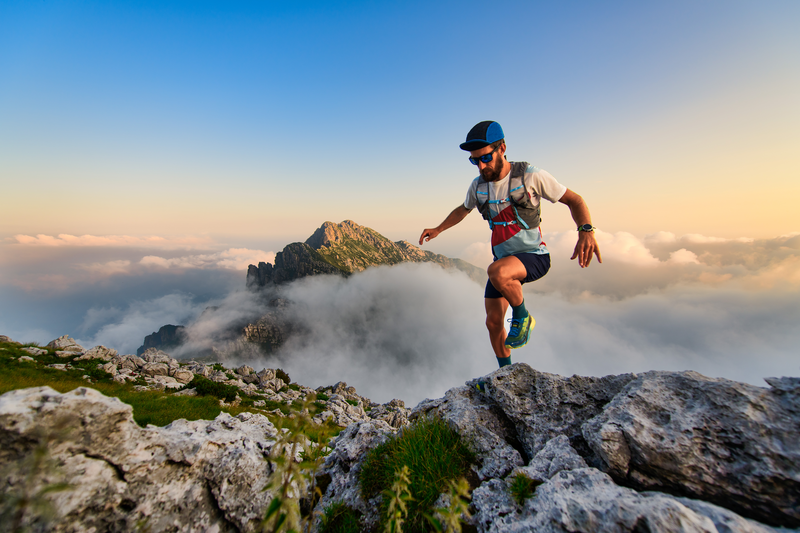
95% of researchers rate our articles as excellent or good
Learn more about the work of our research integrity team to safeguard the quality of each article we publish.
Find out more
REVIEW article
Front. Sustain. Food Syst. , 16 November 2021
Sec. Agroecology and Ecosystem Services
Volume 5 - 2021 | https://doi.org/10.3389/fsufs.2021.767998
This article is part of the Research Topic Nitrogen Use To Improve Sustainable Yields in Agricultural Systems View all 11 articles
Legumes improve soil fertility through the symbiotic association with microorganisms, such as rhizobia, which fix the atmospheric nitrogen and make nitrogen available to the host and other crops by a process known as biological nitrogen fixation (BNF). Legumes included in the cropping system improve the fertility of the soil and the yield of crops. The advantages of legumes in the cropping system are explained in terms of direct nitrogen transfer, residual fixed nitrogen, nutrient availability and uptake, effect on soil properties, breaking of pests' cycles, and enhancement of other soil microbial activity. The best benefits from the legumes and BNF system can be utilized by integrating them into cropping systems. The most common practices to integrate legumes and their associated BNF into agricultural systems are crop rotation, simultaneous intercropping, improved fallows, green manuring, and alley cropping. However, the level of utilizing nitrogen fixation requires improvement of the systems, such as selecting appropriate legume genotypes, inoculation with effective rhizobia, and the use of appropriate agronomic practices and cropping systems. Therefore, using legumes at their maximum genetic potential, inoculation of legumes with compatible rhizobia, and using appropriate agronomic practices and cropping systems are very important for increasing food production. Importantly, the utilization of legumes as an integral component of agricultural practice in promoting agricultural productivity has gained more traction in meeting the demand of food production of the world populace. Priority should, thus, be given to value the process of BNF through more sustainable technologies and expansion of knowledge to the system.
Most soils are facing a decline in soil nutrient status, which is a basic limitation to food production (Sanginga et al., 2003). It was projected, in Africa, that the yearly net nutrient depletion exceeds 30 kg/ha of N and 20 kg/ha of K for arable land in Ethiopia, Kenya, Malawi, Rwanda, and Zimbabwe (Smaling, 1993). The replenishment and enhancement of soil fertility are, thus, progressively regarded as serious to the practice of alleviating poverty. Additionally, developing countries experience the demand of more costs for synthetic fertilizer utilization although their use has adverse and unpredictable problems in the environment: mainly soil, water, and natural area contamination. Consequently, legumes increase soil fertility through the action of microorganisms, which are imperative to affect the soil properties, including soil biological, chemical, and physical properties (Stagnari et al., 2017; Nanganoa et al., 2019; Vasconcelos et al., 2020). The increasing attention of low-input crop production and sustainable agricultural systems from an environmental, economic, and managerial standpoint opened the door for sustained adoption and inclusion of legumes in the agricultural systems (Ghosh et al., 2007; Stagnari et al., 2017; Kebede, 2020b). This is because of the contribution of legumes through their nitrogen (N2)-fixing capabilities and ability to restore soil fertility and break the cycles of diseases and other pests attacking crops (Kebede, 2020a).
The total nitrogen fixation in the world is estimated to be about 1.75 × 1011 Kg, of which symbiotic nitrogen fixation in legumes accounts for about 8.0 × 1010 Kg by fixing, on average, 20–200 kg N fixed ha−1 year−1, and the other near half is industrially fixed while producing N fertilizers (about 8.8 × 1010 Kg) (Shah et al., 2021). Biological nitrogen fixation (BNF) through rhizobia–legume symbiosis is, thus, the best alternative and a more sustainable process by a group of symbiotic bacteria, so-called rhizobia, which fix the atmospheric N2 and make the fixed nutrient available to the host legume and other crops in the cropping system (Stagnari et al., 2017). Increments in crop yield following nitrogen-fixing legumes arises from the role of this system in modifying the activity of soil organisms; the chemical or physical characteristics of the soil; and/or through breaking the cycles of diseases, insects, and other pests (Wani et al., 1995). The use of legumes in agricultural systems and utilization of associated BNF systems provides economically feasible and environmentally sound ways of decreasing external inputs and improving the soil nutrient content and, hence, can be suggested for the nutrition of sustainable agriculture (Peoples et al., 1995; Postgate, 1998; Kebede, 2020a).
Full utilization of BNF and maximal benefit from BNF systems can be recognized through integrating legumes into agricultural systems in which the benefits of BNF can be extended to crops and cropping systems (Fujita et al., 1992; Stagnari et al., 2017; Kebede, 2020a). The well-known agricultural systems of integrating legumes into cropping systems include crop rotation, simultaneous intercropping, improved fallows, green manuring, and alley cropping (Ghosh et al., 2007; Meena et al., 2018; Nanganoa et al., 2019; Kebede, 2020a; Lengwati et al., 2020). Wider legume technology adoption and utilization, including methods that improve the BNF system and integrate it into agricultural cropping systems are imperative to enhance agricultural production. Consequently, an increment in the level of nitrogen fixed could be attained by adopting management practices that influence BNF in agricultural production systems, such as selecting legume genotypes, inoculating with effective rhizobia, and the use of good agronomic practices and cropping systems (Wani et al., 1995; Montañez, 2000; Vanlauwe et al., 2019). Therefore, improvement and utilization of BNF are very important, particularly in the developing world, where much of the increases in food production must come to accommodate the increasing world population. Hence, this paper aims to review the contribution, utilization, and improvement of legumes-based BNF in agricultural systems.
BNF enables legume crops to rely upon atmospheric nitrogen, which is essential in legume-based agricultural systems in which nitrogen fertilizers are limited as legumes integrated within different cropping systems increase the fertility of the soil (Ghosh et al., 2007; Meena et al., 2018; Lengwati et al., 2020). Legume crops, such as common bean, cowpea, soybean, lablab, and groundnut, are important hosts for rhizobia to perform BNF. In addition to providing the fixed nitrogen in the cropping system, legumes also aid in solubilizing unsolvable phosphorus (P) in the soil, increasing soil microbial activity, ameliorating the soil physical environment, restoring organic matter, and smothering weeds (Giller, 2001; Stagnari et al., 2017). BNF by legumes (e.g., fava bean, lentil, pea, chickpea, alfalfa, red clover, etc.) ranges from 21 to 389 kg ha−1 (Vasconcelos et al., 2020). Stagnari et al. (2017) indicated that the magnitude of BNF and associated contribution varies across legume species, soil properties, climatic conditions, and cropping systems (i.e., monoculture, mixed culture, crop rotations, etc.) as well as soil management strategies. The BNF capability of commonly cultivated legumes across the world is shown in Table 1, and the tables indicated that BNF capability varied across legume species and locations.
Furthermore, soil fertility can be restored with nitrogen obtained from legume residue decomposition, which mainly depends on how their residues are exploited (whether incorporated, which is of more benefit; totally removed from the field; or burned) (Ghosh et al., 2007; Thilakarathna et al., 2016). Legume species commonly used for grain production and green manure can fix nitrogen ranging from 100 to 300 kg ha−1 from the atmosphere (Fujita et al., 1992). In a normal ecosystem, legumes can fix nitrogen in the range of 11.34–34.02 kg of nitrogen per acre per year. In cropping systems, for example, perennial legume crops, such as alfalfa, sweet clovers, true clovers, and vetch, can fix up to 250–500 lb of N per acre per year (Walley et al., 1996). Similarly, legumes such as cowpeas, peanuts, fava beans, and soybeans can fix up to 113.4 Kg nitrogen ha−1. The use of these legumes in a cropping system, including rotation, intercropping, green manure, and legume-enriched pastures, has significant advantages over sole cropping systems in terms of fertilizer use and, hence, emissions of the greenhouse gases CO2 and N2O (Peoples et al., 1995; Kebede, 2020a). Stagnari et al. (2017) considered legumes to be competitive crops in terms of both environmental and socioeconomic benefits with the potential to be included in modern agricultural systems, which are characterized by a reducing crop diversity and excessive use of fertilizers and agrochemical inputs. Particularly, the positive contributions of legumes to the agricultural system mainly arise from legume-specific traits of fixing atmospheric nitrogen into nitrogen-rich organic compounds and indirectly from their reduced reliance on agronomic inputs (Vasconcelos et al., 2020). Besides this, the legume-based nitrogen fixation is of importance to the cropping systems as it is used by the N2 fixing and nonfixing crops growing nearby as the benefits can be supplemented from the fixing plants (Vanlauwe et al., 2019; Kebede, 2020a,b; Shah et al., 2021).
