- Department of Plant, Soil, and Microbial Sciences, Michigan State University, East Lansing, MI, United States
Through symbiotic biological nitrogen fixation (BNF), grain legumes, such as groundnuts, can enhance soil nitrogen (N) and be an important source of N fertility, as well as a critical component of human nutrition and food security. Because legumes obtain N from soil N stocks as well as BNF, legume residues are key to capturing potential N benefits for soils, which may contribute to increased yields and food production. Here, we conducted a detailed survey at household and field level within a six-village corridor along the western boundary of Kibale National Park (KNP) in western Uganda. We focused on groundnut production and residue management practices and soil organic carbon (SOC) and total N (TN) in fields managed by 100 different households. We also determined SOC and TN in adjacent uncultivated KNP soils. We tested for relationships between socioeconomic factors and farmer groundnut management practices. We calculated a partial N balance and estimated potential N benefits under three scenarios for groundnut BNF. Within the study area, groundnut residue management varied greatly with 51% of surveyed farmers retaining residues on fields through spreading or incorporation, and 49% removing residues, either by transfer to banana groves or burning. Groundnut population density was relatively high with 43% of fields having >30 plants m−2. Despite providing net N inputs of up to 27 kg N ha−1, there was no observed effect of groundnut residue management practices on SOC, TN, or soil C:N ratios. Compared to uncultivated KNP soils, groundnut fields had lower mean levels of SOC and TN and wider C:N ratios. These values are consistent with cultivated soils; however, losses of SOC and TN were lower compared to losses previously reported for conversion from tropical forest to agricultural use. We found that farmer valuation and perception of groundnut residues were influential factors in residue management practices. Overall, we estimated that groundnut residues have the potential to contribute to SOC and TN stocks if retained in the field, but, conversely, removal will result in sizable losses. We find that both environmental and social contexts must be considered when recommending legumes for N provisioning services.
Introduction
Nutrient depletion is a primary factor in soil degradation and low and declining crop yields across sub-Saharan Africa (SSA) (Stoorvogel and Smaling, 1990; Tully et al., 2015a). Among countries in SSA, Uganda experiences some of the highest rates of land degradation, resulting in lower agricultural productivity (Stoorvogel and Smaling, 1990; Wortmann and Kaizzi, 1998; Nkonya et al., 2005). Compared to global averages, inorganic fertilizer use across SSA is very low at 16 kg ha arable land−1 year−1, and it is extremely low in Uganda at 1.8 kg ha arable land−1 year−1 (World Bank, 2016). In Uganda, inorganic fertilizer accessibility, availability, and affordability is limited for smallholder farmers (Omamo, 2003). Organic inputs are often the main option for smallholder farmers and are a potentially more environmentally sustainable nutrient source. However, resource-limited farmers often find organic fertilizers, such as animal manure or compost, challenging to obtain or employ, especially at recommended amounts (Nandwa and Bekunda, 1998). Similarly, farmers face multiple pressures and trade-offs with crop residues, which in addition to being organic inputs, are frequently used as livestock feed and cooking fuel, among other purposes (Erenstein et al., 2015; Tittonell et al., 2015; Valbuena et al., 2015). Nationally, only 15% of Ugandan households reported adding organic or inorganic fertilizers or pesticides to common beans (Phaseolus vulgaris L.), and only 14% added these inputs to maize (Zea mays L.) (UBOS, 2013).
Nitrogen (N) is most often the main limiting nutrient for plant growth and crop yields (Sanchez et al., 1997). In natural ecosystems and low-input cropping systems such as those found across SSA, legumes can play a vital role in N provisioning. Legumes access N from the atmosphere via biological N fixation (BNF) to support growth and the production of high protein grains and N-rich residues (Snapp et al., 1998; Giller, 2001). Legume residues can supply immediate and short-term N to subsequent crops, as well as contribute to long-term N and soil fertility by stimulating microbial biomass production, nutrient cycling and maintenance of or gains in soil organic matter (SOM) (McDonagh et al., 1993; Toomsan et al., 1995; Promsakha Na Sakonnakhon et al., 2005; Srichantawong et al., 2005; Franke et al., 2018; Kermah et al., 2018). Legumes are therefore widely recommended as an organic N source for low-input, resource-limited agroecosystems in SSA where they can supply N critical to both healthy soil functioning and crop production and potentially replenish SOM in degraded soils (Snapp et al., 1998, 2018; Giller, 2001). Importantly, legumes are also critical for human health, nutrition, and dietary diversity as a key source of protein, diverse amino acids, micronutrients, dietary fiber, and phytochemicals (Messina, 1999; Foyer et al., 2016). Indeed, poorer households in SSA rely on legumes for a large proportion of their dietary protein (Akibode and Maredia, 2012) and because of their importance, legumes can often be sold for high prices at local and international markets, generating substantial income for resource-poor households (Snapp et al., 2018). Because of their many potential benefits, legumes are recommended as part of ecological nutrient management and conservation agriculture schemes in SSA with the ultimate goal of improving soil health, and thus the sustainability and resiliency of low-input agroecosystems (Thierfelder et al., 2013; Drinkwater et al., 2017).
Across SSA, farmers grow grain legumes for provision of food and income, in addition to BNF benefits. The two most widely grown grain legumes in SSA and in Uganda are common bean and groundnut (Arachis hypogaea L.) (UBOS, 2014; Snapp et al., 2018). Because common bean often has low rates of BNF, supplying limited amounts of N in rotation with cereals, we chose to focus on groundnuts. Groundnuts are capable of fixing substantial amounts of N and have a moderate-to-low harvest index, and so can supply a relatively high quantity of N in rotation with cereals (Giller et al., 1997; Ojiem et al., 2014; Franke et al., 2018). Despite N-rich grain removal with harvest, grain legumes with a relatively low harvest index can deliver substantial N benefits to soil; groundnut residues have been found to provide up to 139 kg N ha−1 (Ojiem et al., 2014). However, N credits or gains from legumes are notoriously challenging to determine in the field (Cadisch et al., 2000; Unkovich and Pate, 2000). Fixation efficiency and total quantity of N fixed can vary dramatically depending on legume variety, agroecological conditions (e.g., site, climate, weather, soil type, and fertility), and management practices (e.g., cropping patterns, fertilization) (Peoples and Craswell, 1992; Wani et al., 1995; Dakora and Keya, 1997; Mokgehle et al., 2014). Because legume N fixation is highly variable across cultivar, agroecology, and management, legume N credits are almost always an estimation of potential legume N contributions. In order to fully understand the potential for legume N credits on smallholder farms, we need more long-term data and site and context specific information, including farmer residue management practices, which are key to maximizing both N and C contributions of legume residues to soil (Wani et al., 1995; Giller et al., 1997; Kermah et al., 2018).
Within these agroecosystem contexts, including soil fertility, climate, and management practices, nutrient balances that calculate the N inputs and outputs of a farming system can serve to estimate or quantify legume N benefits (Tully et al., 2015b). Nutrient balances help to highlight the advantages and/or disadvantages of inputs, outputs, and/or management practices in terms of economic, agricultural, and ecological sustainability (Nkonya et al., 2005; Haileslassie et al., 2007). In conjunction with nutrient balances, examination of the relationships among household demographic/socioeconomic characteristics and management practices can elucidate the factors driving farmer decision-making and further contextualize and assess the sustainability of agroecosystems (Nkonya et al., 2005; Ebanyat et al., 2010a). Socioeconomic and demographic factors such as gender of the household head or crop planner (Nijuki et al., 2008; Tanellari et al., 2014; Mugisa et al., 2015), land tenure (Place and Otsuka, 2002; Kassie et al., 2015), ethnic group (Naughton-Treves, 1997; Kirner, 2010), and field distance from the homestead (Tittonell et al., 2005, 2013; Zingore et al., 2007a) have been shown to drive farmer practices and affect farmer access to, use, and decisions regarding resources like residues (Barrett and Bevis, 2015).
Few studies have examined the potential N benefit from grain legumes in SSA while simultaneously adjusting and accounting for legume crop management practices and different fates of legume residues. We present a case study of farm management and residue practices with a focus on groundnuts within smallholder agroecosystems in western Uganda. We collected soils, GIS, socioeconomic and management data and estimated three levels of potential N addition via groundnut BNF. Our objectives were: (1) to assess differences in groundnut residue management; (2) to estimate the potential N benefit from groundnut residues based on their management; (3) to determine if groundnut residue management impacts SOC and TN; and (4) to explore soil and socioeconomic factors driving groundnut residue management practices. We hypothesized that groundnut residue retention in fields had the potential to deliver positive N balances at the field-scale. We expected TN to be greater and soil C:N to be narrower in fields in which groundnut residues were consistently retained vs. fields in which groundnut residues were continually removed. Lastly, we predicted that socioeconomic factors previously identified in the literature as drivers of farmer practices would also be linked to groundnut residue management practices.
Materials and Methods
Study Area
Research was conducted along the western border of Kibale National Park (KNP) in Burahya County, Kabarole district, western Uganda (Figure 1). KNP and the surrounding area fall within the Albertine Rift, a biodiversity hotspot that is part of Africa's Rift Valley (Lepp and Holland, 2006). KNP received its national park designation in 1993, but it has existed as a forest preserve since 1932 (Struhsaker, 1997). The park is a remnant of transitional forest isolated within a densely populated agricultural landscape; in 2006, the population density within 5 km of the park boundary was estimated to be ~300 individuals km−2 (Hartter and Southworth, 2009). Outside KNP, the hilly landscape is dominated by small-scale agriculture, tea plantations, grassland, and fuelwood plantations (Chapman and Lambert, 2000; Majaliwa et al., 2010). Smallholder farms adjacent to the park are impacted by crop raiding by park animals and subsequent crop losses are fairly common, though it mainly impacts those within 1 km of the boundary (Hartter, 2010). The main regional cash crops are banana (Musa spp.), tea (Camellia sinensis L.), coffee (Coffea arabica and Coffea canephora), and maize, but smallholder farmers grow over 20 species of subsistence crops (Hartter and Southworth, 2009). Kabarole district is characterized as an area of “high agricultural potential” (de Jager et al., 2004), and ~84% of households engage in crop growing or livestock agriculture (UBOS, 2014). The district had the highest maize production in the western region (UBOS, 2010). Kabarole district lies within the Lake Albert Crescent zone, which has good to moderate soils (FAO, 2010). Related to the high population density, the western region has the smallest average landholdings at 0.8 ha compared to the national average of 1.1 ha (UBOS, 2010). The small landholdings and high population density are driven in part by limited land (Hartter and Southworth, 2009).
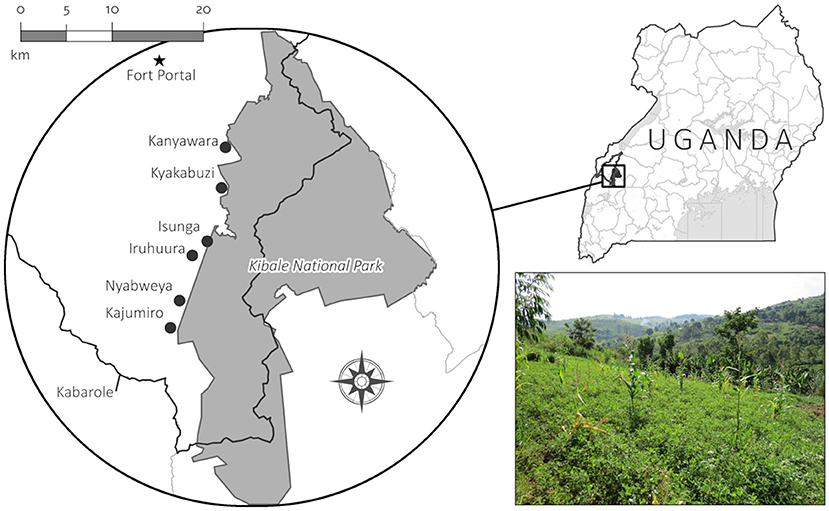
Figure 1. Map of study area, with a focus on areas surrounding six villages along the western border of Kibale National Park in Kabarole district, western Uganda. The inset photo shows a surveyed field in which groundnut is planted as a ground cover with maize sparsely interplanted within a hilly terrain, representative of the majority of fields surveyed for this study.
