- 1Laboratory of Soil Microbiology, Faculty of Agricultural and Veterinary Sciences, Department of Agricultural Production Sciences, São Paulo State University, Jaboticabal, Brazil
- 2Phytopathology, Microbial and Molecular Farming Lab, Centre D'Etudes et Recherche Appliquée-Haute Ecole Provinciale du Hainaut Condorcet, Ath, Belgium
This study aimed to verify the potential of three Aspergillus and Bacillus species as growth promoters in cotton plants under greenhouse conditions. The experiment was conducted with a completely randomized design with seven treatments (six microorganisms plus one control) and five replicates until the flowering stage at 70 days after emergence. The inoculation of cotton plants with Bacillus velezensis (Bv188) and Bacillus subtilis (Bs248 and Bs290) had a positive effect on total nitrogen extraction (899.31, 962.18, and 755.41 mg N/kg dry matter, respectively) compared to the control (459.31 mg N/kg dry weight), total phosphorus extraction (121.94, 124.31, and 99.27 mg P/kg dry matter, respectively) compared to the control (65.10 mg P/kg dry matter), and total dry matter (41.08, 43.59, and 49.86 g/plant, respectively) compared to the control (26.70 g/plant), as well as biomass carbon (72.26, 35.18, and 14.7 mg/kg soil, respectively). Cotton plants inoculated with Aspergillus brasiliensis (F111), Aspergillus sydowii (F112), and Aspergillus sp. (versicolor section) (F113) had higher total nitrogen extraction (953.33, 812.59, and 891.62 mg N/kg dry matter, respectively) compared to the control (459.31 mg N/kg dry matter), a higher total phosphorus (122.30, 104.86, and 118.45 mg P/kg dry matter, respectively) compared to the control (65.10 mg P/kg dry matter), a higher total dry matter (37.52, 37.41, and 53.02 g/plant) compared to the control (26.70 g/plant), and greater respiratory activity (14.98, 10.43, and 7.11 mg CO2/100 g soil, respectively) compared to the control (3.5 mg CO2/100 g soil). The fungi A. brasiliensis (F111) and A. sydowii (F112) promoted higher phosphorus absorption by cotton plants, which was reflected by the lower amount of nutrients in the soil (7.10 and 16.96 g P/dm3 soil) than in the control (26.91 g P/dm3 soil). The results suggest that B. subtilis 248 promoted an increase in phosphorus extracted from the roots and total and phosphorous compounds from the root dry matter and increased the value of soil respiratory activity, and this bacterium could be used as an inoculant in cotton crops.
Introduction
Cotton (Gossypium spp.) is a crop of economic importance for Brazil because the country reached production of 2.49 million tons in the 2020/2021 harvest (CONAB, 2021). The uses of cotton extend far beyond fiber, although cotton fiber is the main source for the textile industry. When the boll is opened, the seed of cotton can be used as raw material in the oil industry for human consumption or animal feed (Constable and Bange, 2015; Lima et al., 2016).
Cotton crops have high production costs due to the high demand for mineral fertilizers and pesticides (Khan et al., 2017). Nitrogen is needed in greater quantities than other nutrients in cotton production systems (Hou et al., 2007), and its excessive use causes economic losses and environmental risks. Global estimates indicate that between 85 and 90 million metric tons of nitrogen fertilizer are applied to soil every year, and 40–70% is lost by leaching, with only a small part used by plants; thus, additional nitrogen fertilizer is applied to the soil (Good et al., 2004; Wu and Liu, 2008). Another nutrient that plays an important role in plants is phosphorus, which forms biological molecules; however, its low availability and high fixation in the soil are problems that lead to deficiencies; thus, phosphorus is a limiting factor for plant growth (Anand et al., 2016). In view of the above, the nutritional requirements for cotton production can be addressed by using plant growth-promoting microorganisms (Diaz et al., 2019).
Various microorganisms, such as bacteria, actinobacteria, and fungi, can promote plant growth by fixing atmospheric nitrogen, solubilizing nutrients (not available in special soil types), and increasing nutrient absorption (Spaink, 2000; Harrison, 2005). These microorganisms have the ability to synthesize hormones, including indole acetic acid (IAA), cytokinins, auxins, and gibberellins, which are essential to promote plant growth (van Loon, 2007; Contreras-Cornejo et al., 2009). In addition, they can increase plant growth through the synthesis of secondary metabolites, volatile compounds, and enzymes and increase photosynthesis (Zhang et al., 2008; Vacheron et al., 2013). These microorganisms can also improve plant health and trigger resistance to pathogens and herbivorous insects, thereby inducing systemic defense responses (Van Wees et al., 2008; Segarra et al., 2009; Hossain et al., 2016).
Studies have shown that the use of plant growth-promoting microorganisms as inoculants is a viable strategy in current agricultural production systems (Romero-Perdomo et al., 2017; Numan et al., 2018; Diaz et al., 2019); therefore, the search for new microorganisms must be constant to face current and future problems in cotton crops.
In this context, the aim of this study was to verify whether the microorganisms Bacillus velezensis, Bacillus subtilis, Aspergillus brasiliensis, Aspergillus sydowii, and Aspergillus sp. (versicolor section) have the ability to promote growth in cotton plants under greenhouse conditions.
Materials and Methods
Study Location
The experiment was carried out in a greenhouse in the Horticulture Sector of the “Júlio de Mesquita Filho” São Paulo State University (UNESP), Campus of Jaboticabal, São Paulo, Brazil (21°14′28″S, 48°17′23″W). According to the Köppen and Geiger classification, the local climate corresponds to a tropical climate with a dry winter season (Peel et al., 2007), an average temperature of 22°C and an average annual rainfall of approximately 1,340 mm. The predominant soil at the study site is classified as eutrophic red latosol with a clayey texture (52% clay, 23% silt, and 24% total sand) (EMBRAPA Solos, 2018).
Experimental Design and Experiment Management
The experiment was carried out in a completely randomized design with seven treatments (six microorganisms and one control without inoculation) and five replicates. The experiment was carried out until the flowering stage at 70 days after emergence (DAE). Pots with a capacity of 5 L were filled with sieved soil (particles smaller than 1 cm in diameter). Fertilization was carried out according to a soil analysis and according to nutritional recommendations for pot experiments proposed by Malavolta et al. (1989) for cotton crops. The amounts of nutrients used in the soil were as follows: nitrogen (N: 3.33 g urea/pot), phosphorus (P: 5.5 g P2O5/pot), potassium (K: 1.66 g KCl/pot), calcium (Ca: 6.25 g of Super simple SS/pot), magnesium (Mg: 0.5 g MgO/pot), sulfur (S: 3.125 g of SS/pot), zinc (Zn: 0.125 g ZnSO4/pot), boron (B: 0.025 g H3BO3/pot), molybdenum (Mo: 0.002 g molybdate/pot), copper (Cu: 0.03 g CuSO4/pot), and manganese (Mn: 0.08 g MnSO4/pot). All nutrients were mixed with sieved soil 1 week before sowing. The moisture content of the pots was maintained at approximately 70% of the field capacity with daily irrigation. Four cotton seeds (Gossypium hirsutum-IMA7501 WS) were sown per pot, and thinning was carried out 15 days after seedling emergence, maintaining one plant per pot.
Inoculum Preparation
The microorganisms used in the study belong to the collection of the Laboratory of Soil Microbiology, UNESP, Campus of Jaboticabal. These microorganisms (bacteria and fungi), B. subtilis strain Bs248 (Access Number MZ133755), B. subtilis strain Bs290 (Access Number MZ133476), B. velezensis strain Bv188 (Access Number MZ133757), A. brasiliensis strain F111 (Access Number MZ133758), A. sydowii strain F112 (Access Number MZ133759), and Aspergillus sp. versicolor section strain F113 (Access Number MZ133456), were selected because they have growth promotion characteristics, such as phosphorus solubilization, biological nitrogen fixation, and IAA production (Baron et al., 2018; Diaz et al., 2019; Milani et al., 2019).
Each bacterial isolate was multiplied in an Erlenmeyer flask containing 90 ml of sterile nutrient broth and incubated for 24 h at 28°C in a bacteriological incubator. After 24 h of incubation, absorbance readings of each isolate were performed in a spectrophotometer at 630 nm, and in parallel, 100 μl of each isolate was sown in Petri dishes (Kloepper et al., 1989) containing nutrient agar to determine the concentration and adjustment for 108 colony-forming units (CFU/ml).
