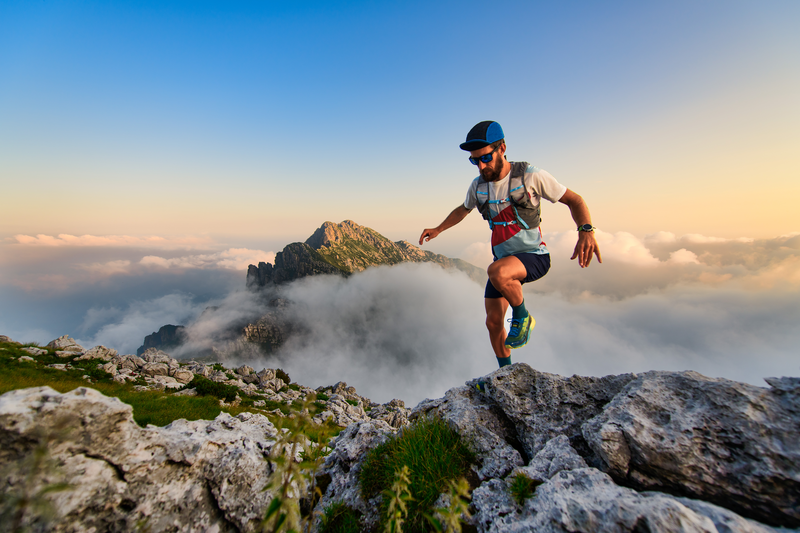
94% of researchers rate our articles as excellent or good
Learn more about the work of our research integrity team to safeguard the quality of each article we publish.
Find out more
ORIGINAL RESEARCH article
Front. Sustain. Food Syst. , 24 December 2021
Sec. Water-Smart Food Production
Volume 5 - 2021 | https://doi.org/10.3389/fsufs.2021.734681
The Mexican Bajío region is the country's main barley (Hordeum vulgare) producing area. Barley is commonly produced during the dry autumn–winter season using furrow irrigation with ground water, following which rainfed maize (Zea mays) is grown in the spring–summer season using supplementary irrigation. Ground water levels in the region are steadily dropping, and the introduction of water-saving technologies in agriculture is urgently required. Drip irrigation can reduce water use but is costly. Conservation agriculture—the combination of minimal tillage, permanent soil cover and crop diversification—might reduce water use, but studies in irrigated systems are scarce. We compared water use and grain yield in tillage-based conventional agriculture and conservation agriculture, both with furrow irrigation and drip irrigation, in a 3-year (six growing seasons) barley-maize field experiment. Additionally, side-by-side demonstrations of conventional and conservation agriculture were installed simultaneously in farmers' fields and yields, water use and fuel use were recorded. In the field experiment, yields did not differ significantly between production systems, but irrigation water use was on average 17% lower in conservation agriculture than in conventional agriculture, ~36% lower with drip irrigation compared with furrow irrigation in conventional tillage, and 40% lower with drip irrigation and conservation agriculture combined compared with conventional agriculture with furrow irrigation. Water use reductions differed strongly between years, depending on weather. The water saving through conservation agriculture in farmers' fields was similar to the water saving in the controlled experiment with about 17%. Additionally, in farmer's fields conservation agriculture reduced greenhouse gas emissions by 192 kg CO2 ha−1 and improved soil health. The implementation of conservation agriculture would be a cost-effective method to reduce water use in the barley-maize production system in the Mexican Bajío, while simultaneously reducing greenhouse gas emissions.
Mexico is currently the largest exporter of beer and the second largest importer of barley (Hordeum vulgare L.) malt in the world (FAO, 20201). Barley in Mexico is mainly produced in irrigated agriculture in the Bajío region, specifically in Guanajuato and Queretaro states (SIAP, 2020). However, the region is increasingly suffering from water stress, which hampers agricultural production. The agricultural sector uses 84% of the state's water to irrigate ~3,36,000 ha of crops and is the largest water consumer in the state (Hoogesteger and Wester, 2017; SIAP, 2020). Water for irrigation in Guanajuato comes mainly from aquifers (69% of water used), while the remaining 31% comes from surface water. As a result of the intensive agriculture in the region, 19 of the 20 aquifers in the state are now overexploited, and the groundwater level is dropping at a rate of up to 3 m year−1 (Wester et al., 2011; Romero et al., 2017; Flores et al., 2018). In order to assure the continued production of barley in the region, it is thus important to drastically reduce agricultural water consumption.
Irrigated barley in the Bajío is grown between December and May, with the optimal planting date being around the 15th of December. Water is provided through furrow irrigation in 3 to 4 heavy (100–300 mm) irrigations. Between May and December, maize (Zea mays L.) is generally grown, and this may receive 1 or 2 furrow irrigations depending on the amount of rainfall in the particular growing season. Precipitation is projected to decrease under all climate change scenarios, which will increase the need for irrigation to maize production in the region (Murray-Tortarolo et al., 2018). There are several options for reducing water use in this barley-maize production system. Water use in irrigation is commonly reduced by investing in advanced irrigation systems such as sprinklers or drip irrigation (Yadvinder-Singh et al., 2014; Sandhu et al., 2019); however, the costs of the necessary equipment are high and may not be cost effective in the barley-maize production system. Ideally, in a system with low profitability, like the maize-barley system, less costly interventions that increase water use efficiency are identified. For example, the laser leveling of unleveled fields, which can save up to 50% of irrigation water and increase crop yields (Jat et al., 2009, 2015), or the training and incentivizing of irrigators, who currently have no incentive to make irrigation more efficient, to reduce water use. Well-executed furrow irrigation can be relatively efficient compared to advanced irrigation systems, with irrigation efficiencies of 45–80% depending on the configuration, while sprinkler irrigation systems can reach 65–90% efficiency, and drip irrigation systems 80–95% (Postel et al., 2001; Irmak et al., 2011), so improving the furrow irrigation system may be the most economical option.
