- 1Department of Plant Science, Central University of Kerala, Kasaragod, Kerala, India
- 2Department of Environmental Science, PGDAV College, University of Delhi, New Delhi, India
- 3Laboratory of Genetics, Biotechnology and Seed Sciences, Faculty of Agronomic Sciences, University of Abomey-Calavi, Cotonou, Benin
- 4School of Life Sciences, Jawaharlal Nehru University, New Delhi, India
Purslane (Portulaca oleracea L.) is a popular orphan crop used for its nutritional properties in various parts of the world. It is considered one of the richest terrestrial sources of omega-3 and omega-6-fatty acids (ω-3 and 6-FAs) suggesting its importance for human health. This ethnomedicinal plant is also an important part of traditional healing systems among the indigenous people. Many studies have indicated its tolerance against multiple stresses and found that it easily grows in a range of environmental gradients. It has also been considered one of the important biosaline crops for the future. Despite its huge nutritional, economic, and medicinal importance, it remains neglected to date. Most of the studies on purslane were focused on its ethnomedicinal, phytochemical, pharmacological, and stress-tolerance properties. Only a few studies have attempted genetic dissection of the traits governing these traits. Purslane being an important traditional food crop across the globe can be valorized for a sustainable food security in the future. Therefore, this review is an attempt to highlight the distribution, domestication, and cultivation of purslane and its importance as an important stress-tolerant food and a biosaline crop. Furthermore, identification of genes and their functions governing important traits and its potential for improvement using genomics tools for smart and biosaline agriculture has been discussed.
Introduction
The Portulaca L. is the only genus in the family Portulacaceae as per APG III classification of angiosperms (The Angiosperm Phylogeny Group, 2009; Ocampo and Columbus, 2012) and comprises more than 100 species which are widely distributed and adapted to a range of environmental conditions (Nyffeler and Eggli, 2010). The genus Portulaca shows a great phenotypic plasticity in its various traits such as flower, fruit, leaves, stem, and growing habit (Coelho and Giulietti, 2010). The members of Portulaca genus are either herbaceous perennials or annuals usually with tuberous roots, slightly succulent stem, and leaves with alternate or rarely opposite phyllotaxy and cymose terminal inflorescences (Nyffeler and Eggli, 2010; Walters et al., 2011; Ocampo and Columbus, 2012; Dalavi et al., 2018). The flowers are sessile or rarely pedicellate and are easily distinguishable by their inflorescence and fruits which are operculate capsules termed as pyxidia (Nyffeler and Eggli, 2010). The Portulaca is characterized by thick and dense homogenous populations in nature (Walters et al., 2011). Due to the presence of facultative C4/CAM photosynthetic pathways in purslane, it has become an important model crop for facultative C4/CAM photosynthesis evolution in plants and its future role for food security (D'Andrea et al., 2014; Ferrari et al., 2020). The species of Portulaca are adapted to the shady and moist environments, show extensive branching (Matthews and Levins, 1985), and are generally characterized by tuberous roots (Geesink, 1969; Carolin, 1987). The leaves are succulent in nature, and fruits are round or egg-shaped operculated capsules and produce tiny, oval-shaped reddish-, brown-, or black-colored seeds which are rich in phytochemicals (Matthews and Levins, 1985; Grubben, 2004). The leaves and inflorescences show the presence of trichomes (Matthews and Levins, 1985). It produces many seeds with very high dormancy (Alam et al., 2014e). Most of its species such as P. oleracea, Portulaca grandiflora, Portulaca amilis, Portulaca molokiniensis, Portulaca pilosa, and Portulaca umbraticola show C4 photosynthesis (Koch and Kennedy, 1982; Guralnick et al., 2002; Voznesenskaya et al., 2010). Interestingly, some C4 species such as P. oleracea, P. grandiflora, P. australis, P. pilosa, Portulaca digyna, and Portulaca cyclophylla also show Crassulacean acid metabolism (CAM) pathway, a special type of photosynthesis found in the succulent plants adapted to desert conditions (Sage, 2002). Most of the species except a few such as Portulaca cryptopetala show Kranz anatomy (Ocampo et al., 2013). However, the CAM pathway in the C4 Portulaca species operates only during drought conditions when water availability is reduced (Lara et al., 2004; D'Andrea et al., 2014). Besides a model plant for C4/CAM photosynthesis, it is also an important crop for understanding the salt-tolerance mechanisms in plants (Borsai et al., 2018, 2020). Of the more than 100 species of Portulaca, P. oleracea L. (hereafter purslane; Figures 1a–d) is the most important and well-studied species. Purslane also shows wide phenotypic plasticity in its flower colors that make it an important ornamental plant (Figure 2). Purslane is an important plant rich in important phytochemicals and nutritional components possessing medicinal, nutritional, medicinal, phytoremediation, stress-tolerance, and pharmacological properties (Alam et al., 2014c, 2015b; Uddin et al., 2014; Zhou et al., 2015). Purslane is also traditionally used as an ethnomedicinal plant (Sultana and Raheman, 2013; Iranshahy et al., 2017). Despite its multiple benefits, such as its nutritional and phytochemical richness, purslane still remains a neglected food crop of the indigenous communities, and studies on the genetic regulation of important traits and their improvement strategies is limited. Except for the C4-CAM switch, very limited information on distribution, domestication, and cultivation of purslane is available. Information on application of genetics and genomics for the improvement of nutritional and stress-resilient traits of purslane, and hence to be adopted for the extensive cultivation and industrial applications is not available. Furthermore, since the purslane grows profusely across a range of environmental gradients, and shows salinity tolerance, it is not surprising that the species holds a potential as a biosaline crop for urban agriculture. Taken together with this background, this review summarizes current knowledge on various aspects of purslane with an emphasis on its distribution, cultivation, domestication, and its importance as a nutritional, medicinal, and biosaline crop. It further discusses a futuristic approach combining genomics and gene-editing tools for the editing of genes governing important traits for urban and biosaline agriculture.
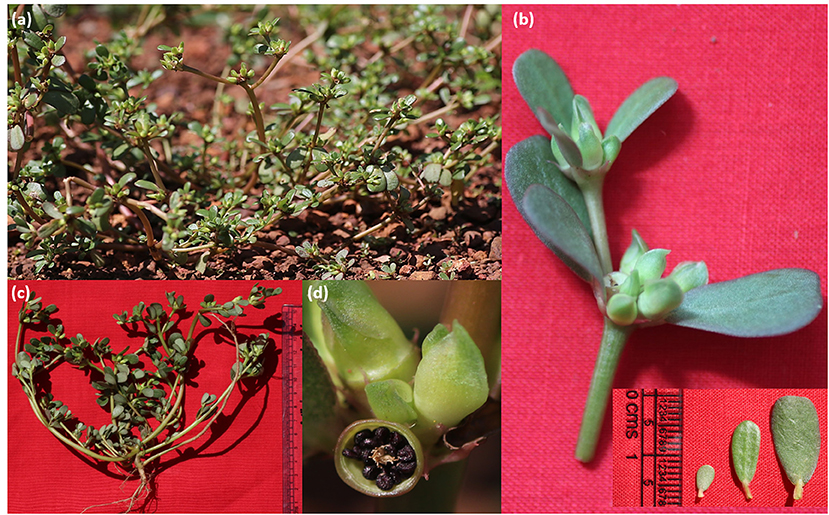
Figure 1. Natural habitat and parts of purslane plant. (a) Purslane plant in natural habitat; (b) young stem with leaves; (c) harvested plant showing roots; and (d) young fruits, known as pyxidia with black-colored seeds.
Distribution and Diversity of Purslane
Purslane is the eighth most commonly distributed plant in the world (Anastácio and Carvalho, 2013). The molecular phylogenetic study of the genus Portulaca shows that it is a monophyletic genus with two major clades, namely, opposite leaves (OL) and alternate leaves (AL; Ocampo and Columbus, 2012). The study further showed that OL clade includes species that possess opposite leaves and are distributed in Africa, Asia, and Australia, whereas AL clade represents species that possess alternate to subopposite leaves and are distributed in the New World. There was a debate around its origin in the Old World and its further introduction to the New World countries during the Columbus period. However, the presence of Pre-Columbian archaeological evidence in the New World suggests that purslane is distributed in the Old World as well as New World countries thereby ending the debate whether it was introduced by Columbus (Byrne and McAndrews, 1975). Recovery of the carbonized fossils of purslane from prehistoric human settlement sites suggests its importance as an important food plant for the indigenous communities since prehistoric times (Chapman et al., 1973; Byrne and McAndrews, 1975; Simopoulos et al., 1995). Simopoulos et al. (1995) suggested Mexico as the center of origin of purslane because the maximum number of species including its three ploidy types exists there. Although germplasm collections and their characterization for various important traits such as stress tolerance, superior metabolic and nutritional traits, and biomass accumulation are important for improvement of purslane; however, no such study reports are available in plenty.
Domestication and Cultivation of Purslane and Measures to Upscale ITS Production and Consumption
A very few studies have focused on the cultivation and domestication of purslane. Cros et al. (2007) carried out comparative study of its cultivation using peat, vermiculite, coir, perlite, and mixtures of peat and perlite and found that plants grown on peat showed the highest total fatty acid, alpha-linolenic acid, and linoleic acid. Application of Rhizophagus irregularis, an arbuscular mycorrhizal fungi (AMF) increased the phosphorus uptake, grain yield, biomass weight, total antioxidant activity, and unsaturated fatty acid, leaf essential oil, and leaf ω3 and improved the soil cation exchange capacity (CEC) of plants (Hosseinzadeh et al., 2020, 2021). Application of nitrogen fertilizers also improved phosphorus and nitrogen uptake, biomass weight, flavonoid content, total antioxidant capacity, and fatty acid content (Hosseinzadeh et al., 2020). The best season for its sowing is spring of fall, and it completes its life cycle in 60–75 days (Alam et al., 2014e). In Malaysia, nearly seven cultivars are cultivated for ornamental purposes and are propagated through cuttings (Alam et al., 2014e). However, the wild purslane can germinate through the seeds (Alam et al., 2014e). Although it is nutritionally and medicinally important across different cultures, it is considered a weed in several parts of the world (Banerjee and Mukherjee, 2002; Rapoport and Drausal, 2013; CABI, 2021).
Safdari and Kazemitabar (2009) have attempted tissue culture in wild and cultivated races of purslane using different explants and hormone concentrations, and the results show that 10 μM IBA combined with 5 or 10 μM BAP sufficiently induce callus formation in the wild race whereas 2.5 μM IBA was enough for root generation in both the races. A study on the development of in vitro plant regeneration and in vitro flowering using various concentrations of PGRS showed that 0.5 mg L−1 of kinetin is optimum for maximum shooting and 0.2 mg L−1 of GA3 induced bud formation in purslane (Sharma et al., 2011). Shekhawat et al. (2015) observed maximum shooting when MS medium was supplemented with 2.0 mg L−1 BAP. These studies provide a basis for the cultivation of purslane. However, to better understand the best performance conditions, further field trial experiments are needed. Moreover, the standardization of tissue culture protocols in purslane would be helpful for future breeding programs aimed at upscaling cultivation of purslane. The future studies should be aimed at the methods for improved productivity, phytochemical composition, and its suitability for urban agriculture. Dewanti et al. (2021) found the highest amount (9.73 mg kg−1) of vitamin C in purslane obtained from lowland areas.
Importance of Purslane
The purslane is cultivated in various parts of the world mostly for ornamental purposes because of its phenotypic diversity in its flower color and for nutritional and medicinal purposes because of its richness in important phytochemicals (Simopoulos et al., 1992; Coelho and Giulietti, 2010; Melilli et al., 2020). Figure 3 provides an overview of the importance of purslane for sustainable food security and human benefits.