For fungi, a suspension of conidia was prepared by scraping Petri dishes containing fungi grown on potato dextrose agar for 7–10 days at 25°C. Fungi were scraped with 0.1% Tween 80 in sterile distilled water. Fungi suspensions were filtered to remove excess mycelium. The concentration of each fungus was determined according to the conidia count in a Neubauer chamber and adjusted to 108 spores/ml.
Cotton seeds were individually inoculated with microorganisms (bacteria or fungi) by immersion for 8 h at 25°C (Jaber and Enkerli, 2016). Immersion was carried out in the dark under agitation on a shaking platform at 130 rpm. After the immersion period, cotton seeds were sown in pots containing sieved and fertilized soil as previously described. Cotton seedlings were inoculated every 15 days with 10 ml of suspension containing the respective microorganism at a concentration of 108 CFU or spores/ml. Inoculations were carried out by applying the inoculum to the plant base and stem using a graduated micropipette (Kasvi single-channel premium black k1–1000 PB). Treatments were as follows: Bv188 = B. velezensis strain Bv188, Bs248 = B. subtilis strain Bs248, Bs290 = B. subtilis strain Bs290, F111 = A. brasiliensis strain F111, F112 = A. sydowii strain F112, F113 = Aspergillus sp. versicolor section strain F113, and Control = No inoculation.
Biometric Plant Parameters
Shoot and Root Dry Matter
At 70 DAE, the experiment was disassembled, and the roots of the cotton plants were washed with running water to remove excess soil. After washing, the plants were separated into roots and shoots and placed in paper bags for drying in an oven with air circulation at 65°C until reaching constant weight. The weight of the root and shoot dry matter was determined using an analytical scale (Mettler Toledo model AB204).
Preparation of Soil Samples
Thirty-five soil samples were separated into two subsamples of approximately 100 g each. One subsample was sieved and dried at room temperature for chemical analysis, and the other was kept in a refrigerator for microbiological analysis. After harvest, the presence of the inoculated microorganisms was checked from each soil sample.
Phosphorus Determination
Soluble phosphorus in soil was determined using the method proposed by Watanabe and Olsen (1965). For the determination of phosphorus in plants, the phosphorus concentrations in roots and shoots were determined according to the methodology proposed by Sarruge and Haag (1974) and modified by Bezerra Neto and Barreto (2011). A sample of 0.5 g of plant tissue from the digester tube was added to 5 ml of concentrated nitric acid and 1 ml of concentrated perchloric acid. The mixture was left to rest for 1 day. Then, complete digestion was performed using a block digester. The material was washed with distilled water to obtain 50 ml of extract. A reading was performed in a spectrophotometer at 470 nm using 5 ml of extract plus 1 ml of specific reagent composed of a mixture of 5% ammonium molybdate and 0.25% ammonium vanadate.
Total Nitrogen Concentration in Plant and Soil
The nitrogen concentration in the shoots and roots was determined according to Sarruge and Haag (1974) with sulfuric digestion of the plant material to measure the nitrogen concentration associated with obtaining 90% dry matter production. The total nitrogen in the soil was determined according to the methodology proposed by Bremner (1996) and modified by Wilke (2005).
Microbial Respiratory Activity
Respiratory activity was determined by the method of quantifying the released CO2 according to Jenkinson and Powlson (1976) using wide-mouthed glass flasks, to which 100 g of soil (dry or wet) was added. Inside the flasks, two beakers were used (one containing 20 ml of NaOH and the other 20 ml of distilled water). Beakers were placed inside glass flasks that were sealed with plastic film and incubated in the dark for 7 days. The microbial respiratory activity was measured based on the amount of CO2 released from the soil samples. After incubation, the remaining NaOH was quantified by titration with HCl.
Microbial Biomass Carbon
Microbial biomass carbon was determined by the irradiation-extraction method (Islam and Weil, 1998; Mendonça and Matos, 2017) using a microwave oven with a power of 900 W (Panasonic model NN-ST 252 WRUN) and frequency of 2,450 MHz. After irradiation, samples were subjected to a 0.5-mol/L potassium sulfate extractor, and microbial biomass carbon was determined by oxidation with 0.066 mol/L potassium dichromate and titration with 0.033 mol/L ferrous ammonium sulfate (Brookes et al., 1982).
Nitrogen and Phosphorus Extraction
Nitrogen extraction from the roots was calculated by multiplying the nitrogen content in the root and root dry matter for each replicate. Nitrogen extraction from the shoots was calculated by multiplying the nitrogen content in the shoot and shoot dry matter for each replicate. Total nitrogen extraction was calculated by multiplying the sum of the nitrogen content of the roots and shoots and the sum of the root and shoot dry matter for each replicate. For the phosphorus extraction calculation, the procedure was the same as that used for nitrogen.
Presence of Microorganisms Previously Inoculated
To verify the presence of the microorganisms previously inoculated in the soil sample, 10 g of rhizospheric soil was added to 95 ml of 0.1% pyrophosphate solution (w/v) and subjected to serial dilution (Wollum, 1982) up to a concentration of 10−4. A 0.1-ml aliquot from the dilution (10−4) was transferred to Petri plates containing Bunt and Rovira medium (Bunt and Rovira, 1955), pH 7.4, for bacteria and maltose agar for fungi. Then, plates containing the bacterial and fungal inoculum were kept at 30°C for 72 h and 7 days, respectively. After this period, the colonies were visualized with the aid of a magnifying glass at 6 × magnification, and a Gram stain test was performed.
Statistical Analysis
Data were log (x + 1) transformed to avoid violating the assumption of the ANOVA. Data normality and homogeneity of variance were assessed by the Shapiro–Wilk and Levene tests (α = 0.05), respectively. The parameters under study were analyzed by the ANOVA F test (α = 0.05) to identify differences. Subsequently, multiple comparisons of averages were performed using Duncan's test (α = 0.05) to identify significant differences between treatments. Statistical analyses were performed using R software for Windows (R Core Team, 2020).
Results
Inoculations with Bv188, Bs248, Bs290, F111, F112, and F113 increased the amount of nitrogen extracted from the roots (177.14, 173.48, 166.73, 197.09, 158.71, and 193.50 mg N/kg dry matter, respectively) compared to the control (66.31 mg N/kg dry matter), from the shoots (722.27, 704.68, 588.68, 756.24, 653.88, and 698.12 mg N/kg dry matter, respectively) compared to the control (393.00 mg N/kg dry matter), and from the total (899.31, 962.18, 755.41, 953.33, 812.59, and 891.62 mg N/kg dry matter, respectively) compared to the control (459.31 mg N/kg dry matter) (Figure 1); moreover, they increased the amount of phosphorus extracted from the roots (47.17, 46.75, 40.68, 40.61, 42.22, and 42.31 mg P/kg dry matter, respectively) compared to the control (21.87 mg P/kg dry matter), from the shoots (74.78, 77.56, 58.59, 81.69, 62.64, and 76.15 mg P/kg dry matter, respectively) compared to the control (43.23 mg P/kg dry matter), and from the total (121.94, 124.31, 99.27, 122.30, 104.86, and 118.45 mg P/kg dry matter, respectively) compared to the control (65.10 mg P/kg dry matter) in cotton plants (Figure 2).
