- 1Sustainable Impact Platform, International Rice Research Institute, Los Baños, Philippines
- 2African Plant Nutrition Institute, Mohammed VI Polytechnic University Experimental Farm, Benguérir, Morocco
- 3Sustainable Impact Platform, International Rice Research Institute, New Delhi, India
- 4Analytical Services Laboratory, Department of Soil Science, Agricultural Systems Institute, College of Agriculture and Food Sciences, University of the Philippines, Los Baños, Philippines
Fertilizer use and genetic improvement of cereal crops contributed to increased yields and greater food security in the last six decades. For rice, however, fertilizer use has outpaced improvement in yield. Excess application of nutrients beyond crop needs, especially nitrogen (N), is associated with losses to the environment. Environmental pollution can be mitigated by addressing fertilizer overuse, improving N use efficiency, while maintaining or improving rice productivity and farmers' income. A promising approach is the site-specific nutrient management (SSNM), developed in the 1990s to optimize supply to meet demand of nutrients, initially for rice, but now extended to other crops. The SSNM approach has been further refined with the development of digital decision support tools such as Rice Crop Manager, Nutrient Expert, and RiceAdvice. This enables more farmers to benefit from SSNM recommendations. In this mini-review, we show how SSNM can foster sustainability in rice production systems through improved rice yields, profit, and N use efficiency while reducing N losses. Farmer adoption of SSNM, however, remains low. National policies and incentives, financial investments, and strengthened extension systems are needed to enhance scaling of SSNM-based decision support tools.
Introduction
Optimal nutrient management in rice is important for food security, climate change mitigation, adaptation and transformation, and attainment of several sustainable development goals (Cakmak, 2002; Kanter et al., 2019; Lal et al., 2020). Fertilizer use has reduced agriculture expansion into natural ecosystems by increasing crop productivity on existing land. However, while yields increased with fertilizer use in the 1960s, they stagnated in intensive rice systems in the mid-1980s despite the development of varieties with greater yield potential (Dawe and Dobermann, 1999). This resulted in large yield gaps. This was largely due to excessive or imbalanced fertilizer use based on increased reliance on blanket fertilizer application, coupled with a rapid decline in the efficiency of fertilizer uptake by plants, indicating that increased fertilizer use outpaced yield improvements (Cassman and Pingali, 1995; Tilman et al., 2002). The orientation of producing more food, associated with fertilizer overuse, particularly nitrogen (N), has caused a deterioration in soil physical, chemical, and microbiological properties and functions and increased soil and water pollution (Pingali, 2012; Srivastava et al., 2020). With increasing pressure to meet global food demand while fostering environmental sustainability, a paradigm shift is needed to a more judicious use of N fertilizer.
About 50% of global N fertilizer is applied to major cereals: Zea mays (maize; 17%), Triticum aestivum (wheat; 18%), and Oryza sativa (rice; 16%) (Heffer et al., 2017). However, globally, N use efficiency, a measure of the short-term balance between N used for grain production and N lost to the environment, has remained below 40% (Omara et al., 2019), indicating that more than 60% of applied N remains unused or is lost from soil (Dobermann, 2000; Ladha et al., 2005). Increasing N use efficiency in rice agri-food systems becomes all the more important, given that the commodity is a staple food for more than half the global population (GRiSP, 2013). More than 90% of the rice is produced in Asia, mostly by smallholder farmers. Due to high subsidy on urea fertilizer, farmers tend to apply large quantities of N fertilizer in excess of plant requirements (Ladha et al., 2005). However, grain yield response diminishes as N fertilizer rate increases and may cause lodging and susceptibility to pest and disease damage when overapplied (Balasubramanian et al., 1998; Duy et al., 2004). Excess reactive N has detrimental effects in agroecosystems, such as nitrous oxide emissions, increased soil acidity, decreased biodiversity, and groundwater contamination (Galloway et al., 2003).
Nitrogen Use Efficiency Trends in Rice Production Systems
In rice production, farmers apply large amounts of N fertilizer to maximize yield, but only 20–50% of N is taken up by the crop. N use efficiency remains low with a global average partial factor of productivity N (PFP N) of about 40 kg grain kg−1 N applied (Figure 1). This is largely due to farmers applying large quantities of N fertilizer at early growth stages when the rice plants have not fully developed the root system. The resulting loss of the applied N, which is a mobile nutrient leads to increased water and land pollution and greenhouse gas (GHG) emissions (Shaviv and Mikkelsen, 1993; Xu et al., 2012; Zhang et al., 2013). Dobermann (2007) reviewed the commonly used N use efficiency indices in agronomy research, which include agronomy efficiency, recovery efficiency, internal efficiency, physiological efficiency, and PFP N. PFP N is commonly used in agronomy and is useful when comparing across different management practices and where there are no N omission plots to enable calculation of other indices (Dobermann, 2007). PFP N is an aggregate index which integrates indigenous N supply from the soil and that applied from external sources. It generally declines with increasing N application rates.
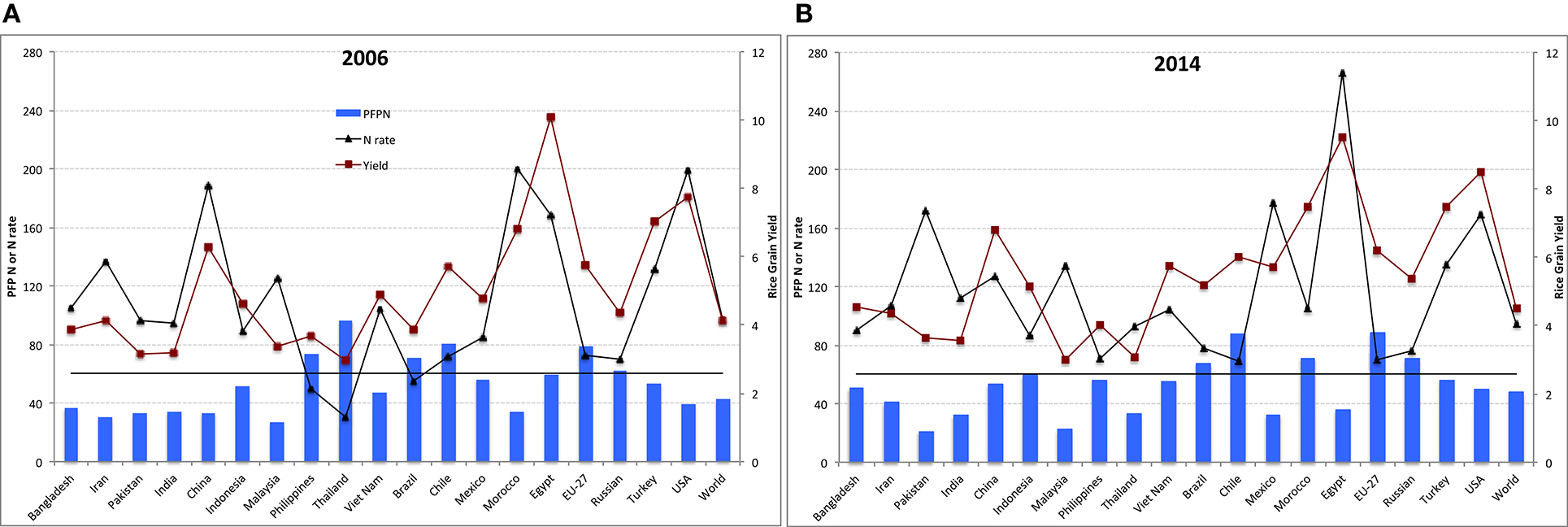
Figure 1. Rice grain yield (Mg ha−1), partial factor of productivity (PFP N; kg grain kg−1 N), and nitrogen rate (kg N ha−1) for selected rice growing countries in (A) 2006 and (B) 2014. The black line indicates PFP N = 60 kg grain kg−1 N (Data source: Heffer et al., 2017 and FAOSTAT, 2019).