The study area covered the villages of Kanyawara, Kyakabuzi, Isunga, Iruhuura, Nyabweya, and Kajumiro, which fall along an ~22-km north-south transect along the edge of the KNP's western boundary (Figure 1). The study area is located between latitude 0.57–0.39°N and longitude 30.35–30.32°E and lies along an elevational gradient north to south from 1,550 to 1,100 m above sea level. The climate is tropical with an average daily temperature range of 15–23°C (Struhsaker, 1997). Rainfall in the region is bimodal with two rainy seasons separated by two dry seasons. The first dry season from early December to late February is followed by a rainy season occurring from approximately early March through mid-to-late May. A second dry season extends until early September followed by a rainy season from September through November (Hartter et al., 2012). Planting commences at the start of each rainy season, allowing for two growing seasons each year. Mean annual rainfall ranges from 1,100 to 1,700 mm with rainfall decreasing and temperature increasing when moving from north to south along the elevational gradient (Struhsaker, 1997). Soils are classified as eutrophic volcanic ash and ferralitic sandy clay loams. Study area soils were previously established to be inherently medium to highly fertile (Jameson, 1970).
Surveys and Data Collection
We conducted a survey and soil sampling within the six village areas in July 2015, coinciding with the final growing stage and harvest of groundnuts and maize (July harvest for groundnut and July to early August harvest for maize) planted at the start of the first rainy season (March–April planting for groundnut and February–March for maize). The study comprised 100 households that had actively been growing groundnuts (Kanyawara n = 9, Kyakabuzi n = 9, Isunga n = 21, Iruhuura n = 18, Nyabweya n = 16, Kajumiro n = 27). All households were located within ~1.6 km from the closest park boundary. Ugandan field assistants translated survey questions and responses from English into Rutooro and Rukiga, the respective languages of the resident Batooro and Bakiga ethnic groups. Households within each village were approached at random and asked if they grew groundnuts and were willing to participate in a survey. A two-part survey instrument was used: (1) a household socioeconomic and overall farm survey, which collected information on family size and composition, education level, ownership status and size of agricultural fields, livestock ownership, crop planting and harvesting dates, crop yields, crop use, income received for specific crops, perceived causes for declines in crop yields, land management decisions, and resource concerns; and (2) a survey of farmer management for the farmer-identified primary groundnut field, which provided information on any and all inputs and outputs into that field, field preparation, any steps taken or practices used to increase or maintain soil fertility, the field's cropping history for the two previous seasons (September 2014–February 2015 and March–August 2014), and detailed information on all crops grown in the field that season (March–August 2015), including planting and harvesting dates and methods, yields, or expected yields for that season, crop use (household, saved, or sale), and detailed residue management with reasons for specific practices. The residue management practices described by respondents were categorized into four main practices: (1) “remove” included residues removed from the field and transported to another location, (2) “burn” included residues burned within the field, (3) “spread” comprised residues that were kept on the field as mulch and spread on the field surface, and (4) “incorporate” constituted residues that were retained and buried into the soil. A simple relative wealth ranking of the study area farms was constructed by assigning a value to assets of homestead dwelling construction and livestock ownership (“yes” and “no” responses to whether they owned cattle, pigs, goats, chickens, other; Hockett and Richardson, 2018); values were summed and then categorized into “below average,” “average,” and “above average” based on the interquartile range.
The first part of the survey instrument (the household and whole farm survey) had previously been implemented with 14 households in July 2013 and eight households in June 2014. We used these prior responses in our analysis. In July 2015, these households participated in the second part of the survey instrument, the field survey.
The survey was administered at the homestead and at the field. The homestead and the corresponding field were marked as waypoints on a handheld Garmin GPS 62s unit. Respondents or a capable household member walked us around or clearly indicated the perimeter of the groundnut field, which was saved as a track to the GPS unit. The GPS data was retrieved from each unit and read into ArcGIS 10.4 software. A map of the household locations, field locations, and field perimeter tracks was created using ArcGIS. We calculated the area within the perimeter track to determine the size of each surveyed field and determined the Euclidean distance between the homestead and groundnut field. Fields within 50 m of the homestead were categorized as “homefields,” and fields further than 50 m were categorized as “outfields” (Zingore et al., 2007b).
Within each surveyed field, we used quadrats and total plant counts to measure plant density for groundnut and any other crop present. In each field, we measured the groundnut crop by counting the number of individual plants within four 50 × 50 cm quadrats; the location of each of the four quadrats was randomly determined along a diagonal field transect. The large variation in the density of crops interplanted in a groundnut field necessitated different measurement techniques according to intercrop species and/or field size. Intercrop plant density was measured either by counting plants within four 50 × 50 cm quadrats (beans) or three 3 × 3 m quadrats (all other crops except banana and coffee) or by counting the total number of plants in the field (coffee, banana, or intercrops in fields smaller than ~0.03 ha). If groundnut or an intercrop had already been fully harvested, we asked respondents to provide an estimate of the crop density by indicating the plant layout within a quadrat.
Soil Sampling and Analysis
In each groundnut field, three soil samples were taken at random to a depth of 15 cm using a 2-inch diameter soil probe and composited to represent each field. We used a set of KNP reference soils (n = 12) collected from uncultivated forest areas (Tiemann, unpublished data) proximal to each village in the study area as a baseline comparison to groundnut field SOC and N values. All fields were within 4 km of the proximal reference soils, and in prior exploratory analyses there were no significant differences in texture between field and park soils. It is likely soils were from the same parent material, and oxalate extractions of these soils show no significant differences in the iron and aluminum oxides between field and proximal park soils (Tiemann, unpublished data). Soils were air-dried in Uganda and shipped to Michigan State University (MSU) in East Lansing, MI, USA, for analysis.
Soil samples were passed through a 2 mm mesh sieve and 5 g placed into 20 ml scintillation vials to oven-dry at 60°C for 24 h. Oven-dried soils were ground on a roller mill and subsamples weighing ~20 mg were packed into tins to measure SOC and TN on an elemental analyzer (ECS 4010, Costech Analytical Technologies, Inc., Valencia, CA, USA).
Partial N Balance
To construct partial N balances, we first used the literature to determine a harvest index for groundnut of 0.23 (Phoomthaisong et al., 2003; Ncube et al., 2007; Kermah et al., 2018). Using the farmer reported yields and field size calculated from the collected GPS data, we calculated total groundnut aboveground productivity (ANPP, kg biomass ha−1). Next, we subtracted the harvested grain amount (grain, kg groundnuts ha−1) from the total biomass and converted this remaining aboveground residue biomass to biomass N (stover N, kg N ha−1) assuming groundnut residues contain 2% N (Kanmegne et al., 2006; Ncube et al., 2007). Assuming a grain N content of 3.8% N (Kanmegne et al., 2006; Ncube et al., 2007) we converted total grain yield to grain N (grain N, kg ha−1). We summed the stover N and grain N to determine the total crop N (crop N, kg N ha−1).
Because the proportion of N derived from BNF can be highly variable, we conducted a sensitivity analysis whereby we adjusted the proportion of total plant N from fixation, or what we term the BNF efficiency, to 30, 50, and 70%. In other words, we assumed 70, 50, or 30% of total plant N was mined from the soil rather than obtained via BNF. These proportions of N derived via BNF fall within the range of published groundnut N and fixation values for SSA (Kanmegne et al., 2006; Ncube et al., 2007; Ojiem et al., 2007; Ebanyat et al., 2010b; Nyemba and Dakora, 2010; Mokgehle et al., 2014; Franke et al., 2018; Kermah et al., 2018; Oteng-Frimpong and Dakora, 2018). We multiplied the crop N by the proportion of N derived via BNF to find the fixed N (fixed N, kg N ha−1). Our final calculations then accounted for farmer reported management of stover (proportion stover retained %). When farmers reported removing or burning all groundnut residue, we estimated that residues would provide 5% (incomplete burning or removal) of their total potential N benefit to soils, or if farmers provided an estimated percentage of residues remaining, this value was used instead. For groundnut residues that were reported incorporated into the soil or spread on the field surface, we estimated that 100% of the potential N benefit could be delivered. We calculated N mined from soil as:
Finally, the partial N balance is the difference between N mined from soil and removed in grain harvest vs. retained in stover as based on management and calculated as:
Additionally, to explore the total amount of N potentially conferred by a groundnut crop we calculated a second partial N balance assuming that 30% of fixed N was present belowground (Unkovich et al., 2008). Including the 30% fixed N contribution from roots (fixed N/0.70; Kermah et al., 2018), the total N mined from soil was calculated as:
And the season N balance plus belowground contributions (Total season N balance, kg N ha−1 season−1) as:
We calculated the partial, single season, field-level N balance for 77 groundnut fields; out of the 100 fields, 12 were missing field area measurements because of an error with the handheld GPS, four were missing groundnut plant density measurements, and seven fields were excluded because the reported groundnut yield weights were extreme outliers (>3,000 kg ha−1, more than 1.5 times interquartile range).
Data Analysis
Descriptive statistics, Pearson's chi-square tests, Wilcoxon rank-sum test, and analysis of variance (ANOVA) were performed with STATA/IC 14.2 statistical software (StataCorp, College Station, TX).
Pearson's chi-square test of independence was used to measure the strength of relationships between the four residue management practices and socioeconomic factors, including ethnicity, village, gender of the household head, crop planner, land tenure, wealth ranking, distance from the field to the homestead, and factors related to a household's valuation of groundnut. The factors related to valuation of groundnut were derived from three different survey questions asking: “which crop do you sell the most of?;” “which crop do you make the most profit on?,” and “which crop is the best to plant if you want to improve crop yields/soil fertility?” Because data were not normally distributed, we applied the Wilcoxon rank sum test to test for differences between groundnut field soils and KNP reference soils (Corder and Foreman, 2009).
Groundnut field SOC and TN values were normalized by calculating the difference from proximal KNP baseline soils. We performed a one-way analysis of variance (ANOVA) on the normalized C and N values by groundnut residue management practice.
Results
Household and Farm-Level Characteristics
Household demographic and socioeconomic characteristics are presented in Table 1. Households ranged in size from 1 to 20 people with a mean of 6.3 members (sd = 3.30), with 61% of members under the age of 15. Most respondents identified as belonging to the Bakiga ethnic group (72%) and 25% identified as Batooro. Households were predominately designated as male-headed (74%). Despite this, 44% identified a woman as the crop planner, i.e., the person responsible for planning the planting and harvesting schedule (29% of male-headed households had a female crop planner), 26% identified a man, and 29% identified multiple planners.
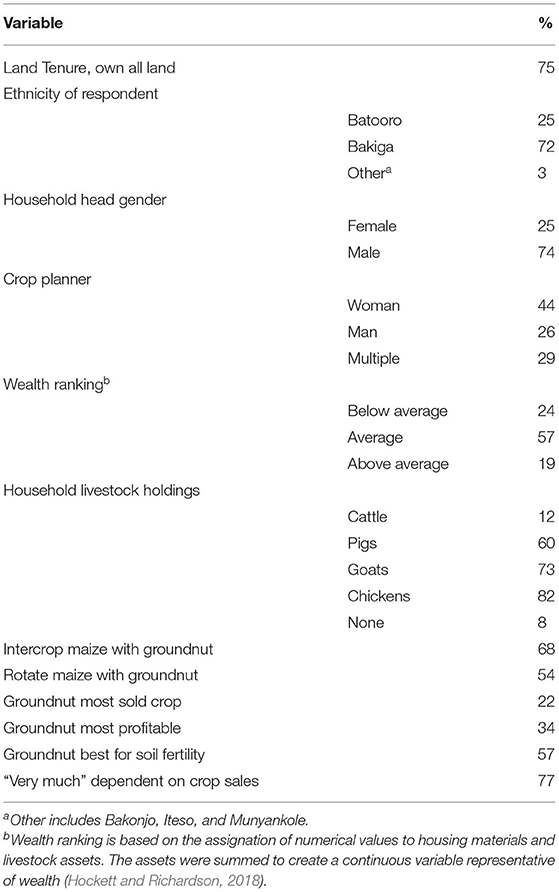
Table 1. Demographic and socioeconomic characteristics of the 100 surveyed groundnut-growing households along the western edge of Kibale National Park in western Uganda.
