- Institute of Aquaculture, University of Stirling, Stirling, United Kingdom
Sustainability analyses of aquaculture typically ignore the fate and value of processing by-products. The aim of this study was to characterise the nutritional content of the common processing by-products (heads, frames, trimmings, skin, and viscera) of five important finfish species farmed in Europe; Atlantic salmon (Salmo salar), European seabass (Dicentrarchus labrax), gilthead seabream (Sparus aurata), common carp (Cyprinus carpio), and turbot (Psetta maxima) to inform on best utilisation strategies. Our results indicate a substantially higher total flesh yield (64–77%) can be achieved if fully processed, compared to fillet only (30–56%). We found that heads, frames, trimmings and skin from Atlantic salmon, European seabass, gilthead seabream and turbot frames showed medium to high edible yields, medium to high lipid, and medium to high eicosapentaenoic acid (EPA) and docosahexaenoic acid (DHA) content, indicating significant potential for direct use in human food. By-products which are unattractive for use in food directly but have low ash content and medium to high crude protein, lipid and EPA and DHA content, such as viscera, could be directed to animal feed. Skin showed interesting nutritional values, but has more potential in non-food applications, such as the fashion, cosmetic and pharmaceutical industries. The results indicate potential to increase the direct food, animal feed and non-food value of European aquaculture, without an increase in production volumes or the use of additional resources. The importance of changing consumer perceptions and addressing infrastructure and legislative barriers to maximise utilisation is emphasised.
Introduction
Seafood consumption in the European Union (EU) increased by ~25% to 24.4 kg capita−1 year−1 between 2005 and 2017 (EUMOFA, 2018, 2019). The EU supply (14.61 million MT, live weight equivalent) in 2017 constitutes product from local (EU member states) capture fisheries (28%) and aquaculture production (9%), together with imports from non-EU member states derived from capture fisheries (49%) and aquaculture (14%). Norway, not an EU member state, but part of the European Economic Area (EEA), fulfils an important role, supplying 25% of total seafood imports into the EU, mainly farmed Atlantic salmon (Salmo salar) which represents 35% of the total estimated consumption of aquaculture products and 15% by volume of all fish and seafood products imported (EUMOFA, 2019). However, supply could be increased by more efficient processing and strategic value addition. Currently, processing by-products from both wild capture and aquaculture are “underutilized” in Europe as a whole (Jackson and Newton, 2016). Such under-utilisation can occur either when quantities of by-products are limited such as when fish are marketed whole or when markets for segregated by-products are undeveloped. Consequently, this results in the accumulation and discard of by-products at the processor or household level, highlighting an opportunity to increase production, while enhancing sustainability. These limitations have been acknowledged as an action point under the forthcoming EU “Farm-to-Fork Strategy” (EC, 2008, 2020) and being a key element of sustainable development goal (SDG)12, “ensuring sustainable consumption and production patterns” (UNDP, 2020).
Omega-3 (n-3) long-chain polyunsaturated fatty acids (LC-PUFA), eicosapentaenoic acid (EPA), and docosahexaenoic acid (DHA) are key micronutrients for human and animal health (Tocher et al., 2019; Hamilton et al., 2020). Additionally, seafood consumption can improve intake of other key micronutrients such as vitamin D and B12, iodine, selenium, and other minerals (Aakre et al., 2019), as well as being a good source of bioavailable protein. An estimated one third of all EPA/DHA originating from wild and farmed fish globally is discarded (Hamilton et al., 2020). A global shortage and increase in value of these nutrients creates incentives to use by-products more efficiently.
Increasing demand for low environmental impact feed ingredients for Europe's livestock and aquaculture sectors has become a major driver for more efficient strategies to use processing by-products within a circular economy. European aquaculture production is highly dependent on imported feed ingredients from both marine and terrestrial systems, such as fishmeal, fish oil, and soy (Newton and Little, 2018), which expose the sector to external economic shocks (Troell et al., 2014) and criticisms of “off-shoring” environmental impacts associated with their production. Feed production is the most significant source of environmental impact of fed aquaculture production (Bohnes et al., 2018). A continued dependence on fish meal and oil for the marine carnivorous species mainly farmed in Europe (Naylor et al., 2021) has led to sustainability concerns remaining focused on the use of marine ingredients in the context of “ocean health” (Tlusty et al., 2019) despite longstanding efforts to substitute them with alternative ingredients (Naylor et al., 2009; Froehlich et al., 2018). Such transformations, demonstrated most obviously by the high levels of soy products now fed to salmon (Newton and Little, 2018), have their own challenges, not least of which has been a shift in sustainability concerns from the oceans to sensitive terrestrial ecosystems (Newton and Little, 2018; Malcorps et al., 2019). Moreover, the substitution of marine ingredients with plant ingredients in aquafeeds risks both compromising the health and welfare of the cultured animal (Rana et al., 2009; Saito et al., 2020) and can also affect micro- and macro nutrient levels in the final consumed product (Nichols et al., 2014; Sprague et al., 2016; Saito et al., 2020). Therefore, it is important to take a larger food system approach into account, as unintended consequences of shifts in feed type used may occur along the entire value chain (Cook et al., 2015). A re-evaluation of the potential to increase the supply of marine ingredients from under-utilised by-product resources has received far less attention.
A comprehensive analysis of the nutritional value of by-products from the main aquaculture species in Europe remains largely undocumented. Norway is an example where full processing takes place and most aquaculture processing by-products are utilised, but there is still potential to increase volumes and value addition (Olafsen et al., 2014). However, elsewhere mixing by-products is still a common practise, with more directed to animal nutrition (Stevens et al., 2018). The absence of sorting and grading of fish by-products can dilute overall nutritional value and limit potential application. Stevens et al. (2018) showed that better by-product separation offered opportunities for value addition in farmed salmon in Scotland. Therefore, the main aim of this study is to characterise the nutritional content, and discuss potential industry applications, of the different by-products derived from the major farmed finfish species in Europe.
Methodology
Species and By-Products
Triplicate samples of heads, frames (the central skeleton of the fish including the tail), trimmings, skin (incl. scales) and viscera of Atlantic salmon (Salmo salar), European seabass (Dicentrarchus labrax), and gilthead seabream (Sparus aurata) were obtained from commercial processors in Norway and Spain, whereas by-products of turbot (Psetta maxima) and carp (Cyprinus carpio) were provided by local producers in Spain and Poland, respectively, to commercial specifications. All species fed formulated feed, except for common carp, which was grown on a natural diet with low levels of cereal supplementation. The fish from which the by-products originated were weighed before processing to calculate the share of each by-product. By-product components were frozen, weighed, packed and transported on ice in polystyrene boxes by air freight. The analyses were performed at the Institute of Aquaculture (University of Stirling), using internationally recognised analytical procedures and calibration standards (AOAC, 2005; ISO, 2005). All samples were kept frozen at −20°C until analysed.
Flesh Yields of the By-Products
The samples were weighed (precision ±0.01 g) before removing the edible flesh from each by-product fraction (except viscera) with a scalpel. The weight of the removed flesh was subtracted from the total weight of the by-product to determine the flesh yield (%). After completion, separated flesh and other parts were recombined for each separate sample and homogenised for further analysis.
Proximate Analyses
The proximate composition of samples was determined using standard methods (AOAC, 1990). Moisture content was determined by weighing ~1 g of homogenised wet sample and placing into a drying oven for 20 h at 105°C. Ash content was determined by weighing ~1 g of dried sample and placing into a muffle furnace overnight set at 600°C. Crude protein was measured by weighing ~0.25 g dried sample before addition of copper catalyst tablets and 5 ml sulphuric acid (analytical reagent grade, Fisher Scientific, Loughborough, UK). Samples were digested at 400°C for 1 h (Foss Digestor 2040, Foss Analytical AB Högnäs, Sweden). Total nitrogen levels were measured by Kjeldahl (Foss Kjeltec™ 2300, Foss Analytical AB, Högnäs, Sweden) and the crude protein level calculated as N × 6.25. The moisture content was used to convert results to a wet weight basis. Total lipid was extracted from homogenised wet samples using 20 vol of ice-cold chloroform/methanol (2:1, v/v) using an Ultra-Turrax tissue disruptor (Fisher Scientific, Loughborough, UK) according to (Folch et al., 1957). Non-lipid impurities were isolated by washing with 0.88% KCl and the lipid weight determined gravimetrically after evaporation of solvent using oxygen-free nitrogen and desiccation in vacuo before making up to a known concentration and storing at −20°C.