A PFP N of 60 kg grain kg−1 N applied or greater indicates well-managed systems (Dobermann, 2005). PFP N has remained well below this threshold in many rice growing Asian countries compared to developed countries (Heffer et al., 2017). While PFP N increased between 2006 and 2014 in China, Indonesia, and Vietnam, it stagnated in India at 33 kg grain kg−1 N (Figure 1). In contrast, in the Philippines, Thailand, Iran, and Pakistan, PFP N declined during the same period. It should be noted, however, that available data on fertilizer use by crop and country are unreliable. The contrasting PFPs could be due to management, e.g., the reduction in N fertilizer rate associated with the controls introduced in China (Chen et al., 2014) could account for the increase in PFP N. The Rice Research Institute of the Guangdong Academy of Agricultural Sciences introduced the Three Controls Technology to control the amount on N fertilizer. This technology includes controls on the amount and timing of N fertilizer using site-specific nutrient management (SSNM) approach, controls on the number of tillers and controls on the use of pesticides and herbicides.
While increasing N use efficiency in rice systems is essential for sustainability, low input rice systems in Sub-Saharan Africa (SSA) are characterized by high N use efficiency, sometimes > 100 kg grain kg−1 N applied. These systems are associated with mining of nutrients, resulting in land degradation (Dobermann, 2005, 2007; Edmonds et al., 2009). Africa contributes about 4.5% to global rice production (FAOSTAT, 2019). This is not enough to meet rice demandin Africa, which is increasing as dietary preferences shift from the traditional coarse grains owing to urbanization and changing family occupational structure. Rice production in SSA has increased in recent years, largely due to expansion of area than increased productivity, i.e., yield per unit area (FAOSTAT, 2019), but has been outpaced by consumption demand; much of which has been supported by imports, mostly from Asia. While global rice yields average at 4 t ha−1, yields in SSA average 2 t ha−1 (GRiSP, 2013); <50% of attainable yield. This is caused by a myriad of issues, among them; low soil fertility and limited fertilizer use, use of home retained seeds and traditional varieties, labor shortage, weak markets, and lack of infrastructure and equipment for irrigation. Rice productivity in SSA can be increased via the introduction of improved cultivar and agronomic management practices.
Exploitable rice yield gaps remain in Asia and SSA (Stuart et al., 2016). There is a need for tailored solutions that are sustainable and meet the increasing global demand for food, feed, and energy while protecting the environment. Rice production also needs to be profitable for farmers; this can be partly achieved by farmers applying appropriate types and amounts of fertilizers. Fertilizers typically constitute 20% of the input costs in rice production (Pampolino et al., 2007) and achieving efficient fertilizer management is challenging in smallholder farming systems where soils and crop management can vary even within short distances. SSNM enable farmers to apply adequate and appropriate amounts of nutrients to suit soil, crop variety, and climate, hence mitigating the potential trade-offs between productivity and environmental health.
The SSNM Approach
The SSNM approach was developed in the 1990s to calculate field-specific requirements for fertilizer N, P, and K for cereal crops, taking into consideration the indigenous nutrient supply and the target yield (Dobermann et al., 2002, 2004). SSNM was initially conceptualized for small holder rice producers in Asia, where fields tend to be small with large spatial variability in terms of nutrient status and management. SSNM is based on the principles of the Quantitative Evaluation of the Fertility of Tropical Soils (QUEFTS) model (Janssen et al., 1990) to estimate the requirement for a fertilizer nutrient from the gap between the total amount of nutrient required to achieve a specific target yield and the indigenous supply of the nutrient (Witt and Dobermann, 2004). The approach allows balanced application of major nutrients. Timing of fertilizer application is adjusted to meet peak nutrient demand of the crop to enhance nutrient use efficiency and foster environmental sustainability. Using SSNM principles, field-, crop-, and season-specific requirements for N, P, and K are calculated at the beginning of the season (Dobermann et al., 2002; Buresh et al., 2010).
SSNM-Based Decision Support Tools
SSNM has evolved along a research to impact pathway, with refinement in the science and methods, expansion to new geographies, and the development of decision support tools for its dissemination. Leaf color charts, typically plastic strips containing four to six panels with colors ranging from yellowish green to dark green, were developed to monitor leaf greenness, which is related to N status and thus aid assessment and adjustment of N requirements during the season (Singh et al., 2002; Witt et al., 2005). An ICT decision support tool, the Nutrient Manager, was developed to give field-specific fertilizer recommendations for rice production, initially as Microsoft Access, but it evolved to be web-based (Buresh et al., 2014) and was eventually developed as Rice Crop Manager [RCM] (Buresh et al., 2019; Sharma et al., 2019a). RCM is a web-based and open access decision tool that provides farmers with simplified, easy to follow, and appropriate nutrient management recommendations (https://phapps.irri.org/ph/rcm/, http://webapps.irri.org/in/od/rcm/). Similar tools were developed for different regions and crops: Nutrient Expert (Pampolino et al., 2012; Xu et al., 2017a) and RiceAdvice in West Africa (Zossou et al., 2020; Arouna et al., 2021). These tools can be integrated with GIS and remote sensing for holistic and precise knowledge delivery to greater numbers of farmers. The tools provide information on yield predictions, which is useful for more accurate estimation of nutrient requirements, and allow for dynamic nutrient management with mid-season nutrient management adjustments to match crop condition and needs.
Methods
Data from 46 published articles with studies conducted between 2001 and 2020 were compiled in a database (Table 1). These studies were conducted in 11 countries: eight in Asia and three in Africa. Using Web of Science, Science Direct, and Google Scholar, we used the following search terms: SSNM, SSNM rice, SSNM maize, SSNM wheat, SSNM cereals, and SSNM vs. farmers' fertilizer practice (FFP). The studies included peer reviewed journal publications, book chapters, and technical reports that show direct comparison between SSNM and FFP in the same fields. We excluded studies when SSNM was compared with other treatments such as the no input control, blanket fertilizer recommendation, government or institute recommendation, or soil test-based recommendations. We included only studies that followed the generic SSNM approach and excluded studies where factors other than fertilizer management differed between SSNM and FFP. When multiple publications reported the data from the same experiments, we used the paper with the most complete dataset. Studies were excluded if the experimental method was vague and when there are varied factors other than fertilizer management between SSNM and FFP treatments. Hence, agronomic practices except nutrient management were the same or similar in both treatments. Of the 46 studies, 23 of them conducted N omission treatment thus enabling them to calculate and report AEN (Equation 1). In some cases, AEN values were extracted from the papers. Partial factor of productivity of N (PFP N) was calculated for all studies (Equation 2).