Legumes in the mixed agricultural system can lead to more efficient use of soil nutrients and the significant release and transfer of fixed N during the cereal phase, which also results in an improved N yield of the mixed cereal and the overall mixture (Louarn et al., 2015). Direct nutrient transfer is the transfer of nutrients from a nitrogen-fixing legume to another crop during the growth of the crop in an intercrop association with a legume's component and/or as remaining nitrogen for the advantage of a subsequent crop. Thilakarathna et al. (2016) stated that nutrient transfer in a legume-based BNF system is the transfer of nutrients from a donor plant (legume) to receiver plants (cereals) either without undergoing mineralization or through mineralization followed by the uptake of nutrients by the receiver plants. In many instances, it is predictable that an amount of the fixed nitrogen by the intercropped legume crop is made accessible to the accompanying non-legume during the cropping season, and the direct transfer of fixed nitrogen from legumes to a companion crop happens in a mixed cropping system (Fujita et al., 1992). Confirmation of nutrient transfer from legumes to cereals is achieved in intercropping and rotation studies through the excretion of the root, nutrients leached from plant leaves, and leaf fall (Yusuf et al., 2009). The main ways of nutrient transfers can be grouped into above- and below-ground nutrient transfer. According to Thilakarathna et al. (2016), nitrogen is obtained from decayed legume nodules, roots, root border cells, root caps, sloughed cells, and the epidermis (water-insoluble resources), which more importantly donate to the below-ground nutrient, primarily nitrogen, transfers.
When examining various mechanisms of nitrogen transfers, nodules, and root decomposition are more significant but can differ significantly by legume species. It is projected that nitrogen ranging from 3 to 102 kg ha−1 yr−1 can be transferred through the decomposition of nodules and roots in legumes, which is equal to 2–26% of the biologically fixed nitrogen in legumes (Thilakarathna et al., 2016). The below-ground nutrient transfer comprises three different pathways, i.e., decomposition, root exudation, and mycorrhizae-mediated nutrient transfer and is shown in Figure 1.
Figure 1. Potential mechanisms of below-ground nitrogen transfers from legumes to non-legumes (decomposition of nodules and roots, root exudates, and mycorrhizal-mediated nitrogen transfer) and the wide range of abiotic and biotic aspects that influence nutrient transfers. Source: Thilakarathna et al. (2016).
The transfer of biologically fixed nitrogen to neighboring and/or succeeding crop plants is highly variable and can range from as low as 0% to as high as 73%, depending on various factors (Islam and Adjesiwor, 2017), which can be the highest reported levels of 75–110 kg N ha−1 y−1 (Louarn et al., 2015). A common practice in agricultural systems for utilizing nutrient transfer from BNF is intercropping N2-fixing legumes with non-N2-fixing crops. A study by Eaglesham et al. (1981) revealed that 24.9% of nitrogen fixed by cowpea can be transferred to intercropped maize. Up to 35% of N in maize grown after pigeon pea was revealed by isotope dilution to be from N fixation, and part of the fixed N was from below-ground parts. Similarly, Osunde et al. (2004) revealed that the quantity of nitrogen derived from N2 fixation is 40% in the intercropped soybean and 30% in the sole crop without the addition of fertilizer. Furthermore, Mandimba (1995) reported that the N input of groundnut to the growth and yield of maize in an intercropping system is equal to the fertilization of 96 kg of N/ha at a proportion of plant population densities of individual maize plants to four groundnut plants. The estimates of the amount of plant phosphorus and nitrogen derived from symbiotic N2 fixation for frequently produced legume crops in tropical and subtropical agricultural systems are shown in Table 2.
Table 2. The proportion of phosphorus and amount of plant nitrogen attained from symbiotic N2 fixation of grain legumes frequently produced in tropical and subtropical systems as adapted from Peoples and Craswell (1992).
The degree of nitrogen transfer depends upon the quantity and concentration of legume N, microbial mineralization and immobilization in the rhizosphere, the availability of other N sources, and the degree of utilization by the associated crop (Peoples and Craswell, 1992; Thilakarathna et al., 2016; Islam and Adjesiwor, 2017). The amount of N available and the pathway of transfer are also seen to depend on the legume species (Wolfe and Cormack, 2002). For instance, Dubach and Russelle (1994) measured 13 kg/ha of symbiotically fixed N could be released from decomposing alfalfa roots but only 2 kg/ha of fixed N from decomposing three-leaved plant roots. By comparison, the three-leaved plant was seen to have further root nodules, estimated to provide 6 kg/ha N to the top 30 cm soil as compared with only 2 kg/ha from alfalfa nodules. The quantities of nitrogen fixed and the amount transferred to the soil and/or subsequent crops in different agricultural systems are indicated in Table 3.
Table 3. The quantities of nitrogen fixed and the amount transferred to soil or subsequent plants in different agricultural systems as taken from Islam and Adjesiwor (2017).
Decomposition of nodules and roots of legumes are also believed to be significant in the transfer of nitrogen (Thilakarathna et al., 2016). Though these parts, generally, have only a portion of the entire plant's nutrients, the amount of the plant root system, which might be decaying during plant growth, has not been estimated yet. Moreover, the likelihood of nitrogen released from the living roots of legumes can also contribute to the transfer of nitrogen (Fujita et al., 1992). On the other hand, the transfer of nutrients to companion non-legume crops within the cropping system and growing season depends on the legume species, the amounts of the plant components in the stand, the comparative maturities of the accompanying crops, and the vigor and period of plant growth (Thilakarathna et al., 2016). Soil-related factors also influence the efficiency of nutrient transfer. The estimates of probable N2 fixation are also unlike in legumes and under various farming systems. The levels of nitrogen fixation and amount of nutrient transfer are also reliant on soil moisture and water supply, rhizobia inoculation, cropping system, nitrogen fertilizer applications, and soil fertility status (Peoples and Craswell, 1992).
Part of the symbiotically fixed nitrogen in legumes is available to succeeding crops through the decomposition and mineralization of the legume residues (Thilakarathna et al., 2016; Islam and Adjesiwor, 2017). The residues of legumes can be a source of more mineral nitrogen to succeeding crops than the residues of cereal due to their relatively high nitrogen contents and relatively low C:N ratio in legume residue as compared with cereal residues. Nutrients derived from decomposed roots, nodules, root caps, root border cells, sloughed cells, and the epidermis (water-insoluble materials) significantly contribute to below-ground nutrient transfer (Louarn et al., 2015). Cereals cultivated in sequence with legume crops obtain nitrogen benefits as compared with cereal monoculture. The utilization of legume crops in various cropping systems can cause a significant and progressive yield increment on subsequent non-legume crops as compared with rotations with non-legume crops (Kebede, 2020a). Many factors are assumed to explain these results, including enriched nitrogen availability following the legume and other rotational effects, such as decrease of disease and other pests and higher mycorrhizal colonization rate and diversity in the soils. Murphy-Bokern et al. (2017) stated that the nitrogen-rich root, shoot, and leaf biomass of legumes, which is enabled by BNF, improves the availability of N to neighboring or succeeding nonlegume crop plants as exudates, and living and senescent biomasses provide additional below-ground N-enriched input to the soil. However, reliable estimates of nitrogen fixation and residual soil nitrogen are required to determine nitrogen contribution of legume to subsequent or associated crops (Adeleke and Haruna, 2012).
Nutrients derived from decomposed roots, nodules, root caps, root border cells, sloughed cells, and the epidermis (water-insoluble materials) significantly contributes to below-ground nutrient transfer (Louarn et al., 2015). Cropping systems, such as crop rotation comprising legumes, can decrease the amount of nitrogen fertilizer applied in succeeding crops. According to Mayer et al. (2003), nitrogen derived from legume rhizodeposits contribute to an increase of 35–44% in residual nitrogen content in the soil and constituted 79–85% of the below-ground nitrogen of plants at the maturity stage. Besides this, Jensen (1996) revealed that 47% of the whole below-ground nitrogen derived from plants can be acquired from legume root depositions. Moreover, enhanced BNF through the use of rhizobia inoculants helps in improving available nitrogen and phosphorus in the soil once the crop is harvested, and it can be utilized by the next crop (Matse et al., 2020).