Fatty Acid Analysis
The fatty acid (FA) profile of samples were determined by fatty acid methyl esters (FAME) prepared from total lipid extracts that had undergone acid-catalysed transmethylation at 50°C for 16 h using 2 ml 1% (v/v) sulphuric acid (95%, Aristar, BDH Chemicals, Poole, UK) in methanol and 1 ml toluene (Christie, 2003). FAME were extracted and purified according to (Tocher and Harvie, 1988) and separated and quantified by gas-liquid chromatography. Individual FAME were identified by comparison to known standards (in-house marine oil and Restek 20-FAME Marine Oil Standard; Thames Restek UK Ltd., Buckinghamshire, UK), in addition to published literature (Ackman, 1980; Tocher and Harvie, 1988). Data were collected and processed using Chromcard for Windows (Version 2.11; Thermo Fisher Scientific Inc., Milan, Italy). Fatty acid content per g sample was calculated using heptadecanoic acid (17:0) as internal standard.
Statistics
Results were analysed using Minitab® v18.1 statistical software package (Minitab Inc., Pennsylvania, USA). Comparisons were made by Analysis of Variance (ANOVA; General linear model with Tukey Pairwise comparisons) between the triplicate samples (five species and five by-products) on a flesh yield, proximate composition, and EPA+DHA resolution.
Results
The by-product proportions, edible (flesh) yields, proximate composition, and fatty acid profiles of the various by-products of the European species are described below. All results are presented on a wet weight (ww) basis. Absolute values and standard deviations are available in the Supplementary Information (Nutritional Composition of the By-products), organised by species in Supplementary Tables 1–5. Not included in these tables in the Supplementary Information are the fillet yields, which have been included in Figure 1 and Table 1 within the main text.
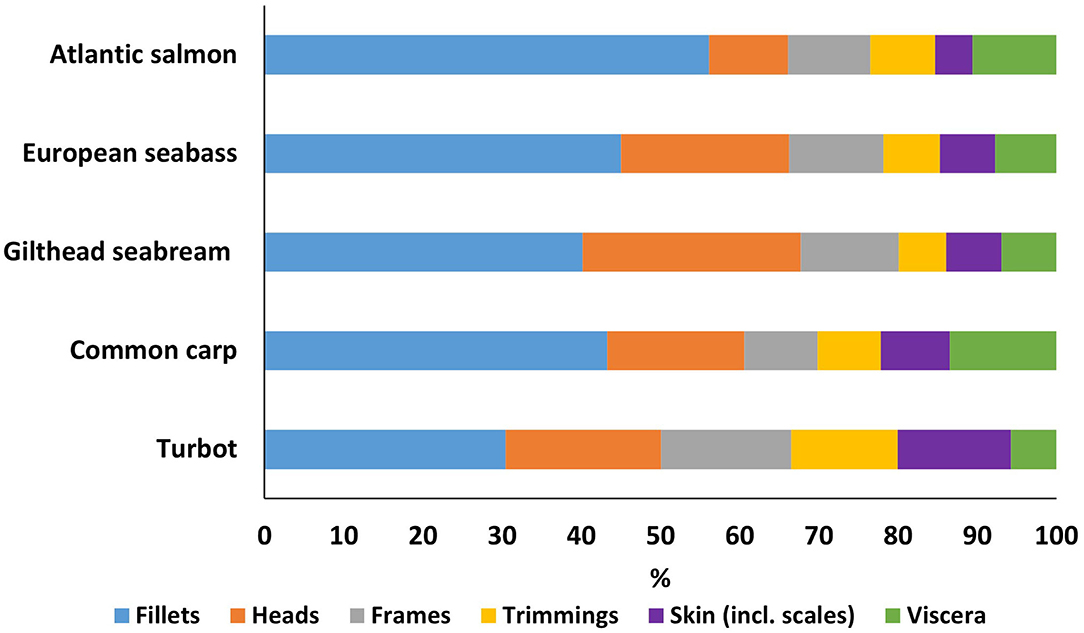
Figure 1. Proportion (%) of different processing fractions for five important European aquaculture species.
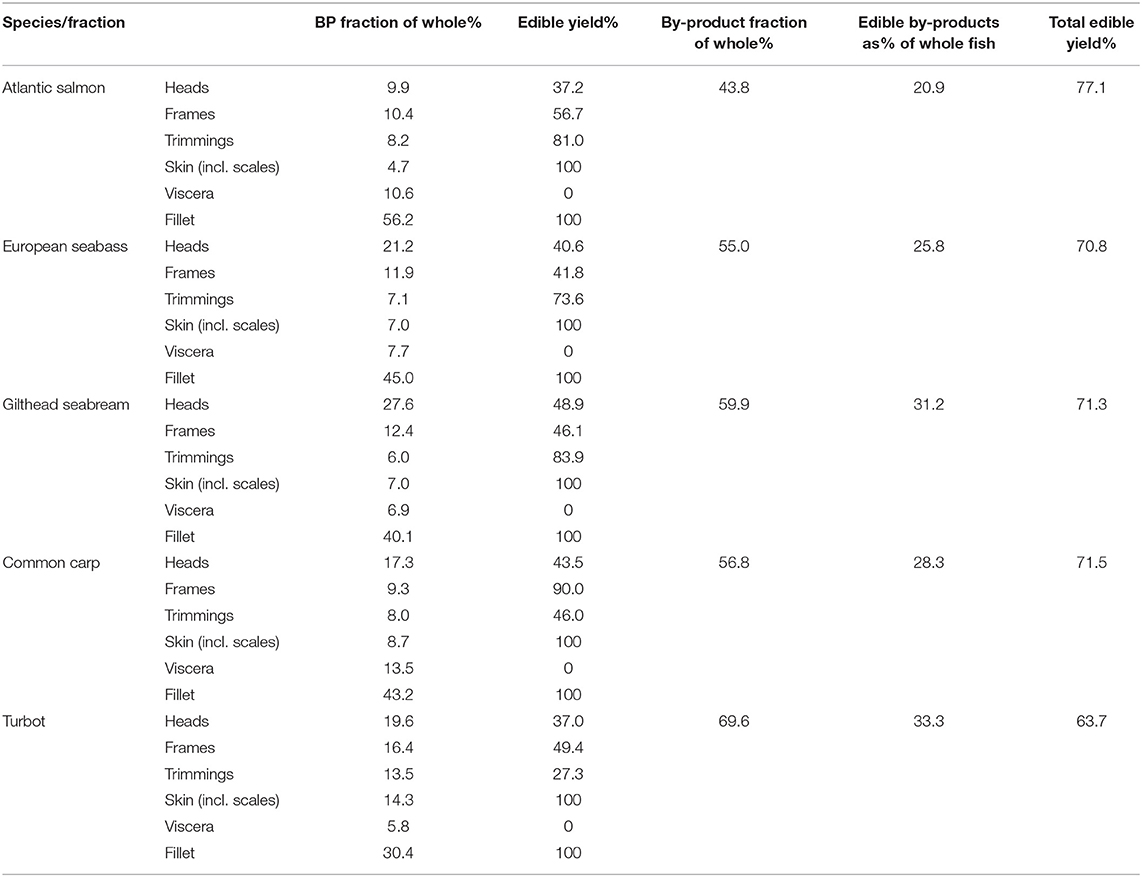
Table 1. By-product fractions and associated edible yield and total edible yield of the 5 European aquaculture species.
By-Product Proportions and Edible Yields
Fillet yield, and hence the by-product proportion, differs between species (Figure 1). Our results indicate that Atlantic salmon has the largest fillet yield of the species studied at almost 56.2 ± 1.6% of the total bodyweight, compared to turbot fillets which were the lowest at 30.5 ± 1.7% of bodyweight. Gilthead seabream showed the largest head proportion at 27.6%, while turbot had the highest share of trimmings and skin at almost 13.5 and 14.3% of the total bodyweight, respectively. Viscera proportions ranged between 5.8% (turbot) and 13.5% (common carp).
Extraction of edible components (Table 1, Figure 2A) from each of the by-product fractions, demonstrated that the potential total edible yield exceeded 70.0% of harvested yield for 4/5 of the species studied, compared to 56.2% or less from the fillet alone. Edible yields from heads showed a low variability, 37.2% (Atlantic salmon) to 48.9% (Gilthead seabream), compared to frames ranging from 41.8% (European seabass) to 90.0% (common carp) and trimmings 27.3% (turbot) to 83.9% (Gilthead seabream). Overall, total edible yields were the highest for Atlantic salmon at up to 77.1%, while turbot was at the low end with 63.7%. However, turbot showed the highest increase in total edible yield from by-products by up to 33.3%, while Atlantic salmon showed the lowest by up to 20.9% (Table 1).