Nutritional Value
A number of studies have shown that purslane is very rich in important nutritional components such as vitamins, proteins, carbohydrates, ω-3-FAs, carotenoids, and minerals (Simopoulos et al., 1995, 2005; Aberoumand, 2009; Uddin et al., 2014; Petropoulos et al., 2016; Alam et al., 2021). It shows a very high amount of ω-3-fatty acids which is not generally found in vegetarian diets suggesting its important role as a functional food (Palaniswamy et al., 2001; Alam et al., 2014e; Petropoulos et al., 2016). Several studies have done analysis of its various nutritional constituents. The purslane contain high quantity of protein, ash, fiber content, and minerals (Aberoumand, 2009; Almasoud and Salem, 2014; Uddin et al., 2014; Chugh et al., 2019). It is also a very good source of various types of vitamins including vitamins A and C (Guil-Guerrero and Rodríguez-García, 1999; Chugh et al., 2019). The high nutritional composition of purslane indicates its potential as an important nutraceutical food for the future.
Medicinal Potential
Purslane is an important traditional ethnomedicinal plant as its leaves, roots, and stems contains rich medicinally important phytochemicals such as alkaloids, flavonoids, phenolic acids, homoisoflavonoids, lignans, polysaccharides, and catecholamines (Banerjee and Mukherjee, 2002; Xiang et al., 2005; Lim and Quah, 2007; Alam et al., 2014b; Zhou et al., 2015). Several authors have reviewed the phytochemical composition of purslane. It has been a part of indigenous healthcare systems across the continents (Xiang et al., 2005; Bosi et al., 2009; Sultana and Raheman, 2013; Hwess et al., 2018; Sdouga et al., 2020; Zaman et al., 2020). Many ethnobotanical studies have reported its use against multiple diseases and ailments (Ahmad and Beg, 2001; Bosi et al., 2009; Nedelcheva, 2013; Sultana and Raheman, 2013; Hwess et al., 2018; Chaachouay et al., 2019; Manzanero-Medina et al., 2020; Nanagulyan et al., 2020). Recent pharmacological studies also suggest its important medicinal benefits against a number of diseases such as cancer, diabetes, and viral, bacterial, and fungal infections (Dong et al., 2010; Ye et al., 2015; Jin et al., 2017; Park and Han, 2018; Zhao et al., 2018; da Silva et al., 2019; Li et al., 2019; El-Desouky, 2021; Park et al., 2021; Tleubayeva et al., 2021). These results of pharmacological studies provided important evidence toward the potential of purslane in drug development against diseases.
Potential for Biosaline Agriculture
Salinity is also an important stressor that limits crop productivity. There is plenty of saline water, and if salinity-tolerant crops are available, we can use the saline water for agriculture and can reduce the usage of freshwater in agriculture (Flowers, 2004; Yamaguchi and Blumwald, 2005; Roy et al., 2014; Fita et al., 2015). Moreover, the current agricultural practices which involve the use of chemicals have increased the salinity of agricultural soils (Shrivastava and Kumar, 2015). Therefore, soils have become less fertile and the normal varieties cannot survive in the saline environments. Plants respond to salinity stresses in a variety of ways including expression of important genes related to salinity tolerance (Chandna et al., 2014; Borsai et al., 2018). Therefore, looking for crop plants which can withstand salinity condition should be one strategy so that saline environment can be utilized for agriculture.
Purslane is considered salinity tolerant and can perform even under high salinity conditions (Yazici et al., 2007; Kafi and Rahimi, 2011; Alam et al., 2014b,e; Hniličková et al., 2019). Experimental evidences by several studies showed that purslane could grow in saline soils, therefore, it could be popularized as an important biosaline crop (Hasanuzzaman et al., 2014; Panta et al., 2014; Dagar, 2018; Elouafi et al., 2020). The plant responds to salinity stress by the production of proline which is an important osmolyte and helps in salt tolerance (Yazici et al., 2007; Rahdari et al., 2012). Similar response is observed in another species, P. grandiflora suggesting its adaptability to stresses (Kichenaradjou et al., 2018). Studies have shown reduced accumulation of proteins and lipids following salinity stress and increased accumulation of carbohydrates (Morgan, 1992; Dhanapackiam and Ilyas, 2010). Unlike salt-sensitive crops, purslane is known to keep its photosynthetic activity on even under higher salinity conditions through increased chloroplast biosynthesis and chlorophyll accumulation (Rahdari et al., 2012), although, the application of salinity decreases its germination potential. Another study found that intermediate levels of salinity are not detrimental to purslane as they continue to perform well under moderate salinities (Teixeira and Carvalho, 2009). Several metabolites in response to salinity have been identified in P. oleracea (Zaman et al., 2020). Increased accumulation of proline in the salt-tolerant purslane genotypes correlated with higher expression of pyrroline-5-carboxylate synthetase (PC5S) gene which is a key enzyme for the biosynthesis of proline (Sdouga et al., 2019). Increased accumulation of proline and its correlation with PC5S show important transcriptional control of salt tolerance in purslane. Due to its salt-tolerance potential, it is suggested to be an appropriate crop, with greater pharmacological and nutritional potential that can be grown in areas where the irrigation water is saline and solar radiation levels are high (Franco et al., 2011).
Potential for Urban Agriculture
Another issue that we face today is the nonavailability of land resources in urban areas (Fischel, 1982; Fazal, 2000; Satterthwaite et al., 2010; Kapil, 2019). The urban populations are dependent on market foods that are not fresh and sometimes, it takes many days to reach the kitchens of urban areas (Satterthwaite et al., 2010). However, if crops are devised that takes very less space and gives high biomass, it can be adopted in the future especially by urban people/consumers to have quick chemical-free fresh vegetables and foods. As purslane takes very less space and produces huge biomass rich in very important nutrients and health-promoting phytochemicals, this crop has tremendous potential for urban agriculture (Ren and White, 2019).
Rich Terrestrial Source of Omega-3-Fatty Acids
Several quantitative studies from various parts of the world have reported very high amounts of ω-3-fatty acids in its various plant parts of purslane (Davis and Kris-Etherton, 2003; Simopoulos et al., 2005; Uddin et al., 2014). It is considered one of the richest sources of ω-3-fatty acids. Marine algae are also rich in ω-3-fatty acids; however, they are not available beyond the coastal areas. Moreover, cultivation of algae needs skill and expertise. In contrast, cultivation of purslane is very easy and does not require special care or expertise. Vegetarian and vegan diets are relatively low in alpha-linolenic acid (ALA), an important ω-3-fatty acid (Davis and Kris-Etherton, 2003). ALA is further broken down to eicosapentaenoic acid (EPA) and docosahexaenoic acid (DHA) and the latter are essential for cardiovascular health. However, plasma, tissue, and blood of vegetarians and vegans are also low EPA as well as DHA as these (Davis and Kris-Etherton, 2003; Rosell et al., 2005; Saunders et al., 2013). Lower EPA and DHA in vegetarians and vegans may lead to cardiovascular-related health issues. Dieticians and health experts suggest increased intake of ALA to vegetarians and vegan people since their diets are deficient in ALA (Davis and Kris-Etherton, 2003). The presence of a high amount of ALA in purslane suggests its suitability for vegetarians and vegans as an important source of ALA (Uddin et al., 2014). Therefore, the promotion of consumption of purslane among vegetarians and vegans is an important step toward enriching their diets with ALA, EPA, and DHA.
Wide Phenotypic Diversity and Adaptability to a Range of Environmental Gradients
The P. oleracea is a cosmopolitan species, and huge phenotypic plasticity is observed for various traits such as flower color, phytochemical constitution, leaf size and number, growth habit, and overall plant size (Danin et al., 1978; Ezekwe et al., 1999; Ren et al., 2011; Alam et al., 2014d). Ren et al. (2011) reported genetic variation study in purslane using amplified fragment length polymorphic markers in 10 drought-tolerant accessions from different geographical locations, namely, POS, PO, and Turkey (Turkey), Keren and Tokombiya (Eritrea), Golden E (UK), Golden G and T (Netherlands),Wild Greece (Greece), and Egyptium (Egypt). They reported Tokombiya and Egyptium as the most tolerant accessions to drought stress at the adult stage (Ren et al., 2011). Commercial cultivars, Glystrida 0425 and Purslane Dark Green, and local populations, Sari, Gorgan, and Aliabad originating from Iran had relatively higher biomass yield (Karkanis and Petropoulos, 2017). Ezekwe et al. (1999) have characterized eight accessions namely P. oleracea, P. sativa, Golden Gerber (GG Dutch), Garden (Gn Dutch), Golden (G England), and wild (W) accessions (Beltsville, Egyptian, and Greece) of purslane from different geographical regions for chemical composition, crude protein, total lipids, and carbohydrate content. They reported the highest crude protein content (27.1%) in Wild Greek accession and the highest omega fatty acid content in Dutch Garden accession (Ezekwe et al., 1999). Alam et al. (2014d) studied 45 accessions of purslane from the Western Peninsula of Malaysia and grouped them into seven types based on their characters such as leaf type and color, stem color, and flower type and color. Molecular analysis of these 45 accessions of purslane using expressed sequence tag (EST)-derived simple sequence repeat markers (ES-SSR) showed high genetic diversity among the accessions (Alam et al., 2015a). Evaluation of antioxidant compounds, antioxidant activities, and mineral composition of 13 collected purslane accessions showed wide phenotypic diversity (Alam et al., 2014c). Several accessions of purslane were screened for high salt tolerance (Alam et al., 2014b), and it was found that salinity affects phenolic compounds and antioxidant activities of 13 collected purslane germplasms (Alam et al., 2015b). Characterization of 45 accessions of West Peninsular, Malaysia for various morphological and physiological parameters and mineral composition identified potential accessions that can be used for supplementing the nutritional and mineral requirements (Alam et al., 2014a,e). Liu et al. (2000) have evaluated 12 varieties of purslane (nine Australian wild, two North American origins and one local), and the results of the study showed a wide variation in fatty acids and β-carotene composition. Lim and Quah (2007) characterized six cultivars (PO1–PO6) of purslane for total phenol content (TPC) and found that the ornamental cultivars have higher total phenolic (TPC) content and antioxidant activities than the common variety (PO1). Among the six varieties, PO6 showed the highest TPC and antioxidant activity. Tiwari et al. (2008) found that two species of Portulaca, i.e., P. tuberosa and P. oleracea are good heavy metal accumulators. Zhu et al. (2010) used 11 Portulaca accessions from five provinces of China and obtained consistent GC-MS and IR spectral fingerprints that are important for quality assurance. A comparative study of two subspecies of Portulaca, i.e., P. oleracea subs. granulatostellulata and P. oleracea subs. edulis for their growth and nutritional quality showed that both the subspecies were rich in minerals. However, K content was higher in P. oleracea subs. edulis, whereas the Ca content was higher in P. oleracea subs. granulatostellulata (Yun et al., 2016). Analysis of physicochemical properties of oil from seed, leaf, and stem of purslane from the Dire Dawa region of Ethiopia showed the highest oil content in seeds (11.25%) compared with leaf and stem (Desta and Cherie, 2018). Furthermore, the analysis of 13 common Malaysian-cultivated accessions of purslane showed differential morphological responses to salinity stress (Alam et al., 2016). The results showed that except plant height in Ac 1 (accession 1), all morphological parameters showed negative correlation with salinity and Ac 13 was the most affected accession among all. Egea-Gilabert et al. (2014) characterized 12 accessions of purslane using morphological, molecular, agronomical, and biochemical analyses and identified CM 13-00809 as an important accession with superior traits such as yield, dry matter, and potassium content. Kaşkar et al. (2009) analyzed agronomic parameters of several accessions of purslane such as Golden purslane, “C,” one local Turkish accession, and three Spanish accessions and found variation in various parameters such as nitrate and oxalate content, plant height, number of leaves, leaf area, and yield component. They further found that accession “C” which was obtained from Pasa Seeds company showed better agronomic traits which includes plant height, number of leaves, leaf area, and yield component. The Turkish accession showed minimum amounts of oxalate and nitrates (undesirable/antinutrients) suggesting their suitability for human consumption. Analysis of 19 characters in four populations of purslane showed wide interpopulation heterogeneity in Tunisia (Salah and Chemli, 2004). Sdouga et al. (2020) found wide diversity in morphological and biochemical characters between wild populations and cultivated varieties. Karkanis and Petropoulos (2017) studied comparative physiological responses under Mediterranean semiarid conditions between two commercial cultivars, three local populations of Iran, and one local population of Greece and found that the Aliabad, a local population of Iran showed the highest yields. Balabanova et al. (2020) analyzed 33 Bulgarian and five Greek accessions of purslane and reported 50 metabolite markers. Metabolic profiling of leaves and roots of two purslane genotypes, Tall Green (TG) and Shandong Wild (SD), under the control and saline exposures was performed by Zaman et al. (2020) who found a huge variation in the concentration of 132 metabolites under control and treated conditions. Furthermore, salinity-induced reduction in the number of leaves and roots, diameter of the stem, and length of roots was reported.
