- 1Laboratory of Biochemistry and Molecular Biology, Faculty of Natural Sciences and Mathematics, National University Federico Villarreal, Lima, Peru
- 2Division of Biotechnology, Department of Chemistry, Lund University, Lund, Sweden
Various strategies are being suggested to solve the challenges in the food system, such as changing the source of nutrients, including the use of non-traditional food sources such as insects. Although insects are promoted as a cheap and sustainable source of protein, consumers are reluctant to eat them. The mycoproteins produced by fungi, on the other hand, are very well received and appreciated by consumers. Thus, in this work we have studied the use of the entomopathogenic fungi Beauveria bassiana (Ascomycota: Hypocreales) for the production of protein using insects as feed. B. bassiana was cultivated in culture medium containing entire insects from the species Eurysacca and Hypothenemus or single carbon sources such as glucose or laminarin from Laminaria digitata. The results showed that B. bassiana can produce up to 16-fold more biomass and 8-fold more protein when grown in insect-based medium than when grown in glucose. The results also indicated that the production of proteins continuously increased when B. bassiana was grown in medium containing insects, reaching its maximum at 9 days (up to 3 mg/mL). On the other hand, when cultivated in glucose-supplemented medium, the production of proteins was constantly low (~0.5 mg/mL). In conclusion, B. bassiana was a large biomass producer and exuded a large amount of protein when grown in medium containing insect powder, making it an ideal intermediate link between insects and protein. Furthermore, the proteins produced by fungi such as B. bassiana can be used in the food, health, and cosmetic industries.
Introduction
Food production is one of the largest sources of greenhouse gas (GHG) emissions, emitting ~30% of the world total (Rosenzweig et al., 2020). A study by Clark et al. (2020) showed that even if all non–food GHG emissions were stopped immediately, emissions from the food system alone would likely exceed the 1.5°C emissions limit by 2050. Therefore, it is urgent to reduce GHG emissions in the food system (Clark et al., 2020; Lynch et al., 2021). However, it is equally urgent to ensure food security and nutrition to avoid inadequate access and availability of safe food. In many regions of the world, high food production costs constitute a barrier in choosing foods that meet nutritional requirements. Thus, threatening the health of the most vulnerable groups such as women, children, and the elderly, with the lower-income families choosing foods that do not meet nutritional requirements (Lo et al., 2009; Abdelmenan et al., 2020).
To solve the challenges of the food system there is no single silver bullet, but rather a set of different strategies, such as reducing food waste, increasing the efficiency of food production, and changing eating habits and the source of nutrients (Sovacool et al., 2021). This last strategy includes the use of non-traditional food sources such as insects (Rumpold and Schlüter, 2015), mycoproteins (Hashempour et al., 2020; Derbyshire and Delange, 2021), algae (Cherry et al., 2019), and meat analogs (Devi et al., 2020; Hwang et al., 2020).
Sustainable proteins are receiving increasing attention given their great importance at all development ages, from newborns to the elderly (Semba, 2016; Sato et al., 2020; Cohen et al., 2021). Insects are being promoted as a very rich source of sustainable protein and, although they are common foods in various parts of the world (Jongema, 2017), their introduction to Western cultures is proven very slow (Collins et al., 2019). People in most Western countries view entomophagy with feelings of disgust and most are reluctant to even consider eating insects (Wendin and Nyberg, 2021).
Fungal-derived mycoproteins, on the other hand, are gaining widespread popularity and acceptance due to their healthy nutritional profile, ability to be produced cheaply, environmental benefits, and resistance to landscape constraints such as floods or droughts (Hashempour et al., 2020). A recent systematic review found strong evidence supporting the role of mycoproteins in lowering total cholesterol levels (Ruxton and McMillan, 2010). Mycoproteins also appear to be a promising bioavailable source of essential amino acids that could induce muscle protein synthesis (Derbyshire and Delange, 2021).
The use of entomopathogenic fungi (insect pathogens) as an intermediate link to produce proteins would increase the use of insects. In this way, it would be possible to produce high quality sustainable protein that is widely accepted by consumers. Entomopathogenic fungi exude chitinolytic enzymes, in addition to proteins, to penetrate the outer cuticle of insects (Mondal et al., 2016; Sánchez-Pérez et al., 2016). These chitinases degrade chitin polymers to N-acetylglucosamine units and are used in the food industry for the fermentation of shellfish, crab, and shrimp shells (Le and Yang, 2019). They are also used in the clinical and pharmaceutical industries for the production of single-cell proteins, chithioligosaccharides, N-acetyl D-glucosamines, and antioxidants, all of which have shown improvements in human health thanks to their antitumor, immunomodulatory, antioxidant, and anti-inflammatory properties (Schmacht et al., 2017; Zhang and Feng, 2018).
