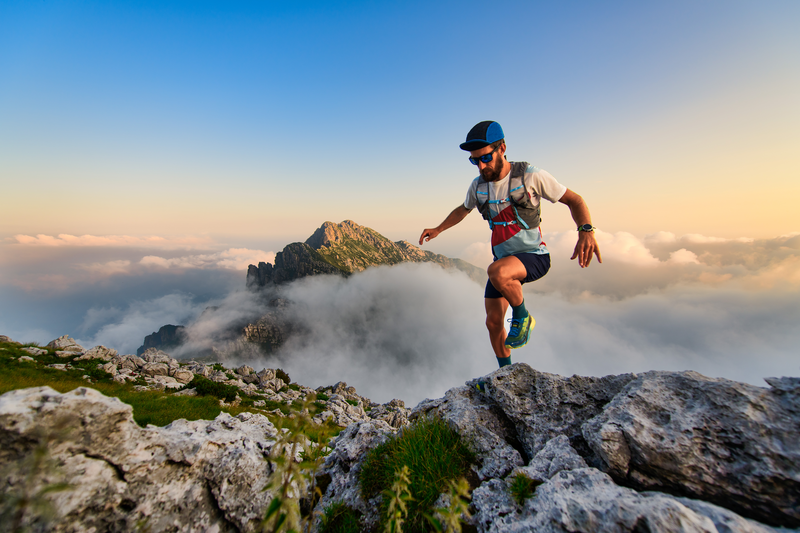
94% of researchers rate our articles as excellent or good
Learn more about the work of our research integrity team to safeguard the quality of each article we publish.
Find out more
REVIEW article
Front. Sustain. Food Syst. , 26 October 2021
Sec. Sustainable Food Processing
Volume 5 - 2021 | https://doi.org/10.3389/fsufs.2021.734921
Nutraceuticals, functional foods, immunity boosters, microcapsules, nanoemulsions, edible packaging, and safe food are the new progressive terms, adopted to describe the food industry. Also, the rising awareness among the consumers regarding these has created an opportunity for the food manufacturers and scientists worldwide to use food as a delivery vehicle. Packaging performs a very imminent role in the food supply chain as well as it is a consequential part of the process of food manufacturing. Edible packaging is a swiftly emerging art of science in which edible biopolymers like lipids, polysaccharides, proteins, resins, etc. and other consumable constituents extracted from various non-conventional sources like microorganisms are used alone or imbibed together. These edible packaging are indispensable and are meant to be consumed with the food. This shift in paradigm from traditional food packaging to edible, environment friendly, delivery vehicles for bioactive compounds have opened new avenues for the packaging industry. Bioactive compounds imbibed in food systems are gradually degenerated, or may change their properties due to internal or external factors like oxidation reactions, or they may react with each other thus reducing their bioavailability and ultimately may result in unacceptable color or flavor. A combination of novel edible food-packaging material and innovative technologies can serve as an excellent medium to control the bioavailability of these compounds in food matrices. One promising technology for overcoming the aforesaid problems is encapsulation. It can be used as a method for entrapment of desirable flavors, probiotics, or other additives in order to apprehend the impediments of the conventional edible packaging. This review explains the concept of encapsulation by exploring various encapsulating materials and their potential role in augmenting the performance of edible coatings/films. The techniques, characteristics, applications, scope, and thrust areas for research in encapsulation are discussed in detail with focus on development of sustainable edible packaging.
The food industry and allied sectors like food-packaging industry and nutraceutical industry, face complex challenges on a regular basis due to change in production practices and consumer preferences. The end consumer is more conversant about the food profile and even more apprehensive about the farm to fork journey of the component that he/she is about to consume. Additionally, an increase in global trading of food is leading the food technologists to produce food products of high quality and safety. There is a need for circular food supply chains which are transparent and interconnected in nature such that the waste from one industry can help in the value addition of another product in a different food industry (Lavelli, 2021). The concepts like green foods with bioactive components that bestow numerous health benefits lure the consumers (Rostamabadi et al., 2021). Entrapment of bioactive substances in food with controlled release strategy is one such area where the scientific fraternity is working diligently to ensure food products with abundant health benefits. One of the canonical concepts to achieve the same is encapsulation and techniques like nanoemulsions (Jafari et al., 2017) and liposome technology (Ajeeshkumar et al., 2021) are used to encapsulate desired materials in food products.
Encapsulation is gaining popularity for use not only in food products but in edible films and coatings as well, which are a sustainable alternative to the conventional food-packaging systems. These days, attempts are being made to improve the sustainable packaging system by adding bioactive compounds and probiotics, which in return enhance the nutritive value, functionality, and overall performance of the edible films. Bioactives in encapsulated films not only improve the quality of edible film but also maneuver the food product quality parameters like shelf life, rate of lipid peroxidation, browning index, bactericidal/bacteriostatic effects, and flavor profile (Díaz-Montes and Castro-Muñoz, 2021).
Bioactive compounds exhibiting antioxidant and antimicrobial properties form the main category of materials that are added to edible films. Polyphenols (like tannins, anthocyanins, flavonoids, phenolic acids), volatile components in essential oils (Zhang W. et al., 2019) (like cinnamon, lemon, oregano, thyme oil, clove oil, etc.), natural pigments, vitamins, polypeptides, and nutraceuticals are the common substances that form the core material of the encapsulated edible films (Chen et al., 2021).
Encapsulation is a superior technology as it overcomes the disadvantages of incorporating bioactive ingredients as food additives. Encapsulation improves heat stability and solubility and offers UV protection and ability of controlled release (Quirós-Sauceda et al., 2014). Encapsulation matrix serves as a perfect vehicle for the delivery of antimicrobials, antioxidants, probiotics, and flavor compounds. Additionally, it gives a better chance at ensuring food safety and sensory acceptance although one needs to ensure minimum damage to the natural flavor of the food that is packaged in the same (Tripathi et al., 2021).
The conventional approach of edible film formation simply requires the addition of the bioactive ingredient to the film-forming solution, followed by casting of the film (Rodríguez et al., 2020). The alternative approach is to encapsulate the active ingredient with colloidal particles prior to mixing with the film-forming materials (Muhammad et al., 2020). This review discusses the latter in detail with focus on understanding the concept of encapsulation, role of colloidal particles, methods of encapsulation, and their impact on the future of sustainable food-packaging industry.
Encapsulation in edible films can be defined as the entrapment of one ingredient (usually bioactive) within another ingredient where the former is identified as core material and the later as wall material (Liu et al., 2021). Film-forming material prepared by encapsulation can be categorized into two groups on the basis of their dimension size, namely, microencapsulation and nanoencapsulation (Figure 1). The microemulsions have a diameter of 3 to 800 μm, whereas the nanoemulsions have a particle size ranging from 10 to 1,000 nm (1 μm) (Ye and Chi, 2018).
Figure 1. (A,B) Encapsulation systems and their structures: microencapsulation and nanoencapsulation (Adapted from Delshadi et al., 2021).
Furthermore, encapsulation technology can be grouped into two broad groups, i.e., true encapsulation where a liquid core is entrapped in a gelatinous capsule. The other one includes all the recent techniques where active ingredients are entrapped in the matrix encapsulant or wall material made up of various carriers (El-Kader and Abu Hashish, 2020).
The success of an effective encapsulation process depends upon selection of three factors, namely, target bioactive molecules, wall materials, and a suitable encapsulation method (Rostamabadi et al., 2021). The encapsulated materials in edible coatings can either function as releasing systems (antimicrobials, antioxidants, CO2 emitters, Ethanol emitters, enzymes, etc.) or as absorbing systems (O2 scavengers, CO2 scavengers, ethylene scavengers, moisture absorbents, aroma absorbents) depending upon the final application (Almasi et al., 2020).
Enhanced functionality of the edible film due to encapsulation technology can be attributed to the entrapment of active ingredient in the wall materials (solid, liquid, or gas) which form the matrix (Boostani and Jafari, 2021). It can be exploited as means of active packaging if incorporated in edible films where encapsulant is the matrix material and bioactive is the functional component.
Controlled release packaging (CRP) is a particular type of slow-release technology where the bioactive is discharged via edible film, into the food product over a period of time in a controlled mode. Deployment of controlled release ensures effective and long-lasting action of bioactives and in desired concentration on the surface of food (Almasi et al., 2020). Additionally, restricted release of bioactives in edible films endorses reproducibility, maneuverability, and predictability of rate of release (Chen et al., 2019). CRP is finalized after taking into consideration all the factors like agent to be encapsulated and its release kinetics, initiation and duration of release, mechanism and trigger of release, quantity, and speed of release into the food product. A fast rate of release would be ineffective in extending shelf life of food products and a very slow rate would fail to check growth of microorganisms or autolytic alterations in the edible film or food product.
It becomes important to have comprehensive knowledge of the release kinetics of the encapsulated active substances in controlled released edible films. Some of the release mechanisms that help in setting the bioactive free into the desired product are depicted in Figure 2 and explained below (McClements, 2019).
Figure 2. Mechanisms for the release of active ingredient from the edible films/coatings into the desired food product (Adapted from Boostani and Jafari, 2021).
It is a method of releasing bioactive materials by dissolving in the surroundings or food medium. Either the particle can be directly released into the surrounding or it dissolves into the matrix first, and then is released in the food product when the entire matrix dissolves. Dissolution begins at the surface and is followed by dissolving the inner framework of the nanoemulsion.
As the solvent particles are absorbed by the core (bioactive) material, the entire structure swells and bioactive is released when the structural integrity is no longer maintained. The release is related to an increase in the internal pore size of the matrix which becomes greater than the size of the bioactive, causing its release.
It is an erosive process where release is a function of the degrading forces. Numerous factors like pH, temperature, strong acids or bases, degrading enzymes, physiochemical processes, etc. lead to release of the bioactive material from the encapsulation matrix.
The bioactive material is released from the encapsulation matrix into the food product or surroundings. The rate of diffusion is dependent upon the physicochemical nature of the core and matrix materials and the gradient between the core and matrix.
It implies disruption of the encapsulation matrix such that the wall materials breakdown due to stress. The stress can be physical, chemical, or biological (enzymatic) in nature. The amount of bioactive released depends upon the fragments formed and as well as the nature and magnitude of applied stress.
Release profile is the cascade of events which result in liberation of bioactives from the wall material at the target site. Release mechanisms of the core material in the matrix affect the release profile of the bioactives. Figure 3 shows various release profiles plotted with time on x-axis and respective concentration of bioactives at y-axis. While designing encapsulated edible films, a thorough understanding of release profile of bioactives, would aid in an improved fabrication of the film-forming material. Release profiles can be adjusted or altered to improve the overall performance of the edible films. For instance, a release profile which is passive in nature can be modified into an active one, which is highly desirable in edible film applications where strong antimicrobial or antioxidant potential is required. Burst release, sustained release, controlled release, delayed release, triggered/ stimuli responsive release, and targeted release are some of the release profiles that unfetter the active ingredient at a preferred concentration and at an appropriate time (Boostani and Jafari, 2021).
