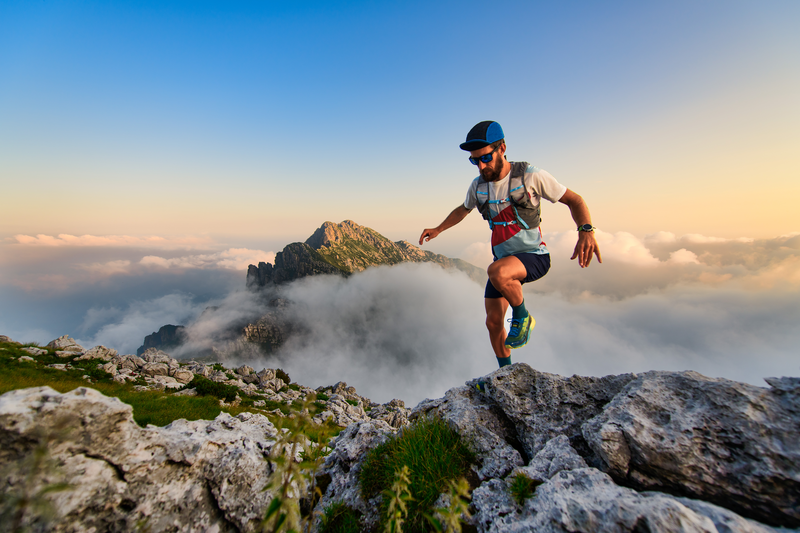
94% of researchers rate our articles as excellent or good
Learn more about the work of our research integrity team to safeguard the quality of each article we publish.
Find out more
ORIGINAL RESEARCH article
Front. Sustain. Food Syst. , 02 December 2021
Sec. Climate-Smart Food Systems
Volume 5 - 2021 | https://doi.org/10.3389/fsufs.2021.778144
This article is part of the Research Topic Mixed Farming Technologies for Increased Resilience, Mitigation and Adaptation to a Changing Climate for Smallholder Farmers in Southern Africa View all 7 articles
Poor soil fertility is a major challenge to crop production in the communal farming areas of Zimbabwe. Intercropping legumes and cereals is a common soil fertility management technology among the farmers. A 3-year field experiment was conducted to evaluate cowpea–sorghum relay intercropping advantages in response to different cattle manure application rates. A 3 × 4 factorial experiment laid in a completely randomized block design (CRBD) with three replicates was conducted. The treatments were three cropping systems (sorghum sole, cowpea sole, and cowpea–sorghum intercrop) and four cattle manure application rates (0, 50, 75, and 100%). Crop growth rate (CGR), grain yield, harvest index (HI), relative competitive ability of each crop, and land equivalent ratio (LER) were measured. Analysis of variance and non-linear regression analyses were done to determine the yield benefits of cowpea–sorghum intercrop and estimate the relative competitive ability, respectively. Application of >75% cattle manure in a cowpea–sorghum intercrop enhanced the sorghum grain yield (75%) and HI (125%) of unmanured cowpea–sorghum plots. Cowpeas had higher CGR (159.6, 166.7 and 149.5 g m−2 day−1 at 7, 21, and 35 days after planting, respectively) at >75% cattle manure application rates on both intercrop and sole cropping than sorghum but with lower grain yield (1.4 t ha−1). Intraspecific competitive stress in sorghum was reduced at a high (>75%) quantity of manure applied. The effects of the intraspecific competition in cowpea were stronger (0.693) on grain yield than biomass at >75% manure application rates. The LER was >1 in all the treatments and was highest (2.73) under the cowpea–sorghum relay intercrop without cattle manure. Cattle manure application at 75% in a cowpea–sorghum intercrop enhanced the grain yield in sorghum and vegetative growth in cowpeas. It is therefore recommended to use the >75% cattle manure application rate in the intercrop if one wants higher grain in sorghum and high biomass in cowpeas possibly for fodder. Further studies are recommended to quantify the rate of increase in available N under the cowpea–sorghum relay intercrop with >75% cattle manure application rates.