Beauveria bassiana is an entomopathogenic fungus, used to control crops pests (Wakil et al., 2014, 2021). This fungus has the potential to be included in protein production due to its high accessibility, pan-global distribution, wide range of host insects, and vast ecological niches (Hyde et al., 2019; McGuire and Northfield, 2020). With the aim of producing high quality mycoprotein, we studied the production of protein by B. bassiana cultivated with five different substrates (carbon sources) that included two species of insects: Eurysacca melanocampta (Meyrick, 1917) (Lepidoptera: Gelechiidae) and Hypothenemus hampei (Ferrari) (Coleoptera: Curculionidae, Scolytinae), a filamentous fungus (Fusarium oxysporum f. sp. melongenae), an oomycete (Peronospora variabilis Gäum) isolated from quinoa (Chenopodium quinoa Willd), and saponins extracted from quinoa. These complex carbon sources were compared with three individual controls composed of simple carbon sources: glucose, laminarin, and colloidal chitin to determine the effect of the carbon source on biomass and total protein production.
The total biomass production and the concentration of soluble proteins exuded were determined in relation to the growth rate of B. bassiana to evaluate its potential as a protein source. The proteins and metabolites produced by fungi have potential applications in the food, pharmaceutical, and cosmetic industries. Thus, this study serves as a proof of concept to increase the use of insects, transformed through entomopathogenic fungi, for the production of high quality sustainable protein with a relatively benign environmental footprint at low cost.
Materials and Methods
Beauveria bassiana CCB-LE262 was acquired from the National Agrarian Health Service (SENASA) in Lima, Peru. The stock was cultivated and maintained in an enriched medium containing 4% glucose, 1% peptone, and 1% yeast extract at pH 5.6 (GLP medium) as described by Laynes et al. (2016) and Acuña-Payano et al. (2017).
Complex Substrates Used for Culture Medium
The substrates used in this study were: (1) Eurysacca melanocampta (Meyrick, 1917) powder produced from 500 larvae collected from a four-month-old quinoa (Chenopodium quinoa) field in Juliaca (southern highlands in Peru) grown without agrochemicals; (2) Hypothenemus hampei (Ferrari) powder produced from 1,000 adults collected from a field of Coffea arabica, also in Juliaca; (3) Fusarium oxysporym (Schltdl) fungus isolated from Passiflora ligularis (A. Juss) plants purchased from SENASA; (4) Oomycete Peronospora variabilis (Gaüm) (formerly Peronospora farinosa) obtained from quinoa leaves; (5) Saponins extracted from quinoa plants that were dried at 50°C for 72 h, crushed and subjected to 20% aqueous extraction (W/V) by mechanical stirring with an orbital shaker (Orbital GenieTM-USA) at a speed of 130 rpm for 15 min. The foam was collected and placed in plates for cold dehydration (refrigeration without ice), then the obtained dry matter was weighed and the amount of saponins was quantified following a protocol in which the addition of the Lieberman-Burchard (LB) reagent forms a colorful product proportional to the concentration of saponins. Reagent LB consists of 16.7% acetic anhydride in concentrated sulfuric acid. Oleanolic acid was used as a standard and readings were taken on a Vis/UV spectrophotometer (Shimadzu, UV-1700, Japan) at 528 nm.
Establishment of B. bassiana Cultures Supplemented With Different Substrates
In total 11 liquid culture media were prepared with different substrates combinations that included the five complex substrates (described above) and simple carbon sources (glucose, laminarin, and colloidal chitin). The 11 culture media tested were: (1) Minimum medium (M); (2) M + laminarin 0,1 % w/v (L); (3) M + glucose 0,1 % w/v (G), (4) M + colloidal chitin 1,8% w/v (CQ); (5) M + E. melanocampta powder 0,1% w/v (EM); (6) M + H. hampei 0.1% w/v (HH); (7) M + F. oxyparum 0,1 % w/v (F); (8) M + P. variabilis 0,1 % w/v (PF); (9) M + saponins 0,036 % w/v (S1); (10) M + saponins 0.09% w/v (S2); and (11) M + saponins 0.179 % w/v (S3). The minimum medium M was prepared according to Fernandes et al. (2012). All the culture media were prepared according to Acuña-Payano et al. (2017).
Determination of Biomass
One ml of culture medium samples were taken at 24, 96, and 216 h after inoculation from all treatments in triplicate. They were centrifuged at 10,000 rpm for 15 min, the supernatant was discarded and the pellet was allowed to dry at 40°C for 24 h before being weighted on a precision analytical balance (Ohaus, USA) to determine the dry weight of biomass (dwb).
Total Proteins
One milliliter samples were taken from each culture at 24, 48, 96, 144, 168, and 216 h and centrifuged at 10,000 rpm for 5 min to quantify the total protein concentration (mg/mL) in the supernatant, using a fluorometer (Qubit 2.0, Canada). The assay is highly selective for proteins and is designed to be accurate in the presence of reducing reagents. Common contaminants, such as reducing reagents (DTT, β-mercaptoethanol), salts, free nucleotides, amino acids, solvents, or DNA, but not detergents, are well tolerated in the assay. As a reference, the bovine serum albumin protein (BSA) standards provided by the Kit were used.
Experimental Design
The data obtained in the biomass production and the quantification of soluble proteins exuded in the different culture media were collected in Excel (Microsoft 2010) and for the statistical analysis, the R program was used.
