- 1Department of Environmental Science and Land Resources Management, South Eastern Kenya University, Kitui, Kenya
- 2Department of Environmental Science and Education, Kenyatta University, Nairobi, Kenya
Deteriorating soil fertility, low unreliable rainfall and soil moisture stress has resulted to low crop yields among farmers of sub-Saharan Africa (SSA), necessitating a search for more sustainable production practices. Zai technology has the ability to promote soil moisture retention and enhances soil fertility. A four-seasons field experiment was conducted to assess the impact of Zai technology combined with cattle manure and inorganic fertilizer on selected soil properties and sorghum yields in Kabati, Kitui County. The experiment was set up in a Randomized Complete Block Design (RCBD) with eight treatments replicated thrice with sorghum Gadam as the test crop. Soil sampling was done at the beginning of the first season and at the end of the fourth season at a dept of 0–15 cm across each plot for laboratory analyses. From the results, the increase in electrical conductivity was significant at p < 0.05 in all the treatments after four cropping seasons. Total organic carbon significantly increased in Zai with cattle manure (p = 0.045), conventional with no input (p = 0.038) and conventional with cattle manure (p = 0.045). Available phosphorous significantly (p < 0.05) increased in treatments under Zai technology while total nitrogen significantly (p < 0.05) reduced after the four cropping seasons. There was a significant (p < 0.05) interactive effect of the tested factors on soil pH, electrical conductivity, total nitrogen, and available phosphorous at the end of the experiment. Moreover, there was significant (p < 0.05) interactive effects on grain yields (SR18 and SR19 seasons) and stover yields (SR18, LR19, and SR19 seasons), with higher yields being recorded in treatments under Zai technology. This study demonstrates the importance of Zai technology in increasing crop yield by trapping water and enhancing its retention and infiltration into the soil for uptake by plants. This study concluded that positive impacts on important soil properties and crop yield could be realized when Zai technology is utilized alongside either sole inorganics or a combination of organic and inorganic amendments and this could be used as a strategy to improve crop production in eastern Kenya and other similar areas.
Introduction
According to FAO (2010), rain-fed agriculture contributes to about 60% of the world crop production. Despite this, low nutrient, inadequate water supply in quality and quantity (Hengsdijk and Langeveld, 2009; Nelly, 2010) and soil moisture stress (Rockstrom and Karlberg, 2010) have been found to be the major drawbacks that limit crop productivity and are major restraints to agricultural development in many areas of the world. Water scarcity and the resultant food insecurity are of particular concern in most tropical regions of the world, where many countries are less developed (Nelly, 2010).
For most countries in Sub-Saharan Africa (SSA), rain-fed agriculture is a significant sector of their economy with close to 70% of the total population living in rural areas and largely depending on small-scale subsistence agriculture for their livelihood security (Rockstrom, 2000). In this region, rain-fed agriculture, which is characterized by droughts in most parts (Rockstrom and Karlberg, 2010), has threatened the food demand projected by 2050 (Grafton et al., 2015). With such projections, rain-fed agriculture is one of the most vulnerable sectors to climate variability and change as reported by (Intergovernmental Panel on Climate Change, 2007).
The uncertainty and risk for current farm-level production associated with variability of rainfall amount and distribution within and between the seasons have downplayed the likely impact of innovations aimed at improving farm productivity (Biazin et al., 2012). This is because the uncertainty discourages the decision to invest in improved farming practices by the farming communities and the wide range of stakeholders in agriculture (Cooper and Coe, 2011). The uncertainty of rainfall amounts and distribution and the high evapotranspiration makes crop production a risky enterprise in semi-arid areas, as a result of agricultural drought and intra-seasonal dry spells (ISDS) leading to reduced yield in rain-fed agriculture (Kahinda et al., 2007).
Despite this, it is imperative to recognize that the situations in many African countries are manageable. The global crop yield growth average of the major cereals in the world varies between 0.9 and 1.6% per year and the increase rates have fallen in the past two decades (Grafton et al., 2015). Rosegrant et al. (2002) reported that grain (maize, millet, sorghum, and teff) yields from rain-fed agriculture were ~1.5 t ha−1 in developing countries. There is need to produce more to sustainably meet the food needs of the ever-growing human population. Thus, this necessitates the need to develop new strategies for agricultural production in sub-Saharan Africa. These situations suggest that the challenges of limited yields in rain-fed farming systems in SSA might be overcome with soil water conservation techniques combined with fertility management technologies (Rockstrom and Karlberg, 2010). These combinations generate systems that further increase water efficiency and yields in smallholder farms (Winterbottom et al., 2013).
Studies have shown that Kenya is one of the most vulnerable countries to climate variability and changes due to its low adaptive capacity and over-dependence on climate sensitive sectors such as rain-fed agriculture (Kurukulasuriya et al., 2006; Herrero et al., 2010; FAO, 2011). Like many other countries in SSA, Kenya has had significant drought events with the 2004–2006 one affecting food availability for 2.5 million people and 2008–2010 affecting 10 million people (Rarieya and Fortun, 2009). This can be attributed to low soil fertility and moisture deficits inhibiting crop production in the arid and semi-arid areas (Gichangi et al., 2006). Use of suitable water and soil management technologies such as Zai pit (Evett and Tolk, 2009) increases rainfall use efficiency and bridge intra-seasonal dry spells (Dile et al., 2013).
Zai pit system is one of the successful interventions that ensure reduction in runoff and evaporation, improved precipitation capture, and improved agricultural productivity (Evett and Tolk, 2009). Since most lands under cultivation are characterized by compaction, reduced permeability, limited plant root development, and inadequate aeration (Zougmoré et al., 2014), pitting facilitates more water infiltration as runoff water is harvested due to the earthen bund formed downslope of the pits (Kabore and Reij, 2004). Despite this, Zai pits have been found to be efficient when combined with other soil moisture conservation methods and organic and inorganic soil inputs (Burpee et al., 2015).
The improvement of water status in the soil and the increased decomposition and nutrient release results in a beneficial impact of the Zai systems on crop performance under semi-arid conditions (Zougmoré et al., 2014). Zai pit is a form of ancient dryland farming technique that was first initiated in Burkina Faso although some literature points it to Dogon in Northern Mali (Danjuma and Mohammed, 2015). It involves the utilization of basins or holes of 20–120 cm and about 10–60 cm in depth for agricultural activities (Sawadogo, 2011). Their utilization has been found to minimize the effects of droughts since they ensure soil maintenance, soil erosion control and water preservation (Figure 2). Zai have been found to be capable of collecting up to 25% or more of a run-off coming from 5 times its area (Malesu et al., 2006). Zai pits are known to allow crops do well in areas with high risk of crop failure as a result of harsh climatic conditions (Critchley and Gowing, 2012). Water stored in the Zai delay the onset and occurrence of severe water stress thereby buffering the crop against damage caused by water deficits during dry periods (Nyamadzawo et al., 2013). Zai pits increase the amount of water stored in the soil profile by trapping or holding rainwater where it falls (Mutunga, 2001). Besides enhancing water storage, Zai pits increases water infiltration and reduces run-off for plant uptake during the dry periods (Drechsel et al., 2005). The pits play a key water harvesting role.
The application of soil fertility management inputs enhances soil nutrient availability and improves nutrient uptake by crops from soil reserves (Kar et al., 2013). Positive impacts of Zai pit combined with integrated soil fertility management (ISFM) inputs on agricultural productions of different crops have been recorded in other studies (Kabore and Reij, 2004; Sawadogo, 2011). Zai pits combined with cattle manure has been known to promote soil fertility (Fatondji et al., 2006; Sawadogo, 2011) and increase organic matter content (Barry et al., 2009) in soil as compared to use of mineral fertilizer. Other studies have recorded increased yields in maize (Recha et al., 2014), pearl millet grain (Fatondji, 2002), and sorghum (Sawadogo, 2011).
Besides supplying macronutrients and micronutrients to the soil (Negassa et al., 2001; Tirol-Padre et al., 2007) cattle manure has been found to improve the physical and chemical properties of soil (Tirol-Padre et al., 2007). Cattle manure application increases soil (Mucheru-Muna et al., 2007, 2014; Mugwe et al., 2009; Mutegi et al., 2012; Wildemeersch et al., 2015), electrical conductivity (Cai et al., 2014; Zhao et al., 2014; Ozlu and Kumar, 2018; Zhu et al., 2018) and Soil organic matter concentration (Bayu et al., 2005; Mucheru-Muna et al., 2007; Xin et al., 2016; Zhou et al., 2016; Omenda et al., 2021) as compared to inorganic fertilizer. Cattle manure has been known to provide higher Phosphorous levels to soil as compared to other organic inputs (Mucheru-Muna et al., 2007; Tirol-Padre et al., 2007; Opala et al., 2012; Muui et al., 2013). By raising soil organic matter, it increases cation exchange capacity and improves soil physical properties (Bationo et al., 2006). The use of cattle manure generally increases sorghum yields (Gateri et al., 2011), wheat grain and straw yield (Coventry et al., 2011), and maize yields (Omenda et al., 2021) by providing plant nutrients and increasing the soil's capacity to hold those nutrients. Increased yields due to use of animal manure has also been reported in other studies in central Kenya (Lekasi et al., 2003; Kimani et al., 2004).
Most farmers in eastern Kenya are entirely depended on rain-fed agriculture as a source of livelihood sustenance and to meet their daily food demands. The study area, which is located in eastern Kenya, is characterized by low, erratic and unreliable rainfall, high temperatures and thus high evaporation rates. These, coupled with low soil fertility has resulted to low, insufficient crop yields among farmers thus resulting to food insecurity. This necessitates the need of research aimed at improving crop production for food security in the area. The present study therefore sought to investigate how selected soil parameters (Soil pH, Electrical conductivity, Total nitrogen, Total organic carbon and Available phosphorous) and sorghum yields are affected by the use of Zai pit technology combined with integrated nutrient management amendments (cattle manure and mineral fertilizer) in Kabati, Kitui County, Kenya.
