- 1College of Plant Health and Medicine, Qingdao Agricultural University, Qingdao, China
- 2Beijing Key Laboratory of New Technology in Agricultural Application, Beijing University of Agriculture, Beijing, China
Sowing plants that provide food resources in orchards is a potential habitat management practice for enhancing biological control. Flowering plants (providing pollen and nectar) and grasses (providing alternative prey) can benefit natural enemies in orchards; however, little is known about their relative importance. We studied the effect of management practices (flower strips, grass strips, and spontaneous grass) on arthropod predators under organic apple management regimes in apple orchards in Beijing, China. Orchards located at two different sites were assessed for 3 years (2017–2019). The cover crops had a significant impact on the abundance and diversity of arthropod predators. The grass treatment consistently supported significantly greater densities of alternative prey resources for predators, and predators were more abundant in the grass than in the other treatments. The Shannon–Wiener diversity was significantly higher for the cover crop treatment than for the control. Community structure was somewhat similar between the grass and control, but it differed between the flower treatment and grass/control. Weak evidence for an increase in mobile predators (ladybirds and lacewings) in the orchard canopy was found. Ladybirds and lacewings were more abundant in the grass treatment than in the other treatments in 2019 only, while the aphid abundance in the grass treatment was lowest. The fact that grass strips promoted higher predator abundance and stronger aphid suppression in comparison to the flower strips suggests that providing alternative prey for predators has great biocontrol service potential. The selection of cover crops and necessary management for conserving natural enemies in orchards are discussed in this paper.
Introduction
Agriculture intensification has led to the loss of ecological heterogeneity, consequently threatening farmland biodiversity (Benton et al., 2003). Implementing management practices that increase agricultural biodiversity is important for mediating the negative impacts of intensive agriculture. At landscape scales, diversified landscapes hold the most potential for the conservation of biodiversity and sustaining pest control function (Bianchi et al., 2006). At local scales, the allocation of habitats to enhance local diversity in agroecosystems may compensate for local high-intensity management (Tscharntke et al., 2005). To enhance pest control, habitats have been managed to conserve natural enemies (Tscharntke et al., 2007; Fiedler et al., 2008; Isaacs et al., 2009). Habitat management that intentionally conserves non-crop habitats and establishes flower strips can reduce pest density by enhancing functional biodiversity and associated ecosystem services (Landis et al., 2000; Gurr et al., 2017; Lundin et al., 2019).
Orchards are susceptible to damage by a wide range of arthropod pests. Moreover, orchards are often characterized by an intense use of pesticides. The reduced pesticide use in organic orchards can reduce disturbance intensity and promote arthropod predators (Galloway et al., 2021). Predators and parasitoids that are natural enemies of orchard pests can potentially suppress pests to below damaging levels if they are present in sufficient numbers at the appropriate time. The potential for ecosystem services provided by natural enemies has been highlighted in orchards (Simon et al., 2010). Creating diversified hedgerows and cover crops in alleys or the understory may improve the level of biodiversity and ecosystem services (Demestihas et al., 2017). Previous studies have confirmed the role of functional agrobiodiversity as a means of potentially reducing insecticide use in orchards (Cahenzli et al., 2019; Penvern et al., 2019), and manipulating orchard habitat vegetation to promote natural enemies is a pest management practice that has gained increasing interest in recent decades.
Cover crops, grass cover, ecological infrastructures, flowering plants, island habitats, and hedgerows have been used to boost natural enemy diversity and abundance in orchards (Bugg and Waddington, 1994; English-Loeb et al., 2003; Fernández et al., 2008; Bone et al., 2009; Geldenhuys et al., 2021). However, the agroecological principles and practical applications are poorly understood (Duru et al., 2015), and thus scientific support and experiential knowledge could help in the design of diversified farming systems and landscapes.
The selection of optimal plant species for the promotion of natural predators is based on information on the ecological mechanisms of how they benefit the predators. Based on their functions and characteristics, cover crops can be divided into two types: (1) flowering plants that provide pollen and nectar (Gontijo et al., 2013; Lu et al., 2014), and (2) grass plants that provide alternative prey/hosts (Wyss, 1996; Gomez-Marco et al., 2016). Floral resources could benefit the longevity and fecundity of predators (Robinson et al., 2008; He et al., 2021) and also increase the natural enemy assemblages (Cloyd, 2020). However, few studies have considered both criteria when selecting suitable cover plants. The choice of optimal plants for use as cover crops in orchards should be considered a key decision that requires adequate research at the local scale. A comparison of the effects of grass strips and flower strips is necessary, as their performance in the same orchard is not known.
The aim of this study was to evaluate the potential effects of flower strips and grass strips on natural enemies and aphid pests in apple orchards in North China. We conducted a 3-year field experiment in apple orchards by sowing two types of cover crops, including flower strips sown with marigold (Tagetes erecta) and China aster (Callistephus chinensis) and grass strips sown with alfalfa (Medicago sativa) and ryegrass (Lolium perenne). Marigold is a fragrant plant species that has been tested as a cover crop in apple orchards (Song and Han, 2020). We also added an untested flowering plant, namely China aster, to the flower strips. The selection of ryegrass was based on its supply of alternative prey for ladybirds in field crops (Dong et al., 2012), and alfalfa is also reported as a traditional cover crop (Yan et al., 1997). Our primary objective was to evaluate the performance of these crops on the conservation of natural enemies and aphid suppression.
Materials and Methods
Site Descriptions and Experimental Design
The study was carried out in two commercial organic apple orchards. One orchard was located in Yanqing district (Beijing city, 116°6′ 5″ E, 40°35′38″ N) in North China, which consisted of 12-year-old trees. In this orchard, field experiments were conducted during 2017 and 2018. The other orchard was located in Changping district (Beijing city, 116°7′50″ E, 40°12′59″ N) in North China, consisting of 5-year-old trees, where the study was conducted in 2019. Both sites had <700 mm mean annual rainfall.
At Yanqing, the study area was ~3.4 ha (68 m long, 50 m wide). There were three treatments with four replicates per treatment, arranged in a randomized block design. Each plot was 283 m2. Apple trees of the “Guoguang” variety were planted with a tree spacing of 5 × 1.8 m. At Changping, three replicates of each of three treatments were established in a randomized block design. The study area in the Changping orchard was ~1.2 ha (40 m long, 30 m wide). Each plot was 135 m2 and included the apple cultivar “Red Fuji” planted in five rows, with row distances of 3 m and a distance of 1 m between single trees.