The protein assay was based on a two-way linear additive model (11 treatments = culture media with different substrates, for 6 sampling times), based on the following equation:
and for biomass it was based on a two-way linear additive model (8 treatments = culture media, for 3 sampling times) following the equation:
Where: i = evaluation time (24, 48, 72, 96, 168, or 216 h); j = treatment with inducing media (M, L, G, CQ, EM, HH, F, PF, S1, S2 y S3); k = repetitions; μ = is the effect of the general mean of the response variable; αi = denotes the effect of the i-th time; βj(i) = denotes the effect of the j-th treatment, and ε(ij)k = denotes the effect of the interaction between the i-th time and the j-th treatment, in a design for the variables to be analyzed.
Statistical Analysis
The normal distribution of the data was assessed using the Shapiro-Wilk test (Shapiro and Wilk, 1965). For the homogeneity of variances, the Bartlett test (Arsham and Lovric, 2011) was used. Each of the variables was analyzed by ANOVA. To compare the means of each sample, Tukey's multiple test was used, and the Pearson statistic between variables was used for correlation.
Results
The statistical analysis of the protein and biomass data showed significant differences between the substrates (treatments) and the growth curve (time) of B. bassiana.
Total Biomass
Studies were conducted on the biomass of B. bassiana to understand the basic aspects of its development and its nutritional requirements to grow and spore in insects-based medium. When performing multiple comparison analysis of the variance of means between the treatments and the biomass, at p < 0.01, it was found that EM, F, and HH are associated with the highest biomass production. The CQ medium produced the highest variability while M, G, L, and PF were associated with the lowest biomass production (Figure 1). Furthermore, the distribution and median were found to be higher in EM and F, >7.0 and 4.0 mg/mL, respectively. The lowest biomass production (<1 mg/mL) was obtained with cultures M, G, L, and PF. Looking specifically at the media with complex substrates, twice the biomass was produced in the EM and F media than in the HH and QC media, indicating that the first two media were more complete in nutrients than the last two.
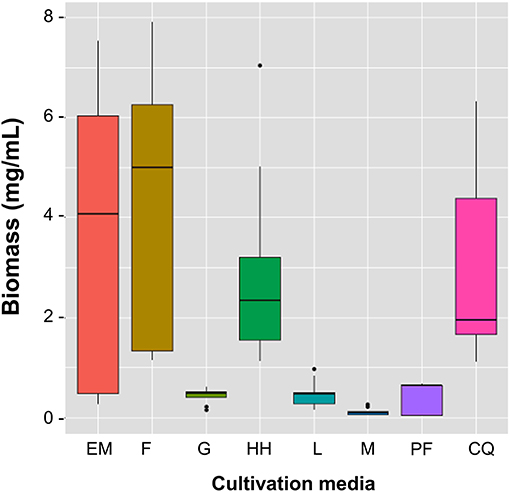
Figure 1. Total biomass of B. bassiana produced in cultures containing Minimal Medium (M), minimal medium with simple carbon substrate (G, glucose; L, laminarin; CQ, colloidal chitin), and minimum medium with complex carbon substrate (EM, E. melanocampta powder; HH, H. hampei powder; F, F. oxyparum powder; PF, P. variabilis powder). The boxes display the central tendency and spread for each treatment. The horizontal line inside each box shows the median for each treatment. EM, F, and HH are associated with the highest biomass production. The CQ medium produces the greatest variability while M, G, L, and PF are associated with the lowest biomass production. The dots outside the boxes represent outliers. p < 0.05, n ≥ 3 independent cultivations.
When it comes to the time when the highest biomass yield occurred, EM and F are associated with a constant increase in yield over time, while HH and CQ yields decrease after 96 h. G, L, and PF show poor yield increase over time, while M shows decreasing yields from the beginning of the cultivation. The EM and F cultures reached the highest dwb at 216 h (9 days) with 7.5 and 7.9 mg/mL, respectively (Figure 2). HH and CQ reached their highest biomass at 96 h (almost 7 mg/mL) but then at 216 h they fell to values below 2 mg/mL. These results show that B. bassiana grows better in media containing complex substrates, such as those based on insects or other fungi, compared to media containing simple carbon sources such as G and L, where only about 0.5 mg/mL dwb were obtained. The biomass obtained in medium with simple carbon sources is not significantly higher than the amount obtained with minimum medium (M) where only 0.5 mg/mL dwb was obtained. In summary, B. bassiana can produce up to 16-fold more biomass when grown in insect-based medium than when grown in glucose. Furthermore, according to the results, when using an insect-based medium, it is better to harvest B. bassiana after seven days of culture to obtain the highest dwb.