Figure 3. Various release profiles plotted with time on x-axis and respective concentration of bioactives at y-axis (Adapted from Boostani and Jafari, 2021).
Encapsulation is a technique for coating bioactive compound(s) within a carrier material and is considered a useful tool for improving the delivery of active compounds as well as living cells in food products. Encapsulating an active principle meets the following main objectives:
1 Confinement or delayed release:
• Volatile conglomerates (e.g., aroma, flavor compounds)
• Steady bioprocessing (e.g., microflora, enzyme)
2 Safeguard/stabilization:
• Sustaining and conserving the bioactive additives from extrinsic environmental influences (e.g., oxygen, light, temperature, water, pH)
• Safeguard processors and end users from harsh chemicals and various enzymes (e.g., pesticide, soaps and detergents, proteases)
3 Sustained release (release at specific time and place, provoked by a relevant initiator):
• Diffusion, rehydration, degeneration, bursting
• Set off release under a particular condition like change in temperature, pH value, ionic strength, enzymatic hydrolysis, and so on
• Sustained release of bioactives like vitamins, flavor compounds, vitamins, etc. with explicit kinetics
4 Structuration/functionalization:
• Transformation of liquid or gas to solid
• Camouflage aroma, taste, or color
• Rheological and surface characteristics of powders
• Visual perspective as well as marketing conceptualization
Nature of the core material, structural and chemical characteristics of the coating materials, interactions between the core material, and the coating material as well as the working conditions of the technique employed govern the physiochemical characteristics of the microcapsule. Diverse encapsulation strategies have been researched relying upon the attributes of the core and the contemplated applications of the resulting goods. The complexity of these procedures varies. Enrobing a bioactive with an apt shell material is the simplest form of encapsulation employed in the food industry. It entails encapsulation of core material with help of fluidized bed or by spraying, succeeded by drying. Alternative approaches encompass emulsifying a core component (just as an enzyme or an oil molecule) along a suspension of wall material (typically a polycarbohydrates/protein or their synergy), followed by drying of the encapsulated compound. Encapsulation techniques on a broader view could be prorated into three primary classes viz. physical, physicochemical, and chemical. Hence, it is critical to summarize the information pertaining to various encapsulation techniques; their basic principle, nature of the core material, particle size, merits, and demerits are tabulated below (Table 1).
Table 1. Overview of encapsulation techniques, basic principle, nature of the core material, particle size, merits, and demerits.
Sensations alluded by the interaction of taste, odor, and texture are commonly referred to as flavor. In general, flavor compounds can be grouped under two classes: liable for taste and the one liable for odor or aroma. Flavor and aroma may be the inherent property of the food; it may evolve at the time of processing or may be supplemented intentionally during the process to meet customer satisfaction. Aroma and flavor compounds are volatile molecules that can be perceived by olfactory receptors. These are responsible for the fabrication of flavorings thus imparting flavor and also help in enhancing the appeal of the product. Hence, flavor and aroma are organic compounds with low molecular weight, volatile in nature, vulnerable to heat, light, pH, acid, enzymes, and moisture (Fuciños et al., 2017). For the retention of flavor and aroma compounds and to minimize degradation of flavor, encapsulation technique is employed in the food-processing industry (Dubey and Dubey, 2020).
Ten aroma compounds were encapsulated in ι-carrageenan emulsion-based edible film, deducing that these films performed better with polar aroma compounds. It was also hypothesized that carrageenan edible films were apt to uphold the volatile aroma compounds amid the film-forming procedure and sustained release later (Marcuzzo et al., 2010). A very interesting research by Gong et al. (2020) revealed that heat stability of cinnamon flavor could be enhanced by ultrasonication-assisted microencapsulation using chitosan and pectin polymers thereby reducing the interaction of cinnamaldehyde with the yeast during the baking process.
Edible packaging helps in achieving superior quality of products, helps in protection against biological, chemical, and physical deterioration. By applying edible coatings or films on food products, physical properties of food like strength can be enhanced, also helps in improving aggregation of particles and appearance. In addition, it creates a semipermeable barrier that protects the bioactive substance against moisture, oil, oxygen, microorganisms, and light thereby enhancing shelf life and ensuring food safety (Assadpour and Jafari, 2019a; Lopez-Polo et al., 2021). Edible packaging with antimicrobial components had led to the development of the hypothesis of active packaging. This helps in reduction, inhibition, or complete arrest of proliferation of microflora on the food surface. A number of essential oils from spices like cloves, cinnamon, coriander, etc.; vegetables such as garlic, onion, and citrus fruits having antimicrobial properties could be embodied into edible packaging material to aid the purpose.
In recent years, more thrust is being imparted into developing potent, persistent, and natural antimicrobial compounds by enhancing the efficacy of the integrated antimicrobial agent. More potent antimicrobial substances of natural origin, like bacteriophages, bacteriocins (Ge et al., 2017), and essential oils and their fractions (Evangelho et al., 2019) have been favored over synthetic antimicrobial compounds, and innovative encapsulating techniques are utilized for attaining the goal. Advantages of encapsulation include sustained deliverance and restrained release of active components in the food system. As a result, this technique can be implied to manage the movement of the bioactive components from the edible packaging. In addition to this, encapsulation ensures better interaction of lipophilic compounds with hydrophilic natural polymers of films, thereby ensuring the stability of active components during processing. Studies pertaining to the impregnation of antimicrobial components in edible packaging by using encapsulation techniques to improve the quality and safety of food products are summarized in Table 2.
Table 2. Highlights of studies pertaining to incorporation of encapsulated antimicrobial conglomerates in edible packaging.
The biggest challenge of the food industry in the food-processing industry is limited storage life, which may be caused by microbial contamination and oxidation reactions. Antioxidants are progressively significant food additives in the food-processing industry. The conventional role of antioxidants, as proposed by their name, is to prohibit the advancement of oxidative rancidity in high fat-containing foods, in particular fried food products, foods of animal origin, and dairy-based foods. Synthetic antioxidants like butylated hydroxytoluene (BHT), tertiary butyl hydroquinone (TBHQ), propyl gallate (PG), or else butylated hydroxyanisole (BHA) are utilized generally to prevent food from oxidation. During recent times, considering the increasing awareness among consumers and their demand for less use of chemicals and minimally processed foods, major consideration is being paid to find natural antioxidants. As a result, food products preserved with natural importance are getting popular with the masses.
Some of the important natural antioxidants are tocopherols, essential oils, volatile phenols, flavanols, organic acids, and so on. Addition of antioxidants directly to food has certain limitations like interaction of food components with antioxidants may neutralize it rendering it less effective thereby making food more prone to be unsafe. Going deep into the mechanism of lipid oxidation reaction, one may find that the majority of these reactions are initiated at the surface of food. Hence, efforts are being made by the food scientists to encapsulate the natural antioxidants and then imbibe them into edible packaging to complement the storage life by shielding food products from oxidation, oxidative rancidity, and color distortion. A more extensive compilation of works in which antioxidants were incorporated in edible films and coatings are shown in Table 3.
Table 3. Research findings related to the effect of encapsulated antioxidants in edible packaging on oxidative stability of food products.
There are a number of food components that cannot be incorporated into food products in their native form like carotenoids, omega 3 fatty acids, fat-soluble vitamins A, D, E, and K, minerals, probiotics, prebiotics, and conjugated linoleic acid therefore these must be encapsulated first into a suitable encapsulating matrix. Just adding additives to food products to boost nutritional value can jeopardize the sensorial characteristics of the product. Furthermore, they may degrade with the passage of time, and as a result, may lose their effectiveness or become toxic because of oxidation process (Grootveld et al., 2020; Khoshnoudi-Nia et al., 2020). These bioactives may also interact with other components of food thereby reducing their bioavailability. Hence, to preserve their stability, nutritional value, taste, and aroma and to provide endurance against processing conditions, encapsulation as well as viability encapsulation of nutraceuticals (Assadpour and Jafari, 2019b) in edible films is emerging as an excellent choice.
Food-grade surfactants and edible oils were utilized to develop stable oil-in-water edible nanoemulsions as conveyor of vitamin D3 for fortifying whole fat milk and was able to impart stability against particle growth and gravitational separation for 10 days (Golfomitsou et al., 2018). In another study, nanoparticle encapsulation of vitamin C by ionic gelation followed by preparation of nanocomposite film represented a decline in degradation of vitamin C thereby culminating in more than 30% storage of vitamin as compared with the control after 15 days of storage. Furthermore, on exposure to high temperature, 80% of the original concentration was retained (Nascimento et al., 2019). It was demonstrated that coating of fish oils by chitosan safeguarded the emulsified fish oils from the action of gastric enzymes, therefore ensuring their controlled release in the intestine (Chang et al., 2018). Xu et al. (2021) in one of his research experiments exhibited that pectin and xanthan gum (anionic polysaccharides) encouraged the digestion of fish oil which may be attributed to impediment to the flocculation of protein-coated fish oil droplets and hence increment in surface area for the action of lipase enzyme.
Diet has a significant effect on overall health. Nowadays, people are being more aware about their health, wellbeing, and consumption pattern of foods having probiotics. In order to confer health benefits and get the desired outcome from probiotics, a dosage of 108-109 viable cells is usually recommended. To confer its health benefits in modulating the immune system, the survival of probiotic strain is a key factor. It is quite challenging to meet the desirable dose of probiotics due to their sensitivity to the change in environmental conditions. Use of encapsulated probiotics in edible packaging is a promising approach which may help beneficial microbes survive longer in food products.
A study by Bekhit et al. (2018) revealed that hydroxypropyl methylcellulose films impregnated with encapsulated Lactococcus lactis subsp. Lactis showed effective inhibition against Listeria monocytogenes. Employment of crystalline nanocellulose, whey protein isolate, and inulin as wall material microencapsulated probiotic powder of Lactobacillus rhamnosus was prepared by employing freeze-drying technique and was later checked for their viability under simulated gastrointestinal conditions. It was endowed from the results that survivability of probiotic improved exceptionally (p < 0.05) (Maleki et al., 2020). Soukoulis et al. (2014) formulated prebioti- based edible films using inulin wheat dextrin, poly dextrose, and glucose oligosaccharides as a conveyor for encapsulating probiotic Lactobacillus rhamnosus GG. It was manifested that all the four prebiotics film helped in ameliorating the stability of the probiotic strain.