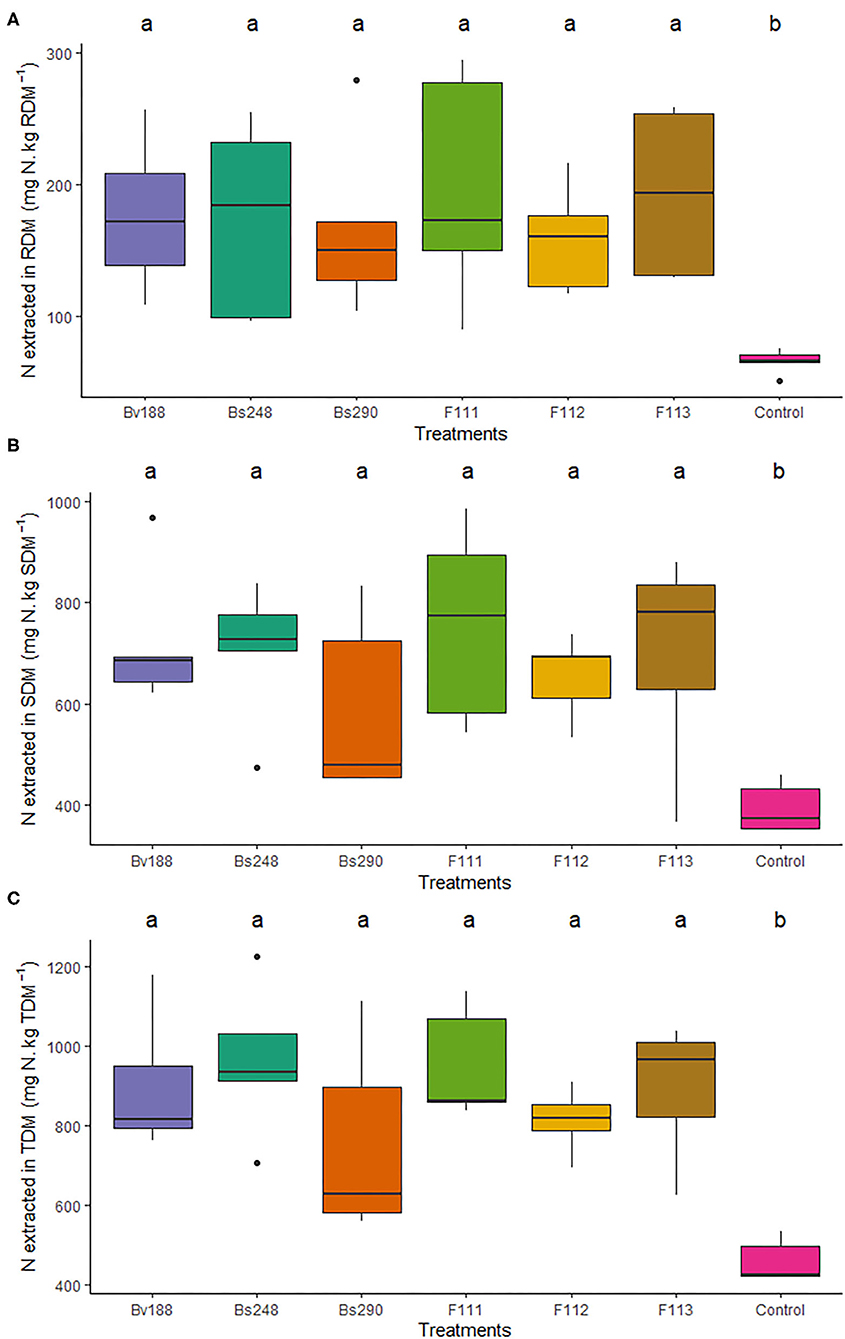
Figure 1. Boxplots (median and quartiles) of N extracted from the roots (A), shoots (B), and total dry matter (C) of cotton plants inoculated with the treatments. Different letters in the rows indicate significant differences between means (Duncan's, p < 0.05). RDM, root dry matter; SDM, shoot dry matter; TDM, total dry matter; Bv188, Bacillus velezensis strain Bv188; Bs248, Bacillus subtilis strain Bs248; Bs290, B. subtilis strain Bs290; F111, Aspergillus brasiliensis; F112, Aspergillus sydowii; F113, Aspergillus sp. versicolor section; Control, no inoculation.
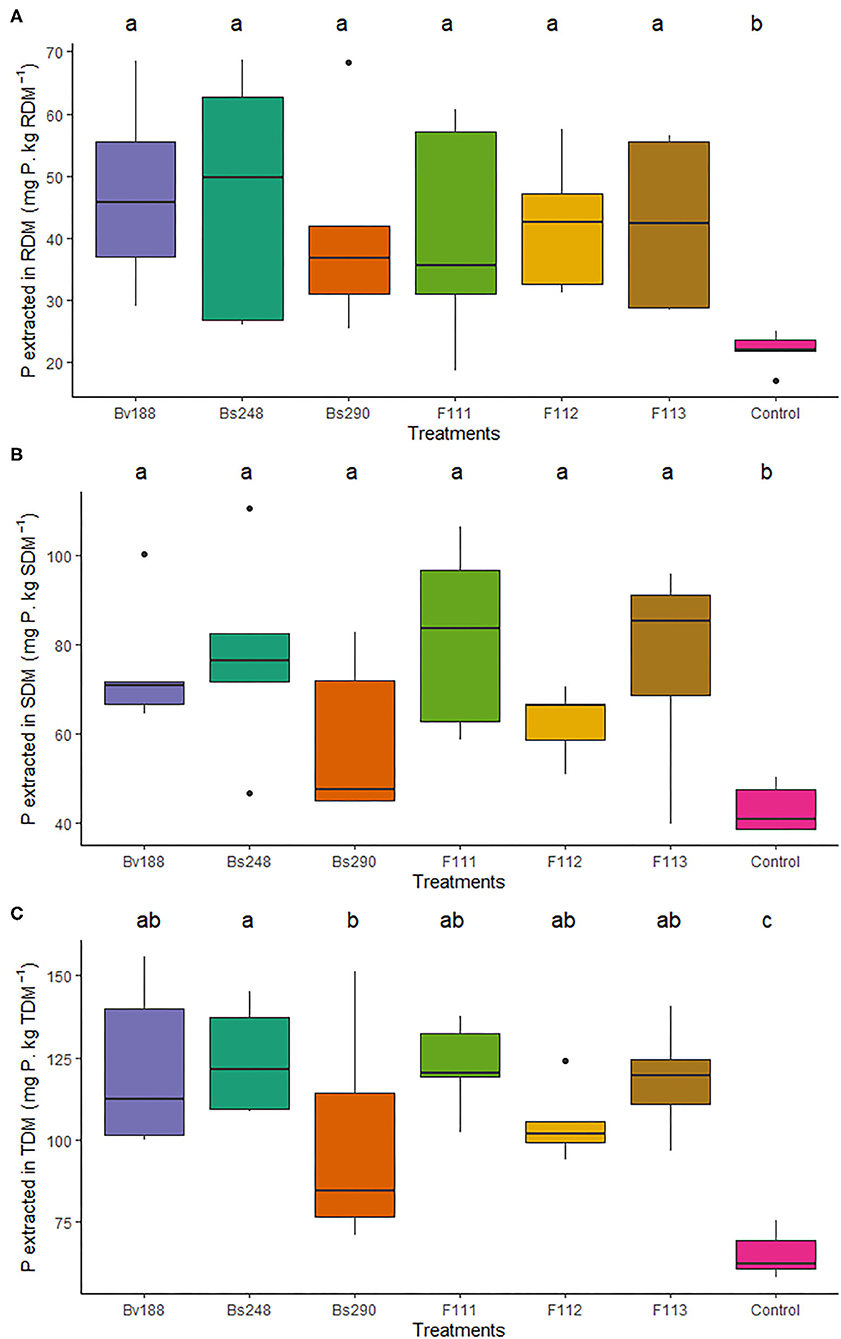
Figure 2. Boxplots (median and quartiles) of P extracted from the roots (A), shoots (B), and total dry matter (C) of cotton plants inoculated with the treatments. Different letters in the rows indicate significant differences between means (Duncan's, p < 0.05). RDM, root dry matter; SDM, shoot dry matter; TDM, total dry matter; Bv188, Bacillus velezensis strain Bv188; Bs248, Bacillus subtilis strain Bs248; Bs290, B. subtilis strain Bs290; F111, Aspergillus brasiliensis; F112, Aspergillus sydowii; F113, Aspergillus sp. versicolor section; Control, no inoculation.
Cotton plants inoculated with bacteria Bv188, Bs248, and Bs290 showed higher weight of the root dry matter (12.37, 15.03, and 20.60 g/plant, respectively) compared to the control (7.05 g/plant), of the shoot dry matter (28.71, 28.56, and 29.27 g/plant, respectively) compared to the control (19.65 g/plant), and of the total dry matter (41.08, 43.59, and 49.86 g/plant, respectively) compared to the control (26.70 g/plant) (Figure 3). In the case of fungi, inoculation with F111, F112, and F113 presented greater weights of root dry matter (13.17, 11.87, and 21.28 g/plant, respectively) and total dry matter (37.52, 37.41, and 53.02 g/plant, respectively).