A majority (>70%) of Zimbabwe's 14.5 million people live in the communal areas and more than three-quarters of these communal areas are characterized by low and unreliable rainfall and inherently low soil fertility (Anderson and D'Souza, 2014). Many researchers are recommending the growing of drought-tolerant grain crops in most of the communal areas in Zimbabwe, particularly in natural regions III, IV, and V (Parwada et al., 2020). Anderson and D'Souza (2014) observed that N, P, and K were depleting at estimated rates of 20–40, 3.5–6.6, and 17–33 kg−1 ha−1 year−1, respectively, in Zimbabwe's agricultural soils. However, crop productivity is still low due to poor soil fertility management among these communal farmers. The farmers do not usually apply any soil-improving remedies, resulting in soil nutrient mining causing a continuous decline of crop yield. Addo-Quaye et al. (2011) concluded that soil fertility was the major agronomic constraint to crop production even in well-watered areas of southern and eastern Africa. The low crop productivity has been exacerbated by the changing climate in Zimbabwe; the rainfall is becoming more and more unreliable and unpredictable, hence the difficulties in the planning cropping schedules among the farmers (Parwada et al., 2020). Food security is also increasingly threatened, and farmers have to be innovative in their food production methods. There is a need for sustainable crop production methods because many of the communal farmers are poor and cannot afford to purchase off-farm inputs. Numerous sustainable crop production methods are available for the farmers; e.g., good agricultural practices like crop rotation, mixed cropping, and intercropping can offer many advantages to these resource-poor farmers.
Good cropping systems like rotating of cereal–cereal–legume–small grain and intercropping of legume–cereals had been recommended for the communal areas in Zimbabwe. The intercropped legumes fix most of their N from the atmosphere and so do not compete with cereals for N resources (Vesterager et al., 2008). Therefore, the cereal–grain legume intercropping can significantly reverse soil nutrient depletion on smallholder farms (Gitari et al., 2019). Besides the N fixation, the legumes are an important source of nutrition for both humans and livestock (Nadwa et al., 2011). A major benefit of intercropping is that more efficient utilization of the available resources is achieved so there is increased productivity compared with each sole crop of the mixture (Addo-Quaye et al., 2011). Nevertheless, the intercropping is a more complex production system than sole cropping and its efficiency depends directly on the species and management practices. In cases where growth requirements are limiting, intra- and interspecies competition will increase too.
Maximum benefits of intercropping will be achieved if the competition among crop species is reduced (Blanchart et al., 2006). Farmers have to be careful on the combinations of crops to include in an intercropping system; some crops are more competitive than others, and this will reduce the productivity of the other crop. Growth requirements such as water and soil fertility have to be checked and corrected in an intercrop. Based on this understanding, it was difficult to quantify the benefits of intercropping especially in areas where a lot of factors that limit growth exist, e.g., in dry areas and inherently infertile soils. The question is “How beneficial is intercropping in the dry and inherently infertile soils?”
Farmers can also use organic manure for soil fertility amelioration. Organic manure has a long-term positive effect on soil organic matter (SOM) which in turn modifies many biological, chemical, and physical soil properties (Parwada et al., 2020). This is essential to achieving sustainable soil fertility through recycling nutrients and creating soil physical conditions favorable for plant growth. However, insufficient quantities of manure among smallholder farmers are a major challenge especially farmers with few or no livestock (Anderson and D'Souza, 2014). Therefore, there is need to supplement or combine the use of the available organic manure with other soil fertility-improving technologies such as intercropping legumes and cereals.
Evaluation of the beneficial of an intercrop can be done in consideration of usefulness of the crops to the farmer (e.g., crude protein, calories), yield and land use complementarity, and intercrop competitiveness (Gitari et al., 2019). Crop complementarity of an intercrop occurs when the intercrop yields are more than the yields obtained from an equivalent land area planted in a sole crop (Tittonell et al., 2005). The most important index of biological advantage is the land equivalent ratio (LER). The LER is defined by the relative land area required as the sole crop to produce the same yields when intercropped (Gitari et al., 2019). LER provides a standardized basis so that crops can be added to form combined yields. In addition, the total LER can be taken as a measure of the yield advantage; e.g., an LER value of 1.2 indicates a yield advantage of 20% (Gitari et al., 2018).
There are other mechanisms associated with higher yield advantages in intercrops, but the most probable one is that component crops in the intercrop can complement each other and environmental resources are used more efficiently than sole crops (Gitari et al., 2018). However, the actual nature of complementarity and the mechanism involved in efficient utilization of environmental resources by crops, and the actual mechanism involved in intercrop crops, remain unclear. In addition, inter- and intraspecies competition always exists when two crops are grown together. Interspecific competition is very important in intercropping systems as it results in low yields of the component crops. Alternating rows of sorghum and cowpea contribute to reduce interspecific competition and increase sorghum and cowpea yields in this intercropping system (Zhang et al., 2011). However, if not carefully managed, intraspecies competition could be more intense than the interspecific competition.