The knowledge of different physicochemical, mechanical, and other techno-functional properties of films is of scientific as well as technological importance owing to their wider industrial applications including the packaging and encapsulation of bioactive components. The incorporation of bioactive compounds/nutraceuticals in the edible film/coatings concomitantly modify the structure due to the physical and chemical interactions with different biopolymers present in matrix and hence structural, mechanical, optical properties are improved/altered (Malherbi et al., 2019; Nogueira I. F. et al., 2019; Chen et al., 2021). Studies report that some of the encapsulated bioactive components have potent antioxidant and antimicrobial properties which further result in the improvement of applicability of bioactive-encapsulated films/coating thereby improving the shelf stability of food systems (Benbettaïeb et al., 2019).
The optical properties are one of the important characteristics for commercial applicability of edible films. A film is supposed to be optically transparent in some circumstances and turbid or opaque in others. Furthermore, the film/coatings prepared should have the ability for selective transmission of light as it could ensure the shelf stability as well as quality of light-sensitive food ingredients and components viz, fats, carotenoids, vitamins, curcumin, etc. (Chen et al., 2021).
Refractive index, light scattering, particle size, and concentration of encapsulated bioactive material govern the optical properties of edible films. The refractive index of edible films refers to reflection or transmission of light through their surfaces. The higher the refractive index, the stronger is the reflection and lower is the transmission. As the solids content increases in films, the effective refractive index also increases. The clarity/opacity of the films is influenced by scattering and selective absorption of different light waves. Extent of light scattering depends on particle size, concentration, and relative refractive index of the incorporated bioactive ingredients into an edible film. When the particle concentration is low or when the particles have the same refractive index with respect to the surrounding film, the edible film may appear transparent, contrarily, it appears turbid or opaque when the particle concentration is high or the particles have a greatly different refractive index. When the particle size ranges from 200 to 2,000 nm, then the degree of light scattering reaches its maximum value. The edible film/coating is optically transparent when the particle size falls within the range of 40–50 nm. The selective absorption of light waves by the bioactive ingredients in edible films/coatings has great influence on determination of color. In many food systems where color is an important quality characteristic, adding natural pigments along with bioactive compounds, viz., carotenoids, curcumin and anthocyanins, while formulating the edible films/coatings can be controlled (Silva-Weiss et al., 2013; Chen et al., 2021). Anthocyanin-encapsulated maltodextrin edible film reported to form clear transparent edible film with lower opaqueness with insufficient protection against light (Stoll et al., 2016).
The incorporation of bioactive components alter the interactions among biopolymer molecules in edible films, which in turn affect the mechanical properties viz., tensile strength, elongation at break, Young's modulus, etc. (Liang et al., 2017). As a result, it is important to have discussion about characteristics, concentrations, and types of bioactive components affecting the mechanical properties of edible films. The primary factors for imparting varied mechanical properties to edible films are both intermolecular and intramolecular interactions between the components in the film-forming matrix (Basiak et al., 2017).
The mechanical characteristics of edible films are influenced by the biopolymer utilized. According to a research study, incorporating nisin into whey protein isolate (WPI) films dramatically enhanced tensile strength, which was ascribed to the ability of nisin to stimulate protein-protein interactions (Ko et al., 2001). Another study found that incorporating nisin into tapioca starch/hydroxypropyl methylcellulose edible films decreased breaking stress by 85%, which was ascribed to the plasticizer effect of nisin (Basch et al., 2013). Depending on their interaction with the biopolymer matrix, different kinds of essential oils have been found to have varied impacts on the mechanical characteristics of edible films (Shen and Kamdem, 2015). Clove oil, for example, is more plasticizing than cinnamon oil, resulting in chitosan/gum arabic films with lower tensile strength (Xu et al., 2019a).
The mechanical characteristics of edible films are also affected by the particle size of the active ingredient and the relative humidity of the environment. Smaller oil droplet sizes can help build a continuous network inside the biopolymer matrix, increasing interactions between biopolymers and resulting in edible films with greater tensile strengths (Xu et al., 2019b). High relative humidity leads biopolymers to absorb water and expand/swell, resulting in decreased tensile strength in sodium alginate films (Ruan et al., 2019).
The concentrations of bioactive compounds are also reported to have an impact upon mechanical properties of edible films/coatings. Decrease in tensile strength and increase in elongation at break was noticed in eugenol/corn starch/chitosan films as the eugenol content varied from 3 to 6% (w/w) (Zheng et al., 2019). This is because the intramolecular and intermolecular cross-links between eugenol, chitosan, and starch initially condensed the biopolymer structure; however, as the eugenol concentration increased, the tensile strength of the composite film decreased, possibly because the biopolymer network ruptured (Zheng et al., 2019). A similar phenomenon was observed in a study of citric acid/potato starch/chitosan films. As the concentration of citric acid increased from 5 to 15% (w/w), the tensile strength of the film increased gradually from 9.27 to 12.6 ± 1.0 MPa but further increase (up to 20%), resulting in the decrease in the tensile strength of the film. The reason could possibly be attributed to high citric acid concentrations, which serve as a plasticizer and diminish the interaction between macromolecules, resulting in a decrease in tensile strength of the edible film (Garavand et al., 2017; Wu et al., 2019).
Gelatin films prepared from anthocyanin-loaded nanocomplexes along with chitosan hydrochloride and chitosan carboxymethyl were reported to have improved tensile strength, elongation at break, thermal stability, and antioxidant properties in comparison with pure gelatin films without nanocomplexes. This improvement in properties of films might be ascribed to bonding between the hydroxyl groups and the polar groups of gelatin that resulted in uniform distribution of nanocomplexes within the gelatin network (Wang et al., 2019).
Furthermore, curcumin-encapsulated native and acetylated starch nanocomplexes (0.01 to 0.1%) were reported to enhance the tensile strength of aloe vera gel and banana starch-based edible films. Native starch nanoparticles in the films had numerous hydroxyl groups which resulted in the formation of strong hydrogen bonding within the film matrix that resulted in increased tensile strength. On the contrary, the acetylated starch nanoparticles had less number of hydroxyl groups due to acetylation of starch; therefore, these films had decreased/lower tensile strength and elongation at break. Additionally, curcumin is a strong hydrophobic molecule; it inhibits the interaction of water with the film matrix, thus, lowering water vapor permeability and solubility. These findings show that the film properties are strongly dependent on the nature and properties of encapsulated bioactive compounds, as well as the compatibility of both the encapsulating and film-forming materials with bioactive compounds (Nieto-Suaza et al., 2019).
The extent of moisture and gas permeation is a crucial functional property of the edible film/coatings. Incorporation/encapsulation of bioactive components having different functional groups like hydroxyl and carbonyl groups, give them both hydrophilic and hydrophobic characteristics. Therefore, when such bioactive components are added to film formulations, hydrophilicity and hydrophobicity affect the water transfer between the film and surrounding materials. Bioactive compounds make cross-linkages with the groups of biopolymers present in formulation and increase the compactness of the edible film structure, thereby affecting/reducing the rate of permeation of water vapor, oxygen, carbon dioxide, and other gases. For example, essential oils are hydrophobic bioactive ingredients that can reduce the penetration of water vapor by increasing the hydrophobicity of edible films (Sanchez-Gonzalez et al., 2011). However, in a study on cassava starch or cassava starch/hydroxypropyl methylcellulose (HPMC) films, it was found that the addition of nisin led to an increase in WVP, which may be due to the fact that nisin has a disruptive effect on the structure of the biopolymer matrix (Basch et al., 2013). On the other hand, Dammak et al. (2017) observed an increase in water vapor permeation of gelatin films loaded with rutin nanoemulsions.
Curcumin was encapsulated in nanohydrogels to the carboxymethyl cellulose (CMC) film-forming solutions. It was noticed that incorporation of encapsulated curcumin significantly altered the mechanical as well as functional properties of CMC films. Water vapor permeability was lowered by the presence of nanoencapsulated curcumin which might be due to change in density of film matrix that led to decreased diffusion of gases (Bourbon et al., 2021). On the same lines, another study conducted by Mirzaei-Mohkam et al. (2020) also reported decreased WVP values in the case of nanoencapsulated vitamin E in CMC films. The decrease was attributed to porosity caused by the nanocapsules which altered CMC film matrix.
Edible films incorporated with antimicrobial ingredients are important for preventing food deterioration thus ensuring safe food. Antimicrobial ingredients can be added both in free as well as encapsulated form. Antimicrobials are encapsulated to enhance their properties like dispersibility, compatibility, as well as stability in terms of better retention and release characteristics by maintaining concentration above the minimum inhibitory concentration (MIC) within the edible films. Furthermore, the efficacy of antimicrobial action of edible films depends upon types of spoilage microorganisms, film-forming materials used, and antimicrobial agents/compounds (Benbettaïeb et al., 2019). Adding bioactive ingredients to edible films can significantly improve their antimicrobial properties. Studies demonstrated that eugenol incorporated in chitosan/acorn starch (CS/AS) films exhibited significant inhibition action against Escherichia coli and Staphylococcus aureus (Zheng K. S. et al., 2019). As discussed above, encapsulation improves the efficacy of antimicrobial agents in edible films, but in few cases, contradictory findings are also reported. Cinnamon oil encapsulated within nanoliposomes was not able to enhance the antibacterial activity of the films, which might be attributed to conceivably less penetration of essential oil into the bacterial cell membranes (Wu et al., 2015). Akin to this finding, bioactive compound nisin encapsulated within liposomes was also reported to have placid inhibition action on food-borne pathogens in comparison with non-encapsulated/free nisin (Imran et al., 2012). Therefore, it can be concluded from the above studies that while researching the antimicrobial activity of encapsulated active compounds in edible films, it is critical to examine the homogenous distribution of bioactive compounds between the film and the bacteria because it will affect its minimum inhibitory concentration. Nanoemulsions combined with ground meat or fish products help to even distribution of bioactive components throughout the product, whereas nanoemulsions dipped or coated on the surface of meat or fish goods aid in preventing surface spoilage in meat or fish products (Das et al., 2020).
Edible coatings incorporated with oregano essential oil nanoemulsion applied on tomato surface were found to have significant inhibitory action on the growth of spoilage microbes. However, these nanoemulsion-based edible coatings also retained the other quality characteristics of the tomatoes stored at ambient temperature conditions for more than 2 weeks (Pirozzi et al., 2020). Alginate-based edible coating encapsulating lemongrass essential oil applied on fresh-cut Fuji apples prevented the activity of Escherichia coli (Salvia-Trujillo et al., 2015). Gelatin films loaded with curcumin nanoemulsions exposited significant antimicrobial activity against pathogenic bacteria—Salmonella typhimurium and Escherichia coli. The bioactive compounds found in different essential oils are known to have broad spectrum antimicrobial activity by destroying bacterial cell integrity, inhibiting the respiration and ion transport mechanisms ultimately causing death (Al-Tayyar et al., 2020).