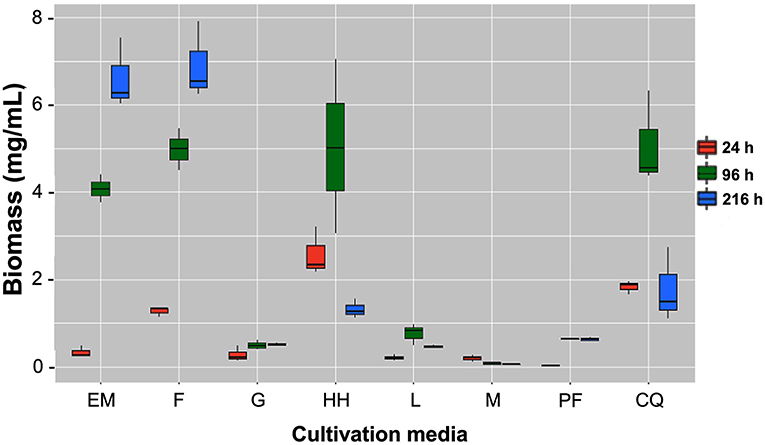
Figure 2. Biomass yield through time. B. bassiana grown in M: minimal medium, minimal medium plus simple carbon substrate (G, glucose; L, laminarin; CQ, colloidal chitin), and minimum medium plus complex carbon substrate (EM, E. melanocampta powder; HH, H. hampei powder; F, F. oxyparum powder; PF, P. variabilis powder). The boxes display the central tendency and spread for each treatment at three different times: 24 h (red), 96 h (green), and 216 h (blue). The horizontal line inside each box shows the median for each treatment. EM and F are associated with constant yield increase through time while HH and CQ yields decreases after 96 h. G, L, and PF display poor yield increase through time with M showing decreasing yields from the beginning of the cultivation. p < 0.05, n ≥ 3 independent cultivations.
Total Proteins
Total proteins are not usually considered an important parameter when studying the pathogenicity of B. bassiana and are only taken into account for the calculation of the specific activity of enzymes (Peteira et al., 2011). However, in this study it is important to determine the amount of protein that B. bassiana excretes when grown in different types of substrate, especially when insects are used as the main source of nutrients. The total proteins obtained with the different media are graphically represented in Figure 3. The results show that a greater quantity of protein was extruded to the medium in those cultures that contained substrates based on insects (EM and HH) (Figure 3A). This high protein productions was maintained throughout the duration of the experiments (9 days) and was up to 3.0 mg/mL higher with respect to substrates that were not based on insects (G, L, M, CQ, S1-S3) (Figure 3B). The total protein concentration in L, G, and M was 0.25, 0.36, and 0.21 mg/mL, respectively. Therefore, B. bassiana can produce about 8-fold more protein when grown in insect-based medium than when grown on glucose. Other substrates, such as F and PF, also had high levels of protein in the exudates, but their protein production stopped or began to decline after 96 h of culture (Figures 3B,C). In all other substrates, which were not based on insects, the total concentration of total proteins obtained was <0.5 mg/mL (Figure 3A).
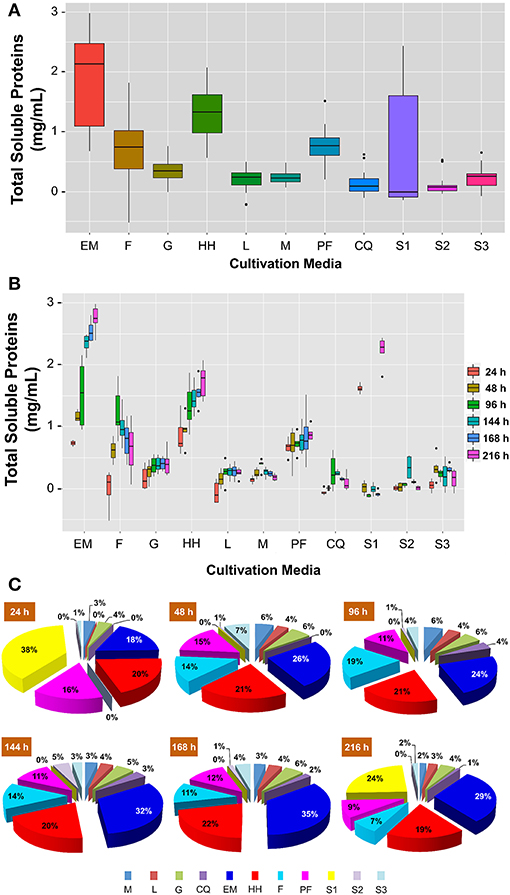
Figure 3. Total soluble proteins exuded by B. bassiana grown in 11 different culture media. (A) Total proteins exuded after 9 days (216 h) of cultivation. The boxes display the central tendency and spread for each culture medium. (B) Total soluble protein exuded by B. bassiana through time in 11 culture media. The boxes display the central tendency and spread for each treatment at six different times: 24 h (red), 48 h (yellow), 96 h (green), 144 h (turquoise), 168 h (blue), and 216 h (pink). For (A,B), the horizontal line inside each box shows the median for each treatment and the dots outside the boxes represent outliers. (C) Total soluble proteins exuded by B. bassiana as a percentage of the total protein exuded at the time of sampling (24, 48, 96, 144, 168, and 216 h). The cultures containing EM and HH consistently show the highest protein concentrations through the 9-day experiments. Minimal Medium (M), minimal medium with simple carbon substrate (G, glucose; L, laminarin; CQ, colloidal chitin; S1, S2, and S3, Media with three saponin concentration 0.036, 0.09, and 0.179% w/v, respectively), and minimum medium with complex carbon substrate (EM, E. melanocampta powder; HH, H. hampei powder; F, F. oxyparum powder; PF, P. variabilis powder). p < 0.05, n = 6 independent cultivations.