In furrow irrigation, irrigation efficiency depends mainly on inflow rate, cut-off time, furrow length and runoff (Eldeiry et al., 2005). Infiltration rate and surface roughness also determine irrigation efficiency, but are often considered as constants in a field; however, increasing infiltration and surface roughness to increase advance rate could be achieved by agronomic interventions such as implementing conservation agriculture (CA) (Verhulst et al., 2011). Jat et al. (2009) reported a 12–20% reduction in water use by implementing zero tillage, which was combined with a higher soil aggregate stability and infiltration rate. Wagger and Cassel (1993) found no difference in water use efficiency between tilled and direct seed maize production in a experiment in the southeastern USA, while Sidhu et al. (2019) and Choudhary et al. (2020) found increased water use efficiency with zero tillage in the rice-wheat system in India. In a long-term experiment in San Luis Potosi, CA led to increased soil health, resulting in an increase in infiltration rate, improved irrigation, and a higher maize yield (Fonteyne et al., 2019; Martínez Gamiño et al., 2019), but a study across 20 sites in Mexico indicated that CA generally increased maize yields, while improvements in infiltration rate were site-specific (Fonteyne et al., 2021). The combination of improved irrigation systems and CA could potentially reduce water consumption even more (Sidhu et al., 2019). Although CA could potentially increase irrigation efficiency, the relationship between water use in furrow irrigation and tillage has not been studied thoroughly (Bryant et al., 2020).
To evaluate potential water saving practices, we set up a field experiment in Guanajuato, Mexico, to compare water use with two tillage systems [conventional tillage (CT) and CA] and two irrigation systems (furrow irrigation and drip irrigation) in barley and maize production from 2016 to 2020. Additionally, we compared water use in CT and CA in 20 side-by-side comparisons in farmer's fields. Our hypothesis was that drip irrigation, CA and the combination of these can reduce water use in barley and maize production in the Mexican Bajío in compared with the conventional practice of furrow irrigation and CT.
The field experiment was conducted at the Ex-Hacienda El Copal site of the Universidad de Guanajuato in Irapuato, Guanajuato, Mexico (N20.745, W101.327) at an elevation of 1,742 m above sea level (Figure 1). The site has a semi-arid climate with an average precipitation of 700 mm falling mainly between June and October, and a Vertisol soil type (Figure 2). At the beginning of the experiment, the soil had an organic matter content of 2.1%, an electrical conductivity of 6.8 dS m−1 and a bulk density of 1.29 g cm−3. Moisture tension at field capacity is 8 kPa at a volumetric moisture content of 46.8%, while moisture tension at the permanent wilting point is 295 kPa at a volumetric moisture content of 23.6%. Reference evapotranspiration exceeds precipitation by an average of 800 mm per year between the months of November and April. The average temperature is 18oC, with the highest temperatures and reference evapotranspiration in the months of April and May when the barley crop is ripening. The experiment was initiated in November 2016 and terminated in June 2020.
Figure 1. Location of field experiment (blue square) and farmers' fields (green circles) in the Mexican Bajío.
Figure 2. Mean monthly maximum and minimum temperatures, mean monthly precipitation and mean monthly evapotranspiration during the experiment (November 2016–June 2020).
Concurrently with the field experiment, side-by-side comparison experiments of CT and CA were installed in collaboration with local farmers (Figure 1). Twenty different fields with similar characteristics to the site of the field experiment were selected in the barley-producing areas of the Bajío region in the states of Guanajuato and Querétaro.
The fields belonged to farmers involved in the “Cultivando un Mexico Mejor” project, funded by Heineken. They were supported by farm advisors in implementing CA and other sustainable agricultural practices in their fields. The side-by-side experiments were implemented to evaluate the experimental treatments under real farm conditions, but also as part of CIMMYT's extension strategy, which relies on field validation and demonstrations, and on co-design of interventions with local stakeholders (Gardeazabal et al., 2021; Govaerts et al., 2021).
The treatments of the field experiment consisted of two tillage practices: CT and CA and two irrigation methods: furrow irrigation (FI) and drip irrigation (DI). Each of the tillage systems was evaluated with both irrigation methods (Table 1). The experiment evaluated four treatments in a randomized complete block design with two replications. Plots were 18.24 m wide and 62 m long. A 1.5 m border was maintained between plots with drip irrigation, and a 3.6 m border between plots with furrow irrigation. The field was laser-leveled before the initiation of the experiment.
Table 1. Treatments evaluated in the field experiment in Irapuato, Mexico from November 2016 to June 2020.
Conventional tillage consisted of two passes with a disk plow, followed by two passes with a disc harrow, and raised bed formation before planting barley. Maize was direct seeded in the barley stubble. All residues were removed. In the barley cycle in the winter of 2018–2019 tillage was not possible due to atypical rains in December, and the crop was direct seeded in all treatments; the only difference from CA in that year was the removal of the crop residues. CA consisted of preparing the field as for the conventional practice in November 2016, after which the raised beds were used for the duration of the experiment, with furrow reformation only before planting. To provide soil cover, 50 to 100% of the crop residues were left in the field. Furrow irrigation was provided through a gated pipe, while the drip irrigation system consisted of polyethylene laterals with an inside diameter of 10 mm laid parallel to crop rows. The laterals were provided with in-line emitters of 0.75 to 1 L h−1 capacity at a pressure of 103 kPa and spaced at 20 cm, so that the complete bed area was wetted. Irrigation was applied when the moisture sensors at 30 cm indicated a moisture tension of 60 kPa, given that at the site the range of readily available moisture is between 8 and 60 kPa. One irrigation was given after sowing and three to four irrigations were given during the growing season (Table 2).
Table 2. Moment [Days after sowing (DAS)] and quantity of irrigation applied (m3 ha−1) per treatment and growing cycle.
Barley was sown at the end of December and harvested at the end of April or beginning of May. The cultivar sown was Esperanza in 2016, 2017 and 2018; in 2019 the cultivar Prunella was sown, which is a newer cultivar with the same yield potential but with a better malting quality. Sowing was done at a rate of 110 kg ha−1 in 6 rows per bed. Beds were 1.52 m wide from furrow to furrow. Barley fertilization was 210-90-00 NPK and a commercial micronutrient mixture, with 110-90-00 applied at sowing and 100-00-00 applied before stem extension. Weeds were controlled with one application of prosulfuron (40 g a.i. ha−1), and aphids with one application of pirimicarb (500 g a.i. ha−1).