Flower mixture and grass mixture were sown in the between-row space at each site (Figure 1). Seeds of the flowers (marigold and China aster) and grasses (alfalfa and ryegrass) were separately mixed with garden sand to ease handling and distribution. These mixes were drilled manually in the spring of 2017, 2018, and 2019 (Table 1). The area within tree rows was left natural. The weed management was according to the local practices. The naturally growing wild grass underneath the trees was mowed three times per year in spring and summer. In the control (CK) plots, the native vegetation consisted mainly of four species: Echinochloa crus-galli, Setaria viridis, Artemisia lavandulaefolia, and Rorippa dubia. The percentage of ground cover was assessed every month during the plant growing season in one of the between-row spaces of each assessment plot.
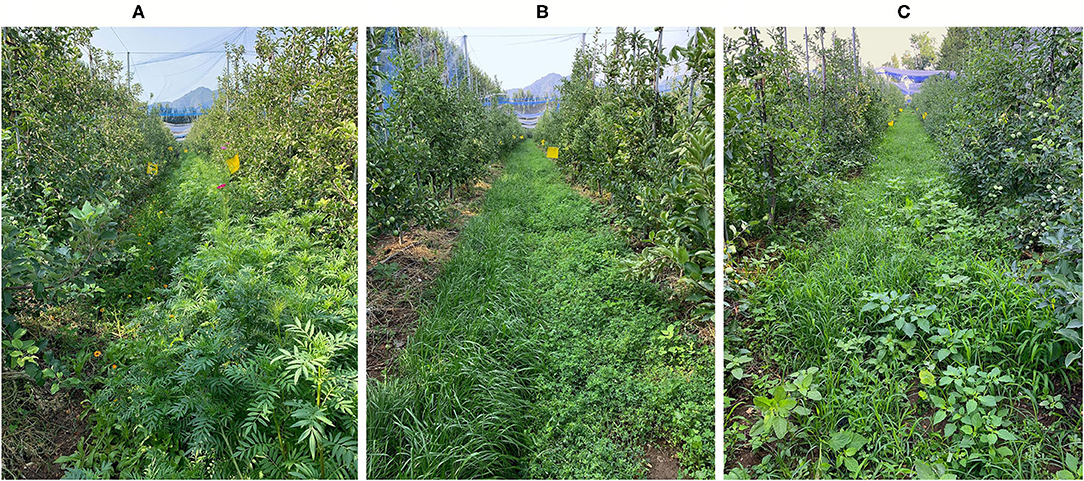
Figure 1. Example of the cover plants in the three treatments: the sown flower strip (A), the sown grass strip (B), and the control (C).

Table 1. Date of cover crop planting and percent area occupied by cover crops in the between-row space of orchards in Yanqing and Changping at 8 weeks after planting (July).
Arthropod Sampling and Monitoring
To assess the influence of treatments on natural enemies in the cover crops and tree canopy, two approaches were used: sweep netting (38 cm diameter) and yellow sticky cards (240 × 100 mm). The sweep net was used to sample arthropods fortnightly in the cover crops. Thirty sweeps in each plot were conducted on each sample date. The sampled arthropods were delivered to the laboratory and identified to the species or genus level based on morphological characteristics. Sticky trap sampling was repeated over 5 months from June through October, with sticky traps placed out for the first week of each month. Yellow sticky cards were suspended on the branches at a height of 1.5 m. The main observed predators were ladybirds and lacewings. Some hoverflies were also seen but were not included in the analysis due to their low numbers.
Aphid density on the tree leaves was measured by directly searching for aphids each week. Within each plot, four apple trees were selected at random as sampling points, and each tree was sampled from four directions (east, south, west, and north). On each side of the tree, 20 leaves were observed, and the numbers of aphids and natural enemies (ladybirds and lacewings) were recorded. However, the densities of ladybirds and lacewings detected on the leaves were low, and therefore these data were not analyzed further.
Data Analysis
Arthropod abundance and percentage of plant cover are presented as means ± standard error of the mean. All of the statistical analyses were completed using R 3.6.3 (R Core Team, 2020).
To determine whether crop type affected the composition of the predator community, we used non-metric multidimensional scaling (NMDS, based on Bray-Curtis dissimilarity) with the vegan package (Oksanen et al., 2020). The assemblages of arthropod predators were compared between the plots with cover and the controls by PERMANOVA using the adonis function, and the Euclidean distances were calculated using the vegdist function; these two functions are available in the vegan package. The Shannon–Wiener diversity index of predators for each sample date was calculated using the total number of captures of each of the taxa using the diversity function in the vegan package. Linear mixed-effects models (LMMs) in the lme4 package (Bates et al., 2015) were used to estimate the effect of the cover crops on the Shannon–Wiener diversity index. Sampling date was introduced in the models as a random factor.
To determine if crop type affected arthropod abundances in cover crops, we used general linear mixed effects models (GLMM) fitted to a poisson distribution. The models included the abundance of total predators (or each group of predators) as dependent variables and year as fixed effects, with date as a random effect. The χ2- and P-values were obtained using the ANOVA function in the car package (Fox and Weisberg, 2019). We then used the glht function in the package multcomp (Hothorn et al., 2008) for post-hoc pairwise comparisons. The effects of cover crops on the aphid abundance on apple tree leaves and herbivore in cover crops were also tested using GLMM with a poisson distribution. Each response variable (aphid and herbivores) included the data of 2017 and 2019. The data of aphid and herbivores in 2018 was not available because no specific counting was recorded.
Results
Percentage of Ground Cover
The cover crop planting dates varied among the 3 years. The planting date in 2019 was a week earlier than in 2017 and 3 weeks earlier than in 2018. The cover crop percentage increased as the growing season progressed. Eight weeks after planting, the mean percentage of cover crops almost exceeded 50.0% and varied across the 3 years (Table 1). In contrast to the coverage in 2017 and 2018, the percentage of cover crops in 2019 had already reached 100.0% after 8 weeks.
Predator Community and Abundance
Overall, we observed arthropod predators in the cover crops from four different families. The observed predators included ladybirds (Coccinellidae), lacewings (Chrysopidae), hoverflies (Syrphidae), and spiders (mainly Philodromidae). The complete list of dominant species can be read in in the Supplementary Table 1.