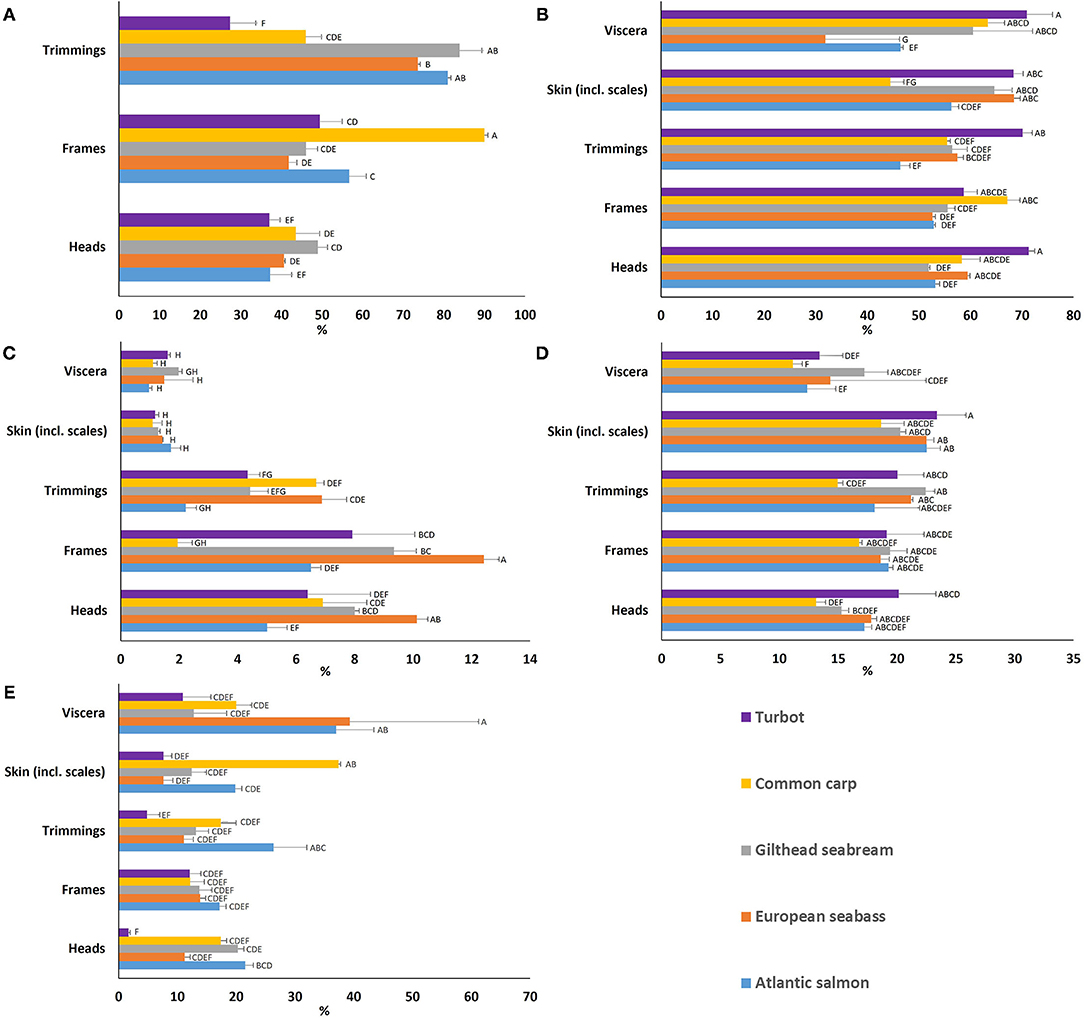
Figure 2. (A) Edible yield and proximate composition (% of wet weight) [(B) moisture, (C) ash, (D) crude protein, and (E) total lipid] of the various by-products [heads, frames, trimmings, skin (incl. scales), and viscera] for each of the European aquaculture species studied. Means that do not share letter(s) (A–H) are significantly different.
Proximate Analysis
All results are presented on a ww basis (Figure 2), and available in Supplementary Tables 1–5. Moisture variability (Figure 2B) between species was very low in heads, frames, skin, and trimmings, while viscera showed high variability ranging from 31.9% for European seabass to 70.9% for turbot. Ash content (Figure 2C) was very low between 1.0 and 2.0% for skin and viscera for all species, but more variable among species for trimmings between 2.2 and 6.9%, heads between 5.0 and 10.1%, and frames between 1.9 and 12.4%. Ash content was highest for European seabass frames at 12.4%. Crude protein (Figure 2D) values ranges were as follows; for heads between 13.1 and 20.2%, frames between 16.8 and 19.4%, and viscera between 11.1-17.2%, but was relatively constant within the skin between species at around 20%, with turbot skin at the high end at 23.4%. Trimmings exhibited similar upper values with more variability between species, with the lowest value of 15% crude protein for common carp. Total lipid content (Figure 2E) variability was low for frames, but high for heads, skin, viscera and trimmings between the species. Atlantic salmon demonstrated high lipid content in heads at 21.5%, frames at 17.2%, and trimmings at 26.4%. However, lipid levels in European seabass viscera at 39.3%, and common carp skin stood out at 37.4%. Highest variability was observed for European seabass viscera at 21.9% (Figure 2E).
EPA and DHA Content
By species, the highest EPA+DHA (g. 100 g−1 ww) values, were observed for Atlantic salmon in heads at 1.53 g. 100 g−1, trimmings at 1.74 g. 100 g−1, and skin at 1.21 g. 100 g−1 (Figure 3, Supplementary Tables 1–5). However, for by-products, Atlantic salmon viscera showed the second largest EPA+DHA content at 1.10 g. 100 g−1, after European seabass at 1.99 g. 100 g−1, and in salmon frames at 1.18 g. 100 g−1, after turbot at 1.32 g. 100 g−1.Turbot showed a relatively high EPA+DHA content in frames at 1.32 g. 100 g−1, skin at 0.85 g. 100 g−1, and viscera at 0.83 g. 100 g−1, while heads and trimmings showed significant lower values compared to most species. The lowest values were observed for common carp, the only freshwater species analysed in this study.
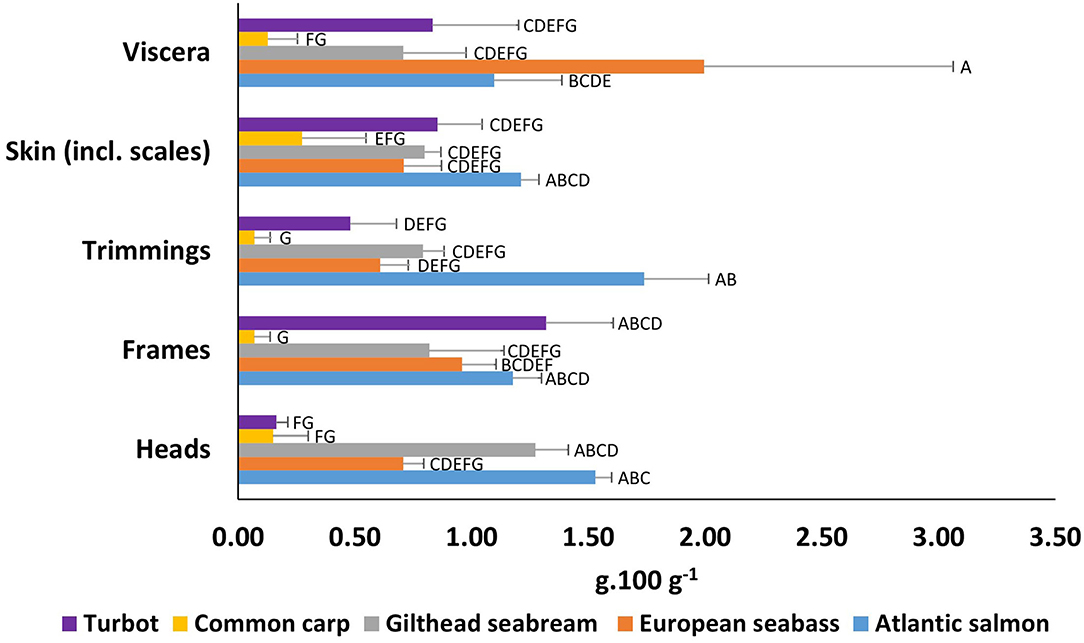
Figure 3. Total EPA+DHA content (g.100 g−1) of wet weight by-products from European aquaculture species. Means that do not share letter(s) (A–G) are significantly different.