Addition of antioxidants to edible films/coatings leads to increase in shelf stability of lipid-based foods, also aids in inhibition of oxidation and browning reactions, thus better retention of color as well as quality nutrients of foods (Bonilla et al., 2013). Generally, the antioxidant properties of a film depend upon encapsulated bioactive ingredient, film-forming material, encapsulation technique, and type of food system in which it is to be added. The addition of antioxidant components will alter the microstructure and mechanical characteristics of the film, which are dependent on the properties of the active ingredients and biopolymers, influencing the antioxidant capacity of edible film (De'Nobili et al., 2013).
Polyphenols, essential oils, tocopherols, carotenoids, flavonoids, etc. are known to possess excellent antioxidant properties (Tian et al., 2013). Antioxidants exhibit their action via different mechanisms, such as chelation of metals, singlet oxygen scavenging, inhibition of peroxide enzyme, scavenging free radicals, and their stabilization through hydrogen radical transfer. Therefore, selection of suitable bioactive ingredients with potent antioxidant activity is a crucial parameter to be taken into account while encapsulation in edible films for enhancing antioxidant properties (Gomez-Estaca et al., 2014). Studies report that the antioxidant property of edible films is directly proportional to the concentration of the bioactive compounds encapsulated (Norajit et al., 2010). Ellagic acid, an antioxidant, incorporated in edible films showed noticeable increase in antioxidant activity (from 0 to 28%) with increase in its concentration (from 0.5 to 5.0%) (Vilela et al., 2017). Akin to this study, the antioxidant efficacy of tannins and catechins incorporated sodium caseinate films was examined. The antioxidant property of tannic acid-encapsulated films was higher than that of catechin-encapsulated films, which was ascribed to variations in molecular weight, aromatic ring number, and hydroxyl radical substitution (Helal et al., 2012). Bioactive compounds with antioxidant characteristics and edible film/coating components should be compatible in order to obtain uniform antioxidant distribution and enhanced antioxidant retention and release in the edible film. Several research investigations have shown that edible films containing antioxidants can be used to extend the shelf life of foods that are vulnerable to oxidation (Stoll et al., 2016; Khan et al., 2020). Carboxymethyl xylan films formed with encapsulated licorice oil significantly improved shelf stability by inhibiting lipid oxidation and scavenging free radicals thus arresting the chain propagation of peroxide formation (Luis et al., 2019). Food-grade nanoemulsion loaded with bioactive compound, curcumin incorporated in gelatin-based edible films showed maximum free radical scavenging/antioxidant property thus improving the shelf life/quality of fresh broiler meat up to 17 days as compared with non-encapsulated gelatin films (Khan et al., 2020) Microbial growth in red beef during storage was significantly inhibited by alginate films containing encapsulated pineapple peel antioxidants (Lourenço et al., 2020).
A considerable amount of research available on encapsulation of bioactive ingredients deals with critical evaluation of different microencapsulation technologies along with their potential applications and limitations. There is still a substantial gap of knowledge between development of various microencapsulation technologies and their effective commercial application. No single method can be applied to all types of core material, and an individual design approach is required for different cores and different applications. Moreover, the food processing industry has to address a number of challenges pertaining to storability and stability of encapsulated bioactives.
Methods like spray drying which involve high temperatures might damage sensitive compounds like vitamin C, β-carotene, lycopene, anthocyanins, various colors, and flavors. In the dryers, dry particles may stick to the walls and not be recovered, which could lead to a lower product yield (Kanwate et al., 2019). Various economic, time aspects and factors such as type of wall material, heat sensitivity, and solubility of encapsulated bioactive compounds, presence of other constituents should be considered. To dehydrate heat-sensitive materials, freeze drying is an apt technique but requires a long process time and the need for reduced pressure condition, achieved by a vacuum pump results in higher cost due to increased energy consumption. Extrusion method of edible film formation limits the use of various polymers because of the ability to only process raw material blends that are low in moisture and can withstand high temperature. Also, cost of maintenance and requirement of specialized equipment adds to the economic aspect.
Among physicochemical methods, microencapsulation by lysosomes involves a series of complex posttreatment steps which might be tedious (Trucillo et al., 2018). There are other limitations such as a broad range of particle size distribution, poor physical and chemical stability, lipid oxidation, and improvement of the process at an acceptable cost level. Lysosome microencapsulation gives high availability bioactive compounds but decreased physical stability and chemical stability are a concern. Interfacial polymerization technique is limited by factors like altered biological activity of proteins during polymerization and less control over quality and yield of polymer membrane. Also, the use of organic solvents in the oil phase may lead to environmental pollution due to volatile organic compound emission (Xiao et al., 2018).
Type of edible film materials used is another important factor for microencapsulation. Polysaccharide-based films are hydrophilic in nature and thus are not good as water barriers, which might lead to dehydration (Dehghani et al., 2018). Protein coatings are also hydrophilic with little mechanical strength, thus limiting their application with preference to other polymers. Lipid-based films, although hydrophobic, might be prone to rancidity while their waxy texture and taste are also major problems (Jeevahan et al., 2017).
The economic aspect of microencapsulation is also an important factor to consider as the cost of materials, high capital, and operating cost make the formulation process more expensive than standard formulations. There may be difficulty in selection of appropriate technique and methodology since a lot of information on microencapsulation is still patented. Also, additional skill and knowledge required, difficulty to achieve uniform coating, problems with large-scale manufacturing, reduced shelf life, and inadequate stability of sensitive pharmaceuticals such as hygroscopic drugs are factors that limit the efficient use of microencapsulation technique.
Over the last decade, there has been a considerable increase in research and commercial interest in bioactive compounds having antimicrobial and antioxidant activity. To satisfy customer demands and provide a product that is free of pathogenic contaminants or without any changes in its organoleptic properties, new food preservation techniques such as encapsulation are being studied and new avenues are continuously being explored. Various food products such as meat, milk, eggs, yogurt drinks, peanut butter, various spreads, fruit juices, and bread are being incorporated with various bioactive ingredients due to soaring demand of functional foods such as probiotics, omega-3 fatty acids, vitamins, and phytochemicals.
In various industrial applications, the most commonly used microencapsulation method is spray-drying based, but new encapsulation methods such as electrospraying, complex coacervation, and inclusion complexes for various bioactive compounds are continually being explored with new knowledge added to the existing information field. Research on the complex coacervation technique has indicated a higher product yield and stability of the resulting product, but a major drawback remains in the limited availability of shell material. Gelatin has been the most prominent protein to be successfully used on a commercial scale using coacervation technique along with gum arabic. However, in the presence of polysaccharides, plant proteins have also been found to be capable of forming complex coacervates (Muhoza et al., 2020). Their lower cost, higher availability, and ecofriendly nature with other functional properties are also some of the factors that should encourage more research in this field. Various reports have suggested that complex coacervation microcapsules of essential oils can potentially control microbial growth in stored fresh and processed foods such as fruits, milk, vegetables, juice, and cakes.
Formation of inclusion complexes is recently being studied for release control of hydrophobic active compounds. Inclusion complexes are made up of a host and a guest molecule bonded by non-covalent interactions. The host cavity contains hydrophobic molecules or parts of molecules that, in the presence of water, are able to fit into the host cavity (Adel et al., 2019). This formation results in changes to the guest molecule solubility but without modifying it chemically. Cyclodextrin inclusion complex improves stability, antioxidant, antibacterial, and biological properties of active compounds (Tian et al., 2020). Electrospraying also has a lot of potential for food packaging and functional foods, since antioxidant and antimicrobial bioactive compounds can be integrated with electrosprayed particles with ease. Most commonly used natural polymers for electrospraying like zein, chitosan, and gelatin are relatively expensive and might lead to undesirable flavors, so testing new polymers which are preferably water-soluble and natural should be a priority. Electrospraying has low throughput, so commercialization of encapsulated compounds through electrospraying should also be explored.
Antimicrobial packaging is another avenue to be considered as the focus is shifting from the use of oil-derived, non-biodegradable and non-compostable synthetic antimicrobials to biodegradable, eco-friendly packaging material such as cellulose, chitosan, and zein are incorporated with compounds like plant extracts, essential oils, bacteriophages, and bacteriocins. Problems due to inherent volatilization of essential oils and other compounds decreased stability of naturally derived compounds due to proneness to degradation by light and heat which are the bases of the search for new strategies that focus on durability and efficiency of the new antimicrobial packaging materials. Focusing on surface-functionalized nanoparticles in the future studies to further boost the activity of antimicrobial agents can be explored. Most of the in vivo and in vitro studies have focused on bioavailability and release of encapsulation products, so a thorough evaluation of interaction of encapsulated antimicrobial compounds with other ingredients in formulations and assessment of their activity and release is required. Modification of organoleptic properties and texture of functional foods, boosting oxidative resistance of high-oil foods and preventing hindering microbial growth in food systems are various promising aspects that should be focused upon in the future.
Encapsulation has a variety of purposes in the food industry, like improvement of stability and bioavailability of functional foods. Various targeted delivery systems like nanoemulsions, emulsion bilayers, surfactant micelles, etc. for provision and encapsulation of functional foods are being used (Jampilek et al., 2019). Due to public demand and health issues, production of bioactives dubbed as generally recognized as safe (GRAS) is important. For marketing purposes, sensory acceptance is an important parameter. Enhanced bioaccessibility, increased solubility, better stability when produced or stored, increased antitumor activity, antimicrobial food packaging, and improving food safety are some key applications and influences of encapsulation technology.
Emulsion-based delivery systems for encapsulation of essential oils with food-grade material to reduce lipid oxidation, restrict microbial growth, and improve the shelf-life of foods have also been explored. Edible films can also be used for preservation of foods such as meat, milk products, yogurt, fruits, bread, and vegetables. Antioxidant activity of double emulsion fortified with encapsulated Murraya koenigii berry extract improved oxidative stability of reduced-fat meat batter with more stable meat matrices (Kumar and Kumar, 2020). Fresh-cut pineapples which are high in fibers, minerals, and ascorbic acid but with a significantly short shelf life have been fortified with citral nanoemulsion incorporating edible coating successfully with better microbial quality and shelf life (Prakash et al., 2020). Encapsulation of vitamin D3 through nanostructured lipid carriers for producing fortified lassi showed positive results and higher acceptability after sensory/organoleptic evaluation (Maurya and Aggarwal, 2019).
Use of food by-products as functional foods using encapsulation technology, like irradiated onion scales encapsulated in alginate beads can have considerable potential as functional ingredients in various foods (Kanatt et al., 2017). Deriving nanoemulsions from fruit peels discarded by the food processing industry like citrate peel and carrot peel has a potential for further utilization. Development of β-carotene-loaded nanoemulsion from citrus peel resulted in increased retinol activity equivalents and bioaccessibility of β-carotene (Barman et al., 2020).
C-Phycocyanin, a phycobiliprotein used in food industry as a natural dye (dairy products, chewing gum, ice cream jellies), therapeutics, biomarkers, pharmaceuticals, and healthy food, when microencapsulated with extrusion dripping technique was found to have a slow degradation at higher temperature with improved stability and shelf life (Pradeep and Nayak, 2019).