Besides its tolerance to a range of environmental conditions (Miller et al., 1984; Simopoulos, 2011), it produces a very high number of seeds with very good germination rate even under stress conditions (Fernández et al., 2008; Chauhan and Johnson, 2009; Alam et al., 2014e; Ren and White, 2019). Purslane grows profusely even on the less fertile and waste lands (Nyffeler and Eggli, 2010; Subramanyan, 2017). Its adaptability to various climatic conditions makes it a suitable crop for extensive agriculture. Various varieties are regionally important, and people collect it from the natural habitats (Turner et al., 2011; Uddin et al., 2014; Borelli et al., 2020). In some cases, people also cultivate it at a small scale especially for ornamental purposes (Osma et al., 2014). Efforts must be taken to exploit its genetic diversity for regional agricultural programs based on the availability and suitability of Portulaca species/cultivar. Moreover, except for P. oleracea, only a few other species of Portulaca are studied to date. This suggests that further studies on other species of Portulaca will help us to identify potential genotypes including higher content of ω-3-fatty acids and other nutritional and phytochemical components.
Important Model Crop for C4/CAM Facultative Photosynthetic Pathways
Portulaca oleracea is an important plant that uses both C4 and CAM photosynthetic pathways depending upon the prevailing situations (Lara et al., 2004; D'Andrea et al., 2014). The C4-CAM switching depending upon the prevailing environmental conditions makes it an important and desirable crop for the twenty-first century. The scientists are working toward development of climate-smart crops using the gene and genome engineering/editing tools (DeLisi et al., 2020; Zaidi et al., 2020). However, purslane is a naturally occurring smart crop that operates its machinery smartly (D'Andrea et al., 2014). Due to the presence of C4 and CAM enzymes in purslane, it has become an important model crop for C4-CAM facultative photosynthesis studies. It behaves as a C4 plant when grown under normal conditions whereas it switches to CAM pathway under drought conditions. Since CAM pathway is a highly water-efficient system of photosynthesis, switching into CAM by purslane surely helps it to survive under drought conditions (D'Andrea et al., 2014). Further investigation on the mechanisms governing C4-CAM switching in purslane and the identification of the genes would provide important insights into this important facility used by purslane (Winter and Holtum, 2014). The enzymes that are activated during these situations have been studied (Lara et al., 2003, 2004; D'Andrea et al., 2014, 2015).
Emergency Food During COVID-19-Like Situations
Various indigenous communities rely on traditional food plants including purslane for their nutritional requirements (Kuhnlein and Receveur, 1996; Pieroni et al., 2005; Muthoni and Nyamongo, 2010; Uddin et al., 2014; Pawera et al., 2020). Traditional plants are also used for medicinal purposes in various parts of the world (Mahomoodally et al., 2012; Shikov et al., 2017; Kumar et al., 2019). As discussed, purslane is also one of the most important ethnomedicinal plants among various countries (Ross, 2003; Bosi et al., 2009; Iranshahy et al., 2017). The traditional food plants are regionally very important and local communities rely on them for their nutritional needs. During the coronavirus disease 2019 (COVID-19)-like situations, purslane can be one of the important emergency foods for far-flung areas where food aid is disrupted due to COVID-19-like situations in the future. It is not surprising that COVID-19 has disturbed the global supply chains of the foods, and in the future, such crops can be important for sustaining the communities that lack basic food distribution.
Stress Tolerance in Purslane
The depletion of water resources is one of the biggest challenges to modern agriculture in the world (FAO, 2011). It directly translates to reduced productivity and yields, and if arising in a large-scale agricultural land, would lead to global food insecurity (Falkenmark, 2013; Misra, 2014). Scientists across the globe are putting efforts to devise and innovate strategies to engineer new varieties of crops that can perform well even under extreme conditions such as drought condition (Zhang et al., 2000, 2018; Jewell et al., 2010; Vandenbroucke and Metzlaff, 2013; Jaganathan et al., 2018; Zaidi et al., 2020). Considering the global water shortages, and the susceptibility of the modern crop cultivars to drought conditions, it is highly desirable to discover new crops/varieties that are naturally drought tolerant.
Researchers are putting efforts to use genetic engineering tools to modify currently available crops for increased stress tolerance. Some studies have shown encouraging results, although, not up to the mark. Therefore, the alternative option is to discover naturally occurring stress-tolerant plants that can perform well under stressful conditions. Among all, drought, salinity, heat, and temperature stresses are some of the important abiotic stresses concerning agriculture (Yang et al., 2012; Jin et al., 2015). Drought is also an important environmental stress which limits plant growth and development and affects yields (Ren et al., 2011). Several studies have indicated that purslane is drought tolerant and can easily grow in extreme drought conditions (Ren et al., 2011; Rahdari et al., 2012). Jin et al. (2015) studied drought and heat stress tolerance of purslane and observed significant increase in the malondialdehyde (MDA), proline, electrolyte leakage, reactive oxygen species (ROS), and antioxidant activities and decrease in leaf water content (LWC) and chlorophyll content during the progressive drought treatment. The rehydration following drought stress reversed the negative parameters such as LWC and chlorophyll content. Activation and regulation of various physiological mechanisms makes purslane more efficient to cope up with stress conditions and recover during rehydration (Jin et al., 2015). Earlier study has also shown rapid recovery of purslane plants following dehydration (D'Andrea et al., 2014). Purslane responds to stresses at multiple levels using various mechanisms. One study suggests that switching of CAM pathways enhances the water use efficiency and drought tolerance in purslane (Liu et al., 2018). Differences in the metabolites and the enzymes required during their synthesis in the well-irrigated and drought conditions are also observed, suggesting a biochemical basis of salt tolerance in purslane (Lara et al., 2003, 2004). It also improves its tolerance by enhancing the production of antioxidants, proline content, and heat-shock proteins which are all important for survival under high stress conditions (Yang et al., 2012).
Traditional Food Systems That Are Based On Purslane
Traditional food plants are an important part of indigenous diets, and they are produced within the restricted local areas (FAO, 1988). Culturally accepted food patterns evolved from available local resources are referred to as traditional food systems (Kuhnlein and Receveur, 1996). Traditional food systems reflect the culture indigenous communities and their dietary patterns (Trichopoulou et al., 2007). Traditional food plants are healthy, nutritious, and serve as the sustainable food resources for indigenous rural communities (Legwaila et al., 2011). A wide variety of food plants form a part of traditional food systems across the world (Turner et al., 2011). Various studies have reported the use of purslane as an important part of traditional food systems (Welcome and Van Wyk, 2019). It is consumed as a vegetable in a variety of ways across the globe, and the mode of preparation and consumption of purslane varies from region to region. It is used as a raw salad in Turkey, Kufla ka saag in India, and as traditionally processed papads in India (Kapoor et al., 2010; Renu and Waghray, 2016; Borelli et al., 2020). It is also consumed in many other countries and forms a basis for novel traditional foods. Table 1 presents a summary of traditional food systems that are based on purslane across 18 countries. This table provides information related to its local names, mode of preparation, and consumption.
Genetics of Phytochemical, Nutritional, and Stress-Resilient Traits
Since purslane is known for its medicinal and nutritional properties due to the synthesis of important nutrients and phytochemicals such as alkaloids, flavonoids, catecholamines, lignans, terpenoids, betalains, carotenoids, vitamins, and ω-3-fatty acids (Oliveira et al., 2009; Mulla and Swamy, 2010; Singh et al., 2011; Patil et al., 2012; Petropoulos et al., 2016; Verma et al., 2016; Fernández-Poyatos et al., 2021) and tolerance toward several stresses such as drought, temperature, salinity, moisture, and heat (Ichimura and Suto, 1998; Yazici et al., 2007; Alam et al., 2014b; Jin et al., 2016; Borsai et al., 2020; Xing et al., 2020), it is attaining greater attention (Sultana and Raheman, 2013; Uddin et al., 2014). Many plant-derived secondary metabolites have been used as drugs in modern times as these compounds have specific biological activities (Kumar et al., 2019). It is important to isolate and identify the genes that govern stress-resilient traits and biosynthesis of metabolites. Identification of genes involved in biosynthetic pathways of important metabolites and stress-resilient traits is useful for the trait improvement programs and for the development of superior varieties. Only a few studies have reported the genes regulating important traits in purslane. Among the various phytochemicals synthesized in purslane, dopamine is an important catecholamine with a neuroprotective potential (Chen et al., 2003; Chugh et al., 2019). It is important to identify and characterize the genes that synthesize dopamine. Recently, genes for two enzymes tyrosine decarboxylase and tyrosinase have been identified from hair roots using A. rhizogenes (Babashpour et al., 2018). Two genes encoding ω-3 fatty acid desaturases viz. FAD7 and FAD8 which are involved in the conversion of linoleic to ω-3-linolenic acid have been identified from purslane (Teixeira et al., 2010). In addition to synthesizing ω-3 fatty acids, purslane also produces ω-6-fatty acids. Du et al. (2021) identified 94 genes involved in the biosynthetic pathway of unsaturated fatty acids. Three genes encoding ω-6 fatty acid desaturases viz. FAD2-1, FAD2-2, and FAD-6 have been isolated (Teixeira et al., 2009). FAD2 genes are plasticidal whereas FAD6 is microsomal. Differential expressions of several genes in response to drought stress and after rewatering have been reported in purslane (D'Andrea et al., 2015). The study showed that three important genes, namely, Ribosomal protein S15A (RPS15A), Ribosomal protein L2 (RPL2), and ω-6-fatty acid desaturase 2 (FAD2) were induced at higher levels following rewatering/recovery of purslane, suggesting their important roles in imparting stress tolerance. FAD2 and stearoyl-acyl-carrier-protein desaturase (SAD) are also important genes involved in ω-3 fatty acid biosynthesis, and there differential expression is reported in stem and leaves of P. oleracea (Du et al., 2021). C4/CAM switching is one of the most important mechanisms reported from purslane in response to the differences in environmental conditions. It uses C4 machinery at normal irrigated conditions whereas it switches to CAM when water scarcity is sensed. The enzymes that are activated during C4 and CAM environmental conditions have been studied (Lara et al., 2003, 2004; D'Andrea et al., 2014, 2015). However, the identification of genes governing C4/CAM switch which enables plants to survive under different environmental conditions would be immensely helpful to manipulate this mechanism in purslanes by genetic engineering.