Unfortunately, the intraspecies competition in an intercrop is usually ignored; hence, this may significantly reduce the economic yield of the crop. Knowledge of the level of intraspecies competition of a crop will assist in determining the crops to combine in an intercrop. Crops with high intraspecies competition will perform poorly in an intercrop competition due to the interspecies competition. It is therefore difficult to exclusively and precisely quantify the benefits of an intercrop; hence, the benefits of intercrops are generalized. The communal farmers in Zimbabwe rely on organic manure such as cattle, poultry, and goat manure as fertilizers. The quantities of manure required per hectare are sometimes too high and therefore can be under-applied leading to low crop productivity. The cowpea–sorghum intercropping system is widely practiced by farmers in Zimbabwean semiarid areas (Parwada et al., 2020). This study was aimed at determining the effects of combining intercropping and manure application at various rates on the yield benefits of relay intercropping cowpea and sorghum, and the intraspecies competition of sole cropping.
A 3-year field experiment was conducted from 2015 to 2017 to investigate the growth and yield of sorghum (Sorghum bicolor L.) and cowpeas (Vigna unguiculata Walp) grown alone and in intercropping with and without cattle manure applied at different rates. The experiment was done in the summer seasons (November to April) of 2015, 2016, and 2017 at a farmer field in Muzokomba area, Buhera, Manicaland Province, Zimbabwe. The farm is >800 m in altitude and is in the agroecology region V, receiving an annual rainfall of 300–450 mm. The area is characterized by severe droughts. The field was under mixed cropping of millet, watermelons, pumpkins, and sweet sorghum before the experiment. The area is characterized by predominantly coarse sands, with pockets of sandy loams to sandy clay loams that can be classified as Lixisols (IUSS Working Group WRB, 2015). The area received a total annual rainfall of 235.1, 245.2, and 238.4 mm in 2015, 2016, and 2017, respectively. The area received below normal rainfall of ≥300 mm per year throughout the study period (Figure 1).
The research field is characterized by two seasons within a year that are hot, wet summers (October–April) and cool dry, winters (May–August). The average monthly summer and winter temperatures for the research field during the study period were 31.2 and 24.3°C, respectively (Figure 1).
A 3 × 4 factorial experiment laid in a completely randomized block design (CRBD) with three replicates was used. The planting time was used as the blocking factor. Each plot was 3 × 1.0 m with three rows in each plot and a 0.50-m space in between plots. A 1:1 crop arrangement was used according to the recommended planting densities of each crop in the study area. The sorghum was planted at 75 × 5 cm and cowpea at 45 × 15 cm. This resulted in planting densities of 266,666 and 148,148 plants ha−1 for sorghum and cowpea, respectively.
Macia and CBC1 sorghum and cowpea varieties were used in this study, respectively. Macia is a short-season variety that matures in 100 days and yields about 3 t ha−1. CBC1 is also a short-seasoned cowpea variety that requires 70–90 days to maturity and yields about 2.5 t ha−1. The sorghum was first to be grown in summer (October to April) of 2015, 2016, and 2017. The sorghum was established in the third week of October, then cowpea was planted 2 weeks later each year for the relay intercrop. The sole crops were established in the third week of October in each year. Cattle manure used in this study was decomposed for 14 weeks before use to achieve partial decomposition. Compound D (7% N, 14% P, 7% K) was used as basal fertilizer at the blanket recommended rate (300 kg ha−1, i.e., 21 kg N ha−1). The cattle manure application rates were then determined according to the N quantities supplied by the compound D application rate. Hence, the quantity of the cattle manure applied was determined using the amount of extractable NO2/NO3 (mg kg−1) in the manure. The manure was applied at 17.789 t ha−1 for the 100% organic manure application rate (Table 1). This manure application rate was then reduced according to the application rates of each treatment combination (Table 1). No irrigation was done during the study period the experiment was rainfed.
Six soil samples were collected at a depth of 0–250 mm using a graduated soil auger in August 2015. Soil samples were taken per plot and then thoroughly mixed to make a composite sample before analyses. The cattle manure was sourced from the farmer's cattle kraal and sun dried for 1 week in order to achieve a uniform moisture content. After 1 week of drying, 500 g of manure was randomly taken for analysis and the bulky stored in a cool shade before use.