Materials and Methods
Profile of the Study Area
The study was carried out in Kabati area, located in Kitui County, Kenya (Figure 1). Based on the stratification of the agro-ecological zones, Kabati represents the semi-arid areas of Kitui County. It lies between 400 m and 1,830 m above sea level and generally slopes eastwards with the highest regions being Mutitu Hills and Kitui Central (KCIDP, 2018). The County lies between latitudes 0010′ and 300′ South and longitudes 37,050′ and 3,900′ East (Figure 1).
The region has a bimodal rainfall distribution in a year, the long rains (LR) which are experienced between March and May and the short rains (SR) between October and December. The total annual rainfall average is between 500 and 1,150 mm with a mean annual temperature of 24°C (Jaetzold et al., 2006). February and September are the hottest months of the year with temperatures ranging to a maximum of 26–34°C as compared to temperature ranges of 14–22°C experienced in the other months. Agriculture, both livestock keeping and crop farming, is the backbone of people's economy in the area and a major source of most households' income (Republic of Kenya, 2005; KCIDP, 2018).
Various food crops [maize (Zea mays L.), beans (Phaseolus vulgaris), green gram (Vigna radiata), cassava (Manihot esculenta), sorghum (Sorghum bicolor), millet (Pennisetum glaucum), and pigeon peas (Cajanus cajan)], fruits [mangoes (Mangifera indica), water melons (Citrullus lanatus), oranges (Citrus sinensis), lemons (Citrus limon), bananas (Musa acuminata), and pawpaw (Carica papaya)], and vegetables [kales (Brassica oleracea), tomatoes (Solanum lycopersicum), spinach (Spinacia oleracea), and onions (Allium cepa)] are grown for local consumption with the surplus being sold to traders (Republic of Kenya, 2005; NDMA, 2017; KCIDP, 2018). The major soil type in the study area is lixisols (red soils; Jaetzold et al., 2006). The soils are predominantly sandy to loamy sand in texture; hence they are generally poorly drained, susceptible to erosion by runoff and are limited in their capacity to retain water and nutrients.
Experimental Design
The field experiments were laid out in a randomized Compete block design (RCBD). Each experimental plot measured 6 m by 4.5 m with a 1 m wide alley separating plots within a block and 2 m wide alley left between blocks. The field experiment ran for four consecutive cropping seasons; short rains 2018 (SR18) and 2019 (SR19) and long rains 2019 (LR19) and 2020 (LR20). There was a total of eight treatments (Table 1). The treatments comprised of two systems, either Zai pit or convention system (no Zai pits), both with four levels of fertilization (no input - control), sole cattle manure, sole mineral fertilizer and both cattle manure and mineral fertilizer). The Zai pits measured 60 cm by 60 cm with a 30 cm depth and was spaced at 70 and 75 cm inter and intra-row, respectively (Figure 2). Dryland Sorghum Gadam variety was used as the experimental test crop with three seeds planted per hill, at a spacing of 75 and 20 cm inter and intra- row, respectively and later thinned out to two seedlings per hill two weeks after emergence to achieve the recommended plant density per ha−1. External nutrient amendments were applied, based on the Phosphorous and Nitrogen contents as determined through a lab analysis of soil samples, at the beginning of every season to give an equivalent amount of 60 kg N ha−1 and 60 kg P ha−1, the recommended Nitrogen and Phosphorous rates in the study area (FURP, 1994; Karanja et al., 2014). Cattle manure was broadcasted before plowing for conventional plots and placed in the prepared pits for Zai plots where it was thoroughly mixed with soil. Mineral fertilizers were pre-weighed for each plot before going to the field and applied using dollop cups to ensure uniform distribution within the plot. Weeding was done twice using hand hoe weeding.
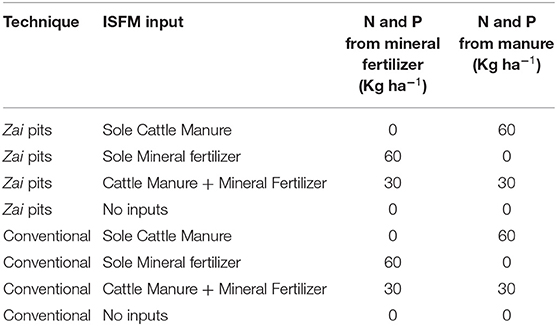
Table 1. Experimental treatments during the short rains 2018, long rains 2019, short rains 2019, and long rains 2020 seasons in Kabati, Kitui County.
Data Collection
Soil Sampling and Laboratory Analysis
The initial sampling was done beginning of SR18 season while the final sampling was done end of LR20 season. Soil samples were collected using an alderman auger at soil depth of 0–15 cm. Soil auguring was done at five spots following a W pattern across each plot and thoroughly mixed to make a composite sample for each plot. Before sampling at every subsequent plot, the soil auger and the collection buckets were thoroughly cleaned to avoid sample contamination. A representative sample was taken from each plot's composite sample, packed in bags with marked tags indicating the plot number and transported to the laboratory for analyses. The fresh-field moist soils were then air-dried, grounded and sieved before subjecting them chemical analysis in the laboratory. All the laboratory analyses on the chemical properties of the soil were done using the standard methods for analyzing soils by Motsara (2008). Soil pH was determined at a 1:1 soil/water ratio using an electronic pH while electrical conductivity was measured using an electrical conductivity probe. Total nitrogen was determined using Kjeldahl method as recently used by Bolo et al. (2021), Soil organic carbon was determined using the Walkley and Black (1934) wet oxidation method and available phosphorus was determined using the Bray and Kurtz (1945) P-1 soil test phosphorus (P) method.
Harvesting and Yield Determination
Sorghum grain yield and stover yield were harvested at physiological maturity from the net plot. The heads were manually separated from the stover. All heads from each plot were collected and separately placed in different sacks which were well-labeled. Heads were then sun-dried, hand-threshed and weighed at 12.5 % moisture content. Maize stover was cut at ground level and total above-ground fresh weight determined. Both grain and Stover yields were then converted from kilograms per net area to tons per hectare (t/ha).
Statistical Analysis
Both sorghum yield and soil data were subjected to a two-way analysis of variance (ANOVA) in R-studio program to obtain an F-value of the effect of the model. Pair-wise comparison of selected soil parameters differences between the start and the end of the experiment were analyzed using t-test. Differences between treatment means were examined using least significance difference (LSD) at p = 0.05.
Results
Rainfall Distribution During the Four Experimental Seasons
There was seasonal rainfall variability over the four seasons of the study (Figure 3). The four consecutive cropping seasons SR18, LR19, SR19, and LR20 were characterized by different rainfall patterns, with higher rainfall occurring in the short rain seasons (SR18-938.1 mm and SR19- 442.71 mm) compared to the long rainy seasons (LR19-116.3 mm and LR20- 228 mm).
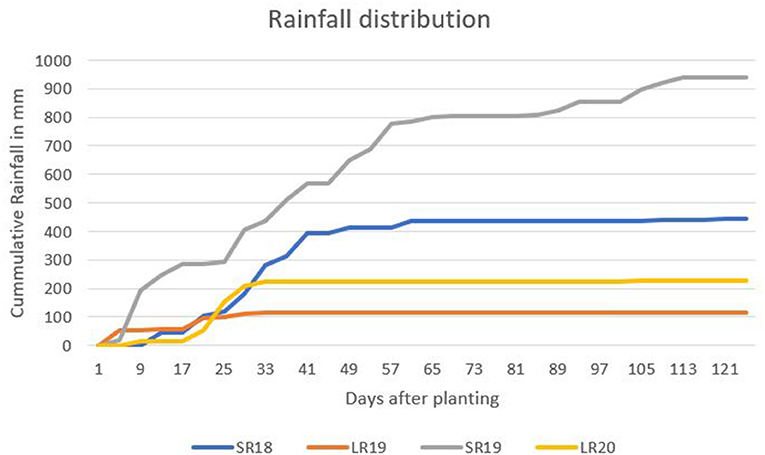
Figure 3. Rainfall distribution from 2018 to 2020 for short rains 2018, long rains 2019, short rains 2019, and long rains 2020 seasons.
The total number of rainy days varied in the four growing seasons with SR19 having the highest number of rainy days (47 days) followed by SR18 (31 days) (Table 2). LR19 and LR20 had the lowest number of rainy days i.e., 10 and 11 days, respectively. Much of the rainfall during the SR18 and SR19 seasons was received during the first 2 months with a prolonged dry spell period occurring from day 64 after planting and prolonging toward the end of the planting seasons.
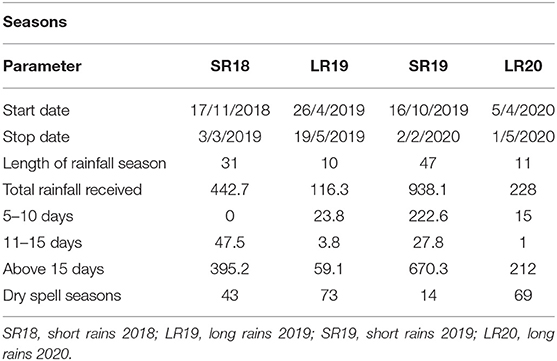
Table 2. Seasonal Rainfall characteristics for short rains 2018 and 2019 and long rains 2019 and 2020.