Fatty Acid Profiles
The relative proportion of the fatty acid classes is related to the total lipid content (Figure 2E). There are similarities across the different species and their derived by-products, except for common carp (Figure 4, Supplementary Tables 1–5). Total saturated FAs are variable across the by-products and species but are relatively higher in the viscera of Atlantic salmon at 4.44 g. 100 g−1 (of ww sample), European seabass at 7.02 g. 100 g−1 and common carp at 4.07 g. 100 g−1. Additionally, common carp skin also showed relatively high absolute values for total saturated FA at 7.92 g. 100 g−1. Total n-6 PUFA showed variability and has relatively higher values for viscera of Atlantic salmon at 4.05 g. 100 g−1 and European seabass at 5.31 g. 100 g−1 and trimmings of Atlantic salmon at 3.83 g. 100 g−1.
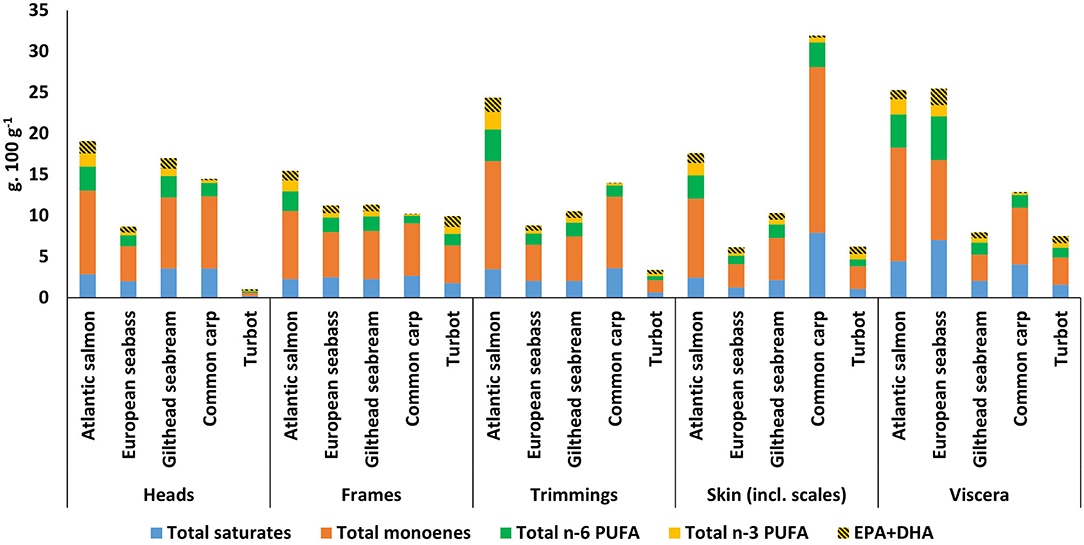
Figure 4. Fatty acid group contents (g.100 g−1 of wet weight sample) per by-product and per species (excluding 16:2, 16:3, and 16:4). The totals of the fatty acid groups could slightly differ from the total lipids (Figure 2E), as means of fatty acid groups were used. The absolute and % of fatty acid group values and standard deviations are available in the Supplementary Information (Supplementary Tables 1–5).
The highest inclusion of n-3 PUFA can be found in the by-products of Atlantic salmon, ranging between 3.88 g. 100 g−1 for trimmings and 2.47 g. 100 g−1 for frames. European seabass viscera also showed a high value at 3.40 g. 100 g−1. The content of EPA+DHA as a percentage of total n-3 PUFAs showed variability averaged across heads, frames, trimmings, skin (incl. scales) and viscera of 44.6% (±4.5) for salmon, 64.1% (±3.8) for European seabass, 58.1% (±0.8) for gilthead seabream and 61.7% (±5.8) for turbot. However, common carp showed lower EPA+DHA values across by-products with an average of 29.8% (±5.2) as a percentage of total n-3 PUFAs.
Discussion
Improved utilisation of the “whole fish” is a key component of the sustainable intensification of seafood value chains (Little et al., 2018), and through reducing waste supporting progress toward ensuring sustainable consumption and production patterns (UN, 2020). Our analysis on the potential total edible yields showed a range of 64–77% for turbot and Atlantic salmon, respectively, while fillet yields between these species range significantly between 30 and 56%, respectively. Total edible yields could potentially double compared to fillet only depending on the type of species, and total edible yield is much more similar between species than from fillet alone. A focus on the nutritional value of the by-products is required to get the best out of these high edible yields. In the following sections the nutritional value is discussed in relation to available literature and the food recovery hierarchy for fish by-products (Stevens et al., 2018; USEPA, 2020), followed by challenges associated with the strategic utilisation of by-products and future research perspectives.
Nutritional Characteristics of the By-Products
Most of the nutritional differences between our study and assessed literature could be explained by age, sex, environmental factors, and nutrient intake (Huss, 1995). The latter could explain the high variability for most nutritional parameters within viscera for all European species, as viscera is often the area where most lipid deposition occurs especially if feed is not balanced, or fish is overfed. In addition, efficiency of the investigators in terms of obtaining flesh from bones and heads, differences in origin (aquaculture vs. fisheries), intensity (intensive vs. extensive) production systems (land vs. cage culture) harvest size and weight. Homogenisation strategies, i.e., if whole fish are homogenised or analysis is made on tissue samples can result in overlooking the retention of nutrients in certain by-product fractions. For example one study from Aas et al. (2019a) found that salmon retained only 37% of EPA+DHA from which 23% was retained in the fillets and the rest in by-products, explained by a disproportionate accumulation of fat in the viscera relative to the muscle (Aas et al., 2019b).
Our findings for Atlantic salmon when compared to that of Stevens et al. (2018), found significant differences in edible yield for heads (37 and 25%), frames (57 and 32%), and trimmings (81 and 68%, trimmings and belly flaps combined), respectively, despite lab conditions and methods being similar. The higher edible yield in our analysis is likely to affect the nutritional composition of the homogenised sample, explaining some of the nutritional differences. The differences in EPA+DHA content (Supplementary Table 1) could be explained by the nutritional content of the feed reflected in the fatty acid content of the harvested fish (Sprague et al., 2016). Marine ingredient inclusion in Norwegian salmon feed has declined drastically in the last three decades (Ytrestøyl et al., 2015) in comparison to fish farmed in Scotland (Shepherd et al., 2017).
Our findings for European seabass from Spain (Supplementary Table 2) were relatively similar with the values for seabass sourced from an intensive and extensive farm in Italy, and their derived homogenised by-products (Messina et al., 2013). Difference in processing cuts could also explain the higher skin lipid content (17%) in the study of Munekata et al. (2020) from seabass homogenised by-products sourced at a Spanish market, as our homogenised skin sample (8%) had flesh attached to it.
Gilthead seabream by-products (Supplementary Table 3) had similar nutritional values compared to the study of Pateiro et al. (2020), who used fishery derived male seabream purchased from a local market in Spain, and their derived by-products. However, the head proportion from this study was 28 ± 0.5%, while Pateiro et al. (2020) reported 21%. Additionally, skin, moisture, total lipid and DHA content differed with Pateiro et al. (2020) for reasons already stated.
Polish common carp aquaculture is characterised by “natural” extensive production systems (Raftowicz and Le Gallic, 2019), and is the only freshwater species assessed in this study. Lipid content of homogenised by-product samples was higher, while EPA and DHA was lower (Supplementary Table 4), compared to the muscle tissue samples from three (intensive, low-intensive, and semi-extensive) production systems (Kłobukowski et al., 2018). While sample difference could explain the differences, production systems and their associated feed ingredient use could impact the fatty acid profile according to the study of Kłobukowski et al. (2018). EPA and DHA is more common in the marine food chain, but some freshwater species could also serve as a source of EPA and DHA. However, in general the freshwater food chain contains higher levels of linoleic acid (LOA) (C18:2 n-6) and alpha-linolenic acid (ALA) (C18:3 n-3) (Strobel et al., 2012). Nevertheless, common carp requires n-3 and n-6 fatty acids in their diet (Takeuchi et al., 2002; Glencross, 2009), which can be manipulated through enriched feed (Eljasik et al., 2020).