Maize was sown at the end of May each year and harvested at the end of November. The hybrid sown was XR20A (Ceres) in 2017 and 2061Y (Dekalb) in 2018 and 2019, both yellow maize hybrids. Maize was direct seeded in all treatments, as that is the most common farmer's practice in the region, at a rate of 90,000 seeds ha−1 in two rows per bed with a distance of 76 cm between rows, using a SD-2011-DSC-4 direct seeder by Sembradores del Bajio. Maize fertilization was 260-80-00 kg NPK ha−1 and a commercial micronutrient mixture. One hundred and thirty kg ha−1 of N and all the P were applied at sowing and 130 kg ha−1 of N was applied at the six leave stage. Weeds were controlled with tembotrione (300 ml a.i. ha−1), atrazine (1 kg a.i. ha−1) and mesotrione (60 g a.i. ha−1). Fall armyworm (Spodoptera frugiperda) was controlled using spinetoram (75 ml ha−1) or emamectin benzoate (100 ml ha−1). One irrigation was given at sowing and more irrigation was applied if needed.
Yield and biomass samples were hand harvested in five subsamples with an area of 5 by 1.52 m (width of one bed) each. Moisture content was determined with a humidity meter and adjusted to 12% for barley and 14% for maize. WATERMARK Soil Moisture Sensors (Irrometer, Riverside, USA) were placed at 30, 60 and 90 cm depths in the middle of the central bed in each plot to measure moisture tension. Moisture tension was read every three days using a multimeter. Applied water was measured using a propeller flow meter and outflow was measured using a Cipoletty weir. Weather data was collected using an on-station weather station. Reference evapotranspiration was calculated with the Penmann-Montheith equation using the Cropwat 8.0 (FAO, Rome, Italy).
Conventional tillage and CA were compared side-by-side in the same field, except for three fields compared in 2018, when CT was implemented in one field and CA in a neighboring field. The management of the fields was decided between the collaborating farmer and the farm advisor. In CT all farmers did conventional tillage before sowing barley and most direct seeded maize, although some farmers passed a disk harrow before planting maize. Yield was determined as described in CIMMYT (2013a). Fields were irrigated according to common local practices, using either water provided by the irrigation district trough a canal or using pumped subterranean water. All fields were furrow irrigated, apart from one field with access to drip irrigation. Water was provided to the furrows either using a gated pipe or by digging an opening in the border of the canal. Water use was recorded by measuring the time of irrigation and inflow rate; in fields with gated pipe irrigation the outflow per gate was measured, while in fields irrigated with a canal the inflow was calculated using the dimensions of the canal and a flow probe. All management, yield and water-use data were captured by farm advisors through the Bitacora Electronica MasAgro (BEM). Soil health measurements were taken during the 2020–2021 barley cropping cycle. Soil was sampled from 0 to 30 cm depth in a at least 10 points per field: a composite sample was made and analyzed as described in Fonteyne et al. (2021). Penetration resistance was measured using a dynamic penetrometer (CIMMYT, 2013b). Infiltration rate was determined by infiltrating 500 ml of water using a 100 mm diameter infiltrometer, in three repetitions per plot. Fuel use was recorded from the tractor during field operations in the 2020 summer cycle and the 2020–2021 winter cycle. Greenhouse gas emissions were calculated as CO2-equivalents according to the guidelines of SEMARNAT (SEMARNAT, 2015).
With ECO2 the greenhouse gas emission in CO2-equivalents, V: the volume of fuel used (L), C the calorific value of the fuel (35.5374 MJ L−1 for Diesel fuel), FCO2 the emissions factor for CO2 (0.0000741 t CO2 MJ−1), FCH4 the emissions factor for CH4 (0.0000039 kg CH4 MJ−1) and FN2O the emissions factor for N2O (0.0000039 kg N2O MJ−1).
The complete dataset is available on Dataverse at https://hdl.handle.net/11529/10548578. Water use efficiency (WUE) was calculated by dividing grain yield (kg ha−1) by the volume of applied irrigation water (m3 ha−1). Statistical analysis was performed in R 3.1.1 (R core team, Vienna, Austria). Homogeneity of variances was tested with the Levene's test using the “leveneTest” function from the “car” package. Data was normally distributed, this was tested with the Shapiro-Wilk's test using the “shapiro.test” function from the “stats” package. The effect of the treatments in the field experiment and the farmers' fields on grain yield and water use using the “glm” and “aov” functions from the “stats” package with the following model:
Where: Y is the response variable, m is the overall mean, T are the effects of the factor “tillage system” (CA or CT), I are the effects of the factor “irrigation type” (furrow or drip), R are the repetition effects and G the effects of the year. The growing cycle and the repetition were considered random factors. Each side by side comparison of CA and CT in a farmers' field was considered a repetition (n = 24 for barley and n = 10 for maize). Post-hoc analysis was performed to determine differences between treatments using the “HSD” function from the “agricolae” package which performs a Tuckey test. In 2016 CA was not yet established, as the field had to be prepared before sowing, therefore the 2016–2017 barley season was not included in the analysis. Data from farmers' fields were compared using paired t-tests. Results were considered significant at P ≤ 0.05.