In Contrast, the long rains seasons (LR19 and LR20) experienced interchanging periods of rainy days and consecutive dry days during the 1st month. An intra-seasonal dry spell of 73 and 69 days was experienced during the LR19 and LR20 respectively, whereas during the SR18 and SR19 drought periods of 43 and 14 days respectively, were experienced (Table 2). The rainfall in Eastern Kenya is distributed within two seasons annually, the long rains which are experienced between March and May and the short rains between October and December. Much of the total annual rainfall is received during the short rains season and thus the short rains are considered more reliable. It is during this season that higher crop yields are realized as compared to the long rains season. In this study, highest total season rainfall amounts were experienced during the short rains and this had a positive impact on sorghum yield as compared to the long rains' seasons.
Effects of Zai Pits in Combination With Selected ISFM Options on Selected Soil Chemical Properties
Generally, there was observed variation in pH levels across the treatments with all treatments that had manure application whether solely or in combination with mineral fertilizer increasing while those with sole application of mineral fertilizer decreasing at the end of the experiment. There was a significant (p < 0.05) interactive effect on soil pH at the end of the experiment. The increase in soil pH was significant at p < 0.05 in Zai with manure (p = 0.02234), conventional with manure (p = 0.04697), Zai pit with manure and half rate mineral fertilizer (p = 0.02597), and conventional with manure and half rate mineral fertilizer (p = 0.04697).
Electrical conductivity significantly increased at p <0.05 in all the treatments at the end of the experiment (Table 3); Zai pit with no input (p = 0.015), Zai pit with manure (p = 0.022), Zai pit with full rate fertilizer (p = 0.0058), Zai pit with both manure and half rate mineral fertilizer (p = 0.013), conventional with no input (p = 0.001), conventional with manure (p = 0.006), conventional with full rate mineral fertilizer (p = 0.019), and conventional with both manure and half rate mineral fertilizer (p = 0.001).
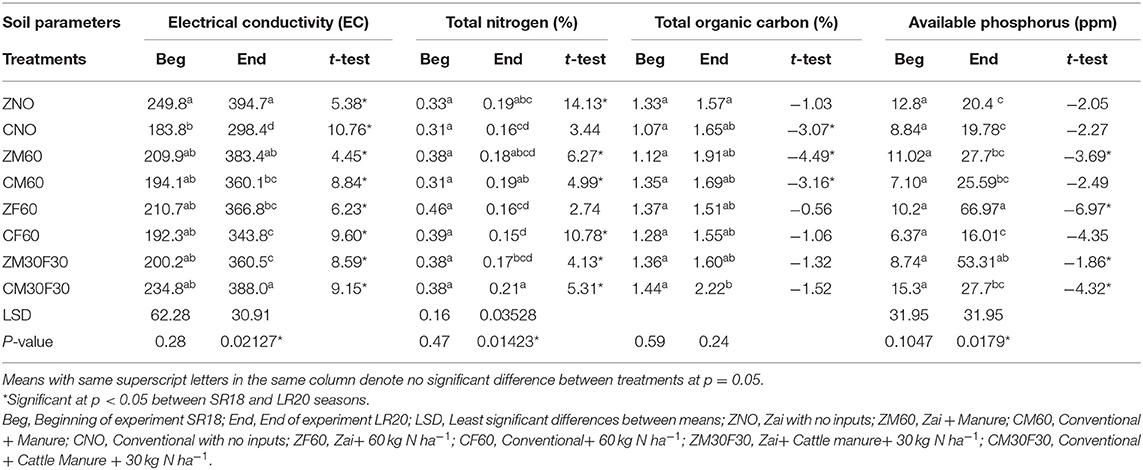
Table 3. Soil chemical properties (0–15 cm) at the beginning of short rains 2018 and end of long rain 2020 seasons at Kabati, Kitui County.
All the treatment under Zai pit system had a significant (p < 0.05) positive effect on electrical conductivity of soil as compared to their counterparts under conventional system. Zai with full rate mineral fertilizer and Zai with cattle manure and half rate mineral fertilizer treatments significantly (p = 0.021) increased electrical conductivity as compared to conventional with full rate mineral fertilizer and conventional with cattle manure and half rate mineral fertilizer. Zai with no input and Zai with sole cattle manure recorded 27 and 6% higher electrical conductivity values compared to Conventional with no input and Conventional with sole cattle manure, respectivelyAt the end of the experiment, total nitrogen significantly decreased (Table 3) at p < 0.05 in Zai pit with no input (p = 0.0003), Zai pit with sole cattle manure (p = 0.012), Zai pit with manure and half rate mineral fertilizer (p = 0.047), Conventional with sole cattle manure (p = 0.027), conventional with full rate mineral fertilizer (p = 0.0005) and conventional with cattle manure and half rate mineral fertilizer (p = 0.018). Zai with no input significantly (p = 0.014) increased total nitrogen as compared to conventional with no input treatment. Conventional with cattle manure and half rate fertilizer had a significant positive effect on total nitrogen than Zai with cattle manure and half rate mineral fertilizer.
Significant (p < 0.05) increases in Total organic carbon between the beginning and end of the experiment were recorded under Zai pit with sole cattle manure treatment (p = 0.045), conventional with sole cattle manure treatment (p = 0.045) and conventional with no input treatment (p = 0.038). Under the Zai pit system, Zai with sole cattle manure increased Total organic carbon by 36, 22, and 19% higher as compared to Zai with full rate mineral fertilizer, Zai with no input and Zai with cattle manure and half rate mineral fertilizer respectively (Table 3).
The amount of available phosphorous significantly (p < 0.05) increased at the end of the long rains 2020 season in Zai with sole cattle manure (p = 0.022), Zai with full rate mineral fertilizer (p = 0.013), Zai with cattle manure and half rate mineral fertilizer (0.021), and conventional treatment with cattle manure and half rate mineral fertilizer (p = 0.039). At the end of the experiment, Zai with full rate mineral fertilizer and Zai with cattle manure and half rate mineral fertilizer treatments significantly (p = 0.018) increased phosphorous as compared to Conventional with full rate mineral fertilizer and Conventional with cattle manure and half rate mineral fertilizer. Zai with no input and Zai with sole cattle manure recorded 3 and 8% higher available phosphorous amounts compared to Conventional with no input and Conventional with sole cattle manure, respectively (Table 3).
Effects of Zai Pits in Combination With Selected ISFM Options on Sorghum Yields
Generally, higher grain and stover yields were recorded during the short rains' seasons (SR18 and SR19) as compared to the long rains' seasons (LR19 and LR20) (Table 4). Even though treatments under Zai system recorded higher grain yields in LR19 and LR20, the difference was not significant at P = 0.05 (Table 4). Zai treatments recorded significantly (p < 0.05) higher grain yields during the SR18, SR19 and LR19 seasons as compared to the treatments under the conventional system. In SR18, Zai with no input, Zai with sole cattle manure, Zai with full rate mineral fertilizer and Zai with cattle manure and half rate mineral fertilizer recorded 33, 25, 42, and 28% significantly higher grain yield than conventional with no input, conventional with sole cattle manure, conventional with full rate mineral fertilizer and conventional with cattle manure and half rate mineral fertilizer, respectively (Figure 5).
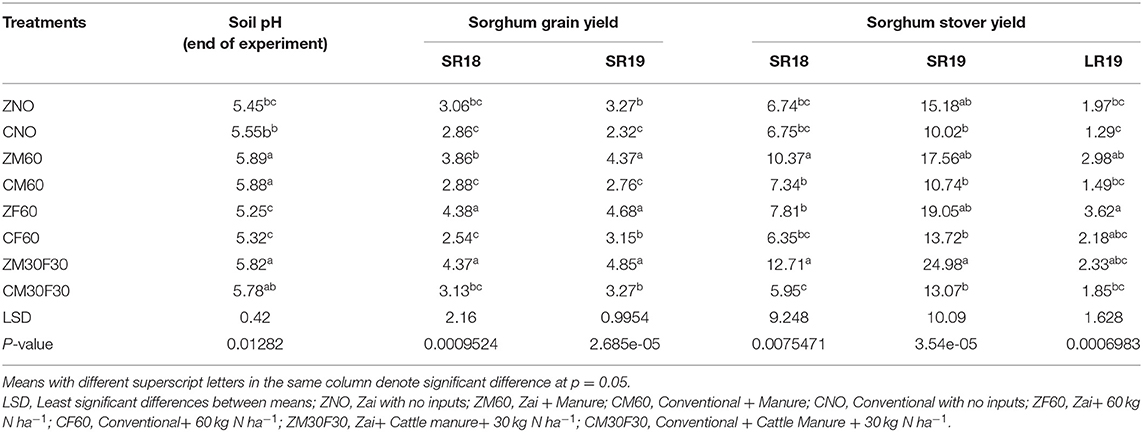
Table 4. Interaction effects of Zai technology and selected nutrient amendment options on Soil pH and sorghum grain and stover yields in the short rains' seasons of 2018 and 2019 (SR18 and SR19) and the long rain season of 2019 (LR19).
The highest grain yields (4.85 t ha−1 in SR19 and was recorded under the Zai pit system with cattle manure and half rate mineral fertilizer and this was significantly (p < 0.05) higher than the similar treatment under conventional system (Table 4). During the SR19 season, Zai pit with cattle manure and half rate mineral fertilizer, Zai with sole cattle manure, Zai with full rate mineral fertilizer and Zai with no input recorded 48, 58, 39, and 40% significantly (p < 0.05) higher yields than their counterpart treatments under conventional system (Figure 4). The interaction effect on Sorghum grain yield between water management and nutrient amendment options was significant at p = 0.05 in SR18 and SR19 (Figure 5). In the LR19 and LR20 seasons, all the treatments under Zai system recorded higher grain yield than their conventional counterpart treatments, with the highest (1.39 t ha−1) grain yield recorded under Zai with sole cattle manure in LR20 being 21% higher than that of conventional with sole cattle manure (Table 4).