Soil pH and electrical conductivities (EC) for both the soil and manure were determined in a soil water suspension (ratio of 1:5) using a TPS meter according to Okalebo et al. (2000). Total elemental concentrations of K, Ca, Zn, Mg, Na, and Cu were determined in the digests using an atomic absorption spectrometer (iCE 3300 Atomic Absorption Spectrometer, Thermo Scientific, Waltham, MA, USA). The soil was also analyzed for texture using the hydrometer method as described by Okalebo et al. (2000). The total carbon (C), nitrogen (N), Olsen extractable P, exchangeable ammonium, and nitrate and nitrite in both the soil and cattle manure were analyzed according to Parwada and Van Tol (2018).
Data was collected from three and five randomly selected cowpea and sorghum plants per plot respectively for the crop growth rate (CGR) analysis. The CGR was collected at a 2-week interval starting from 7 to 35 days after planting (boot stage of Macia and initial flowering stage of CBC1).
where W1 and W2 are the whole plant dry weight at time t1 and t2, respectively, and ρ is the ground area of which W1 and W2 were recorded.
The harvest index (HI) was measured at the physiological maturity stages of each crop, i.e., at 90 and 100 days after planting for the cowpea and sorghum, respectively. The HI was defined as the ratio of grain yield weight (t ha−1) and the total aboveground biomass weight (t ha−1) at maturity and was calculated as follows:
LER was calculated using the following equation:
where YC is the yield (t ha−1) of cowpeas and YS is the yield (t ha−1) of sorghum.
Thus, the LER expresses how much land in a monoculture system is needed to produce the same amount of yield in an intercropping system. Generally, a LER value of 1.0 indicates no difference in yield between the intercrop and the collection of monocultures, value >1.0 indicates a yield advantage for intercrop, and LER value <1.0 indicates a disadvantage of the intercrop.
To estimate the relative competitive ability of the crops with respect to productivity of sorghum (first crop) and productivity of cowpeas (second crop) under the different cattle manure application rates, the original model of Spitters (1983),
was rewritten as:
where Wm1 = the weight of an isolated plant (= 1/b1.0).
a = a parameter characterizing intraspecific competition (= b1,1/b1,2).
ε = relative competitive ability explaining how many individuals crop 2 each individual of crop 1 is equivalent to (= b1,1/b1,2). b1,1 indicates the intraspecific competition between sorghum plants, and b1,2 measures the intraspecific competition effects of cowpea on productivity of sorghum.
The data were checked for normality if confirming the normal distribution; hence, a three-factor analysis of variance (ANOVA) was run using Statistix software version 10.0 to determine the effects of the cropping system, days after planting, and growing season (year) on the growth rate and LER of the cowpeas and sorghum. Means were separated using Tukey's honestly significant difference (HSD) test (a = 0.05). A non-linear regression analysis was also conducted to determine the best fit and to obtain an estimate for the relative competitive ability. Relative competitiveness of the crop species with respect to productivity of sorghum (Sb,b/Sb,o) and productivity of cowpea (So,o/bo,b) was determined. Both estimates were then used for the computation of niche differentiation indices [(bb,b/bb,o) × (bo,o/bo,b)] as described by Spitters (1983). Sb,b is the sorghum biomass under manure, and Sb,o is the sorghum biomass under no manure. All data were analyzed using JMP version 11.0.0 statistical software.
The sorghum requires an average of 85 kg N ha−1, but the soil contained only 58.4 kg N ha−1 (extractable NO2/NO3). This indicated that the extractable N was a limiting nutrient for sorghum production in the Muzokomba area (Table 2). Soil pH (4.2) was acidic and had low CEC and EC values of 65 cmol(+)kg−1 and 4.1 dSm−1, respectively. Generally, the soil was low in total C, N, P, and Ca which were consistent with the lower [8.0 cmol(+)kg−1] cation exchange capacity (CEC) compared to the cattle manure. However, the soil recorded higher quantities of K and Mg compared to the cattle manure (Table 2). The cattle manure and the initial soil had C/N ratios of 7.3 and 1.4, respectively (Table 2).
Table 2. The initial chemical properties of the soil at the Muzokomba area, experimental field, and cattle manure used in the study.