The two-dimensional NMDS plots of the predator community in 2017, 2018, and 2019 had stress values of 0.069, 0.108, and 0.107, respectively (Figure 2). The PERMANOVA analysis indicated that the community composition in 2017 varied significantly among treatments (P = 0.001). The post-hoc analysis indicated that communities occurring in the three treatments differed from one another (flower vs. grass: P = 0.045, grass vs. control: P = 0.006, flower vs. control: P = 0.018). The community composition in 2018 also differed significantly among treatments (P = 0.002). Post-hoc analysis indicated that communities occurring in the flowers differed from the control (P = 0.036) and grass (P = 0.009), while the control and grass did not differ from each other (P = 0.081). In 2019, the community composition was not affected by treatment (P = 0.739).
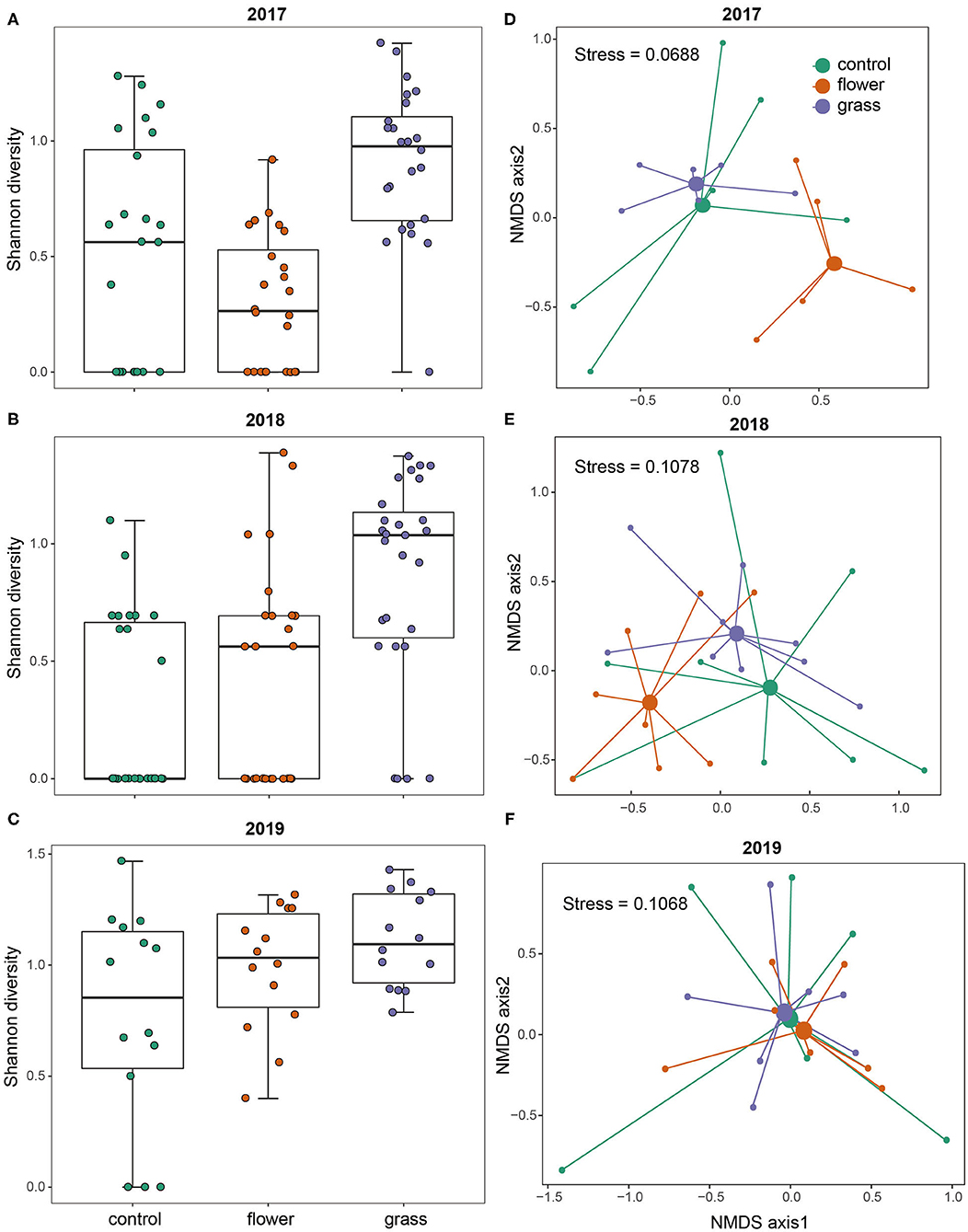
Figure 2. Diversity and community structure of the predators in the control, flower, and grass cover plants during the 3 years (2017–2019). Each year is presented separately: 2017 (A,D), 2018 (B,E), and 2019 (C,F). NMDS plots are presented based on Bray–Curtis similarity.
Predator diversity varied significantly with treatment (χ2 = 63.803, df = 2, P < 0.0001) and year (χ2 = 19.837, df = 2, P < 0.0001). The mean predator diversity was higher in the grass treatment than in the flower and control treatments. Through analyzing the data in each year separately, the post-hoc tests showed that predator diversity in the grass was consistently higher than in the control in the 3 years (all P < 0.001, Figure 2). On the contrary, the flower treatment had a similar predator diversity with the control in the 3 years (all P > 0.05, Figure 2).
Treatment and year significantly affected predator abundances (Table 2). The results from the post-hoc tests showed that the abundances of total predators were highest in the grass, followed by the flowers, and lowest in the control (Figure 3C). The interactions of treatment and year were significant in most cases (Table 2). Both the grass and flower treatments possessed more predators than the control. Hoverflies, lacewings, and ladybirds were higher in the grass than in the flowers, while the spiders were high in the flower as well as in the grass (Figure 3). Herbivore abundance was significantly higher in the grass treatment than in the flower and control treatments (Table 2; Figure 3F). In 2017 and 2019, the lowest abundance was recorded in the flower treatment. Both the grass treatment and the control exhibited relatively high abundance of herbivores. The herbivores were mainly composited of small sucking insect, such as Aphididae, Miridae, and Delphacidae.