Barley yields varied significantly over the years, as the average yield was 4.80 t ha−1 in 2018, and 7.48 t ha−1 and 7.41 t ha−1 in 2017 and 2019 respectively, but there was no significant interaction between year and tillage or irrigation type (Table 3). Yields were lower in 2018 because unseasonal rains in November and December 2017 delayed sowing, and late sowing reduces yield. Overall, drip irrigation obtained significantly higher yields, with an average yield of 6.40 t ha−1 using drip irrigation and 6.00 t ha−1 using furrow irrigation. CA resulted in a slightly higher yield than CT (P = 0.088), with an average yield of 6.39 t ha−1 under CA and 6.05 t ha−1 under CT. There was a marginally significant interaction between tillage type and irrigation type (P = 0.068). When analyzed per irrigation type, there was no difference between CA and CT with drip irrigation, but with furrow irrigation, CA yielded significantly more than CT. Under CA there was no difference in yields between the two types of irrigation, but under CT, drip irrigation yielded significantly better than furrow irrigation. Maize yields were significantly higher in 2018, with an average yield of 16.4 t ha−1 compared to an average of 12.7 t ha−1 and 11.3 t ha−1 in 2017 and 2019 respectively. Maize yield was not significantly different between treatments overall (Table 3). Yields were slightly higher under CA than under CT (P = 0.082), with an average yield of 12.6 t ha−1 under CA and 11.9 t ha−1 under CT. With drip irrigation there was no difference between yields, while with furrow irrigation the average yield was higher under CA than under CT (P = 0.152).
Table 3. Mean and standard error of barley grain yield and water use efficiency per treatment and per growing cycle.
Barley required 4 to 7 irrigations with drip irrigation and 3 to 4 irrigations with furrow irrigation (Table 2). Barley irrigation water use varied between 2,900 m3 ha−1 under CA-DI in 2018 and 6,230 under CT-FI in 2017. The conventional treatment, CT-FI, used on average 5,208 m3 ha−1 of irrigation water to produce a crop. CA-FI used 898 m3 ha−1 (17%) less water than CT-FI, while CT-DI used 1,058 m3 ha−1 (20%) less water than CT-FI. Combining CA and DI (CA-DI) saved on average 1441 m3 ha−1 (27%) compared to CT-FI. Outflow in furrow irrigation was similar in CA and CT, with on average 740 m3 ha−1 in 2017 and 340 m3 ha−1 in 2019. Maize was mostly rainfed so required only 1 irrigation in 2017 and two irrigations in 2018 and 2019, because of the later onset of the rains in those years. Maize irrigation water use varied between 800 m3 ha−1 in 2017 under drip irrigation and 3,025 m3 ha−1 under CT-FI in 2018 (Table 3). CT-FI required on average 2,658 m3 ha−1 of irrigation water for maize production. CA-FI used on average 458 m3 ha−1 (17%) less water than CT-FI, while both CA-DI and CT-DI saved 1,762 m3 ha−1 (66%) with drip irrigation. On a yearly basis, CT-FI required on average 7,866 m3 ha−1 for both crops, while CT-DI needed 5,046 m3 ha−1, CA-FI 6,510 m3 ha−1 and CA-DI 4,663 m3 ha−1. Changing from CT to CA while maintaining furrow irrigation would thus save 1,356 m3 ha−1 (17%) yearly, while installing drip irrigation in CT would save 2,820 m3 ha−1 (36%) yearly. Combining both CA and drip irrigation would save 3,203 m3 ha−1 (40%) of irrigation water yearly.
Water use efficiency differed significantly between the treatments. For barley, CA-DI had a significantly higher WUE than the other treatments with an average WUE of 1.06 kg m−3 (Table 3). CA-FI and CT-DI had a similar WUE at 1.42 kg m−3 and 1.41 kg m−3, respectively. CT-FI had a significantly lower WUE than the other treatments with 1.06 kg m−3. CA had a significantly higher WUE than CT across both irrigation systems and drip irrigation had a significantly higher WUE across both tillage systems. For maize, over all the years, the two treatments with drip irrigation had a significantly higher WUE than the other treatments, and CA-FI had a significantly higher WUE than CT-FI (Table 3).
Moisture tension sensors at 30 cm depth indicated that CA treatments retained moisture longer than CT treatments (Figure 3). A similar tendency was observed at 60 cm depth, while at 90 cm depth there was little difference between tillage systems (Supplementary Figures 1, 2). This effect was more pronounced after the first two irrigations at the beginning of the growing season, when moisture loss was mainly due to evaporation, than after later irrigations, when moisture loss was mainly due to transpiration. In the winter of 2018–2019, under CA and DI, one irrigation could be avoided due to slower drying out, allowing a saving of 750 m3 ha−1 of irrigation water over the course of the growing season. At the end of the barley growing season, no irrigation is applied as the crop is left to dry. This is reflected in the moisture tension reaching values over 100 kPa. During the maize growing season, moisture tension sensors indicated that CA treatments retained moisture longer than CT treatments, although moisture tension at 30 cm depth remained below 60 kPa for most of the growing season (Figure 4). In 2017 and 2019 there were dry periods during the maize growing season; during these dry periods, at 30 cm CT treatments reached a moisture tension higher than 60 kPa several times, while under CA no moisture stress occurred. In 2018, a wet year, moisture tension at 30 cm depth was near zero for most of the growing season, indicating almost complete saturation. At 60 and 90 cm depth, the soil was saturated with moisture for most of the maize growing season (Supplementary Figures 3, 4).
Figure 3. Moisture tension at 30 cm depth during the barley growing season, separated per irrigation type. The dotted line indicates the limit of 60 kPa of usable moisture.
Figure 4. Moisture tension at 30 cm depth during the barley growing season, separated per irrigation type. The dotted line indicates the limit of 60 kPa of usable moisture.
Comparison of CA and CT in farmers' fields corroborated the results of the field experiment under the conditions of the farmers of the Bajio. Water use for barley was on average 7236 ± 2411 m3 ha−1 under CT while it was only 6041 ± 2181 m3 ha−1 under CA (Table 4), an average reduction of 1,195 m3 ha−1 or a 17% reduction in water use (Table 4). For maize the reduction in water use was lower, 723 m3 ha−1 on average, because most of the water was supplied through rainfall; however, this still amounted to a 16% reduction in irrigation water use. Yields were higher under CA: the average increase was 0.47 t ha−1 for barley and 0.59 t ha−1 for maize. While the difference in water use for barley was only marginally significant (P = 0.0827), the combination of higher yields and lower water use led to a significantly higher WUE for barley under CA compared to CT (P = 0.0101). Drip irrigation was only evaluated in one field during two consecutive crop cycles: due to high costs the farmer did not want to continue after those cycles. When CA and CT were compared in the same field with drip irrigation, water savings were similar to the other fields based on the data from the one field where this was evaluated; however, compared with the average water use when using furrow irrigation in CT in the same year, CA + DI reduced water use by 5,821 and 2,540 m3 ha−1 for barley and maize respectively.