Encapsulation technology has come up as a unique and latest technology. It provides new opportunities and helps in solving the challenges faced by food as well as pharmaceutical industry. In the arena of edible films, the encapsulation technology has emerged as a boon as it incorporate various bioactive materials which are released/delivered at target areas in a very precise manner to improve stability, flavor, color, and texture of foods during processing and storage. Furthermore, the strategic use of these bioactive edible films as vehicles for the tailored/targeted delivery of bioactive ingredients has been proven beneficial for safety as well as shelf-life extensibility of different foods. However, there are challenges in the way of encapsulation technology which limits its commercialization. Processing problems, high costs, concept of controlled release of the bioactive compound into the food, and issues related to standardizing edible film properties need to be addressed so that this technology can be utilized in a much better way in the field of development of edible films that are sustainable, active, and economical, which may enhance the overall potential of the food product. As a result, it is reasonable to conclude that, if properly applied, encapsulation technology can pave the way for a brighter future and commercialization for edible films in particular and the food sector in general.
VC and NT: conceptualization. VC, NT, PK, and ST: writing and draft. SP: editing and review. All authors contributed to the article and approved the submitted version.
The authors declare that the research was conducted in the absence of any commercial or financial relationships that could be construed as a potential conflict of interest.
All claims expressed in this article are solely those of the authors and do not necessarily represent those of their affiliated organizations, or those of the publisher, the editors and the reviewers. Any product that may be evaluated in this article, or claim that may be made by its manufacturer, is not guaranteed or endorsed by the publisher.
Adel, A., Ibrahim, A., El-Shafei, A., and Al-Shemy, M. (2019). Inclusion complex of clove oil with chitosan/β-cyclodextrin citrate/oxidized nanocellulose biocomposite for active food packaging. Food Packag. Shelf Life. 20:100307. doi: 10.1016/j.fpsl.2019.100307
Agarwal, H., Joshi, R., and Gupta, M. (2021). Optimization of pearl millet-derived bioactive peptide microspheres with double emulsion solvent evaporation technique and its release characterization. Food Struct. 29:100200. doi: 10.1016/j.foostr.2021.100200
Ajeeshkumar, K. K., Aneesh, P. A., Raju, N., Suseela, M., Ravishankar, C. N., and Benjakul, S. (2021). Advancements in liposome technology: Preparation techniques and applications in food, functional foods, and bioactive delivery: a review. Compr. Rev. Food Sci. Food Saf. 20, 1280–1306. doi: 10.1111/1541-4337.12725
Almasi, H., Jahanbakhsh Oskouie, M., and Saleh, A. (2020). A review on techniques utilized for design of controlled release food active packaging. Crit. Rev. Food Sci. Nutr. 17, 1–21. doi: 10.1080/10408398.2020.1783199
Al-Tayyar, N. A., Youssef, A. M., and Al-Hindi, R. R. (2020). Edible coatings and antimicrobial nanoemul-sions for enhancing shelf life and reducing food borne pathogens of fruits and vegetables: a review. Sustainable Mater Technol. 26, 1–12. doi: 10.1016/j.susmat.2020.e00215
Amjadi, S., Almasi, M., and Ramazani, S. (2020). Multifunctional betanin nanoliposomes- incorporated gelatin/chitosan naofiber/ ZnO nanoparticles nanocomposite film for fresh beef preservation. Meat Sci. 167:108161. doi: 10.1016/j.meatsci.2020.108161
Antigo, J. L. D., Bergamasco, R. D. C., and Madrona, G. S. (2018). Effect of pH on the stability of red beet extract (Beta vulgaris L.) microcapsules produced by spray drying or freeze drying. Food Sci. Technol. 38, 72–77. doi: 10.1590/1678-457x.34316
Assadpour, E., and Jafari, S. M. (2019a). A systematic review on nanoencapsulation of food bioactive ingredients and nutraceuticals by various nanocarriers. Crit. Rev. Food Sci. Nutr. 59, 3129–3151. doi: 10.1080/10408398.2018.1484687
Assadpour, E., and Jafari, S. M. (2019b). Advances in spray-drying encapsulation of food bioactive ingredients: from microcapsules to nanocapsules. Annu. Rev. Food Sci. Technol. 10, 103–131. doi: 10.1146/annurev-food-032818-121641
Barman, K., Chowdhury, D., and Baruah, P. (2020). Development of β-carotene loaded nanoemulsion using the industrial waste of orange (Citrus reticulate) peel to improve in vitro bioaccessibility of carotenoids and use as natural food colorant. J. Food Process. Preserv. 44:14429. doi: 10.1111/jfpp.14429
Basch, C. Y., Jagus, R. J., and Flores, S. K. (2013). Physical and antimicrobial properties of tapioca starch-HPMC edible films incorporated with nisin and/or potassium sorbate. Food Bioproc. Tech. 6, 2419–2428. doi: 10.1007/s11947-012-0860-3
Basiak, E., Lenart, A., and Debeaufort, F. (2017). Effect of starch type on the physico-chemical properties of edible films. Int. J. Biol. Macromol. 98, 348–356. doi: 10.1016/j.ijbiomac.2017.01.122
Bekhit, M., Arab-Tehrany, E., Kahn, C., Cleymand, F., Fleutot, S., Desobry, S., et al. (2018). Bioactive films containing alginate-pectin composite microbeads with lactococcus lactis subsp. lactis: Physicochemical characterization and antilisterial activity. Int. J. Mol. Sci. 19:574. doi: 10.3390/ijms19020574
Benbettaïeb, N., Debeaufort, F., and Karbowiak, T. (2019). Bioactiveedible films for food applications: mechanisms of antimicrobial and antioxidant activity. Crit. Rev. Food Sci. Nutr. 59, 3431–3455. doi: 10.1080/10408398.2018.1494132
Bonilla, J., Atares, L., Vargas, M., and Chiralt, A. (2013). Properties of wheat starch film-forming dispersions and films as affected by chitosan addition. J. Food Eng. 114, 303–312. doi: 10.1016/j.jfoodeng.2012.08.005
Boostani, S., and Jafari, S. M. (2021). A comprehensive review on the controlled release of encapsulated food ingredients; fundamental concepts to design and applications. Trends Food Sci. Technol. 1:40. doi: 10.1016/j.tifs.2021.01.040
Bosnea, L. A., Moschakis, T., Nigam, P. S., and Biliaderis, C. G. (2017). Growth adaptation of probiotics in biopolymerbased coacervate structures to enhance cell viability. LWT - Food Sci. Technol. 77, 282–289. doi: 10.1016/j.lwt.2016.11.056
Bourbon, A. I., Costa, M. J., Maciel, L. C., Pastrana, L., Vicente, A. A., and Cerqueira, M. A. (2021). Active carboxymethylcellulose-based edible films: influence of free and encapsulated curcumin on films' properties. Foods. 10:1512.
Bushra, J., Rashda, A., Shahid, A., Muhammad, I., Khan, S. U., Ihsan, A., et al. (2016). Encapsulation of cardamom essential oil in chitosan nano-composites: in-vitro efficacy on antibiotic-resistant bacterial pathogens and cytotoxicity studies. Front. Microbiol. 7:1580. doi: 10.3389/fmicb.2016.01580
Castro, N., Durrieu, V., Raynaud, C., Rouilly, A., Rigal, L., and Quellet, C. (2016). Melt extrusion encapsulation of flavors: a review. Polym. Rev. 56, 137–186. doi: 10.1080/15583724.2015.1091776
Chang, D., Hayat, K., Abbas, S., and Zhang, X. (2019). Ascorbic acid encapsulation in a glassy carbohydrate matrix via hot melt extrusion: preparation and characterization. Food Sci. Technol. 39, 660–666. doi: 10.1590/fst.02918
Chang, H. W., Tan, T. B., Tan, P. Y., Abas, F., Lai, O. M., Wang, Y., et al. (2018). Microencapsulation of fish oil using thiol-modified β-lactoglobulin fibrils/chitosan complex: a study on the storage stability and in vitro release. Food Hydrocoll. 80, 186–194. doi: 10.1016/j.foodhyd.2018.02.002
Chen, W., Ma, S., Wang, Q., McClements, D. J., Liu, X., Ngai, T., et al. (2021). Fortification of edible films with bioactive agents: a review of their formation, properties, and application in food preservation. Crit Rev Food Sci Nutr. 18, 1–27. doi: 10.1080/10408398.2021.1881435
Chen, X., Chen, M., Xu, C., and Yam, K. L. (2019). Critical review of controlled release packaging to improve food safety and quality. Crit. Rev. Food Sci. Nutr. 59, 2386–2399. doi: 10.1080/10408398.2018.1453778
Choudhury, N., Meghwal, M., and Das, K. (2021). Microencapsulation: An overview on concepts, methods, properties and applications in foods. Food Front. 2, 1–17. doi: 10.1002/fft2.94
Coronel-Aguilera, C. P., and San Martín-González, M. F. (2015). Encapsulation of spray dried β-carotene emulsion by fluidized bed coating technology. LWT—Food Sci. Technol. 62, 187–193. doi: 10.1016/j.lwt.2014.12.036
Cui, H., Bai, M., Rashed, M. M., and Lin, L. (2018). The antibacterial activity of clove oil/chitosan nanoparticles embedded gelatin nanofibers against Escherichia coli O157: H7 biofilms on cucumber. Int. J. Food Microbiol. 266, 69–78. doi: 10.1016/j.ijfoodmicro.2017.11.019
Cui, H., Wu, J., Li, C., and Lin, L. (2017). Improving anti-listeria activity of cheese packaging via nanofiber containing nisin-loaded nanoparticles. LWT—Food Sci. Technol. 81, 233–242. doi: 10.1016/j.lwt.2017.04.003
Cutrim, C. S., Alvim, I. D., and Cortez, M. (2019). Microencapsulation of green tea polyphenols by ionic gelation and spray chilling methods. J. Food Sci.Technol. 56, 3561–3570. doi: 10.1007/s13197-019-03908-1
Dammak, I., de Carvalho, R. A., Trindade, C. S. F., Lourenço, R. V., and Sobral, P. J. A. (2017). Properties of active gelatin films incorporated with rutin-loaded nanoemulsions. Int. J. Biol. Macromol. 98, 39–49. doi: 10.1016/j.ijbiomac.2017.01.094
Das, A. K., Nanda, P. K., Bandyopadhyay, S., Banerjee, R., Biswas, S., and McClements, D. J. (2020). Application of nanoemulsion-based approaches for improving the quality and safety of muscle foods. Comp. Rev. Food Sci. Food Saf. 19, 2677–2700. doi: 10.1111/1541-4337.12604
Dehghani, S., Hosseini, S. V., and Regenstein, J. M. (2018). Edible films and coatings in seafood preservation: a review. Food Chem. 240, 505–513. doi: 10.1016/j.foodchem.2017.07.034
Delshadi, R., Bahrami, A., Assadpour, E., Williams, L., and Jafari, S. M. (2021). Nano/microencapsulated natural antimicrobials to control the spoilage microorganisms and pathogens in different food products. Food Control. 10:8180. doi: 10.1016/j.foodcont.2021.108180
De'Nobili, M. D., Perez, C. D., Navarro, D. A., Stortz, C. A., and Rojas, A. M. (2013). Hydrolytic stability of l-(þ)-ascorbic acid in low methoxyl pectin films with potential antioxidant activity at food interfaces. Food Bioproc. Tech. 6, 186–197. doi: 10.1007/s11947-011-0684-6
Dhital, R., Joshi, P., Becerra-Mora, N., Umagiliyage, A., Chai, T., Kohli, P., et al. (2017). Integrity of edible nano-coatings and its effects on quality of strawberries subjected to simulated in-transit vibrations. LWT- Food Sci. Technol. 80, 257–264. doi: 10.1016/j.lwt.2017.02.033
Díaz-Montes, E., and Castro-Muñoz, R. (2021). Edible films and coatings as food-quality preservers: an overview. Foods. 10:249. doi: 10.3390/foods10020249
Dubey, N. K., and Dubey, R. (2020). “Edible films and coatings: An update on recent advances,” in Biopolymer-Based Formulations, ed Pal et al. (New York, NY: Elsevier), 675–695.