These results indicate that non-complex nutrient sources such as glucose or laminarin are not sufficient to satisfy the nutritional requirements of B. bassiana. Complex substrates such as insect powders are required for this fungus to produce a large amount of total protein. In the case of saponins, previous research showed that they could inhibit proteins secretion (Wang et al., 2020), that is why they were included in the proteins tests but not in biomass experiments. In general, the results obtained with the media containing saponins confirm the previous results, B. bassiana does not exude much protein. Although an unexpected high protein concentration was observed at 24 and 216 h in the S1 medium, the mean median (0.13 mg/mL) was lower than in the cultures with simple carbon sources or without carbon source (Figures 3B,C). Finally, this study shows that protein production by B. bassiana is constant throughout (24–216 h) when using insect-based media, EM, and HH (Figure 3C).
Interrelation of Variables: Culture Medium, Biomass, and Total Protein
The analysis of the correlation between the culture medium, the biomass yield, and the concentration of total exuded proteins clearly showed that these three variables were significantly correlated (59%, p < 0.001). This means that the carbon source in the culture medium used to grow B. bassiana has a great effect on the final biomass and the amount of protein exuded. When looking only at the correlation between total exuded protein and biomass, the correlation is even higher (>80%, p < 0.001) which basically means that by having a high biomass yield it is possible to have a high concentration of total protein too.
Discussion
The aim of this study was to investigate the conversion of insect feed into protein using a fungus. The organism used for this transformation was the entomopathogenic fungus B. bassiana. This fungus was chosen because of its pan-global distribution, wide range of host insects, and vast ecological niches (McGuire and Northfield, 2020). As hypothesized, our results showed that B. bassiana is an efficient protein producer when grown in a medium prepared with insects. Since B. bassiana feeds on a wide range of insect species, it has the potential to be a great source of proteins, secondary metabolites, and small molecular compounds for various biotechnological applications. We believe that this is the beginning of a path of discovery of all the benefits that B. bassiana can bring, especially to the food industry, if it is discovered that its proteins have the same or better qualities than the mycoproteins currently on the market (Finnigan, 2011).
Insects are being touted as a very rich source of sustainable protein and, although they are common food in several parts of the world, their introduction to Western cultures has been proven challenging as most people are reluctant to eat them (Collins et al., 2019; Wendin and Nyberg, 2021). The extraction of protein from insects for human food products could be a useful way to increase acceptability among wary consumers. However, at present, the cost of extracting protein from insects is prohibitive. Furthermore, the removal of chitin-rich body parts such as the cuticle, wings, and legs, which has to be done by hand, further increases costs (van Huis, 2013). There is no doubt that more research is needed to find optimal, cost-effective, and environmentally friendly processing conditions for insect proteins and their by-products (Liceaga, 2021).
On the other hand, proteins from fungi have been consumed for many years and are low in fat and high in protein and fiber (Denny et al., 2008; Smith et al., 2015). Positive effects on blood Cholesterol concentration (Ruxton and McMillan, 2010) and glycemic response (Denny et al., 2008; Bottin et al., 2016) have been reported. They are also more efficient in use of land and water (Smetana et al., 2018).
Beauveria bassiana is an entomopathogenic fungus mainly used to control some pests of plant crops (Wakil et al., 2014). It has the ability to exude different protein and metabolites, which can vary depending on the substrate on which the fungus feeds. In addition to proteins, which have been shown to have a high nutritional value, B. bassiana has also developed an antioxidant machinery that produces, among other relevant antioxidants, glutathione (GSH). GSH is widely used in the food industry (as a flavor stabilizer) and in the pharmaceutical and cosmetic industries (Schmacht et al., 2017; Zhang and Feng, 2018).
Our results also showed that single carbon sources, such as glucose, did not provide all the nutrients required by B. bassiana to stably produce large biomass and a high percentage of proteins. Another reason to use insects as feed instead of single carbon sources.
Recent research clearly shows that fungal mycoproteins are becoming a well-established food source with potential uses in health benefits. The evidence about its benefits has accumulated over the last half century, along with its wide acceptance. The use of entomopathogenic fungi as an intermediate link would increase the use of insects while providing high quality proteins that are widely accepted by consumers.
There is a growing demand for sustainable food proteins using technologies that are both green (eco-innovative) and cost-effective (Fasolin et al., 2019). Additionally, the COVID-19 pandemic has put the spotlight on meat supply chains and food safety around the world, further driving demand for novel-food alternatives. Mycoproteins are produced at scale using an aerobic fermentation system and carbohydrate and nutrient substrates (Finnigan et al., 2019). The use of insects as a feeding substrate would allow the production of high-quality proteins with a relatively benign environmental footprint. Since single carbon sources, e.g., glucose, do not provide all the nutrients that B. bassiana needs to produce a large amount of biomass and proteins, the use of insects as a cheap source of carbon is clearly a good option.