Several reports reveal that BNF can be improved by inoculating host legumes with compatible rhizobia (Wani et al., 1995; Zahran, 1999; Adeleke and Haruna, 2012; Bhowmik and Das, 2018). The improved nitrogen fixation can leave crop residual nitrogen in the soil, and it contributes to organic matter and can be a source of inexpensive nutrients for the next cropping season. In food legumes, the nitrogen is divided into either the harvested seed or the non-harvested vegetative parts of the crops, such as nodulated roots, stems, and leaves, which usually stay as crop residues. The leaf falls throughout legume crop growth in the nodulated roots are also testified to comprise up to 40 kg N ha−1 (Buresh and De Datta, 1991). Ghosh et al. (2007) stated that legumes, such as soybean, pigeon pea, cowpea, and groundnut, cultivated as an intercrop in maize had a positive residual contribution on the yield of the following wheat crop. The use of legume crops in the cropping system and utilization of BNF is, therefore, considered to have soil fertility enhancement and economic benefits (Ndakidemi et al., 2006).
During the harvest of legume seeds and residue incorporation in the soil, the entire nutrient found in the plant is unequally dispersed between the various vegetative parts (Louarn et al., 2015; Thilakarathna et al., 2016). These parts also vary from each other in the level to which they release mineral nutrients to the soil and, eventually, to the subsequent crops. The nutrient alterations happening during decomposition of crop residues are influenced by residue management, temperature, soil physical and chemical properties, and whether the soils are flooded and/or remain aerobic (Buresh and De Datta, 1991). However, decomposition of the residues may be improved by lignin and polyphenol content even though tissue nitrogen concentration, C:N ratio, and soil water status are the principal aspects affecting the rate of mineralization and obtainability of legume residues to succeeding crops (Peoples and Herridge, 1990). When legume green manure is grown and intended to be used in the agricultural system, however, all plant parts are returned to the soil. In this case, the number of nutrients left in the soil and nutrient concentration in the legume's parts returned to the soil are expected to be greater than when the seed is harvested from food legumes. As a result, decomposition might also be anticipated to be quick, and the proportion of legume nitrogen mineralized is high (Peoples and Herridge, 1990). According to Beri et al. (1989), a considerable increment in yield of cereals following legume green manuring could range from 50 to 120 kg ha−1 of nitrogen fertilizer. Therefore, legume green manuring can offer substantial quantities of nutrients to the soil that can significantly benefit succeeding crops.
Legumes-based cropping systems improve several aspects of soil fertility, including soil organic carbon and humus content, N and P availability and uptake, and organic C and N as well as releasing hydrogen gas as a by-product of BNF, which promotes bacterial legume nodules' development in the rhizosphere (Stagnari et al., 2017). Plant nutrient availability and uptake are dependent mainly on the amount, concentrations, and activities taking place in the root zone of the soils along with the ability of the soils to replace the limiting nutrients in the soil's solution (Makoi et al., 2013). Nitrogen and phosphorus are the principal elements that are abundantly available in the soil and atmosphere, respectively, but found in forms inaccessible to plants. These elemental nutrients are usually the limiting factors for the growth and development of plants (Mmbaga et al., 2014). Stagnari et al. (2017) divided the agricultural contribution of grain legumes in two as (1) the “nitrogen effect” component, which is due to the nutrient provision from BNF, which is highest in situations of low N fertilization to subsequent crop cycles, and (2) the “break crop effect” component, which includes nonlegume-specific benefits, such as improvements of soil organic matter and structure, phosphorus mobilization, soil water retention and availability, and reduced pressure from diseases and weeds. Hence, the contributions of legumes are highest in legumes included in diverse cropping systems.
Legumes-based BNF has a positive effect on the availability and real chemistry of soil nutrients, which, thus, stimulate the availability and uptake of plant nutrients. For instance, Fujita et al. (1992) showed that the maximum wheat nitrogen uptake was obtained when the crop is cropped following a maize–soybean or maize–groundnut intercrop system than after lone maize cropping. Besides this, microbial inoculants, especially rhizobia species, have come to be a promising solution to some of the problems associated with intensive agriculture by enhancing nutrient availability and uptake and eventually enhanced yield. Makoi et al. (2013) revealed improvement in the uptake of N and P following inoculation of different legumes with effective strains of rhizobia. Nyoki and Ndakidemi (2014) found that Bradyrhizobium japonicum inoculation complemented with phosphorus application in cowpea enhanced the uptake of nutrients such as N and P. Similarly, Desta et al. (2015) indicated that the inoculation of legume crops can improve nodulation capacity and nitrogen fixation, which, in turn, enhance nutrient uptake of fava bean.
Legume species also have mechanisms to solubilize and recover phosphorus from unavailable forms in association with rhizobia. For instance, Stagnari et al. (2017) reported an increase in P availability at the rhizosphere level in the intercropping system than in sole cropping. One mechanism is the organic acid exudation from the root legumes, which reduces the pH in the soil neighboring the roots and solubilizes and discharges phosphorus. The second mechanism is the release of phosphatase enzymes into the soil, which can decompose organic material containing phosphorus. The third mechanism is an interaction reaction between the root surface of the legume and the insoluble phosphorus surrounding the plant roots (Ae and Shen, 2002). However, the degree of the contribution of legumes in phosphorus acquisition to the cropping system is greatly reliant on the type of soil and existing soil atmosphere. More broadly, Peoples and Craswell (1992) described the benefits of legumes in the agricultural system in the following ways:
i Improvements in soil structure following legumes or improvements in soil water-holding and buffering capacity and increased nutrient availability associated with incorporation of legume residues.
ii Breaking of cycles of crop pests and diseases and phytotoxic and allelopathic effects of different crop residues.
iii Improvement of soil microbial activity and probably heterotrophic nitrogen fixation following the addition of legume residues.
iv Additional residual nitrate found in the soil resulting from a legume rather than after a cereal or other non-legume, which might be associated with fewer nitrates being taken up by the legume or result from stimulation of mineralization rates under a legume cropping system.
Legumes are considered as competitive crops in terms of both environmental and socioeconomic benefits with the potential to be included in modern agricultural systems, which are characterized by a reduced crop diversity and excessive use of fertilizers and agrochemical inputs (Stagnari et al., 2017). They play fundamental roles at the production system level due to their ability to fix atmospheric nitrogen, making them potentially highly suitable for inclusion in low-input agricultural systems as well as at cropping-system levels as diversification crops in agroecosystems, breaking the cycles of pests and diseases, and balancing the soil nutrient deficit in the agricultural system. Full utilization of legumes-driven BNF and maximal benefit from these systems can be recognized through investigation and resolution of the most important limitations to the ideal performance in the field, their adoption, and practices by farmers (Fujita et al., 1992). There are practices utilized to integrate the BNF benefits into agricultural systems in which the benefits of BNF can be extended to crops and cropping systems. The most common agricultural systems of integrating legumes into cropping systems include crop rotation, simultaneous intercropping, improved fallows, green manuring, and alley cropping. These cropping systems are used worldwide to exploit the nitrogen-fixing symbiotic relationship, and they can be applied in separation or hybrid mixtures. However, the selection of an appropriate cropping system is only one management step; i.e., pairing the system with a nitrogen-fixing symbiotic relationship adds dimension to the decision-making process.
Crop rotation is the oldest agricultural practice that comprises the long-term, everlasting farming of well-ordered sequences of crops on the same land. Crops are planted in accordance with their nutrient needs in which deep-rooted plants are followed by their shallow-rooted counterparts to allow nutrients to restore at various depths. Legumes are usually handled as components of crop rotations to optimize the management of pests, weeds, and diseases and to exploit nutrient availability through the soil profile (Murphy-Bokern et al., 2017). For example, deep-rooted legume crops, such as the common bean, cowpea, and pigeon pea can access nutrients below the cereal rhizosphere (1–3 m) rather than cereal crops. The incorporation of these legumes into crop rotations has proven to increase the yields of subsequent crops (Peoples et al., 2009). For example, Sanginga et al. (2003) described that the real proportions of nitrogen fixed by soybeans and the residual nitrogen contributions to the succeeding cereal crops in a crop rotation system range from 38 to 126 kg N ha−1. According to Lengwati et al. (2020), nitrogen contribution obtained from Bambara groundnut, groundnut, mung bean, black gram, and cowpea is 83, 67, 39, 36, and 32 kg ha−1, respectively. The fixed nitrogen benefit of these five legume crops to the subsequent maize crop, which is assessed using grain yield, shoot biomass, and total biomass as compared with monocropping and zero nitrogen plots is shown in Figure 2.
Figure 2. The interactive effects of previous crops and levels of nitrogen fertilizers on (A) grain yield, (B) total biomass, and (C) shoot biomass of maize planted in rotation with five different legumes. The horizontal straight line indicates increments in yield caused by nitrogen levels of 20, 40, and 60 kg ha−1 relative to 0 kg ha−1. Source: Lengwati et al. (2020).
Legume rotations are widely recognized to improve soil structure, permeability, microbial activity, water storage capacity, organic matter content, and resistance to erosion, thereby increasing crop yields and sustainability of the agricultural systems (Murphy-Bokern et al., 2017). Therefore, the addition of legume crops in crop rotation systems by smallholder farmers is a profitable and ecologically sound way of improving the nutrition of both the legume and subsequent crops (Kebede, 2020a). Consequently, the net outcome is an increment in the yields of crops and soil nutrient uptake and availability, thus reducing the use of synthetic fertilizers. For instance, a comparison of the grain yields from zero nitrogen application in maize planted subsequently after different legumes with grain yields of maize under sole cropping receiving nitrogen fertilizer indicate that the symbiotic nitrogen contribution of the legumes to the maize was about 20 kg N ha−1 for legumes, such as groundnut, black gram, cowpea, mung bean, and Bambara groundnut (Lengwati et al., 2020).