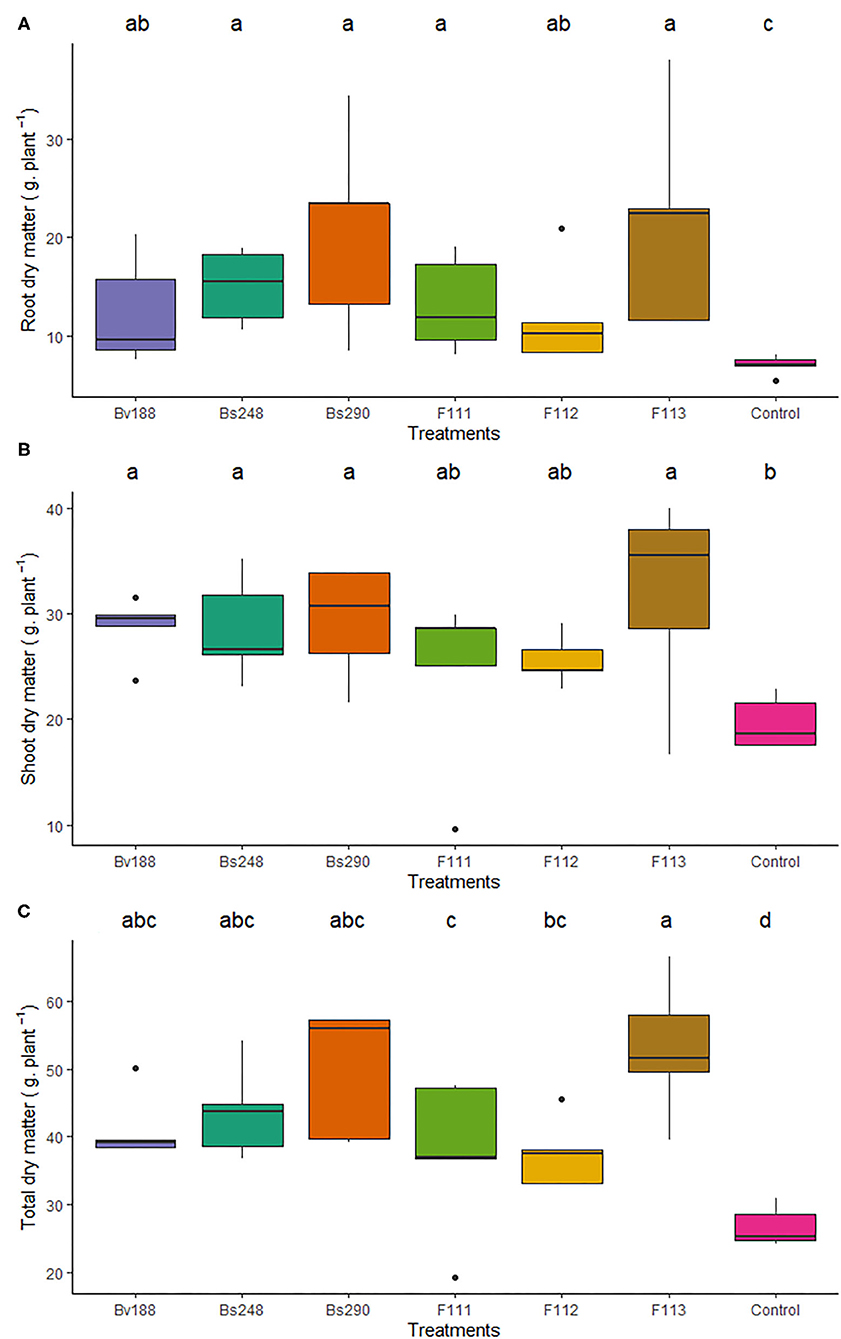
Figure 3. Boxplots (median and quartiles) of the root (A), shoot (B), and total dry matter (C) of cotton plants inoculated with the treatments. Different letters in the rows indicate significant differences between means (Duncan's, p < 0.05). Bv188, Bacillus velezensis strain Bv188; Bs248, Bacillus subtilis strain Bs248; Bs290, B. subtilis strain Bs290; F111, Aspergillus brasiliensis; F112, Aspergillus sydowii; F113, Aspergillus sp. versicolor section; Control, no inoculation.
The nitrogen content in the shoot dry matter (10.16 g N/kg dry matter) (Figure 4A) and phosphorus content in the root dry matter (4.28 g P/kg dry matter) were higher in cotton plants inoculated with Bv188 (Figure 4B) and Bs248 (Figure 5A) compared to the control (20.02 N/kg dry matter). There were no differences in the nitrogen content in the root dry matter (p = 0.175), and the phosphorus content in the shoot dry matter was not different from that in the control except for F112, which was lower (p < 0.003) (Figure 5B).
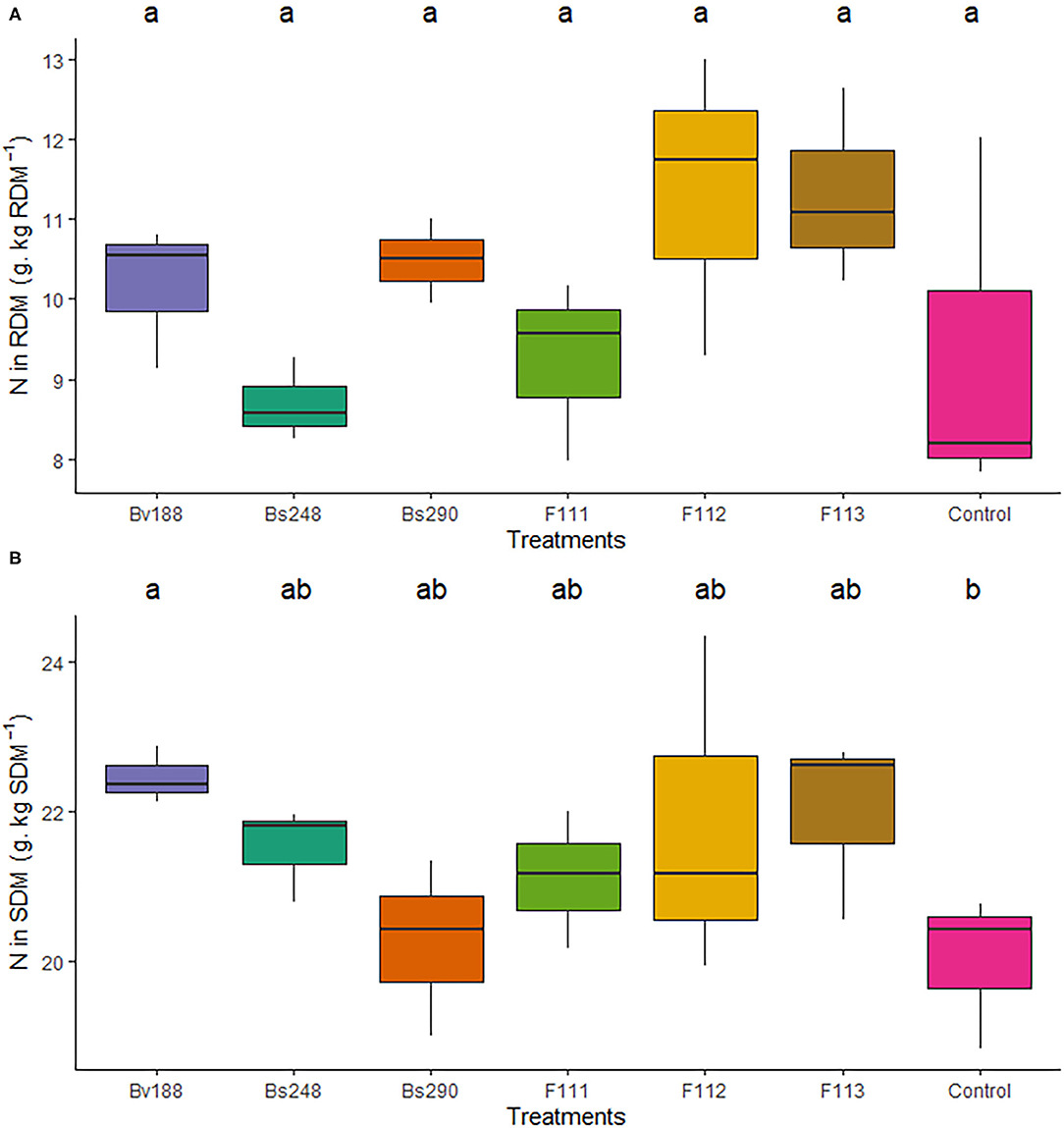
Figure 4. Boxplots (median and quartiles) of the N content in the root (A) and shoot (B) dry matter of cotton plants inoculated with the treatments. Different letters in the rows indicate significant differences between means (Duncan's, p < 0.05). RDM, root dry matter; SDM, shoot dry matter; Bv188, Bacillus velezensis strain Bv188; Bs248, Bacillus subtilis strain Bs248; Bs290, B. subtilis strain Bs290; F111, Aspergillus brasiliensis; F112, Aspergillus sydowii; F113, Aspergillus sp. versicolor section; Control, no inoculation.