Table 4. Average water use, yield and water use efficiency with standard deviation in CT and CA in farmers' fields with side-by-side comparisons of CT and CA.
Soil health measurements in side-by-side comparisons indicated improvements in soil health under CA. Soil organic matter was significantly higher under CA, with an average of 2.93% under CA and 2.27% under CT (Figure 5). Soil compaction was significantly lower under CA, with a penetration resistance of 9.2 kg cm−2 for CA and 13.0 kg cm−2 for CT. Consequently, the average infiltration rate was higher under CA than under CT, with 12.0 mm min−1 for CA and 4.75 mm min−1 for CT, but the difference was not significant (P = 0.13) due to large variation between fields. Apart from the reduction in water use and improvement in soil health under CA, the practice had additional benefits in terms of reducing the climate impact of agriculture. Diesel use was lower in CA mainly due to the reduction in field preparation (Table 5). In the summer cropping cycle in 2020, on average 41.0 L diesel fuel ha−1 were needed for field preparation for CT, while CA used on average 10.3 L diesel fuel ha−1, mainly for furrow reformation; in the winter cropping cycle of 2020–2021 on average 47.3 L diesel fuel ha−1 were needed for field preparation for CT, while CA used on average 9.0 L diesel fuel ha−1. In terms of greenhouse gas emissions this amount to a yearly emission of 514 kg CO2 equivalent ha−1 in CT, while emission are approximately 37% lower in CA, with 322 kg CO2 equivalent ha−1.
Table 5. Fuel use per operation type and associated CO2-equivalent greenhouse gas emissions per crop and production system in the farmers field experiments in the summer cycle of 2020 for maize and the 2020–2021 autumn-winter cycle for barley.
Substantial reductions in irrigation water use are possible in the barley-maize production system in the Mexican Bajío. CA reduced water use by ~20% in comparison with the conventional production system; drip irrigation reduced water use by about 30% in comparison to furrow irrigation, while combining both practices reduced water use by ~40% on a yearly basis. In farmer's fields, the irrigation quantity and the management factors depended on the conditions of each field and preferences of each farmer. However, even though the conditions in the farmer's fields were less controlled, similar results were observed under those conditions as under controlled conditions in the field experiment. The effect of CA on WUE was even greater in farmers' fields than in the field experiment, probably because the farmers' fields were larger and therefore less precisely irrigated, while the shorter furrows in the field experiment allowed for more efficient irrigation (Zerihun et al., 1997). If CA could be applied in the 60,000 ha of irrigated barley in Guanajuato and Querétaro (SIAP, 2020), the ~1,000 m−3 ha−1 of irrigation water saved per hectare that was observed in the farmers' fields would amount to ~60 Mm3 in irrigation water saved per growing season, while simultaneously reducing greenhouse gas emissions by ~11,520 t CO2 equivalents. The reduced irrigation requirements would bring substantial savings in pumping costs and aquifer depletion, but might also be used to increase production by increasing irrigated cropland (Fishman et al., 2015). However, according to Jägermeyr et al. (2016), if farmer-implementable practices such as CA or water harvesting could be implemented on a global scale, this would substantially increase agricultural production by 18–41% through the expansion of irrigated land, while simultaneously reducing groundwater withdrawal rates; thus, the implementation of CA could both increase agricultural output and reduce water use in the Bajío. Our water-use findings were similar to those found in studies in the double-cropped intensive production systems in northwestern India, where CA reduced irrigation water use by about 20% in comparison with CT, while increasing maize, wheat and rice yields and improving profitability (Das et al., 2014; Parihar et al., 2016; Jat et al., 2019). In the same region, drip irrigation can reduce water use by 32% in wheat and 65% in maize (Sandhu et al., 2019). The similarity between the results from our dedicated field experiment, the farmers' fields and the scientific literature shows that the implementation of CA is a viable option for reducing agricultural water use.
Drip irrigation increased barley yields, which may have been an effect of reduced flooding of the root zone that creates anoxic conditions. After furrow irrigation the soil is saturated completely with water, and the plant suffers anoxic conditions for several days. With drip irrigation the soil is not completely saturated, and plants suffer no stress from flooding. The soil moisture sensors indicated that soil under CA retained moisture for longer; this helps the crop to overcome periods of drought stress, which is common with surface water irrigation that is available according to schedule and not according to crop needs because distribution is decided centrally for the whole irrigation district. In irrigated wheat under CA, Verhulst et al. (2011) found that yields were similar under full irrigation, but under reduced irrigation CA yielded 19–26% more. In our experiment the soil under barley in CA had a higher moisture content at each irrigation, which may have led to a lower irrigation requirement. With maize, drip irrigation had no significant effect on yield because maize is mostly rainfed, and there was little difference in moisture conditions between treatments. Both CA and drip irrigation increased maize yields by ~1 t ha−1 in our field experiment, although the difference was not statistically significant. In farmer's fields, CA also increased barley and maize yields, even though a range of different varieties, fertilizations and crop management practices were applied by the cooperating farmers.