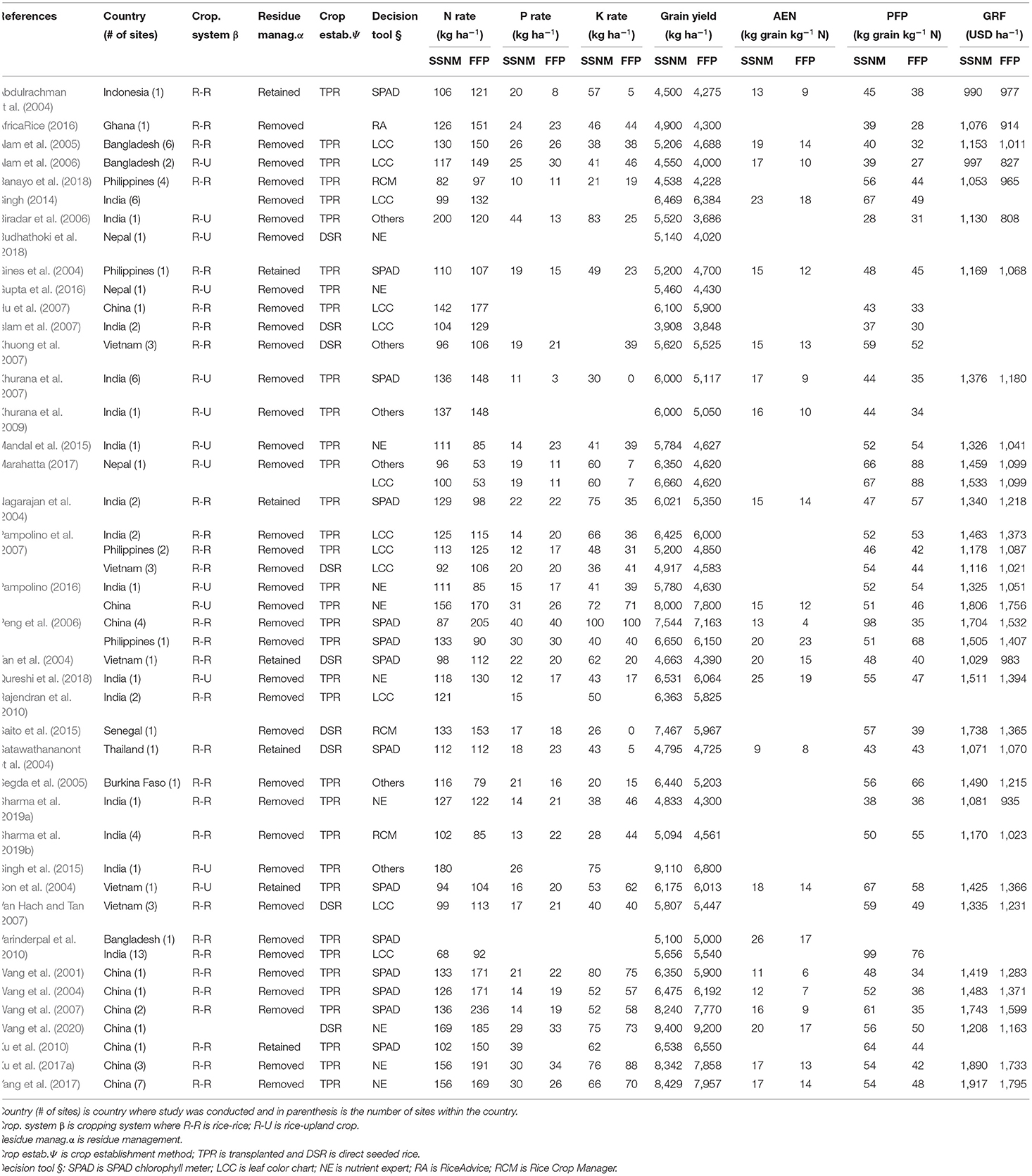
Table 1. A synthesis of studies conducted in rice cropping systems on different sites in different countries in Asia and Africa under different agronomic management practices that evaluated the site-specific nutrient management (SSNM) compared to the farmer fertilizer practice (FFP) in terms of grain yield, agronomic efficiency of N (AEN), partial factor productivity of N (PFP N), and gross return above fertilizer cost (GRF).
Where GYN is the grain yield in a treatment with N application.
GY0 is the grain yield in a treatment without N application.
Averages of yields and AEN of both SSNM and FFP were obtained from each study, while in some instances, manual estimation from the figures was performed when these data were only presented in figures. Individual studies have variable number of replicates with 323 as the highest number. Also, nutrient rates among the fields varied and were reported as a range because there is a high spatial variability even for neighboring fields. Thus, the average between the minimum and maximum values reported was determined across the replicates and was used as nutrient rate for the reported grain yield. The most common sources of fertilizers used in the studies were urea for nitrogen, DAP for P, and muriate of potash (KCl) for potassium.
The cost benefits were reported as gross return above fertilizer cost (GRF) in this paper, which was derived from the other two economic performance metrics: total fertilizer cost (TFC; Equation 3) and gross return (Equation 4). GRF was calculated as in Equation 5. Fertilizer prices of the most common sources: urea (46-0-0) for N, DAP (18-46-0) for N and P, and KCl (0-0-60) for K (urea, DAP, and KCl), were estimated from the 10-year average across countries listed in the database (Indexmundi, 2020; https://www.indexmundi.com). The prices were calculated as per unit of nutrient leading to US$0.642 kg−1 N, US$2.151 kg−1 P, and US$0.633 kg−1 K. Farm gate price of paddy rice was used at US$0.25 kg−1 paddy rice based on the trend of the market price for the past 25 years (Indexmundi, 2020; https://www.indexmundi.com).
Where pN, pP, pK = prices of N, P and K fertilizers, respectively (US $ kg−1).
Nrate, Prate, Krate = amount of N, P, and K applied (kg ha−1).
FGP = farm gate price of paddy rice, maize, or wheat (US$ kg−1).
GY = grain yield of paddy rice, maize, and wheat (kg ha−1).
Performance of SSNM
We conducted a mini-review using 46 studies (43 from Asia and three from SSA). This shows the paucity of research in SSA, despite the low rice productivity against an increasing rice demand in the region. Our analysis shows that on average, the implementation of SSNM recommendations resulted in 644 kg ha−1 (11.4%) more rice yield compared to the farmer fertilizer practice (FFP; Table 1). This was associated with 38.2, 18.2, and 8.6% greater agronomic efficiency of N, PFP N, and gross return above fertilizer cost (GRF; a measure of economic performance) with SSNM compared to FFP, respectively. These benefits accrued while using 14% less N fertilizer than FFP (Table 1), similar to observations by Peng et al. (2010). The lower N fertilizer also resulted in an increased GRF by US$178 ha−1 and a higher agronomic N use efficiency (AEN) under SSNM (17 kg grain kg−1 N) than FFP (12 kg grain kg−1 N applied) and PFP N (58 vs. 47 kg grain kg−1 N). The FFP is often based on blanket recommendations with unbalanced nutrient application in many cases (Wang et al., 2001; Dobermann et al., 2002; Peng et al., 2010).