Improvement of Purslane Using Genomics and Gene-editing Tools for Superior Traits and Urban Agriculture
Crop improvement involves strategies for the trait enhancement of the plants for superior characters (Singh et al., 2020). Various strategies including conventional and modern approaches have been used for crop improvement programs (Breseghello and Coelho, 2013). The characterization and identification of diverse germplasm is an important step toward further crop-improvement programs (Ezekwe et al., 1999; Alam et al., 2014b; Egea-Gilabert et al., 2014). Few researchers have attempted and successfully standardized tissue culture protocols and Agrobacterium-mediated transformation (Rossi-Hassani et al., 1995; Sedaghati et al., 2019, Sedaghati et al., 2021). Although the advancement of molecular techniques including genomics and other omics technologies enabled the analysis of genomes, proteomes, metabolomes, and ionomes of the crop plants (Salt, 2004; Kumari et al., 2015; Ramalingam et al., 2015), however, such studies are lacking in purslane. The genomics studies in combination with other omics studies such as metabolomics and proteomics will provide useful information about the regulatory networks that contribute to the stress tolerance, diversity of phytochemicals, and other nutritional traits in purslane besides shortening the time required for breeding superior cultivars (Singh et al., 2020). Furthermore, the gene-editing tools can also be exploited for editing the important genes and customization of the purslane cultivars for urban and biosaline agriculture (Abdallah et al., 2015). The purslane improvement studies must focus on traits such as biomass, yield, maturity, flowering, seeds, and stress tolerance (Alam et al., 2014e). Since purslane is highly nutritious orphan crops with a number of superior traits, attempts should be made to decipher its genetic makeup and other regulators such as noncoding RNAs and epigenetic marks using genomics tools. The identification of genes will enable to manipulate and improve desirable traits including enhancement of ω-3-FAs, its CAM pathway, nutritional content, stress tolerance/resistance, and other important traits using conventional and modern genome-editing tools. Furthermore, a large-scale profiling of nutrients and essential mineral elements using metabolomics and ionomics and establishing links with their genes would help us to decipher the importance of genetics of these traits. Figure 4 represents an integrative strategy involving the use of genomics and gene editing for the improvement of purslane.
Challenges to Cultivation and Improvement of Purslane
Purslane is a nutritionally rich traditionally important orphan crop which is not either cultivated or consumed on a large scale. Although it has multiple desirable traits, it has unpalatable taste due to which it is not widely consumed by people. To increase its production and consumption, awareness programs about its health benefits must be conducted. Although wide variation of purslane is reported, detail collection and characterization to identify potential germplasm to be used in crop improvement has not been reported (Ramanatha Rao and Hodgkin, 2002: Alam et al., 2014a, 2015a). The lack of germplasm collections in different gene/germplasm banks across the globe is also one of the reasons why it remained neglected to date. Furthermore, there are very limited genetic and genomics resources of purslane to date. Future attempts should be made to increase germplasm collections; their characterization, phenotyping, and genotyping using various omics techniques should be attempted for purslane to be used in improvement programs.
Conclusion and Future Directions
Purslane, being an important traditional crop with multiple health benefits and inherent stress-tolerant mechanism has tremendous potential to be adopted for cultivation during this time of global climate change, salinity, drought, and urbanization-related problems. The climate-smart crops should be able to grow and adapt in stressful environments such as drought, high temperature, and submergence machinery, and these properties are inherently present in purslane. Purslane uses water-efficient CAM pathway for photosynthesis during drought conditions and switches to C4 machinery when the temperature is very high (Simopoulos et al., 1995; Kamil et al., 2000; Ferrari et al., 2020), which is a potential trait for designing climate-smart crop (D'Andrea et al., 2014). Facultative CAM pathway of purslane and C4 metabolism makes it an important crop for water-scarce regions of the world (Welkie and Caldwell, 1970; Koch and Kennedy, 1980; Ocampo et al., 2013; Winter and Holtum, 2014; Liu et al., 2018). Therefore, its cultivation can also be attempted in the drought-prone regions of the world. Furthermore, its salinity-tolerance character makes it fit for biosaline agriculture where freshwater (normal) is not in plenty (Borsai et al., 2018, 2020). However, the mechanisms of salinity tolerance still need to be investigated in detail. The development of transformation protocols is important for gene editing or genetic modification of crops. A research group has standardized and developed an efficient protocol of Agrobacterium-mediated transformation in P. oleracea (Sedaghati et al., 2019). The induction of hairy root is a key step toward in vitro production of secondary metabolites, and it has been successfully achieved in P. oleracea (Moghadam et al., 2014). Therefore, the availability of Agrobacterium-mediated transformation protocol may ensure genetic manipulation experiments once the genes governing important traits are identified. Furthermore, other gene editing technologies may also be attempted in purslane for manipulating genes governing important traits. The application of multi-omics high-throughput technologies such as genomics, transcriptomics, metabolomics, proteomics, and ionomics and their integrated analysis would help to identify genes, understand the regulatory mechanism, and help in manipulation of important traits. Lastly, we suggest and recommend for popularizing the cultivation and consumption of traditional orphan food crop purslane in a large scale owing to its high nutrition and health beneficial compound content and easy to cultivate in less space and multiple stress environments.
Author Contributions
All authors have directly and significantly contributed to this work and approved the final draft for publication.
Conflict of Interest
The authors declare that the research was conducted in the absence of any commercial or financial relationships that could be construed as a potential conflict of interest.
Publisher's Note
All claims expressed in this article are solely those of the authors and do not necessarily represent those of their affiliated organizations, or those of the publisher, the editors and the reviewers. Any product that may be evaluated in this article, or claim that may be made by its manufacturer, is not guaranteed or endorsed by the publisher.
Acknowledgments
The authors would like to acknowledge Aysha Rooby MK, Aryasree M, Arjun Dinesh M, Vaishnav S. Babu, and Somitta Augusthy for contributions to Figure 2. AK extends gratitude to the Central University of Kerala for the support during the work.
References
Abdallah, N. A., Prakash, C. S., and McHughen, A. G. (2015). Genome editing for crop improvement: challenges and opportunities. GM Crops Food 6, 183–205. doi: 10.1080/21645698.2015.1129937
Aberoumand, A. (2009). Identification of fatty acids in edible wild plants by gas chromatography. Food Anal. Methabermods 2, 208–211. doi: 10.1007/s12161-008-9063-y
Achigan-Dako, E.M.W P, Komlan, F., N'Danikou, S., Dansi, A., et al. (2010). Traditional Vegetables in Benin. Cotonou: Institut National des Recherches Agricoles du Bénin, Imprimeries du CENAP
Ahmad, I., and Beg, A. Z. (2001). Antimicrobial and phytochemical studies on 45 Indian medicinal plants against multi-drug resistant human pathogens. J. Ethnopharmacol. 74, 113–123. doi: 10.1016/S0378-8741(00)00335-4
Alam, A., Juraimi, A. S., Yusop, M. R., Hamid, A. A., and Hakim, A. (2014a). Morpho-physiological and mineral nutrient characterization of 45 collected purslane (Portulaca oleracea l.) accessions. Bragantia 73, 426–437. doi: 10.1590/1678-4499.253
Alam, M. A., Juraimi, A. S., Rafii, M. Y., Abdul Hamid, A., and Aslani, F. (2014b). Screening of purslane (Portulaca oleracea L.) accessions for high salt tolerance. Sci. World J. 2014. doi: 10.1155/2014/627916
Alam, M. A., Juraimi, A. S., Rafii, M. Y., Abdul Hamid, A., Aslani, F., Hasan, M. M., et al. (2014c). Evaluation of antioxidant compounds, antioxidant activities, and mineral composition of 13 collected purslane (Portulaca oleracea L.) accessions. BioMed Res. Int. 2014:296063. doi: 10.1155/2014/296063
Alam, M. A., Juraimi, A. S., Rafii, M. Y., Hamid, A. A., Arolu, I. W., and Latif, M. A. (2015a). Application of EST-SSR marker in detection of genetic variation among purslane (Portulaca oleracea L.) accessions. Rev. Bras. Bot. 38, 119–129. doi: 10.1007/s40415-014-0103-0
Alam, M. A., Juraimi, A. S., Rafii, M. Y., Hamid, A. A., Aslani, F., and Alam, M. Z. (2015b). Effects of salinity and salinity-induced augmented bioactive compounds in purslane (Portulaca oleracea L.) for possible economical use. Food Chem. 169, 439–447. doi: 10.1016/j.foodchem.2014.08.019
Alam, M. A., Juraimi, A. S., Rafii, M. Y., Hamid, A. A., Aslani, F., and Hakim, M. A. (2016). Salinity-induced changes in the morphology and major mineral nutrient composition of purslane (Portulaca oleracea L.) accessions. Biol. Res. 49, 1–19. doi: 10.1186/s40659-016-0084-5
Alam, M. A., Juraimi, A. S., Rafii, M. Y., Hamid, A. A., Kamal Uddin, M., Alam, M. Z., et al. (2014e). Genetic improvement of purslane (Portulaca oleracea L.) and its future prospects. Mol. Biol. Rep. 41, 7395–7411. doi: 10.1007/s11033-014-3628-1
Alam, M. A., Juraimi, A. S., Rafii, M. Y., Hamid, A. A., and Aslani, F. (2014d). Collection and identification of different purslane (Portulaca oleracea L.) accessions available in Western Peninsular Malaysia. Life Sci. J. 11, 431–437.
Alam, M. A., Nadirah, T. A., Mohsin, G. M., Saleh, M., Moneruzzaman, K. M., Aslani, F., et al. (2021). Antioxidant compounds, antioxidant activities, and mineral contents among underutilized vegetables. Int. J. Veg. Sci. 27, 157–166. doi: 10.1080/19315260.2020.1748785
Almasoud, A. G., and Salem, E. (2014). Nutritional quality of purslane and its crackers. Middle East J. Appl. Sci. 4, 448–454.
Anastácio, A., and Carvalho, I. S. (2013). Accumulation of fatty acids in purslane grown in hydroponic salt stress conditions. Int. J. Food Sci. Nutr. 64, 235–242. doi: 10.3109/09637486.2012.713915
Babashpour, S., Piri, K., Saify-Nabiabad, H., and Sabouni, F. (2018). Identification, cloning and structural analysis of major genes from Portulaca oleracea L. hairy roots that involved in the biosynthesis of dopamine. J. Med. Plants By-products 7, 153–162. doi: 10.22092/jmpb.2018.118143
Balabanova, V., Hristov, I., Zheleva-Dimitrova, D., Sugareva, P., Lozanov, V., and Gevrenova, R. (2020). Bioinformatic insight into Portulaca oleracea L. (purslane) of bulgarian and Greek origin. Acta Biol. Cracoviensia Ser. Bot. 62, 7–21. doi: 10.24425/abcsb.2020.131662
Banerjee, G., and Mukherjee, A. (2002). Biological activity of a common weed - Portulaca oleracea L. II. Antifungal activity. Acta Bot. Hung. 44, 205–208. doi: 10.1556/ABot.44.2002.3-4.1
Beidokhti, M. N., Lobbens, E. S., Rasoavaivo, P., Staerk, D., and Jäger, A. K. (2018). Investigation of medicinal plants from Madagascar against DPP-IV linked to type 2 diabetes. South Afr. J. Bot. 115, 113–119. doi: 10.1016/j.sajb.2018.01.018
Borelli, T., Hunter, D., Powell, B., Ulian, T., Mattana, E., Termote, C., et al. (2020). Born to eat wild: an integrated conservation approach to secure wild food plants for food security and nutrition. Plants 9:1299. doi: 10.3390/plants9101299
Borsai, O., Al Hassan, M., Negruşier, C., Raigón, M. D., Boscaiu, M., Sestraş, R. E., et al. (2020). Responses to salt stress in Portulaca: insight into its tolerance mechanisms. Plants 9, 1–24. doi: 10.3390/plants9121660
Borsai, O., Hassan, M., Bo_caiu, M., Sestras, R., and Vicente, O. (2018). The genus Portulaca as a suitable model to study the mechanisms of plant tolerance to drought and salinity. EuroBiotech J. 2, 104–113. doi: 10.2478/ebtj-2018-0014
Bosi, G., Guarrera, P., Rinaldi, R., and Mazzanti, M. (2009). Ethnobotany of purslane (Portulaca oleracea L.) in Italy and morphobiometric analyses of seeds from archaeological sites in the Emilia Romagna Region (Northern Italy), in Plants and Culture: Seeds of the Cultural Heritage of Europe, eds Morel, J. P., and Mercuri, A. M., (Bari, Italy: Edipuglia), 129–139.
