress has been reported previously (Shah et al., 2017; Mbarki et al., 2018; Afsar et al., 2020). However, these reports confirmed the reduction of the total biomass as corresponding to the stress condition. To this incident, Shah et al. (2017) explained that biomass reduction was due to the lower surface area per leaf mass which contributed to less transpiration and conservation of water. As said, plants produced secondary metabolites as to prevent itself from oxidative damage and flavonoids are among the metabolites produced via this event (Gao et al., 2011; Ebrahimian and Bybordi, 2012; Liu et al., 2012). The accumulation of defensive compounds such as flavonoids increased osmotic pressure, which helps plant to withstand unfavorable conditions (Gao et al., 2011). The physiological responses with nitrogen fertigation are shown in Figures 4B,D. The score plots accounted for >90% of all variables in PC1 and PC2. The higher nitrogen supply level correlated with all biomass data as well as the contents of phenolic acid, flavonoid, and menthol. The antioxidants as determined by DPPH and ABTS assays were influenced by the nitrogen deficits (N105 and N0). This is in line with previous report which advised that the increase in antioxidant potential could be as a response to the build-up of water-soluble antioxidants such as vitamin C and minerals after the stress (Ibrahim et al., 2012). In contradiction, nitrogen is able to mitigate the stress by promoting osmoregulation, alleviating lipid peroxidation and thereby improving plant physiology and level of active ingredients (Nematpour et al., 2019).
Figure 4. Principal component analysis biplots of physical (A) and biochemical (C) responses of Mentha arvensis grown in the evaporative greenhouse with different water regimes and of physical (B) and biochemical (D) responses of Mentha arvensis grown in the evaporative greenhouse with different levels of nitrogen fertigation.
In the evaporative greenhouse system of Japanese mint, temperature and humidity were maintained. Drought condition induces leaf stomatal conductance, which enhances the rate of photosynthesis through carbon assimilation and transpiration. Loss of H2O from the leaf surface increases water uptake, where limited water supply stimulates the biosynthesis of water-soluble metabolites that alter water potential. Nitrogen was used in the plant to subtle stress including the increase in osmotic regulation, reducing reactive oxygen species, and consequently the total biomass of plants can be improved.
The raw data supporting the conclusions of this article will be made available by the authors, without undue reservation.
SS designed the concept of this study. NK, CJ, and CI drafted the methodology. NK, CJ, PN, and MW conducted the formal analyses and investigation. SS wrote the original draft of the manuscript. Writing—review and editing were completed by SS, CI, HD, and RC. SS and CI were the project supervisors. Funding acquisition was done by HV and RC. All authors contributed to the article and approved the submitted version.
This research project was supported by Fundamental Fund 2022, Chiang Mai University.
The authors declare that the research was conducted in the absence of any commercial or financial relationships that could be construed as a potential conflict of interest.
All claims expressed in this article are solely those of the authors and do not necessarily represent those of their affiliated organizations, or those of the publisher, the editors and the reviewers. Any product that may be evaluated in this article, or claim that may be made by its manufacturer, is not guaranteed or endorsed by the publisher.
Afsar, S., Bibi, G., Ahmad, R., Bilal, M., Naqvi, T. A., Baig, A., Shah, M. M., Huang, B., and Hussain, J. (2020). Evaluation of salt tolerance in Eruca sativa accessions based on morpho-physiological traits. PeerJ 8, e9749. doi: 10.7717/peerj.9749
Albaho, M., Thomas, B., and Christopher, A. (2008). Evaluation of hydroponic techniques on growth and productivity of greenhouse grown bell pepper and strawberry. Int. J. Veg. Sci. 14, 23–40. doi: 10.1080/19315260801890492
Alinejad, S., Sarabi, V., Bakhtvari, A. R. S., and Hashempour, H. (2020). Variation in physiological traits, yield and secondary metabolites of jimsonweed (Datura stramonium L.) under different irrigation regimes and nutrition systems. Ind. Crops Prod. 143, 111916. doi: 10.1016/j.indcrop.2019.111916
Alsafar, M. S., and Al-Hassan, Y. M. (2009). Effect of nitrogen and phosphorus fertilizers on growth and oil yield of indigenous mint (Mentha longifolia L.). Biotechnology 8, 380–384. doi: 10.3923/biotech.2009.380.384
Arjun, P., Kumar Semwal, D., Badoni Semwal, R., Malaisamy, M., Sivaraj, C., and Vijayakumar, S. (2017). Total phenolic content, volatile constituents and antioxidative effect of Coriandrum sativum, Murraya koenigii and Mentha arvensis. Nat. Prod. J. 7, 65–74. doi: 10.2174/2210315506666161121104251
Arve, L., Torre, S., Olsen, J., and Tanino, K. (2011). Stomatal Responses to Drought Stress and Air Humidity. Rijeka: IntechOpen, 267–280.