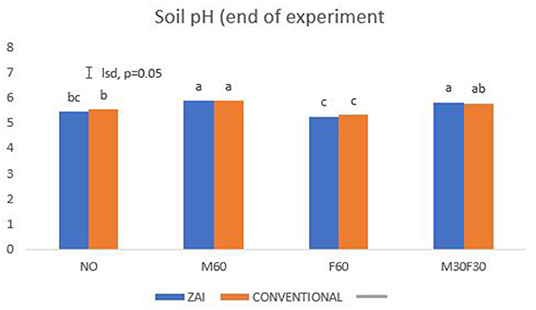
Figure 4. Interaction effect of Zai technology and selected soil nutrient amendment options on Soil pH at the end of the LR20 season. Beg, Beginning of experiment SR18; End, End of experiment LR20; ZNO, Zai with no inputs; ZM60, Zai + Manure; CM60, Conventional + Manure; CNO, Conventional with no inputs; ZF60, Zai + 60 kg N ha−1; CF60, Conventional + 60 kg N ha−1; ZMF30, Zai + Cattle manure + 30 kg N ha−1; CMF30, Conventional + Cattle manure + 30 kg N ha−1. The error bar denote the least significant difference (lsd) per season at p = 0.05. Means followed by same letters in the same column denote no significant difference between treatments at p = 0.05.
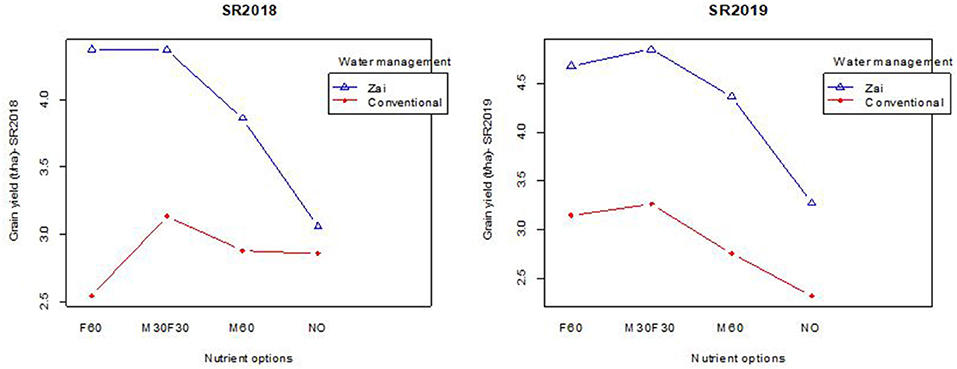
Figure 5. Effect of interaction of Zai technology and selected nutrient options on Sorghum yields in the Short rains' seasons 2018 and 2019 (SR18 and SR19). NO, No inputs; M60, Sole manure; F60, Full rate mineral fertilizer (60 kg N ha−1); M30F30, Manure + half rate mineral fertilizer (30 kg N ha−1).
Highest stover yields were recorded during the SR19 season while the lowest was recorded in the LR19 season. Significant (p < 0.05) positive interaction effects on stover yields were recorded in treatments under Zai system in the SR18, LR19, and SR19 seasons as compared to the treatments under conventional system (Figure 6). In SR18, Zai with cattle manure and half rate mineral fertilizer recorded 53% significantly (p < 0.05) higher stover yields than conventional with cattle manure and half rate mineral fertilizer. During the LR19 season, Zai with no input, Zai with sole cattle manure, Zai with full rate mineral fertilizer and Zai with cattle manure and half rate mineral fertilizer recorded 35, 50, 40, and 21% significantly (p < 0.05) higher stover yields than their conventional counterparts. In SR19 season, significantly (p < 0.05) higher stover yields were recorded under Zai treatments as compared to similar treatments under conventional system, with the highest (13.14 t ha−1) under Zai with cattle manure and half rate mineral fertilizer being 35% higher than the similar treatment under conventional system (Figure 6).
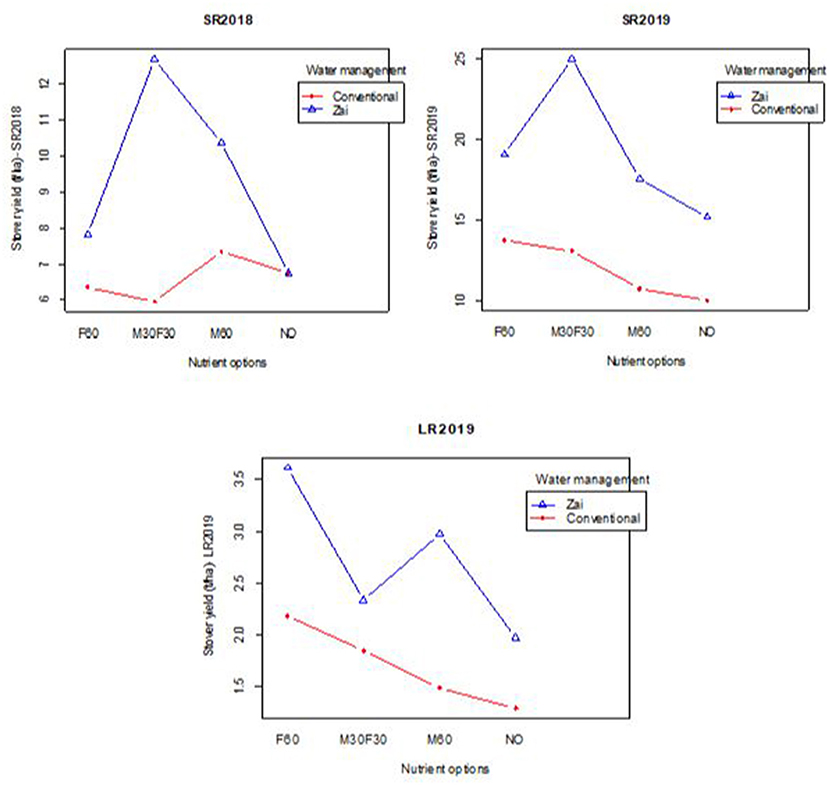
Figure 6. Interaction effect of Zai technology and selected nutrient amendment options on Sorghum stover yield in the Short rains' seasons, 2018 and 2019 (SR18 and SR19) and long rain season 2019 (LR19). NO, No inputs; M60, Sole manure; F60, Full rate mineral fertilizer (60 kg N ha−1); M30F30, Manure + half rate mineral fertilizer (30 kg N ha−1).
Generally, across all the treatments in both systems in the four study seasons, treatments with fertility inputs recorded higher sorghum grain and stover yields as compared to both Zai and conventional with no input. Furthermore, treatments with cattle manure application either solely or in combination with mineral fertilizer recorded significantly (p < 0.05) higher yields as compared to the treatments with sole mineral fertilizer application in both systems. All the treatments with sole cattle manure application or with combined manure and mineral fertilizer application recorded significantly higher yields as compared to Zai pit treatments without cattle manure application. The effect of interaction on Sorghum grain yield between the selected water management strategy and the nutrient amendment options was significant in the SR18 and the SR19. The two seasons recorded highest grain yield with Zai treatments recording higher yields than the treatments under conventional system (Figure 5).
Discussion
Generally, there was observed variation in pH levels across the treatments with all treatments that had manure application whether solely or in combination with mineral fertilizer increasing while those with sole application of mineral fertilizer decreasing at the end of the experiment (Figure 4).
The decrease in soil pH in mineral fertilizer-amended treatments could be as a result of the H+ ions, which are added on the cation exchange complex of soils from the mineral fertilizer. Nitrogen fertilization acidifies the soil by the oxidation of dry-deposited compounds, loss of basic cations through ion exchange, and plant uptake and nitrification of ammonium (Cai et al., 2019). Synthetic nitrogen application significantly reduces exchangeable base cations in soils, which lead to declined soil pH. A decline in base saturation is symptomatic of soil acidification (Stevens et al., 2009). Additionally, synthetic nitrogen application shifts soils into the Al3+ buffering stage. Aluminum is released into solution at a pH below 5 by the hydrolysis of both Al-hydroxides and silicates on clay mineral surfaces (Cai et al., 2019). Decrease in soil pH increases the availability of potentially toxic heavy metals and also contribute to the reduction of the microbial organisms beneficial in root functions (Stevens et al., 2009). The increase in soil pH in manure-amended soils could be attributed to the reduction of exchangeable aluminum in acidic soils (pH 4.5–5.5) which is considered to occur through aluminum precipitation or chelation on organic colloids (Hue and Amien, 1989; Mucheru-Muna et al., 2007) or by complexation of soluble aluminum by organic molecules, especially organic acids (Hue and Amien, 1989). Generally, the ash-alkalinity nature of manure is associated with protons to neutralize soil acidity (Rukshana et al., 2013). The alkalinity of organic materials following the decarboxylation of organic anions and the ammonification of organic N (Cai et al., 2019) are the major causes of the recorded increase in soil pH in manure-amended soils.
The observed pH decreases in fertilizer amended soils corroborate with the findings by Cai et al. (2019) where lowered soil pH was recorded in treatments with sole application of N fertilizers which resulted in no yield after 12 years of study. Accordingly, other studies reported that synthetic fertilizer application could significantly decrease soil pH (Mucheru-Muna et al., 2007; Cai et al., 2014; Zhu et al., 2018). The increase in soil pH recorded in manure-amended soils correspond with the findings by Wildemeersch et al. (2015) where increment in soil pH to 5.0 from the initial 4.2 was recorded following manure application. A number of other studies have also reported significant increases in pH with manure treatment (Eghball, 2002; Bayu et al., 2005; Mucheru-Muna et al., 2007, 2014; Mugwe et al., 2009; Mutegi et al., 2012).