Sorghum biomass and harvestable yield responded in the same way. Yield increased with increased cattle manure application, indicating that higher quantities of manure were required to maximize biomass and grain yield in sorghum. This suggests that intraspecific competitive stress between individual sorghum plants was reduced with an increase in the application of manure (Table 3). The sorghum biomass and grain yield intraspecific competition values were 0.006 and 0.067 on the sorghum plus 100% cattle manure (SM100), respectively (Table 3). The cowpea intraspecific competition values for both the biomass (0.048) and grain yield (0.693) were highest on cowpea + 100% cattle manure (CM100%), but the intra-specific competition was lowest under cowpea + 50% cattle manure (CM50%) (Table 3).
Table 3. Estimated parameters and intraspecific competitive stress for sorghum and cowpea in monoculture.
Maximum grain yield of cowpea was attained at lower quantities (CM75%) of cattle manure applied compared to the maximum grain yield obtained for sorghum. Generally, the intraspecific competition between sorghum plants was much greater than that of cowpea plants in the study area (Table 3). The high value for harvestable yield of both the cowpea and sorghum indicates that the effects of intraspecific competition for grain yield was stronger than for biomass. This suggests that lower (<75%) cattle manure application rates are required for high grain yield in cowpea than in sorghum.
There were significant (p < 0.05) interactions of intercropping (I) × manure application rate × growing season (year) on the LER of the cowpea–sorghum intercrop (Table 4).
Table 4. Analysis of variance for the land equivalent ratios (LERs) in the yield of cowpea–sorghum intercrop and their respective pure stands.
Cowpea grain yield was highest (average 1.6 t ha−1) and lowest (average 0.53 t ha−1) under CSM75 and CS, respectively (Table 5). Higher (1.84 t ha−1) cowpea grain yield was recorded on sole crop + 75% cattle manure than sole crop + 100% cattle manure. We observed a lower grain yield and HI of cowpea at 100% cattle manure than at <100% manure application rates in both intercrop and sole crop (Table 5). Nevertheless, sorghum grain yield and HI were increased with an increase in quantity of cattle manure applied in both intercrop and sole cropping. The sorghum grain yield was highest on 100% cattle manure compared to other manure application rates for both the intercrop and sole crop (Table 5).
Table 5. Grain yield, land equivalent ratio (LER), and harvest index (HI) of sorghum grown in a pure stand and mixed with cowpeas under the different cattle manure application rates.
The higher the HI value, the higher the economic yield value of a crop. In this study, the inclusion of the manure significantly (p < 0.05) affected the HI of the cowpea and sorghum. The HI and grain yield of the crops showed a similar trend on grain yield for each respective crop in both intercrop and sole cropping systems (Table 5). On average, HI values of sorghum for the 3 years were highest (0.82) and lowest (0.26) on cowpea–sorghum + 100% cattle manure and sorghum without manure sole crop, respectively (Table 5). The HI for cowpea was highest at cowpea–sorghum + 75% cattle manure and lowest at cowpea–sorghum + 100% cattle manure (Table 5).
The LER was highest (3.12) under cowpea–sorghum without manure (CS) and lowest on the cowpea–sorghum + 50% cattle manure (CSM50) (Table 5). The LER increased by 82 and 52% in the cowpea–sorghum + 100% cattle manure and cowpea–sorghum without manure, respectively, from 2015 to 2017 (Table 5). The CGR of cowpea and sorghum varied according to the amount of cattle manure applied and days after planting (DAP) in each year. The CGRs of both cowpea and sorghum were highest at 21 DAP and lowest at 35 DAP in all the treatments (Table 6).
Table 6. Effects of cropping system × days after planting (DAP) × growing season (year) on the growth rate of cowpeas and sorghum.
The CGR increased from 7 to 21 DAP and then declined at 35 DAP (Table 6). The CGR of cowpeas increased by 8.18% from 178.3 g m−2 day−1 at 7 DAP to 192.9 g m−2 day−1 at 21 DAP and decreased by 17.78% from 21 DAP to 158.6 g m−2 day−1 at 35 DAP on the cowpea–sorghum + 100% cattle manure (Table 6). This was a similar trend with the growth rate of cowpea under sole cropping; however, marginal changes were observed under cowpea without manure at 7, 21, and 35 DAP (Table 6).
In the cowpea–sorghum intercrop, CRGs of sorghum followed a similar trend observed on the cowpea growth rate. The average CGRs of sorghum were 140.6, 181.4, and 141.2 g m−2 day−1 at 7, 21, and 35 DAP, respectively. This indicated a 29.0% increase from 7 to 21 DAP and a 22.16% decrease at 35 DAP. The average CGRs of sole sorghum crop + 100% cattle manure (SM100) were 189.9, 191.2, and 170.0 g m−2 day−1 at 7, 21, and 35 DAP respectively (Table 6). This trend was observed throughout the other sole sorghum cropping systems used in this study.