The nutritional results for farmed turbot are relatively similar to other literature with the exception of lipid and ash results, which are much higher for frames, skin, and viscera (Supplementary Table 5) compared to the values shown in Martinez et al. (2010). While our DHA results are relatively similar to Martinez et al. (2010), our EPA values are significantly lower. Differences can be explained by the fact that Martinez et al. (2010) used fillet muscle samples rather than homogenised by-product fractions.
Strategic Utilisation Pathways
It should be a top priority to strategically manage by-products to maximise the edible yield from whole fish for direct human consumption, according to the Food Recovery Hierarchy for Fish By-products (Stevens et al., 2018). Heads, frames, and trimmings from all species assessed in this study show potential to increase the food supply. For example, 10% of the Norwegian salmon by-products (heads and frames) are considered a high value export product to Asian countries where they are used in e.g., soups (Stevens et al., 2018). Another pathway could be the utilisation in processed foods, such as fish sausages, sauces, and cakes (FAO, 2018). Based on our crude protein, EPA and DHA results, skin, trimmings and frames from Atlantic salmon, European seabass, gilthead seabream, and turbot show potential for these types of applications, assuming separation is economically feasible. Alternatively, a higher economic value might be obtained if these by-products were processed into food extracts and nutraceuticals. This could be the case for salmon by-products processed into protein powders, hydrolysates, salmon oil, and collagen supplements (Stevens et al., 2018). On a crude protein, lipid and EPA+DHA level, our analysis indicates potential for the heads, frames, trimmings and skin for most species (except common carp, and turbot heads) for these types of applications. A full cost-benefit and market analysis has not been performed but is a pertinent area of future research.
If by-product quality is too low and the requirements to maintain “food quality” with appropriate HACCP and GMP standards too costly or prohibitive in any way, feed ingredients, such as fishmeal and fish oil may be more appropriate (Stevens et al., 2018; Glencross, 2019). This is particularly the case for viscera which has little to no value for direct human consumption but showed excellent nutritional properties for oil extraction and hydrolysis, as is the case for Atlantic salmon in Norway (Deepika et al., 2014; Aspevik, 2016), showing a similar fatty acid profile compared to a salmon fillet (Sun et al., 2006). Our study indicates potential for feed applications for by-products, such as skin and viscera from all species (except common carp) due to high protein and EPA+DHA content, but low ash content. Salmon heads, frames and trimmings also show potential, as their ash content is relatively low compared to the other species. In a controlled environment they could be processed into fish protein hydrolysate (FPH), showing high-ranking nutritional characteristics, such as an excellent amino acid composition and digestible proteins that are often applied in animal feed due to their odour and flavour (Kristinsson and Rasco, 2000; Chalamaiah et al., 2012; Tilami and Sampels, 2017). Alternatively, when small volumes of fish by-products are available, a simple and inexpensive method could be the preservation by acid silage. This has a relatively lower quality compared to FPH, but is considered an inexpensive ingredient or feed additive (Olsen and Toppe, 2017). These by-products and derived ingredients could contribute to the human food chain indirectly through livestock or pet food ingredients, adding to the global pool of marine ingredients, especially where by-products exhibit high levels of valuable omega-3 fatty acids (Rustad et al., 2011; Newton et al., 2014). This could be more efficient than direct human consumption, especially when the flesh is technically difficult to extract and/or whole by-product is utilised, rather than just the obtainable flesh yield (Newton, 2020). It could also reduce the need for commonly used marine ingredient substitutes, such as plant ingredients, which affect EPA+DHA content in the final aquaculture product (Sprague et al., 2016) and increase the pressure on agricultural resources (Malcorps et al., 2019). The inclusion of relatively “low economic value” fish by-products in aquafeed reduces the demand for pelagic fish in the form of marine ingredients. This results in a lower Fish In: Fish Out (FIFO) ratio, creating economic and environmental incentives to utilise by-products (Kok et al., 2020).
Alternatively, industrial applications could be considered, such as cosmetics (Alves et al., 2017), pharmaceuticals (e.g., bandages) (Rothwell et al., 2005; Sharp et al., 2012; Afifah et al., 2019) and packaging (de la Caba et al., 2019). Fish skin offers opportunities for the extraction of collagen and gelatine, as an alternative source than bovine or porcine (Nurilmala et al., 2017), and applications in the fashion industry in the form of fish skin leather (Palomino, 2020).
Challenges Associated With the Strategic Utilisation of By-Products
Processing and hence, by-product utilisation strategies depend on the broader food environment, including consumption preferences that can vary greatly across Europe from whole fish compared to various processed forms of different species (EUMOFA, 2017). Additionally, fish and seafood could contain chemical contaminants with associated health implications (Thomsen et al., 2021), and requires investments in HACCP and decontamination to meet food grade requirements. This also accounts for fish by-products processed into aquafeed ingredients such as protein meals and oils, in which these contaminants could cause a significant reduction in nutritional value, food safety, and even fish health (Glencross et al., 2020).
Countries and industries face major infrastructure challenges, such as the scale and accessibility of processing facilities, which limits the economic incentives to utilise by-products (Tyler, 2019). Additionally, obtaining certain ingredients from by-products has technological challenges and is often a cost-intensive process, consequently favouring cheaper alternatives (Olsen et al., 2014). Automated systems (Torrissen et al., 2011), which have been successfully implemented in the poultry industry (Asche et al., 2018), require significant capital investment.
Apart from higher uniformity and freshness of aquaculture by-products compared to those from most fisheries (Newton et al., 2014), feed ingredients influence the nutritional quality of the final aquaculture product (Kwasek et al., 2020; Sprague et al., 2020). Contaminant levels in farmed salmon in Europe are generally lower compared to wild salmon, which can be explained by quality control in the ingredients used (EFSA, 2012; Lundebye et al., 2017; Glencross et al., 2020). The suitability of by-products as feed ingredient depends on multiple factors but their widespread use requires a standardised assessment process to evaluate quality and reduce risk (Glencross, 2020). A separate issue are the legislation and documentation requirements which can be a key utilisation barrier (Olsen et al., 2014) for processors to meet necessary conditions and standards.
Future Research
The low sample size could be expanded in future research and a broader sample set (incl. fillets) from different farms (and feed formulations) could be taken into account. The nutrient analysis could also have been expanded with key amino acids, selenium and vitamins (Lund, 2013; Tilami and Sampels, 2017).
Blood from slaughter facilities represents around 2% of the total production volume (Stevens et al., 2018), but was not investigated in this study. It shows potential for high value applications in the pharmaceutical industry (Sharp et al., 2012).
Conclusion
Certain aquaculture by-products show more potential than others based on their nutritional composition. Nutritional variability among raw materials can have a significant impact on the risk involved in using them. Nevertheless, they are an underutilised resource, which has the potential to support the sustainable growth of European and global aquaculture if more strategically utilised. For species with low fillet yields, the level to which value can be added to the by-products could significantly affect the profitability of production, because of similar total edible yields across the European species. However, sorting and utilising may not always be cost competitive where markets for separated by-products remain undeveloped.
Overall, most by-products showed promising characteristics in terms of nutritional value, which could create incentives to overcome infrastructure and legislative barriers. Most by-products show potential for direct human consumption, but this requires processing and transformation into attractive products, and the incentive to do so, which has occurred within the salmon industry (Torrissen et al., 2011), but yet to do so with other species. Alternatively, by-products that are unattractive for food products might be better direct into feed or industrial applications. Nevertheless, the most strategic application also requires an economic analysis to determine market acceptability.
This analysis showed the different nutritional profiles of by-product fractions, indicating that by-product separation could offer better opportunities to maximise value addition and nutritional efficiency. This could create processing and utilisation incentives, which could enable the aquaculture industry to diversify its products, while using marine resources more efficiently. Consequently, increasing aquaculture output in terms of volume and value without using more resources, showing a perfect example of eco-intensification.
Data Availability Statement
The original contributions presented in the study are included in the article/Supplementary Material, further inquiries can be directed to the corresponding author.
Author Contributions
WM, RN, and DL: conceptualization and resources. WM and MS: formal analysis and visualisation. WM: investigation, methodology, and writing—original draft. RN and DL: project administration. DL, RN, and MS: supervision. WM, RN, MS, and BG: validation. WM, RN, DL, MS, and BG: writing—review and editing. All authors contributed to the article and approved the submitted version.