Mixed and relay cropping are two practices that involve simultaneous cropping of two or more crops. Mixed cropping is the same as intercropping; however, it does not include a precise geometric pattern, such as rows. Relay cropping is a cropping system that involves establishing the second crop directly onto the first crop before harvesting, thus, permitting the cultivation of two crops during the same year. In a mixed cropping system, the action of rhizodeposition advances the nitrogen uptake in the companion crop (Fustec et al., 2010). For instance, intercropping of legumes and cereals provides an opportunity to increase the input of fixed N into an agricultural system in both the short term through direct N transfe, and the long term through mineralization of residues (Murphy-Bokern et al., 2017). Yield increment and other benefits from simultaneous cropping as compared with monocropping are usually accredited to joint balancing effects of the component crops, such as improved total utilization of existing nutrients and resources (Fujita et al., 1992). Ghosh et al. (2007) stated that the yield of sorghum in sorghum–legume intercropping is by far greater than that of the maximum yield of sorghum in sole cropping systems (Figure 3). The authors ascribed the possible reason to improved growth and enhanced uptake of N, P, and K by sorghum along with effective weed oppressing by the legume's intercrops. Murphy-Bokern et al. (2017) also revealed that intercropping has positive effects on phosphorus availability and uptake as phosphorus can be released into the soil solution in the form of phosphate ions and become available for plant uptake.
Figure 3. Sorghum intercropped with various legumes. Source: Ghosh et al. (2007).
Improved fallows involve planting a beneficial legume crop during the fallow period to restore soil fertility to enhance subsequent crop production. It is the planting of rapid-growing legume tree/shrub species as a replacement to normal fallow to attain the advantages of the latter in a smaller time. In legume tree fallows, the wood can be harvested, and N-rich components (leaves, pods, and green stems) tend to be incorporated into the soil before the rainy season (Sanchez, 1999). The nutrient supply, such as nitrogen, is greater in improved fallow than in cropped land as the plants store nutrients from the air and bottomless layers of the soil, and falls their leaf litter to improve the soil and preserve moisture (Lemage and Tsegaye, 2020). Nanganoa et al. (2019) indicated that different legume fallow systems had variable effects on the soil physicochemical properties of which the maximum distinguished effect is the total nitrogen content (ranging from 0.19 to 0.24%), organic carbon (SOC) content (ranging from 2.77 to 3.36%), and available phosphorus (ranging from 12.50 to 16.12 mg/kg) (Table 4).
Table 4. Effect of five different fallow systems (natural, soybean, groundnut, cowpea, and common bean) on soil physicochemical properties (Mean ± SD) after 19 weeks of crop cultivation.
In particular, the off-season farming of improved legume fallows and their succeeding incorporation as green manure is vital to improve soil production and productivity by adding nutrients such as N and organic C and by smothering the weeds (Lemage and Tsegaye, 2020). Nair et al. (1999) observed that 1-year Sesbania sesban fallow increased the yields of succeeding maize crops by 50–80%, and 2-year fallows showed yield increases of 150–270%. The residual benefits of different legume fallows were observed for 4 years after fallowing, and yields were three times greater than monocropped maize. Lemage and Tsegaye (2020) showed that abandoned agricultural land can be rehabilitated using improved legume fallow, which can enhance soil pH, organic carbon, available phosphorus, available potassium, and total nitrogen content. Besides this, Nanganoa et al. (2019) indicated that different legume fallow systems had varying grain yield and economic benefits, ranging from enhanced soil nutrient contents, increased grain yield, and increased economic value of the fallow land. The authors revealed that grain yield ranging from 1.0 to1.9 t/ha can be obtained using different legume fallow systems with the highest in groundnut (1.9 t/ha), followed by soybean (1.6 t/ha), common bean (1.3 t/ha), and cowpea (1.0 t/ha) (Figure 4).
Figure 4. Yield (t ha−1 ± SD) of four different fallow grain legumes (soybean, groundnut, cowpea, and bean). Data with different letters are significantly different (P < 0.05). Source: Nanganoa et al. (2019).
Green manures are locally produced and non-decomposed plant matter, especially legumes, which are applied to the soil surface (as in conservation agriculture) or tilled into the soil so that they help as a mulch and soil amendment. Green manures are cultivated for the specific purpose of providing nutrients to the agricultural system through biomass decomposition (Gangwar et al., 2004; Fageria, 2007; Ghosh et al., 2007). Legume-based green manures are grown with the specific aim of increasing N availability in a system by making use of the N fixed from the atmosphere by the legume (Murphy-Bokern et al., 2017). Green manuring is advantageous for improving the yields of crops and the fertility of the soil. Legume crops are higher-ranking green manure crops as compared with non-leguminous crops due to their ability to fix atmospheric nitrogen. Incorporation of legume green manures and their decomposition has a solubilizing consequence of macronutrients, such as N, P, and K, and micronutrients (Zn, Fe, Mn, and Cu) in the soil and can also alleviate deficiency of different nutrients by recycling nutrients through green manuring (Ghosh et al., 2007). Further, Meena et al. (2018) revealed that legume green manuring can increase the sustainability of agriculture by enhancing retention of different nutrients, improving soil fertility, and decreasing soil erosion and global warming. The BNF process and mineralization of legume green manure crops in soils are shown in Figure 5.
Figure 5. Nitrogen fixation and mineralization of legumes green manure in the soil. Source: Meena et al. (2018).
Gangwar et al. (2004) revealed that Leucaena incorporated into the soil as green manure at the rate of 6 t ha−1 year−1 before sowing of rice and wheat contributed about 80 kg organic N ha−1 year−1, which can substitute 25% mineral N fertilizer and substantiated to be more financially rewarding than monocropping of rice–wheat. The integration of legumes' green manure into the soil provides organic constituents, such as organic acid, amino acids, sugars, vitamins, and mucilage, during crop growth and after decomposition. These materials are important for binding soil particles together and forming better soil aggregates, which result in increased hydraulic conductivity, water infiltration, water-holding capacity, and overall pore space of the soil, which are desirable characteristics to enhance soil fertility and crop yield (Meena et al., 2018). The green manuring legumes can also increase the uptake of phosphorus in subsequent crops by changing the unavailable natural and residual phosphorus to more available forms. Furthermore, legume green manure residue decomposition can produce bicarbonates (H2CO3), which can solubilize soil mineral phosphorus and, accordingly, result in higher phosphorus availabilities to plants (Fageria, 2007). The contents of different nutrients and C:N ratios found in the above-ground portions of various important legume green manure crops are shown in Table 5.
Table 5. The contents of nutrient and C:N ratios of above-ground portions of some important green manure crops (Source: Ghosh et al., 2007).
Alley cropping is a cropping practice in which cultivated crops are planted in alleys designed by trees or shrubs, well-known primarily to restore soil fertility and enhance productivity. It is the simultaneous growing of crops between hedgerows of trees that are nitrogen-fixing trees. Alley cropping with nitrogen-fixing trees appears to have a greater impact on long-term nutrient acquisition from deep soil strata. Besides this, the alley cropping approach guarantees the utilization of leaf manures as the trees/shrubs are trimmed during the cropping season (Ghosh et al., 2007). Green leaf manures falling from nitrogen-fixing trees/shrubs supplement the efficacy of applied fertilizers, sustain the soil health and, thus, improve the crop yields and returns. A report on the decomposition residues in alley cropping systems by Wilson et al. (1986) revealed that 50% of the supplemented legume nitrogen can be available to the crops within 1–9 weeks although it depends on the primary nitrogen contents and predominant ecological conditions.
According to Ghosh et al. (2007), the pruning of Leucaena leucocephala in an alley cropping system provides a considerable quantity of nutrients that aids the companion crop. In addition, the requirement for fertilizers is decreased, and the system offers an alternative system for achieving sustained yield with low agricultural inputs. Ghosh et al. (2007) also showed that there is an average decrease of 38, 34, and 29% in the yield of maize, black gram, and cluster bean, respectively, as compared with pure crops when grown as an intercrop with Leucaena leucocephala. The authors indicated that the highest earnings can be achieved when Leucaena is grown in alley cropping with cluster bean and black gram than growing of sole maize or Leucaena. Kang and Shannon (2001) also indicated that nitrogen yields are typically around 200–300 kg ha−1 in alley cropping of which ~50% of the nitrogen is derived from the atmosphere (Giller, 2001). The continued presence of alley cropping, which utilizes nitrogen-fixing legume trees, can, thus, act as a way of utilizing the BNF system. The influence of sole cropping, alley cropping systems, green leaf manures, and nitrogen levels on seed yields of castor is shown in Table 6.
Table 6. Seed yields (kg ha−1) of castor as influenced by sole cropping, alley cropping, green leaf manures, and nitrogen levels.