Essentially cowpea grain yield, HI, and growth rate were higher in a sole crop than in an intercrop at all <100% cattle manure application rates. The extension report estimates crop yields among smallholder farmers in Zimbabwe to be 0.40 t ha−1 (cowpea) and 0.70 t ha−1 (sorghum) and in a normal rainy season (>300 mm per year) (Parwada et al., 2020). Grain yields of cowpea and sorghum obtained from this study were at least 50% higher than those in the extension report in all the intercrop treatments (Table 5). Addition of manure in the intercrops reduced the intraspecies competitive stress of the cowpea and sorghum plants and hence increased the grain yield and HI under the intercrops. Intraspecific competitive stress of cowpea was shown to reduce at 75 and 50% cattle manure application rates (Table 3). In a sole crop, the competitive stress induced by light, water, and nutrients was minimal compared to the intercrop. The addition of the organic manure in the inherently infertile soils (Table 2) can reduce the soil nutrient competitive stress in crops. This caused the high biomass productivity of cowpeas at a 100% cattle manure application rate and a high sorghum grain yield and HI at higher cattle manure application rates. Zhang et al. (2011) noted that when two species are associated, they interact in such a way that when one exerts a negative effect on the other, the principle of competition is established. In this study, addition (>75%) of cattle manure in the intercrop resulted in vegetative growth of cowpeas and low grain yield; this lowered the LER (Table 5). Reducing the quantities of manure enhanced the normal growth of the cowpea and hence increased LER observed on the cowpea–sorghum without manure (CS) throughout the study period.
The LER of the CS was higher than in all other intercrop systems; this indicated that addition of high (100% application rate) quantities of manure in a cowpea–sorghum intercrop was less beneficial compared to lower (<100%) rates of manure application. There was more competition in cowpea–sorghum + manure systems than sole cowpea. However, these results agreed with Kandhro et al. (2007) who observed a decrease in yield of the legume crop by 23.9% in a sunflower–mungo bean intercrop with different fertilizer application rates, as the mungo bean had prolonged vegetative growth under high N which resulted in reduced grain yield. The mungo bean was vigorously growing at the expense of reproduction which caused a low grain yield. High available N resulted in a prolonged vegetative growth phase of the cowpea and hence the lower grain yield observed on >75% than ≤ 75% manure application rates (Tables 5, 6). Generally, the beneficial effects of intercropping may be attributed to less competition for growth resources and the eventual productivity by both crops. The addition of cattle manure reduced both intra- and interspecies competition on soil nutrients hence high grain yield. The cowpea–sorghum relay intercrop showed yield advantage (LER > 1) in all treatments. Similar results were reported by Tajudeen (2010) who observed high LER (1.16) in sorghum–cowpea relay intercrop which indicated high bioeconomic efficiency. In this study, we used a 1:1 crop row arrangement and Kandhro et al. (2007) noted that the intercropping pattern was also influential on the grain yields in cowpea/sorghum intercrop. Tajudeen (2010) then recommended a 1:1 crop row arrangement for grain and stover yield stability in sorghum particularly in the semiarid savanna ecology.
The high values of the CGR from day 7 to 21 DAP indicate that the vegetative phases of the crop varieties are between 7 and 21 DAP. These findings were similar with the earlier findings by Vesterager et al. (2008). The HI refers to the % ratio of the economic and biological yield of a crop. Results from this study showed considerable variations in the HI value range (0.6–0.8) among the intercropping systems and 0.2–0.6 on sole cropping (Table 6). The observed variation in the HI could be due to the varying competitive effects of cowpea on sorghum caused by the increased N availability from the cattle manure. The different applied quantities of the cattle manure had a significant effect on the growth rate of both the cowpea and the sorghum. The 75 and 100% manure application rates caused vigorous vegetative growth in cowpea which was in turn beneficial to the sorghum growth rate and grain yield (Tables 5, 6).