El-Kader, A., and Abu Hashish, H. (2020). Encapsulation techniques of food bioproduct. Egypt. J. Chem. 63, 6–7. doi: 10.21608/ejchem.2019.16269.1993
Esposto, B. S., Juregi, P., Tapia-Blacido, D. R., and Martelli-Tosi, M. (2021). Liposomes vs. chitosomes: Encapsulating food bioactives. Trends Food Sci. Technol. 108, 40–48. doi: 10.1016/j.tifs.2020.12.003
Evangelho, J. A., Silva Dannenberg, G., da., Biduski, B., El Halal, S. L. M., Kringel, D. H., et al. (2019). Antibacterial activity, optical, mechanical, and barrier properties of corn starch films containing orange essential oil. Carbohydr. Polym. 222:114981. doi: 10.1016/j.carbpol.2019.114981
Farheen, T., Shaikh, A., and Shahi, S. (2017). a review on a process: microencapsulation. Int. J.Pharma Res. Health Sci. 5, 1823–1830.
Fuciños, C., Míguez, M., Fuciños, P., Pastrana, L. M., and Rúa, M. L. (2017). Vicente Creating functional nanostructures: encapsulation of caffeine into α-lactalbumin nanotubes. Innov. Food Sci. Emerg. Technol. 40, 10–17. doi: 10.1016/j.ifset.2016.07.030
Garavand, F., Rouhi, M., Razavi, S. H., Cacciotti, I., and Mohammadi, R. (2017). Improving the integrity of natural biopolymer films used in food packaging by cross linking approach: a review. Int. J. Biol. Macromol. 104, 687–707. doi: 10.1016/j.ijbiomac.2017.06.093
Ge, L., Zhu, M., Xu, Y., Li, X., Li, D., and Mu, C. (2017). Development of antimicrobial and controlled biodegradable gelatin-based edible films containing nisin and amino-functionalized montmorillonite. Food Bioproc. Tech. 10, 1727–1736. doi: 10.1007/s11947-017-1941-0
Golfomitsou, I., Mitsou, E., Xenakis, A., and Papadimitriou, V. (2018). Development of food grade O/W nanoemulsions as carriers of vitamin D for the fortification of emulsion based food matrices: a structural and activity study. J. Mol. Liq. 268, 734–742. doi: 10.1016/j.molliq.2018.07.109
Gomez-Estaca, J., Lopez-de-Dicastillo, C., Hernandez-Munoz, P., Catala, R., and Gavara, R. (2014). Advances in antioxidant active food packaging. Trends Food Sci Technol. 35, 42–51. doi: 10.1016/j.tifs.2013.10.008
Gong, C., Lee, M. C., Godec, M., Zhang, Z., and Abbaspourrad, A. (2020). Ultrasonic encapsulation of cinnamon flavor to impart heat stability for baking applications. Food Hydrocoll. 99:105316. doi: 10.1016/j.foodhyd.2019.105316
Grootveld, M., Percival, B. C., Leenders, J., and Wilson, P. B. (2020). Potential adverse public health effects afforded by the ingestion of dietary lipid oxidation product toxins: Significance of fried food sources. Nutrients. 12:974. doi: 10.3390/nu12040974
Guo, J., Li, P., Kong, L., and Xu, B. (2020). Microencapsulation of curcumin by spray drying and freeze drying. LWT- Food Sci. Technol. 132:109892. doi: 10.1016/j.lwt.2020.109892
He, L., Hu, J., and Deng, W. (2018). Preparation and application of flavor and fragrance capsules. Polym. Chem. 9, 4926–4946. doi: 10.1039/C8PY00863A
Helal, A., Tagliazucchi, D., Conte, A., and Desobry, S. (2012). Antioxidant properties of polyphenols incorporated in casein/sodium caseinate films. Int. Dairy J. 25, 10–15. doi: 10.1016/j.idairyj.2011.12.002
Hemmatkhah, F., Zeynali, F., and Almasi, H. (2020). Encapsulated cumin seed essential oil-loaded active papers: characterization and evaluation of the effect on quality attributes of beef hamburger. Food Bioprocess Technol. 13, 533–547. doi: 10.1007/s11947-020-02418-9
Hu, S., Yu, J., Wang, Z., Li, L., Du, Y., Wang, L., et al. (2017). Effects of sorbic acid-chitosan microcapsules as antimicrobial agent on the properties of ethylene vinyl alcohol copolymer film for food packaging. J. Food Sci. 82, 1451–1460. doi: 10.1111/1750-3841.13731
Imran, M., Revol-Junelles, A. M., Rene, N., Jamshidian, M., Akhtar, M. J., Arab-Tehrany, E., et al. (2012). Microstructure and physico-chemical evaluation of nano-emulsion-based antimicrobial peptides embedded in bioactive packaging films. Food Hydrocoll. 29, 407–419. doi: 10.1016/j.foodhyd.2012.04.010
Ishizuka, F., Stenzel, M. H., and Zetterlund, P. B. (2018). Microcapsule synthesis via RAFT photopolymerization in vegetable oil as a green solvent. J. Polym. Sci. Part A: Polym. Chem. 56, 831–839. doi: 10.1002/pola.28958
Jafari, S. M., Paximada, P., Mandala, I., Assadpour, E., and Mehrnia, M. A. (2017). Encapsulation by nanoemulsions in nanoencapsulation technologies for the food and nutraceutical industries. Cambridge, MA: Academic Press, 36–73.
Jampilek, J., Kos, J., and Kralova, K. (2019). Potential of nanomaterial applications in dietary supplements and foods for special medical purposes. Nanomater. 9:296. doi: 10.3390/nano9020296
Jeevahan, J., Chandrasekaran, M., Durairaj, R., Mageshwaran, G., and Joseph, G. B. (2017). A brief review on edible food packing materials. Int. J. Res. Sci. Eng. 1, 9–19.
Kanatt, S., Tari, S., and Chawla, S. (2017). Encapsulation of extract prepared from irradiated onion scales in alginate beads: a potential functional food ingredient. J. Food Meas. Charact. 12, 848–858. doi: 10.1007/s11694-017-9699-7
Kanwate, B., Ballari, R., and Kudre, T. (2019). Influence of spray-drying, freeze-drying and vacuum-drying on physicochemical and functional properties of gelatin from Labeo rohita swim bladder. Int. J. Biol. Macromol. 121, 135–141. doi: 10.1016/j.ijbiomac.2018.10.015
Khan, M. R., Sadiq, M. B., and Mehmood, Z. (2020). Development of edible gelatin composite films enriched with polyphenol loaded nanoemulsions as chicken meat packaging material. CyTA—J. Food. 18, 137–146. doi: 10.1080/19476337.2020.1720826
Khoshnoudi-Nia, S., Forghani, Z., and Jafari, S. M. (2020). A systematic review and meta-analysis of fish oil encapsulation within different micro/nanocarriers. Crit. Rev. Food Sci. Nutr. 18, 1–22. doi: 10.1080/10408398.2020.1848793
Kizilbey, K. (2019). Optimization of rutin-loaded plga nanoparticles synthesized by single-emulsion solvent evaporation method. ACS Omega. 4, 555–562. doi: 10.1021/acsomega.8b02767
Ko, S., Janes, M. E., Hettiarachchy, N. S., and Johnson, M. G. (2001). Physical and chemical properties of edible films containing nisin and their action against Listeria monocytogenes. J. Food Sci. 66, 1006–1011. doi: 10.1111/j.1365-2621.2001.tb08226.x
Kumar, C. G., Sripada, S., and Poornachandra, Y. (2018). “Status and future prospects of fructooligosaccharides as nutraceuticals,” in Role of Materials Science in Food Bioengineering, eds A. M. Grumezescu, A.M. Holban (Academic Press: Cambridge, Massachusetts), 451–503.
Kumar, Y., and Kumar, V. (2020). Effects of double emulsion (W1/O/W2) containing encapsulated Murraya koenigii berries extract on quality characteristics of reduced-fat meat batter with high oxidative stability. LWT-Food Sci. Technol. 127:109365. doi: 10.1016/j.lwt.2020.109365
Lavelli, V. (2021). Circular food supply chains–Impact on value addition and safety. Trends Food Sci. Technol. 114, 323–332. doi: 10.1016/j.tifs.2021.06.008
Li, L., Song, W., Shen, C., Dong, Q., Wang, Y., and Zuo, S. (2020). Active packaging film containing oregano essential oil microcapsules and their application for strawberry preservation. J. Food Process. Preserv. 44:e14799. doi: 10.1111/jfpp.14799
Liang, J., Yan, H., Zhang, J., Dai, W., Gao, X., Zhou, Y., et al. (2017). Preparation and characterization of antioxidant edible chitosan films incorporated with epigallocatechin gallate nanocapsules. Carbohydr. Polym. 171, 300–306. doi: 10.1016/j.carbpol.2017.04.081
Lin, L., Gu, Y., and Cui, H. (2019). Moringa oil/chitosan nanoparticles embedded gelatin nanofibers for food packaging against Listeria monocytogenes and Staphylococcus aureus on cheese. Food Packag. Shelf Life. 19, 86–93. doi: 10.1016/j.fpsl.2018.12.005
Liu, B., Jiao, L., Chai, J., Bao, C., Jiang, P., and Li, Y. (2021). “Encapsulation and targeted release,” in Food Hydrocolloids (Singapore: Springer), 369–407.