Land ownership was high with 75% of households owning all their land and 24% of households renting a portion of their land. Mean, farmer-estimated, household land use was 3.3 ha. The majority (57%) of households had similar, average wealth, while 24% were below average and 19% were above average. The above average wealth ranking includes households that owned cattle (12%) in addition to other livestock and had a dwelling constructed of concrete (10%); average households owned goats, pigs, and/or chickens and had homes with mud-wattle construction and an iron-sheet roof; and below average households owned chickens or no livestock and had traditional thatch-roofed homes or homes with dirt floors.
In addition to groundnuts, households grew a large diversity of crops at the farm-level with, in order of frequency, maize, common bean, banana, cassava, potato, and sweet potato grown by over half the households (Table 2). Crop production at the farm-level was strongly characterized by intercropping (99%) and crop rotation (82%). Maize was often intercropped with groundnut (68%), and of the 70% of farms that reported practicing a set, planned crop rotation, 77% reported that they included groundnut in the rotation. The top three reasons for intercropping were limited land (45%), greater harvest (22%), and greater profit (16%). When asked which crop(s) were best to plant for improving soil fertility, 57% included groundnut, though 30% of respondents also listed at least one non-legume crop (sorghum, maize, potato, millet, rice, and bananas were also mentioned).
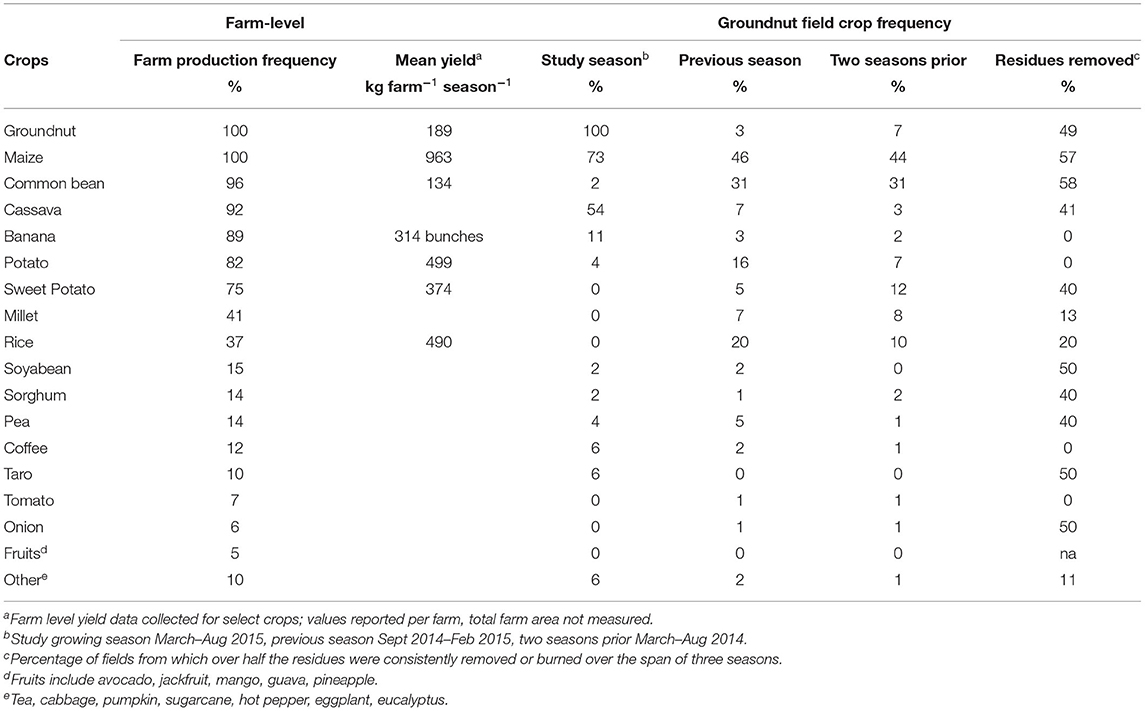
Table 2. Frequency of each crop grown at the household level with normal seasonal yields and the frequency of each crop grown in the groundnut field surveyed during the 2015 study season (crops other than groundnut indicate intercrops) and for the two prior growing seasons.
Maize was reported as the most sold crop (48%) with groundnut the second most sold crop (22% of households). Groundnut was reported to be the most profitable crop in 34% of households, followed closely by maize (31%), then rice (14%) and potato (10%). Most households (77%) categorized themselves as “very much” dependent on income from crop sales.
The large majority of farmers (93%) reported seeing year-to-year declines in crop yields with declines most often reported in maize (68%), followed by groundnut (48%), common bean (46%), and potato (27%); 6% of farmers reported declines in all crops. The reasons cited for declining crop yields included factors relating to soil fertility (soil fertility loss, old soils, poor soils, 43%), climate (heavy rains, drought, delayed rains, climate change, 34%), crop management (poor seeds, crop type, delayed planting, 5%), and a combination of soil, crop, and climate factors (11%); 7% said they did not know the reason.
Groundnut Field Characteristics: Production and Use
Groundnut fields ranged from 0.01 up to 0.58 ha with a mean area of 0.095 ha (Table 3). The distance between the surveyed groundnut fields and the homestead was at minimum 5 m and maximum of 1.7 km. The distance was <50 m for 40% of households thereby characterized as homefields and >50 m for 60%, which were classified as outfields.
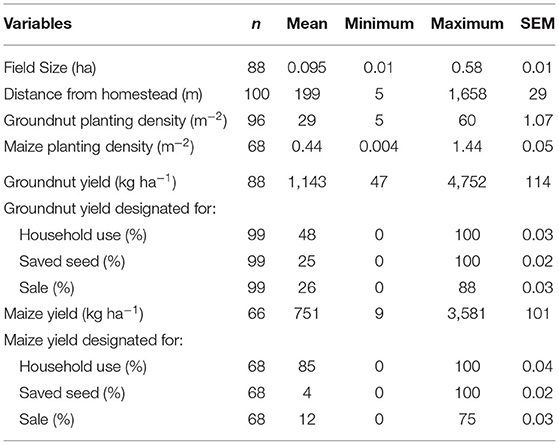
Table 3. Groundnut field characteristics and agronomic data across 100 smallholder farms along the western edge of Kibale National Park in western Uganda.
All fields were rainfed and were prepared and worked manually with a hand hoe. Only 14 fields had received any kind of external input; seven fields had manure added, four had herbicide applications, and application of chemical fertilizer, household waste, or residues from another source occurred in single fields. The remaining 86 fields did not receive any external inputs other than seeds or starts at planting. Weed biomass was retained on 96% of fields, and 93% of fields were weeded 1–2 times per season with the remainder weeded more frequently.
Of the three seasons surveyed, all fields were planted to groundnut during the 2015 survey season, but only 10% of households reported planting groundnut in the surveyed fields during the two prior seasons. This is contextualized by the fact that groundnut was included every third season in 83% of reported rotations. Approximately 52% of households grew maize and 50% grew common bean at least once during the two prior seasons. In 66% of surveyed fields, farmers reported using crop rotations with a variety of crops, including groundnut (79%), common bean (74%), maize (56%), potato (56%), rice (36%), cassava (21%), and sweet potato (21%; Table 2). Crop combinations, densities and rotations in these groundnut fields were highly variable. We found anywhere from one to five different intercrops planted with groundnuts in a variety of combinations, the most common of which was groundnut-maize-cassava in 21% of fields. On average, farmers had planted groundnut in the surveyed field for ~4 seasons total out of seven years in crop production.
For the surveyed groundnut field, household consumption accounted for around half of the groundnut harvest (48%), while 26% of the harvestwas sold and 25% was saved for seed. All households except for one intended a portion of the groundnut yield for household use, 80% of households saved part of the harvest for seed and 58% sold a portion of the groundnut harvest.
Bunch-type groundnut was found in all fields with varieties identified as local. The mean planting density for groundnut was 29 plants m−2 (Table 3), with 43% of households planting 30 or more groundnut plants m−2. In all surveyed fields, groundnut was planted as a ground cover over the entire field. Maize and cassava were interplanted at much lower densities and widely dispersed with respective mean planting densities of 0.44 (SEM = 0.62) and 0.18 (SEM = 0.04) m−2. Planting density for the other less common intercrops (Table 2) was also low, ranging from <0.01 to 0.63 plants m−2 with a mean of 0.136 m−2 (SEM = 0.03). Regression of groundnut yield on groundnut planting density indicated no linear relationship (R2 = 0.015) between the two; the exclusion of outliers did not increase the R2 above 0.1.
All farmers harvested groundnuts by pulling the entire plant out of the ground. Of the 100 groundnut-producing households, 49% removed or burned groundnut residues and 51% retained groundnut residues on fields, either incorporating or spreading the stover as a mulch (Table 2). Groundnut residues were removed from 19% of fields, burned in 30%, spread on the surface for 31%, and incorporated into the soil in 20%. Residues from maize, the most common intercrop, were removed to mulch bananas in 46% of fields, surface spread in 32%, incorporated in 11%, and burned in 11%. Approximately 41% of cassava residues were removed to use as firewood or animal feed, and 59% of residues were replanted as stem cuttings or remained in the fields.
Respondents provided a variety of reasons and explanations for residue management practices for groundnut and other crops planted in the field over the course of the three seasons (Figure 2). However, of all the different crop residues, only groundnut residues were described as having potentially negative impacts on the soil or crop yields (Figure 2). A total of 18 respondents said they burned or removed groundnut residues because the residues were either bad for the soil or caused infertility. Conversely, residues were described as adding fertility by 26 respondents who spread, incorporated, or removed residues to use as mulch in other fields. Residue decomposition was mentioned often with 10 respondents stating that they burned or removed groundnut residues because they did not easily decompose, whereas 11 respondents said they spread or incorporated residues so they would decompose.
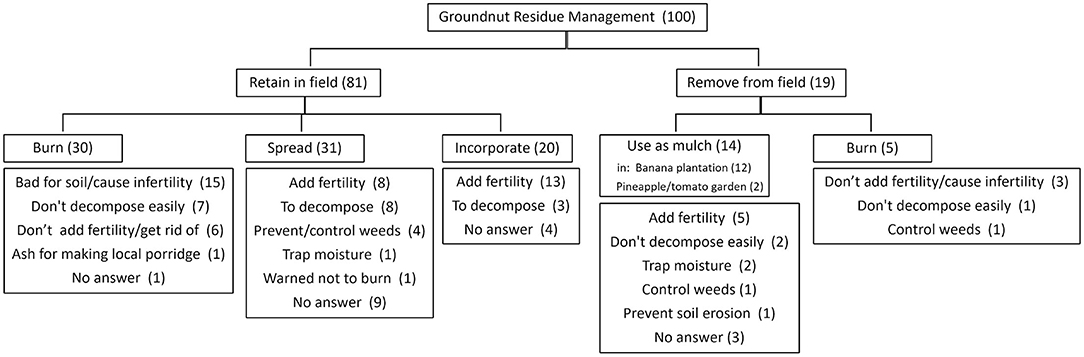
Figure 2. Tree diagram illustrating potential fates of groundnut residues on smallholder farms in Kabarole district, western Uganda, with farmer provided explanations for each of the five practices. Number of farmer responses for each management practice or explanation are in parentheses.
Soil Fertility
Study area soils are high in organic matter with relatively high SOC and TN values and low C:N (Table 4). In comparison to uncultivated reference soils from KNP that represent total potential soil nutrient stocks, the cultivated groundnut field soils contained 24% less total SOC, 44% less TN, and had a 35% wider C:N ratio. In two of the villages, Kyakabuzi and Isunga, mean SOC values were higher than proximal KNP reference soils, but TN values were lower and C:N ratios wider. An analysis of variance on the normalized groundnut field SOC and TN values found that groundnut residue practices did not significantly alter SOC (P = 0.695) or TN (P = 0.742) (Figure 3).