The potential of nitrogen fixation in every legume system is dependent on the plant's reliance upon nitrogen fixation for growth and development and plant nitrogen yield. Improving the BMF for higher nitrogen productivity and gain will increase the sustainability of agricultural production systems. Improving the contribution of BNF by legumes to agricultural systems@ is currently central to different strategies to mitigate the environmental impacts of agriculture as it contributes to recoupling the carbon and nitrogen cycles in agro-ecosystems and enhancing soil nutrient pools while increasing nutrient use efficiency at the levels of diverse cropping systems (Louarn et al., 2015). Various strategies are used to improve the process in an existing symbiotic relationship and widen the scope to non-symbiotic commercial crops, and the success of these strategies depends on how well the process of BNF is understood (Goyal et al., 2021). As a whole, an increment in the level of nitrogen fixed could be attained by exploiting the legumes yield within the constraints imposed by agronomic, nutritional, or ecological factors. In addition, several management practices influence BNF in agricultural production systems. The strategies widely recognized and adopted for improving BNF of legumes and their associated contributions in the agricultural systems include the selection of legume genotypes, inoculation of legumes with effective rhizobia and their improvements, and the use of appropriate agronomic practices and cropping systems.
Enhancement in agricultural sustainability needs the utilization of BNF as a key source of nutrients for plant growth and development. Mixed cropping and crop rotations of a non-legumes with legumes have been utilized for centuries to exploit BNF from legumes. The selection of appropriate legume hosts plays a dominant role in interaction with rhizobia (Liu et al., 2020; Plett et al., 2021). The selection of improved host legumes must be developed if higher levels of production and sustainability are to be achieved. Best production from any environment can only be achieved by the use of the most appropriate biological material most matched for a particular environment and adopting management practices intended to avoid or minimize the particular ecological stress most likely to affect the symbiosis. According to Plett et al. (2021), understanding how a genotype supports nodulation and N-fixation in legumes is important to maximize the benefit of N-fixation and reduce reliance on nitrogenous fertilizers. Vanlauwe et al. (2019) stated that the primary methods to improve the potential of an effective BNF are (i) breeding of legume genotypes with increased BNF efficiency with elite strains; (ii) choosing of grain legumes (legume genotypes) that are satisfactorily promiscuous to nodulate successfully with the native rhizobia existing in the soils; and (iii) inoculating of legumes with effective and superior rhizobia strains.
Legume–rhizobial symbiosis is a highly specific interaction, such that particular legume genotypes form an efficient symbiosis with only a specific set of rhizobial strains (Liu et al., 2020). However, the importance of the legume genotypes in regulating nitrogen fixation has commonly been given negligible attention. Sinclair and Nogueira (2018) indicated that breeding programs ignore plant traits that might be related to improved nitrogen fixation potentials due to the constraints in tracking nodulation capacity and nitrogen fixation activity. In more recent research on legumes N2 fixation, it is increasingly becoming clear that the host plant has a leading role in influencing N2 fixation. The selection of legume genotypes now appears to be necessary to improve N2 fixation potential and to have better growth and physiological capability, which can provide better nitrogen input to the plant. Therefore, host plant breeding is compulsory to increase BNF, particularly if inoculation with elite rhizobia strains is anticipated to improve crops yield. Efforts toward announcing BNF as a key property to be considered in plant breeding programs could have thoughtful influences on symbiotic potentials (Vanlauwe et al., 2019).
On the other hand, various stages of the plant–bacteria interaction could also be optimized to maximize the amount and benefit of nitrogen fixation. Incompatibility occurring among host legumes and nodulating rhizobia at the initial stages of the interaction can block bacterial infection and nodule organogenesis, whereas incompatibility happening after nodule development can result in the development of infected nodules incapable of fixing nitrogen (Yang et al., 2017; Wang et al., 2018). Hence, it can be noted that nitrogen fixation is dependent on the capability of legumes to form an association with rhizobia in a given soil and the host's ability to selectively interact with the most mutualistic partners from a group of compatible indigenous strains. Therefore, it is important to select appropriate legumes and improve their ability to choose and cooperate with the best mutualistic rhizobial symbionts. For this purpose, Liu et al. (2020) suggested that genetic and genomic methods should be employed to identify genes and alleles through harnessing abundant genetic and phenotypic variation present in the legume varieties. According to Goyal et al. (2021), the chemical communication between the legume host and bacteria can be improved to enhance the number of nodules formed and favor occupation by desirable strains. Flavonoids produced by the plant are the earliest signals that promote the symbiotic interaction and induce the genes (nod genes) that are responsible for the synthesis of nod factors. Nod genes are lipochitooligosaccharides that allow the legume to initiate nodule formation, and hence, manipulation of these chemicals, particularly for their expression under stress and suboptimal environmental conditions can substantially improve BNF. Prithiviraj et al. (2003) indicated that increasing the availability of nod factors at low soil temperatures can improve nodulation and BNF. Modulation of stress response genes, phytohormone biosynthesis, phosphate solubilization, and antibiotic production in host plants are also important techniques for improving the symbiotic interaction and the effectiveness of BNF. Kebede (2021) also delineated that rhizosphere engineering can be an alternative approach through which plants are genetically modified to discharge compounds that boost the association and proliferation of beneficial microorganisms.
The most antique microbe used as inoculants is “rhizobia” bacteria that can colonize the rhizosphere and establish a symbiotic association with legumes, which are used as a plant growth promoter and protector through BNF, mobilization and solubilization of nutrients, production of siderophores, and discharge of phytohormones (Kebede, 2021). According to Murphy-Bokern et al. (2017), inoculation of the legume with the appropriate strain of rhizobia is necessary production technology if it is to be grown where it or a related species has not been produced within the previous 5 years, and this inoculation often results in improved BNF and, hence, soil fertility and crop yields. Hence, legume inoculation with rhizobia is a way of ensuring that the strain of rhizobia appropriate for the legume cultivar being grown exists in the soil at the proper period and in numbers adequate to make a rapid and effective infection and succeeding nitrogen fixation. The inventive objective of this practice is to stimulate BNF to offer nitrogenous nutrients to a particular legume and other crops, and its return is an increment in plant growth, nutrient uptake, yield, seed protein, and other traits and a reduction in the use of chemical fertilizers with the subsequent decrease of ecological contamination (Wolde-meskel et al., 2018; Kebede, 2021).
Inoculation of legumes with rhizobia can be beneficial in providing a sufficient number of viable N-fixing rhizobia to offer early and effective symbiosis in legumes in the field. Moreover, inoculating the appropriate rhizobia results in the early formation of effective nodules for efficient nitrogen fixation. The utilization of rhizobial inoculants has also permitted the effective introduction of legumes to new agricultural systems in which compatible rhizobia were absent from the soils. A better knowledge of the questions of when, where, and how many inoculants to apply to the soil is the main factor to ensure effective inoculation and colonization of roots in competition with soil rhizobia (Wani et al., 1995). Vanlauwe et al. (2019) suggested future research consideration of an understanding of factors that regulate the persistence of inoculated rhizobia, and this may vary broadly among various rhizobial species and strains. Therefore, the use of appropriate inoculants in legumes offers an opportunity for improving the productivity of legumes and other crops grown in integrated cropping systems, such as crop rotation, intercropping, alley cropping, and green manuring. As a result, the phenomenon of rhizobial inoculation has gotten consideration due to its increasing contribution to agricultural productivity. Further, using rhizobia strains that nodulate well and are persistent in the soils when their host legumes are not cropped will be beneficial for farmers. Besides this, the necessity to inoculate should be well-understood along with the most suitable and effective rhizobium supply systems and improved supply chain conditions.
For effective nodulation and subsequent improvement in BNF, it is also imperative to improve the rhizosphere competence and survival of rhizobial inoculants and enhance their adaptation to diverse environments, which can be advantageous in producing a robust approach for usage by farmers (Kebede, 2021). It is reported that legume inoculation with effective rhizobia offers stimulation and accumulation of phenolic compounds, such as isoflavonoid phytoalexins, and triggering of enzymes such as L-phenylalanine ammonia-lyase (PAL), chalcone synthase (CHS), peroxidase (POX), and polyphenol oxidase (PPO), which are involved in phenylpropanoid and isoflavonoid pathways, a rich source of metabolites in plants (Das et al., 2017; Kebede, 2021). Furthermore, Das et al. (2017) recommended novel rhizobial formulation technologies, including polymer-based formulations, water-in-oil emulsion technology for producing liquid formulations, biofilm-based formulations, and application of nanotechnology for the manufacture of an effective inoculant that can ensure enhanced stability, survival, and competence as biofertilizers and biocontrol agents under adverse ecological conditions.
Symbiotic nitrogen fixation in rhizobia is mostly controlled by the nod, nif , and fix genes. Goyal et al. (2021) revealed that rhizobial strains could be improved at any stage beginning with their perception of flavonoids to initiate the nodulation process through all the steps up to the delivery of fixed nitrogen to the host plant. Consequently, molecular studies and improvements and in-depth knowledge of factors responsible for rhizobial survival and persistence can play a vital role in improving the underlying mechanism of communication, occupation in the host, and nitrogen-fixing ability. For instance, Goyal et al. (2021) described that nitrogen reduction by overexpression of genes from nif and fix groups and modulation of stress response genes, phytohormone biosynthesis, phosphate solubilization, and antibiotic production are among technologies that are being explored to enhance rhizobial effectiveness. Tremendous efforts over a long period that have resulted in significant progress in different molecular modifications and improvements in rhizobial strains and their effect on host-bacteria symbiotic processes are presented in Table 7.