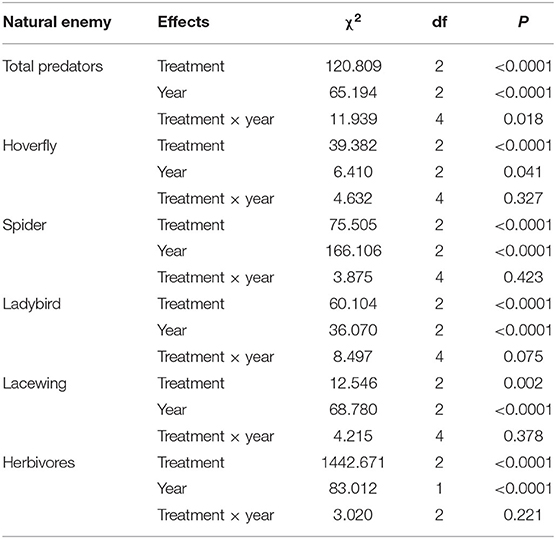
Table 2. Coefficients and statistics of the generalized linear mixed effects models (GLMM) to test for the effect of the cover plant type and year on the abundance of natural enemies and herbivores collected from the sweep net.
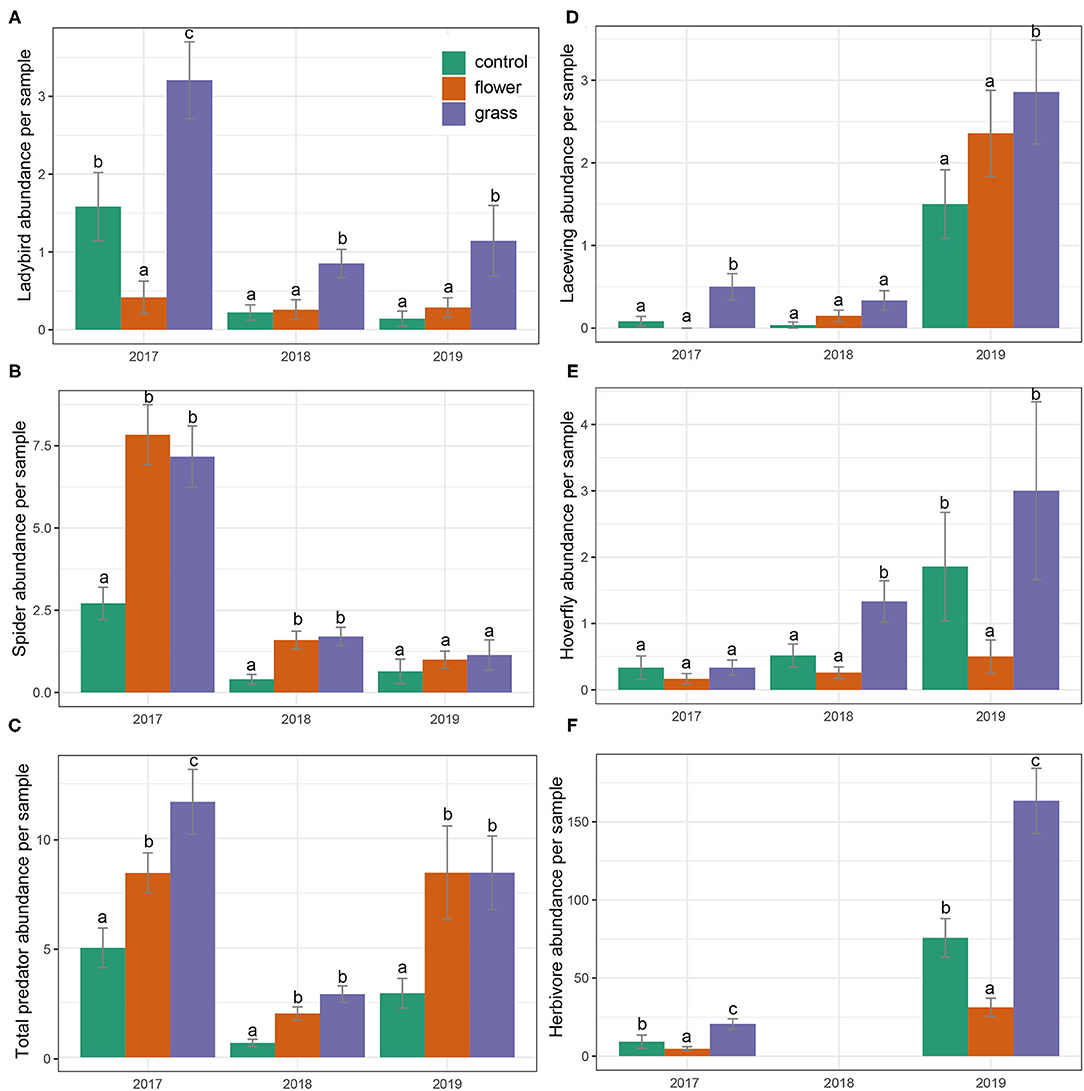
Figure 3. (A-F) Predator and herbivore abundances (mean ± SE) in the cover crops during the 3 years (2017–2019). Within each year, bars capped with the different letter are significantly different (P < 0.05).
Main Predators in Tree Canopy
For the canopy sampling, we used yellow sticky cards to monitor the abundance of flying predators. Ladybirds and lacewings were the most abundant taxa in the sticky card samples. There were three species of ladybird (Harmonia axyridis, Propylaea japonica, and Chilocorus kuwanae) and one species of lacewing (Chrysoperla sinica).
We observed 2,857 individual predators during the 3 years (2017: 802, 2018: 556, 2019: 1499). Ladybirds and lacewings accounted for 72% and 28% of the number of predators captured, respectively. A low number of hover flies (Syrphidae) were captured but were not included in the analysis.
There were significant differences in the occurrence of predators (lacewings and ladybirds) (Table 3). The abundance of ladybirds in the tree canopy in 2019 varied significantly by cover crop treatment (χ2 =1688.3, P = 0.003). Post-hoc analysis indicated that the abundances of ladybirds in the flowers and grass were higher than in the control treatment. In 2017 and 2018, ladybird abundance did not differ among the treatments. Lacewings consistently exhibited similar abundances across the three treatments, with the exception of 2018. Lacewing abundance was highest in the flower treatment, followed by the grass and control, and no differences in lacewing abundance were detected between the grass and control (Figure 4).