Liu, F., Antoniou, J., Li, Y., Yi, J., Yokoyama, W., Ma, J., et al. (2015). Preparation of gelatin films incorporated with tea polyphenol nanoparticles for enhancing controlled-release antioxidant properties. J. Agric. Food Chem. 63, 3987–3995. doi: 10.1021/acs.jafc.5b00003
Liu, H., Xie, M., and Nie, S. (2020). Recent trends and applications of polysaccharides for microencapsulation of probiotics. Food Front. 1, 45–59. doi: 10.1002/fft2.11
Lopez-Polo, J., Monasterio, A., Cantero-Lopez, P., and Osorio, F. A. (2021). Combining edible coatings technology and nanoencapsulation for food application: a brief review with an emphasis on nanoliposomes. Int. Food Res. J. 110402.
Lourenço, S. C., Fraqueza, M. J., Fernandes, M. H., Moldão-Martins, M., and Alves, V. D. (2020). Application of edible alginate films with pineapple peel active compounds on beef meat preservation. Antioxidants. 9:667. doi: 10.3390/antiox9080667
Luis, A., Pereira, L., Domingues, F., and Ramos, A. (2019). Development of a carboxymethyl xylan film containing licorice essential oil with antioxidant properties to inhibit the growth of food borne pathogens. LWT—Food Sci. Technol. 111, 218–225. doi: 10.1016/j.lwt.2019.05.040
Maleki, O., Khaledabad, M. A., Amiri, S., Asl, A. K., and Makouie, S. (2020). Microencapsulation of Lactobacillus rhamnosus ATCC 7469 in whey protein isolate-crystalline nanocellulose-inulin composite enhanced gastrointestinal survivability. LWT- Food Sci. Technol. 126:109224. doi: 10.1016/j.lwt.2020.109224
Malherbi, N. M., Schmitz, A. C., Grando, R. C., Bilck, A. P., Yamashita, F., Tormen, L., Fakhouri, F. M., et al. (2019). Corn starch and gelatin-based films added with guabiroba pulp for application in food packaging. Food Packag. Shelf Life. 19, 140–146. doi: 10.1016/j.fpsl.2018.12.008
Marcuzzo, E., Sensidoni, A., Debeaufort, F., and Voilley, A. (2010). Encapsulation of aroma compounds in biopolymeric emulsion based edible films to control flavour release. Carbohydr. Polym. 80, 984–988. doi: 10.1016/j.carbpol.2010.01.016
Maurya, V., and Aggarwal, M. (2019). Fabrication of nano-structured lipid carrier for encapsulation of vitamin D3 for fortification of ‘Lassi’; A milk based beverage. J. Steroid Biochem. Mol. Biol. 193:105429. doi: 10.1016/j.jsbmb.2019.105429
McClements, D. J. (2019). Nanoparticle-and microparticle-based delivery systems. Encapsulation, protection and release of active compounds. Boca Raton, FL: CRC press.
Mihalcea, L., Turturic,ă, M., Ghinea, I. O., Barbu, V., Ioni,t,ă, E., Cotârlet, M., et al. (2017). Encapsulation of carotenoids from sea buckthorn extracted by CO2 supercritical fluids method within whey proteins isolates matrices. Innov. Food Sci. Emerg. Technol. 42, 120–129. doi: 10.1016/j.ifset.2017.06.008
Mirzaei-Mohkam, A., Garavand, F., Dehnad, D., Keramat, J., and Nasirpour, A. (2020). Physical, mechanical, thermal and structural characteristics of nanoencapsulated vitamin E loaded carboxymethyl cellulose films. Prog. Org. Coat. 138, 105383. doi: 10.1016/j.porgcoat.2019.105383
Muhammad, D. R. A., Doost, A. S., Gupta, V., bin Sintang, M. D., Van de Walle, D., Van der Meeren, P., et al. (2020). Stability and functionality of xanthan gum–shellac nanoparticles for the encapsulation of cinnamon bark extract. Food Hydrocoll. 100:105377. doi: 10.1016/j.foodhyd.2019.105377
Muhoza, B., Xia, S., Wang, X., Zhang, X., Li, Y., and Zhang, S. (2020). Microencapsulation of essential oils by complex coacervation method: preparation, thermal stability, release properties and applications. Crit. Rev. Food Sci. Nutr. 18, 1–20. doi: 10.1080/10408398.2020.1843132
Nami, Y., Lornezhad, G., Kiani, A., Abdullah, N., and Haghshenas, B. (2020). Alginate-persian gum-prebiotics microencapsulation impacts on the survival rate of Lactococcus Lactis ABRIINW-N19 in orange juice. LWT-Food Sci. Technol. 124:109190. doi: 10.1016/j.lwt.2020.109190
Naranjo-Durán, A. M., Quintero-Quiroz, J., Rojas-Camargo, J., and Ciro-Gomez, G. L. (2021). Modified-release of encapsulated bioactive compounds from annatto seeds produced by optimized ionic gelation techniques. Sci. Rep. 11:1317. doi: 10.1038/s41598-020-80119-1
Nascimento, J. A., de, A., Gomes, L. K. S., Duarte, D. S., Lima, M.A.C.de, and Britto, D. (2019). Stability of nanocomposite edible films based on polysaccharides and vitamin c from agroindustrial residue. Mater. Res. 22:57. doi: 10.1590/1980-5373-mr-2019-0057
Nieto-Suaza, L., Acevedo-Guevara, L., Sánchez, L. T., Pinzón, M. I., and Villa, C. C. (2019). Characterization of Aloe vera-banana starch composite films reinforced with curcumin-loaded starch nanoparticles. Food Struct. 22:100131. doi: 10.1016/j.foostr.2019.100131
Niu, B., Shao, P., Chen, H., and Sun, P. (2019). Structural and physiochemical characterization of novel hydrophobic packaging films based on pullulan derivatives for fruits preservation. Carbohydr. Polym. 208, 276–284. doi: 10.1016/j.carbpol.2018.12.070
Nogueira, G. F., Soares, C. T., Cavasini, R., Fakhouri, F. M., and de Oliveira, R. A. (2019). Bioactive films of arrow root starch and blackberry pulp: physical, mechanical and barrier properties and stability to pH and sterilization. Food Chem. 275, 417–425. doi: 10.1016/j.foodchem.2018.09.054
Nogueira, I. F., Fakhouri, F. M., and Oliveira, R. A. (2019). Incorporation of spray dried and freeze dried blackberry particles in edible films: morphology, stability to pH, sterilization and biodegradation. Food Packag. Shelf Life. 20:100313. doi: 10.1016/j.fpsl.2019.100313
Norajit, K., Kim, K. M., and Ryu, G. H. (2010). Comparative studies onthe characterization and antioxidant properties of biodegradable alginate films containing ginseng extract. J. Food Eng. 98, 377–384. doi: 10.1016/j.jfoodeng.2010.01.015
Paret, N., Trachsel, A., Berthier, D. L., and Herrmann, A. (2019). Developing multi stimuli-responsive core/shell microcapsules to control the release of volatile compounds. Macromol. Mater. Eng. 304:1800599. doi: 10.1002/mame.201800599
Passos, R. B., dos, Bazzo, G. C., Almeida, A., da, R., Noronha, C. M., and Barreto, P.L.M. (2019). Evaluation of oxidative stability of mayonnaise containing poly ε-caprolactone nanoparticles loaded with thyme essential oil. Braz. J. Pharm. Sci. 55:177. doi: 10.1590/s2175-97902019000118177
Pellicer, J. A., Fortea, M. I., Trabal, J., Rodríguez-López, M. I., Gabaldón, J. A., and Núñez-Delicado, E. (2019). Stability of microencapsulated strawberry flavour by spray drying, freeze drying and fluid bed. Powder Technol. 347, 179–185. doi: 10.1016/j.powtec.2019.03.010
Pérez-Córdoba, L. J., Norton, I. T., Batchelor, H. K., Gkatzionis, K., Spyropoulos, F., and Sobral, P. J. A. (2018). Physico-chemical, antimicrobial and antioxidant properties of gelatin-chitosan based films loaded with nanoemulsions encapsulating active compounds. Food Hydrocoll. 79, 544–559. doi: 10.1016/j.foodhyd.2017.12.012
Pieczykolan, E., and Kurek, M. A. (2019). Use of guar gum, gum arabic, pectin, beta-glucan and inulin for microencapsulation of anthocyanins from chokeberry. Int. J. Biol. Macromol. 129, 665–671. doi: 10.1016/j.ijbiomac.2019.02.073
Pirozzi, A., Del Grosso, V., Ferrari, G., and Dons,ì, F. (2020). Edible coatings containing oregano essential oil nanoemulsion for improving postharvest quality and shelf life of tomatoes. Foods 9:1605. doi: 10.3390/foods9111605
Pradeep, H., and Nayak, C. (2019). Enhanced stability of C-phycocyanin colorant by extrusion encapsulation. J. Food Sci. Technol. 56, 4526–4534. doi: 10.1007/s13197-019-03955-8
Prakash, A., Baskaran, R., and Vadivel, V. (2020). Citral nanoemulsion incorporated edible coating to extend the shelf life of fresh cut pineapples. LWT 118:108851. doi: 10.1016/j.lwt.2019.108851
Procopio, F. R., Oriani, V. B., Paulino, B. N., do Prado-Silva, L., Pastore, G. M., Sant'Ana, A. S., et al. (2018). Solid lipid microparticles loaded with cinnamon oleoresin: Characterization, stability and antimicrobial activity. Food Res. Int. 113, 351–361. doi: 10.1016/j.foodres.2018.07.026
Quirós-Sauceda, A. E., Ayala-Zavala, J. F., Olivas, G. I., and González-Aguilar, G. A. (2014). Edible coatings as encapsulating matrices for bioactive compounds: a review. J. Food Sci. Technol. 51, 1674–1685. doi: 10.1007/s13197-013-1246-x
Robledo, N., Vera, P., López, L., Yazdani-Pedram, M., Tapia, C., and Abugoch, L. (2018). Thymol nanoemulsions incorporated in quinoa protein/chitosan edible films; antifungal effect in cherry tomatoes. Food Chem. 246, 211–219. doi: 10.1016/j.foodchem.2017.11.032
Rodríguez, G. M., Sibaja, J. C., Espitia, P. J., and Otoni, C. G. (2020). Antioxidant active packaging based on papaya edible films incorporated with Moringa oleifera and ascorbic acid for food preservation. Food Hydrocoll. 103:105630. doi: 10.1016/j.foodhyd.2019.105630
Rostamabadi, H., Falsafi, S. R., Boostani, S., Katouzian, I., Rezaei, A., Assadpour, E., et al. (2021). “Design and formulation of nano/micro-encapsulated natural bioactive compounds for food applications” in Application of Nano/Microencapsulated Ingredients in Food Products (Boca, Raton: Academic Press), 1–41.