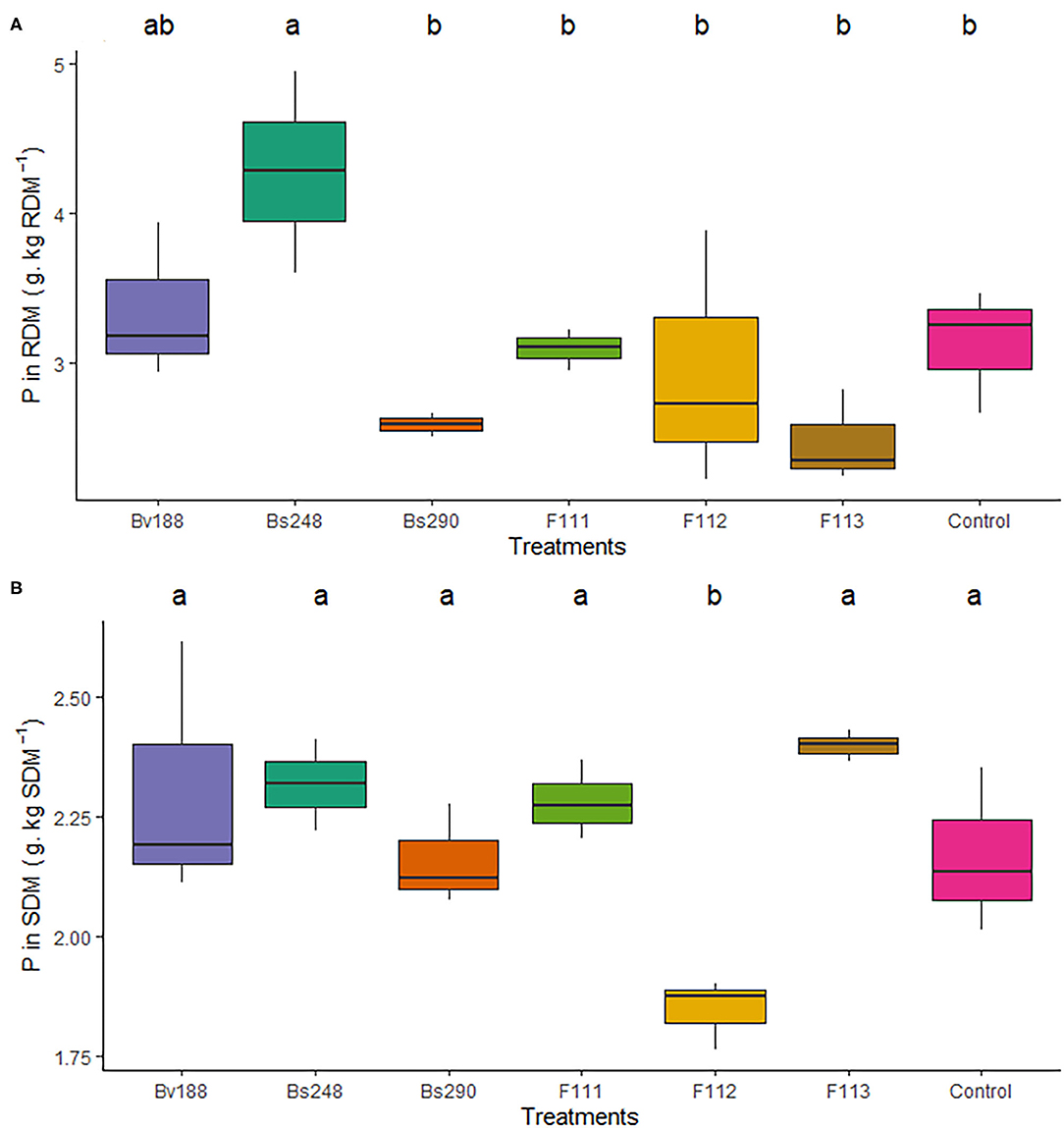
Figure 5. Boxplots (median and quartiles) of P extracted in root (A) and shoot (B) total dry matter of cotton plants inoculated with the treatments. Different letters in the rows indicate significant differences between means (Duncan's, p < 0.05). RDM, root dry matter; SDM, shoot dry matter; Bv188, Bacillus velezensis strain Bv188; Bs248, Bacillus subtilis strain Bs248; Bs290, B. subtilis strain Bs290; F111, Aspergillus brasiliensis; F112, Aspergillus sydowii; F113, Aspergillus sp. versicolor section; Control, no inoculation.
The nitrogen percentage was lower in soils inoculated with Bv188, Bs248, Bs290, F111, F112, and F113 (5.12, 5.23, 8.07, 5.23, 5.23, and 6.02%, respectively) than in the control (8.77%) (Figure 6A). The soluble phosphorus content was lower only in soils inoculated with fungi F111 and F112 (7.10 soil and 16.97 mg/dm3 soil, respectively) compared to the control (26.91 mg/dm3 soil) (Figure 6B). The biomass carbon results indicated that inoculation with Bv188, Bs248, Bs290, F111, and F113 (72.26, 35.18, 32.54, 38.29, and 45.16 mg C/kg soil, respectively) positively affected this variable compared to the control (6.41 mg C/kg soil) (Figure 6C). In the case of respiratory activity, a positive effect was only observed for inoculation with microorganisms Bv188, Bs248, F11, F112, and F113 (16.41, 23.21, 14.98, 10.43, and 7.11 mg CO2/100 g soil, respectively) compared to the control (3.50 mg CO2/100 g soil) (Figure 6D).
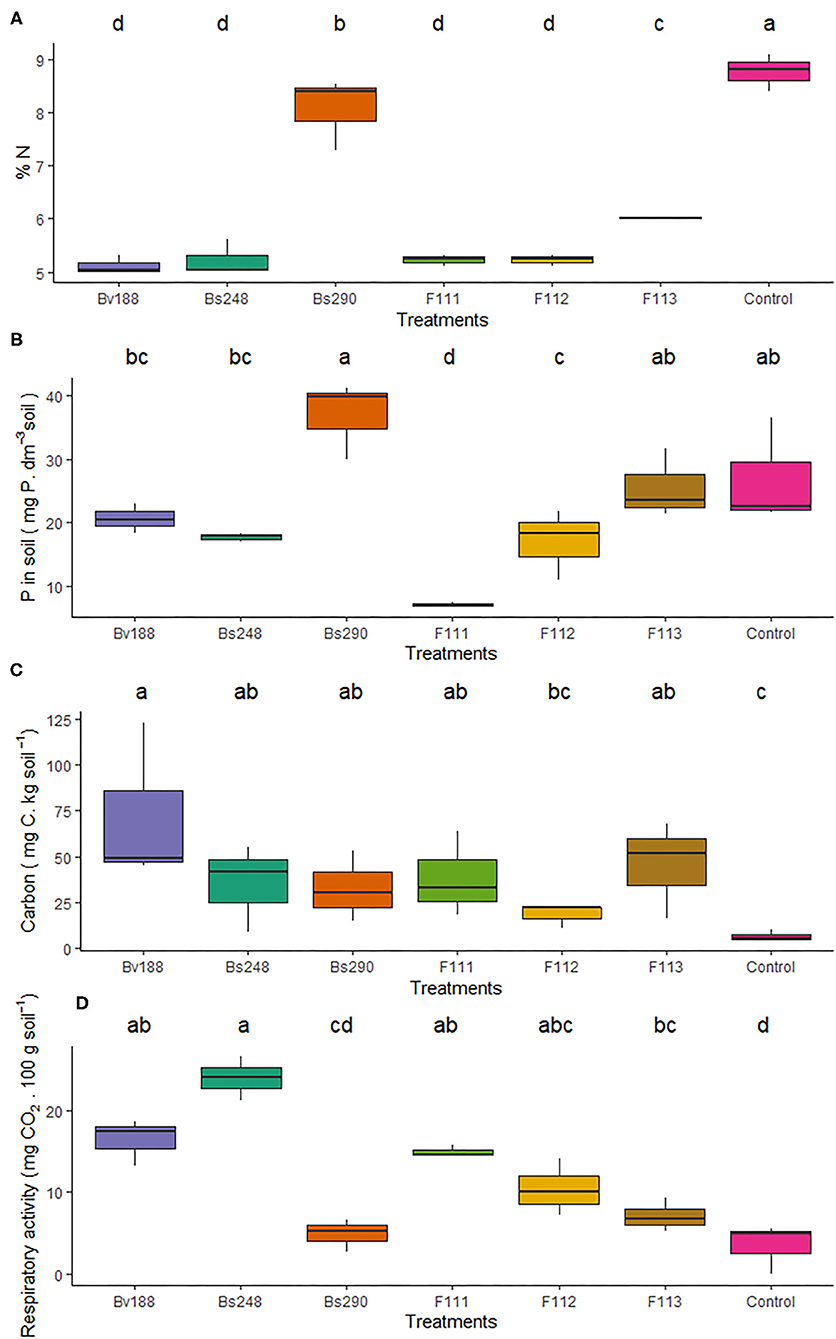
Figure 6. Boxplots (median and quartiles) of the N percentage (A), soluble phosphorus (B), biomass carbon (C), and respiratory activity (D) in soil inoculated with the treatments. Different letters in the rows indicate significant differences between means (Duncan's, p < 0.05). RDM, root dry matter; SDM, shoot dry matter; TDM, total dry matter; Bv188, Bacillus velezensis strain Bv188; Bs248, Bacillus subtilis strain Bs248; Bs290, B. subtilis strain Bs290; F111, Aspergillus brasiliensis; F112, Aspergillus sydowii; F113, Aspergillus sp. versicolor section; Control, no inoculation.