Table 7. Summarized molecular modifications of rhizobial strains and explored improvements in survival and nitrogen fixation characteristics.
Overall, the use of rhizobial inoculants is a common agronomic practice to ensure adequate nitrogen availability as ~80% of biologically fixed N comes from this symbiosis, having the potential to fix up to 300 kg N/ha/year in different legume crops (Nosheen et al., 2021). In a mixed cropping system, these species improve the growth of nonlegumes by inducing changes in root morphology and growth physiology. One important approach to ensure the success of these species nowadays is to use their mixtures, which increase the competency of each component under field conditions. According to Vassilev et al. (2015), the interest in using mixed inoculants is based on the fact that species of microorganisms in the soil normally exist in communities in which they release diverse metabolites related to their association with plants as well as other microorganisms involved in naturally existing processes of defense and/or competition for space and nutrients. In this regard, Kebede (2021) delineated that the mixture of rhizobial inoculants allows persistence in the rhizosphere for an extended period even during the nonexistence of host legumes and exploitation of broader mechanisms of action that improve their efficiency and reliability. For instance, Kebede (2021) indicated that the co-inoculated mixture of rhizobia can produce signaling molecules, such as nodulation factors (nod factors) and polysaccharides that can stimulate root nodulation and improve the efficiency of biological nitrogen fixation. Hence, the use of mixtures of inoculants, especially various inoculants with diverse proven benefits to the plants, such as nitrogen fixing, phosphorus solubilizing, potassium mobilizing, and biocontrol of diseases, is essential.
Understanding the key agronomic constraints influencing legume yields is a prerequisite for improving the BNF in legumes. Stagnari et al. (2017) indicated that the magnitude of BNF and associated plant nutrient contributions varies across legume species, soil properties, climatic conditions, and cropping systems (i.e., monoculture, mixed culture, crop rotations, etc.) as well as soil management strategies. Inorganic nitrogen sources are reported to decrease the nodulation of legumes and reduce nitrogen fixation. The degree of inhibition achieved is reliant on numerous factors comprising the concentration and form, the time of application, and also the host plant and bacterial strain. Sometimes a small amount of inorganic N, which is known to be starter nitrogen, stimulates early seedling growth and nodulation, leading to an increment in the quantity of nitrogen fixed per plant. In such cases, the nitrogen overcomes the period of nitrogen stress, which the legumes may experience before the commencement of vigorous N fixation. According to George and Singleton (1992), the amount of N gained from fixation is influenced strongly by the N level in the soil as well as nodule weight, nodule number, nodule size, and the amount of N fixed being inversely related to the increase in fertilizer N.
Phosphorus is also critical for both establishments of nodulation and N2 fixation. Poor nodulation and poor plant vigor are observed in legumes grown in soils low in extractable phosphorus (Desta et al., 2015; Wolde-meskel et al., 2018). Uchida (2000) revealed that phosphorus is vital in several key plant functions, including photosynthesis, transfer of energy, conversion of sugars and starches, movement of nutrients within the plant, and transmission of genetic traits from one generation to the next. Rhizobia utilize phosphorus as a vital element in transforming atmospheric N2 to nitrate or ammonium (NH4), a form that is uptaken by plants (Dakora and Keya, 1997). Thus, nodulation, N fixation, and detailed nodule activity are directly correlated to the phosphorus supply in the soil. Under phosphorus stress, strains of rhizobia vary in their capability to extract and incorporate phosphorus from the external environment (Ahiabor et al., 2014; Desta et al., 2015; Wolde-meskel et al., 2018). Phosphorus is, thus, the basis for effective legume nodulation and efficiency of symbiotic nitrogen fixation. Under adequate conditions of P, potassium stimulates infection and N fixation, but high levels of K are inhibiting if P levels are low. Potassium is also well-known for stimulating nodule activity by improving carbohydrate supplies. Similarly, S deficiency affects nodulation by reducing nodule number, size, and N fixation. It is revealed that sulfur deficiency causes a failure of protein synthesis whether nitrogen is available to the plant symbiotically or in a combined form. Some disorders due to deficiency of trace elements are shown to affect the growth of legumes, including Ca, Mg, Mn, Al, etc. (Campo, 1995). Therefore, nutrient management is essential to maximize BNF and obtain the potential benefit from this system.
Crop agronomic practices, such as the frequency of tillage and cropping system practices, can change edaphic, chemical, and biophysical factors and also indirectly affect BNF. Therefore, agronomic management practices that enhance BNF are a promising avenue to increase yields of legumes and other crops in different cropping systems (Montañez, 2000). The potential of BNF and the amount of soil nutrients accessible to a non-legume crop can also be affected by soil and crop management and cropping systems. Legumes grown directly after a cereal crop can fix significantly higher nitrogen than when grown in formerly fallowed soil. With an appropriate selection of cropping systems and consideration of nutritional aspects, BNF can be managed for enhancing N2 fixation. In areas in which water logging is a problem, the use of different drainage mechanisms, such as broad bed furrows, cumber plots, and furrows, improve the stress imposed by excess water on the growth of crops (Bergersen et al., 1989). Besides this, the most important agronomic practices for enhancing BNF are using improved pest management practices, enhancing soil structures, transforming from conventional tillage to minimal or zero tillage, enhancing the general fertility status of the soils, and maintaining the levels of existing soil nutrients (Montañez, 2000).
Legumes are essential sources of foods and feed proteins and are often integrated into agricultural systems for their beneficial effects on soil fertility through BNF, which makes a substantial contribution in cropping systems. Direct nitrogen transfer, decomposition, and mineralization of legume residues and mineral nutrient availability and uptake enhancement by crops are among the contribution of BNF in the cropping systems. To effectively utilize these returns in production systems, integrated cropping systems, such as crop rotation, intercropping, improved fallows, green manuring, and alley cropping, are commonly used. An increase in the amount and utilization of the potential benefits in different cropping systems could be realized by exploiting legumes yield within the limitations imposed by different agronomic, nutritional, and ecological factors. Particularly, the BNF and its associated benefit in the agricultural system can be improved by selecting legume genotypes, inoculating with effective rhizobia, and using good crop and soil management practices. Generally, the use of legumes in the cropping system and adoption of BNF technology, such as an expansion of knowledge and development of economic applications and management systems should be given priority. Future research should engage different approaches to improving inoculant quality with special emphasis on the development of rhizobial strains, inoculant production and application methods, and utilization of legumes in different cropping systems.
The author confirms being the sole contributor to this work and has approved it for publication.
The author declares that the research was conducted in the absence of any commercial or financial relationships that could be construed as a potential conflict of interest.
All claims expressed in this article are solely those of the authors and do not necessarily represent those of their affiliated organizations, or those of the publisher, the editors and the reviewers. Any product that may be evaluated in this article, or claim that may be made by its manufacturer, is not guaranteed or endorsed by the publisher.
Adeleke, M. A., and Haruna, I. M. (2012). Residual nitrogen contributions from grain legumes to the growth and development of succeeding maize crop. Int. Scholarly Res. Network 2021:213729. doi: 10.5402/2012/213729
Ae, N. B., and Shen, R. F. (2002). Root cell-wall properties are proposed to contribute to phosphorus (P) mobilization by groundnut and pigeon pea. Plant. Soil 245, 95–103. doi: 10.1023/A:1020669326602
Ahiabor, B., Lamptey, S., Yeboah, S., and Bahari, V. (2014). Application of phosphorus fertilizer on soybean [(Glycine max L. (Merril)] inoculated with rhizobium and its economic implication to farmers. Am. J. Exp. Agric. 11:1420. doi: 10.9734/AJEA/2014/10400
Bergersen, F. J., Brockwell, J., Gault, R. R., Morthorpe, L., People, M. B., and Turner, G. L. (1989). Effects of the available soil nitrogen and rates of inoculation on nitrogen fixation by irrigated soybean and evaluation of 15N methods for measurement. Aus. J. Agric. Res. 40, 763–780. doi: 10.1071/AR9890763
Beri, V., Meelu, O. P., and Khind, C. S. (1989). Biomass production, N accumulation, symbiotic effectiveness and mineralization of green manures in relation to yield of wetland rice. Trop. Agric. 66, 11–16.
Bhowmik, N. S., and Das, A. (2018). “Biofertilizers: a sustainable approach for pulse production,” in Legumes for Soil Health and Sustainable Management, eds R., Meena, A., Das, G., Yadav, R., Lal eds (Berlin/Heidelberg: Springer), 445–485. doi: 10.1007/978-981-13-0253-4_14
Buresh, R. J., and De Datta, S. K. (1991). Nitrogen dynamics and management in rice-legume cropping systems. Adv. Agron. 45, 1–59. doi: 10.1016/S0065-2113(08)60037-1
Campo, R. J. (1995). Residual Effects of Aluminium on Bradyrhizobium japonicum in Defined Medium and Soil Solution. Ph. D. Thesis. The University of Reading, Reading.