It is known that micellar augmentation requires an adequate carbon/nitrogen ratio and nutrients such as chitin, starch, fatty acids, and N-acetylglucosamine, among others compounds (Gaderer et al., 2017). Our results indicate that the insect-based media provides a sufficient amount of all these nutrients to B. bassiana throughout its growth and for a prolonged period (up to 9 days).
Current studies indicate that filamentous fungi are very efficient protein producers. Aspergillus niger, for instance, can produce at least 25–30 g/L of proteins. This efficiency can be further increased with the use of genetic engineering and biotechnology (Ward, 2012; Wang et al., 2020). Overall, these results show that the use of insects as feed for the cultivation of fungi and the production of proteins is promising. As a next step, the proteins and metabolites produced by B. bassiana need to be further characterized and the fermentation scaled-up to industrial levels.
Data Availability Statement
The raw data supporting the conclusions of this article will be made available by the authors, without undue reservation.
Author Contributions
AG and PL carried out the cultivation of B. bassiana with the different carbon sources and reported the results in writing. RA did the statistical analysis and corrected the draft of the manuscript. AG and ON supervised the laboratory work and corrected the manuscript. CS-C analyzed the data, corrected it, and revised the manuscript. AG and NL did the literature search, conceived the idea for the manuscript, and wrote most of it. All authors have approved the final version of the manuscript.
Funding
The authors thank the National Program of Innovation for Competitiveness and Productivity (PNICP) in Perú for financing this project (Agreement No. 189-FINCyT-IB-2013).
Conflict of Interest
The authors declare that the research was conducted in the absence of any commercial or financial relationships that could be construed as a potential conflict of interest.
Publisher's Note
All claims expressed in this article are solely those of the authors and do not necessarily represent those of their affiliated organizations, or those of the publisher, the editors and the reviewers. Any product that may be evaluated in this article, or claim that may be made by its manufacturer, is not guaranteed or endorsed by the publisher.
References
Abdelmenan, S., Berhane, H., Jirström, M., Trenholm, J., Worku, A., Ekström, E. C., et al. (2020). The social stratification of availability, affordability, and consumption of food in families with preschoolers in Addis Ababa: the EAT Addis Study in Ethiopia. Nutrients 12:3168. doi: 10.3390/nu12103168
Acuña-Payano, R., Quiroz-Farfan, D., Laynez-Zela, P., Nolasco-Cárdenas, O., and Gutiérrez-Román, A. (2017). Hypoyhenemus hampei (Coleoptera: Curculionidae) induces in vitro laminarinasa y quitinasa de Beauveria bassiana (Hypocreales: Clavicipitaceae). Rev. Colomb. Entomol. 43, 7–13. doi: 10.25100/socolen.v43i1.6639
Arsham, H., and Lovric, M. (2011). “Bartlett's test,” in International Encyclopedia of Statistical Science, ed M. Lovric (Berlin; Heidelberg: Springer). doi: 10.1007/978-3-642-04898-2_132
Bottin, J. H., Swann, J. R., Cropp, E., Chambers, E. S., Ford, H. E., Ghatei, M. A., et al. (2016). Mycoprotein reduces energy intake and post-prandial insulin release without altering glucagon-like peptide-1 and peptide tyrosine-tyrosine concentrations in healthy over-weight and obese adults: a randomised-controlled trial. Br. J. Nutr. 116, 360–374. doi: 10.1017/S0007114516001872
Cherry, P., O'Hara, C., Magee, P., McSorley, E., and Allsopp, P. (2019). Risks and benefits of consuming edible seaweeds. Nutr. Rev. 77:66. doi: 10.1093/nutrit/nuy066
Clark, M. A., Domingo, N. G. G., Colgan, K., Thakrar, S. K., Tilman, D., Lynch, J., et al. (2020). Global food system emissions could preclude achieving the 1.5° and 2°C climate change targets. Science 370:6517. doi: 10.1126/science.aba7357
Cohen, K. K., Muhardi, L., Parikh, P., Basso, M., Jan Mohamed, H. J., Prawitasari, T., et al. (2021). Nutritional Support of Neurodevelopment and Cognitive Function in Infants and Young Children-An Update and Novel Insights. Nutrients, 13(1):199. doi: 10.3390/nu13010199
Collins, M., Vaskou, P., and Kountouris, Y. (2019). Insect food products in the western world: assessing the potential of a new ‘Green’ market. Ann. Entomol. Soc. Am. 112, 518–528. doi: 10.1093/aesa/saz015