Breseghello, F., and Coelho, A. S. G. (2013). Traditional and modern plant breeding methods with examples in Rice (Oryza sativa L.). J. Agric. Food Chem. 61, 8277–8286. doi: 10.1021/jf305531j
Bussmann, R., Zambrana, N., Sikharulidze, S., Kikvidze, Z., and Kikodze, D. (2016). Medicinal and food plants of Svaneti and Lechkhumi, Sakartvelo (Republic of Georgia), Caucasus. Med. Aromat. Plants 5, 2167–0412. doi: 10.4172/2167-0412.1000266
Byrne, R., and McAndrews, J. H. (1975). Pre-Columbian purslane (Portulaca oleracea L.) in the new world. Nature 253, 726–727. doi: 10.1038/253726a0
CABI (2021). Portulaca oleracea (purslane). CABI Invasive Species Compend. Available online at: https://www.cabi.org/isc/datasheet/43609 (accessed May 1 2021).
Carolin, R. (1987). A review of the family Portulacaceae. Aust. J. Bot. 35, 383–412. doi: 10.1071/BT9870383
Chaachouay, N., Benkhnigue, O., Fadli, M., El Ibaoui, H., and Zidane, L. (2019). Ethnobotanical and ethnopharmacological studies of medicinal and aromatic plants used in the treatment of metabolic diseases in the Moroccan Rif. Heliyon 5, e02191–e02191. doi: 10.1016/j.heliyon.2019.e02191
Chandna, R., Azooz, M., and Ahmad, P. (2014). Recent advances of metabolomics to reveal plant response during salt stress. Salt Stress Plants Signal. Omics Adapt. 2013, 1–14. doi: 10.1007/978-1-4614-6108-1_1
Chapman, J., Stewart, R. B., and Yarnell, R. A. (1973). Archaeological evidence for precolumbian introduction of Portulaca oleracea and mollugo verticillata into Eastern North America. Econ. Bot. 28, 411–412. doi: 10.1007/BF02862857
Chauhan, B., and Johnson, D. E. (2009). Seed germination ecology of Portulaca oleracea L.: an important weed of rice and upland crops. Ann. Appl. Biol. 155, 61–69. doi: 10.1111/j.1744-7348.2009.00320.x
Chen, J., Shi, Y.-P., and Liu, J.-Y. (2003). Determination of noradrenaline and dopamine in Chinese herbal extracts from Portulaca oleracea L. by high-performance liquid chromatography. J. Chromatogr. A 1003, 127–132. doi: 10.1016/S0021-9673(03)00786-6
Chugh, V., Mishra, V., and Sharma, K. (2019). Purslane (Portulaca oleracea L.): an underutilized wonder plant with potential pharmacological value. Pharma Innov. 8, 236–246.
Coelho, A. A. O. P., and Giulietti, A. M. (2010). The genus Portulaca L. (Portulacaceae) in Brazil. Acta Bot. Bras. 24, 655–670. doi: 10.1590/S0102-33062010000300009
Cros, V., Martínez-Sánchez, J. J., Fernández, J. A., Conesa, E., Vicente, M. J., Franco, J. A., et al. (2007). Salinity effects on germination and yield of purslane (Portulaca oleracea L.) in a hydroponic floating system. Acta Hortic. 747, 571–579. doi: 10.17660/ActaHortic.2007.747.74
da Silva, J. D. F., da Silva, S. P., da Silva, P. M., Vieira, A. M., de Araújo, L. C. C., de Albuquerque Lima, T., et al. (2019). Portulaca elatior root contains a trehalose-binding lectin with antibacterial and antifungal activities. Int. J. Biol. Macromol. 126, 291–297. doi: 10.1016/j.ijbiomac.2018.12.188
Dagar, J. C. (2018). Utilization of degraded saline habitats and poor-quality waters for livelihood security. Sch. J. Food Nutr. 1, 1–19. doi: 10.32474/sjfn.2018.01.000115
Dalavi, J., Deshmukh, P., Jadhav, V., and Yadav, S. (2018). Two new species of Portulaca (Portulacaceae) from india. Phytotaxa 376, 68–76. doi: 10.11646/phytotaxa.376.1.7
D'Andrea, R. M., Andreo, C. S., and Lara, M. V. (2014). Deciphering the mechanisms involved in Portulaca oleracea (C4) response to drought: Metabolic changes including crassulacean acid-like metabolism induction and reversal upon re-watering. Physiol. Plant. 152, 414–430. doi: 10.1111/ppl.12194
D'Andrea, R. M., Triassi, A., Casas, M. I., Andreo, C. S., and Lara, M. V. (2015). Identification of genes involved in the drought adaptation and recovery in Portulaca oleracea by differential display. Plant Physiol. Biochem. 90, 38–49. doi: 10.1016/j.plaphy.2015.02.023
Danin, A., Baker, I., and Baker, H. G. (1978). Cytogeography and taxonomy of the Portulaca oleracea L. polyploid complex. Isr. J. Bot. 27, 177–211.
Davis, B. C., and Kris-Etherton, P. M. (2003). Achieving optimal essential fatty acid status in vegetarians: current knowledge and practical implications. Am. J. Clin. Nutr. 78, 640S−646S. doi: 10.1093/ajcn/78.3.640S
DeLisi, C., Patrinos, A., MacCracken, M., Drell, D., Annas, G., Arkin, A., et al. (2020). The role of synthetic biology in atmospheric greenhouse gas reduction: prospects and challenges. BioDesign Res. 2020:1016207. doi: 10.34133/2020/1016207
Desta, Z. Y., and Cherie, D. A. (2018). Determination of antioxidant and antimicrobial activities of the extracts of aerial parts of Portulaca quadrifida. Chem. Cent. J. 12:146. doi: 10.1186/s13065-018-0514-2
Dewanti, F. D., Pujiasmanto, B., and Sukendah Yunus, A. (2021). Analysis of ascorbic acid content (vitamin C) of purslane (Portulaca oleracea L.) at various altitudes in East Java, Indonesia. IOP Conf. Ser. Earth Environ. Sci. 637:012074 doi: 10.1088/1755-1315/637/1/012074
Dhanapackiam, S., and Ilyas, M. (2010). Effect of salinity on chlorophyll and carbohydrate contents of Sesbania grandiflora seedlings. Indian J. Sci. Technol. 3, 64–66. doi: 10.17485/ijst/2010/v3i1.20
Dong, C.-X., Hayashi, K., Lee, J.-B., and Hayashi, T. (2010). Characterization of structures and antiviral effects of polysaccharides from Portulaca oleracea L. Chem. Pharm. Bull. (Tokyo) 58, 507–510. doi: 10.1248/cpb.58.507
Du, H., Zaman, S., Hu, S., and Che, S. (2021). Single-molecule long-read sequencing of purslane (Portulaca oleracea) and differential gene expression related with biosynthesis of unsaturated fatty acids. Plants 10:655. doi: 10.3390/plants10040655
Ediriweera, E. R. H. S. S. (2010). A review on medicinal uses of weeds in Sri Lanka. Trop. Agric. Res. Ext. 10, 11–16. doi: 10.4038/tare.v10i0.1865
Egea-Gilabert, C., Ruiz-Hernández, M. V., Parra, M. T., and Fernández, J. A. (2014). Characterization of purslane (Portulaca oleracea L.) accessions: suitability as ready-to-eat product. Sci. Hortic. 172, 73–81. doi: 10.1016/j.scienta.2014.03.051
El-Desouky, T. A. (2021). Evaluation of effectiveness aqueous extract for some leaves of wild edible plants in Egypt as anti-fungal and anti-toxigenic. Heliyon 7:e06209. doi: 10.1016/j.heliyon.2021.e06209
Elouafi, I., Shahid, M. A., Begmuratov, A., and Hirich, A. (2020). The Contribution of Alternative Crops to Food Security in Marginal Environments, in Emerging Research in Alternative Crops, eds Hirich, A., Choukr-Allah, R., and Ragab, R., (Cham: Springer International Publishing), 1–23. doi: 10.1007/978-3-319-90472-6_1
Ezekwe, M. O., Omara-Alwala, T. R., and Membrahtu, T. (1999). Nutritive characterization of purslane accessions as influenced by planting date. Plant Foods Hum. Nutr. 54, 183–191. doi: 10.1023/A:1008101620382
Falkenmark, M. (2013). Growing water scarcity in agriculture: future challenge to global water security. Philos. Trans. R. Soc. Math. Phys. Eng. Sci. 371:20120410. doi: 10.1098/rsta.2012.0410
FAO (1988). Traditional food plants: a resource book for promoting the exploitation and consumption of food plants in arid, semi-arid and sub-humid lands in Eastern Africa. Rome: FAO. Food and nutrition paper 42.
FAO (2011). The State of the World's Land and Water Resources for Food and Agriculture (SOLAW)—Managing Systems at Risk. London: Food and Agriculture Organization of the United Nations, Rome and Earthscan.
Fazal, S. (2000). Urban expansion and loss of agricultural land—a GIS based study of Saharanpur City, India. Environ. Urban. 12, 133–149. doi: 10.1177/095624780001200211
Fernández, J. A., Navarro, A., Vicente, M. J., Peñapareja, D., and Plana, V. (2008). Effect of seed germination methods on seedling emergence and earliness of purslane (Portulaca oleracea L.) cultivars in a hydroponic floating system. Acta Hortic. 782, 207–212. doi: 10.17660/ActaHortic.2008.782.24
Fernández-Poyatos, M. P., Llorent-Martínez, E. J., and Ruiz-Medina, A. (2021). Phytochemical composition and antioxidant activity of Portulaca oleracea: influence of the steaming cooking process. Foods 10:94. doi: 10.3390/foods10010094
Ferrari, R. C., Cruz, B. C., Gastaldi, V. D., Storl, T., Ferrari, E. C., Boxall, S. F., et al. (2020). Exploring C4–CAM plasticity within the Portulaca oleracea complex. Sci. Rep. 10:14237. doi: 10.1038/s41598-020-71012-y
Fischel, W. A. (1982). The urbanization of agricultural land: a review of the national agricultural lands study. Land Econ. 58, 236–259. doi: 10.2307/3145899
Fita, A., Rodríguez-Burruezo, A., Boscaiu, M., Prohens, J., and Vicente, O. (2015). Breeding and domesticating crops adapted to drought and salinity: A new paradigm for increasing food production. Front. Plant Sci. 6:978. doi: 10.3389/fpls.2015.00978
Flowers, T. J. (2004). Improving crop salt tolerance. J. Exp. Bot. 55, 307–319. doi: 10.1093/jxb/erh003
Franco, J. A., Cros, V., Vicente, M. J., and Martínez-Sánchez, J. J. (2011). Effects of salinity on the germination, growth, and nitrate contents of purslane (Portulaca oleracea L.) cultivated under different climatic conditions. J. Hortic. Sci. Biotechnol. 86, 1–6. doi: 10.1080/14620316.2011.11512716
Geesink, R. (1969). An account of the genus Portulaca in Indo-Australia and the Pacific (Portulacaceae). Blumea Biodivers. Evol. Biogeogr. Plants 17:275–301.
Grubben, G. J. H., (ed.). (2004). Plant Resources of Tropical Africa 2. Vegetables. Wageningen, Netherlands: PROTA Foundation/Backhuys Publishers.