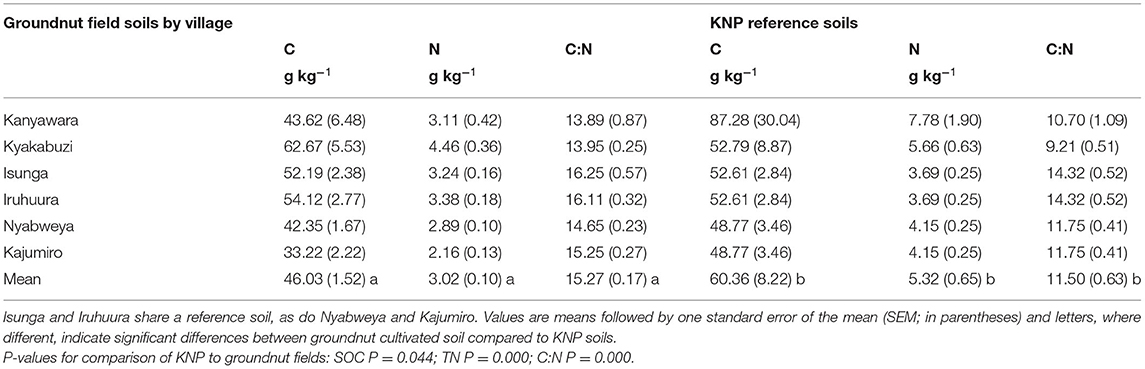
Table 4. SOC, TN, and soil C:N ratios in soils collected from groundnut fields and corresponding, proximal reference soils from Kibale National Park (KNP).
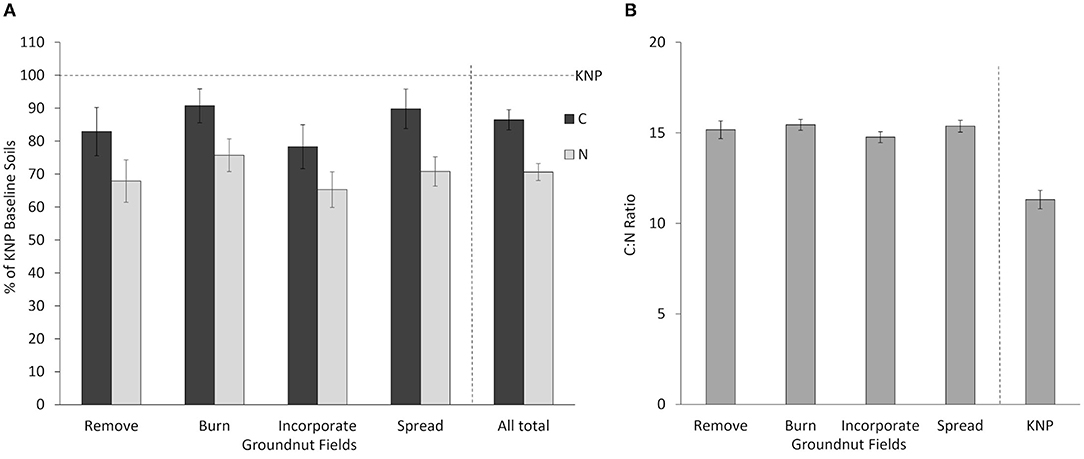
Figure 3. Mean groundnut field (n = 100) SOC and TN as a percentage of uncultivated Kibale National Park (KNP) reference soils (n = 12) (A) and groundnut field soil C:N ratios compared to the C:N ratio of KNP soils (B). Across all groundnut fields, SOC (P = 0.044) and TN (P = 0.000) were significantly reduced compared to KNP soils. By residue management practice, there were no significant differences between KNP and groundnut fields in SOC, TN or soil C:N ratios. Data are means ± one standard error.
Groundnut Field N Balance
The partial N balance scenarios showed there were N benefits at 50 and 70% BNF efficiency but only if residues were retained, i.e., spread or incorporated, in which case, mean BNF efficiency benefits ranged from ~8 up to 27 kg N ha−1 (Figure 4). Removal and burning of groundnut residues resulted in N loss at all levels of BNF efficiency with the greatest losses of 76 and 60 kg N ha−1, respectively, at 30% BNF efficiency (Figure 4). Although belowground biomass estimates for groundnut are not well-characterized, using values reported in the literature, and assuming 30% N fixed was allocated to belowground productivity, at the highest level of BNF efficiency (70%) root N could balance N lost through residue removal (Supplementary Figure 1).
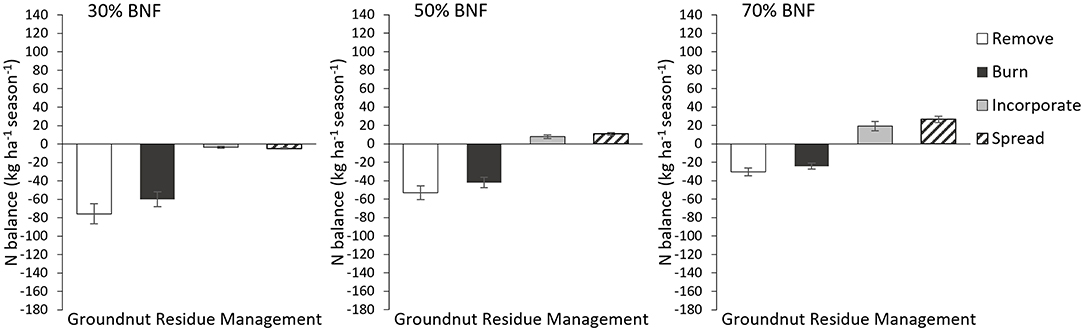
Figure 4. Potential single-season N balances for groundnut fields (n = 77) surveyed in 2015 in Kabarole district, western Uganda. N balances account for N removal through grain harvest calculated at three BNF efficiencies, i.e., percentage groundnut plant N from BNF relative to total plant N demands, and grouped by residue management practice. Data are means ± one standard error.
Determinants of Groundnut Residue Management
Household socioeconomic characteristics were not strongly related to groundnut residue management practices, but variables related to valuation of groundnut were. Pearson's chi-square measures of association did not find significant relationships between groundnut residue management and the ethnicity of respondent, gender of the household head, crop planner, land tenure, wealth ranking, distance from the field to the homestead, or if groundnut was the most sold crop (Table 5). There was a significant relationship between the removal of groundnut residues and village with more respondents than expected removing residues in Kanyawara, and fewer than expected in the remaining villages, except for Isunga (P < 0.05).
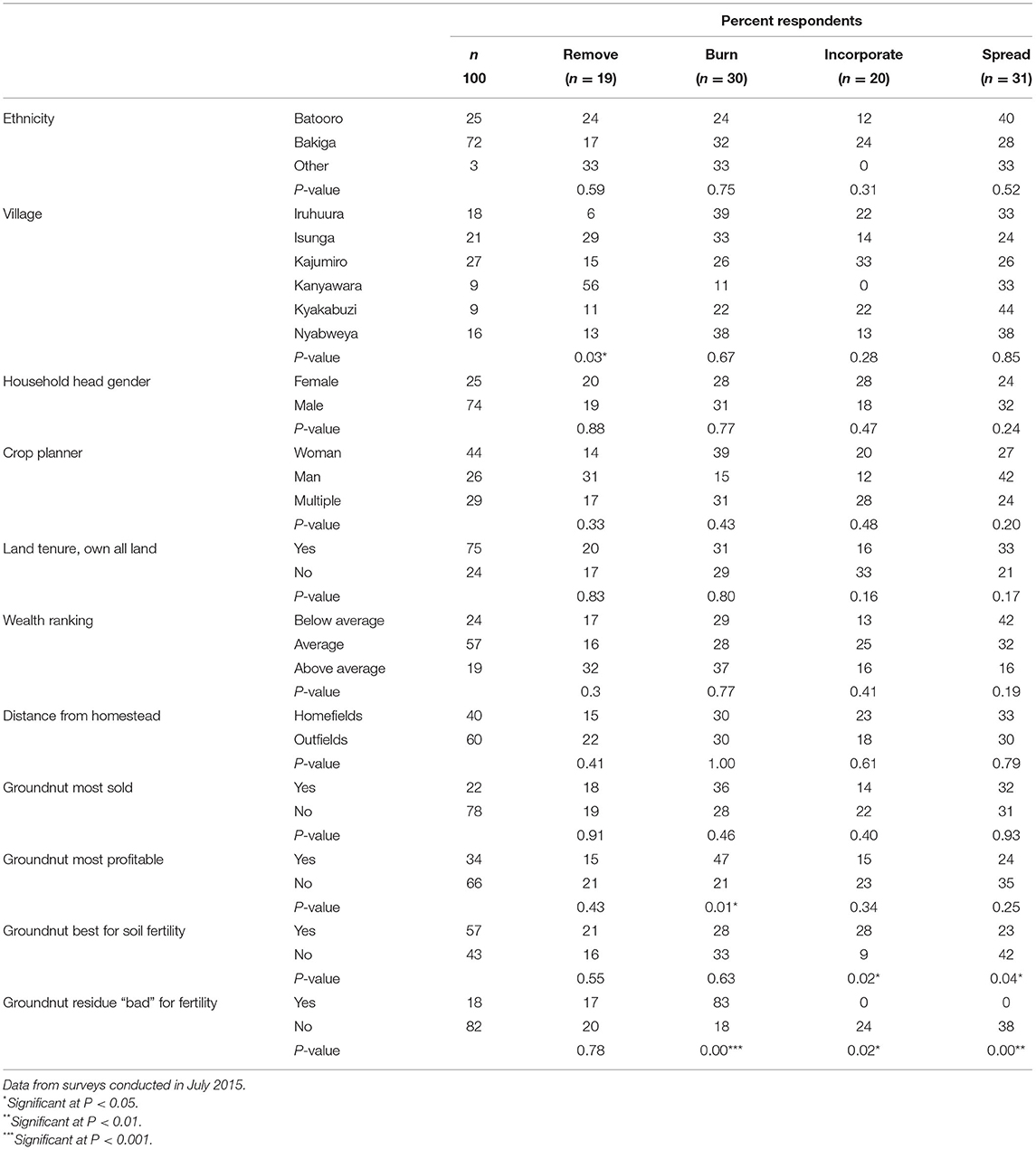
Table 5. Relationships between demographic and socioeconomic characteristics, farmer preferences, and groundnut residue management practices in smallholder farm fields along the western border of Kibale National Park (n = 100).
Households that considered groundnut as one of the best crops for improving soil fertility were significantly associated with residue incorporation and residue spread (P < 0.05) (Table 5). Households that designated groundnut as the most profitable crop were significantly associated with burning (Table 5). Finally, farmers who perceived groundnut residues as “bad” for soil or crop fertility were significantly associated with burning (P < 0.0001) and were not associated with spreading (P < 0.01) or incorporating residues (P < 0.05).
Discussion
In this study we took an agroecological approach, integrating biophysical, social, and economic data, to determine the extent and drivers of SOC and TN relative to groundnut management within smallholder farm fields in western Uganda. We documented the smallholder household, farm and groundnut field characteristics in the western region, an agroecosystem that is not well-profiled in the literature. We found that SOC and especially TN have been depleted relative to uncultivated soils. Despite groundnut appearing to be the most promising source of N for these fields, contrary to our hypothesis there were no discernible significant differences by groundnut residue management practice on SOC, TN, or C:N. It appears that groundnut residues have not had a large impact on soil C and N, which could be due to: residue application methods (timing, location, quantity, etc.); limited impact of aboveground residues compared to belowground contributions throughout the season; cropping system heterogeneity, including a complex variety of intercropped and rotated crops and planting densities. Estimated partial N balances supported our hypothesis that groundnut residues could deliver positive N balances at the field-scale. Residues could make up for grain N losses and deliver considerable N in these low input fields at close to 70% BNF. Importantly, only half of surveyed farmers retained groundnut stover in their fields, and removal or burning of residues resulted in N losses at all levels of BNF. Residue management practices were not clearly linked to socioeconomic factors related to gender and wealth, but rather highly driven by perception and valuation of groundnut residues as either good or bad for soil fertility or crop yields. We identify important knowledge gaps with respect to groundnut management, residue management and SOM or N benefits from legumes in SSA, as well as the importance of including information about residue management and variety selection to maximize BNF efficiency when legumes are recommended as a component of ecological nutrient management.