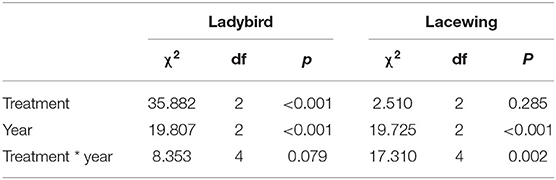
Table 3. Coefficients and statistics of the generalized linear mixed effects models (GLMM) to test for the effect of the cover plant type and year on the abundance of predators collected from the yellow sticky card trap.
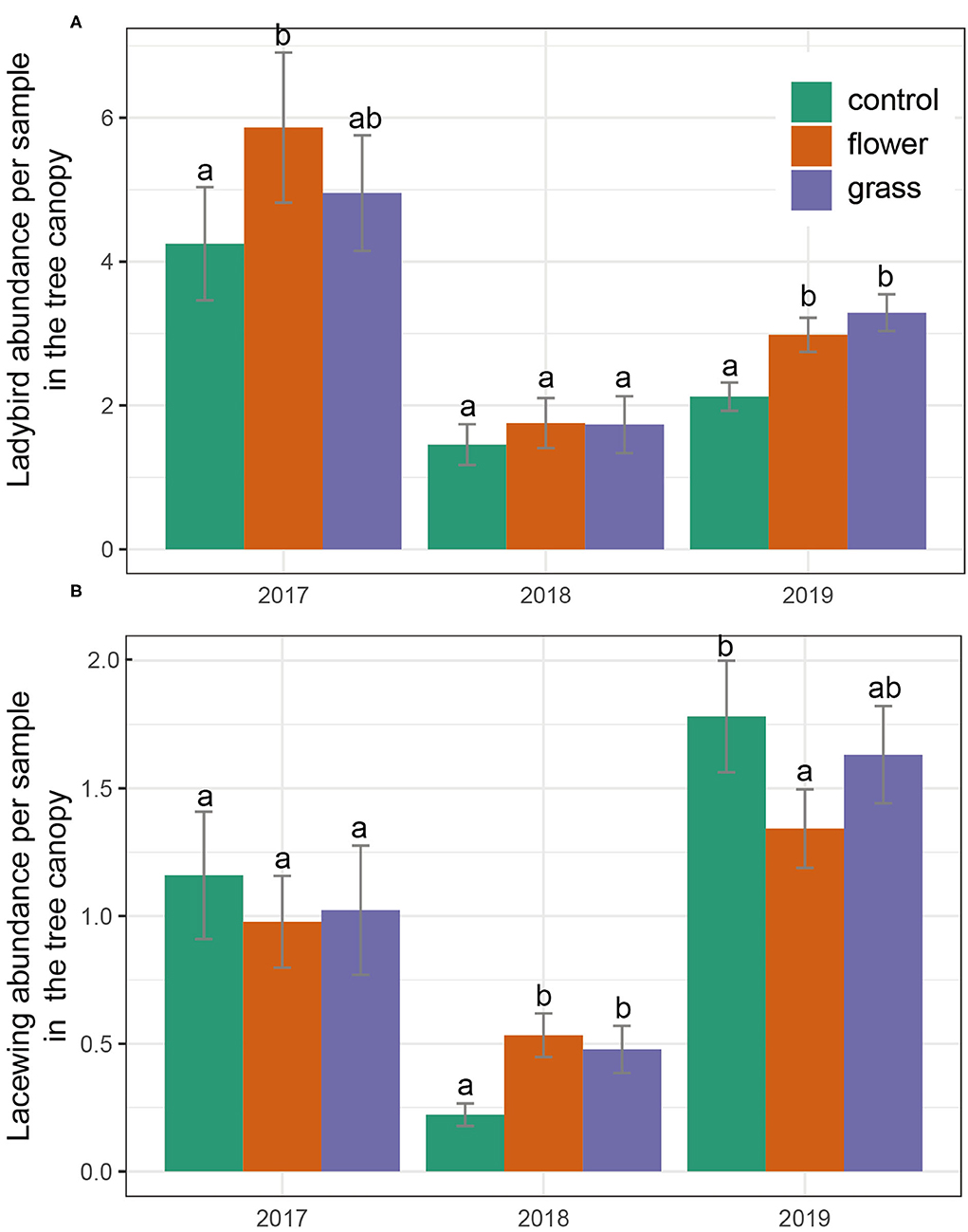
Figure 4. (A,B) Predator abundances (mean ± SE) in the tree canopy during the 3 years (2017–2019). Within each year, bars capped with the different letter are significantly different (P < 0.05).
Aphid Suppression
The aphid abundance in 2017 did not differ significantly among the treatments (χ2 = 2.391, df = 2, P = 0.303). On the contrary, aphid abundance in 2019 differed among the three treatments (χ2 = 58.031, df = 2, P < 0.001), with the abundances in the grass and flower treatments being significantly lower than in the control. As the growing season progressed, the aphid abundance increased and peaked in June and July, following which it declined gradually. In 2019, aphid abundance in the grass treatment was constantly lower than in the flower treatment and control (Figure 5).
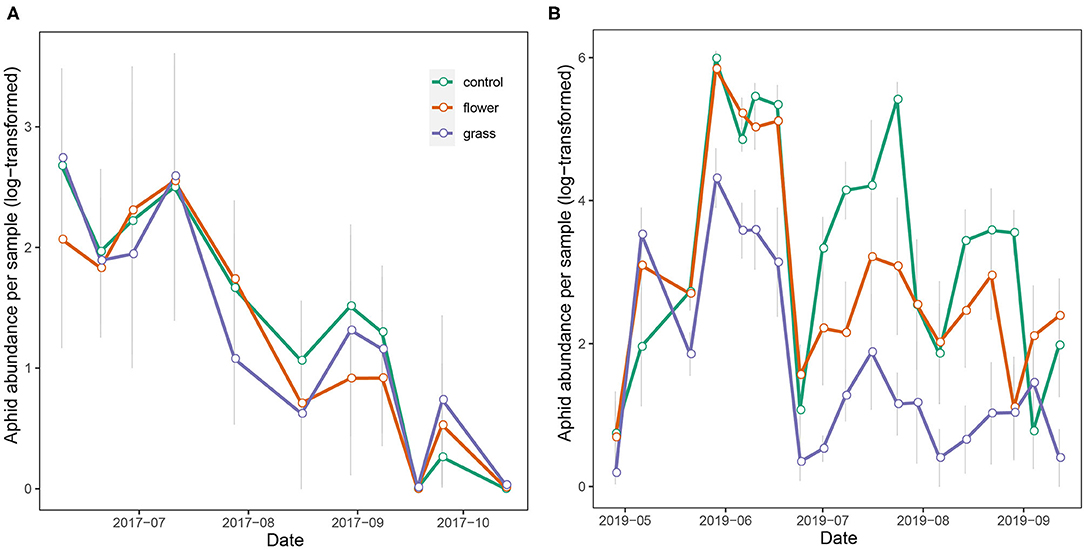
Figure 5. Temporal abundance of aphid (mean ± SE) in the treatments of the apple trees during 2017 (A) and 2019 (B).