Azizi, A., Yan, F., and Honermeier, B. (2009). Herbage yield, essential oil content and composition of three oregano (Origanum vulgare L.) populations as affected by soil moisture regimes and nitrogen supply. Ind. Crops Prod. 29, 554–561. doi: 10.1016/j.indcrop.2008.11.001
Bahadori, M. B., Zengin, G., Bahadori, S., Dinparast, L., and Movahhedin, N. (2018). Phenolic composition and functional properties of wild mint (Mentha longifolia var. calliantha (Stapf) Briq.). Int. J. Food Properties 21, 183–193. doi: 10.1080/10942912.2018.1440238
Behera, M., Mahapatra, P., Singandhupe, R., and Kanan, K. (2015). Fertigation studies in Japanese mint (Mentha arvensis L.) under humid climate in Odisha, India. Afr. J. Agric. Res. 10, 1320–1330. doi: 10.5897/AJAR2013.7575
Behera, M., Mahapatra, P., Singandhupe, R., Kundu, D., Kannan, K., Mandal, K., and Amarpreet, S. (2014). Effect of drip fertigation on yield, water use efficiency and water productivity of Mint (Mentha arvensis L.). J. Agric. Phys. 14, 37–43. Available online at: www.researchgate.net/publication/292982190
Berger, R. G. (2007). Flavours and Fragrances: Chemistry, Bioprocessing and Sustainability. New York, PA: Springer Science & Business Media.
Bhattacharya, S. (2016). “Chapter 3 - cultivation of essential oils,” in Essential Oils in Food Preservation, Flavor and Safety, editor V. R. Preedy. San Diego: Academic Press. pp. 19–29.
Brar, A. S., Buttar, G. S., Singh, M., Singh, S., and Vashist, K. K. (2021). Improving bio-physical and economic water productivity of menthol mint (Mentha arvensis L.) through drip fertigation. Irrigat. Sci. 39, 505–516. doi: 10.1007/s00271-021-00722-6
Brown, B., Hart, J., Wescott, M., and Christensen, N. (2003). The critical role of nutrient management in mint production. Better Crops 87, 9–11. Available online at: eurekamag.com/research/003/965/003965655.php
Chen, F., Liu, C.-J., Tschaplinski, T. J., and Zhao, N. (2009). Genomics of secondary metabolism in Populus: interactions with biotic and abiotic environments. Crit. Rev. Plant Sci. 28, 375–392. doi: 10.1080/07352680903241279
Ciriminna, R., Fidalgo, A., Delisi, R., Tamburino, A., Carnaroglio, D., Cravotto, G., Ilharco, L., and Pagliaro, M. (2017). Controlling the degree of esterification of citrus pectin for demanding applications by selection of the source. ACS Omega 2, 7991–7995. doi: 10.1021/acsomega.7b01109
Cowan, I. R. (1982). “Regulation of water use in relation to carbon gain in higher plants,” in Physiological Plant Ecology II: Water Relations and Carbon Assimilation, eds O. L. Lange, P. S. Nobel, C. B. Osmond, H. Ziegler, editors (Berlin, Heidelberg: Springer Berlin Heidelberg), 589–613.