Current Soil Fertility
On average, the cultivated groundnut field soils are degraded compared to the uncultivated KNP reference soils. However, the mean difference in SOC (24%) is less than the reported C decline in other studies comparing tropical forest soils to cultivated fields (Tiessen et al., 1994; Moebius-Clune et al., 2011). Time of conversion from forest to field is unknown and likely variable for these fields as pockets of remnant forest outside the park boundary were cleared for agriculture over a broad time frame. However, it is likely that most fields were cleared by the 1970s when large waves of migrants settled in the region (Ryan and Hartter, 2012). A global meta-analysis examining SOC stocks after land use change found that conversion from native forest to crop resulted in a decline of ~50% in the top 30 cm (Guo and Gifford, 2002). A chronosequence in a region of Kenya with similar bimodal precipitation found that the degree of soil degradation in cultivated fields vs. primary forest was highly influenced by soil parent material (Moebius-Clune et al., 2011). The andic soils in our study area have relatively young overlays or rift volcanics that exhibit inherently high levels of fertility, renewed through mineral weathering, and characterized by amorphous mineral colloids with large active surfaces to which organic matter readily binds (Young, 1976). The soils have low bulk density, high water holding capacity, and good drainage, making them optimal for plant growth (Shoji et al., 1993). These properties have likely buffered the soils against degradation and C loss in the surveyed fields.
Farmer field management may also have contributed to maintaining or even recouping SOC lost due to forest conversion as farmers intercrop and/or rotate a large diversity of crops (Table 2). According to a review of SOC change after adoption of different management practices in tropical croplands, the strongest predictors of C change were quantity of C inputs, experiment duration, and management practices; soil, and climate variables did not have an effect (Fujisaki et al., 2018). The review determined that the management practice that resulted in the highest SOC was diversified crop rotation. In the current study, farmers practiced diversified crop rotation, but high rates of crop residue removal (Table 2) diminished the quantity of organic matter inputs. Removal of groundnut stover, relatively high in N content, not only removes important organic matter from the system but also a prime N source. The wider C:N ratio found in groundnut fields compared to uncultivated KNP soils is indicative of high N demands that are not being met by organic matter inputs. Instead, competition for N would tend to be high, which may result in microbial N mining of extant SOM (Craine et al., 2007). The addition of high-quality groundnut residues could provide N and help to narrow the C:N ratio of SOM in farmer fields.
Impact of Groundnuts on SOC and TN
The potential N contribution from groundnut stover if efficiently recycled to soil could increase N availability and thus boost yields and biomass of following crops, creating a positive cycle for C and N additions to the soil (Figure 4). However, we did not detect evidence of positive benefits of groundnut residue retention to SOM (Figure 3). SOC, TN, and C:N ratios did not differ significantly from uncultivated KNP soils across groundnut fields based on groundnut residue management practices. While these results are somewhat surprising, there are several potential explanations. First, higher biomass and yields of other intercrops or rotated crops with subsequent removal of their residues may reduce or cancel out potential benefits of groundnuts to the soil. For example, we found that 57% of farmers consistently removed maize residues, either through removal to the banana plantation as mulch or through burning (Table 2). For other commonly planted crops like common bean, cassava, and sweet potato, residue removal was also high at 58, 40, and 37%, respectively (Table 2). Without residual biomass retention from maize, common bean, and other crops within these fields, potential for SOM gains from groundnut stover are severely limited.
Second, groundnut residues alone may not be enough to influence SOM and TN, though they may positively impact crop fertility. The N inputs from groundnut would be expected to be relatively short-lived in the soil with residue N being mineralized and then immobilized by microbes and/or taken up by plants. The N provided by groundnut residue has the potential to stimulate productivity of intercropped or rotated crops such that residue inputs are increased with positive impacts on SOM and TN. Approximately 43% of farmers reported rotating groundnut every third season (i.e., every other year, similar to legume-maize rotations in tropical systems with unimodal precipitation) on the surveyed groundnut field. However, in this case, the N inputs from groundnut stover retention vs. non-retention were possibly not great enough, frequent enough, or available at the necessary time or place to significantly impact productivity and residue inputs from other crops.
Finally, the lack of a detectable effect of retention vs. removal of groundnut residues is also surprising given that in the study area, groundnut was planted at a density higher than the official recommendation by Uganda's National Agricultural Research Organization (NARO) of 15 plants m−2 for unirrigated production and closer to the recommended 30 plants m−2 for irrigated fields (Okello et al., 2013). Also, all farmers planted groundnut over the whole field rather than the recommended spacing of 30–45 cm rows (Okello et al., 2013); broad field coverage has many possible advantages including reduced soil erosion and weed competition. “Square spacing,” or the equal spacing of groundnut plants over the growing area, has been shown to have positive benefits and to maximize both total plant biomass and groundnut yield (Jaaffar and Gardner, 1988; Gardner and Auma, 1989). Results in the literature are mixed regarding groundnut plant population density and its effect on grain yield and stover production, groundnut variety, and growth habit (bunch vs. runner). Environmental conditions are critical to the density at which yields and biomass are maximized; maximum density values ranged from 20 to 50 plants m−2 (Bell et al., 1987; Bell and Wright, 1998; Tarimo and Blarney, 1999). Aboveground biomass has been shown to increase with increasing plant density, while pod yield has been shown to be less responsive and to decline at densities >25 plants m−2 (Bell et al., 1987; Tarimo and Blarney, 1999). Documentation of on-farm (vs. researcher-managed) groundnut planting density and spacing is scant in the literature (e.g., Nyemba and Dakora, 2010), and our study documents relatively high plant densities with yields equivalent to on-farm trial yields in similar agroecological zones in western Kenya where somewhat lower planting densities were employed (Ojiem et al., 2007). If the high planting density equated to a large volume of aboveground biomass with correspondingly moderate-to-low grain yield as suggested by previous studies (Bell et al., 1987; Tarimo and Blarney, 1999), the potential net C and N input should be sizable, yet we saw no evidence of this potential benefit in SOC and TN.
Groundnut Residue Management Practices
If aboveground residues are retained on fields, farmers can maximize the full N benefits from groundnut BNF but, in the current study, we found that 49% of farmers did not retain groundnut residues, although almost two thirds of farmers were using residues as some sort of soil amendment across their farm (Figure 2). The assumption that legumes like groundnuts can improve soil fertility, increase crop yields, and produce high-protein, more nutrient dense crops is largely based on best management practices. Studies that specifically address smallholder farmer management of groundnut residues are rare in the literature, and the existing research often does not represent smallholder contexts well. Several studies in Thailand present what may be considered an optimal potential N credit from groundnut residues as the groundnut crop was seeded, fertilized, and managed according to recommended best practices (McDonagh et al., 1993; Toomsan et al., 1995, 2000; Phoomthaisong et al., 2003; Srichantawong et al., 2005). In these studies, groundnut residues were chopped to 10 cm lengths, which would greatly impact rates of decomposition and timing of N availability to a subsequent crop, and is a labor-intensive step that the majority of smallholder farmers are unlikely to take (McDonagh et al., 1993; Toomsan et al., 1995, 2000; Phoomthaisong et al., 2003; Srichantawong et al., 2005). While groundnut residues retained on fields could contribute N to a following crop, another potential hurdle is the timing of N release from residues, and the N demand by a following crop is difficult to predict and synchronize (Robertson et al., 1997). Two studies that looked at the time gap between the planting of the next crop and the post-harvest surface-application or incorporation of groundnut residues found no significant differences in N delivery from surface-applied vs. incorporated residues, although trends suggested higher residue N conservation in soil and efficiency of N recycling, as well as slightly higher yields, with incorporation (Promsakha Na Sakonnakhon et al., 2005; Srichantawong et al., 2005). One study also tested removing and storing the groundnut residues before incorporating them just prior to maize planting and found that this significantly boosted maize yield compared to immediate post-harvest residue incorporation, surface application, and removal; however, the authors concluded that the storage facility and labor requirements made this practice difficult for smallholder farmers to implement (Promsakha Na Sakonnakhon et al., 2005). These studies emphasize the value of keeping residues in the system irrespective of application method. Overall, there is a lack of studies on groundnut residue contributions to soil N and none seem to fully replicate resource-limited, smallholder farmer management practices.
Factors Driving Groundnut Residue Management Practices
In the study area, groundnut residue management practices appear to be driven by perceptions and valuation of groundnut stover. Respondents gave various explanations for groundnut residue management decisions, and these decisions seem to be largely based on the perception of groundnut residue fertility or utility, and human values and behaviors that are part of the social context (Figure 2). Most farmers explained that they incorporated or spread groundnut residues in the field or as mulch in the banana plantation because residues added fertility. Bananas are the main staple food crop, and the transfer of residues to the banana plantation to boost yields through the benefits of added fertility, trapped soil moisture or weed prevention, makes sense in these resource-limited agroecosystems. On the contrary, most farmers who burned residues in the field, or removed residues and burned them elsewhere, perceived groundnut residues as “bad” for the soil, causing soil infertility or not benefiting soil fertility (Figure 2). Respondents were often not able to explain their reason for believing groundnut stover was “bad,” but several farmers mentioned burning had been recommended in the past as means to eradicate disease and/or pests (e.g., rats), which are noted concerns with residue retention (Erenstein, 2002). The basis for the negative perception of groundnut residue within the study area warrants further investigation.
We found no strong relationships between groundnut residue management practices and social and economic factors that have previously been shown in the literature to be drivers of farmer management decisions in SSA (e.g., gender of the household head, crop planner, ethnic group, land ownership, wealth rank, field distance from the homestead). Perhaps, in this region of Uganda, these socioeconomic drivers are less important than those related to farmer perception of groundnut fertility or there are other factors related to farmer resources that are more important determinants of residue management practices (Table 5). A commonly identified tradeoff in the literature is the use of residues as livestock feed (Tittonell et al., 2015; Valbuena et al., 2015), but livestock holdings are low in the study area and no household indicated that groundnut residues were used to feed livestock; only sweet potato and cassava residues were distinguished as animal feed. Respondents who listed groundnut as their most profitable crop were more likely to burn the residues, which is a relationship that requires further exploration as it could be linked to various different drivers, such as time and labor availability, residue biomass amount, and management at farm-scale.
In order to make effective recommendations and to enhance adoption of beneficial practices it is important for any extension or agricultural development agency working within the region to know and understand drivers of management practice. This knowledge is necessary for devising and implementing local or regional policy, for example residue burning regulations.
Groundnut Residue Management Impacts on Soil N Balance
The groundnut field N balances were calculated using estimated N input from groundnut residues minus the N exported by groundnut grain while also factoring in residue management practices (i.e., spreading, incorporating, removing, or burning). The N input from fully-retained, i.e., incorporated or spread, groundnut residues could provide a substantial N credit at 70% BNF (Figure 4). The maximum N benefit from groundnut residues at 70% BNF is ~27 kg N ha−1 season−1, which is considerable in a no-to-low-input system. Giller and Cadisch (1995) estimated that to offset N losses in SSA, a legume crop needed to fix an average of 30 kg N ha−1 year−1, and with retained residues and belowground inputs groundnut could likely achieve this at close to 70% BNF efficiency (Supplementary Figure 1). However, there are a number of factors that combine to determine the N provisioning potential of groundnut stover, most of which have been inadequately researched, including: stover quantities and N concentrations under different climates and soil types; roots and rhizodeposition; BNF efficiencies across varieties and environmental conditions and intercrop arrangements; and management of residues (e.g., timing of addition, spreading vs. burying, etc.).