Increased N use efficiency in SSNM was attributed to the distribution of N fertilizer applications (i.e., timing, amount, and frequency), resulting in an optimized balance between N supply and crucial stages of crop growth and demand for N. On average, there were 3.5 N-fertilizer splits under SSNM compared to 3.0 under FFP. In addition to reduced N fertilizer, the SSNM approach ensures balanced N, P, and K application contributing to increased N use efficiency. In our review, the largest increases in N use efficiency with SSNM were observed in China, where farmers generally use excessive amounts of N fertilizer (Wang et al., 2001; Peng et al., 2010). Peng et al. (2010) reviewed the performance of SSNM across 107 sites in China conducted over 10 seasons and showed on average, higher N input in FFP (195 kg N ha−1) compared to SSNM (133 kg N ha−1). While that study showed a 5% yield advantage with SSNM, the greater benefits were observed with AEN, which was 61% higher compared to FFP. This suggests significant reduced N losses.
Although most of the reviewed studies were in irrigated lowland ecosystems, where the SSNM approach was developed, Biradar et al. (2006) and Banayo et al. (2018) in India and in the Philippines, respectively, conducted studies under rain-fed ecosystems and reported higher rice yield and GRF under SSNM compared to FFP. However, the higher yield for SSNM in India was achieved with about 1.7 times more N fertilizer than in FFP, resulting in a lower PFP N under SSNM. It is likely that the algorithms of SSNM evaluated a greater N requirement in rain-fed systems but that could be an overestimation since SSNM has not been optimized with limited trials under rain-fed systems. Overestimation could lead to lower PFPN, thus further evaluation and calibration of SSNM under rain-fed environments are needed.
Rice yield, N use efficiencies and GRF responses to SSNM recommendations varied depending on crop establishment methods. Greater benefits from SSNM compared to FFP were observed for transplanted than direct-seeded rice (DSR; Table 1). Faced with labor and water scarcity in transplanted systems (Pampolino et al., 2007), farmers are increasingly adopting DSR (Kumar et al., 2018). However, weeds are a major constraint in DSR systems, leading to reduced crop productivity. A key strategy to enhance rice yield under DSR is to apply N late in the season (Liu et al., 2019), this is in line with SSNM which emphasizes the need to time N supply with demand. There are opportunities for improving SSNM recommendations for DSR, encompassing local conditions and weed management.
Traditional tools based on leaf greenness to assess N status (leaf color charts and SPAD or chlorophyll meter) were used in 21 of the studies and increased rice yield, PFP N and GRF by 6.8, 18.9, and 9.1%, respectively, compared to FFP (Table 1). On the other hand, the use of SSNM-based digital decision-support tools (RCM, Nutrient Expert, RiceAdvice) increased rice yield by 11.7%, PFP N by 11.5%, and GRF by 11.8%. However, RiceAdvice was only used in one study (AfricaRice, 2016). Digital tools provide pre-season recommendations, and allow for in-season N fertilizer adjustments to improve the performance of the recommendations given by the tools. However, extensive adoption of SSNM-based digital tools by farmers in the field has been limited by factors like poor access to the tools, non-availability of internet facilities, low penetration of digital devices in rural areas, non-availability of recommended fertilizers, limited credit for buying the fertilizers, labor shortage lack of concentrated efforts by the local extension agents (Florey et al., 2020). Integrating digital tools with geospatial approaches facilitates improved yield targets and in-season N adjustment based on crop performance and enhances scaling of SSNM recommendations (Xu et al., 2017b).
Discussion
Our mini-review shows SSNM as an effective N management strategy for improving rice productivity and profit for farmers while increasing N use efficiency, thus attaining environmental benefits. On average, optimized nutrient management reduced N fertilizer inputs, improved yields, and hence, increased N use efficiency. While N use efficiency has been used to indicate the balance between N used for grain production and losses to the environment (Dobermann, 2007; Omara et al., 2019), only a few studies have quantified N losses under SSNM. For example, a recent study using Nutrient Expert showed both agronomic and environmental benefits of SSNM in a rice–maize cropping systems in China (Wang et al., 2020). In that study, N losses and GHG emissions with SSNM were 10.1 and 6.6% lower than FFP for rice and 46.9 and 37.2% for maize, respectively. The reduced losses arose from increased N use efficiency. Nutrient Expert was also used for winter wheat in North China where N fertilizer rates were 41.4% lower with SSNM than FFP, leading to a 70% increase in agronomic N use efficiency and 55% lower emissions of N2O (Zhang et al., 2018).
Similarly, using Nutrient Expert in a wheat cropping system in India, GHG emissions were 16–42% lower under SSNM than FFP, both under conventional and no-till, but with greater benefits under no-till (Sapkota et al., 2014). Sapkota et al. (2021) observed a 2.5% reduction in global warming potential associated with reduced GHG emissions, increased rice yields and profit in India when Nutrient Expert was compared to FFP. Earlier studies in the Philippines and Vietnam (Pampolino et al., 2007) and in China (Wang et al., 2007) also showed increased N use efficiency with reduced N losses through leaching, runoff, and N2O emissions with SSNM compared to FFP. SSNM, hence, provides a climate mitigation nutrient management option compared with the FFP. Considering the wide range of conditions where rice is grown, there is need to evaluate the benefits of SSNM in more locations and using different digital tools. However, adoption of SSNM recommendations has been low, highlighting the need to strengthen extension systems through public and private partnerships.
Currently, the SSNM-based digital tools, including RCM, provide pre-season nutrient management recommendations and focus on balanced application of N, P, and K with little emphasis on micronutrients. On the other hand, farmers generally lack awareness of the importance of micronutrients and there are less obvious and/or immediate yield gains and profit associated with micronutrient fertilization. This has resulted in mining of these micronutrients from rice soils, while malnutrition, particularly due to zinc and iron deficiency, is common for communities relying on rice-based diets (Palanog et al., 2019). While much of the research on improving micronutrient nutrition in rice have been through breeding (Dixit et al., 2019), there is also need to optimize micronutrient management, along with major nutrients, for the production of healthier rice through agronomic bio-fortification as soil or foliar application (Hakoomat et al., 2014). Given that more iron and zinc is needed during the early growth stages of rice, application in the soil is more practical than foliar application, which requires the plant leaves to have been developed significantly in order to effectively take up the foliar applied nutrients. However, zinc has been shown to convert to unavailable forms immediately after the application of zinc sulfate in flooded soils (Bunquin et al., 2017). Thus, soil application of zinc is not highly effective in flooded rice fields. Although foliar application of zinc at later growth stages of rice does not result in yield gain, it has been shown to increase grain zinc content and is therefore important for the production of healthier high-zinc rice (Hakoomat et al., 2014; Rubianes et al., 2018).
While micronutrient fertilization improves the nutrition value of rice grain, farmers are often not compensated for the extra input costs. They are unwilling to invest in micronutrient management in the absence of other incentives. Policy shifts are needed to reward farmers for the production of more nutritious and healthier rice; some of them necessarily require active public–private partnerships. For example, the Sustainable Rice Platform, which is promoting a premium price for sustainably produced rice (SRP, 2019). Such efforts can foster environmental sustainability, ensuring that rice systems in the Global South deliver essential ecosystem services while also improving farmers' livelihoods. The changing climate and other driving forces like shortage of labor and water dictate shifts from continuous intensive rice systems to changes in agronomic management practices, such as direct seeded rice, non-puddled rice, and water saving technologies. Increasing rice productivity while minimizing adverse environmental consequences require the adoption of integrated nutrient and crop management practices that increase system-level efficiency.