The HI of sorghum in an intercropping was significantly (p < 0.05) greater than that in sole crop but was proportionally increased with the increase in quantity of manure applied in all cropping systems (Table 5). Intercropping cowpea and sorghum were advantageous to the grain yield of the sorghum. However, a study by Egbe (2010) showed no significant difference in HI between sole crop and intercrop which contradicts our findings. The noted differences between our results and those of Egbe (2010) could be due to the inclusion of cattle manure in our study; addition of manure in the intercrop resulted in an increased economic yield (high HI) of the sorghum. The HI of cowpea in the intercrop was significantly (p < 0.05) greater at 75% than 100% cattle manure application rates. The cattle manure in both cowpea–sorghum intercrop and sole cowpea crop influenced the vegetative growth of the cowpea due to increased available N within the cowpea rhizosphere. The 100% inclusion of cattle manure in both cowpea–sorghum intercrop and sole cowpea crop resulted in prolonged vegetative growth of the cowpea, causing a reduction in grain yield of the cowpea. Although this study did not quantify the additive effects of the manure and biologically fixed N on the available N, the 100% manure application rate showed to promote vegetative growth than reproduction in cowpea which caused low grain yield and high biomass. Reducing the manure application rate to <100% promoted a high grain yield in the cowpeas as an intercrop or sole cropping.
Cowpea–sorghum + cattle manure had beneficial effects (LER > 1) on the yield of the cowpea and sorghum. The grain yield and HI of sorghum were increased by 100% cattle manure inclusion but were reduced in cowpea. Addition of manure (>75%) in the cowpea–sorghum intercrop promoted vegetative growth of the cowpea which had an indirect positive effect on the growth rate and grain yield of the sorghum. Cowpea productivity, grain yield, and HI were inversely proportional to the quantities of cattle manure applied. The cowpea grain yield and HI were highest at the 75% cattle manure application rate in both intercrop and sole crop. Increasing cattle manure (>75% application rates) reduced the intraspecific competition of sorghum plants. Farmers can increase the amount of manure in a legume–cereal relay intercrop if they aim to achieve a high grain yield of the cereal crop, as well as high biomass and low grain yield for the legume crop. Further, studies are recommended to quantify the relationship between the high quantities (100% application rate) of organic manure and the legume effect in a legume–cereal relay intercrop.
The original contributions presented in the study are included in the article/supplementary material, further inquiries can be directed to the corresponding author.
CP conceptualized the research idea, designed the research, monitored data collection, analyzed the data, draft, and write the final manuscript. TC managed the experiment, collected data, and did a literature review. All authors contributed to the article and approved the submitted version.
The research did not receive any specific funding, but was performed as part of employment at the Zimbabwe Open University, Zimbabwe.
The authors declare that the research was conducted in the absence of any commercial or financial relationships that could be construed as a potential conflict of interest.
All claims expressed in this article are solely those of the authors and do not necessarily represent those of their affiliated organizations, or those of the publisher, the editors and the reviewers. Any product that may be evaluated in this article, or claim that may be made by its manufacturer, is not guaranteed or endorsed by the publisher.
The authors gratefully acknowledge Mr. T. Muzokomba for the resources to carry out this study at his field.
Addo-Quaye, A. A., Darkwa, A. A., and Ocloo, G. K. (2011). Yield and productivity of component crops in a maize-soybean intercropping system as affected by time of planting and spatial arrangement. J. Agric. Biol. Sci. 9, 50–57.
Anderson, J. A., and D'Souza, S. (2014). From adoption claims to understanding farmers and contexts: a literature review of conservation agriculture (CA) adoption among smallholder farmers in Southern Africa. Agric. Ecosyst. Environ. 187, 116–132. doi: 10.1016/j.agee.2013.08.008
Blanchart, E., Villenave, C., Viallatoux, A., Barthès, B., Girardin, C., Azontonde, A., et al. (2006). Long-term effect of a legume cover crop (Mucuna pruriens var. utilis) on the communities of soil macrofauna and nematofauna, under maize cultivation, in southern Benin. Eur. J. Soil Biol. 42, S136–S144. doi: 10.1016/j.ejsobi.2006.07.018
Egbe, O. M. (2010). Effects of plant density of intercropped soybean with tall sorghum on competitive ability of soybean and economic yield at Otobi, Benue State, Nigeria. J. Cereals Oilseeds 1, 1–10. doi: 10.5897/JCO.9000005
Gitari, H. I., Gachene, C. K. K., Karanja, N. N., Kamau, S., Sharma, K., and Schulte-Geldermann, E. (2018). Optimizing yield and economic returns of rain-fed potato (Solanum tuberosum L.) through water conservation under potato-legume intercropping systems. Agric. Water Manage. 208, 59–66. doi: 10.1016/j.agwat.2018.06.005
Gitari, H. I., Nyawade, S. O., Gachene, C. K. K., Karanja, N. N., Kamau, S., Sharma, K., et al. (2019). Increasing potato equivalent yield increases returns to investment under potato-legume intercropping system. Open Agric. 4, 623–629. doi: 10.1515/opag-2019-0062
IUSS Working Group WRB (2015). World Reference Base for Soil Resources 2014, Update 2015, International Soil Classification System for Naming Soils and Creating Legends for Soil Maps. World Soil Resources Reports No. 106. Rome: FAO.