We performed a sensitivity analysis to examine a span of BNF efficiencies, not only to reflect the fact that BNF can fluctuate by variety and season-to-season (Mokgehle et al., 2014; Oteng-Frimpong and Dakora, 2019), but also because there is no precedent for groundnut BNF on soils with such high TN, where legume nodulation and BNF may be suppressed by large pools of available soil N (Giller and Cadisch, 1995). Studies in SSA have contrasting conclusions about the impact of soil N on BNF efficiencies, for example, one determined that high endogenous levels of soil N led to lower N fixation (Mokgehle et al., 2014), while another found that BNF was lower in low fertility vs. high fertility fields and BNF generally decreased with soil fertility levels (Ojiem et al., 2007). Intercropping legumes with cereals and other non-N fixing crops, as was the case in 88% of the groundnut fields we surveyed, can lead to reductions in soil N concentrations that then promote greater nodulation and BNF (Giller and Cadisch, 1995), but only if BNF is not limited by other nutrients.
Residue management is also critical to achieving an N benefit and if groundnut stover were removed, groundnut would be a heavy miner of soil N at <70% BNF efficiency (Figure 4). Even our simplified N balances confirm the importance of management in combination with BNF as field balances were only positive when residues were spread and incorporated (Figure 4). We chose to use the maximum of 100% N delivery for residues that were spread or incorporated to help illustrate the full potential N benefit of groundnut stover. If there was loss of retained residue (e.g., through livestock grazing or pests) or loss of retained residue N (e.g., through ammonia volatilization, denitrification, or nitrate leaching), which is likely, then nutrient balances would be reduced (Figure 4). Further, we chose to use a minimum of 5% N delivery for residues that were burned or removed, though it is probable that the total combustion of residues is inconsistent, as is the proportion of residue material left in the field. Importantly, the field-scale partial N balance establishes reference points for farmers, extension agents, and policymakers when estimating a potential N credit from groundnut residues within the context of management practices.
While the partial N balances do not account for the N loss from the diverse number of additional crops grown at the field-scale and other potential inputs and outputs, the estimated N inputs suggest that full retention of residues at the higher levels of BNF could reduce or counter additional N exports over a full cropping cycle. The average seasonal maize yield for western Uganda is ~2,600 kg ha−1 season−1 (UBOS, 2010), which would remove about 41 kg N ha−1 season−1 at a maize grain N concentration of 1.57% (Kaizzi et al., 2012). This output could be balanced by groundnut N inputs if BNF efficiency was close to or >70% and residues were retained (Figure 4; Supplementary Figure 1). Notably, when a second set of N balances were calculated considering the belowground N inputs from unrecovered roots and nodules and rhizodeposition contributions, which have been estimated to account for 30 to 50% of plant N (Giller et al., 1997; Unkovich and Pate, 2000; Herridge et al., 2008), we see that there is potential for even greater N input from groundnut (Supplementary Figure 1). Additionally, there could be N contributions from the other legume crops grown on these farms (i.e., common bean and pea), but relative to groundnut their N contributions are likely far less as common bean has been shown to be poor at BNF and pea is not widely grown (Herridge et al., 2008; Franke et al., 2018).
With fields planted to groundnut receiving the only substantial N inputs (through BNF), our results suggest that overall N balances at the farm-level would be negative considering the mean yields and diversity of other crops, and the lack of other N inputs (fertilizer or manure) to these crops (Table 2). Negative farm-level N balances would be in line with previously published nutrient balances in Uganda which found negative or near zero N, P, and K values at all levels of scale across all regions of the country, with few exceptions (Stoorvogel and Smaling, 1990; Wortmann and Kaizzi, 1998; Briggs and Twomlow, 2002; Bekunda and Manzi, 2003; de Jager et al., 2004; Sheldrick and Lingard, 2004; Nkonya et al., 2005; Ebanyat et al., 2010a; Mubiru et al., 2011; Lederer et al., 2015). More positive farm-level N balances might be achieved through an increase in groundnut production, or inclusion of non-harvested legumes such as those in cover crops or agroforestry species.
Increasing groundnut grown through more frequent rotations or land planted to groundnut could contribute to greater N inputs, but after household groundnut needs are met, there would need to be market opportunities to support greater production. Planting groundnut more frequently could lead to greater incidences of pest and disease, and advice from Uganda's NARO is to plant groundnut every three years or more to prevent such buildups (Okello et al., 2014). Households are already planting groundnut more frequently than this recommendation as ~43% reported rotating groundnut every third season on the surveyed field. Farmers in the study area are land-limited as evidenced by the small field sizes, and 45% of farmers who intercropped said they did so because of limited land. Thus, expanding the area cropped to groundnut may not be feasible or meet household needs.
Groundnut yield increases are challenged by the fact that an estimated 80% of the groundnut seed is saved, may be of lower quality, and it is overwhelmingly from traditional, low-yielding varieties (Okello et al., 2010). However, there is a tradeoff between grain production and soil inputs because yield increases can lead to larger amounts of N exported in grain resulting in lower soil N balances, thus, in this regard, low-yielding varieties, and those that offer both moderate yields and abundant biomass, could be considered advantageous for soil fertility (Ojiem et al., 2007; Kermah et al., 2018). Crop yields and BNF are affected by climate and water availability, and within the study region, rainfall has been shown to be highly variable in its timing, and, while total rainfall has not changed significantly, the intra-seasonal distribution has (Hartter et al., 2012). Climate change and changes to the timing and distribution of rainfall heighten the uncertainty for all crop production, including groundnut, which in turn heightens the impact and importance of farmer management decisions and practices that can affect factors like soil moisture retention and nutrient availability.
Conclusions
Grain legumes like groundnut have the potential to contribute N-rich residues to boost SOC and TN and increase N available to other crops. Here, we estimated groundnut residue N delivery within minimal input, smallholder fields and found that there was a potentially substantial net N input at 70% BNF efficiency, but only if farmers retained residues on fields. However, after normalizing surveyed field soils using uncultivated reference soils from KNP, we did not find any evidence of differences in SOC or TN from fields where groundnut residues were retained vs. fields where they were removed. The high soil fertility inherent to the study area and the prevalence of diverse crop rotations, intercropping, and residue practices may have masked effects of residue management.
While our study focused on groundnut contributions to soil health, sustainability, and agricultural productivity, groundnut also provides essential nutrition and generates crucial income to support the health and well-being of smallholder farmers. Approximately half of the groundnut harvest in the surveyed fields was intended for household consumption, while ~25% of the harvest was sold. Nutrient-rich groundnuts increase food security and diversify diets by providing protein, micronutrients, and phytochemicals to resource-poor households. Sale of groundnuts can generate high profits and bring in important income that may also contribute to food security. Though groundnut was second to maize in terms of household crop sales, households identified groundnut as the most profitable crop (34%) with maize a close second (31%). The majority of households (77%) categorized themselves as “very much” dependent on income from crop sales, thus groundnut generates vital income for these smallholder households.
Our study presents a valuable snapshot of a growing season, but multi-year studies are needed to fully assess the impact of legumes such as intercropped or rotated groundnut, as well as similar ecological nutrient management practices, on SOC and TN. There is a dearth of long-term studies examining the effects of grain legume rotations on SOM and soil properties in SSA. We recognize that there is a need to move beyond examination of legumes' potential benefits to soil and to institute trials to document changes over the long-term, including trials that collaborate with farmers to compare practices side-by-side on the same soils. Studies have mainly focused on changes in SOC and TN, as we did here, but we recommend quantification and analysis of more management sensitive, early indicators of SOC and N change, such as C and N within aggregates, and dissolved organic C and N. We used literature values to construct the N balance, and, in the process, we found few studies that examined groundnut plant total N, plant N partitioning, and N derived via BNF in farmer fields. We also did not find studies examining high plant population density and spacing effects on groundnut N uptake and BNF. Similarly, few studies examine the effects of farmer practices like removal, burning, incorporation, and surface spreading of groundnut residues on N retention in soil and N availability. Future research is needed to address these knowledge gaps, to gain a better understanding of groundnut's impact on soil fertility and to elucidate residue management practices that maximize short-term and long-term benefits to soils, human nutrition, and food systems within these smallholder contexts.
Data Availability Statement
The raw data supporting the conclusions of this article will be made available by the authors, without undue reservation.
Ethics Statement
The studies involving human participants were reviewed and approved by Michigan State University IRB. Written informed consent for participation was not required for this study in accordance with the national legislation and the institutional requirements.
Author Contributions
AW and LT devised the study and conducted the field work. AW performed the lab work, analyzed the data, and wrote the manuscript. LT contributed to interpretation of results and commented on the manuscript. All authors reviewed and approved the final manuscript.
Funding
Funding for this project was provided by the NSF SEES Fellowship Program (Award #1215750).
Conflict of Interest
The authors declare that the research was conducted in the absence of any commercial or financial relationships that could be construed as a potential conflict of interest.
Publisher's Note
All claims expressed in this article are solely those of the authors and do not necessarily represent those of their affiliated organizations, or those of the publisher, the editors and the reviewers. Any product that may be evaluated in this article, or claim that may be made by its manufacturer, is not guaranteed or endorsed by the publisher.
Acknowledgments
We acknowledge and thank the farmers who participated in this study. This work would not have been possible without the field assistants in Uganda: Emmanuel Tugume, Kato Micheal, Kiiza Kiizito, Richard Ategeka, Edith Mbabazi, and King Solomon. Thanks to Dr. Stephanie Grand, the Makerere University Biological Field Station, and the Uganda Wildlife Authority.
Supplementary Material
The Supplementary Material for this article can be found online at: https://www.frontiersin.org/articles/10.3389/fsufs.2021.691786/full#supplementary-material
Supplementary Figure 1. Potential single-season N balances for groundnut fields (n = 77) surveyed in 2015 in Kabarole district, western Uganda, accounting for belowground N input and N removal through grain harvest calculated at three BNF efficiencies, i.e., percentage groundnut plant N from BNF relative to total plant N demands, and grouped by residue management practice. Data are means ± one standard error.