Discussion
The microorganisms used in the present study showed specific abilities to promote growth in cotton plants according to the parameters evaluated. There was no microorganism that improved all the parameters analyzed.
Concerning the shoot and root dry matter, higher values were found for Aspergillus sp. versicolor and B. subtilis 290, although there was no significant difference between them. When bacteria promote an increase in shoot dry mass, the plant has a great chance to improve its photosynthetic efficiency, producing more metabolites for growth. When root development is increased, plants increase their efficiency in exploring the soil and their capacity to absorb water and nutrients (Hu and Chabbi, 2021). The abilities shown for these two microorganisms are important for plant growth.
Bacillus sp. isolated from the wheat rhizosphere showed the ability to promote plant growth by increasing the shoot and root length, fresh weight, dry weight, production of siderophores, phosphorus solubilization activity, production of IAA, and inhibitory action on the growth of Fusarium oxysporum in medicinal plants (Zhao et al., 2015). El-Deeb et al. (2012) isolated B. subtilis from roses and evaluated their colonization capacity to increase the content of dry matter, solubilization of phosphorus, production of siderophores and extracellular enzymes, and production of hydrolytic enzymes. B. subtilis in the rhizosphere promotes a decrease in soil pH, production of organic acids in the rhizosphere, and anion exchange and chelation, thus demonstrating its potential to improve phosphorus absorption in cotton crops (Ahmad et al., 2021).
In the present study, B. velezensis (Bv188) isolated from maize plants promoted an increase in the total and root dry matter, nitrogen absorption in the shoots, and phosphorus solubilization in the roots. Similar results were found by Meng et al. (2016), who demonstrated that inoculation with B. velezensis BAC03 promoted greater biomass gain, IAA production, and enzymatic activity in beet, carrot, cucumber, and radish plants.
Bacillus velezensis FZB42, a strain previously classified as Bacillus amyloliquefaciens ssp. plantarum FZB42, due to the morphology, physiology, chemotaxonomy, and phylogenetic similarity related to them, sharing the same phenotype and genotype (Dunlap et al., 2016). This bacterium isolated from the beet rhizosphere has been investigated as a new species that is widely found in the rhizosphere and has potential for agricultural use due to its inhibitory action on fungi and biocontrol skills (Perron et al., 2020).
The two isolates of the genus Aspergillus used in this study were sequenced and identified as A. brasiliensis (F111) and A. sydowii (F112) by Baron et al. (2018). The results demonstrate that these fungi inoculated in cotton plants favor phosphorus and nitrogen absorption (Figure 6B). Both A. brasiliensis and A. sydowii showed great biotechnological potential due to the production of enzymes and other substances of interest and can be used to aid in the absorption and solubilization of phosphorus and nitrogen, which are important for plant growth.
Interestingly, A. brasiliensis promoted the lowest value of phosphorus in the soil. This result suggests that this fungus has a great ability to mineralize this nutrient, promoting its greater availability to plants. The ability to solubilize phosphorus is important because this nutrient is essential to plant growth, including cotton crops, and easily, this nutrient is absorbed by soil clay, becoming unavailable to plants (Wan et al., 2020). Phosphorus is responsible for the storage and transfer of energy such as glucose, fructose, and ATP (Habib et al., 2015; Mumtaz et al., 2019). Similar results were found by Liang et al. (2020), who demonstrated that phosphorus-solubilizing bacteria also influence the physical, chemical, and biological soil properties by significantly increasing the growth and productivity of tomato plants. Another essential nutrient is nitrogen, which is an important element for life. It is present in the structures of essential biochemicals, such as nucleotides and proteins. Fungi do not have the ability to fix nitrogen from the atmosphere; however, fungi mineralize soil organic matter, making this nutrient available to plants (Baron et al., 2018).
Filamentous fungi belonging to the genera Aspergillus and Trichoderma are recognized as producers of cellulolytic enzymes used in the bioethanol industry (Zoglowek et al., 2015). Studies have shown that A. brasiliensis is grouped in the section of black aspergillus and produces large amounts of citric acid and enzymes, such as xylanases, thermostable β-xylidasidases, α-glucosidase, and amylases, with enzymes being one of the main factors responsible for the characteristic phosphorus solubilization in soil (Nahas and de Assis, 1992; Schneider et al., 2010; de Oliveira Mendes et al., 2014).
Another important highlight in this work is verified by the biomass (Figure 6C) and microbial respiration (Figure 6D) in the soil. Inoculation positively affected the biological characteristics of the soil, which contributed to an increase in the microbial community (bacterial biomass and respiratory activity).
Biomass carbon is directly proportional to the colonization ability of microorganisms. The microorganisms that stood out in this parameter were B. velezensis 188 and B. subtilis 248. Another parameter that reinforces this result is the increase in respiratory activity related to soil microbial activity. Ju et al. (2019) demonstrated the beneficial impact of the inoculation of rhizospheric microorganisms on plant growth in addition to the increase in microbial biomass, which positively contributed to the biochemical properties of soils planted with lettuce.
As previously mentioned, no microorganism showed a great ability to increase all parameters. On the other hand, all microorganisms improved one or more parameters compared to the control, which did not receive microbial inoculation.
Conclusion
The results suggest that the Aspergillus sp. versicolor tends to improve plant growth and development. Nevertheless, A. brasilense can improve the phosphorus availability in soil, and B. subtilis 248 promoted an increase in phosphorus extracted from the roots and total and phosphorous compounds from the root dry matter and increased the value of soil respiratory activity.
Based on the results, the bacterium B. subtilis 248 could be used as an inoculant in cotton crops.
Data Availability Statement
The raw data supporting the conclusions of this article will be made available by the authors, without undue reservation.
Author Contributions
All authors listed have made a substantial, direct and intellectual contribution to the work, and approved it for publication.
Conflict of Interest
The authors declare that the research was conducted in the absence of any commercial or financial relationships that could be construed as a potential conflict of interest.
Publisher's Note
All claims expressed in this article are solely those of the authors and do not necessarily represent those of their affiliated organizations, or those of the publisher, the editors and the reviewers. Any product that may be evaluated in this article, or claim that may be made by its manufacturer, is not guaranteed or endorsed by the publisher.
Acknowledgments
The first author thanks the Coordination for the Improvement of Higher Education Personnel (CAPES) for providing a scholarship.