Denny, A., Aisbitt, B., and Lunn, J. (2008). Mycoprotein and health nutr ition. Bulletin 33, 298–310. doi: 10.1111/j.1467-3010.2008.00730.x
Derbyshire, E., and Delange, J. (2021). Fungal protein – what is it and what is the health evidence? A systematic review focusing on mycoprotein. Front. Sustain. Food Syst. 5:581682. doi: 10.3389/fsufs.2021.581682
Devi, R., Kaur, T., Guleria, G., Rana, K., Kour, D., Yadav, N., et al. (2020). “Fungal secondary metabolites and their biotechnological applications for human health,” in Trends of Microbial Biotechnology for Sustainable Agriculture and Biomedicine Systems: Perspectives for Human Health, eds A. A. Rastegari, A. N. Yadav, and N. Yadav (Amsterdam: Elsevier), 147–161. doi: 10.1016/B978-0-12-820528-0.00010-7
Fasolin, L. H., Pereira, R. N., Pinheiro, A. C., Martins, J. T., Andrade, C. C. P., Ramos, O. L., et al. (2019). Emergent food proteins - towards sustainability, health and innovation. Food Res. Int. 125:108586. doi: 10.1016/j.foodres.2019.108586
Fernandes, E. G., Valério, H. M., Feltrin, T., and Van Der Sand, S. T. (2012). Variability in the production of extracellular enzymes by entomopathogenic fungi grown on different substrates. Brazil J. Microbiol. 43, 827–833. doi: 10.1590/S1517-83822012000200049
Finnigan, T. J. A. (2011). “Mycoprotein: origins, production and properties,” in Handbook of Food Proteins, eds G. O. Phillips, and P. A. Williams (Cambridge: Woodhead Publishing), 335–352. doi: 10.1533/9780857093639.335
Finnigan, T. J. A., Wall, B., Wilde, P., Stephens, F., Taylor, S. L., and Freedman, M. (2019). Mycoprotein: the future of nutritious nonmeat protein, a symposium review. Curr. Dev. Nutr. 3:nzz021. doi: 10.1093/cdn/nzz021
Gaderer, R., Seidl-Seiboth, V., de Vries, R., Seiboth, B., and Kappel, L. (2017). N-acetylglucosamine, the building block of chitin, inhibits growth of Neurospora crasa. Fungal Genetics Biol. 107, 1–11. doi: 10.1016/j.fgb.2017.07.005
Hashempour, B. F., Khosravi, D. K., Hosseini, H., Farshi, P., Reihani, S., and Fatemeh, S. (2020). Mycoproteins as safe meat substitutes. J. Clean. Product. 253:119958. doi: 10.1016/j.jclepro.2020.119958
Hwang, J., You, J., Moon, J., and Jeong, J. (2020). Factors affecting consumers' alternative meats buying intentions: plant-based meat alternative and cultured meat. Sustainability 12:5662. doi: 10.3390/su12145662
Hyde, K. D., Xu, J., Rapior, S., et al. (2019). The amazing potential of fungi: 50 ways we can exploit fungi industrially. Fungal Diversity 97, 1–136. doi: 10.1007/s13225-019-00430-9
Jongema, Y. (2017). Worldwide List of Recorded Edible Insects. Wageningen: Department of Entomology, Wageningen University & Research.
Laynes, P., Acuña, R., and Gutiérrez, A. (2016). Obtención de esporas y biomasa de Beauveria bassiana en medios líquidos suplementados con Eurysacca melanocampta y Peronospora farinosa. Biologist 14, 75–87. doi: 10.24039/rtb201614188
Le, B., and Yang, S. H. (2019). Microbial chitinases: properties, current state and biotechnological applications. World J. Microbiol. Biotech. 35:144. doi: 10.1007/s11274-019-2721-y
Liceaga, A. M. (2021). Processing insects for use in the food and feed industry. Curr. Opin. Insect Sci. 48, 32–36. doi: 10.1016/j.cois.2021.08.002
Lo, Y. T., Chang, Y. H., Lee, M. S., and Wahlqvist, M. L. (2009). Health and nutrition economics: diet costs are associated with diet quality. Asia Pacific J. Clin. Nutr. 18, 598–604.
Lynch, J., Cain, M., Frame, D., and Pierrehumbert, R. (2021). Agriculture's Contribution to Climate Change and Role in Mitigation Is Distinct From Predominantly Fossil CO2-Emitting Sectors. Front Sustain Food Syst. 4:518039. doi: 10.3389/fsufs.2020.518039
McGuire, A. V., and Northfield, T. D. (2020). Tropical occurrence and agricultural importance of Beauveria bassiana and Metarhizium anisopliae. Front. Sustain. Food Syst. 4:6. doi: 10.3389/fsufs.2020.00006
Mondal, S., Baksi, S., Koris, A., and Vatai, G. (2016). Journey of enzymes in entomopathogenic fungi. Pacific Sci. Rev. A 18, 85–99. doi: 10.1016/j.psra.2016.10.001
Peteira, B., Gonzales, I., Fernández, A., Miranda, I., and Martinez, B. (2011). Caracterización bioquímica de seis aislamientos de Beauveria bassiana (Balsamo) vuillemin. Rev. Protec. Veg. 26, 16–22.