Dakora, F. D., and Keya, S. O. (1997). Contribution of legume nitrogen fixation to sustainable agriculture in sub-Saharan African. Soil Biol. Biochem. 29, 809–817. doi: 10.1016/S0038-0717(96)00225-8
Das, K., Prasanna, R., and Saxena, A. K. (2017). Rhizobia: a potential biocontrol agent for soilborne fungal pathogens. Folia Microbiol. 62, 425–435. doi: 10.1007/s12223-017-0513-z
Desta, Y., Habtegebrial, K., and Woldu, Y. (2015). Inoculation, Phosphorus and Zinc fertilization effect on nodulation, yield and nutrient uptake of Faba bean (Vicia faba L) grown on calcaric Cambisol of Semi-Arid Ethiopia. J. Soil Sci. Environ. Manage. 6, 9–15. doi: 10.5897/JSSEM2013.0406
Dubach, M., and Russelle, M. P. (1994). Forage legume roots and nodules and their role in nitrogen transfer. Agron. J. 86, 259–266. doi: 10.2134/agronj1994.00021962008600020010x
Eaglesham, A. R. J., Ayanaba, A., Ranga Rao, V., and Eskew, D. L. (1981). Improving the nitrogen nutrition of maize by intercropping with cowpea. Soil Biol. Biochem. 13, 169–171. doi: 10.1016/0038-0717(81)90014-6
Fageria, N. K. (2007). Green manuring in crop production. J. Plant Nutr. 30, 691–719. doi: 10.1080/01904160701289529
Fujita, K., Ofosu-Budu, K. G., and Ogata, S. (1992). Biological nitrogen fixation in mixed legume-cereal cropping systems. Plant Soil 141, 155–176. doi: 10.1007/BF00011315
Fustec, J., Lesuffleur, F., Mahieu, S., and Cliquet, J. B. (2010). Nitrogen rhizodeposition of legumes. a review. Agron. Sustain. Dev. 30, 57–66. doi: 10.1051/agro/2009003
Gangwar, K. S., Sharma, S. K., and Tomar, O. K. (2004). Alley cropping of subabul (Leucaena leucocephala) for sustaining higher crop productivity and soil fertility of rice (Oryza sativa)-wheat (Triticum aestivum) system in semi-arid conditions. Indian J. Agron. 49, 84–88.
George, T., and Singleton, P. W. (1992). Nitrogen assimilation traits and dinitrogen fixation in soybean and common bean. Agron. J. 84, 1020–1028. doi: 10.2134/agronj1992.00021962008400060022x
Ghosh, P. K., Bandyopadhyay, K. K., Wanjari, R. H., Manna, M. C., Misra, A. K., Mohanty, M., et al. (2007). Legume effect for enhancing productivity and nutrient use-efficiency in major cropping systems–an Indian perspective: a review. J. Sustain. Agric. 30, 59–86. doi: 10.1300/J064v30n01_07
Giller, K. E. (2001). Nitrogen Fixation in Tropical Cropping Systems. 2nd edn. Wallingford: CAB International. doi: 10.1079/9780851994178.0000
Goyal, R. K., Schmidt, M. A., and Hynes, M. F. (2021). Molecular biology in the improvement of biological nitrogen fixation by rhizobia and extending the scope to cereals. Microorganisms 9:125. doi: 10.3390/microorganisms9010125
Islam, M. A., and Adjesiwor, A. T. (2017). Nitrogen Fixation and Transfer in Agricultural Production Systems. Nitrogen in Agriculture-Updates, Amanullah and Shah Fahad. IntechOpen. doi: 10.5772/intechopen.71766
Jensen, E. S. (1996). Rhizodeposition of N by pea and barley and its effect on soil N dynamics. Soil Biol. Biochem. 28, 65–71. doi: 10.1016/0038-0717(95)00116-6
Kang, B. T., and Shannon, D. A. (2001). Agroforestry with focus on alley cropping. sustaining soil fertility in West Africa. Soil Sci. Soc. Am. 58, 211–224. doi: 10.2136/sssaspecpub58.ch10
Kebede, E. (2020a). Grain legumes production and productivity in Ethiopian smallholder agricultural system, contribution to livelihoods and the way forward. Cogent Food Agric. 6:1722353. doi: 10.1080/23311932.2020.1722353
Kebede, E. (2020b). Grain legumes production in Ethiopia: a review of adoption, opportunities, constraints and emphases for future interventions. Turkish J. Agricult. Food Sci. Technol. 8, 977–989. doi: 10.24925/turjaf.v8i4.977-989.3254
Kebede, E. (2021). Competency of rhizobial inoculation in sustainable agricultural production and biocontrol of plant diseases. Front. Sustain. Food Syst. 5:728014. doi: 10.3389/fsufs.2021.728014
Lemage, B., and Tsegaye, M. (2020). Evaluation of legume shrubs improved fallow for abandoned agricultural land rehabilitation. Int. J. Agril. Res. Innov. Tech. 10, 64–70. doi: 10.3329/ijarit.v10i1.48095
Lengwati, D. M., Mathews, C., and Dakora, F. D. (2020). Rotation benefits from N2-fixing grain legumes to cereals: from increases in seed yield and quality to greater household cash-income by a following maize crop. Front. Sustain. Food Syst. 4:94. doi: 10.3389/fsufs.2020.00094
Liu, J., Yu, X., Qin, Q., Dinkins, R. D., and Zhu, H. (2020). The impacts of domestication and breeding on nitrogen fixation symbiosis in legumes. Front. Genet. 11:973. doi: 10.3389/fgene.2020.00973
Louarn, G., Pereira-Lopès, E., Fustec, J., Mary, B., Voisin, A. S., de Faccio Carvalho, P. C., et al. (2015). The amounts and dynamics of nitrogen transfer to grasses differ in alfalfa and white clover-based grass-legume mixtures as a result of rooting strategies and rhizodeposit quality. Plant Soil 389, 289–305. doi: 10.1007/s11104-014-2354-8
Makoi, J. H., Bambara, S., and Ndakidemi, P. A. (2013). Rhizobium inoculation and the supply of Molybdenum and lime affect the uptake of macro elements in common bean (P. vulgaris L.) plants. Aust. J. of Crop Sci. 7, 784–793.
Mandimba, G. R. (1995). Contribution of nodulated legumes on the growth of Zea mays L. under various cropping systems. Symbiosis 19, 213–222.
Matse, D. T., Huang, C., Huang, Y., and Yen, M. (2020). Effects of coinoculation of Rhizobium with plant growth promoting rhizobacteria on the nitrogen fixation and nutrient uptake of Trifolium repens in low phosphorus soil. J. Plant Nutr. 43, 739–752. doi: 10.1080/01904167.2019.1702205
Mayer, J., Buegger, F., Jensen, E. S., Schloter, M., and Hess, J. (2003). Estimating N rhizodeposition of grain legumes using a 15N in situ stem labeling method. Soil Biol. Biochem. 35, 21–28. doi: 10.1016/S0038-0717(02)00212-2
Meena, B. P., Fagodiya, R. K., Prajapat, K., Dotaniya, M., Kaledhonkar, M., Sharma, P., et al. (2018). “Legume green manuring: an option for soil sustainability,” in Legumes for Soil Health and Sustainable Management, eds R. Meena, A. Das, G. Yadav, R. Lal (Singapore: Springer), 387–408. doi: 10.1007/978-981-13-0253-4_12
Mmbaga, G. W., Mtei, K. M., and Ndakidemi, P. A. (2014). Extrapolations on the use of rhizobium inoculants supplemented with phosphorus (P) and potassium (K) on growth and nutrition of legumes. Agric. Sci. 5, 1207–1226. doi: 10.4236/as.2014.512130
Montañez, A. (2000). Overview and Case Studies on Biological Nitrogen Fixation. Perspectives and Limitations. FAO, Science in Agriculture, 1–11.
Murphy-Bokern, D., Stoddard, F. L., and Watson, C. A. (2017). Legumes in Cropping Systems. CABI. doi: 10.1079/9781780644981.0000
Nair, P. K. R., Buresh, R. J., Mugendi, D. N., and Latt, C. R. (1999). “Nutrient cycling in tropical agroforestry systems: myths and science,” in Agroforestry in Sustainable Agricultural Systems, eds L. E. Buck et al. (Boca Raton, FL: CRC Press), 1–31.