Discussion
The overall impact of cover crops on predator abundance in apple orchards was significant in comparison to the impact of spontaneous grasses (control). We hypothesized that different cover crops had a differential impact on the conservation of natural enemies. We found that the mean abundance of total predators was higher in the grass treatment than in the flower treatment. Moreover, predator diversity was also higher in the grass treatment than in the flower treatment. In theory, the composition and size of the pool of natural enemies available for orchard pest suppression could vary significantly with the composition of cover crops in orchards. In cover crop management, a mix of herbaceous plants or flowering plants is often used to house natural enemies in orchards (Eric, 1995; Marliac et al., 2016; de Pedro et al., 2020). For example, it is suggested that perennial flower strips should be placed in orchards to maintain natural enemies (Cahenzli et al., 2019). Considering the functional differences in cover crops, relatively little information on the comparison between flower strips and grass strips is available. Our results suggest that grass strips might offer a promising approach for augmenting natural enemy abundance and diversity.
The grass treatment demonstrated higher predator abundance and diversity than the control and flower treatments. A number of factors may contribute to the increase in predator abundance in the grass treatment. One explanation is that the alternative prey benefits predators during periods of food shortage or disturbance (Happe et al., 2018). In the present study, the herbivores in the grasses were more abundant than in the flowering plants and may have provided food resources to predators. The microclimate created by cover crops has also been cited as a mechanism behind increased predator abundance and suppressed prey populations (Bugg and Waddington, 1994). In our apple orchards, the control treatment (spontaneous grass) was also under organic management. Wild grass on the ground would likely provide microclimate benefits to natural enemies. The community structure of the predators showed similarity in the grass treatment and control, but these treatments differed from the flower treatment. This might be explained by the microclimate, which was similar in the grass treatment and control. However, the spontaneous grass under organic management did not perform as well as the cover crop treatment. Higher predator abundances were observed in the cover crops than in the control. This supports the idea that in organic orchards, a promising approach for conserving natural enemies is the use of functional plants.
Flower strips may have limited capacity to influence predators in our region due to the relatively late planting date of the cover crop. Flowering plants have often been grown in orchards, vegetables, and field crops to improve biological control and reduce dependence on chemical pesticides (Lu et al., 2014). Previous results suggest that flower strips that include fragrant plants favor an increase in natural enemy abundance in orchards (Song et al., 2012; Albert et al., 2017). However, we should also note that nectar or pollen is a limited resource that is usually available for only a short time in the plant growing season. We expected the flowers in the cover crop to attract natural enemies, providing a source of predators that would enhance biocontrol in the orchard. However, if the pest density in orchards is low, the predators will move to other habitats instead of staying in the orchards. Therefore, herbivore populations acting as alternative prey are critical for maintaining a certain number of predators. As most of the predators are highly mobile, the time and duration that they respectively arrive and stay are both important.
Although the cover crops in the apple orchards had a strong impact on the predator abundance and communities in the cover crops, we found weak evidence of an increase in mobile predators in the orchard canopy. Only in 2019 was the predator abundance in the canopy associated with more ladybirds in the grass treatment and flower treatment than in the control. A similar situation was reported by Bone et al. (2009), who found no increase in natural enemies in the orchard canopy when using cover crops. An increase in cover crop benefits did not translate into increases in canopy numbers in the orchards. Such unexpected results may be partly due to the high mobility of ladybirds and lacewings, which might blur the impacts of cover crop. Mobile predators (e.g., ladybirds and lacewings) can offer local population sources of predators. Although the cover crop is not necessarily related to canopy predators, the functions of cover crops are potentially large, especially regarding how they adjust the behavior of predators in orchards. We hypothesized that cover crops could increase biocontrol services in apple orchards by offering food resources for predators. However, only in 1 year did the cover crop improve aphid suppression. It was also reported that increases in natural enemies in cover crops often do not translate into the control of pests in the crop itself (Bone et al., 2009; Markó et al., 2013).
Many studies favor the use of perennial plants to reduce planting costs (Cahenzli et al., 2019), and annual cover crops are also intensive to sow. In the present study, the growing conditions in 2017 and 2018 were not as good as in 2019. This may have resulted from the management practices in these regions, which were inadequate for the cultivation of cover crops. Habitat management through cover crops may be more successful when careful field management can be implemented, e.g., by facilitating the establishment of cover crops by watering dry areas. A greater cover crop biomass may produce greater opportunities for predators and their prey resources to colonize cover crop fields. Vegetative density has been correlated with predator abundance (Rebek et al., 2005). The percentage of ground cover was 100% higher in 2019 than the other years. Implementing practices to plant cover crops earlier may increase the cover crop percentage and potentially increase biocontrol efficiency.
Data Availability Statement
The raw data supporting the conclusions of this article will be made available by the authors, without undue reservation.
Author Contributions
ZD and ZZ conceived and designed the research. CL and BM conducted experiments. MX contributed to the research design. ZD wrote the manuscript. All of the authors read and approved the manuscript.
Funding
This study was supported by the National Key R&D Program of China (2017YFD0200307) and National Natural Science Foundation of China (No. 31501646).
Conflict of Interest
The authors declare that the research was conducted in the absence of any commercial or financial relationships that could be construed as a potential conflict of interest.
Acknowledgments
We thank Shifeng Liu, Hongru Kou, Yanchao Sun, and Pengyuan Sun for field and lab assistance.