Rosenzweig, C., Mbow, C., Barioni, L. G., et al. (2020). Climate change responses benefit from a global food system approach. Nat. Food 1, 94–97. doi: 10.1038/s43016-020-0031-z
Rumpold, B. A., and Schlüter, O. (2015). Insect-based protein sources and their potential for human consumption: nutritional composition and processing. Anim. Front. 5:15. doi: 10.2527/af.2015-0015
Ruxton, C., and McMillan, B. (2010). The impact of mycoprotein on blood cholesterol levels: a pilot study. Br. Food J. 112:109. doi: 10.1108/00070701011080221
Sánchez-Pérez, L., Rodriguez-Navarro, S., Marin-Cruz, V. H., Ramos-Lopez, M. A., Ramos, A. P., and Barranco-Florido, J. E. (2016). Assessment of Beauveria bassiana and their enzymatic extracts against Metamasius spinolae and Cyclocephala lunulata in laboratory. Adv. Enzyme Res. 4, 98–112. doi: 10.4236/aer.2016.43010
Sato, H., Tsukamoto-Yasui, M., Takado, Y., Kawasaki, N., Matsunaga, K., Ueno, S., et al. (2020). Protein deficiency-induced behavioral abnormalities and neurotransmitter loss in aged mice are ameliorated by essential amino acids. Front. Nutr. 7:23. doi: 10.3389/fnut.2020.00023
Schmacht, M., Lorenz, E., and Senz, M. (2017). Microbial production of glutathione. World J. Microbiol. Biotechnol. 33:106. doi: 10.1007/s11274-017-2277-7
Semba, R. D. (2016). The rise and fall of protein malnutrition in global health. Annals Nutr. Metabol. 69, 79–88. doi: 10.1159/000449175
Shapiro, S. S., and Wilk, M. B. (1965). An analysis of variance test for normality (complete samples). Biometrika. 52, 591–611. doi: 10.1093/biomet/52.3-4.591
Smetana, S., Aganovic, K., Irmscher, S., and Heinz, V. (2018). Agri-Food Waste Streams Utilization for Development of More Sustainable Food Substitutes. Designing Sustainable Technologies, Products and Policies: From Science to Innovation. Cham: Springer, 145–215. doi: 10.1007/978-3-319-66981-6_17
Smith, H., Doyle, S., and Murphy, R. (2015). Filamentous fungi as a source of natural antioxidants. Food Chem. 185, 389–397. doi: 10.1016/j.foodchem.2015.03.134
Sovacool, B. K., Bazilian, M., Griffiths, S., Kim, J., Foley, A., and Rooney, D. (2021). Decarbonizing the food and beverages industry: a critical and systematic review of developments, sociotechnical systems and policy options. Renew. Sustain. Energy Rev. 143:110856. doi: 10.1016/j.rser.2021.110856
van Huis, A. (2013). Potential of insects as food and feed in assuring food security. Annu. Rev. Entomol. 58, 563–583. doi: 10.1146/annurev-ento-120811-153704
Wakil, W. N. G, Kavallieratos, M. U., Ghazanfar, M., Usman, A., Habib, H. A. F., and El-Shafie (2021). Efficacy of different entomopathogenic fungal isolates against four key stored-grain beetle species. J. Stored Prod. Res. 93:101845. doi: 10.1016/j.jspr.2021.101845
Wakil, W. M. U., Ghazanfar, M., and Yasin. (2014). Naturally occurring entomopathogenic fungi infecting stored grain insect species in Punjab, Pakistan. J. Insect Sci. 14:44. doi: 10.1093/jisesa/ieu044
Wang, Q., Zhong, C., and Xiao, H. (2020). Genetic engineering of filamentous fungi for efficient protein expression and secretion. Front. Bioeng. Biotechnol. 8:293. doi: 10.3389/fbioe.2020.00293
Ward, O. P. (2012). Production of recombinant proteins by filamentous fungi. Biotechnol. Adv. 30, 1119–1139. doi: 10.1016/j.biotechadv.2011.09.012
Wendin, K., and Nyberg, M. (2021). Factors influencing consumer perception and acceptability of insect-based foods. Curr. Opin. Food Sci. 40, 67–71. doi: 10.1016/j.cofs.2021.01.007
Keywords: Beauveria bassiana, insect-based media, mycoproteins, insects, fungal biomass
Citation: Gutiérrez Román AIF, Laynes Zela PF, Acuña Payano RK, Nolasco Cárdenas OP, Santa-Cruz Carpio CM and Leiva Eriksson NR (2022) Production of Sustainable Proteins Through the Conversion of Insects to Proteins Using Beauveria bassiana Cultures. Front. Sustain. Food Syst. 5:760274. doi: 10.3389/fsufs.2021.760274
Received: 17 August 2021; Accepted: 20 December 2021;
Published: 20 January 2022.
Edited by:
Waqas Wakil, University of Agriculture, PakistanReviewed by:
Muhammad Usman Ghazanfar, University of Sargodha, PakistanShi Wangpeng, China Agricultural University, China
Copyright © 2022 Gutiérrez Román, Laynes Zela, Acuña Payano, Nolasco Cárdenas, Santa-Cruz Carpio and Leiva Eriksson. This is an open-access article distributed under the terms of the Creative Commons Attribution License (CC BY). The use, distribution or reproduction in other forums is permitted, provided the original author(s) and the copyright owner(s) are credited and that the original publication in this journal is cited, in accordance with accepted academic practice. No use, distribution or reproduction is permitted which does not comply with these terms.
*Correspondence: Ana I. F. Gutiérrez Román, agutierrez@unfv.edu.pe; Nélida R. Leiva Eriksson, nelida.leiva_eriksson@biotek.lu.se