Higher increases in electrical conductivity recorded in manure amended soils (Table 3) could be as a result of presence of dissolved salts in the cattle manure used as a result of feed additives which when mixed with water, they dissolve and break into tiny electrically charged ions, hence increasing the ability of the soil solution to conduct electricity. The increase could also be attributed to the resultant increases in exchangeable potassium ions and organic matter, which supplies a pool of nutrients and ions that can be released in the soil solution. This observation corresponds with the findings by Ozlu and Kumar (2018) who reported increased Electrical conductivity by 2.2 times in manure-amended soils as compared to that of fertilizer for 0–10 cm depth. The higher increase in electrical conductivity in the treatments with manure application as compared to non-manure amendments is consistent with the findings of several other studies (Dong et al., 2001; Eghball, 2002; Eigenberg et al., 2002; Cai et al., 2014; Zhao et al., 2014; Carmo et al., 2016; Miller et al., 2017; Zhu et al., 2018).
Generally, there was reduction in Total Nitrogen in all the treatments at the end of the experiment (Table 3). The observed decrease in total nitrogen content could be attributed to uptake for utilization by sorghum plants. The recorded nitrogen losses could also as a result of erosion by run off since the experiments were carried out during rainy seasons and the soils in the study area, being predominantly sandy to loamy sand in texture, are poorly drained, susceptible to erosion and are limited in their capacity to retain water and nutrients. Furthermore, the reduction recorded in manure-amended treatments could be as a result of increased microbial biomass which could utilize the nitrogen and losses through leaching, denitrification and ammonia volatilization. These results correspond with the findings of other studies where reduction in total nitrogen were recorded and the losses attributed to erosion (Jaetzold et al., 2006), utilization through microbial activity (Van Diepeningen et al., 2006) and losses through denitrification and ammonia volatilization (Ju et al., 2009).
The recorded significant increases in total organic carbon in treatments with sole application of manure (Table 3) could be as a result of addition of more carbon source through manure application. Manure directly increases carbon inputs into the soil and also influences crop residues, which potentially sequestrates agricultural soil organic carbon and nutrient release. These finding is corroborated by the finding by Hati et al. (2007) who attributed the observed increase in soil organic carbon among different fertility treatments after 28 years of study to the addition of carbon source through crop residues, root biomass, and farmyard manure. Similarly, other studies have reported a significant increase in soil organic carbon in treatments that had sole cattle manure application (Dunjana et al., 2012; Bedada et al., 2014) and in those with manure and half rate fertilizer (Kaur et al., 2007; Bedada et al., 2014). Several other studies have reported increases in organic carbon content in soil as a result of manure application as compared to use of mineral fertilizer (Mucheru-Muna et al., 2007; Lal, 2008; Bandyopadhyay et al., 2010; Dunjana et al., 2012; Liang et al., 2012; Kuzyakov and Blagodatskaya, 2015; Xin et al., 2016; Zhou et al., 2016).
There was increase in available phosphorous in all the treatment at the end of the experiment (Table 3). The higher levels of phosphorous recorded in treatments with sole fertilizer or fertilizer combined with manure application was as a result of phosphorous available in the NPK fertilizer used. Zai treatments with either sole cattle manure application or manure combined with mineral fertilizer had higher available phosphorous levels at the end of the experiment as compared to similar treatments under conventional system. This could be as a result of the composition of cattle manure, which contains both organic and inorganic phosphorous. Plants take up phosphorous in form of inorganic orthophosphates and it generally constitutes 45–90% of the phosphorous in manure (Buckley and Makorto, 2004), making manure an important source of phosphorous. Despite the effect of nutrient amendment inputs, all the treatments under Zai system recorded higher phosphorous levels as compared to conventional treatments. This could be due to the ability of the Zai pit system to hold nutrients in place by preventing nutrient loss through run-off by erosion. The current trend of results is in consonance with the findings of studies by Mucheru-Muna et al. (2007), Tirol-Padre et al. (2007), and Muui et al. (2013). Cattle manure supplies macronutrients and micronutrients to the soil (Gemechu, 2020) and has been known to provide higher phosphorous levels to soil as compared to other organic inputs (Opala et al., 2012).
Generally, the two short rains seasons (SR18 and SR19) recorded higher grain and stover yields as compared to the two long rains seasons (LR19 and LR20). This could be as a result of the high rainfall amounts (442.71 and 938.1 mm for SR18 and SR19, respectively) recorded in the short rains seasons (Figure 1) which were higher than those recorded in the long rain seasons (166.3 and 228 mm for LR19 and LR20, respectively). The amount of rainfall received during the planting season has an effect on yield. The number of rainy days were also more during these two short seasons (Table 2) with more rainy days occurring during the first 62 days after planting (critical growth and developmental stages). According to Hansen (2004), agricultural activities in eastern Kenya depends on the OND (October, November, and December) short rainfall seasons which have been found to be more predictable and reliable. Thus, better annual yields are received during the OND seasons in most agriculture-depended households (Amissah-Arthur et al., 2002; Barron et al., 2003). The lower yields recorded during the LR19 and the LR20 seasons was as a result of the lower unreliable rainfall amounts, fewer number of rainy days and poor distribution during the season (McCarl et al., 2008). These two seasons experienced long intra-seasonal dry spells (73 and 69 days for LR19 and LR20, respectively) which resulted to soil moisture stress, hence the low recorded yields. Intra-seasonal dry spells are a characteristic feature of semi-arid areas in East Africa (Barron et al., 2003) and South Africa (Usman and Reason, 2004). The occurrence of these dry spells has had major detrimental effects on crop yields. In their study, Craufurd and Peacock (1993) found out that sorghum grain yields were reduced by 87% as a result of water stress which occurred during critical crop developmental stages such as flowering and stem swelling (McCarl et al., 2008). According to Bewket (2009) and Mulat et al. (2004), the amount and temporal distribution of rainfall is generally the major determinant of inter seasonal fluctuations observed in crop production levels.
Zai pit is a form of ancient dryland farming technique which involves the utilization of basins or holes for agricultural activities so as to minimize the effects of droughts since they ensure soil maintenance, soil erosion control and water preservation (Sawadogo, 2011; Danjuma and Mohammed, 2015). In Kenya, Zai pits technology has been promoted as a water harvesting technique for maize production in eastern region (Recha et al., 2014). The results of this study indicated that treatments under Zai pit technology with the selected soil nutrient amendment options had a significant (p < 0.05) interaction effect on sorghum grain and stover yields as compared to similar treatments under conventional system. Furthermore, higher yields were realized in Zai treatments containing either sole cattle manure or manure combined with mineral fertilizer.
Despite the fertility inputs, the higher yields recorded under Zai treatments could be attributed to the potential of Zai pits in increasing the amount of water stored in the soil profile by trapping or holding rainwater where it falls (Mutunga, 2001), therefore delaying the onset and occurrence of severe water stress thus buffering the crop against damage caused by water deficits during dry periods (Nyamadzawo et al., 2013). Besides enhancing water storage, Zai pits increases water infiltration and reduces run-off for plant uptake (Drechsel et al., 2005) thereby, boosting crop yield. The results of this study correspond with other studies whose results indicated a positive effect of Zai pit utilization on crop yield.
Fatondji et al. (2006) found out that the use of Zai pits in Burkina Faso led to increased harvests of pearl grain Millet. They attributed this increase to the use of Zai pits as well as the use of organic fertility inputs. A study by Sawadogo (2011) reported increased yields variations from 300 to 400 kg ha−1 by the Zai system in degraded land. Sawadogo (2011) reported substantial sorghum grain yield increases in farmer's fields from 319 to 642 kg ha−1 without Zai pit system to 975 to 1,600 kg ha−1 with Zai pit system. The use of potential of Zai pit system in increasing crop yield has also been reported in Zambia (Haggblade and Tembo, 2003; Thierfelder and Wall, 2009), South Africa (Magombeyi and Taigbenu, 2008), Niger (Fatondji et al., 2009), Ethiopia (Amede et al., 2011), and in Zimbabwe (Thierfelder and Wall, 2009; Gumbo et al., 2012). Besides its positive effect on soil moisture, Zai pits have a good potential to increase soil biological activities and to promote nutrient use and agronomic efficiency (Fatondji, 2002).
Conclusion
Significant differences in soil properties and sorghum grain and stover yields among different fertilization treatments under Zai and conventional systems were recorded in this study. Application of sole manure or combined with mineral fertilizer significantly increased soil pH, electrical conductivity, soil organic carbon and available phosphorous as compared to treatments with sole mineral fertilizer at the end of the experiment.
There was significant (p < 0.05) interaction effect of Zai technology and the selected soil nutrient amendment options on the selected soil properties and sorghum grain and stover yields. Generally, treatments under Zai system recorded higher pH, electrical conductivity, organic carbon, phosphorous, and nitrogen levels as compared to conventional treatments and this could be due to the ability of the Zai pit system to hold nutrients in place by preventing nutrient loss through run-off by erosion. The significant effects resulting from the interaction of Zai system and the selected soil nutrient amendment options on sorghum grain and stover yields as compared to the conventional system could be attributed to moisture retention and increased nutrient use efficiency enhanced by the Zai pits. The results of this study demonstrates that significant positive interaction effects on crop yields could be achieved when Zai technology is combined with inorganic nutrient amendments either solely or in combination with organic inputs. The ability of Zai technology to improve crop yield is largely attributed to its pit design. Apart from capturing water, Zai pits enhances soil moisture retention and infiltration thereby increasing the amount of water in the soil that is available for uptake by plants.
Data Availability Statement
The original contributions presented in the study are included in the article/Supplementary Material, further inquiries can be directed to the corresponding author/s.
Author Contributions
MK conceived the study, collected data, performed analysis, and drafted the manuscript. FM-N, CN, and MM-M assisted in designing the field experiments, supervised data collection, reviewed, and corrected and commended on the manuscript. All authors contributed to the article and approved the submitted version.