Conclusion
Our mini-review clearly shows that SSNM in rice cropping systems increases rice yield, profit, and N use efficiency while reducing N losses and GHG emissions when compared with the farmer practice. AEN and PFP were 38.2 and 18.2% greater with SSNM than the farmer practice. This was achieved using 14% lower N fertilizer. The superior performance of SSNM compared to the farmer practice is mainly due to better distribution with more splits of N fertilizer during the growing season coupled with balanced fertilization. However, SSNM has mainly focused on the major nutrients, ignoring micronutrients, and thus, impacting human nutrition for those whose diets are rice-based, while potentially mining the soils of the micronutrients. SSNM-based digital decision support tools enable dissemination of SSNM recommendations at scale, but this requires a pluralistic approach that fosters collaboration among multiple organizations and service providers with support from governments. Additionally, linking the digital tools with GIS and remote sensing tools allows fine-tuning of SSNM recommendations, addressing the huge spatial variability in smallholder farming systems. SSNM research and evaluation has focused on favorable environments in Asia, despite the increasing demand and production in Africa. More research is needed on SSNM under diverse management practices, such as direct seeding, and in marginal environments along with quantification of nutrient losses, along with inter-disciplinary approaches to enhance farmer uptake of SSNM.
Author Contributions
PC, SS, and JH conceived the project. MB extracted data from publications. PC and MB conducted analyses. PC wrote the manuscript draft. All authors contributed to the literature search, interpretation of the results, and writing of the final paper.
Funding
The Swiss Agency for Development and Cooperation (SDC; Grant No. 81016734) through the Closing the Rice Yield Gap (CORIGAP) project provided support for this review. The SSNM approach reviewed here was initially developed under the Reversing Trends of Declining Productivity in Intensive Irrigated Rice Systems project (RTDP), also funded by SDC. SSNM was field tested and fine-tuned through subsequent SDC funded projects including Irrigated Rice Research Consortium (IRRC), a predecessor of CORIGAP.
Conflict of Interest
The authors declare that the research was conducted in the absence of any commercial or financial relationships that could be construed as a potential conflict of interest.
Publisher's Note
All claims expressed in this article are solely those of the authors and do not necessarily represent those of their affiliated organizations, or those of the publisher, the editors and the reviewers. Any product that may be evaluated in this article, or claim that may be made by its manufacturer, is not guaranteed or endorsed by the publisher.
Acknowledgments
We are grateful to SDC for supporting the study.
References
Abdulrachman, S., Susanti, Z., Pahim, A. D., Dobermann, A., and Witt, C. (2004). “Site-specific nutrient management in intensive irrigated rice systems of West Java, Indonesia,” in: “Increasing Productivity of Intensive Rice Systems Through Site-Specific Nutrient Management” (Los Baños: Science Publishers, Inc.), 171–192.
AfricaRice (2016). Terminal Report on Collaboration Project Between Syngenta Foundation for Sustainable Agriculture (SFSA) and Africa Rice Center (AfricaRice): Validating the Services of a Decision Support System for Nutrient Management for Rice in West Africa.
Alam, M. M., Ladha, J. K., Foyjunnessa, R.Z, Khan, S. R., Harun-ur-Rashid Khan, A. H., et al. (2006). Nutrient management for increased productivity of rice–wheat cropping system in Bangladesh. Field Crops Res. 96, 374–386. doi: 10.1016/j.fcr.2005.08.010
Alam, M. M., Ladha, J. K., Khan, S. R., Khan, A. H., and Buresh, R. J. (2005). Leaf color chart for managing nitrogen fertilizer in lowland rice in Bangladesh. Agron. J. 97, 949–959. doi: 10.2134/agronj2004.0206
Arouna, A., Michler, J.D., Yergo, W.G., and Saito, K. (2021). One size fits all? Experimental evidence on the digital delivery of personalized extension advice in Nigeria. Am J Agric Econ. 103, 596–619.
Balasubramanian, V., Morales, A., Cruz, R., and Abdulrachman, S. (1998). On-farm adaptation of knowledge-intensive nitrogen management technologies for rice systems. Nutrient Cycling Agroecosyst. 53, 59–69. doi: 10.1023/A:1009744605920
Banayo, N. P., Haefele, S. M., Desamero, N. V., and Kato, Y. (2018). On-farm assessment of site-specific nutrient management for rainfed lowland rice in the Philippines. Field Crops Res. 220, 88–96. doi: 10.1016/j.fcr.2017.09.011
Biradar, D., Aladakatti, Y., Rao, T., and Tiwari, K. (2006). Site-specific nutrient management for maximization of crop yields in Northern Karnataka. Better Crops 90, 33–35.
Budhathoki, S., Amgain, L. P., Subedi, S., Iqbal, M., Shrestha, N., and Aryal, S. (2018). Assessing growth, productivity and profitability of drought tolerant rice using nutrient expert-rice and other precision fertilizer management practices in Lamjung, Nepal. Acta Sci. Agricul. 2, 153–158.
Bunquin, M. A. B., Tandy, S., Beebout, S. J., and Schulin, R. (2017). Influence of soil properties on zinc solubility dynamics under different redox conditions in non-calcareous soils. Pedosphere 27, 96–105. doi: 10.1016/S1002-0160(17)60299-6
Buresh, R. J., Castillo, R., van den Berg, M., and Gabinete, G. (2014). Nutrient Management Decision Tool for Small-Scale Rice and Maize Farmers. Technical Bulletin 190. Taipei, Taiwan: Food and Fertilizer Technology Center.
Buresh, R. J., Castillo, R. L., Torre, J. C. D., Laureles, E. V., Samson, M. I., Sinohin, P. J., et al. (2019). Site-specific nutrient management for rice in the Philippines: calculation of field-specific fertilizer requirements by Rice Crop Manager. Field Crops Res. 239, 56–70. doi: 10.1016/j.fcr.2019.05.013
Buresh, R. J., Pampolino, M. F., and Witt, C. (2010). Field-specific potassium and phosphorus balances and fertilizer requirements for irrigated rice-based cropping systems. Plant Soil 335, 35–64. doi: 10.1007/s11104-010-0441-z
Cakmak, I. (2002). Plant nutrition research: Priorities to meet human needs for food in sustainable ways. Plant Soil 247, 3–24. doi: 10.1023/A:1021194511492
Cassman, K. G., and Pingali, P. L. (1995). “Extrapolating trends from long-term experiments to farmers' fields: the case of irrigated rice in Asia,” in Agricultural Sustainability in Economic, Environmental, and Statistical Terms, eds V. Barnett, R. Payne, R. Steiner (London: Wiley), 64–84.
Chen, X., Cui, Z., Fan, M., Vitousek, P., Zhao, M., Ma, W., et al. (2014). Producing more grain with lower environmental costs. Nature 514:486. doi: 10.1038/nature13609
Dawe, D., and Dobermann, A. (1999). De®ning productivity and yield. IRRI Discussion Papers Series No. 33. Makati City, Philippines: International Rice Research Institute.