Demidchik, V., Straltsova, D., Medvedev, S. S., Pozhvanov, G. A., Sokolik, A., and Yurin, V. (2014). Stress-induced electrolyte leakage: the role of K+-permeable channels and involvement in programmed cell death and metabolic adjustment. J. Exp. Bot. 65, 1259–1270. doi: 10.1093/jxb/eru004
Dhifi, W., Jelali, N., Mnif, W., Litaiem, M., and Hamdi, N. (2013). Chemical composition of the essential oil of mentha spicata l. from tunisia and its biological activities. J. Food Biochem. 37, 362–368. doi: 10.1111/j.1745-4514.2012.00656.x
Doymaz, I. (2006). Thin-layer drying behaviour of mint leaves. J. Food Eng. 74, 370–375. doi: 10.1016/j.jfoodeng.2005.03.009
Ebrahimian, E., and Bybordi, A. (2012). Influence of ascorbic acid foliar application on chlorophyll, flavonoids, anthocyanin and soluble sugar contents of sunflower under conditions of water deficit stress. J. Food Agric. Environ. 10, 1026–1030. Available online at: www.cabdirect.org/cabdirect/abstract/20123098946
El-Sharkaway, M. A., and Cock, J. H. (1984). Water use efficiency of cassava. I. Effects of air humidity and water stress on stomatal conductance and gas exchange 1. Crop Sci. 24, 497–502. doi: 10.2135/cropsci1984.0011183X002400030017x
Erlandsson, G. (1975). Rapid effects on ion and water uptake induced by changes of water potential in young wheat plants. Physiol. Plant. 35, 256–262. doi: 10.1111/j.1399-3054.1975.tb03903.x
Fahmy, F. H., Farghally, H. M., Ahmed, N. M., and Nafeh, A. (2012). Modeling and simulation of evaporative cooling system in controlled environment greenhouse. Smart Grid Renewable Energy 3, 67–71. doi: 10.4236/sgre.2012.31010
Gao, J.-J., Zhang, Z., Peng, R.-H., Xiong, A.-S., Xu, J., Zhu, B., and Yao, Q.-H. (2011). Forced expression of Mdmyb10, a myb transcription factor gene from apple, enhances tolerance to osmotic stress in transgenic Arabidopsis. Mol. Biol. Rep. 38, 205–211. doi: 10.1007/s11033-010-0096-0
Garcia-Mata, C., and Lamattina, L.. (2001). Nitric oxide induces stomatal closure and enhances the adaptive plant responses against drought stress. Plant Physiol. 126, 1196–1204. doi: 10.1104/pp.126.3.1196
Goudarzian, A., Pirbalouti, A. G., and Hossaynzadeh Menthol, M. (2020). Balance of menthol/menthone, and essential oil contents of mentha × Piperita L. under foliar-applied chitosan and inoculation of arbuscular Mycorrhizal Fungi. J. Essential Oil Bearing Plants 23, 1012–1021. doi: 10.1080/0972060X.2020.1828177
Halliwell, B. (2006). Reactive species and antioxidants. Redox biology is a fundamental theme of aerobic life. Plant Physiol. 141, 312–322. doi: 10.1104/pp.106.077073
Hamed, K., and Seyed Ali Mohammad, M. S. (2018). Yield and oil content of mint under different nitrogen fertilizer treatments. Notulae Sci. Biol. 10, 92. doi: 10.15835/nsb10110221
Hasanuzzaman, M., Hossain, M. A., da Silva, J. A. T., and Fujita, M. (2012). “Plant response and tolerance to abiotic oxidative stress: antioxidant defense is a key factor,” in Crop Stress and its Management: Perspectives and Strategies, B. Venkateswarlu, A. k. Shanker, C. Shanker, M. Maheswari (Dordrecht: Springer Netherlands), 261–315.