The lower water use in CA compared to the CT system is likely due to several factors, such as increased infiltration rate through improved soil structure, less evaporation as a result of using residues for soil cover (Zhang et al., 2005; Ranaivoson et al., 2017), and higher moisture retention with increased organic matter (Govaerts et al., 2007; Basche and DeLonge, 2019). Since the relationship between tillage and irrigation water use has been little studied, it is unclear which aspects of CA contribute the most to water saving, whether it is the soil cover, the improved soil health, increased infiltration rate, or another aspect. Further study of the factors that lead to water use reduction and yield increases under CA with furrow or drip irrigation, as well as further studies in different production systems, is desirable to further develop CA as a water-saving practice. Reduced irrigation costs and higher yields make both CA and drip irrigation attractive options for farmers. However, while CA reduced production costs, drip irrigation required a high initial investment. In the long term, it is likely that yields would increase further due to improved soil health, while production costs may be reduced through the reduction in tillage and weed management (Fonteyne et al., 2019, 2020; Wall et al., 2020). Drip irrigation was evaluated in one farmer's field but was discontinued because it was not profitable due to the high costs. Experience with drip irrigation for cereals in the region is lacking however, it is likely that a well-managed drip fertigation system could increase yields and reduce fertilization costs enough to offset the investment in drip irrigation, especially for maize (Sidhu et al., 2019). Additionally, the dropping aquifer levels make pumping ever more costly, so saving water becomes more profitable every year. Drip irrigation would be most beneficial for farmers with the use of subterranean pumped water, while when using surface water there is no incentive to save water as it does not reduce costs, even though collective action could increase water availability for everyone. Overall, to reduce water use in grain production, CA appears the most rapidly scalable option as it reduces costs and increases profits, while drip irrigation needs further improvement to be a profitable option.
Conservation agriculture with both furrow irrigation and drip irrigation could be further improved to increase water savings and profitability. In furrow-irrigated CA, improved irrigation methods such as surge irrigation or irrigating alternate furrows should be evaluated in terms of savings in water use. Since furrow irrigation is already generally done using gated pipes, these practices could be implemented relatively easily. Drip irrigation could be made more attractive to farmers by optimizing drip fertigation for the barley-maize system in the region. Drip fertigation can reduce fertilizer use and increase yields through more timely application of the nutrients where they are needed (Sidhu et al., 2019). Drip fertigation for maize increases the efficiency of applying nutrients compared to the flood irrigation system (Sampath Kumar and Pandian, 2010). In the Bajío, maize yields of 20 t ha−1 and barley yields of 8 t ha−1 are possible given optimal management (Saldivia Tejeda et al., 2020). Drip fertigation could help to attain those yields consistently, which would make the investment economical. Furthermore, the use of sensors, weather data and modeling could improve irrigation scheduling and consequently reduce irrigation water use. Conservation agriculture, in which the soil is not tilled, could allow the installation of buried drip lines, which would drastically reduce the costs of replacing drip lines and improve water use efficiency (Sidhu et al., 2019). Problems with rodent damage and leakage should be resolved first however. An increased use of sensors to inform the optimal irrigation moment could reduce water use, as we observed in the 2019 barley season. Production systems vary widely across Mexico, and the effects of the evaluated treatments could be different in other water-stressed regions, for example in the northwest where only one crop is grown per year, or the Laguna area, where tillage is performed two or three times per year (Fonteyne et al., 2021). There is thus probably a large potential to reduce water use, but research is necessary to adapt the technologies to the conditions of the diverse cropping systems across Mexico.
Water use in the barley-maize production system of the Mexican Bajío can be reduced by the implementation of conservation agriculture or drip irrigation or the combination of both by 20 to 40%. Conservation agriculture retained moisture longer resulting in less moisture stress, which may have resulted in a higher yield in barley. Furthermore, in farmers' fields it improved soil health and reduced fuel consumption. While conservation agriculture has generally been promoted as a soil-conserving and labor-reducing practice mainly in rainfed areas, our study indicates that the promotion of conservation agriculture in irrigated regions is advisable in order to reduce irrigation water use.
The datasets presented in this study can be found in online repositories. The names of the repository/repositories and accession number(s) can be found below: CIMMYT Research Data and Software Repository Network, https://hdl.handle.net/11529/10548578.
ÁFG and SF contributed to the conception and design of the study. ÁFG performed the investigation and curated the data. SF wrote the first draft of the publication. NV supervised the project and reviewed and edited the draft. All authors contributed to revision of the manuscript, and read and approved the submitted version.
The experiments were funded by the Cultivando un Mexico Mejor project funded by Heineken. The data analysis and writing of the article was funded by CCAFS and MasAgro Guanajuato. The work of CCAFS is supported by CGIAR Fund Donors and through bilateral funding agreements. For details please visit https://ccafs.cgiar.org/donors. MasAgro Guanajuato is funded by the Secretaría de Desarrollo Agroalimentario y Rural of the state of Guanajuato. Any opinions, findings, conclusion, or recommendations expressed are those of the authors and do not necessarily reflect the view of the donors.
The authors declare that the research was conducted in the absence of any commercial or financial relationships that could be construed as a potential conflict of interest.
All claims expressed in this article are solely those of the authors and do not necessarily represent those of their affiliated organizations, or those of the publisher, the editors and the reviewers. Any product that may be evaluated in this article, or claim that may be made by its manufacturer, is not guaranteed or endorsed by the publisher.
We thank Zeferino Fernández Vera, Blanca Albarrán Contreras, Amador Aguillón Aguillón, Erick Ortiz Hernandez and Samuel Flores Galarza for their help in the project, as well as the many students involved in the trial for data collection, Juan Arista Cortes for the map, and Elizabeth Waygood for language editing.
The Supplementary Material for this article can be found online at: https://www.frontiersin.org/articles/10.3389/fsufs.2021.734681/full#supplementary-material
1. ^FAO (2021). Available online at: http://www.fao.org/faostat/en/#rankings/countries_by_commodity_exports (accessed January 7, 2021).
Basche, A. D., and DeLonge, M. S. (2019). Comparing infiltration rates in soils managed with conventional and alternative farming methods: a meta-analysis. PLoS ONE 14:e0215702. doi: 10.1371/journal.pone.0215702
Bryant, C. J., Locke, M. A., Krutz, L. J., Reynolds, D. B., Golden, B. R., Irby, T., et al. (2020). Furrow-irrigation application efficiency in mid-southern U.S. conservation tillage systems. Agron. J. 113, 397–406. doi: 10.1002/agj2.20468
Choudhary, M., Panday, S. C., Meena, V. S., Singh, S., Yadav, R. P., Pattanayak, A., et al. (2020). Long-term tillage and irrigation management practices: Strategies to enhance crop and water productivity under rice-wheat rotation of Indian mid-Himalayan Region. Agric. Water Manag. 232, 106067. doi: 10.1016/j.agwat.2020.106067
CIMMYT (2013a). Penetration Resistance: A Practical Guide for Comparing Crop Management Practices. Texcoco: CIMMYT.