Guil-Guerrero, J. L., and Rodríguez-García, I. (1999). Lipids classes, fatty acids and carotenes of the leaves of six edible wild plants. Eur. Food Res. Technol. 209, 313–316. doi: 10.1007/s002170050501
Guralnick, L. J., Edwards, G., Ku, M. S. B., Hockema, B., and Franceschi, V. R. (2002). Photosynthetic and anatomical characteristics in the C4-crassulacean acid metabolism-cycling plant, Portulaca grandiflora. Funct. Plant Biol. 29, 763–773. doi: 10.1071/PP01176
Hasanuzzaman, M., Nahar, K., Alam, M. M., Bhowmik, P. C., Hossain, M. A., Rahman, M. M., et al. (2014). Potential use of halophytes to remediate saline soils. BioMed Res. Int. 2014, 589341. doi: 10.1155/2014/589341
Hniličková, H., Hnilička, F., Orsák, M., and Hejnák, V. (2019). Effect of salt stress on growth, electrolyte leakage, Na+ and K+ content in selected plant species. Plant Soil Environ. 65, 90–96. doi: 10.17221/620/2018-PSE
Hosseinzadeh, M. H., Ghalavand, A., Boojar, M. M.-A., Modarres-Sanavy, S. A. M., and Mokhtassi-Bidgoli, A. (2021). Application of manure and biofertilizer to improve soil properties and increase grain yield, essential oil and ω3 of purslane (Portulaca oleracea L.) under drought stress. Soil Tillage Res. 205, 104633. doi: 10.1016/j.still.2020.104633
Hosseinzadeh, M. H., Ghalavand, A., Mashhadi-Akbar-Boojar, M., Modarres-Sanavy, S. A. M., and Mokhtassi-Bidgoli, A. (2020). Increased medicinal contents of purslane by nitrogen and arbuscular mycorrhiza under drought stress. Commun. Soil Sci. Plant Anal. 51, 118–135. doi: 10.1080/00103624.2019.1695828
Hwess, H., Ayadi, R., Mahouachi, W., Rezgui, M., Balti, H., and Hamrouni, L. (2018). Ethnobotanical and ethnopharmacological notes on Portulaca oleracea (L.). Phytotherapie 16, S215–S219. doi: 10.3166/phyto-2019-0151
Ichimura, K., and Suto, K. (1998). Environmental factors controlling flower opening and closing in a Portulaca hybrid. Ann. Bot. 82, 67–70. doi: 10.1006/anbo.1998.0642
Iranshahy, M., Javadi, B., Iranshahi, M., Jahanbakhsh, S. P., Mahyari, S., Hassani, F. V., et al. (2017). A review of traditional uses, phytochemistry and pharmacology of Portulaca oleracea L. J. Ethnopharmacol. 205, 158–172. doi: 10.1016/j.jep.2017.05.004
Irawan, D., Hariyadi, P., and Wijaya, C. (2003). The potency of Krokot (Portulaca oleracea) as functional food ingredients. Indones. Food Nutr. Prog. 10, 1–12. doi: 10.22146/jifnp.89
Ismail, B. H. (2013). Edible wild vegetables used in North West of Tunisia. Paripex Indian J. Res. 9, 219–221.
Jaganathan, D., Ramasamy, K., Sellamuthu, G., Jayabalan, S., and Venkataraman, G. (2018). CRISPR for crop improvement: an update review. Front. Plant Sci. 9:985. doi: 10.3389/fpls.2018.00985
Jewell, M. C., Campbell, B. C., and Godwin, I. D. (2010). Transgenic Plants for Abiotic Stress Resistance, in Transgenic Crop Plants, eds Kole, C., Michler, C. H., Abbott, A. G., and Hall, T. C., (Berlin, Heidelberg: Springer), 67–132. doi: 10.1007/978-3-642-04812-8_2
Jin, H., Chen, L., Wang, S., and Chao, D. (2017). Portulaca oleracea extract can inhibit nodule formation of colon cancer stem cells by regulating gene expression of the notch signal transduction pathway. Tumor Biol. 39:1010428317708699. doi: 10.1177/1010428317708699
Jin, R., Shi, H., Han, C., Zhong, B., Wang, Q., and Chan, Z. (2015). Physiological changes of purslane (Portulaca oleracea L.) after progressive drought stress and rehydration. Sci. Hortic. 194, 215–221. doi: 10.1016/j.scienta.2015.08.023
Jin, R., Wang, Y., Liu, R., Gou, J., and Chan, Z. (2016). Physiological and metabolic changes of purslane (Portulaca oleracea L.) in response to drought, heat, and combined stresses. Front. Plant Sci. 6:1123. doi: 10.3389/fpls.2015.01123
Kafi, M., and Rahimi, Z. (2011). Effect of salinity and silicon on root characteristics, growth, water status, proline content and ion accumulation of purslane (Portulaca oleracea L.). Soil Sci. Plant Nutr. 57, 341–347. doi: 10.1080/00380768.2011.567398
Kamil, M., Chan, K., and Habibullah, M. (2000). A review on Portulaca species—with special reference to Potulaca oleracea. Aust. J. Med. Herbal. 12, 43–51.
Kapil, S. (2019). Urbanisation to cause huge loss of prime farmland: UNCCD. DownToEarth. Available online at: https://www.downtoearth.org.in/news/agriculture/urbanisation-to-cause-huge-loss-of-prime-farmland-unccd-66562 (accessed May 7, 2021).
Kapoor, A., Kanwar, P., and Gupta, R. (2010). Traditional recipes of district Kangra of Himachal Pradesh. Indian J. Tradit. Knowl. 9, 282–288.
Karkanis, A. C., and Petropoulos, S. A. (2017). Physiological and growth responses of several genotypes of common purslane (Portulaca oleracea L.) under mediterranean semi-arid conditions. Not. Bot. Horti Agrobot. Cluj-Napoca. 45, 569–575. doi: 10.15835/nbha45210903
Kaşkar, Ç., Fernándeza, J. A., Ochoa, J., Niñirola, D., Conesa, E., and Tüzel, Y. (2009). Agronomic behaviour and oxalate and nitrate content of different purslane cultivars (Portulaca oleracea) grown in a hydroponic floating system. Acta Hortic. 807, 521–526 doi: 10.17660/ActaHortic.2009.807.76
Kichenaradjou, M., Jawaharlal, M., Arulmozhiyan, R., Vijayaraghavan, H., and Muralidharan, D. B. (2018). Evaluation of ornamental groundcovers on physiological and quality parameters in salt affected soil condition. J. Pharmacogn. Phytochem. 7, 553–557.
Koch, K., and Kennedy, R. A. (1980). Characteristics of Crassulacean Acid Metabolism in the succulent C4 dicot, Portulaca oleracea L. Plant Physiol. 65, 193–197. doi: 10.1104/pp.65.2.193
Koch, K. E., and Kennedy, R. A. (1982). Crassulacean acid metabolism in the succulent C4 Dicot, Portulaca oleracea L under natural environmental conditions 1. Plant Physiol. 69, 757–761. doi: 10.1104/pp.69.4.757
Kuhnlein, H. V., and Receveur, O. (1996). Dietary change and traditional food systems of indigenous peoples. Annu. Rev. Nutr. 16, 417–442. doi: 10.1146/annurev.nu.16.070196.002221
Kumar, A., Kumar, S., Thomas, T. D., Ramchiary, N., Swamy, M. K., and Ahmad, I. (2019). Linking Omics Approaches to Medicinal Plants and Human Health, in Natural Bio-active Compounds: Volume 3: Biotechnology, Bioengineering, and Molecular Approaches, eds. Akhtar, M. S., and Swamy, M. K., (Singapore: Springer), 31–57. doi: 10.1007/978-981-13-7438-8_2
Kumari, A., Das, P., Parida, A. K., and Agarwal, P. K. (2015). Proteomics, metabolomics, and ionomics perspectives of salinity tolerance in halophytes. Front. Plant Sci. 6:537. doi: 10.3389/fpls.2015.00537
Lara, M. V., Disante, K. B., Podestá, F. E., Andreo, C. S., and Drincovich, M. F. (2003). Induction of a Crassulacean acid like metabolism in the C4 succulent plant, Portulaca oleracea L.: physiological and morphological changes are accompanied by specific modifications in phosphoenolpyruvate carboxylase. Photosynth. Res. 77, 241–254. doi: 10.1023/A:1025834120499
Lara, M. V., Drincovich, M. F., and Andreo, C. S. (2004). Induction of a crassulacean acid-like metabolism in the C4 succulent plant, Portulaca oleracea L.: study of enzymes involved in carbon fixation and carbohydrate metabolism. Plant Cell Physiol. 45, 618–626. doi: 10.1093/pcp/pch073
Legwaila, G. M., Mojeremane, W., Madisa, M., Mmolotsi, R., and Rampart, M. (2011). Potential of traditional food plants in rural household food security in Botswana. J. Hortic. For. 3, 171–177.
Li, Y.-H., Lai, C.-Y., Su, M.-C., Cheng, J.-C., and Chang, Y.-S. (2019). Antiviral activity of Portulaca oleracea L. against influenza A viruses. J. Ethnopharmacol. 241. doi: 10.1016/j.jep.2019.112013
Lim, Y. Y., and Quah, E. P. L. (2007). Antioxidant properties of different cultivars of Portulaca oleracea. Food Chem. 103, 734–740. doi: 10.1016/j.foodchem.2006.09.025
Liu, D., Palla, K. J., Hu, R., Moseley, R. C., Mendoza, C., Chen, M., et al. (2018). Perspectives on the basic and applied aspects of crassulacean acid metabolism (CAM) research. Plant Sci. 274, 394–401. doi: 10.1016/j.plantsci.2018.06.012
Liu, L., Howe, P., Zhou, Y.-F., Xu, Z.-Q., Hocart, C., and Zhang, R. (2000). Fatty acids and β-carotene in Australian purslane (Portulaca oleracea) varieties. J. Chromatogr. A 893, 207–213. doi: 10.1016/S0021-9673(00)00747-0
Magwede, K., van Wyk, B.-E., and van Wyk, A. E. (2019). An inventory of Vhavena useful plants. Ethnobotany 122, 57–89. doi: 10.1016/j.sajb.2017.12.013
Mahomoodally, M. F., Subratty, A. H., Gurib-Fakim, A., Choudhary, M. I., and Nahar Khan, S. (2012). Traditional medicinal herbs and food plants have the potential to inhibit key carbohydrate hydrolyzing enzymes in vitro and reduce postprandial blood glucose peaks in vivo. Sci. World J. 2012:285284. doi: 10.1100/2012/285284
Manzanero-Medina, G. I., Vásquez-Dávila, M. A., Lustre-Sánchez, H., and Pérez-Herrera, A. (2020). Ethnobotany of food plants (quelites) sold in two traditional markets of Oaxaca, Mexico. South Afr. J. Bot. 130, 215–223. doi: 10.1016/j.sajb.2020.01.002
Matthews, J. F., and Levins, P. A. (1985). The genus Portulaca in the Southeastern United States. Castanea 50, 96–104.
Melilli, M. G., Pagliaro, A., Bognanni, R., Scandurra, S., and Di Stefano, V. (2020). Antioxidant activity and fatty acids quantification in Sicilian purslane germplasm. Nat. Prod. Res. 34, 26–33. doi: 10.1080/14786419.2018.1560291
Miller, T. E., Soho Wing, J., and Huete, A. R. (1984). The agricultural potential of selected C4 plants in arid environments. J. Arid Environ. 7, 275–286.
Mishra, V., Chugh, V., Dwivedi, S., and Sharma, K. (2020). Food and nutraceuticals value of purslane (Portulaca oleracea L.): an overview. Pharma Innov. 9, 419–424.
Misra, A. K. (2014). Climate change and challenges of water and food security. Int. J. Sustain. Built Environ. 3, 153–165. doi: 10.1016/j.ijsbe.2014.04.006
Moghadam, Y. A., Piri, K., Bahramnejad, B., and Ghiasvand, T. (2014). Dopamine production in hairy root cultures of Portulaca oleracea (purslane) using Agrobacterium rhizogenes. J. Agric. Sci. Technol. 16, 409–420.