Nanganoa, L. T., Njukeng, J. N., Ngosong, C., Atache, S. K. E., Yinda, G. S., Ebonlo, J. N., et al. (2019). Short-term benefits of grain legume fallow systems on soil fertility and farmers livelihood in the humid forest zone of cameroon. Int. J. Sustain. Agric. Res. 6, 213–223. doi: 10.18488/journal.70.2019.64.213.223
Ndakidemi, P. A., Dakora, F. D., Nkonya, E. M., Ringo, D., and Mansoor, H. (2006). Yield and economic benefits of common bean (Phaseolus vulgaris) and soybean (Glycine max) inoculation in northern Tanzania. Aust. J. Exp. Agric. 46, 571–577. doi: 10.1071/EA03157
Nosheen, S., Ajmal, I., and Song, Y. (2021). Microbes as biofertilizers, a potential approach for sustainable crop production. Sustainability 13:1868. doi: 10.3390/su13041868
Nyoki, D., and Ndakidemi, P. A. (2014). Effects of Bradyrhizobium japonicum inoculation and supplementation with phosphorus on macronutrients uptake in cowpea (Vigna unguiculata L. Walp). Am. J. Plant Sci. 5, 442–451. doi: 10.4236/ajps.2014.54058
Osunde, A. O., Tsado, P. A., Bala, A., and Sanginga, N. (2004). Productivity of a maize promiscuous soybean intercrops as affected by fertilizer in the southern guinea savanna zone of Nigeria. West Afr. J. Appl. Ecol. 5, 1–12. doi: 10.4314/wajae.v5i1.45598
Peoples, M. B., Brockwell, J., Herridge, D. F., Rochester, I. J., Alves, B. I. R., Urquiaga, S., et al. (2009). The contributions of nitrogen-fixing crop legumes to the productivity of agricultural systems. Symbiosis 48, 1–17. doi: 10.1007/BF03179980
Peoples, M. B., and Craswell, E. T. (1992). Biological nitrogen fixation: investments, expectations and actual contributions to agriculture. Plant Soil 141, 13–39. doi: 10.1007/BF00011308
Peoples, M. B., and Herridge, D. F. (1990). Nitrogen fixation by legumes in tropical and subtropical agriculture. Adv. Agron. 44, 155–223. doi: 10.1016/S0065-2113(08)60822-6
Peoples, M. B., Herridge, D. F., and Ladha, J. K. (1995). Biological nitrogen fixation: an efficient source of nitrogen for sustainable agricultural production. Plant Soil 174, 3–28. doi: 10.1007/BF00032239
Plett, K. L., Bithell, S. L., Dando, A., and Plett, J. M. (2021). Chickpea shows genotype-specific nodulation responses across soil nitrogen environment and root disease resistance categories. BMC Plant Biol. 21:310. doi: 10.1186/s12870-021-03102-6
Prithiviraj, B., Zhou, X., Souleimanov, A., Khan, W. M., and Smith, D. L. (2003). A host-specific bacteria-to-plant signal molecule (Nod factor) enhances germination and early growth of diverse crop plants. Planta 216, 437–445. doi: 10.1007/s00425-002-0928-9
Sanchez, P. A. (1999). Improved fallows come of age in the tropics. Agroforestry Syst. 47, 3–12. doi: 10.1023/A:1006287702265
Sanginga, N., Dashiell, K., Diels, J., Vanlauwe, B., Lyasse, O., Carsky, R. J., et al. (2003). Sustainable resource management coupled to resilient germplasm to provide new intensive cereal-grain legume livestock systems in the dry savanna. Agric. Ecosyst. Environ. 100, 305–314. doi: 10.1016/S0167-8809(03)00188-9
Shah, A., Nazari, M., Antar, M., Msimbira, L. A., Naamala, J., Lyu, D., et al. (2021). PGPR in agriculture: a sustainable approach to increasing climate change resilience. Front. Sustain. Food Syst. 5:667546. doi: 10.3389/fsufs.2021.667546
Sinclair, T. R., and Nogueira, M. A. (2018). Selection of host-plant genotype: the next step to increase grain legume N2 fixation activity. J. Exp. Bot. 69, 523–3530. doi: 10.1093/jxb/ery115
Smaling, E. (1993). “Soil nutrient depletion in Sub-Saharan Africa,” in The Role of Plant Nutrients for Sustainable Food Crop Production in Sub Saharan Africa, eds H. Van Reuler, and W. Prins (Leidschendan: VKP (Dutch Association of Fertilizer Producers), 47–61.
Stagnari, F., Maggio, A., Galieni, A., and Pisante, M. (2017). Multiple benefits of legumes for agriculture sustainability: an overview. Chem. Biol. Technol. Agric. 4, 1–13. doi: 10.1186/s40538-016-0085-1
Thilakarathna, M. S., McElroy, M. S., Chapagain, T., Papadopoulos, Y. A., and Raizada, M. N. (2016). Belowground nitrogen transfer from legumes to non-legumes under managed herbaceous cropping systems. A review. Agron. Sustain. Dev. 368. doi: 10.1007/s13593-016-0403-9
Uchida, R. (2000). “Essential nutrients for plant growth: nutrient functions and deficiency symptoms,” Plant Nutrient Management in Hawaii's Soils (Manoa: College of Tropical Agriculture and Human Resources, University of Hawaii), 31–55.
Vanlauwe, B., Hungria, M., Kanampiu, F., and Giller, K. E. (2019). The role of legumes in the sustainable intensification of African smallholder agriculture: lessons learnt and challenges for the future. Agric. Ecosyst. Environ. 284:28406583. doi: 10.1016/j.agee.2019.106583
Vasconcelos, M. W., Grusak, M. A., Pinto, E., Gomes, A., Ferreira, H., Balázs, B., et al. (2020). “The biology of legumes and their agronomic, economic, and social impact,” in The Plant Family Fabaceae (Singapore: Springer), 3–25. doi: 10.1007/978-981-15-4752-2_1
Vassilev, N., Vassileva, M., Lopez, A., Martos, V., Reyes, A., Maksimovic, I., et al. (2015). Unexploited potential of some biotechnological techniques for biofertilizer production and formulation. Appl. Microbiol. Biotechnol. 99, 4983–4996. doi: 10.1007/s00253-015-6656-4
Walley, F. L., Tomm, G. O., Matus, A., Slinkard, A. E., and van Kessel, C. (1996). Allocation and cycling of nitrogen in an alfalfa bromegrass sward. Agron. J. 88, 834–843. doi: 10.2134/agronj1996.00021962008800050025x
Wang, Q., Liu, J., and Zhu, H. (2018). Genetic and molecular mechanisms underlying symbiotic specificity in legume-rhizobium interactions. Front. Plant Sci. 9:313. doi: 10.3389/fpls.2018.00313
Wani, S. P., Repula, O. P., and Lee, K. K. (1995). Sustainable agriculture in the semi-arid tropics through biological nitrogen fixation in grain legumes. Plant Soil 174, 42–45. doi: 10.1007/BF00032240
Wilson, G. F., Kang, B. T., and Mulongoy, K. (1986). Alley cropping: Trees as sources of green-manure and mulch in the tropics. Biological Agric. Hortic. 3, 251–267. doi: 10.1080/01448765.1986.9754474
Wolde-meskel, E., van Heerwaarden, J., Abdulkadir, B., Kassa, S., Aliyi, I., Degefu, T., et al. (2018). Additive yield response of chickpea (Cicer arietinum L.) to rhizobium inoculation and phosphorus fertilizer across smallholder farms in Ethiopia. Agric. Ecosyst. Environ. 261, 144–152. doi: 10.1016/j.agee.2018.01.035
Wolfe, M. S., and Cormack, W. F. (2002). Companion Cropping for Organic field vegetables (OF0181). Report. Terrington: ADAS. Available online at: http://www2.defra.gov.uk/research/Project_Data/More.asp?I=OF0181 (accessed April 06, 2021).
Yang, S., Wang, Q., Fedorova, E., Liu, J., Qin, Q., Zheng, Q., et al. (2017). Microsymbiont discrimination mediated by a host-secreted peptide in Medicago truncatula. Proc. Natl Acad. Sci. U.S.A. 114, 6848–6853. doi: 10.1073/pnas.1700460114
Yusuf, A. A., Iwuafor, E. N. O., Olufajo, O. O., Abaidoo, R. C., and Sanginga, N. (2009). Effect of crop rotation and nitrogen fertilization on yield and nitrogen efficiency in maize in the northern Guinea Savannah of Nigeria. Afr. J. Agric. Res. 4, 913–921. doi: 10.5897/AJAR.9000199
Keywords: biological nitrogen fixation, cropping system, inoculation, legume residue, nutrient transfer
Citation: Kebede E (2021) Contribution, Utilization, and Improvement of Legumes-Driven Biological Nitrogen Fixation in Agricultural Systems. Front. Sustain. Food Syst. 5:767998. doi: 10.3389/fsufs.2021.767998
Received: 31 August 2021; Accepted: 11 October 2021;
Published: 16 November 2021.
Edited by:
Sudhakar Srivastava, Banaras Hindu University, IndiaReviewed by:
Alice Checcucci, University of Bologna, ItalyCopyright © 2021 Kebede. This is an open-access article distributed under the terms of the Creative Commons Attribution License (CC BY). The use, distribution or reproduction in other forums is permitted, provided the original author(s) and the copyright owner(s) are credited and that the original publication in this journal is cited, in accordance with accepted academic practice. No use, distribution or reproduction is permitted which does not comply with these terms.
*Correspondence: Erana Kebede, ZXJhbmFrLm5lZGFAZ21haWwuY29t; ZXJhbmEua2ViZWRlQGhhcmFtYXlhLmVkdS5ldA== orcid.org/0000-0002-3584-6757
Disclaimer: All claims expressed in this article are solely those of the authors and do not necessarily represent those of their affiliated organizations, or those of the publisher, the editors and the reviewers. Any product that may be evaluated in this article or claim that may be made by its manufacturer is not guaranteed or endorsed by the publisher.
Research integrity at Frontiers
Learn more about the work of our research integrity team to safeguard the quality of each article we publish.