Kandhro, M. N., Tunio, S. D., Memon, H. R., and Ansari, M. A. (2007). Growth and yield of sunflower under influence of mungbean intercropping. Pakistan J. Agric. Res. 23, 9–13.
Nadwa, S. M., Bationo, A., Obanyi, S. N., Rao, I. M., Sanginga, N., and Vanlauwe, B. (2011). “Inter and intra-specific variation of legumes and mechanisms to access and adapt to less available soil phosphorus and rock phosphate,” in Fighting Poverty in Sub-Saharan Africa: The Multiple Roles of Legumes in Integrated Soil Fertility Management, eds A. Bationo et al. (Berlin: Springer), 47–83.
Okalebo, J. B., Gathua, K. W., and Woomer, P. L. (2000). Laboratory Methods of Soil and Plant Analysis: A Working Manual. Nairobi: TSBF-KARI-UNESCO.
Parwada, C., and Van Tol, J. (2018). Effects of litter quality on macroaggregates reformation and soil stability in different soil horizons. Environ. Dev. Sustain. 21, 1321–1339. doi: 10.1007/s10668-018-0089-z
Parwada, C., Van Tol, J., Tibugari, H., and Mandumbu, R. (2020). Characterisation of soil physical properties and resistance to erosion in different areas of soil associations. Afric. Crop Sci. J. 28, 93–109. doi: 10.4314/acsj.u28i1.8
Spitters, C. J. T. (1983). An alternative approach to the analysis of mixed cropping experiments. 2. Marketable yield. Neth. J. Agric. Sei. 31, 1–11. doi: 10.18174/njas.v31i1.16957
Tajudeen, O. O. (2010). Evaluation of sorghum-cowpea intercrop productivity in savanna agro-ecology using competition indices. J. Agric. Sci. 2, 229–234. doi: 10.5539/jas.v2n3p229
Tittonell, P., Vanlauwe, B., Leffelaar, P. A., Rowe, E. C., and Giller, K. E. (2005). Exploring diversity in soil fertility management of smallholder farms in western Kenya—I. Heterogeneity at region and farm scale. Agric. Ecosyst. Environ. 110, 149–165. doi: 10.1016/j.agee.2005.04.001
Vesterager, J. M., Nielsen, N. E., and Hogh-Jensen, H. (2008). Effect of cropping history and phosphorous source on yield and nitrogen fixation in sole and intercropped cowpea-maize systems. Nutr. Cycl. Agroecosyst. 80, 61–73. doi: 10.1007/s10705-007-9121-7
Keywords: crop productivity, fertility, interaction, resource poor, soil productivity
Citation: Parwada C and Chinyama TA (2021) Land Equivalent Ratio of Cowpea–Sorghum Relay Intercrop as Affected by Different Cattle Manure Application Rates Under Smallholder Farming System. Front. Sustain. Food Syst. 5:778144. doi: 10.3389/fsufs.2021.778144
Received: 16 September 2021; Accepted: 02 November 2021;
Published: 02 December 2021.
Edited by:
Hupenyu Allan Mupambwa, University of Namibia, NamibiaReviewed by:
Harun Gitari, Kenyatta University, KenyaCopyright © 2021 Parwada and Chinyama. This is an open-access article distributed under the terms of the Creative Commons Attribution License (CC BY). The use, distribution or reproduction in other forums is permitted, provided the original author(s) and the copyright owner(s) are credited and that the original publication in this journal is cited, in accordance with accepted academic practice. No use, distribution or reproduction is permitted which does not comply with these terms.
*Correspondence: Cosmas Parwada, Y3BhcndhZGFAZ21haWwuY29t
Disclaimer: All claims expressed in this article are solely those of the authors and do not necessarily represent those of their affiliated organizations, or those of the publisher, the editors and the reviewers. Any product that may be evaluated in this article or claim that may be made by its manufacturer is not guaranteed or endorsed by the publisher.
Research integrity at Frontiers
Learn more about the work of our research integrity team to safeguard the quality of each article we publish.