Ruan, C., Zhang, Y., Wang, J., Sun, Y., Gao, X., Xiong, G., et al. (2019). Preparation and antioxidant activity of sodium alginate and carboxymethyl cellulose edible films with epigallocatechin gallate. Int. J. Biol. Macromol. 134, 1038–1044. doi: 10.1016/j.ijbiomac.2019.05.143
Salvia-Trujillo, L., Rojas-Gra,ü M. A., Soliva-Fortuny, R., et al. (2015). Use of antimicrobial nanoemul-sions as edible coatings: impact on safety and quality attributes of fresh-cut Fuji apples. Postharvest Biol. Technol. 105, 8–16. doi: 10.1016/j.postharvbio.2015.03.009
Sanchez-Gonzalez, L., Vargas, M., Gonzalez-Martinez, C., Chiralt, A., and Chafer, M. (2011). Use of essential oils in bioactive edible coat-ings: a review. Food Eng. Rev. 3, 1–16. doi: 10.1007/s12393-010-9031-3
Shen, Z., and Kamdem, D. P. (2015). Development and characterization of biodegradable chitosan films containing two essential oils. Int. J. Biol. Macromol. 74, 289–296. doi: 10.1016/j.ijbiomac.2014.11.046
Shukla, S., Haldorai, Y., Hwang, S. K., Bajpai, V. K., Huh, Y. S., and Han, Y. (2017). Current demands for food-approved liposome nanoparticles in food and safety sector. Front. Microbiol. 17:2398. doi: 10.3389/fmicb.2017.02398
Silva-Weiss, A., Ihl, M., Sobral, P. J. A., Gomez-Guillen, M. C., and Bifani, V. (2013). Natural additives in bioactive edible films and coatings: functionality and applications in foods. Food Eng. Rev. 5, 200–216. doi: 10.1007/s12393-013-9072-5
Song, Y., Fan, J., and Wang, S. (2017). Recent progress in interfacial polymerization. Mat. Chem. Front. 1, 1028–1040. doi: 10.1039/C6QM00325G
Soukoulis, C., Behboudi-Jobbehdar, S., Yonekura, L., Parmenter, C., and Fisk, I. D. (2014). Stability of Lactobacillus rhamnosus GG in prebiotic edible films. Food Chem. 159, 302–308. doi: 10.1016/j.foodchem.2014.03.008
Soukoulis, C., and Bohn, T. (2018). A comprehensive overview on the micro- and nano-technological encapsulation advances for enhancing the chemical stability and bioavailability of carotenoids. Crit. Rev. Food Sci. Nutr. 58, 1–36. doi: 10.1080/10408398.2014.971353
Souza, A. L., Hidalgo-Chávez, D. W., Pontes, S. M., Gomes, F. S., Cabral, L. M., and Tonon, R. V. (2018). Microencapsulation by spray drying of a lycopene-rich tomato concentrate: characterization and stability. LWT- Food Sci. Technol. 91, 286–292. doi: 10.1016/j.lwt.2018.01.053
Stoll, L., Costa, T. M. H., Jablonski, A., Flores, S. H., and de Oliveira Rios, A. (2016). Microencapsulation of anthocyanins with different wall materials and its application in active biodegradable films. Food Bioproc. Tech. 9, 172–181. doi: 10.1007/s11947-015-1610-0
Subasi, B. G., Vahapoglu, B., and Capanoglu, E. (2021). Microencapsulation Methods for Food Antioxidants In Plant Antioxidants and Health. Switzerland: Springer Nature, 1–37.
Sun, Y., Zhang, M., Bhandari, B., and Bai, B. (2021). Nanoemulsion-based edible coatings loaded with fennel essential oil/cinnamaldehyde: characterization, antimicrobial property and advantages in pork meat patties application. Food Control. 127:108151. doi: 10.1016/j.foodcont.2021.108151
Tian, B., Xiao, D., Hei, T., Ping, R., Hua, S., and Liu, J. (2020). The application and prospects of cyclodextrin inclusion complexes and polymers in the food industry: a review. Polym. Int. 69, 597–603. doi: 10.1002/pi.5992
Tian, F., Decker, E. A., and Goddard, J. M. (2013). Controlling lipid oxidation of food by active packaging technologies. Food Funct. 4, 669–680. doi: 10.1039/c3fo30360h
Tripathi, A. D., Sharma, R., Agarwal, A., and Haleem, D. R. (2021). Nanoemulsions based edible coatings with potential food applications. Int. J. Biobased Plast. 3, 112–125. doi: 10.1080/24759651.2021.1875615
Trucillo, P., Campardelli, R., and Reverchon, E. (2018). Production of liposomes loaded with antioxidants using a supercritical CO2 assisted process. Powder Technol. 323, 155–162. doi: 10.1016/j.powtec.2017.10.007
Venugopalan, V. K., Gopakumar, L. R., Kumaran, A. K., Chatterjee, N. S., Soman, V., Peeralil, S., et al. (2021). Encapsulation and Protection of Omega-3-Rich Fish Oils Using Food-Grade Delivery Systems. Foods 10:1566. doi: 10.3390/foods10071566
Vilela, C., Pinto, R. J. B., Coelho, J., Domingues, M. R. M., Daina, S., Sadocco, P., et al. (2017). Bioactive chitosan/ellagic acid films with UV-light protection for active food packaging. Food Hydrocoll. 73, 120–128. doi: 10.1016/j.foodhyd.2017.06.037
Wang, S., Xia, P., Wang, S., Liang, J., Sun, Y., Yue, P., and Gao, X. (2019). Packaging films formulated with gelatin and anthocyanins nanocomplexes: physical properties, antioxidant activity and its application for olive oil protection. Food Hydrocoll. 96, 617–624. doi: 10.1016/j.foodhyd.2019.06.004
Wu, H., Lei, Y., Lu, J., Zhu, R., Xiao, D., Jiao, C., et al. (2019). Effect of citric acid induced cross linking on the structure and properties of potato starch/chitosan composite films. Food Hydrocoll. 97:105208. doi: 10.1016/j.foodhyd.2019.105208
Wu, J., Liu, H., Ge, S., Wang, S., Qin, Z., Chen, L., et al. (2015). The preparation, characterization, antimicrobial stability and in-vitro release evaluation of fish gelatin films incorporated with cinnamon essential oil nanoliposomes. Food Hydrocoll. 43, 427–435 doi: 10.1016/j.foodhyd.2014.06.017
Xiao, Y., Wu, B., Fu, X., Wang, R., and Lei, J. (2018). Preparation of biodegradable microcapsules through an organic solvent-free interfacial polymerization method. Polym. Adv. Technol. 30, 483–488. doi: 10.1002/pat.4482
Xiong, Y., Li, S., Warner, R. D., and Fang, Z. (2020). Effect of oregano essential oil and resveratrol nanoemulsion loaded pectin edible coating on the preservation of pork loin in modified atmosphere packaging. Food Control 114:107226. doi: 10.1016/j.foodcont.2020.107226
Xu, T., Gao, C. C., Feng, X., Huang, M., Yang, Y., Shen, X., et al. (2019a). Cinnamon and clove essential oils to improve physical, thermal and antimicrobial properties of chitosan-gum arabic polyelectrolyte complexed films. Carbohydr. Polym. 217, 116–125. doi: 10.1016/j.carbpol.2019.03.084
Xu, T., Gao, C. C., Feng, X., Yang, Y., Shen, X., and Tang, X. (2019b). Structure, physical and antioxidant properties of chitosan-gum arabic edible films incorporated with cinnamon essential oil. Int. J. Biol. Macromol. 134, 230–236. doi: 10.1016/j.ijbiomac.2019.04.189
Xu, X., Sun, Q., and McClements, D. J. (2021). Effects of anionic polysaccharides on the digestion of fish oil-in-water emulsions stabilized by hydrolyzed rice glutelin. Food Res. Int. 127:108768. doi: 10.1016/j.foodres.2019.108768
Ye, C., and Chi, H. (2018). A review of recent progress in drug and protein encapsulation: approaches, applications and challenges. Mater. Sci. Eng. C. 83, 233–246. doi: 10.1016/j.msec.2017.10.003
Yin, C., Huang, C., Wang, J., Liu, Y., Lu, P., and Huang, L. (2019). Effect of chitosan- and alginate-based coatings enriched with cinnamon essential oil microcapsules to improve the postharvest quality of mangoes. Materials 12:2039. doi: 10.3390/ma12132039
Zaghari, L., Basiri, A., and Rahimi, S. (2020). Preparation and characterization of double-coated probiotic bacteria via afluid-bed process: acase study on Lactobacillus reuteri. Int. J. Food Eng. 16:20190384. doi: 10.1515/ijfe-2019-0384
Zhang, X., J., Liu, C., Qian, J., and Jin, C. (2019). Effect of grafting method on the physical property and antioxidant potential of chito-san film functionalized with gallic acid. Food Hydrocoll. 89, 1–10. doi: 10.1016/j.foodhyd.2018.10.023
Zhang, W., Shu, C., Chen, Q., and Cao, J. (2019). The multilayer film system improved the release and retention properties of cinnamon essential oil and its application as coating in inhibition to penicillium expansion of apple fruit. Food Chem. 299:125109. doi: 10.1016/j.foodchem.2019.125109
Keywords: edible packaging, encapsulation, nanoemulsions, bioactive compounds, functional foods
Citation: Chaudhary V, Thakur N, Kajla P, Thakur S and Punia S (2021) Application of Encapsulation Technology in Edible Films: Carrier of Bioactive Compounds. Front. Sustain. Food Syst. 5:734921. doi: 10.3389/fsufs.2021.734921
Received: 01 July 2021; Accepted: 17 September 2021;
Published: 26 October 2021.
Edited by:
Veronica Calado, Federal University of Rio de Janeiro, BrazilReviewed by:
Pamela Pereira, Federal University of Rio de Janeiro, BrazilCopyright © 2021 Chaudhary, Thakur, Kajla, Thakur and Punia. This is an open-access article distributed under the terms of the Creative Commons Attribution License (CC BY). The use, distribution or reproduction in other forums is permitted, provided the original author(s) and the copyright owner(s) are credited and that the original publication in this journal is cited, in accordance with accepted academic practice. No use, distribution or reproduction is permitted which does not comply with these terms.
*Correspondence: Sneh Punia, c25laHB1bmlhNjlAZ21haWwuY29t
Disclaimer: All claims expressed in this article are solely those of the authors and do not necessarily represent those of their affiliated organizations, or those of the publisher, the editors and the reviewers. Any product that may be evaluated in this article or claim that may be made by its manufacturer is not guaranteed or endorsed by the publisher.
Research integrity at Frontiers
Learn more about the work of our research integrity team to safeguard the quality of each article we publish.