References
Akibode, S., and Maredia, M. (2012). Global and Regional Trends in Production, Trade and Consumption of Food Legume Crops. East Lansing, MI: Department of Agricultural, Food and Resource Economics, Michigan State University. doi: 10.22004/ag.econ.136293
Barrett, C. B., and Bevis, L. E. M. (2015). The self-reinforcing feedback between low soil fertility and chronic poverty. Nat. Geosci. 8, 907–912. doi: 10.1038/ngeo2591
Bekunda, M., and Manzi, G. (2003). Use of the partial nutrient budget as an indicator of nutrient depletion in the highlands of southwestern Uganda. Nutr. Cycl. Agroecosystems 67, 187–195. doi: 10.1023/A:1025509400226
Bell, M. J., Muchow, R. C., and Wilson, G. L. (1987). The effect of plant population on peanuts (Arachis hypogaea) in a monsoonal tropical environment. F. Crop. Res. 17, 91–107. doi: 10.1016/0378-4290(87)90085-2
Bell, M. J., and Wright, G. C. (1998). Groundnut growth and development in contrasting environments 1. Growth and plant density responses. Exp. Agric. 34, 99–112. doi: 10.1017/S001447979800101X
Briggs, L., and Twomlow, S. J. (2002). Organic material flows within a smallholder highland farming system of South West Uganda. Agric. Ecosyst. Environ. 89, 191–212. doi: 10.1016/S0167-8809(01)00164-5
Cadisch, G., Hairiah, K., and Giller, K. E. (2000). Applicability of the natural N-15 abundance technique to measure N-2 fixation in Arachis hypogaea grown on an Ultisol. Netherlands J. Agric. Sci. 48, 31–45. doi: 10.1016/S1573-5214(00)80003-2
Chapman, C. A., and Lambert, J. E. (2000). Habitat alteration and the conservation of African primates: Case study of Kibale National Park, Uganda. Am. J. Primatol. 50, 169–185. doi: 10.1002/(SICI)1098-2345(200003)50:3<169::AID-AJP1>3.0.CO;2-P
Corder, G. W., and Foreman, D. I. (2009). Nonparametic Statistics for Non-statisticians. Hoboken, NJ: John Wiley & Sons, Inc. doi: 10.1002/9781118165881
Craine, J. M., Morrow, C., and Fierer, N. (2007). Microbial nitrogen limitation increases decomposition. Ecology 88, 2105–2113. doi: 10.1890/06-1847.1
Dakora, F. D., and Keya, S. O. (1997). Contribution of legume nitrogen fixation to sustainable agriculture in sub-saharan Africa. Soil Biol. Biochem. doi: 10.1016/S0038-0717(96)00225-8
de Jager, A., Onduru, D., and Walaga, C. (2004). Facilitated learning in soil fertility management: assessing potentials of low-external-input technologies in east African farming systems. Agric. Syst. 79, 205–223. doi: 10.1016/S0308-521X(03)00071-4
Drinkwater, L. E., Schipanski, M., Snapp, S., and Jackson, L. E. (2017). “Ecologically based nutrient management,” in Agricultural Systems: Agroecology and Rural Innovation for Development: Second Edition, eds S. Snapp, B. Pound (London: Elsevier Inc), 203–257. doi: 10.1016/B978-0-12-802070-8.00007-4
Ebanyat, P., de Ridder, N., de Jager, A., Delve, R. J., Bekunda, M. a., and Giller, K. E. (2010a). Drivers of land use change and household determinants of sustainability in smallholder farming systems of Eastern Uganda. Popul. Environ. 31, 474–506. doi: 10.1007/s11111-010-0104-2
Ebanyat, P., de Ridder, N., de Jager, A., Delve, R. J., Bekunda, M. A., and Giller, K. E. (2010b). Impacts of heterogeneity in soil fertility on legume-finger millet productivity, farmers' targeting and economic benefits. Nutr. Cycl. Agroecosystems 87, 209–231. doi: 10.1007/s10705-009-9329-9
Erenstein, O. (2002). Crop residue mulching in tropical and semi-tropical countries: An evaluation of residue availability and other technological implications. Soil Tillage Res. 67, 115–133. doi: 10.1016/S0167-1987(02)00062-4
Erenstein, O., Gérard, B., and Tittonell, P. (2015). Biomass use trade-offs in cereal cropping systems in the developing world: overview. Agric. Syst. 134, 1–5. doi: 10.1016/j.agsy.2014.12.001
FAO (2010). Crop Calendar – An Information Tool for Seed Security, Uganda Agro-ecological Zones. Available online at: http://www.fao.org/agriculture/seed/cropcalendar/aezones.do?isocode=UGA (accessed September 11, 2019).
Foyer, C. H., Lam, H. M., Nguyen, H. T., Siddique, K. H. M., Varshney, R. K., Colmer, T. D., et al. (2016). Neglecting legumes has compromised human health and sustainable food production. Nat. Plants 2, 1–10. doi: 10.1038/NPLANTS.2016.112
Franke, A. C., van den Brand, G. J., Vanlauwe, B., and Giller, K. E. (2018). Sustainable intensification through rotations with grain legumes in Sub- Saharan Africa: a review. Agric. Ecosyst. Environ. 261, 172–185. doi: 10.1016/j.agee.2017.09.029
Fujisaki, K., Chevallier, T., Chapuis-Lardy, L., Albrecht, A., Razafimbelo, T., Masse, D., et al. (2018). Soil carbon stock changes in tropical croplands are mainly driven by carbon inputs: a synthesis. Agric. Ecosyst. Environ. 259, 147–158. doi: 10.1016/j.agee.2017.12.008
Gardner, F. P., and Auma, E. O. (1989). Canopy structure, light interception, and yield and market quality of peanut genotypes as influenced by planting pattern and planting date. F. Crop. Res. 20, 13–29. doi: 10.1016/0378-4290(89)90020-8
Giller, K. E., and Cadisch, G. (1995). Future benefits from biological nitrogen fixation: An ecological approach to agriculture. Plant Soil 174, 255–277. doi: 10.1007/BF00032251
Giller, K. E., Cadisch, G., Ehaliotis, C., Adams, E., Sakala, W. D., and Mafongoya, P. L. (1997). “Building soil nitrogen capital in Africa,” in Replenishing Soil Fertility in Africa, eds R. J. Buresh, P. A. Sanchez, and F. Calhoun (Madison, WI: SSSA and ASA), 151–192. doi: 10.2136/sssaspecpub51.c7
Guo, L. B., and Gifford, R. M. (2002). Soil carbon stocks and land use change: a meta analysis. Glob. Change Biol. 8, 345–360. doi: 10.1046/j.1354-1013.2002.00486.x
Haileslassie, A., Priess, J. a., Veldkamp, E., and Lesschen, J. P. (2007). Nutrient flows and balances at the field and farm scale: Exploring effects of land-use strategies and access to resources. Agric. Syst. 94, 459–470. doi: 10.1016/j.agsy.2006.11.013
Hartter, J. (2010). Resource Use and Ecosystem Services in a Forest Park Landscape. Soc. Nat. Resour. 23, 207–223. doi: 10.1080/08941920903360372
Hartter, J., and Southworth, J. (2009). Dwindling resources and fragmentation of landscapes around parks: Wetlands and forest patches around Kibale National Park, Uganda. Landsc. Ecol. 24, 643–656. doi: 10.1007/s10980-009-9339-7
Hartter, J., Stampone, M. D., Ryan, S. J., Kirner, K., Chapman, C. A., and Goldman, A. (2012). Patterns and perceptions of climate change in a biodiversity conservation hotspot. PLoS ONE 7:e0032408. doi: 10.1371/journal.pone.0032408
Herridge, D. F., Peoples, M. B., and Boddey, R. M. (2008). Global inputs of biological nitrogen fixation in agricultural systems. Plant Soil 311, 1–18. doi: 10.1007/s11104-008-9668-3
Hockett, M., and Richardson, R. B. (2018). Examining the drivers of agricultural experimentation among smallholder farmers in Malawi. Exp. Agric. 54, 45–65. doi: 10.1017/S0014479716000673
Jaaffar, Z., and Gardner, F. P. (1988). Canopy development, yield, and market quality in peanut as affected by genotype and planting pattern. Crop Sci. 28, 299–305. doi: 10.2135/cropsci1988.0011183x002800020024x
Kaizzi, K. C., Byalebeka, J., Semalulu, O., Alou, I., Zimwanguyizza, W., Nansamba, A., et al. (2012). Maize response to fertilizer and nitrogen use efficiency in Uganda. Agron. J. 104, 73–82 doi: 10.2134/agronj2011.0181
Kanmegne, J., Smaling, E. M. A., Brussaard, L., Gansop-Kouomegne, A., and Boukong, A. (2006). Nutrient flows in smallholder production systems in the humid forest zone of southern Cameroon. Nutr. Cycl. Agroecosystems 76, 233–248. doi: 10.1007/s10705-005-8312-3
Kassie, M., Teklewold, H., Jaleta, M., Marenya, P., and Erenstein, O. (2015). Understanding the adoption of a portfolio of sustainable intensification practices in eastern and southern Africa. Land Use Policy. 42, 400–411. doi: 10.1016/j.landusepol.2014.08.016
Kermah, M., Franke, A. C., Adjei-nsiah, S., Ahiabor, B. D. K., Abaidoo, R. C., and Giller, K. E. (2018). N2 - fixation and N contribution by grain legumes under different soil fertility status and cropping systems in the Guinea savanna of northern Ghana. Agric. Ecosyst. Environ. 261, 201–210. doi: 10.1016/j.agee.2017.08.028
Kirner, K. (2010). Agricultural Variation and Change Among Batoro and Bakiga. Gainesville, FL: University of Florida.
Lederer, J., Karungi, J., and Ogwang, F. (2015). The potential of wastes to improve nutrient levels in agricultural soils: a material flow analysis case study from Busia District, Uganda. Agric. Ecosyst. Environ. 207, 26–39. doi: 10.1016/j.agee.2015.03.024
Lepp, A., and Holland, S. (2006). A comparison of attitudes toward state-led conservation and community-based conservation in the village of Bigodi, Uganda. Soc. Nat. Resour. 19, 609–623. doi: 10.1080/08941920600742377
Majaliwa, J. G. M., Twongyirwe, R., Nyenje, R., Oluka, M., Ongom, B., Sirike, J., et al. (2010). The effect of land cover change on soil properties around Kibale National Park in South Western Uganda. Appl. Environ. Soil Sci. 2010, 1–7. doi: 10.1155/2010/185689
McDonagh, J. F., Toomsan, B., Limpinuntana, V., and Giller, K. E. (1993). Estimates of the residual nitrogen benefit of groundnut to maize in Northeast Thailand. Plant Soil 267–277. doi: 10.1007/BF00012532
Messina, M. J. (1999). Legumes and soybeans: overview of their nutritional profiles and health effects. Am. J. Clin. Nutr. 70:439. doi: 10.1093/ajcn/70.3.439s
Moebius-Clune, B. N., van Es, H. M., Idowu, O. J., Schindelbeck, R. R., Kimetu, J. M., Ngoze, S., et al. (2011). Long-term soil quality degradation along a cultivation chronosequence in western Kenya. Agric. Ecosyst. Environ. 141, 86–99. doi: 10.1016/j.agee.2011.02.018
Mokgehle, S. N., Dakora, F. D., and Mathews, C. (2014). Variation in N2 fixation and N contribution by 25 groundnut (Arachis hypogaea L.) varieties grown in different agro-ecologies, measured using 15N natural abundance. Agric. Ecosyst. Environ. 195, 161–172. doi: 10.1016/j.agee.2014.05.014
Mubiru, L. S., Romney, D., Halberg, N., and Tenywa, J. S. (2011). Development of nutrient management strategies based on mapping of nitrogen flows and balances in dairy production systems in Uganda. Livest. Sci. 137, 116–123. doi: 10.1016/j.livsci.2010.10.009
Mugisa, I. O., Karungi, J., Akello, B., Ochwo Ssemakula, M. K. N., Biruma, M., Okello, D. K., et al. (2015). Assessing the effect of farmers practices on the severity of groundnut rosette virus disease in Uganda. African J. Agric. Res. 10, 995–1003. doi: 10.5897/ajar2014.9171
Nandwa, S. M., and Bekunda, M. A. (1998). Research on nutrient flows and balances in East and Southern Africa: state-of-the-art. Agric. Ecosyst. Environ. 71, 5–18. doi: 10.1016/S0167-8809(98)00128-5
Naughton-Treves, L. (1997). Farming the forest edge: vulnerable places and people around Kibale National Park, Uganda. Geogr. Rev. 87, 27–46. doi: 10.2307/215656
Ncube, B., Twomlow, S. J., Van Wijk, M. T., Dimes, J. P., and Giller, K. E. (2007). Productivity and residual benefits of grain legumes to sorghum under semi-arid conditions in southwestern Zimbabwe. Plant Soil 299, 1–15. doi: 10.1007/s11104-007-9330-5
Nijuki, J. M., Mapila, M. T., Zingore, S., and Delve, R. (2008). The dynamics of social capital in influencing use of soil management options in the Chinyanja Triangle of Southern Africa. Ecol. Soc. 13, 1–16. doi: 10.5751/es-02539-130209
Nkonya, E., Kaizzi, C., and Pender, J. (2005). Determinants of nutrient balances in a maize farming system in eastern Uganda. Agric. Syst. 85, 155–182. doi: 10.1016/j.agsy.2004.04.004
Nyemba, R. C., and Dakora, F. D. (2010). Evaluating N2 fixation by food grain legumes in farmers' fields in three agro-ecological zones of Zambia, using 15N natural abundance. Biol. Fertil. Soils 46, 461–470. doi: 10.1007/s00374-010-0451-2
Ojiem, J. O., Franke, A. C., Vanlauwe, B., de Ridder, N., and Giller, K. E. (2014). Benefits of legume-maize rotations: assessing the impact of diversity on the productivity of smallholders in Western Kenya. F. Crop. Res. 168, 75–85. doi: 10.1016/j.fcr.2014.08.004
Ojiem, J. O., Vanlauwe, B., de Ridder, N., and Giller, K. E. (2007). Niche-based assessment of contributions of legumes to the nitrogen economy of Western Kenya smallholder farms. Plant Soil 292, 119–135. doi: 10.1007/s11104-007-9207-7
Okello, D. K., Biruma, M., and Deom, C. M. (2010). Overview of groundnuts research in Uganda: past, present and future. African J. Biotechnol. 9, 6448–6459. doi: 10.5897/AJB09.013
Okello, D. K., Monyo, E., Deom, C. M., Ininda, J., and Oloka, H. K. (2013). Groundnut Production Guide for Uganda : Recommended Practices for Farmers. Entebbe: National Agricultural Research Organisation.