CIMMYT (2013b). Yield and Yield Components: A Practical Guide for Comparing Crop Management Practices. Texcoco: CIMMYT, Mexico.
Das, T. K., Bhattacharyya, R., Sudhishri, S., Sharma, A. R., Saharawat, Y. S., Bandyopadhyay, K. K., et al. (2014). Conservation agriculture in an irrigated cotton-wheat system of the western Indo-Gangetic Plains: crop and water productivity and economic profitability. F. Crop. Res. 158, 24–33. doi: 10.1016/j.fcr.2013.12.017
Eldeiry, A. A., Garcia, L. A., El-Zaher, A. S. A., and El-Sherbini Kiwan, M. (2005). Furrow irrigation system design for clay soils in arid regions. Appl. Eng. Agric. 21, 411–420. doi: 10.13031/2013.18460
Fishman, R., Devineni, N., and Raman, S. (2015). Can improved agricultural water use efficiency save India's groundwater? Environ. Res. Lett. 10:084022. Available online at: https://iopscience.iop.org/article/10.1088/1748-9326/10/8/084022
Flores, H., Morales, J. L., Mora-Rodríguez, J., Carreño, G., and Delgado-Galván, X. (2018). Management priorities for aquifers in El Bajío in Guanajuato state, Mexico. Water Policy 20, 1161–1175. doi: 10.2166/wp.2018.202
Fonteyne, S., Burgueño, J., Albarrán Contreras, B. A., Andrio Enríquez, E., Castillo Villaseñor, L., and Enyanche Velázquez, F.. (2021). Effects of conservation agriculture on physicochemical soil health in 20 maize-based trials in different agro-ecological regions across Mexico. L. Degrad. Dev. 32, 1–15. doi: 10.1002/ldr.3894
Fonteyne, S., Martinez Gamiño, M.-A., Saldivia Tejeda, A., and Verhulst, N. (2019). Conservation agriculture improves long-term yield and soil quality in irrigated maize-oats rotation. Agronomy 9:845. doi: 10.3390/agronomy9120845
Fonteyne, S., Singh, R. G., Govaerts, B., and Verhulst, N. (2020). Rotation, mulch and zero tillage reduce weeds in a long-term conservation agriculture trial. Agronomy 10:962. doi: 10.3390/agronomy10070962
Gardeazabal, A., Lunt, T., Jahn, M. M., Verhulst, N., Hellin, J., and Govaerts, B. (2021). Knowledge management for innovation in agri-food systems: a conceptual framework. Knowl. Manag. Res. Pract. doi: 10.1080/14778238.2021.1884010
Govaerts, B., Fuentes, M., Mezzalama, M., Nicol, J. M., Deckers, J., Etchevers, J. D., et al. (2007). Infiltration, soil moisture, root rot and nematode populations after 12 years of different tillage, residue and crop rotation managements. Soil Tillage Res. 94, 209–219. doi: 10.1016/j.still.2006.07.013
Govaerts, B., Negra, C., Camacho Villa, T. C., Chavez Suarez, X., Espinosa, A. D., Fonteyne, S., et al. (2021). One CGIAR and the integrated agri-food systems initiative: from short-termism to transformation of the world's food systems. PLoS ONE 16:e0252832. doi: 10.1371/journal.pone.0252832
Hoogesteger, J., and Wester, P. (2017). Regulating groundwater use: the challenges of policy implementation in Guanajuato, Central Mexico. Environ. Sci. Policy 77, 107–113. doi: 10.1016/j.envsci.2017.08.002
Irmak, S., Odhiambo, L. O., Kranz, W. L., and Eisenhauer, D. E. (2011). Irrigation Efficiency and Uniformity, and Crop Water Use Efficiency. Lincoln, LN: Biological Systems Engineering. Available Online at: https://digitalcommons.unl.edu/cgi/viewcontent.cgi?article=1455&context=biosysengfacpub
Jägermeyr, J., Gerten, D., Schaphoff, S., Heinke, J., Lucht, W., and Rockström, J. (2016). Integrated crop water management might sustainably halve the global food gap. Environ. Res. Lett. 11:025002. doi: 10.1088/1748-9326/11/2/025002
Jat, H. S., Sharma, P. C., Datta, A., Choudhary, M., Kakraliya, S. K., Yadvinder-Singh, et al. (2019). Re-designing irrigated intensive cereal systems through bundling precision agronomic innovations for transitioning towards agricultural sustainability in North-West India. Sci. Rep. 9, 1–14. doi: 10.1038/s41598-019-54086-1
Jat, M. L., Gathala, M. K., Ladha, J. K., Saharawat, Y. S., Jat, A. S., Kumar, V., et al. (2009). Evaluation of precision land leveling and double zero-till systems in the rice-wheat rotation: Water use, productivity, profitability and soil physical properties. Soil Tillage Res. 105, 112–121. doi: 10.1016/j.still.2009.06.003
Jat, M. L., Singh, Y., Gill, G., Sidhu, H. S., Aryal, J. P., Stirling, C., et al. (2015). “Laser-assisted precision land leveling impacts in irrigated intensive production systems of South Asia,” in Soil-Specific Farming, eds R. Lal and B. A. Stewart (Boca Raton, FL: CRC Press), 323–351.