Ibrahim, M. H., Jaafar, H. Z. E., Rahmat, A., and Rahman, Z. A. (2012). Involvement of nitrogen on flavonoids, glutathione, anthocyanin, ascorbic acid and antioxidant activities of malaysian medicinal plant labisia pumila blume (Kacip Fatimah). Int. J. Mol. Sci. 13, 393–408. doi: 10.3390/ijms13010393
Jahani, F., Tohidi-Moghadam, H. R., Larijani, H. R., Ghooshchi, F., and Oveysi, M. (2021). Influence of zinc and salicylic acid foliar application on total chlorophyll, phenolic components, yield and essential oil composition of peppermint (Mentha piperita L.) under drought stress condition. Arabian J. Geosci. 14, 691. doi: 10.1007/s12517-021-07024-3
Janpen, C., Kanthawang, N., Inkham, C., Tsan, F. Y., and Sommano, S. R. (2019b). Physiological responses of hydroponically-grown Japanese mint under nutrient deficiency. PeerJ 7, e7751. doi: 10.7717/peerj.7751
Janpen, C., Kanthawang, N., and Sommano, S. R. (2019a). Visualisation of reactive oxygen species during stress of aromatic crop. Med. Plants Int. J. Phytomed. Relat. Ind. 11, 110–115. doi: 10.5958/0975-6892.2019.00014.5
Kamatou, G. P. P., Vermaak, I., Viljoen, A. M., and Lawrence, B. M. (2013). Menthol: a simple monoterpene with remarkable biological properties. Phytochemistry 96, 15–25. doi: 10.1016/j.phytochem.2013.08.005
Khalid, K. A. (2006). Influence of water stress on growth, essential oil, and chemical composition of herbs (Ocimum sp.). Int. Agrophys. 20, 289–296. Available online at: agris.fao.org/agris-search/search.do?recordID=DJ2012039672
Król, B., Seczyk, Ł., Kołodziej, B., and Paszko, T. (2020). Biomass production, active substance content, and bioaccessibility of Greek oregano (Origanum vulgare ssp. hirtum (Link) Ietswaart) following the application of nitrogen. Ind. Crops Prod. 148, 112271. doi: 10.1016/j.indcrop.2020.112271
Lakra, K., and Verma, S. (2021). Water economization in Japanese mint through crop establishment, irrigation and nitrogen levels. Indian J. Agric. Sci. 91, 792–795. Available online at: www.researchgate.net/publication/353514397
Leuschner, C. (2002). Air humidity as an ecological factor for woodland herbs: leaf water status, nutrient uptake, leaf anatomy, and productivity of eight species grown at low or high vpd levels. Flora Morphol. Distribut. Funct. Ecol. Plants 197, 262–274. doi: 10.1078/0367-2530-00040
Liu, M., Cao, B., Zhou, S., and Liu, Y. (2012). Responses of the flavonoid pathway to UV-B radiation stress and the correlation with the lipid antioxidant characteristics in the desert plant Caryopteris mongolica. Acta Ecol. Sinica 32, 150–155. doi: 10.1016/j.chnaes.2012.04.004
Marschner, H. (2012). Preface to Second Edition, Marschner's Mineral Nutrition of Higher Plants. 3rd Edn. San Diego: Academic Press, ix.
Mbarki, S., Sytar, O., Cerda, A., Zivcak, M., Rastogi, A., He, X., Zoghlami, A., Abdelly, C., and Brestic, M. (2018). Strategies to Mitigate the Salt Stress Effects on Photosynthetic Apparatus and Productivity of Crop Plants, Salinity Responses and Tolerance in Plants. Vol. 1, Gewerbestrasse: Springer, 85–136.