Dixit, S., Singh, U. M., Abbai, R., Ram, T., Singh, V. K., Paul, A., et al. (2019). Identification of genomic region(s) responsible for high iron and zinc content in rice. Sci. Rep. 9:8136. doi: 10.1038/s41598-019-43888-y
Dobermann, A. (2000). “Future intensification of irrigated rice systems,” in Redesigning Rice Photosynthesis to Increase Yield, eds J. E. Sheehy, P. L. Mitchell, B. Hardy (Makati City, Philippines/Amsterdam: International Rice Research Institute/Elsevier), 229–247.
Dobermann, A. (2007). “Nutrient use efficiency–measurement and management,” in Fertilizer Best Management Practices: General Principles, Strategy for Their Adoption and Voluntary Initiatives Versus Regulations (Paris: International Fertilizer Industry Association), 1–28.
Dobermann, A., Witt, C., and Dawe, D. (2004). Increasing the Productivity of Intensive Rice Systems Through Site-Specific Nutrient Management. Los Baños, Philippines: Science Publishers, Inc., Enfield, NH, USA and Int. Rice Res. Inst.
Dobermann, A., Witt, C., Dawe, D., Abdulrachman, S., Gines, H., Nagarajan, R., et al. (2002). Site-specific nutrient management for intensive rice cropping systems in Asia. Field Crops Res. 74, 37–66. doi: 10.1016/S0378-4290(01)00197-6
Dobermann, A. R. (2005). Nitrogen Use Efficiency-State of the Art. Lincoln, NE: Agronomy–Faculty Publications; University of Nebraska. 316. Available online at: https://digitalcommons.unl.edu/agronomyfacpub/316
Duy, P. Q., Abe, A., Hirano, M., Sagawa, S., and Kuroda, E. (2004). Analysis of lodging-resistant characteristics of different rice genotypes grown under the standard and nitrogen-free basal dressing accompanied with sparse planting density practices. Plant Prod. Sci. 7, 243–251. doi: 10.1626/pps.7.243
Edmonds, D. E., Abreu, S. L., West, A., Caasi, D. R., Conley, T. O., Daft, M. C., et al. (2009). Cereal nitrogen use efficiency in Sub Saharan Africa. J. Plant Nutr. 32, 2107–2122. doi: 10.1080/01904160903308184
FAOSTAT (2019). Available online at: http://www.fao.org/faostat/en/#data (accessed November 7, 2019).
Florey, C., Hellin, J., and Balié, J. (2020). Digital agriculture and pathways out of poverty: the need for appropriate design, targeting, and scaling. Enterprise Dev. Microfin. 31, 126–140. doi: 10.3362/1755-1986.20-00007
Galloway, J. N., Aber, J. D., Erisman, J. W., Seitzinger, S. P., Howarth, R. W., Cowling, E. B., et al. (2003). The nitrogen cascade. Bioscience 53, 341–356. doi: 10.1641/0006-3568(2003)053[0341:TNC]2.0.CO;2
Gines, H., Redondo, G., Estigoy, A., and Dobermann, A. (2004). “Site-specific nutrient management in irrigated rice systems of Central Luzon, Philippines,” in Increasing Productivity of Intensive Rice Systems Through Site-Specific Nutrient Management (Los Bañ~os: Science Publishers, Inc.), 145–169.
GRiSP, G. R. S. P. (2013). Rice almanac. 4th Edn. Los Baños, Philippines: International Rice Research Institute. 283p.
Gupta, G., Shrestha, A., Shrestha, A., and Amgain, L. P. (2016). Evaluation of different nutrient management practice in yield and growth in rice in Morang district. Adv. Plants Agric. Res. 3, 187–191. doi: 10.15406/apar.2016.03.00119
Hakoomat, A. L. I., Zuhair, H., Ahmad Naeem, S., Naeem, S., Muhammad, K. Q., Shazia, K., et al. (2014). Nitrogen and Zinc Interaction Improves Yield and Quality of Submerged Basmati Rice (Oryza sativa L.). Notulae Botanicae Horti Agrobotanici Cluj-Napoca 42, 372–379. doi: 10.15835/nbha4229469
Heffer, P., Gruere, A., and Roberts, T. (2017). Assessment of Fertilizer Use by Crop at the Global Level. International Fertilizer Association (IFA) and International Plant Nutrition Institute (IPNI), 2014-2014/5, 20p. Paris.
Hu, R., Cao, J., Huang, J., Peng, S., Huang, J., Zhong, X., et al. (2007). Farmer participatory testing of standard and modified site-specific nitrogen management for irrigated rice in China. Agric. Syst. 94, 331–340. doi: 10.1016/j.agsy.2006.10.002
Islam, Z., Bagchi, B., and Hossain, M. (2007). Adoption of leaf color chart for nitrogen use efficiency in rice: impact assessment of a farmer-participatory experiment in West Bengal, India. Field Crops Res. 103, 70–75. doi: 10.1016/j.fcr.2007.04.012
Janssen, B. H., Guiking, F., Van der Eijk, D., Smaling, E., Wolf, J., and Van Reuler, H. (1990). A system for quantitative evaluation of the fertility of tropical soils (QUEFTS). Geoderma 46, 299–318. doi: 10.1016/0016-7061(90)90021-Z
Kanter, D. R., Bell, A. R., and McDermid, S. S. (2019). Precision agriculture for smallholder nitrogen management. One Earth 1, 281–284. doi: 10.1016/j.oneear.2019.10.015
Khuong, T. Q., Thi, T., Huan, N., Tan, P., and Buresh, R. J. (2007). Effect of site specific nutrient management on grain yield, nutrient use efficiency and rice production profit in the Mekong Delta. Omonrice 158, 153–158.
Khurana, H. S., Phillips, S. B., Dobermann, A., Sidhu, A. S., and Peng, S. (2007). Performance of site-specific nutrient management for irrigated, transplanted rice in northwest India. Agron. J. 99, 1436–1447. doi: 10.2134/agronj2006.0283
Khurana, H. S., Sidhu, A., Singh, B., and Singh, Y. (2009). “Performance of site-specific nutrient management in a rice-wheat cropping system,” in The Proceedings of the International Plant Nutrition Colloqium XVI, UC Davis. Available online at: https://escholarship.org/uc/item/870498gc (accessed June 17, 2020).
Kumar, V., Jat, H. S., Sharma, P. C., Gathala, M. K., Malik, R. K., Kamboj, B. R., et al. (2018). Can productivity and profitability be enhanced in intensively managed cereal systems while reducing the environmental footprint of production? Assessing sustainable intensification options in the breadbasket of India. Agric. Ecosyst. Environ. 252, 132–147. doi: 10.1016/j.agee.2017.10.006
Ladha, J. K., Pathak, H. J, Krupnik, T., Six, J., and van Kessel, C. (2005). Efficiency of fertilizer nitrogen in cereal production: retrospects and prospects. Adv. Agron. 87, 85–156. doi: 10.1016/S0065-2113(05)87003-8
Lal, R., Brevik, E. C., Dawson, L., Field, D., Glaser, B., Hartemink, A. E., et al. (2020). Managing soils for recovering from the COVID-19 pandemic. Soil Syst. 4:46. doi: 10.3390/soilsystems4030046
Liu, H., Won, P. L., Banayo, N. P., Nie, L., Peng, S., and Kato, Y. (2019). Late-season nitrogen applications improve grain yield and fertilizer-use efficiency of dry direct-seeded rice in the tropics. Field Crops Res. 233, 114–120. doi: 10.1016/j.fcr.2019.01.010
Mandal, M. K., Dutta, S., Majumdar, K., Satyanarayana, T., Pampolino, M., Govil, V., et al. (2015). Enhancing Rice Yield, Profitability, and Phosphorus Use Efficiency in West Bengal Using the Nutrient Expert® Fertilizer Decision Support Tool. Better Crops–SouthAsia, 12–14.