Martínez Gamiño, M. Á., Osuna Ceja, E. S., and Espinosa Ramírez, M. (2019). Impacto acumulado de la agricultura de conservación en propiedades del suelo y rendimiento de maíz. Rev. Mex. Ciencias Agrícolas 10, 765–778. doi: 10.29312/remexca.v10i4.1640
Murray-Tortarolo, G. N., Jaramillo, V. J., and Larsen, J. (2018). Food security and climate change: the case of rainfed maize production in Mexico. Agric. For. Meteorol. 253–254, 124–131. doi: 10.1016/j.agrformet.2018.02.011
Parihar, C. M., Jat, S. L., Singh, A. K., Kumar, B., Yadvinder-Singh Pradhan, S., et al. (2016). Conservation agriculture in irrigated intensive maize-based systems of North-Western India: effects on crop yields, water productivity and economic profitability. F. Crop. Res. 193, 104–116. doi: 10.1016/j.fcr.2016.03.013
Postel, S., Polak, P., Gonzales, F., and Keller, J. (2001). Drip irrigation for small farmers: a new initiative to alleviate hunger and poverty. Water Int. 26, 3–13. doi: 10.1080/02508060108686882
Ranaivoson, L., Naudin, K., Ripoche, A., Affholder, F., Rabeharisoa, L., and Corbeels, M. (2017). Agro-ecological functions of crop residues under conservation agriculture. A review. Agron. Sustain. Dev. 37:26. doi: 10.1007/s13593-017-0432-z
Romero, J. H., Palacios, O. L., and Escobar, B. S. (2017). Estimación de la sobreexplotación producida en el acuífero Valle de Celaya (México). Tecnol. y Ciencias del Agua 8, 127–138. doi: 10.24850/j-tyca-2017-04-08
Saldivia Tejeda, A., Albarrán Contreras, B. A., Verhulst, N., and Fonteyne, S. (2020). Avances en agricultura sustentable-Resultados plataformas de investigación, hubs Bajío e Intermedio 2010-2019. Texcoco: CIMMYT.
Sampath Kumar, T., and Pandian, B. J. (2010). Efficiency of applied nutrients and SPAD values in hybrid maize under drip fertigation. Madras Agric. J. 97, 237–241.
Sandhu, O. S., Gupta, R. K., Thind, H. S., Jat, M. L., Sidhu, H. S., and Yadvinder-Singh. (2019). Drip irrigation and nitrogen management for improving crop yields, nitrogen use efficiency and water productivity of maize-wheat system on permanent beds in north-west India. Agric. Water Manag. 219, 19–26. doi: 10.1016/j.agwat.2019.03.040
SEMARNAT (2015). Guía de usuario - Registro Nacional de Emisiones (RENE) para el reporte de emisiones de compuestos y gases de efecto invernadero. Mexico City: SEMARNAT.
SIAP (2020). Estadística de Producción Agrícola. Available online at: http://infosiap.siap.gob.mx/gobmx/datosAbiertos.php (accessed September 22, 2020).
Sidhu, H. S., Jat, M. L., Singh, Y., Sidhu, R. K., Gupta, N., Singh, P., et al. (2019). Sub-surface drip fertigation with conservation agriculture in a rice-wheat system: a breakthrough for addressing water and nitrogen use efficiency. Agric. Water Manag. 216, 273–283. doi: 10.1016/j.agwat.2019.02.019
Verhulst, N., Carrillo-García, A., Moeller, C., Trethowan, R., Sayre, K. D., and Govaerts, B. (2011). Conservation agriculture for wheat-based cropping systems under gravity irrigation: increasing resilience through improved soil quality. Plant Soil 340, 467–479. doi: 10.1007/s11104-010-0620-y
Wagger, M. G., and Cassel, D. K. (1993). Corn yield and water-use efficiency as affected by tillage and irrigation. Soil Sci. Soc. Am. J. 57, 229–234. doi: 10.2136/sssaj1993.03615995005700010040x
Wall, P. C., Thierfelder, C., Hobbs, P. R., Hellin, J., and Govaerts, B. (2020). Benefits of Conservation Agriculture to Farmers and Society. Cambridge: Burleigh Dodds Science Publishing. doi: 10.19103/AS.2019.0049.11
Wester, P., Minero, R. S., and Hoogesteger, J. (2011). Assessment of the development of aquifer management councils (COTAS) for sustainable groundwater management in Guanajuato, Mexico. Hydrogeol. J. 19, 889–899. doi: 10.1007/s10040-011-0733-2
Yadvinder-Singh Kukal, S. S., Jat, M. L., and Sidhu, H. S. (2014). “Improving water productivity of wheat-based cropping systems in South Asia for sustained productivity,” in Advances in Agronomy, Vol. 127, ed D. Sparks (Cambridge, MA: Academic Press), 157–258. doi: 10.1016/B978-0-12-800131-8.00004-2
Zerihun, D., Wang, Z., Rimal, S., Feyen, J., and Mohan Reddy, J. (1997). Analysis of surface irrigation performance terms and indices. Agric. Water Manag. 34, 25–46. doi: 10.1016/S0378-3774(97)00005-X
Keywords: irrigation, tillage, permanent raised beds, Mexico, water use efficiency, corn, CO2 emissions
Citation: Fonteyne S, Flores García Á and Verhulst N (2021) Reduced Water Use in Barley and Maize Production Through Conservation Agriculture and Drip Irrigation. Front. Sustain. Food Syst. 5:734681. doi: 10.3389/fsufs.2021.734681
Received: 01 July 2021; Accepted: 08 December 2021;
Published: 24 December 2021.
Edited by:
Gonçalo Rodrigues, University of Lisbon, PortugalReviewed by:
Yaosheng Wang, Institute of Environment and Sustainable Development in Agriculture, Chinese Academy of Agricultural Sciences (CAAS), ChinaCopyright © 2021 Fonteyne, Flores García and Verhulst. This is an open-access article distributed under the terms of the Creative Commons Attribution License (CC BY). The use, distribution or reproduction in other forums is permitted, provided the original author(s) and the copyright owner(s) are credited and that the original publication in this journal is cited, in accordance with accepted academic practice. No use, distribution or reproduction is permitted which does not comply with these terms.
*Correspondence: Nele Verhulst, bi52ZXJodWxzdEBjZ2lhci5vcmc=
Disclaimer: All claims expressed in this article are solely those of the authors and do not necessarily represent those of their affiliated organizations, or those of the publisher, the editors and the reviewers. Any product that may be evaluated in this article or claim that may be made by its manufacturer is not guaranteed or endorsed by the publisher.
Research integrity at Frontiers
Learn more about the work of our research integrity team to safeguard the quality of each article we publish.