Mekonnen, M., Manahile, B., and Mengesha, B. (2015). Screening of Botanical extracts for the control of Japanese mint (Mentha arvensis L.) Leaf rust (Puccinia menthae) in Greenhouse and field condition. Afr. J. Crop Sci. 3, 201–205. doi: 10.13140/RG.2.1.2256.9689
Men, Y., Wang, D., Li, B., Su, Y., and Chen, G. (2018). Effects of drought stress on the antioxidant system, osmolytes and secondary metabolites of Saposhnikovia divaricata seedlings. Acta Physiol. Plantar. 40, 191. doi: 10.1007/s11738-018-2762-0
Misra, A., and Srivastava, N. (2000). Influence of water stress on Japanese mint. J. Herbs Spices Med. Plants 7, 51–58. doi: 10.1300/J044v07n01_07
Nematpour, A., Eshghizadeh, H. R., and Zahedi, M. (2019). Drought-tolerance mechanisms in foxtail millet (Setaria italica) and proso millet (Panicum miliaceum) under different nitrogen supply and sowing dates. Crop Pasture Sci. 70, 442–452. doi: 10.1071/CP18501
Pandey, A. K., Rai, M. K., and Acharya, D. (2003). Chemical composition and antimycotic activity of the essential oils of corn mint (Mentha arvensis) and lemon grass (Cymbopogon flexuosus) against human pathogenic fungi. Pharm. Biol. 41, 421–425. doi: 10.1076/phbi.41.6.421.17825
Pichersky, E., Sharkey, T. D., and Gershenzon, J. (2006). Plant volatiles: a lack of function or a lack of knowledge? Trends Plant Sci. 11, 421. doi: 10.1016/j.tplants.2006.07.007
Rahimi, A., Hosseini, S. M., Pooryoosef, M., and Fateh, I. (2010). Variation of leaf water potential, relative water content and SPAD under gradual drought stress and stress recovery in two medicinal species of Plantago ovata and P. psyllium. Plant Ecophysiol. 2, 53–60. Available online at: www.researchgate.net/publication/292743283
Sarker, U., Islam, M. T., Rabbani, M. G., and Oba, S. (2018). Phenotypic divergence in vegetable amaranth for total antioxidant capacity, antioxidant profile, dietary fiber, nutritional and agronomic traits. Acta Agric. Scand. Section B Soil Plant Sci. 68, 67–76. doi: 10.1080/09064710.2017.1367029
Sarker, U., and Oba, S. (2018). Response of nutrients, minerals, antioxidant leaf pigments, vitamins, polyphenol, flavonoid and antioxidant activity in selected vegetable amaranth under four soil water content. Food Chem. 252, 72–83. doi: 10.1016/j.foodchem.2018.01.097
Sarker, U., and Oba, S. (2019). Salinity stress enhances color parameters, bioactive leaf pigments, vitamins, polyphenols, flavonoids and antioxidant activity in selected Amaranthus leafy vegetables. J. Sci. Food Agric. 99, 2275–2284. doi: 10.1002/jsfa.9423
Sarker, U., and Oba, S. (2020a). The response of salinity stress-induced A. tricolor to growth, anatomy, physiology, non-enzymatic and enzymatic antioxidants. Front. Plant Sci. 11, 559876. doi: 10.3389/fpls.2020.559876
Sarker, U., and Oba, S. (2020b). Polyphenol and flavonoid profiles and radical scavenging activity in leafy vegetable Amaranthus gangeticus. BMC Plant Biol. 20, 499. doi: 10.1186/s12870-020-02700-0
Shah, S. H., Houborg, R., and McCabe, M. F. (2017). Response of chlorophyll, carotenoid and SPAD-502 measurement to salinity and nutrient stress in wheat (Triticum aestivum L.). Agronomy 7, 61. doi: 10.3390/agronomy7030061
Shormin, T., Khan, A., and Alamgir, D. M. (2009). Response of different levels of nitrogen fertilizer and water stress on the growth and yield of japanese mint (Mentha Arvensis L.). Bangladesh J. Sci. Ind. Res. 44, 137–145. doi: 10.3329/bjsir.v44i1.2723
Singh, V. P., Kothari, S. K., Singh, K., and Singh, D. V. (1989). Effect of irrigation and nitrogen on herbage and oil yields of Japanese mint (Mentha arvensis). J. Agric. Sci. 113, 277–279. doi: 10.1017/S0021859600086883
Skalicka-Wozniak, K., and Walasek, M. (2014). Preparative separation of menthol and pulegone from peppermint oil (Mentha piperita L.) by high-performance counter-current chromatography. Phytochem. Lett. 10, xciv–xcviii. doi: 10.1016/j.phytol.2014.06.007
Sommano, S. (2015). Physiological and biochemical changes during heat stress induced browning of detached Backhousia myrtifolia (cinnamon myrtle) tissues. Trop. Plant Biol. 8, 31–39. doi: 10.1007/s12042-015-9148-x
Sommano, S., Kumpoun, W., and Yusuf, N. A. (2017). Subcellular extraction and enzyme characterisation of polyphenol oxidase and peroxidase in Cinnamon myrtle. Acta Physiol. Plantar. 39, 36. doi: 10.1007/s11738-016-2339-8
Taneja, S. C., and Chandra, S. (2012). 20 - Mint A2 - Peter, K.V, Handbook of Herbs and Spices. 2nd Edn. Sawston, Cambridge: Woodhead Publishing, 366–387.