Funding
This research was funded by GAIN (EU, Horizon 2020 Framework Research and Innovation Programme under GA no. 773330).
Conflict of Interest
The authors declare that the research was conducted in the absence of any commercial or financial relationships that could be construed as a potential conflict of interest.
Publisher's Note
All claims expressed in this article are solely those of the authors and do not necessarily represent those of their affiliated organizations, or those of the publisher, the editors and the reviewers. Any product that may be evaluated in this article, or claim that may be made by its manufacturer, is not guaranteed or endorsed by the publisher.
Acknowledgments
The authors would like to thank the suppliers of the by-products (Giulia Micallef from Gildeskal Forskningsstasjon AS (GIFAS), Remigiusz Panicz and Piotr Eljasik from the Zachodniopomorski Uniwersytet Technologiczny W Szczecinie (ZUT), Mateusz Gzyl from Inskie Centrum Rybactwa - carp ponds in Maliniec, Carmen Gonzalez Sotelo from Agencia Estatal Consejo Superior de Investigaciones Cientificas (CSIC), and Mariló López Belluga from CULMAREX). Additionally, we would like to thank the staff at the Nutritional Analytical Service (NAS), Institute of Aquaculture, University of Stirling, UK, for their excellent lab support and assistance. The authors would like to thank the reviewers and the editor for their valuable comments and suggestions. Additionally, the authors would like to thank the University of Stirling for their financial support regarding the Article Processing Charges (APC) to make this research open access and free for readers.
Supplementary Material
The Supplementary Material for this article can be found online at: https://www.frontiersin.org/articles/10.3389/fsufs.2021.720595/full#supplementary-material
References
Aakre, I., Naess, S., Kjellevold, M., Markhus, M. W., Alvheim, A. R., Dalane, J. O., et al. (2019). New data on nutrient composition in large selection of commercially available seafood products and its impact on micronutrient intake. Food Nutr. Res. 63:3573. doi: 10.29219/fnr.v63.3573
Aas, T. S., Ytrestøyl, T., and Åsgård, T. (2019a). Resource Utilization of Norwegian Salmon Farming in 2016. Tromsø: NOFIMA. Available online at: https://nofima.brage.unit.no/nofima-xmlui/handle/11250/2608436 (Accessed November 10, 2020).
Aas, T. S., Ytrestøyl, T., and Åsgård, T. (2019b). Utilization of feed resources in the production of Atlantic salmon (Salmo salar) in Norway: an update for 2016. Aquacult. Rep. 15:100216. doi: 10.1016/j.aqrep.2019.100216
Ackman, R. G. (1980). Potential for more efficient methods for lipid-analysis. Eur. J. Lipid Sci. Technol. 82, 351–357. doi: 10.1002/lipi.19800820903
Afifah, A., Suparno, O., Haditjaroko, L., and Tarman, K. (2019). “Utilisation of fish skin waste as a collagen wound dressing on burn injuries: a mini review,” in IOP Conference Series Earth and Environmental Science, Vol. 335, 012031 (Bogor). doi: 10.1088/1755-1315/335/1/012031
Alves, A., Marques, A., Martins, E., Silva, T., and Reis, R. (2017). Cosmetic potential of marine fish skin collagen. Cosmetics 4:39. doi: 10.3390/cosmetics4040039
AOAC (1990). Official Methods of Analysis, 15th edn. Gaithersburg, MD: Association of Official Analytical Chemists.
AOAC (2005). Official Methods of Analysis, 18th edn. Gaithersburg, MD: Association of Official Analytical Chemists.
Asche, F., Cojocaru, A. L., and Roth, B. (2018). The development of large scale aquaculture production: a comparison of the supply chains for chicken and salmon. Aquaculture 493, 446–455. doi: 10.1016/j.aquaculture.2016.10.031
Aspevik, T. (2016). Fish Protein Hydrolysates Based on Atlantic salmon By-products. Enzyme Cost-Efficiency and Characterizatoin of Sensory, Surface-Active and Nutritional Properties. Bergen: University of Bergen. Available online at: https://bora.uib.no/bora-xmlui/bitstream/handle/1956/12181/dr-thesis-2016-Tone-Aspevik.pdf?sequence=1&isAllowed=y (accessed January 5, 2021).
Bohnes, F. A., Hauschild, M. Z., Schlundt, J., and Laurent, A. (2018). Life cycle assessments of aquaculture systems: a critical review of reported findings with recommendations for policy and system development. Rev. Aquac. 11, 1061–1079. doi: 10.1111/raq.12280
Chalamaiah, M., Dinesh Kumar, B., Hemalatha, R., and Jyothirmayi, T. (2012). Fish protein hydrolysates: proximate composition, amino acid composition, antioxidant activities and applications: a review. Food Chem. 135, 3020–3038. doi: 10.1016/j.foodchem.2012.06.100
Christie, W. W. (2003). “Preparation of derivatives of fatty acids,” in Lipid Analysis - Isolation, Seperation, Identification and Structural Analysis of Lipids, 3rd edn (Bridgwater: The Oily Press), 205–215.
Cook, S., Silici, L., Adolph, B., and Walker, S. (2015). Sustainable Intensification Revisited. London: IIED. IIED Issue Paper. Available online at: https://pubs.iied.org/sites/default/files/pdfs/migrate/14651IIED.pdf (accessed February 01, 2021).
de la Caba, K., Guerrero, P., Trung, T. S., Cruz-Romero, M., Kerry, J. P., Fluhr, J., et al. (2019). From seafood waste to active seafood packaging: An emerging opportunity of the circular economy. J. Clean. Prod. 208, 86–98. doi: 10.1016/j.jclepro.2018.09.164
Deepika, D., Vegneshwaran, V. R., Julia, P., Sukhinder, K. C., Sheila, T., Heather, M., et al. (2014). Investigation on oil extraction methods and its influence on omega-3 content from cultured Salmon. J. Food Process. Technol. 5, 2157–7110. doi: 10.4172/2157-7110.1000401
EC (2008). Directive 2008/98/ EC of the European Parliament and of the Council of 19 November 2008 on Waste and Repealing Certain Directives (Text with EEA relevance). Document 32008L0098. L 312/3. Luxembourg: European Parliament. Available online at: https://eur-lex.europa.eu/legal-content/EN/TXT/?uri=celex%3A32008L0098 (accessed February 1, 2021).
EC (2020). A New Circular Economy Action Plan For a Cleaner and More Competitive Europe. Brussels: European Commission. Available online at: https://ec.europa.eu/environment/strategy/circular-economy-action-plan_en (accessed February 1, 2021).
EFSA (2012). Update of the monitoring of levels of dioxin and PCBs in food and feed. EFSA J. 10:82. doi: 10.2903/j.efsa.2012.2832
Eljasik, P., Panicz, R., Sobczak, M., Sadowski, J., Barbosa, V., Marques, A., et al. (2020). Plasma biochemistry, gene expression and liver histomorphology in common carp (Cyprinus carpio) fed with different dietary fat sources. Food Chem. Toxicol. 140:111300. doi: 10.1016/j.fct.2020.111300
EUMOFA (2017). EU Consumer Habits Regarding Fishery and Aquaculture Products. Luxembourg: European Market Observatory for Fisheries and Aquaculture Products. doi: 10.2771/758623
EUMOFA (2018). The EU Fish Market 2018 Edition. Luxembourg: European Market Observatory for Fisheries and Aquaculture Products. doi: 10.2771/41473
EUMOFA (2019). The EU Fish Market 2019 Edition. Highlights the EU in the World Market Supply. Consumption, Import, Export Landings in the EU Aquaculture. Luxembourg: European Market Observatory for Fisheries and Aquaculture Products. doi: 10.2771/168390
FAO (2018). The State of World Fisheries and Aquaculture 2018 - Meeting the Sustainable Development Goals. Rome: Food and Agriculture Organization of the United Nations. Available online at: http://www.fao.org/documents/card/en/c/I9540EN/ (accessed November 15, 2020).