Funding
This research was funded by National Research Fund, Kenya, through South Eastern Kenya University Climate Smart Agriculture research project.
Conflict of Interest
The authors declare that the research was conducted in the absence of any commercial or financial relationships that could be construed as a potential conflict of interest.
Publisher's Note
All claims expressed in this article are solely those of the authors and do not necessarily represent those of their affiliated organizations, or those of the publisher, the editors and the reviewers. Any product that may be evaluated in this article, or claim that may be made by its manufacturer, is not guaranteed or endorsed by the publisher.
Acknowledgments
The authors acknowledge South Eastern Kenya University Climate Smart Agriculture research project funded by the National Research Fund, Kenya for funding data collection and soil laboratory analyses costs for the current study. The authors are thankful to The Higher Education Loans Board (HELB), Kenya, for funding tuition fee for PhD studies for the first author. The authors would also like to appreciate the field assistants, casual laborers, and laboratory technicians at Kenya Agricultural and Livestock Research Organization (KALRO) Muguga laboratory for all the assistance they accorded that made the study a success.
Supplementary Material
The Supplementary Material for this article can be found online at: https://www.frontiersin.org/articles/10.3389/fsufs.2021.714212/full#supplementary-material
References
Amede, T., Menza, M., and Awlachew, S. B. (2011). Zai improves nutrient and water productivity in the Ethiopian highlands. Exp. Agric. 47, 7–20. doi: 10.1017/S0014479710000803
Amissah-Arthur, A., Jagtap, S., and Rosenzweig, C. (2002). Spatio-temporal effects of El Nino events on rainfall and maize yield in Kenya. Int. J. Climatol. 22, 1849–1860. doi: 10.1002/joc.858
Bandyopadhyay, K., Misra, A., Ghosh, P., and Hati, K. (2010). Effect of integrated use of farmyard manure and chemical fertilizers on soil physical properties and productivity of soybean. Soil Tillage Res. 7, 115–125. doi: 10.1016/j.still.2010.07.007
Barron, J., Rockström, J., Gichuki, F., and Hatibu, N. (2003). Dry spell analysis and maize yields for two semi-arid locations in East Africa. Agric. For Meteorol. 117, 23–37. doi: 10.1016/S0168-1923(03)00037-6
Barry, B., Olaleye, A. O., Zougmoré, R., and Fatondji, D. (2009). Rainwater Harvesting Technologies in the Sahelian Zone of West Africa and the Potential for Outscaling. Vol. 126. Colombo: International Water Management Institute, H042111.
Bationo, A., Hartemink, A., Lungu, O., Naimi, M., Okoth, P., Smaling, E., et al. (2006). “African soils: their productivity and profitability of fertilizer use,” in Background Paper Prepared for The African Fertilizer Summit June 9-13, 2006. Abuja.
Bayu, W., Rethman, N. F. G., and Hammes, P. S. (2005). The role of manure in sustainable soil fertility management in Sub-Saharan Africa. J. Sustain. Agric. 25, 113–136. doi: 10.1300/J064v25n02_09
Bedada, W., Karltun, E., Lemenih, M., and Tolera, M. (2014). Long-term addition of compost and NP fertilizer increases crop yield and improves soil quality in experiments on smallholder farms. Agric. Ecosyst. Environ. 195, 193–201. doi: 10.1016/j.agee.2014.06.017
Bewket, W. (2009). “Rainfall variability and crop production in Ethiopia: case study in the Amhara region. (conference proceedings),” in 16th International Conference of Ethiopian Studies (Trondheim), 823–836.
Biazin, B., Sterk, G., Temesgen, M., Abdulkedir, A., and Stroosnijder, L. (2012). Rainwater harvesting and management in rainfed agricultural systems in sub-Saharan Africa – a review. Phys. Chem. Earth A/B/C 47–48, 139–151. doi: 10.1016/j.pce.2011.08.015
Bolo, P., Kihara, J., Mucheru-Muna, M., Njeru, E. M., Kinyua, M., and Sommer, R. (2021). Application of residue, inorganic fertilizer and lime affect phosphorus solubilizing microorganisms and microbial biomass under different tillage and cropping systems in a Ferralsol. Geoderma 390, 114–962. doi: 10.1016/j.geoderma.2021.114962
Bray, R. H., and Kurtz, L. T. (1945). Determination of total, organic, and available forms of phosphorus in soils. Soil Sci. 59, 39–46. doi: 10.1097/00010694-194501000-00006
Buckley, K., and Makorto, M. (2004). “Phosphorus in livestock manures,” in Advanced Silage Corn Management. Brandon, MB: Agriculture and Agri-Food Canada.
Burpee, G., Janet, B. S., and Schmidt, A. (2015). Preparing Smallholder Farm Families to Adapt to Climate Change: Pocket Guide 3; Managing Water Resources. Baltimore, MD: Catholic Relief Services.
Cai, A., Xu, M., Wang, B., Zhang, W., Liang, G., Hou, E., et al. (2019). Manure acts as a better fertilizer for increasing crop yields than synthetic fertilizer does by improving soil fertility. Soil Tillage Res. 189, 168–175. doi: 10.1016/j.still.2018.12.022
Cai, Z., Wang, B., Xu, M., Zhang, H., He, X., Zhang, L., et al. (2014). Intensified soil acidification from chemical N fertilization and prevention by manure in an 18-year field experiment in the red soil of southern China. J. Soils Sediments 15, 260–270. doi: 10.1007/s11368-014-0989-y
Carmo, D. L., Lima, L. B., and Silva, C. A. (2016). Soil fertility and electrical conductivity are affected by organic waste rates and nutrient inputs. Rev. Bras. Ciênc. 40:rbcs20150152. doi: 10.1590/18069657rbcs20150152
Cooper, P. J. M., and Coe, R. (2011). Assessing and addressing climate-induced risk in sub-Saharan rain-fed agriculture. Exp. Agric. 47, 179–184. doi: 10.1017/S0014479711000019
Coventry, D. R., Gupra, R. K., Yadav, A., Poswal, R. S., Chhokar, R. S., Sharma, R. K., et al. (2011). Wheat quality and productivity as affected by varieties and sowing time in Haryana, India. Field Crop. Res. 123, 214–225. doi: 10.1016/j.fcr.2011.05.017
Craufurd, P. Q., and Peacock, J. M. (1993). Effect of heat and drought stress on sorghum (Sorghum Bicolor). II. Grain yield. Exp. Agric. 29, 77–86. doi: 10.1017/S0014479700020421
Critchley, W., and Gowing, J. (2012). Water Harvesting in Sub-Saharan Africa. New York, NY: Earthscan from Routledge. doi: 10.4324/9780203109984
Danjuma, M. N., and Mohammed, S. (2015). Zai pit System: a catalyst for restoration in the dry lands. J. Agric. Vet. 8, 1–4. doi: 10.9790/2380-08210104
Dile, Y. T., Karlberg, L., Temesgen, M., and Rockström, J. (2013). The role of water harvesting to achieve sustainable agricultural intensification and resilience against water related shocks in sub-Saharan Africa. Agric. Ecosyst. Environ. 181, 69–79. doi: 10.1016/j.agee.2013.09.014
Dong, J., Yao, L., Zhang, J., Feng, J., and Sa, R. (2001). Feed Additive Manual (in China). Beijing: China Agricultural University Press, 289.
Drechsel, P., Quansah, C., and Penning de Vries, F. (2005). “Stimulation of urban and peri-urban agriculture in West Africa: characteristics, challenges, need for action,” in Urban Agriculture in West Africa. Contributing to Food Security and Urban Sanitation, ed O. B. Smith (Ottawa, ON; Wageningen: International Development Research Centre, Technical Centre for Agricultural and Rural Cooperation). Available online at: http://www.idrc.ca/en/ev-33700-201-1-DO_TOPIC.html (accessed March 23, 2021).
Dunjana, N., Nyamugafata, P., Shumba, A., Nyamangara, J., and Zingore, S. (2012). Effects of cattle manure on selected soil physical properties of smallholder farms on two soils of Murewa, Zimbabwe. Soil Use Manag. 28, 221–228. doi: 10.1111/j.1475-2743.2012.00394.x
Eghball, B. (2002). Soil properties as influenced by phosphorus- and nitrogen-based manure and compost applications. Agron J. 94:128. doi: 10.2134/agronj2002.0128
Eigenberg, R., Doran, J., Nienaber, J., Ferguson, R., and Woodbury, B. (2002). Electrical conductivity monitoring of soil condition and available N with animal manure and a cover crop. Agric. Ecosyst. Environ. 88, 183–193. doi: 10.1016/S0167-8809(01)00256-0
Evett, S. R., and Tolk, J. A. (2009). Introduction: can water use efficiency be modeled well enough to impact crop management? Agron. J. 101, 423–425. doi: 10.2134/agronj2009.0038xs
FAO (2010). The Hague Conference on Agriculture, Food Security and Climate Change. Climate Smart Agriculture: Policies, Practices and Financing for Food Security, Adaptation and Mitigation. Rome: FAO.
Fatondji, D. (2002). “Organic amendment decomposition, nutrient release and nutrient uptake by millet (Pennisetum glaucum L. R. Br) in a traditional land rehabilitation technique (Zai) in the Sahel,” in Ecology and development Series No. 1 2002, eds P. L. Vlek, M. Denich, C. Martius, and N. van de Giesen (PhD dissertation). North Rhine-Westphalia: University of Bonn.