References
Ahmad, I., Ahmad, M., Hussain, A., and Jamil, M. (2021). Integrated use of phosphate-solubilizing Bacillus subtilis strain IA6 and zinc-solubilizing Bacillus sp. strain IA16: a promising approach for improving cotton growth. Folia Microbiol. 66, 115–125. doi: 10.1007/s12223-020-00831-3
Anand, K., Kumari, B., and Mallick, M. A. (2016). Phosphate solubilizing microbes: an effective and alternative approach as biofertilizers. Int. J. Pharm. Pharm. Sci. 8, 37–40.
Baron, N. C., Costa, N. T. A., Mochi, D. A., and Rigobelo, E. C. (2018). First report of Aspergillus sydowii and Aspergillus brasiliensis as phosphorus solubilizers in maize. Ann. Microbiol. 68, 863–870. doi: 10.1007/s13213-018-1392-5
Bezerra Neto, E., and Barreto, L. P. (2011). Análises Químicas e Bioquímicas em Plantas. Recife: Editora Universitária da UFRPE.
Bremner, J. M. (1996). “Nitrogen-total,” in Methods of Soil Analysis Part 3—Chemical Methods, eds D. L. Sparks et al. (Madison, WI: Soil Science Society of America, American Society of Agronomy), 1085–1121. doi: 10.2136/sssabookser5.3.c37
Brookes, P. C., Powlson, D. S., and Jenkinson, D. S. (1982). Measurement of microbial biomass phosphorus in soil. Soil Biol. Biochem. 14, 319–329. doi: 10.1016/0038-0717(82)90001-3
Bunt, J. S., and Rovira, A. D. (1955). Microbiological studies of some subantarctic soils. J. Soil Sci. 6, 119–128.
CONAB (2021). Acompanhamento da safra brasileira grãos (Sétimo levantamento No. 9; v.8-Safra 2020/21, 25–34). Companhia Nacional de Abastecimento. Available online at: https://www.conab.gov.br/info-agro/safras/graos/boletim-da-safra-de-graos (accessed April 29, 2021).
Constable, G. A., and Bange, M. P. (2015). The yield potential of cotton (Gossypium hirsutum L.). Field Crops Res. 182, 98–106. doi: 10.1016/j.fcr.2015.07.017
Contreras-Cornejo, H. A., Macías, R. L., Cortés, P. C., and López, B. J. (2009). Trichoderma virens, a plant beneficial fungus, enhances biomass production and promotes lateral root growth through an auxin-dependent mechanism in arabidopsis. Plant Physiol. 149, 1579–1592. doi: 10.1104/pp.108.130369
de Oliveira Mendes, G., Moreira de Freitas, A.L., Liparini Pereira, O., da Silva, I. R., Vassilev, N. B., and & Costa, M. D. (2014). Mechanisms of phosphate solubilization by fungal isolates when exposed to different P sources. Ann. Microbiol. 64, 239–249. doi: 10.1007/s13213-013-0656-3
Diaz, P. A. E., Baron, N. C., and Rigobelo, E. C. (2019). Bacillus spp. As plant growth-promoting bacteria in cotton under greenhouse conditions. Aust. J. Crop Sci. 13, 2003–2014. doi: 10.21475/ajcs.19.13.12.p2003
Dunlap, C. A., Kim, S.-J., Kwon, S.-W., and Rooney, A. P. (2016). Bacillus velezensis is not a later heterotypic synonym of Bacillus amyloliquefaciens; Bacillus methylotrophicus, Bacillus amyloliquefaciens subsp. plantarum and ‘Bacillus oryzicola' are later heterotypic synonyms of Bacillus velezensis based on phylogenomics. Int. J. Syst. Evol. Microbiol. 66, 1212–1217. doi: 10.1099/ijsem.0.000858
El-Deeb, B., Bazaid, S., Gherbawy, Y., and Elhariry, H. (2012). Characterization of endophytic bacteria associated with rose plant (Rosa damascena trigintipeta) during flowering stage and their plant growth promoting traits. J. Plant Interac. 7, 248–253. doi: 10.1080/17429145.2011.637161
EMBRAPA Solos (2018). Sistema Brasileiro de Classificação de Solos. 5th ed.. Available online at: https://www.embrapa.br/busca-de-publicacoes/-/publicacao/1107206/sistema-brasileiro-de-classificacao-de-solos (accessed April 20, 2021).
Good, A. G., Shrawat, A. K., and Muench, D. G. (2004). Can less yield more? Is reducing nutrient input into the environment compatible with maintaining crop production? Trends Plant Sci. 9, 597–605. doi: 10.1016/j.tplants.2004.10.008
Habib, S. H., Kausar, H., Saud, H. M., Ismail, M. R., and Othman, R. (2015). Molecular characterization of stress tolerant plant growth promoting rhizobacteria (PGPR) for growth enhancement of rice. Int. J. Agric. Biol. 18, 184–191. doi: 10.17957/IJAB/15.0094
Harrison, M. J. (2005). Signaling in the arbuscular mycorrhizal symbiosis. Annu. Rev. Microbiol. 59, 19–42. doi: 10.1146/annurev.micro.58.030603.123749
Hossain, M. T., Khan, A., Chung, E. J., Rashid, Md,. H.-O, and Chung, Y. R. (2016). Biological control of rice bakanae by an endophytic Bacillus oryzicola YC7007. Plant Pathol. J. 32, 228–241. doi: 10.5423/PPJ.OA.10.2015.0218
Hou, Z., Li, P., Li, B., Gong, J., and Wang, Y. (2007). Effects of fertigation scheme on N uptake and N use efficiency in cotton. Plant Soil 290, 115–126. doi: 10.1007/s11104-006-9140-1
Hu, T., and Chabbi, A. (2021). Does the higher root carbon contribution to soil under cropping cycles following grassland conversion also increase shoot biomass? Sci. Total Environ. 752:141684. doi: 10.1016/j.scitotenv.2020.141684
Islam, K. R., and Weil, R. R. (1998). Microwave irradiation of soil for routine measurement of microbial biomass carbon. Biol. Fertil. Soils 27, 408–416. doi: 10.1007/s003740050451
Jaber, L. R., and Enkerli, J. (2016). Effect of seed treatment duration on growth and colonization of Vicia faba by endophytic Beauveria bassiana and Metarhizium brunneum. Biol. Control 103, 187–195. doi: 10.1016/j.biocontrol.2016.09.008
Jenkinson, D. S., and Powlson, D. S. (1976). The effects of biocidal treatments on metabolism in soil V: a method for measuring soil biomass. Soil Biol. Biochem. 8, 209–213. doi: 10.1016/0038-0717(76)90005-5
Ju, W., Liu, L., Fang, L., Cui, Y., Duan, C., and Wu, H. (2019). Impact of co-inoculation with plant-growth-promoting rhizobacteria and rhizobium on the biochemical responses of alfalfa-soil system in copper contaminated soil. Ecotoxicol. Environ. Saf. 167, 218–226. doi: 10.1016/j.ecoenv.2018.10.016
Khan, A., Tan, D. K. Y., Afridi, M. Z., Luo, H., Tung, S. A., Ajab, M., et al. (2017). Nitrogen fertility and abiotic stresses management in cotton crop: a review. Environ. Sci. Pollut. Res. 24, 14551–14566. doi: 10.1007/s11356-017-8920-x
Kloepper, J. W., Lifshitz, R., and Zablotowicz, R. M. (1989). Free-living bacterial inocula for enhancing crop productivity. Trends Biotechnol. 7, 39–44. doi: 10.1016/0167-7799(89)90057-7
Liang, J.-L., Liu, J., Jia, P., Yang, T., Zeng, Q., Zhang, S., et al. (2020). Novel phosphate-solubilizing bacteria enhance soil phosphorus cycling following ecological restoration of land degraded by mining. ISME J. 14, 1600–1613. doi: 10.1038/s41396-020-0632-4
Lima, V. B., de, S., Dourado, L. R. B., Machado, L. P., Biagiotti, D., de Lima, S. B. P., et al. (2016). Cottonseed Oil in Diets for Broilers in the Pre-Starter and Starter Phases. PLoS ONE 11:e0147695. doi: 10.1371/journal.pone.0147695
Malavolta, E., Vitti, G. C., and de Oliveira, S. A. (1989). Avaliação de Estado Nutricional das Plantas: Princípios e Aplicações. Piracicaba: Associação Brasileira para Pesquisa da Potássio e do Fosfato.