Supplementary Material
The Supplementary Material for this article can be found online at: https://www.frontiersin.org/articles/10.3389/fsufs.2021.697864/full#supplementary-material
References
Albert, L., Franck, P., Gilles, Y., and Plantegenest, M. (2017). Impact of agroecological infrastructures on the dynamics of Dysaphis plantaginea (Hemiptera: Aphididae) and its natural enemies in apple orchards in Northwestern France. Environ. Entomol. 46, 528–537. doi: 10.1093/ee/nvx054
Bates, D., Mächler, M., Bolker, B., and Walker, S. (2015). Fitting linear mixed-effects models using lme4. J. Stat. Softw. 67:i01. doi: 10.18637/jss.v067.i01
Benton, T. G., Vickery, J. A., and Wilson, J. D. (2003). Farmland biodiversity: is habitat heterogeneity the key? Trends Ecol. Evol. 18, 182–188. doi: 10.1016/S0169-5347(03)00011-9
Bianchi, F. J., Booij, C. J., and Tscharntke, T. (2006). Sustainable pest regulation in agricultural landscapes: a review on landscape composition, biodiversity, and natural pest control. Proc. R. Soc. B Biol. Sci. 273, 1715–1727. doi: 10.1098/rspb.2006.3530
Bone, N. J., Thomson, L. J., Ridland, P. M., Cole, P., and Hoffmann, A. A. (2009). Cover crops in Victorian apple orchards: effects on production, natural enemies, and pests across a season. Crop Prot. 28, 675–683. doi: 10.1016/j.cropro.2009.03.021
Bugg, R. L., and Waddington, C. (1994). Using cover crops to manage arthropod pests of orchards: a review. Agric. Ecosyst. Environ. 50, 11–28. doi: 10.1016/0167-8809(94)90121-X
Cahenzli, F., Sigsgaard, L., Daniel, C., Herz, A., Jamar, L., Kelderer, M., et al. (2019). Perennial flower strips for pest control in organic apple orchards–a pan-European study. Agric. Ecosyst. Environ. 278, 43–53. doi: 10.1016/j.agee.2019.03.011
Cloyd, R. A. (2020). How effective is conservation biological control in regulating insect pest populations in organic crop production systems? Insects 11:15. doi: 10.3390/insects11110744
de Pedro, L., Perera-Fernández, L. G., López-Gallego, E., Pérez-Marcos, M., and Sanchez, J. A. (2020). The effect of cover crops on the biodiversity and abundance of ground-dwelling arthropods in a Mediterranean pear orchard. Agronomy 10:580. doi: 10.3390/agronomy10040580
Demestihas, C., Plénet, D., Génard, M., Raynal, C., and Lescourret, F. (2017). Ecosystem services in orchards. A review. Agron. Sustain. Dev. 37:12. doi: 10.1007/s13593-017-0422-1
Dong, Z. K., Gao, F. J., and Zhang, R. Z. (2012). Use of ryegrass strips to enhance biological control of aphids by ladybirds in wheat fields. Insect Sci. 19, 529–534. doi: 10.1111/j.1744-7917.2011.01499.x
Duru, M., Therond, O., Martin, G., Martin-Clouaire, R., Magne, M.-A., Justes, E., et al. (2015). How to implement biodiversity-based agriculture to enhance ecosystem services: a review. Agron. Sustain. Dev. 35, 1259–1281. doi: 10.1007/s13593-015-0306-1
English-Loeb, G., Rhainds, M., Martinson, T., and Ugine, T. (2003). Influence of flowering cover crops on Anagrus parasitoids (Hymenoptera: Mymaridae) and Erythroneura leafhoppers (Homoptera: Cicadellidae) in New York vineyards. Agric. For. Entomol. 5, 173–181. doi: 10.1046/j.1461-9563.2003.00179.x
Eric, W. (1995). The effects of weed strips on aphids and aphidophagous predators in an apple orchard. Entomol. Exp. Appl. 75, 43–49. doi: 10.1111/j.1570-7458.1995.tb01908.x
Fernández, D. E., Cichón, L. I., Sánchez, E. E., Garrido, S. A., and Gittins, C. (2008). Effect of different cover crops on the presence of arthropods in an organic apple (Malus domestica Borkh) orchard. J. Sustain. Agric. 32, 197–211. doi: 10.1080/10440040802170624
Fiedler, A. K., Landis, D. A., and Wratten, S. D. (2008). Maximizing ecosystem services from conservation biological control: the role of habitat management. Biol. Control 45, 254–271. doi: 10.1016/j.biocontrol.2007.12.009
Galloway, A. D., Seymour, C. L., Gaigher, R., and Pryke, J. S. (2021). Organic farming promotes arthropod predators, but this depends on neighbouring patches of natural vegetation. Agric. Ecosyst. Environ. 310:107295. doi: 10.1016/j.agee.2020.107295
Geldenhuys, M., Gaigher, R., Pryke, J. S., and Samways, M. J. (2021). Diverse herbaceous cover crops promote vineyard arthropod diversity across different management regimes. Agric. Ecosyst. Environ. 307:10. doi: 10.1016/j.agee.2020.107222
Gomez-Marco, F., Urbaneja, A., and Tena, A. (2016). A sown grass cover enriched with wild forb plants improves the biological control of aphids in citrus. Basic Appl. Ecol. 17, 210–219. doi: 10.1016/j.baae.2015.10.006
Gontijo, L. M., Beers, E. H., and Snyder, W. E. (2013). Flowers promote aphid suppression in apple orchards. Biol. Control 66, 8–15. doi: 10.1016/j.biocontrol.2013.03.007
Gurr, G. M., Wratten, S. D., Landis, D. A., and You, M. S. (2017). Habitat management to suppress pest populations: progress and prospects. Annu. Rev. Entomol. 62, 91–109. doi: 10.1146/annurev-ento-031616-035050
Happe, A. K., Roquer-Beni, L., Bosch, J., Alins, G., Mody, K., and Rroll Dp, J. O. E. E. V. P. (2018). Earwigs and woolly apple aphids in integrated and organic apple orchards: responses of a generalist predator and a pest prey to local and landscape factors. Agric. Ecosyst. Environ. 268, 44–51. doi: 10.1016/j.agee.2018.09.004
He, X. Q., Kiaer, L. P., Jensen, P. M., and Sigsgaard, L. (2021). The effect of floral resources on predator longevity and fecundity: a systematic review and meta-analysis. Biol. Control 153:11. doi: 10.1016/j.biocontrol.2020.104476