Fatondji, D., Martius, C., Bielders, C. L., Vlek, P. L., Bationo, A., and Gerard, B. (2006). Effect of planting technique and amendment type on pearl millet yield, nutrient uptake, and water use on degraded land in Niger. Nutr. Cycl. Agroecosyst. 76, 203–217. doi: 10.1007/s10705-005-6209-9
Fatondji, D., Martius, C., Zougmore, R., Vlek, P. L., Bielders, C. L., and Koala, S. (2009). Decomposition of organic amendment and nutrient release under the Zai technique in the Sahel. Nutr. Cycl. Agroecosyst. 85, 225–239. doi: 10.1007/s10705-009-9261-z
FURP (1994). Fertilizer Recommendations Volumes 1-22, KARI. Nairobi: Fertilizer Use Recommendation Project.
Gateri, M. W., Thuranira, E. G., Njuguna, L. W., Gikaara, D. M., Kamau, E. M., Wepukhulu, S. B., et al. (2011). “Development of agronomic practices for Hydrangea (Hydrangea macrophylla) for dry cut-flower processing in Central Kenya,” in 11th Kenya Agricultural Research Institute Biennial Scientific Conference. Available online at: https://www.yumpu.com/en/document/read/27313284/development-of-agronomic-practices-for-hydrangea-/3 (accessed March 26, 2021).
Gemechu, B. (2020). Effect of integrated farmyard manure and NP fertilizers use on hybrid maize yield and soil properties in western Ethiopia. Malays. J. Med. Biol. Res. 7, 49–58. doi: 10.18034/mjmbr.v7i1.489
Gichangi, A., Maina, M. P., Lelgut, D. K., Kinyua, M. G., Macharia, E., Kamwaga, J., et al. (2006). “Cassava-grain legume inter-crop: its feasibility and implications on household food security in Kenya. Strengthening Kenya's food security and income,” in 10th KARI Biennial Scientific Conference. Nairobi: Kenya Agricultural Research Institute.
Grafton, R. Q., Williams, J., and Jiang, Q. (2015). Food and water gaps to 2050: preliminary results from the global food and water system (GFWS) platform. Food Secur. 7, 209–220. doi: 10.1007/s12571-015-0439-8
Gumbo, D., Snelder, D., Wuta, M., and Nyagumbo, I. (2012). “Zimbabwe: keeping runoff on the land,” in Water Harvesting in Sub-Saharan Africa, eds W. Critchley and J. Gowing. New York, NY: Earthscan, Routledge, 256.
Haggblade, S., and Tembo, G. (2003). Conservation farming in Zambia. EPTD Discussion Paper No. 108. Washington, DC: IFPRI, 112.
Hansen, J. W. (2004). Linking dynamic seasonal climate forecasts with crop simulation for maize yield prediction in semi-arid Kenya. Agric. For Meteorol. 125, 143–157. doi: 10.1016/j.agrformet.2004.02.006
Hati, K. M., Swarup, A., Dwivedi, A., Misra, A., and Bandyopadhyay, K. (2007). Changes in soil physical properties and organic carbon status at the topsoil horizon of a vertisol of central India after 28 years of continuous cropping, fertilization and manuring. Agric. Ecosyst Environ. 119, 127–134. doi: 10.1016/j.agee.2006.06.017
Hengsdijk, H., and Langeveld, J. W. (2009). Yield Trends and Yield Gap Analysis of Major Crops in the World. Wageningen: Wageningen University, 170.
Herrero, M., Ringler, C., Steeg, J., van de Thornton, P., Tingju, Z., Bryan, E., et al. (2010). Climate Variability and Extreme Events and Climate Change and Their Impacts on Kenya's Agricultural Sector. Nairobi: ILRI.
Hue, N. V., and Amien, I. (1989). Aluminum detoxification with green manures. J. Soil Sci. Plant Nutr. 20, 1499–1511. doi: 10.1080/00103628909368164
Intergovernmental Panel on Climate Change (2007). “Intergovernmental panel on climate change. Climate change 2007: synthesis report,” in Contribution of Working Groups I, II and III to the Fourth Assessment Report of the Intergovernmental Panel on Climate Change. Cambridge; New York, NY: Geneva Cambridge University Press.
Jaetzold, R., Schmidt, H., Hornet, Z. B., and Shisanya, C. A. (2006). Farm Management Handbook of Kenya. Natural Conditions and Farm Information (Eastern Province), Vol 11/C, 2nd Edn. Nairobi: Ministry of agriculture/GTZ.
Ju, X., Xing, G., Chen, X., Zhang, S., Zhang, L., Liu, X., et al. (2009). Reducing environmental risk by improving N management in intensive Chinese agricultural systems. Natl. Acad. Sci. 106, 3041–3046. doi: 10.1073/pnas.0813417106
Kabore, P. D., and Reij, C. (2004). The Emergence and Spreading of an Improved Traditional Soil and Water Conservation Practice in Burkina Faso. Environment and Production Technology Division Discussion Paper No. 114. Washington, DC: International Food Policy Research Institute. p. 338.
Kahinda, J. M., Boroto, R. J., and Taigbenu, A. E. (2007). Domestic rainwater harvesting as an adaptation measure to climate change in South Africa. Phys. Chem. Earth 35, 742–751. doi: 10.1016/j.pce.2010.07.004
Kar, R., Bindroo, B. B., Ghosh, M. K., and Majumder, S. K. (2013). Carbon credit in soil under a long-term fertilizer experiment on mulberry. Nature 11, 77–81.
Karanja, D. R., Kisilu, R. K., Kathuli, P., Mutisya, D. L., Njaimwe, A. N., Keya, G., et al. (2014). Enhancing Sorghum Production in Semi-Arid Kenya. Available online at: Kalro.org/Sorghum-training-manual (accessed May 05, 2021).
Kaur, T., Brar, B. S., and Dhillon, N. S. (2007). Soil organic matter dynamics as affected by long-term use of organic and inorganic fertilizers under maize–wheat cropping system. Nutr. Cycl. Agroecosyst. 81, 59–69. doi: 10.1007/s10705-007-9152-0
KCIDP (2018). County Integrated Development Plan 2018–2022. County Government of Kitui. Kitui: Kitui County Integrated Development Plan.
Kimani, S. K., Macharia, J. M., Gachengo, C., Palm, C. A., and Delve, R. J. (2004). Maize production in the central highlands of Kenya using cattle manures combined with modest amounts of mineral fertilizer. Uganda J. Agric. Sci. 9, 480–490. Available online at: https://www.google.com/url?sa=t&rct=j&q=&esrc=s&source=web&cd=&cad=rja&uact=8&ved=2ahUKEwjE5qyI3vPzAhUymnIEHeA8C0kQFnoECAYQAQ&url=https%3A%2F%2Fwww.ajol.info%2Findex.php%2Fujas%2Farticle%2Fview%2F134989%2F124493&usg=AOvVaw0UtXlesPGhrQXT0X7BI1ja
Kurukulasuriya, P., Mendelsohn, R., Hassan, R., Benhin, J., Diop, M., Eid, H., et al. (2006). Will African agriculture survive climate change? World Bank Econ. Rev. 20, 367–388. doi: 10.1093/wber/lhl004
Kuzyakov, Y., and Blagodatskaya, E. (2015). Microbial hotspots and hot moments in soil: concept and review. Soil Biol. Biochem. 83, 184–199. doi: 10.1016/j.soilbio.2015.01.025
Lal, R. (2008). Crop residues as soil amendments and feedstock for bioethanol production. Waste Manage. 28, 747–758. doi: 10.1016/j.wasman.2007.09.023
Lekasi, J. K., Tanner, J. C., Kimani, S. K., and Harris, P. J. (2003). Cattle manure quality in Maragua District, Central Kenya: effect of management practices and development of simple methods of assessment. Agric. Ecosyst. Environ. 94, 289–298. doi: 10.1016/S0167-8809(02)00037-3
Liang, Q., Chen, H., Gong, Y., Fan, M., Yang, H., Lal, R., et al. (2012). Effects of 15 years of manure and inorganic fertilizers on soil organic carbon fractions in a wheat-maize system in the north China plain. Nutr. Cycl. Agroecosyst. 92, 21–33. doi: 10.1007/s10705-011-9469-6
Magombeyi, M. S., and Taigbenu, A. E. (2008). Crop yield risk analysis and mitigation of smallholder farmers at Quaternary catchment level: case study of B72A in Olifants river basin, South Africa. Phys. Chem. Earth A/B/C 33, 744–756. doi: 10.1016/j.pce.2008.06.050
Malesu, M., Sang, J., Oduor, A., Odhiambo, O., and Nyabenge, M. (2006). Rainwater Harvesting Innovations in Response to Water Scarcity: The Lare Experience. Technical Report No.32. Nairobi: Regional Land Management Unit (RELMA-in-ICRAF), Netherlands Ministry of Foreign Affairs and Swedish International Development Cooperation Agency (SIDA).
McCarl, B. A., Villavicencio, X., and Wu, X. (2008). Climate change and future analysis: is stationarity dying? Am. J. Agric. Econ. 90, 1241–1247. doi: 10.1111/j.1467-8276.2008.01211.x
Miller, J., Beasley, B., Drury, C., Larney, F., and Hao, X. (2017). Surface soil salinity and soluble salts after 15 applications of composted or stockpiled manure with straw or wood chips. Compost. Sci. Util. 25, 36–47. doi: 10.1080/1065657X.2016.1176968
Motsara, M. R. (2008). Guide to Laboratory Establishment for Plant Nutrient Analysis. Rome: Food and Agriculture Organization of the United Nations.