Okello, D. K., Okori, P., Puppala, N., Bravo-Ureta, B., Deom, C. M., Ininda, J., et al. (2014). Groundnut Seed Production Manual for Uganda. Entebbe: National Agricultural Research Organisation.
Omamo, S. W. (2003). Fertilizer trade and pricing in Uganda. Agrekon 42, 310–324. doi: 10.1080/03031853.2003.9523626
Oteng-Frimpong, R., and Dakora, F. D. (2018). Selecting elite groundnut (Arachis hypogaea L) genotypes for symbiotic N nutrition, water-use efficiency and pod yield at three field sites, using 15 N and 13 C natural abundance. Symbiosis 75, 229–243. doi: 10.1007/s13199-017-0524-1
Oteng-Frimpong, R., and Dakora, F. D. (2019). Multienvironment testing for trait stability and G × E interaction on N2 fixation, plant development, and water-use efficiency of 21 elite groundnut (Arachis hypogaea L.) genotypes in the Guinea Savanna. Front. Plant Sci. 10:1070. doi: 10.3389/fpls.2019.01070
Peoples, M. B., and Craswell, E. T. (1992). Biological nitrogen fixation: Investments, expectations and actual contributions to agriculture. Plant Soil 141, 13–39. doi: 10.1007/BF00011308
Phoomthaisong, J., Toomsan, B., Limpinuntana, V., Cadisch, G., and Patanothai, A. (2003). Attributes affecting residual benefits of N2-fixing mungbean and groundnut cultivars. Biol. Fertil. Soils. 39, 16–24. doi: 10.1007/s00374-003-0676-4
Place, F., and Otsuka, K. (2002). Land tenure systems and their impacts on agricultural investments and productivity in Uganda. J. Dev. Stud. 38, 105–128. doi: 10.1080/00220380412331322601
Promsakha Na Sakonnakhon, S., Toomsan, B., Cadisch, G., Baggs, E. M., Vityakon, P., Limpinuntana, V., et al. (2005). Dry season groundnut stover management practices determine nitrogen cycling efficiency and subsequent maize yields. Plant Soil 272, 183–199. doi: 10.1007/s11104-004-4780-5
Robertson, G. P., Klingensmith, K. M., Klug, M. J., Paul, E. A., Crum, J. R., and Ellis, B. G. (1997). Soil resources, microbial activity, and primary production across an agricultural ecosystem. Ecol. Appl. 7, 158–170. doi: 10.1890/1051-0761(1997)0070158:SRMAAP2.0.CO;2
Ryan, S. J., and Hartter, J. (2012). Beyond ecological success of corridors: intergrating land use history and demographic change to provide a whole landscape perspective. Ecol.Restor. 30, 320–328. doi: 10.3368/er.30.4.320
Sanchez, P. A., Shepherd, K. D., Soule, M. J., Place, F. M., Buresh, R. J., Izac, A.-M. N., et al. (1997). “Soil fertility replenishment in Africa: an investment in natural resource capital,” in Replenishing Soil Fertility in Africa, eds R. J. Buresh, P. A. Sanchez, and F. Calhoun (Madison, WI: Soil Science Society of America; American Society of Agronomy), 1–46. doi: 10.2136/sssaspecpub51.c1
Sheldrick, W. F., and Lingard, J. (2004). The use of nutrient audits to determine nutrient balances in Africa. Food Policy 29, 61–98. doi: 10.1016/j.foodpol.2004.01.004
Shoji, S., Nanzyo, M., and Dahlgren, R. A. (1993). Volcanic ash soils: genesis, properties and utilization. Volcanic ash soils genesis. Prop. Util. 21, 1–288. doi: 10.1016/0016-7061(95)90034-9
Snapp, S., Rahmanian, M., and Batello, C. (2018). Pulse Crops for Sustainable Farms in Sub-Saharan Africa. Rome: FAO. doi: 10.18356/6795bfaf-en
Snapp, S. S., Mafongoya, P. L., and Waddington, S. (1998). Organic matter technologies for integrated nutrient management in smallholder cropping systems of southern Africa. Agric. Ecosyst. Environ. 71, 185–200. doi: 10.1016/S0167-8809(98)00140-6
Srichantawong, M., Toomsan, B., Limpinuntana, V., Cadisch, G., Jogloy, S., and Patanothai, A. (2005). Evaluation of groundnut stover management strategies in a legume-rice rotation. Biol. Agric. Hortic. 23, 29–44. doi: 10.1080/01448765.2005.9755306
Stoorvogel, J. J., and Smaling, E. M. A. (1990). Assessment of Soil Nutrient Depletion in Sub-Saharan Africa: 1983-2000. Wageningen: Winand Staring Centre.
Struhsaker, T. T. (1997). Ecology of an African Rain Forest: Logging in Kibale and the Conflict Between Conservation and Exploitation. Gainesville, FL: University Press of Florida.
Tanellari, E., Kostandini, G., Bonabana-Wabbi, J., and Murray, A. (2014). Gender impacts on adoption of new technologies: the case of improved groundnut varieties in Uganda. African J. Agric. Resour. Econ. 9, 300–308. doi: 10.22004/ag.econ.197017
Tarimo, A. J. P., and Blarney, F. P. (1999). Effects of plant population density and cultivar on growth, yield and yield components in groundnut (Arachis hypogaea L.). South African J. Plant Soil 16, 74–78. doi: 10.1080/02571862.1999.10634850
Thierfelder, C., Chisui, J. L., Gama, M., Cheesman, S., Jere, Z. D., Trent Bunderson, W., et al. (2013). Maize-based conservation agriculture systems in Malawi: Long-term trends in productivity. F. Crop. Res. 142, 47–57. doi: 10.1016/j.fcr.2012.11.010
Tiessen, H., Cuevas, E., and Chacon, P. (1994). The role of soil organic matter in sustaining soil fertility. Nature 371, 783–785. doi: 10.1038/371783a0
Tittonell, P., Gérard, B., and Erenstein, O. (2015). Tradeoffs around crop residue biomass in smallholder crop-livestock systems – What 's next? Agric. Syst. 134, 119–128. doi: 10.1016/j.agsy.2015.02.003
Tittonell, P., Muriuki, A., Klapwijk, C. J., Shepherd, K. D., Coe, R., and Vanlauwe, B. (2013). Soil heterogeneity and soil fertility gradients in smallholder farms of the East African Highlands. Soil Sci. Soc. Am. J. 77, 525–538. doi: 10.2136/sssaj2012.0250
Tittonell, P., Vanlauwe, B., Leffelaar, P. A., Rowe, E. C., and Giller, K. E. (2005). Exploring diversity in soil fertility management of smallholder farms in western Kenya: I. Heterogeneity at region and farm scale. Agric. Ecosyst. Environ. 110, 149–165. doi: 10.1016/j.agee.2005.04.001
Toomsan, B., Cadisch, G., Srichantawong, M., Thongsodsaeng, C., Giller, K. E., and Limpinuntana, V. (2000). Biological N2 fixation and residual N benefit of pre-rice leguminous crops and green manures. Netherlands J. Agric. Sci. 48, 19–29. doi: 10.1016/S1573-5214(00)80002-0
Toomsan, B., McDonagh, J. F., Limpinuntana, V., and Giller, K. E. (1995). Nitrogen fixation by groundnut and soyabean and residual nitrogen benefits to rice in farmers' fields in Northeast Thailand. Plant Soil 175, 45–56. doi: 10.1007/BF02413009
Tully, K. L., Sullivan, C., Weil, R., and Sanchez, P. (2015a). The state of soil degradation in sub-Saharan Africa: baselines, trajectories, and solutions. Sustainability. 2015, 6523–6552. doi: 10.3390/su7066523
Tully, K. L., Wood, S. A., Almaraz, M., Neill, C., and Palm, C. (2015b). The effect of mineral and organic nutrient input on yields and nitrogen balances in western Kenya. Agric. Ecosyst. Environ. 214, 10–20. doi: 10.1016/j.agee.2015.08.006
UBOS (2010). UBOS Census of Agriculture 2008/2009 Volume IV: Crop Area and Production Report. Kampala: UBOS.
Unkovich, M., Herridge, D., Peoples, M., Cadisch, G., Boddey, B., Giller, K., et al. (2008). Measuring Plant-Associated Nitrogen Fixation in Agricultural Systems. Canberra: Australian Centre for International Agricultural Research, 132–188. doi: 10.1071/AR9940119
Unkovich, M. J., and Pate, J. S. (2000). An appraisal of recent field measurements of symbiotic N2 fixation by annual legumes. F. Crop Res. 65, 211–228. doi: 10.1016/S0378-4290(99)00088-X
Valbuena, D., Tui, S. H. K., Erenstein, O., Teufel, N., Duncan, A., Abdoulaye, T., et al. (2015). Identifying determinants, pressures and trade-offs of crop residue use in mixed smallholder farms in Sub-Saharan Africa and South Asia. Agric. Syst. 134, 107–118. doi: 10.1016/j.agsy.2014.05.013
Wani, S. P., Rupela, O. P., and Lee, K. K. (1995). Sustainable agriculture in the semi-arid tropics through biological nitrogen fixation in grain legumes. Plant Soil 174, 29–49. doi: 10.1007/BF00032240
World Bank (2016). Fertilizer Consumption (Kilograms Per Hectare of Arable Land). Available online at: https://data.worldbank.org/indicator/AG.CON.FERT.ZS (accessed February 5, 2017).
Wortmann, C. S., and Kaizzi, C. K. (1998). Nutrient balances and expected effects of alternative practices in farming systems of Uganda. Agric. Ecosyst. Environ. 71, 115–129. doi: 10.1016/S0167-8809(98)00135-2
Young, A. (1976). Tropical Soils and Soil Survey. Cambridge: Cambridge University Press. doi: 10.2307/2259206
Zingore, S., Murwira, H. K., Delve, R. J., and Giller, K. E. (2007a). Influence of nutrient management strategies on variability of soil fertility, crop yields and nutrient balances on smallholder farms in Zimbabwe. Agric. Ecosyst. Environ. 119, 112–126. doi: 10.1016/j.agee.2006.06.019
Keywords: nitrogen fixation, soil fertility, residue management, groundnut (Arachis hypogaea), smallholder farmers
Citation: Witcombe AM and Tiemann LK (2022) Potential Contribution of Groundnut Residues to Soil N and the Influence of Farmer Management in Western Uganda. Front. Sustain. Food Syst. 5:691786. doi: 10.3389/fsufs.2021.691786
Received: 07 April 2021; Accepted: 17 December 2021;
Published: 20 January 2022.
Edited by:
Steven J. Vanek, Colorado State University, United StatesReviewed by:
Liming Ye, Ghent University, BelgiumDurgesh Kumar Jaiswal, Savitribai Phule Pune University, India
Copyright © 2022 Witcombe and Tiemann. This is an open-access article distributed under the terms of the Creative Commons Attribution License (CC BY). The use, distribution or reproduction in other forums is permitted, provided the original author(s) and the copyright owner(s) are credited and that the original publication in this journal is cited, in accordance with accepted academic practice. No use, distribution or reproduction is permitted which does not comply with these terms.
*Correspondence: Alexia M. Witcombe, d2l0Y29tYmVAbXN1LmVkdQ==