Marahatta, S. (2017). Increasing productivity of an intensive rice based system through site specific nutrient management in Western Terai of Nepal. J. Agric. Environ. 18, 140–150. doi: 10.3126/aej.v18i0.19899
Nagarajan, R., Ramanathan, S., Muthukrishnan, P., Stalin, P., Ravi, V., Babu, M., et al. (2004). “Site-specific nutrient management in irrigated rice systems of Tamil Nadu, India,” in Increasing Productivity of Intensive Rice Systems Through Site-Specific Nutrient Management (Los Baños: Science Publishers, Inc.), 101–123.
Omara, P., Aula, L., Oyebiyi, F., and Raun, W. R. (2019). World cereal nitrogen use efficiency trends: review and current knowledge. Agrosyst. Geosci. Environ. 2, 1–8. doi: 10.2134/age2018.10.0045
Palanog, A. D., Calayugan, M. I. C., Descalsota-Empleo, G. I., Amparado, A., Inabangan-Asilo, M. A., Arocena, E. C., et al. (2019). Zinc and iron nutrition status in the Philippines population and local soils. Front. Nutr. 6:81. doi: 10.3389/fnut.2019.00081
Pampolino, M. (2016). Optimising Fertilizer Formulations for Smallholders in Asia and Africa. Fertilizer Focus, 42–45. Available online at: http://seap.ipni.net/article/SEAP-3202
Pampolino, M. F., Manguiat, I. J., Ramanathan, S., Gines, H., Tan, P., Chi, T., et al. (2007). Environmental impact and economic benefits of site-specific nutrient management (SSNM) in irrigated rice systems. Agric. Syst. 93, 1–24. doi: 10.1016/j.agsy.2006.04.002
Pampolino, M. F., Witt, C., Pasuquin, J. M., Johnston, A., and Fisher, M. J. (2012). Development approach and evaluation of the Nutrient Expert software for nutrient management in cereal crops. Comput. Electron. Agric. 88, 103–110. doi: 10.1016/j.compag.2012.07.007
Peng, S., Buresh, R. J., Huang, J., Yang, J., Zou, Y., Zhong, X., et al. (2006). Strategies for overcoming low agronomic nitrogen use efficiency in irrigated rice systems in China. Field Crops Res. 96, 37–47. doi: 10.1016/j.fcr.2005.05.004
Peng, S., Buresh, R. J., Huang, J., Zhong, X., Zou, Y., Yang, J., et al. (2010). Improving nitrogen fertilization in rice by sitespecific N management. A review. Agron. Sustain. Dev. 30, 649–656. doi: 10.1051/agro/2010002
Pingali, P. L. (2012). Green revolution: impacts, limits, and the path ahead. Proc. Natl. Acad. Sci. 109, 12302–12308. doi: 10.1073/pnas.0912953109
Qureshi, A., Singh, D. K., and Kumar, A. (2018). Climate Smart Nutrient Management (CSNM) for enhanced use efficiency and productivity in rice and wheat under rice-wheat cropping system. Int. J. Curr. Microbiol. Appl. Sci. 7, 4166–4176.
Rajendran, R., Stalin, P., Ramanathan, S., and Buresh, R. J. (2010). Site-Specific Nitrogen and Potassium Management for Irrigated Rice in the Cauvery Delta. Better Crops, 7–9.
Rubianes, F., Swamy, B. M., and Johnson-Beebout, S. (2018). Irrigation management risks and zn fertilization needs in zn biofortification breeding in lowland rice. Exp. Agric. 54:382. doi: 10.1017/S0014479717000084
Saito, K., Diack, S., Dieng, I., and N'Diaye, M. K. (2015). On-farm testing of a nutrient management decision-support tool for rice in the Senegal River valley. Comput. Electron. Agric. 116, 36–44. doi: 10.1016/j.compag.2015.06.008
Sapkota, T. B., Jat, M. L., Rana, D. S., Khatri-Chhetri, A., Jat, H. S., Bijarniya, D., et al. (2021). Crop nutrient management using Nutrient Expert improves yield, increases farmers' income and reduces greenhouse gas emissions. Sci. Rep. 11, 1–11. doi: 10.1038/s41598-020-79883-x
Sapkota, T. B., Majumdar, K., Jat, M. L., Kumar, A., Bishnoi, D. K., McDonald, A., et al. (2014). Precision nutrient management in conservation agriculture based wheat production of Northwest India: profitability, nutrient use efficiency and environmental footprint. Field Crops Res. 155, 233–244. doi: 10.1016/j.fcr.2013.09.001
Satawathananont, S., Chatuporn, S., Niyomvit, L., Kongchum, M., Sookthongsa, J., and Dobermann, A. (2004). “Site-specific nutrient management in irrigated rice systems of Central Thailand,” in Increasing Productivity of Intensive Rice Systems Through Site-Specific Nutrient Management (Los Baños: Science Publishers, Inc.), 125–143.
Segda, Z., Haefele, S. M., Wopereis, M. C. S., Sedogo, M. P., and Guinko, S. (2005). Combining field and simulation studies to improve fertilizer recommendations for irrigated rice in Burkina Faso. Agron. J. 97, 1429–1437. doi: 10.2134/agronj2004.0275
Sharma, S., Panneerselvam, P., Castillo, R. L., Manohar, S., Rajendran, R., Ravi, V., et al. (2019a). Web-based tool for calculating field-specific nutrient management for rice in India. Nutr. Cycling Agroecosyst. 113, 21–33. doi: 10.1007/s10705-018-9959-x
Sharma, S., Rout, R. K., Khanda, C. M., Tripathi, R., Shahid, M., Nayak, A., et al. (2019b). Field-specific nutrient management using Rice Crop Manager decision support tool in Odisha, India. Field Crops Res. 241:107578. doi: 10.1016/j.fcr.2019.107578
Shaviv, A., and Mikkelsen, R. L. (1993). Controlled-release fertilizers to increase efficiency of nutrient use and minimize environmental degradation - A review. Fertilizer Res. 35, 1–12. doi: 10.1007/BF00750215
Singh, B. (2014). Site specific and need based management of nitrogen fertilizers in cereals in India. Adv. Fertilizer Technol. Biofertilizers 2, 576–605. Available online at: http://krishi.icar.gov.in/jspui/handle/123456789/15279
Singh, B., Singh, Y., Ladha, J. K., Bronson, K. F., Balasubramanian, V., Singh, J., et al. (2002). Chlorophyll meter– and leaf color chart–based nitrogen management for rice and wheat in Northwestern India. Agron. J. 94, 821–829. doi: 10.2134/agronj2002.8210
Singh, V. K., Shukla, A. K., Singh, M. P., Majumdar, K., Mishra, R. P., Rani, M., et al. (2015). Effect of site-specific nutrient management on yield, profit and apparent nutrient balance under pre-dominant cropping systems of Upper Gangetic Plains. Indian J. Agric. Sci. 85, 335–343.