Morgan, J. (1992). Osmotic components and properties associated with genotypic differences in osmoregulation in wheat. Funct. Plant Biol. 19, 67–76. doi: 10.1071/PP9920067
Mulla, S. K., and Swamy, P. (2010). Preliminary pharmacognostical and phytochemical evaluation of Portulaca quadrifida Linn. Int. J. PharmTech Res. 2, 1699–1702.
Muthoni, J., and Nyamongo, D. O. (2010). Traditional food crops and their role in food and nutritional security in Kenya. J. Agric. Food Inf. 11, 36–50. doi: 10.1080/10496500903466745
Nanagulyan, S., Zakaryan, N., Kartashyan, N., Piwowarczyk, R., and Łuczaj, Ł. (2020). Wild plants and fungi sold in the markets of Yerevan (Armenia). J. Ethnobiol. Ethnomedicine 16:26. doi: 10.1186/s13002-020-00375-3
Nedelcheva, A. (2013). An ethnobotanical study of wild edible plants in Bulgaria. EurAsian J. Biosci. 7, 77–94. doi: 10.5053/ejobios.2013.7.0.10
Nyffeler, R., and Eggli, U. (2010). Disintegrating portulacaceae: a new familial classification of the suborder portulacineae (Caryophyllales) based on molecular and morphological data. Taxon 59, 227–240. doi: 10.1002/tax.591021
Ocampo, G., and Columbus, J. T. (2012). Molecular phylogenetics, historical biogeography, and chromosome number evolution of Portulaca (Portulacaceae). Mol. Phylogenet. Evol. 63, 97–112. doi: 10.1016/j.ympev.2011.12.017
Ocampo, G., Koteyeva, N. K., Voznesenskaya, E. V., Edwards, G. E., Sage, T. L., Sage, R. F., et al. (2013). Evolution of leaf anatomy and photosynthetic pathways in Portulacaceae. Am. J. Bot. 100, 2388–2402. doi: 10.3732/ajb.1300094
Oliveira, I., Valentão, P., Lopes, R., Andrade, P. B., Bento, A., and Pereira, J. A. (2009). Phytochemical characterization and radical scavenging activity of Portulaca oleracea L. leaves and stems. Microchem. J. 92, 129–134. doi: 10.1016/j.microc.2009.02.006
Osma, E., Ozyigit, I., Demir, G., and Yaşar, Ü. (2014). Assesment of some heavy metals in wild type and cultivated purslane (Portulaca oleracea L.) and soils in Istanbul, Turkey. Fresenius Environ. Bull. 23, 2181–2189.
Palaniswamy, U. R., McAvoy, R. J., and Bible, B. B. (2001). Omega-3 fatty acid concentration in purslane (Portulaca oleracea) is altered by photosynthetic photon flux. J. Am. Soc. Hortic. Sci. 126, 537–543. doi: 10.21273/JASHS.126.5.537
Panta, S., Flowers, T., Lane, P., Doyle, R., Haros, G., and Shabala, S. (2014). Halophyte agriculture: success stories. Environ. Exp. Bot. 107, 71–83. doi: 10.1016/j.envexpbot.2014.05.006
Park, J. E., and Han, J. S. (2018). A Portulaca oleracea L. extract promotes insulin secretion via a K+ATP channel dependent pathway in INS-1 pancreatic β-cells. Nutr. Res. Pract. 12, 183–190. doi: 10.4162/nrp.2018.12.3.183
Park, J. E., Seo, Y., and Han, J. S. (2021). HM-chromanone, a component of Portulaca oleracea L., stimulates glucose uptake and glycogen synthesis in skeletal muscle cell. Phytomedicine 83:153473. doi: 10.1016/j.phymed.2021.153473
Patil, A. G., Joshi, V. S., Koli, S. P., and Patil, D. A. (2012). Pharmacognistical and phytochemical analysis of Portulaca quadrifida Linn. Res. J. Pharm. Biol. Chem. Sci. 3, 90–100.
Pawera, L., Khomsan, A., Zuhud, E. A. M., Hunter, D., Ickowitz, A., and Polesny, Z. (2020). Wild food plants and trends in their use: from knowledge and perceptions to drivers of change in west Sumatra, Indonesia. Foods 9:1240. doi: 10.3390/foods9091240
Petropoulos, S., Karkanis, A., Martins, N., and Ferreira, I. C. F. R. (2016). Phytochemical composition and bioactive compounds of common purslane (Portulaca oleracea L.) as affected by crop management practices. Trends Food Sci. Technol. 55, 1–10. doi: 10.1016/j.tifs.2016.06.010
Pieroni, A., Dibra, B., Grishaj, G., Grishaj, I., and Maçai, S. G. (2005). Traditional phytotherapy of the Albanians of Lepushe, Northern Albanian Alps. Fitoterapia 76, 379–399. doi: 10.1016/j.fitote.2005.03.015
Pieroni, A., Hovsepyan, R., Manduzai, A. K., and Sõukand, R. (2021). Wild food plants traditionally gathered in central Armenia: archaic ingredients or future sustainable foods? Environ. Dev. Sustain. 23, 2358–2381. doi: 10.1007/s10668-020-00678-1
Rahdari, P., Tavakoli, S., and Hosseini, S. (2012). Studying of salinity stress effect on germination, proline, sugar, protein, lipid and chlorophyll content in purslane (Portulaca oleracea L.) leaves. J. Stress Physiol. Biochem. 8, 182–193.
Ramalingam, A., Kudapa, H., Pazhamala, L. T., Weckwerth, W., and Varshney, R. K. (2015). Proteomics and metabolomics: two emerging areas for legume improvement. Front. Plant Sci. 6:1116. doi: 10.3389/fpls.2015.01116
Ramanatha Rao, V., and Hodgkin, T. (2002). Genetic diversity and conservation and utilization of plant genetic resources. Plant Cell Tiss. Org. 68, 1–19. doi: 10.1023/A:1013359015812
Rapoport, E. H., and Drausal, B. S. (2013). Edible Plants, in Encyclopedia of Biodiversity (Second Edition), ed. Levin, S. A., (Waltham: Academic Press), 127–132. doi: 10.1016/B978-0-12-384719-5.00160-X
Ren, S., Weeda, S., Akande, O., Guo, Y., Rutto, L., and Mebrahtu, T. (2011). Drought tolerance and AFLP-based genetic diversity in purslane (Portulaca oleracea L.). J. Biotech Res. 3, 51–61.
Ren, S., and White, L. (2019). Genetic variation of copper stress tolerance and shoot copper accumulation in purslane (Portulaca oleracea). J. Biotech Res. 10, 213–222.
Renu, R., and Waghray, K. (2016). Development of papads: a traditional savoury with purslane, Portulaca oleracea, leaves. Health Scope 5:26735. doi: 10.5812/jhealthscope.20138
Rosell, M. S., Lloyd-Wright, Z., Appleby, P. N., Sanders, T. A., Allen, N. E., and Key, T. J. (2005). Long-chain n−3 polyunsaturated fatty acids in plasma in British meat-eating, vegetarian, and vegan men. Am. J. Clin. Nutr. 82, 327–334. doi: 10.1093/ajcn/82.2.327
Ross, I. A. (2003). Portulaca oleracea, in Medicinal Plants of the World: Volume 1 Chemical Constituents, Traditional and Modern Medicinal Uses, ed. Ross, I. A., (Totowa, NJ: Humana Press), 405–414. doi: 10.1007/978-1-59259-365-1_23
Rossi-Hassani, B. D., Bennani, F., and Zryd, J. P. (1995). Agrobacterium-mediated transformation of large-flowered purslane (Portulaca grandiflora H.). Genome 38, 752–756. doi: 10.1139/g95-095
Roy, S. J., Negrão, S., and Tester, M. (2014). Salt resistant crop plants. Curr. Opin. Biotechnol. 26, 115–124. doi: 10.1016/j.copbio.2013.12.004
Safdari, Y., and Kazemitabar, S. K. (2009). Plant tissue culture study on two different races of purslane (Portulaca oleracea L.). Afr. J. Biotechnol. 8, 5906–5912. doi: 10.5897/AJB09.816
Sage, R. F. (2002). Are crassulacean acid metabolism and C4 photosynthesis incompatible? Funct. Plant Biol. 29, 775–785. doi: 10.1071/PP01217
Salah, K. B. H., and Chemli, R. (2004). Variabilité phénotypique de quelques populations de Pourpier (Portulaca oleracea L.) en Tunisie. Acta Bot. Gallica 151, 111–119. doi: 10.1080/12538078.2004.10516024
Satterthwaite, D., McGranahan, G., and Tacoli, C. (2010). Urbanization and its implications for food and farming. Philos. Trans. R. Soc. Lond. B. Biol. Sci. 365, 2809–2820. doi: 10.1098/rstb.2010.0136
Saunders, A. V., Davis, B. C., and Garg, M. L. (2013). Omega-3 polyunsaturated fatty acids and vegetarian diets. Med. J. Aust. 199, S22–S26. doi: 10.5694/mja11.11507
Sdouga, D., Ben Amor, F., Ghribi, S., Kabtni, S., Tebini, M., Branca, F., et al. (2019). An insight from tolerance to salinity stress in halophyte Portulaca oleracea L.: physio-morphological, biochemical and molecular responses. Ecotoxicol. Environ. Saf. 172, 45–52. doi: 10.1016/j.ecoenv.2018.12.082
Sdouga, D., Branca, F., Kabtni, S., Di Bella, M. C., Trifi-Farah, N., and Marghali, S. (2020). Morphological traits and phenolic compounds in tunisian wild populations and cultivated varieties of Portulaca oleracea L. Agronomy 10, 948. doi: 10.3390/agronomy10070948
Sedaghati, B., Haddad, R., and Bandehpour, M. (2019). Efficient plant regeneration and Agrobacterium-mediated transformation via somatic embryogenesis in purslane (Portulaca oleracea L.): an important medicinal plant. Plant Cell Tiss. Org. 136, 231–245. doi: 10.1007/s11240-018-1509-3
Sedaghati, B., Haddad, R., and Bandehpour, M. (2021). Development of an efficient in-planta Agrobacterium-mediated transformation method for Iranian purslane (Portulaca oleracea L.) using sonication and vacuum infiltration. Acta Physiol. Plant. 43, 17. doi: 10.1007/s11738-020-03185-y
Sharma, M. M., Singh, A., Verma, R. N., Ali, D. Z., and Batra, A. (2011). Influence of PGRS for the in vitro plant regeneration and flowering in Portulaca oleracea (L.): a medicinal and ornamental plant. Int. J. Bot. 7, 103–107. doi: 10.3923/ijb.2011.103.107
Shekhawat, M., Narasingam, K., and Manokari, M. (2015). Propagation of Portulaca oleracea L. in liquid medium: implications of plant growth regulators in culture. J. Microbiol. Biotechnol. Food Sci. 4, 332–335. doi: 10.15414/jmbfs.2015.4.4.332-335
Shikov, A. N., Tsitsilin, A. N., Pozharitskaya, O. N., Makarov, V. G., and Heinrich, M. (2017). Traditional and current food use of wild plants listed in the Russian pharmacopoeia. Front. Pharmacol. 8:841. doi: 10.3389/fphar.2017.00841
Shrivastava, P., and Kumar, R. (2015). Soil salinity: A serious environmental issue and plant growth promoting bacteria as one of the tools for its alleviation. Saudi J. Biol. Sci. 22, 123–131. doi: 10.1016/j.sjbs.2014.12.001
Simopoulos, A. P. (2011). Healthy Agriculture, Healthy Nutrition, Healthy People. Basel: Karger. doi: 10.1159/isbn.978-3-8055-9780-7
Simopoulos, A. P., Norman, H. A., and Gillaspy, J. E. (1995). Purslane in human nutrition and its potential for world agriculture. World Rev. Nutr. Diet. 77, 47–74. doi: 10.1159/000424465
Simopoulos, A. P., Norman, H. A., Gillaspy, J. E., and Duke, J. A. (1992). Common purslane: a source of omega-3 fatty acids and antioxidants. J. Am. Coll. Nutr. 11, 374–382. doi: 10.1080/07315724.1992.10718240
Simopoulos, A. P., Tan, D.-X., Manchester, L. C., and Reiter, R. J. (2005). Purslane: a plant source of omega-3 fatty acids and melatonin. J. Pineal Res. 39, 331–332. doi: 10.1111/j.1600-079X.2005.00269.x
Singh, R. K., Prasad, A., Muthamilarasan, M., Parida, S. K., and Prasad, M. (2020). Breeding and biotechnological interventions for trait improvement: status and prospects. Planta 252:54. doi: 10.1007/s00425-020-03465-4
Singh, S., Singh, D. R., Salim, K. M., Srivastava, A., Singh, L. B., and Srivastava, R. C. (2011). Estimation of proximate composition, micronutrients and phytochemical compounds in traditional vegetables from Andaman and Nicobar Islands. Int. J. Food Sci. Nutr. 62, 765–773. doi: 10.3109/09637486.2011.585961
Smith, N. (1991). Ethnobotanical field notes from the Northern Territory, Australia. J. Adel. Bot. Gard. 14, 1–65.