Mendonça, E., and Matos, E. (2017). Matéria Orgânica do Solo: Métodos de Análises. 2nd ed. Viçosa, MG: Universidade Federal de Viçosa.
Meng, Q., Jiang, H., and Hao, J. J. (2016). Effects of Bacillus velezensis strain BAC03 in promoting plant growth. Biol. Control 98, 18–26. doi: 10.1016/j.biocontrol.2016.03.010
Milani, R., Santos, R. M. D., Bentes, L. L., Lazarovits, G., and Rigobelo, E. C. (2019). Bacillus subtilis isolates with different abilities to promote plant growth in maize, cotton and soybean crops isolation and characterization of bacterial strains. Asian J. Microbiol. Biotechnol. Environ. Sci. 21, 827–836.
Mumtaz, M. Z., Barry, K. M., Baker, A. L., Nichols, D. S., Ahmad, M., Zahir, Z. A., et al. (2019). Production of lactic and acetic acids by Bacillus sp. ZM20 and Bacillus cereus following exposure to zinc oxide: a possible mechanism for Zn solubilization. Rhizosphere 12:100170. doi: 10.1016/j.rhisph.2019.100170
Nahas, E., and de Assis, L. C. (1992). Solubilização de fosfatos de rocha por Aspergillus niger em diferentes tipos de vinhaça. Pesqui. Agropecu. Bras. 27, 325–331.
Numan, M., Bashir, S., Khan, Y., Mumtaz, R., Shinwari, Z. K., Khan, A. L., et al. (2018). Plant growth promoting bacteria as an alternative strategy for salt tolerance in plants: a review. Microbiol. Res. 209, 21–32. doi: 10.1016/j.micres.2018.02.003
Peel, M. C., Finlayson, B. L., and McMahon, T. A. (2007). Updated world map of the Köppen-Geiger climate classification. Hydrol. Earth Syst. Sci. 11, 1633–1644. doi: 10.5194/hess-11-1633-2007
Perron, G. G., Neupane, T., and Mendoza, R. A. (2020). Draft genome sequences of Bacillus subtilis strains TNC1(2019), TNC3(2019), and TNW1(2019), as well as Bacillus velezensis strains TNC2(2019) and TNW2(2019), isolated from Cabbage Kimchee. Microbiol. Resour. Announc. 9, e00085–20. doi: 10.1128/MRA.00085-20
R Core Team (2020). R: A Language and Environment for Statistical Computing. Viena, Austria: R Foundation for Statistical Computing. Available online at: https://www.R-project.org/ (accessed February 20, 2021).
Romero-Perdomo, F., Abril, J., Camelo, M., Moreno-Galván, A., Pastrana, I., Rojas-Tapias, D., et al. (2017). Azotobacter chroococcum as a potentially useful bacterial biofertilizer for cotton (Gossypium hirsutum): effect in reducing N fertilization. Rev. Argent. Microbiol. 49, 377–383. doi: 10.1016/j.ram.2017.04.006
Sarruge, J. R., and Haag, H. P. (1974). Análise química em plantas. Piracicaba: ESALQ/USP, Departamento de química.
Schneider, K. D., Van Straaten, P., De Orduña, R. M., Glasauer, S., Trevors, J., Fallow, D., et al. (2010). Comparing phosphorus mobilization strategies using Aspergillus niger for the mineral dissolution of three phosphate rocks. J. Appl. Microbiol. 108, 366–374. doi: 10.1111/j.1365-2672.2009.04489.x
Segarra, G., Van der Ent, S., Trillas, I., and Pieterse, C. M. J. (2009). MYB72, a node of convergence in induced systemic resistance triggered by a fungal and a bacterial beneficial microbe. Plant Biol. 11, 90–96. doi: 10.1111/j.1438-8677.2008.00162.x
Spaink, H. P. (2000). Root nodulation and infection factors produced by rhizobial bacteria. Annu. Rev. Microbiol. 54, 257–288. doi: 10.1146/annurev.micro.54.1.257
Vacheron, J., Desbrosses, G., Bouffaud, M.-L., Touraine, B., Moënne-Loccoz, Y., Muller, D., et al. (2013). Plant growth-promoting rhizobacteria and root system functioning. Front. Plant Sci. 4:356. doi: 10.3389/fpls.2013.00356
van Loon, L.C. (2007). “Plant responses to plant growth-promoting rhizobacteria,” in New Perspectives and Approaches in Plant Growth-Promoting Rhizobacteria Research, eds P. A. H. M. Bakker, J. M. Raaijmakers, G. Bloemberg, M. Höfte, P. Lemanceau, B. M. Cooke (Dordrecht: Springer). doi: 10.1007/978-1-4020-6776-1_2
Van Wees, S. C., Van der Ent, S., and Pieterse, C. M. (2008). Plant immune responses triggered by beneficial microbes. Curr. Opin. Plant Biol. 11, 443–448. doi: 10.1016/j.pbi.2008.05.005
Wan, W., Qin, Y., Wu, H., Zuo, W., He, H., Tan, J., et al. (2020). Isolation and characterization of phosphorus solubilizing bacteria with multiple phosphorus sources utilizing capability and their potential for lead immobilization in soil. Front. Microbiol. 11:752. doi: 10.3389/fmicb.2020.00752
Watanabe, F. S., and Olsen, S. R. (1965). Test of an ascorbic acid method for determining phosphorus in water and NaHCO3 extracts from soil. Soil Sci. Soc. Am. J. 29, 677–678. doi: 10.2136/sssaj1965.03615995002900060025x
Wilke, B. M. (2005). “Determination of chemical and physical soil properties,” in Monitoring and Assessing Soil Bioremediation, eds R. Margesin, and F. Schinner (Berlin, Heidelberg: Springer), 47–95. doi: 10.1007/3-540-28904-6_2
Wollum, A. (1982). “Cultural methods for soil microorganisms,” in Methods of Soil Analysis. Part 2. Chemical and Microbiological Properties, ed A. L. Page, 781–802.
Wu, L., and Liu, M. (2008). Preparation and properties of chitosan-coated NPK compound fertilizer with controlled-release and water-retention. Carbohydr. Polym. 72, 240–247. doi: 10.1016/j.carbpol.2007.08.020
Zhang, H., Xie, X., Kim, M.-S., Kornyeyev, D. A., Holaday, S., and Paré, P. W. (2008). Soil bacteria augment Arabidopsis photosynthesis by decreasing glucose sensing and abscisic acid levels in planta: photosynthesis augmentation by soil bacteria. Plant J. 56, 264–273. doi: 10.1111/j.1365-313X.2008.03593.x
Zhao, L., Xu, Y., Lai, X.-H., Shan, C., Deng, Z., and Ji, Y. (2015). Screening and characterization of endophytic Bacillus and Paenibacillus strains from medicinal plant Lonicera japonica for use as potential plant growth promoters. Braz. J. Microbiol. 46, 977–989. doi: 10.1590/S1517-838246420140024
Keywords: phosphorus solubilization, nitrogen extraction, dry matter, Aspergillus brasiliensis, Bacillus velezensis
Citation: Escobar Diaz PA, Gil OJA, Barbosa CH, Desoignies N and Rigobelo EC (2021) Aspergillus spp. and Bacillus spp. as Growth Promoters in Cotton Plants Under Greenhouse Conditions. Front. Sustain. Food Syst. 5:709267. doi: 10.3389/fsufs.2021.709267
Received: 13 May 2021; Accepted: 13 July 2021;
Published: 13 August 2021.
Edited by:
Matteo Balderacchi, Independent Researcher, Piacenza, ItalyReviewed by:
Mahloro Hope Serepa-Dlamini, University of Johannesburg, South AfricaAngelica Rodriguez Dorantes, Instituto Politécnico Nacional de México (IPN), Mexico
Copyright © 2021 Escobar Diaz, Gil, Barbosa, Desoignies and Rigobelo. This is an open-access article distributed under the terms of the Creative Commons Attribution License (CC BY). The use, distribution or reproduction in other forums is permitted, provided the original author(s) and the copyright owner(s) are credited and that the original publication in this journal is cited, in accordance with accepted academic practice. No use, distribution or reproduction is permitted which does not comply with these terms.
*Correspondence: Everlon Cid Rigobelo, everlon.cid@unesp.br; Paola Andrea Escobar Diaz, paola.escobar@unesp.br