Folch, J., Lees, M., and Sloan Stanley, G. H. (1957). A simple method for the isolation and purification of total lipides from animal tissues. Biol. Chem. 226, 497–509. doi: 10.1016/S0021-9258(18)64849-5
Froehlich, H. E., Jacobsen, N. S., Essington, T. E., Clavelle, T., and Halpern, B. S. (2018). Avoiding the ecological limits of forage fish for fed aquaculture. Nat. Sustain. 1, 298–303. doi: 10.1038/s41893-018-0077-1
Glencross, B. D. (2009). Exploring the nutritional demand for essential fatty acids by aquaculture species. Rev. Aquac. 1, 71–124. doi: 10.1111/j.1753-5131.2009.01006.x
Glencross, B. D. (2019). Unravelling Fishmeal: A Review of the Quality Assessment of Fishmeals. Independent report to IFFO. Stirling: University of Stirling.
Glencross, B. D. (2020). A feed is still only as good as its ingredients: An update on the nutritional research strategies for the optimal evaluation of ingredients for aquaculture feeds. Aquac. Nutr. 26, 1871–1883. doi: 10.1111/anu.13138
Glencross, B. D., Baily, J., Berntssen, M. H. G., Hardy, R., MacKenzie, S., and Tocher, D. R. (2020). Risk assessment of the use of alternative animal and plant raw material resources in aquaculture feeds. Rev. Aquac. 12, 703–758. doi: 10.1111/raq.12347
Hamilton, H. A., Newton, R., Auchterlonie, N. A., and Müller, D. B. (2020). Systems approach to quantify the global omega-3 fatty acid cycle. Nat. Food 1, 59–62. doi: 10.1038/s43016-019-0006-0
Huss, H. H. (1995). Quality and Quality Changes in Fresh Fish. FAO Fisheries Technical Paper. Rome: Food and Agriculture Organization of the United Nations. Available online at: http://www.fao.org/3/v7180e/V7180E00.htm
ISO (2005). ISO/IEC 17025:2005(en) General Requirements for the Competence of Testing and Calibration Laboratories. Geneva: The International Organization for Standardization. Available online at: https://www.iso.org/obp/ui/#iso:std:iso-iec:17025:ed-2:v1:en (accessed December 8, 2020).
Jackson, A., and Newton, R. W. (2016). Project to Model the Use of Fisheries By-products in the Production of Marine Ingredients With Special Reference to Omega-3 Fatty Acids EPA and DHA. Stirling: IFFO and University of Stirling.
Kłobukowski, J., Skibniewska, K., Janowicz, K., Kłobukowski, F., Siemianowska, E., Terech-Majewska, E., et al. (2018). Selected parameters of nutritional and pro-health value in the common carp (Cyprinus carpio L.) muscle tissue. J. Food Qual. 2018, 1–9. doi: 10.1155/2018/6082164
Kok, B., Malcorps, W., Tlusty, M. F., Eltholth, M. M., Auchterlonie, N. A., Little, D. C., et al. (2020). Fish as feed: using economic allocation to quantify the Fish in: Fish out ratio of major fed aquaculture species. Aquaculture 528:735474. doi: 10.1016/j.aquaculture.2020.735474
Kristinsson, H. G., and Rasco, B. A. (2000). Fish protein hydrolysates: Production, biochemical, and functional properties. Crit. Rev. Food Sci. Nutr. 40, 43–81. doi: 10.1080/10408690091189266
Kwasek, K., Thorne-Lyman, A. L., and Phillips, M. (2020). Can human nutrition be improved through better fish feeding practices? a review paper. Crit. Rev. Food Sci. Nutr. 60, 3822–3835. doi: 10.1080/10408398.2019.1708698
Little, D. C., Young, J. A., Zhang, W., Newton, R. W., Mamun, A. A., and Murray, F. J. (2018). Sustainable intensification of aquaculture value chains between Asia and Europe: a framework for understanding impacts and challenges. Aquaculture 493, 338–354. doi: 10.1016/j.aquaculture.2017.12.033
Lund, E. K. (2013). Health benefits of seafood; is it just the fatty acids? Food Chem. 140, 413–420. doi: 10.1016/j.foodchem.2013.01.034
Lundebye, A. K., Lock, E. J., Rasinger, J. D., Nøstbakken, O. J., Hannisdal, R., Karlsbakk, E., et al. (2017). Lower levels of persistent organic pollutants, metals and the marine omega 3-fatty acid DHA in farmed compared to wild Atlantic salmon (Salmo salar). Environ. Res. 155, 49–59. doi: 10.1016/j.envres.2017.01.026
Malcorps, W., Kok, B., Land, M. v.t, Fritz, M., Doren, D., et al. (2019). The sustainability conundrum of fishmeal substitution by plant ingredients in shrimp feeds. Sustainability 11:1212. doi: 10.3390/su11041212
Martinez, B., Miranda, J. M., Nebot, C., Rodriguez, J. L., Cepeda, A., and Franco, C. M. (2010). Differentiation of farmed and wild turbot (Psetta maxima): proximate chemical composition, fatty acid profile, trace minerals and antimicrobial resistance of contaminant bacteria. Food Sci. Tech. Int. 16, 0435–0437. doi: 10.1177/1082013210367819
Messina, C., Renda, G., Barbera, L., and Santulli, A. (2013). By-products of farmed European sea bass (Dicentrarchus labrax L.) as a potential source of n-3 PUFA. Biologia 68, 288–293. doi: 10.2478/s11756-013-0148-8
Munekata, P. E. S., Pateiro, M., Domínguez, R., Zhou, J., Barba, F. J., and Lorenzo, J. M. (2020). Nutritional characterization of sea bass processing by-products. Biomolecules 10:232. doi: 10.3390/biom10020232
Naylor, R. L., Hardy, R. W., Bureau, D. P., Chiua, A., Elliott, M., Farrelle, A. P., et al. (2009). Feeding aquaculture in an era of finite resources. PNAS 106, 15103–15110. doi: 10.1073/pnas.0905235106
Naylor, R. L., Hardy, R. W., Buschmann, A. H., Bush, S. R., Cao, L., Klinger, D. H., et al. (2021). A 20-year retrospective review of global aquaculture. Nature 591, 551–563. doi: 10.1038/s41586-021-03308-6
Newton, R. (2020). “A more sophisticated way of looking at Fish,” in Fish Out Ratios. New Methodology for Scoring Hierarchies for Fish-Out Options in FIFO Calculations. Aqua Horizons Consultancy. Available online at: https://www.iffo.com/more-sophisticated-way-looking-fish-infish-out (accessed November 01, 2020).
Newton, R., Telfer, T., and Little, D. (2014). Perspectives on the utilization of aquaculture coproduct in Europe and Asia: prospects for value addition and improved resource efficiency. Crit. Rev. Food Sci. Nutr. 54, 495–510. doi: 10.1080/10408398.2011.588349
Newton, R. W., and Little, D. C. (2018). Mapping the impacts of farmed Scottish salmon from a life cycle perspective. Int. J. Life Cycle Assess. 23, 1018–1029. doi: 10.1007/s11367-017-1386-8
Nichols, P. D., Glencross, B., Petrie, J. R., and Singh, S. P. (2014). Readily available sources of long-chain omega-3 oils: is farmed Australian seafood a better source of the good oil than wild-caught seafood? Nutrients 6, 1063–1079. doi: 10.3390/nu6031063
Nurilmala, M., Jacoeb, A. M., Suryamarevita, H., and Ochiai, Y. (2017). “Extraction and characterization of fresh and sea water fish skin as halal gelatin sources,” in The JSFS 85th Anniversary-Commemorative International Symposium “Fisheries Science for Future Generations”. Symposium Proceedings. Available online at: https://www.researchgate.net/publication/323521620_Extraction_and_characterization_of_fresh_and_sea_water_fish_skin_as_halal_gelatin_sources (accessed November 01, 2020).
Olafsen, T., Richardsen, R., Strandheim, R. N., and Kosmo, J. P. (2014). Analysis of Marine By-Products 2013. Tromsø: SINTEF Fisheries & Aquaculture. Available online at: https://docplayer.net/10912320-Analysis-of-marine-by-products-2013-english-summary.html (accessed November 05, 2020).