Tepe, B. (2008). Antioxidant potentials and rosmarinic acid levels of the methanolic extracts of Salvia virgata (Jacq), Salvia staminea (Montbret & Aucher ex Bentham) and Salvia verbenaca (L.) from Turkey. Bioresour. Technol. 99, 1584–1588. doi: 10.1016/j.biortech.2007.04.008
Treadwell, D., Migliaccio, K. W., Olczyk, T., Qian, Y., Li, Y., Hochmuth, G. J., Hochmuth, R. C., Simonne, E. H., Osborne, L. S., and Sprenkel, R. K. (2020). Organic Greenhouse container herb production in south florida: fertilizer and potting medial. EDIS 2020, 1–5. doi: 10.32473/edis-ae408-2020
Turkoglu, S. (2015). Assessment of wild mint from Tunceli as source of bioactive compounds, and its antioxidant Activity. Cell. Mol. Biol. 61, 63–68. doi: 10.14715/cmb/2015.61.8.11
Verma, N., and Shukla, S. (2015). Impact of various factors responsible for fluctuation in plant secondary metabolites. J. Appl. Res. Med. Arom. Plants 2, 105–113. doi: 10.1016/j.jarmap.2015.09.002
Villarreal-Guerrero, F., Kacira, M., Fitz-Rodríguez, E., Kubota, C., Giacomelli, G. A., Linker, R., and Arbel, A. (2012). Comparison of three evapotranspiration models for a greenhouse cooling strategy with natural ventilation and variable high pressure fogging. Sci. Hortic. 134, 210–221. doi: 10.1016/j.scienta.2011.10.016
Keywords: abiotic, bioactive compounds, drought, menthol mint, nutrient deficiency
Citation: Sommano SR, Kanthawang N, Janpen C, Norkum ai P, Wongkaew M, Inkham C, Van Doan H and Cheewangkoon R (2022) Physiological and Oxidative Responses of Japanese Mint Grown Under Limited Water and Nitrogen Supplies in an Evaporated Greenhouse System. Front. Sustain. Food Syst. 5:808327. doi: 10.3389/fsufs.2021.808327
Received: 03 November 2021; Accepted: 13 December 2021;
Published: 28 January 2022.
Edited by:
Sajid Ali, Bahauddin Zakariya University, PakistanReviewed by:
Umakanta Sarker, Bangabandhu Sheikh Mujibur Rahman Agricultural University, BangladeshCopyright © 2022 Sommano, Kanthawang, Janpen, Norkum ai, Wongkaew, Inkham, Van Doan and Cheewangkoon. This is an open-access article distributed under the terms of the Creative Commons Attribution License (CC BY). The use, distribution or reproduction in other forums is permitted, provided the original author(s) and the copyright owner(s) are credited and that the original publication in this journal is cited, in accordance with accepted academic practice. No use, distribution or reproduction is permitted which does not comply with these terms.
*Correspondence: Sarana Rose Sommano, c2FyYW5hLnNAY211LmFjLnRo; Ratchadawan Cheewangkoon, cmF0Y2hhZGF3YW4uY0BjbXUuYWMudGg=
Disclaimer: All claims expressed in this article are solely those of the authors and do not necessarily represent those of their affiliated organizations, or those of the publisher, the editors and the reviewers. Any product that may be evaluated in this article or claim that may be made by its manufacturer is not guaranteed or endorsed by the publisher.
Research integrity at Frontiers
Learn more about the work of our research integrity team to safeguard the quality of each article we publish.