Son, T. T., Van Chien, N., Thoa, V. T. K., Dobermann, A., and Witt, C. (2004). “Site-specific nutrient management in irrigated rice systems of the Red River Delta of Vietnam,” in Increasing Productivity of Intensive Rice Systems Through Site-Specific Nutrient Management (Los Baños: Science Publishers, Inc.), 217–242.
Srivastava, P., Balhara, M., and Giri, B. (2020). “Soil health in India: past history and future perspective,” in Vol 58, Soil Health, Soil Biology Book Series (Springer), 1–19.
SRP (2019). The SRP Standard for Sustainable Rice Cultivation (Version 2.0), Sustainable Rice Platform. Bangkok. Available online at: http://www.sustainablerice.org (accessed November 20, 2019).
Stuart, A. M., Pame, A. R. P., Silva, J. V., Dikitanan, R. C., Rutsaert, P., Malabayabas, A. J. B., et al. (2016). Yield gaps in rice-based farming systems: Insights from local studies and prospects for future analysis. Field Crops Res. 194, 43–56. doi: 10.1016/j.fcr.2016.04.039
Tan, P. S., Tuyen, T. Q., Huan, T. T. N., Khuong, T. Q., Hoai, N. T., Diep, L. N., et al. (2004). “Site-specific nutrient management in irrigated rice systems of the Mekong Delta of Vietnam,” in Increasing Productivity of Intensive Rice Systems Through Site-Specific Nutrient Management (Los Baños: Science Publishers, Inc.), 193–215.
Tilman, D., Cassman, K. G., Matson, P. A., Naylor, R., and Polasky, S. (2002). Agricultural sustainability and intensive production practices. Nature 418:671. doi: 10.1038/nature01014
Van Hach, C., and Tan, P. S. (2007). Study on site-specific nutrient management (SSNM) for high-yielding rice in the Mekong Delta. Omonrice 15, 144–152.
Varinderpal, S., Singh, B., Singh, Y., Thind, H. S., and Gupta, R. K. (2010). Need based nitrogen management using the chlorophyll meter and leaf colour chart in rice and wheat in South Asia: a review. Nutr. Cycling Agroecosyst. 88, 361–380. doi: 10.1007/s10705-010-9363-7
Wang, G., Dobermann, A., Witt, C., Sun, Q., and Fu, R. (2001). Performance of site-specific nutrient management for irrigated rice in southeast China. Agron. J. 93, 869–878. doi: 10.2134/agronj2001.934869x
Wang, G., Sun, Q., Fu, R., Huang, X., Ding, X., Wu, J., et al. (2004). “Site-specific nutrient management in irrigated rice systems of Zhejiang Province, China,” in Increasing Productivity of Intensive Rice Systems Through Site-Specific Nutrient Management (Science Publishers, Inc.), 243–263.
Wang, G., Zhang, Q., Witt, C., and Buresh, R. (2007). Opportunities for yield increases and environmental benefits through site-specific nutrient management in rice systems of Zhejiang province, China. Agric. Syst. 94, 801–806. doi: 10.1016/j.agsy.2006.11.006
Wang, Y., Li, C., Li, Y., Zhu, L., Liu, S., Yan, L., et al. (2020). Agronomic and environmental benefits of nutrient expert on maize and rice in Northeast China. Environ. Sci. Pollut. Res. Int. 27, 28053–28065. doi: 10.1007/s11356-020-09153-w
Witt, C, and A. Dobermann, (2004). “17 toward a decision support system for site-specific nutrient management.” in Increasing Productivity of Intensive Rice Systems Through Site Rice Systems Through Site-Specific Nutrient Management (Los Baños: Science Publishers, Inc.), 359–395.
Witt, C., Pasuquin, E., Mutters, R., and Buresh, R. (2005). New leaf color chart for effective nitrogen management in rice. Better Crops 89, 36–39.
Xu, G., Fan, X., and Miller, A. J. (2012). Plant nitrogen assimilation and use efficiency. Annu. Rev. Plant Biol. 63, 153–182. doi: 10.1146/annurev-arplant-042811-105532
Xu, X., He, P., Yang, F., Ma, J., Pampolino, M. F., Johnston, A. M., et al. (2017a). Methodology of fertilizer recommendation based on yield response and agronomic efficiency for rice in China. Field Crops Res. 206, 33–42. doi: 10.1016/j.fcr.2017.02.011
Xu, X., He, P., Zhang, J., Pampolino, M. F., Johnston, A. M., and Zhou, W. (2017b). Spatial variation of attainable yield and fertilizer requirements for maize at the regional scale in China. Field Crops Res. 203, 8–15. doi: 10.1016/j.fcr.2016.11.013
Xu, Y., Nie, L., Buresh, R. J., Huang, J., Cui, K., Xu, B., et al. (2010). Agronomic performance of late-season rice under different tillage, straw, and nitrogen management. Field Crops Res. 115, 79–84. doi: 10.1016/j.fcr.2009.10.005
Yang, F., Xu, X., Ma, J., He, P., Pampolino, M., and Zhou, W. (2017). Experimental validation of a new approach for rice fertiliser recommendations across smallholder farms in China. Soil Res. 55, 579–589. doi: 10.1071/SR16328
Zhang, J. J., He, P., Xu, X. P., Ding, W. C., Ullah, S., Wang, Y. L., et al. (2018). Nutrient expert improves nitrogen efficiency and environmental benefits for winter wheat in China. Agron. J. 110, 696–706. doi: 10.2134/agronj2017.05.0291
Zhang, W.-f., Dou, Z.-x., He, P., Ju, X.-T., Powlson, D., Chadwick, D., et al. (2013). New technologies reduce greenhouse gas emissions from nitrogenous fertilizer in China. Proc. Natl. Acad. Sci. 110:8375. doi: 10.1073/pnas.1210447110
Keywords: precision nutrient management, sustainability, rice agri-food systems, digital tools, profitability
Citation: Chivenge P, Sharma S, Bunquin MA and Hellin J (2021) Improving Nitrogen Use Efficiency—A Key for Sustainable Rice Production Systems. Front. Sustain. Food Syst. 5:737412. doi: 10.3389/fsufs.2021.737412
Received: 06 July 2021; Accepted: 29 September 2021;
Published: 02 November 2021.
Edited by:
Sudhakar Srivastava, Banaras Hindu University, IndiaReviewed by:
Sudarshan Kumar Dutta, Mohammed VI Polytechnic University, MoroccoShiveshwar Pratap Singh, Dr. Rajendra Prasad Central Agricultural University, India
Copyright © 2021 Chivenge, Sharma, Bunquin and Hellin. This is an open-access article distributed under the terms of the Creative Commons Attribution License (CC BY). The use, distribution or reproduction in other forums is permitted, provided the original author(s) and the copyright owner(s) are credited and that the original publication in this journal is cited, in accordance with accepted academic practice. No use, distribution or reproduction is permitted which does not comply with these terms.
*Correspondence: Pauline Chivenge, cC5jaGl2ZW5nZUBhcG5pLm5ldA==