Olsen, R. L., and Toppe, J. (2017). Fish silage hydrolysates: Not only a feed nutrient, but also a useful feed additive. Trends Food Sci. Technol. 66, 93–97. doi: 10.1016/j.tifs.2017.06.003
Olsen, R. L., Toppe, J., and Karunasagar, I. (2014). Challenges and realistic opportunities in the use of by-products from processing of fish and shellfish. Trends Food Sci. Technol. 36, 144–151. doi: 10.1016/j.tifs.2014.01.007
Palomino, E. (2020). “SDG 14 life below water,” in Actioning the Global Goals for Local Impact. Science for Sustainable Societies, eds I. Franco, T. Chatterji, E. Derbyshire, and J. Tracey (Singapore: Springer). doi: 10.1007/978-981-32-9927-6_15
Pateiro, M., Munekata, P. E. S., Dominguez, R., Wang, M., Barba, F. J., Bermudez, R., et al. (2020). Nutritional profiling and the value of processing by-products from gilthead sea bream (Sparus aurata). Mar. Drugs 18:101. doi: 10.3390/md18020101
Raftowicz, M., and Le Gallic, B. (2019). Inland aquaculture of carps in Poland: Between tradition and innovation. Aquaculture 518:734665. doi: 10.1016/j.aquaculture.2019.734665
Rana, K. J., Siriwardena, S., and Hasan, M. R. (2009). Impact of Rising Feed Ingredient Prices on Aquafeeds and Aquaculture Production. FAO Fisheries and Aquaculture Technical Paper 541. Rome: Food and Agriculture Organization of the United Nations. Available online at: http://www.fao.org/3/i1143e/i1143e.pdf (accessed November 10, 2020).
Rothwell, S. W., Reid, T. J., Dorsey, J., Flournoy, W. S., Bodo, M., Janmey, P. A., et al. (2005). A salmon thrombin-fibrin bandage controls arterial bleeding in a swine aortotomy model. J. Trauma. 59, 143–149. doi: 10.1097/01.TA.0000171528.43746.53
Rustad, T., Storr,ø, I., and Slizyte, R. (2011). Possibilities for the utilisation of marine by-products. Int. J. Food Sci. Technol. 46, 2001–2014. doi: 10.1111/j.1365-2621.2011.02736.x
Saito, T., Whatmore, P., Taylor, J. F., Fernandes, J. M. O., Adam, A. C., Tocher, D. R., et al. (2020). Micronutrient supplementation affects transcriptional and epigenetic regulation of lipid metabolism in a dose-dependent manner. Epigenetics 31, 1–18. doi: 10.1080/15592294.2020.1859867
Sharp, K. G., Dickson, A. R., Marchenko, S. A., Yee, K. M., Emery, P. N., Laidmåe, I., et al. (2012). Salmon fibrin treatment of spinal cord injury promotes functional recovery and density of serotonergic innervation. Exp. Neurol. 235, 345–356. doi: 10.1016/j.expneurol.2012.02.016
Shepherd, C. J., Monroig, O., and Tocher, D.R. (2017). Future availability of raw materials for salmon feeds and supply chain implications: the case of Scottish farmed salmon. Aquaculture 467, 49–62. doi: 10.1016/j.aquaculture.2016.08.021
Sprague, M., Dick, J. R., and Tocher, D. R. (2016). Impact of sustainable feeds on omega-3 long-chain fatty acid levels in farmed Atlantic salmon, 2006-2015. Sci. Rep. 6:21892. doi: 10.1038/srep21892
Sprague, M., Fawcett, S., Betancor, M., Struthers, W., and Tocher, D. (2020). Variation in the nutritional composition of farmed Atlantic salmon (Salmo salar L.) fillets with emphasis on EPA and DHA contents. J. Food Compost. Anal. 94:103618. doi: 10.1016/j.jfca.2020.103618
Stevens, J. R., Newton, R. W., Tlusty, M., and Little, D. C. (2018). The rise of aquaculture by-products: increasing food production, value, and sustainability through strategic utilisation. Mar. Policy 90, 115–124. doi: 10.1016/j.marpol.2017.12.027
Strobel, C., Jahreis, G., and Kuhnt, K. (2012). Survey of n-3 and n-6 polyunsaturated fatty acids in fish and fish products. Lipids Health Dis. 11, 1–10. doi: 10.1186/1476-511X-11-144
Sun, T., Xu, Z., and Prinyawiwatkul, W. (2006). FA composition of the oil extracted from farmed atlantic salmon (Salmo salar L.) viscera. JAOCS 83, 615–619. doi: 10.1007/s11746-006-1247-2
Takeuchi, T., Satoh, S., and Kiron, V. (2002). “Common carp, Cyprius carpio,” in Nutrient Requirements and Feeding of Finfish for Aquaculture, 1st edn., eds C. D. Webster, and C. Lim (Oxfordshire: CABI Publishing), 245–261. doi: 10.1079/9780851995199.0245
Thomsen, S. T., Assunção, R., Afonso, C., Bou,é, G., Cardoso, C., Cubadda, F., et al. (2021). Human health risk–benefit assessment of fish and other seafood: a scoping review. Crit. Rev. Food Sci. Nutr. 6, 1–22. doi: 10.1080/10408398.2021.1915240
Tilami, S. K., and Sampels, S. (2017). Nutritional Value of Fish: Lipids, Proteins, Vitamins, and Minerals. Rev. Fish. Sci. Aquac. 26, 243–253. doi: 10.1080/23308249.2017.1399104
Tlusty, M. F., Tyedmers, P., Bailey, M., Ziegler, F., Henriksson, P. J. G., Béné, C., et al. (2019). Reframing the sustainable seafood narrative. Glob. Environ. Change 59:101991. doi: 10.1016/j.gloenvcha.2019.101991
Tocher, D. R., Betancor, M. B., Sprague, M., Olsen, R. E., and Napier, J. A. (2019). Omega-3 long-chain polyunsaturated fatty acids, EPA and DHA: bridging the gap between supply and demand. Nutrients 11, 1–20. doi: 10.3390/nu11010089
Tocher, D. R., and Harvie, D. G. (1988). Fatty acid compositions of the major phosphoglycerides from fish neural tissues; (n−3) and (n−6) polyunsaturated fatty acids in rainbow trout (Salmo gairdneri) and cod (Gadus morhua) brains and retinas. Fish Physiol. Biochem. 5, 229–239. doi: 10.1007/BF01874800
Torrissen, O., Olsen, R. E., Toresen, R., Hemre, G. I., Tacon, A. G. J., Asche, F., et al. (2011). Atlantic salmon (Salmo salar): the “Super-Chicken” of the sea? Rev. Fish. Sci. 19, 257–278. doi: 10.1080/10641262.2011.597890
Troell, M., Naylor, R. L., Metian, M., Beveridge, M., Tyedmers, P. H., Folke, C., et al. (2014). Does aquaculture add resilience to the global food system? PNAS 111, 13257–13263. doi: 10.1073/pnas.1404067111
Tyler, J. (2019). Why Is Fish Waste a Profitable Waste Stream Often Overlooked by the Industry? Aquafeed.com. Available online at: https://aquafeed.co.uk/entrada/why-is-fish-waste-a-profitable-waste-stream-often-overlooked-by-the-industry−20876/ (accessed March 5, 2021).
UN (2020). SDG 12: Ensure Sustainable Consumption and Production Patterns. Available online at: https://sdgs.un.org/goals/goal12 (accessed January 10, 2021).
UNDP (2020). Responsible Consumption and Production. Available online at: https://www.undp.org/sustainable-development-goals#responsible-consumption-and-production (accessed September 15, 2020).
USEPA (2020). Sustainable Management of Food. Food Recovery Hierarchy. Washington, DC: United States Environmental Protection Agency. Available online at: https://www.epa.gov/sustainable-management-food/food-recovery-hierarchy (accessed September 16, 2020).
Keywords: aquaculture, by-products, nutrition, processing, edible yield, circular economy, value addition, waste reduction
Citation: Malcorps W, Newton RW, Sprague M, Glencross BD and Little DC (2021) Nutritional Characterisation of European Aquaculture Processing By-Products to Facilitate Strategic Utilisation. Front. Sustain. Food Syst. 5:720595. doi: 10.3389/fsufs.2021.720595
Received: 25 June 2021; Accepted: 20 September 2021;
Published: 20 October 2021.
Edited by:
Keitaro Kato, Kindai University, JapanReviewed by:
Guobao Song, Dalian University of Technology, ChinaAmal Biswas, Kindai University, Japan
Copyright © 2021 Malcorps, Newton, Sprague, Glencross and Little. This is an open-access article distributed under the terms of the Creative Commons Attribution License (CC BY). The use, distribution or reproduction in other forums is permitted, provided the original author(s) and the copyright owner(s) are credited and that the original publication in this journal is cited, in accordance with accepted academic practice. No use, distribution or reproduction is permitted which does not comply with these terms.
*Correspondence: Wesley Malcorps, wesley.malcorps@stir.ac.uk