Hothorn, T., Bretz, F., and Westfall, P. (2008). Simultaneous inference in general parametric models. Biom. J. 50, 346–363. doi: 10.1002/bimj.200810425
Isaacs, R., Tuell, J., Fiedler, A., Gardiner, M., and Landis, D. (2009). Maximizing arthropod-mediated ecosystem services in agricultural landscapes: the role of native plants. Front. Ecol. Environ. 7, 196–203. doi: 10.1890/080035
Landis, D. A., Wratten, S. D., and Gurr, G. M. (2000). Habitat management to conserve natural enemies of arthropod pests in agriculture. Annu. Rev. Entomol. 45, 175–201. doi: 10.1146/annurev.ento.45.1.175
Lu, Z. X., Zhu, P. Y., Gurr, G. M., Zheng, X. S., Read, D. M., Heong, K. L., et al. (2014). Mechanisms for flowering plants to benefit arthropod natural enemies of insect pests: prospects for enhanced use in agriculture. Insect Sci. 21, 1–12. doi: 10.1111/1744-7917.12000
Lundin, O., Ward, K. L., and Williams, N. M. (2019). Identifying native plants for coordinated habitat management of arthropod pollinators, herbivores and natural enemies. J. Appl. Ecol. 56, 665–676. doi: 10.1111/1365-2664.13304
Markó, V., Jenser, G., Kondorosy, E., Ábrahám, L., and Balázs, K. (2013). Flowers for better pest control? the effects of apple orchard ground cover management on green apple aphids (Aphis spp.) (Hemiptera: Aphididae), their predators and the canopy insect community. Biocontrol Sci. Technol. 23, 126–145. doi: 10.1080/09583157.2012.743972
Marliac, G., Mazzia, C., Pasquet, A., Cornic, J. F., Hedde, M., and Capowiez, Y. (2016). Management diversity within organic production influences epigeal spider communities in apple orchards. Agric. Ecosyst. Environ. 216, 73–81. doi: 10.1016/j.agee.2015.09.026
Oksanen, J., Blanchet, F. G., Friendly, M., Kindt, R., Legendre, P., McGlinn, D., et al. (2020). vegan: Community Ecology Package. R package version 2.5-7. Available online at: https://CRAN.R-project.org/package=vegan.
Penvern, S., Fernique, S., Cardona, A., Herz, A., Ahrenfeldt, E., Dufils, A., et al. (2019). Farmers' management of functional biodiversity goes beyond pest management in organic European apple orchards. Agric. Ecosyst. Environ. 284:11. doi: 10.1016/j.agee.2019.05.014
R Core Team (2020). R: A Language and Environment for Statistical Computing. Vienna: R Foundation for Statistical Computing.
Rebek, E. J., Sadof, C. S., and Hanks, L. M. (2005). Manipulating the abundance of natural enemies in ornamental landscapes with floral resource plants. Biol. Control 33, 203–216. doi: 10.1016/j.biocontrol.2005.02.011
Robinson, K. A., Jonsson, M., Wratten, S. D., Wade, M. R., and Buckley, H. L. (2008). Implications of floral resources for predation by an omnivorous lacewing. Basic Appl. Ecol. 9, 172–181. doi: 10.1016/j.baae.2007.01.002
Simon, S., Bouvier, J. C., Debras, J. F., and Sauphanor, B. (2010). Biodiversity and pest management in orchard systems. A review. Agron. Sustain. Dev. 30, 139–152. doi: 10.1051/agro/2009013
Song, B. Z., and Han, Z. H. (2020). Assessment of the biocontrol effects of three aromatic plants on multiple trophic levels of the arthropod community in an agroforestry ecosystem. Ecol. Entomol. 45, 831–839. doi: 10.1111/een.12858
Song, B. Z., Zhang, J., Wiggins, N. L., Yao, Y. C., Tang, G. B., and Sang, X. S. (2012). Intercropping with aromatic plants decreases herbivore abundance, species richness, and shifts arthropod community trophic structure. Environ. Entomol. 41, 872–879. doi: 10.1603/EN12053
Tscharntke, T., Bommarco, R., Clough, Y., Crist, T. O., Kleijn, D., Rand, T. A., et al. (2007). Conservation biological control and enemy diversity on a landscape scale. Biol. Control 43, 294–309. doi: 10.1016/j.biocontrol.2007.08.006
Tscharntke, T., Klein, A. M., Kruess, A., Steffan-Dewenter, I., and Thies, C. (2005). Landscape perspectives on agricultural intensification and biodiversity–ecosystem service management. Ecol. Lett. 8, 857–874. doi: 10.1111/j.1461-0248.2005.00782.x
Wyss, E. (1996). The effects of artificial weed strips on diversity and abundance of the arthropod fauna in a Swiss experimental apple orchard. Agric. Ecosyst. Environ. 60, 47–59. doi: 10.1016/S0167-8809(96)01060-2
Keywords: cover crops, understory, habitat management, strip sown, predator, conservation biological control
Citation: Dong Z, Xia M, Li C, Mu B and Zhang Z (2021) A Comparison of Flower and Grass Strips for Augmentation of Beneficial Arthropods in Apple Orchards. Front. Sustain. Food Syst. 5:697864. doi: 10.3389/fsufs.2021.697864
Received: 20 April 2021; Accepted: 03 June 2021;
Published: 29 June 2021.
Edited by:
Fang Ouyang, Institute of Zoology, Chinese Academy of Sciences (CAS), ChinaReviewed by:
Hongying Cui, China Agricultural University, ChinaWeiyi He, Fujian Agriculture and Forestry University, China
Copyright © 2021 Dong, Xia, Li, Mu and Zhang. This is an open-access article distributed under the terms of the Creative Commons Attribution License (CC BY). The use, distribution or reproduction in other forums is permitted, provided the original author(s) and the copyright owner(s) are credited and that the original publication in this journal is cited, in accordance with accepted academic practice. No use, distribution or reproduction is permitted which does not comply with these terms.
*Correspondence: Zhiyong Zhang, enp5JiN4MDAwNDA7YnVhLmVkdS5jbg==; Zhaoke Dong, emhhb2tlX2RvbmcmI3gwMDA0MDsxMjYuY29t