Sultana, A., and Raheman, K. (2013). Portulaca oleracea Linn: a global panacea with ethnomedicinal and pharmacological potential. Int. J. Pharm. Pharm. Sci. 5, 33–39.
Tarkergari, S., Waghray, K., and Gulla, S. (2013). Acceptability studies of value added products with purslane (Portulaca oleracea). Pak. J. Nutr. 12, 93–96. doi: 10.3923/pjn.2013.93.96
Teixeira, M., and Carvalho, I. S. (2009). Effects of salt stress on purslane (Portulaca oleracea) nutrition. Ann. Appl. Biol. 154, 77–86. doi: 10.1111/j.1744-7348.2008.00272.x
Teixeira, M. C., Carvalho, I. S., and Brodelius, M. (2010). ω-3 Fatty acid desaturase genes isolated from purslane (Portulaca oleracea L.): expression in different tissues and response to cold and wound stress. J. Agric. Food Chem. 58, 1870–1877. doi: 10.1021/jf902684v
Teixeira, M. C., Coelho, N., Olsson, M. E., Brodelius, P. E., Carvalho, I. S., and Brodelius, M. (2009). Molecular cloning and expression analysis of three omega-6 desaturase genes from purslane (Portulaca oleracea L.). Biotechnol. Lett. 31, 1089–1101. doi: 10.1007/s10529-009-9956-x
The Angiosperm Phylogeny Group (2009). An update of The Angiosperm Phylogeny Group classification for the orders and families of flowering plants: APG III. Bot. J. Linn. Soc. 161, 105–121. doi: 10.1111/j.1095-8339.2009.00996.x
Tiwari, K. K., Dwivedi, S., Mishra, S., Srivastava, S., Tripathi, R. D., Singh, N. K., et al. (2008). Phytoremediation efficiency of Portulaca tuberosa Rox and Portulaca oleracea L. naturally growing in an industrial effluent irrigated area in Vadodra, Gujrat, India. Environ. Monit. Assess. 147, 15–22. doi: 10.1007/s10661-007-0093-5
Tleubayeva, M. I., Datkhayev, U. M., Alimzhanova, M., Ishmuratova, M. Y., Korotetskaya, N. V., Abdullabekova, R. M., et al. (2021). Component composition and antimicrobial activity of CO2 extract of Portulaca oleracea, growing in the territory of Kazakhstan. Sci. World J. 2021:5434525. doi: 10.1155/2021/5434525
Trichopoulou, A., Soukara, S., and Vasilopoulou, E. (2007). Traditional foods: a science and society perspective. Trends Food Sci. Technol. 18, 420–427. doi: 10.1016/j.tifs.2007.03.007
Turner, N. J., Łuczaj, Ł. J., Migliorini, P., Pieroni, A., Dreon, A. L., Sacchetti, L. E., et al. (2011). Edible and tended wild plants, traditional ecological knowledge and agroecology. Crit. Rev. Plant Sci. 30, 198–225. doi: 10.1080/07352689.2011.554492
Uddin, M. K., Juraimi, A. S., Hossain, M. S., Nahar, M. A. U., Ali, M. E., and Rahman, M. M. (2014). Purslane weed (Portulaca oleracea): a prospective plant source of nutrition, omega-3 fatty acid, and antioxidant attributes. Sci. World J. 2014:951019. doi: 10.1155/2014/951019
Vandenbroucke, K., and Metzlaff, M. (2013). Abiotic Stress Tolerant Crops: Genes, Pathways and Bottlenecks, in Sustainable Food Production, eds. Christou, P., Savin, R., Costa-Pierce, B. A., Misztal, I., and Whitelaw, C. B. A., (New York, NY: Springer), 1–17. doi: 10.1007/978-1-4614-5797-8_415
Verma, S. C., Hazra, K., Devgan, M., Ramaiah, M., and Sarkar, B. K. (2016). Phytochemical and high-performance liquid chromatography analysis of extract of Portulaca quadrifida Linn. Asian J. Pharm. Clin. Res. 9, 163–167.
Voznesenskaya, E. V., Koteyeva, N. K., Edwards, G. E., and Ocampo, G. (2010). Revealing diversity in structural and biochemical forms of C4 photosynthesis and a C3-C4 intermediate in genus Portulaca L. (Portulacaceae). J. Exp. Bot. 61, 3647–3662. doi: 10.1093/jxb/erq178
Walters, M., Figueiredo, E., Crouch, N., Winter, P., Smith, G., Zimmermann, H., et al. (2011). Naturalised and Invasive Succulents of Southern Africa. Belgium: ABC Taxa.
Welcome, A. K., and Van Wyk, B.-E. (2019). An inventory and analysis of the food plants of southern Africa. Ethnobotany 122, 136–179. doi: 10.1016/j.sajb.2018.11.003
Welkie, G. W., and Caldwell, M. (1970). Leaf anatomy of species in some dicotyledon families as related to the C3 and C4 pathways of carbon fixation. Can. J. Bot. 48, 2135–2146. doi: 10.1139/b70-309
Winter, K., and Holtum, J. A. M. (2014). Facultative crassulacean acid metabolism (CAM) plants: Powerful tools for unravelling the functional elements of CAM photosynthesis. J. Exp. Bot. 65, 3425–3441. doi: 10.1093/jxb/eru063
Xiang, L., Xing, D., Wang, W., Wang, R., Ding, Y., and Du, L. (2005). Alkaloids from Portulaca oleracea L. Phytochemistry 66, 2595–2601. doi: 10.1016/j.phytochem.2005.08.011
Xing, J. C., Zhao, B. Q., Dong, J., Liu, C., Wen, Z. G., Zhu, X. M., et al. (2020). Transcriptome and metabolome profiles revealed molecular mechanisms underlying tolerance of Portulaca oleracea to saline stress. Russ. J. Plant Physiol. 67, 146–152. doi: 10.1134/S1021443720010240
Xu, Y., Liang, D., Wang, G.-T., Wen, J., and Wang, R.-J. (2020). Nutritional and functional properties of wild food-medicine plants from the coastal region of South China. J. Evid.-Based Integr. Med. 25, 2515690X20913267–2515690X20913267. doi: 10.1177/2515690X20913267
Yamaguchi, T., and Blumwald, E. (2005). Developing salt-tolerant crop plants: challenges and opportunities. Trends Plant Sci. 10, 615–620. doi: 10.1016/j.tplants.2005.10.002
Yang, Y., Chen, J., Liu, Q., Ben, C., Todd, C. D., Shi, J., et al. (2012). Comparative proteomic analysis of the thermotolerant plant Portulaca oleracea acclimation to combined high temperature and humidity stress. J. Proteome Res. 11, 3605–3623. doi: 10.1021/pr300027a
Yazici, I., Turkan, I., Sekmen, A., and Demiral, T. (2007). Salinity tolerance of purslane (Portulaca oleracea L.) is achieved by enhanced antioxidative system, lower level of lipid peroxidation and proline accumulation. Environ. Exp. Bot. 61, 49–57. doi: 10.1016/j.envexpbot.2007.02.010
Ye, Q., Zhang, N., Chen, K., Zhu, J., and Jiang, H. (2015). Effects of portulacerebroside a on apoptosis of human leukemia HL60 cells and p38/JNK signaling pathway. Int. J. Clin. Exp. Pathol. 8, 13968–13977.
Yun, J., Li, M., Li, Q., Yang, X., Wang, X., and Lu, C. (2016). Growth and nutritional quality comparison between two common purslanes, Portulaca granulatostellulata and P. edulis. J. Food Nutr. Sci. 4:145. doi: 10.11648/j.jfns.20160406.12
Zaidi, S. S.-A., Mahas, A., Vanderschuren, H., and Mahfouz, M. M. (2020). Engineering crops of the future: CRISPR approaches to develop climate-resilient and disease-resistant plants. Genome Biol. 21, 289. doi: 10.1186/s13059-020-02204-y
Zaman, S., Bilal, M., Du, H., and Che, S. (2020). Morphophysiological and comparative metabolic profiling of purslane genotypes (Portulaca oleracea L.) under salt stress. BioMed Res. Int. 2020, 4827045. doi: 10.1155/2020/4827045
Zhang, H., Li, Y., and Zhu, J.-K. (2018). Developing naturally stress-resistant crops for sustainable agriculture. Nat. Plants 4, 989–996. doi: 10.1038/s41477-018-0309-4
Zhang, J., Natalya, Y., Klueva, W., ang, Z., Wu, R., Ho, T.-H. D., and Nguyen, H. T. (2000). Genetic engineering for abiotic stress resistance in crop plants. In Vitro Cell. Dev. Biol. Plant 36, 108–114. doi: 10.1007/s11627-000-0022-6
Zhao, C., Ying, Z., Tao, X., Jiang, M., Ying, X., and Yang, G. (2018). A new lactam alkaloid from Portulaca oleracea L. and its cytotoxity. Nat. Prod. Res. 32, 1548–1553. doi: 10.1080/14786419.2017.1385022
Zhou, Y.-X., Xin, H.-L., Rahman, K., Wang, S.-J., Peng, C., and Zhang, H. (2015). Portulaca oleracea L.: a review of phytochemistry and pharmacological effects. BioMed Res. Int. 2015:925631. doi: 10.1155/2015/925631
Keywords: purslane, sustainable food systems, traditional foods, food security, climate change, climate smart agriculture, biosaline agriculture, ethnic foods
Citation: Kumar A, Sreedharan S, Singh P, Achigan-Dako EG and Ramchiary N (2021) Improvement of a Traditional Orphan Food Crop, Portulaca oleracea L. (Purslane) Using Genomics for Sustainable Food Security and Climate-Resilient Agriculture. Front. Sustain. Food Syst. 5:711820. doi: 10.3389/fsufs.2021.711820
Received: 19 May 2021; Accepted: 13 July 2021;
Published: 12 August 2021.
Edited by:
Matteo Balderacchi, Independent Researcher, Piacenza, ItalyReviewed by:
Maria Grazia Melilli, National Research Council (CNR), ItalyMd. Amirul Alam, Universiti Malaysia Sabah, Malaysia
Copyright © 2021 Kumar, Sreedharan, Singh, Achigan-Dako and Ramchiary. This is an open-access article distributed under the terms of the Creative Commons Attribution License (CC BY). The use, distribution or reproduction in other forums is permitted, provided the original author(s) and the copyright owner(s) are credited and that the original publication in this journal is cited, in accordance with accepted academic practice. No use, distribution or reproduction is permitted which does not comply with these terms.
*Correspondence: Ajay Kumar, botanistajay@gmail.com; Enoch G. Achigan-Dako, e.adako@gmail.com; Nirala Ramchiary, nrudsc@gmail.com
†These authors have contributed equally to this work