- Robert W. Holley Center for Agriculture and Health, United States Department of Agriculture, Agricultural Research Service, Ithaca, NY, United States
Iron biofortification of the common bean (Phaseolus vulgaris) commenced in earnest ~18 years ago. Based on knowledge at the time, the biofortification approach for beans was simply to breed for increased Fe concentration based on 3 major assumptions: (1) The average bean Fe concentration is ~50 μg/g; (2) Higher Fe concentration results in more bioavailable Fe delivered for absorption; (3) Breeding for high Fe concentration is a trait that can be achieved through traditional breeding and is sustainable once a high Fe bean sample is released to farmers. Current research indicates that the assumptions of the high Fe breeding approach are not met in countries of East Africa, a major focus area of bean Fe biofortification. Thus, there is a need to redefine bean Fe biofortification. For assumption 1, recent research indicates that the average bean Fe concentration in East Africa is 71 μg/g, thus about 20 μg/g higher than the assumed value. For assumption 2, recent studies demonstrate that for beans higher Fe concentration does not always equate to more Fe absorption. Finally, for assumption 3, studies show a strong environment and genotype by environment effect on Fe concentration, thus making it difficult to develop and sustain high Fe concentrations. This paper provides an examination of the available evidence related to the above assumptions, and offers an alternative approach utilizing tools that focus on Fe bioavailability to redefine Fe biofortification of the common bean.
Introduction
Iron (Fe) deficiency anemia (IDA) is a global health issue affecting two billion people worldwide, especially women and children. The prevalence is highest in regions such as Africa, South Asia, and Latin America. Sub-Saharan Africa comprises 23.9% of global anemia cases, and in East Africa ~3 in 4 children under 5 years old suffer from anemia (Ngesa and Mwambi, 2014; Pasricha, 2014). In Rwanda, a country located in East Africa, 17% of women of child-bearing age and 38% of children are affected by IDA (Donahue et al., 2017), with the overall prevalence having increased from 17 to 19% from 2010 to 2015. In women of reproductive age, the prevalence was 18.9%, but this value varied depending on factors such as physical environment, socioeconomic status, and level of education (Habyarimana et al., 2018). South Asia constitutes 37.5% of global anemia cases, affecting more than 40% of women of reproductive age in this region (Pasricha, 2014; Petry et al., 2016a). In Central America, the prevalence of anemia reaches 33.9%; in South America 46.2%; and in the Caribbean 42.9%, with highest national rates found in children <11 months old (Hummel et al., 2020).
Biofortification is the nutritional enrichment of food crops via agricultural practices and plant breeding for higher micronutrient levels of Fe, Zn, and provitamin A. Since 2003, substantial research efforts, with about $192 million of beans produced, have been invested in Fe biofortification of the common bean (Phaseolus vulgaris) to bring more of this essential nutrient into the food system of targeted regions such as East Africa, South Asia, and Latin America (PABRA, 2017). In Africa, bean Fe biofortification has been proclaimed a “nutrition success story” with organizations such as HarvestPlus and Pan Africa Bean Research Alliance (PABRA) citing a human efficacy trial (Haas et al., 2016). Numerous testimonials and anecdotal evidence have also been published by these organizations (HarvestPlus, 2019).
The current approach to biofortification for beans is simply to breed for high Fe, a strategy that has been applied for ~18 years. This approach is based on three major assumptions: (1) the mean bean Fe concentration in target marketplaces is 50–55 μg/g; (2) a higher concentration of Fe in bean varieties correlates to greater Fe absorption when consumed; and (3) a high Fe concentration (target value of 94 μg/g) is a stable trait that can be achieved through traditional breeding methods. These assumptions were established based on analysis of a highly diverse “core collection” of beans established at the Center for Tropical Agriculture (CIAT) in Cali, Colombia in ~2,000 (Beebe et al., 2000; Islam et al., 2002; Beebe, 2020).
This collection showed an average bean Fe concentration of ~50–55 μg/g, and a range up to ~90 μg/g. Based on the observations of this collection, nutritionists calculated that a 40–44 μg/g increase would be necessary to provide meaningful nutritional benefit. This calculation factors in assumed values for estimated daily average iron requirements, intake, retention, a 7% estimated fractional bioavailability, and the observed potential for Fe concentration increase through breeding. Given the above, the breeding target for bean Fe biofortification was set to 90–94 ppm (Mulambu et al., 2017).
Over the past several years, a number of studies have been published suggesting that the 3 primary assumptions listed above are often not met (Tako et al., 2014; Cichy et al., 2015; Finkelstein et al., 2019). Most recently, a survey of marketplaces in East Africa demonstrated that multiple varieties released as biofortified were no different in terms of Fe concentration relative to common marketplace varieties (Glahn et al., 2020). Therefore, in this paper assumptions of the high Fe bean biofortification approach will be examined in light of recent studies and various evidence that supports and refutes such assumptions. In addition, evidence for a data driven approach that measures the amount of deliverable Fe from foods will be offered as the way forward to redefine bean Fe biofortification.