Mucheru-Muna, M., Mugendi, D., Kung'u, J., Mugwe, J., and Bationo, A. (2007). Effects of organic and mineral fertilizer inputs on maize yield and soil chemical properties in a maize cropping system in Meru south district, Kenya. Agrofor. Syst. 69, 189–197. doi: 10.1007/s10457-006-9027-4
Mucheru-Muna, M. W., Mugendi, D. N., Pypers, P., Mugwe, J. N., Kung'u, J. B., Vanlauwe, B., et al. (2014). Enhancing maize productivity and profitability using organic inputs and mineral fertilizer in central Kenya small-hold farms. Exp. Agric. 50, 250–269. doi: 10.1017/S0014479713000525
Mugwe, J., Mugendi, D., Mucheru-Muna, M., Odee, D., and Mairura, F. (2009). Effect of selected organic materials and inorganic fertilizer on the soil fertility of a humic Nitisol in the central Highlands of Kenya. Soil Use Manag. 25, 434–440. doi: 10.1111/j.1475-2743.2009.00244.x
Mulat, D., Fantu, G., and Tadele, F. (2004). Agricultural Development in Ethiopia: Are There Alternatives to Food Aid. Addis Ababa: Addis Ababa University.
Mutegi, E. M., Kung'u, J. B., Muna, M., Pieter, P., and Mugendi, D. N. (2012). Complementary effects of organic and mineral fertilizers on maize production in the smallholder farms of Meru south district, Kenya. J. Agric. Sci. 3, 221–229. doi: 10.4236/as.2012.32026
Mutunga, K. (2001). “Water conservation, harvesting and management – Kenyan experience,” in Sustaining the Global Farm: Selected paper from the 10th International Soil Conservation Organization Meeting held May 24-29, 1999 at Purdue University and the USD-ARS National Soil Erosion Research Laboratory, eds D. E. Stott, R. H. Mohtar, and G. C. Steinhardt (West Lafayette, IN: Purdue University), 1139–1143.
Muui, C., Muasya, R. M., and Kirubi, D. T. (2013). Baseline survey on factors affecting sorghum production and use in eastern Kenya. African J. Food Agric. Nutr. Dev. 13, 7339–7342. doi: 10.18697/ajfand.56.11545
NDMA (2017). Kitui County 2016 Short Rains Food Security Assessment Report. A Joint Report by the Kenya Food Security Steering Group1 (KFSSG) and County Steering Group. Kitui County: National Drought Management Authority.
Negassa, W., Negisho, K., Friesen, D. K., Ransom, J., and Yadessa, A. (2001). “Determination of optimum farmyard manure and NP fertilizers for maize on farmers field,” in Seventh Eastern and Southern Africa Regional Maize Conference (Nairobi), 387–393.
Nelly, A. (2010). Rainwater Harvesting in Rural Kenya, Reliability in a Variable and Changing Climate, Physical Geography and Quaternary Geology, 45 HECs. Stockholm: Stockholm University, NKA 27.
Nyamadzawo, G., Wuta, M., Nyamangara, J., and Gumbo, D. (2013). Opportunities for optimization of in-field water harvesting to cope with changing climate in semi-arid smallholder farming areas of Zimbabwe. SpringerPlus 2:100. doi: 10.1186/2193-1801-2-100
Omenda, J., Ngetich, K., Kiboi, M., Mucheru-Muna, M., and Mugendi, D. (2021). Phosphorus availability and exchangeable aluminum response to phosphate rock and organic inputs in the central Highlands of Kenya. Heliyon 7:e06371. doi: 10.1016/j.heliyon.2021.e06371
Opala, P. A., Okalebo, J. R., and Othieno, C. O. (2012). Effects of organic and inorganic materials on soil acidity and phosphorus availability in a soil incubation study. Agronomy 2012:10. doi: 10.5402/2012/597216
Ozlu, E., and Kumar, S. (2018). Response of soil organic carbon, pH, electrical conductivity, and water stable aggregates to long-term annual manure and inorganic fertilizer. Soil Sci. Soc. Am. J. 82, 1243–1251. doi: 10.2136/sssaj2018.02.0082
Rarieya, M., and Fortun, K. (2009). Food security and seasonal climate information, Kenyan challenges. Sustain. Sci. 5, 99–114. doi: 10.1007/s11625-009-0099-8
Recha, C. W., Mukopi, M. N., and Otieno, J. O. (2014). Socio-economic determinants of adoption of Rainwater harvesting and conservation techniques in semi-arid Tharaka Sub-County, Kenya. Land Degrad. Dev. 26, 765–773. doi: 10.1002/ldr.2326
Rockstrom, J. (2000). Water resources management in smallholder farms in eastern and Southern Africa: an overview. Phys. Chem. Earth B Hydrol. Oceans Atmos. 25, 275–283. doi: 10.1016/S1464-1909(00)00015-0
Rockstrom, J., and Karlberg, L. (2010). The quadruple squeeze: defining the safe operating space for freshwater use to achieve a triply Green Revolution in the Anthropocene. Ambio 39, 257–265. doi: 10.1007/s13280-010-0033-4
Rosegrant, M. W., Cai, X., and Cline, S. A. (2002). World Water and Food to 2025: Dealing With Scarcity. Washington, DC: International Food Policy Research Institute.
Rukshana, F., Butterly, C. R., Xu, J. M., Baldock, J. A., and Tang, C. (2013). Organic anion-to acid ratio influences pH change of soils differing in initial pH. Int. J. Soil Sediment. Water 14, 407–414. doi: 10.1007/s11368-013-0682-6
Sawadogo, H. (2011). Using soil and water conservation techniques to rehabilitate degraded lands in northwestern Burkina Faso. Int. J. Agric. Sustain. 9, 120–128. doi: 10.3763/ijas.2010.0552
Stevens, C. J., Dise, N. B., and Gowing, D. J. (2009). Regional trends in soil acidification and exchangeable metal concentrations in relation to acid deposition rates. Environ. Pollut. 157, 313–319. doi: 10.1016/j.envpol.2008.06.033
Thierfelder, C., and Wall, P. C. (2009). Effects of conservation agriculture techniques on infiltration and soil water content in Zambia and Zimbabwe. Soil Tillage Res. 105, 217–227. doi: 10.1016/j.still.2009.07.007
Tirol-Padre, A., Ladha, J. K., Regmi, A. P., Bhandari, A. L., and Inubushi, K. (2007). Organic amendments affect soil parameters in two long-term rice-wheat experiments. Soil Sci. Soc. Am. J. 71, 442–452. doi: 10.2136/sssaj2006.0141
Usman, M., and Reason, C. (2004). Dry spell frequencies and their variability over Southern Africa. Clim. Res. 26, 199–211. doi: 10.3354/cr026199
Van Diepeningen, A. D., De Vos, O. J., Korthals, G. W., and Van Bruggen, A. H. (2006). Effects of organic versus conventional management on chemical and biological parameters in agricultural soils. Appl. Soil Ecol. 31, 120–135. doi: 10.1016/j.apsoil.2005.03.003
Walkley, A., and Black, I. A. (1934). An examination of the degtjareff method for determining soil organic matter, and a proposed modification of the chromic acid titration method. Soil Sci. 37, 29–38. doi: 10.1097/00010694-193401000-00003
Wildemeersch, J. C., Garba, M., Sabiou, M., Sleutel, S., and Cornelis, W. (2015). The effect of water and soil conservation (WSC) on the soil chemical, biological, and physical quality of a Plinthosol in Niger. Land Degrad. Dev. 26, 773–783. doi: 10.1002/ldr.2416
Winterbottom, R., Reij, C., Garrity, D., Glover, J., Hellums, D., McGahuey, M., et al. (2013). Improving Land and Water Management. World Resources Institute Working Paper. Washington, DC: World Resources Institute.
Xin, X., Zhang, J., Zhu, A., and Zhang, C. (2016). Effects of long-term (23 years) mineral fertilizer and compost application on physical properties of fluvoaquic soil in the North China Plain. Soil Tillage Res. 156, 166–172. doi: 10.1016/j.still.2015.10.012
Zhao, S., Qiu, S., Cao, C., Zheng, C., Zhou, W., and He, P. (2014). Responses of soil properties, microbial community and crop yields to various rates of nitrogen fertilization in a wheat–maize cropping system in north-central China. Agric. Ecosyst. Environ. 194, 29–37. doi: 10.1016/j.agee.2014.05.006
Zhou, H., Fang, H., Mooney, S. J., and Peng, X. (2016). Effects of long-term inorganic and organic fertilizations on the soil micro and macro structures of rice paddies. Geoderma 266, 66–74. doi: 10.1016/j.geoderma.2015.12.007
Zhu, Q., Liu, X., Hao, T., Zeng, M., Shen, J., Zhang, F., et al. (2018). Modeling soil acidification in typical Chinese cropping systems. Sci. Total Environ. 613–614. doi: 10.1016/j.scitotenv.2017.06.257
Keywords: Zai technology, sorghum, integrated nutrient management, soil fertility, cattle manure, mineral fertilizer, total nitrogen
Citation: Kebenei MC, Mucheru-Muna M, Muriu-Ng'ang'a F and Ndung'u CK (2021) Zai Technology and Integrated Nutrient Management for Improved Soil Fertility and Increased Sorghum Yields in Kitui County, Kenya. Front. Sustain. Food Syst. 5:714212. doi: 10.3389/fsufs.2021.714212
Received: 24 May 2021; Accepted: 12 October 2021;
Published: 05 November 2021.
Edited by:
Jimmy R. Botella, The University of Queensland, AustraliaReviewed by:
Richard Onwonga, University of Nairobi, KenyaCargele Masso, International Institute of Tropical Agriculture (IITA), Nigeria
Copyright © 2021 Kebenei, Mucheru-Muna, Muriu-Ng'ang'a and Ndung'u. This is an open-access article distributed under the terms of the Creative Commons Attribution License (CC BY). The use, distribution or reproduction in other forums is permitted, provided the original author(s) and the copyright owner(s) are credited and that the original publication in this journal is cited, in accordance with accepted academic practice. No use, distribution or reproduction is permitted which does not comply with these terms.
*Correspondence: Mercy Cheruto Kebenei, mercycheruto@gmail.com