Examination of the Major Assumptions of Bean Iron Biofortification Via Breeding for High Fe
Assumption #1: The Average Bean Fe Concentration Is ~50 μg/g
As stated previously, this mean Fe concentration of 50 μg/g originates from the CIAT core collection of beans assembled back in 2000 (Beebe et al., 2000). This assumption also appears to have been extended to all beans in the food systems of targeted regions, even though the CIAT collection was likely assembled from seed grown in or around Cali, Colombia. Evidence of such assumption can be found in several publications (Haas et al., 2016; Andersson et al., 2017; Boy et al., 2017; Mulambu et al., 2017; Finkelstein et al., 2019; HarvestPlus, 2019).
A recent study (Glahn et al., 2020) indicates that the average bean Fe concentration of 50 μg/g does not apply to all regions. This study collected 76 marketplace samples from multiple countries of East Africa. In this collection, 18 samples were varieties released as “biofortified” samples, and 58 were “non-biofortified.” The average Fe concentration of non-biofortified varieties was 71 ± 9 μg/g (mean ± SD) ranging from 52 to 93 μg/g. The average Fe concentration of the biofortified varieties was 71± 11 μg/g with a range of 55–94 μg/g, essentially identical to non-biofortified samples. In addition to the marketplace samples, the investigators collected 154 genotypes from bean breeders that were known to be representative of the marketplace of the region. These samples were collected as that marketplace samples can sometimes be mixtures of varieties within a color class. Within this collection from breeders, the investigators had 35 biofortified and 119 non-biofortified varieties. The Fe concentration of the non-biofortified varieties was 66 ± 7 μg/g (range of 51–90 μg/g) which was significantly different (P < 0.0001) from the biofortified lines (73 ± 9 μg/g, range of 60–91 μg/g). However, it is important to note that the Fe concentration of the biofortified lines of the breeder collection were not different from the non-biofortified marketplace collection. A summary of these observations is shown in Figures 1, 2.
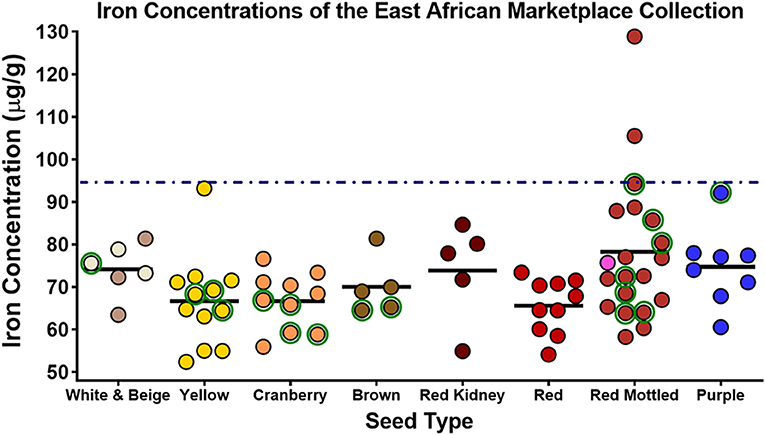
Figure 1. Iron concentrations of seed collected from marketplaces throughout countries of East Africa. Points with green circles indicate lines released as “biofortified.” Dashed line indicates the target value for biofortified beans. Adapted from Glahn et al. (2020).
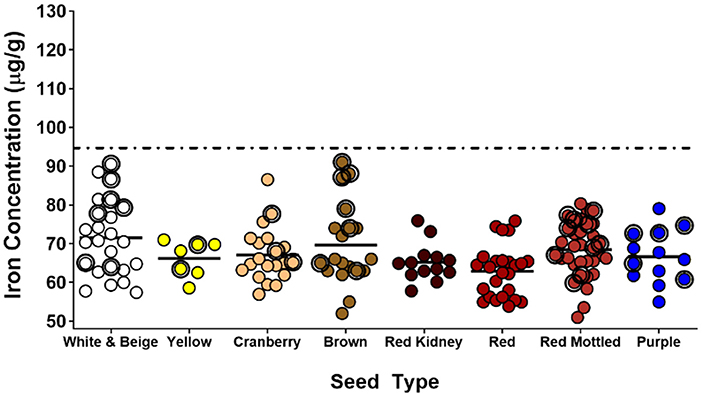
Figure 2. Iron concentrations of seed collected from bean breeders of East Africa. The breeders submitted samples that were deemed representative of varieties present in marketplaces throughout countries of East Africa. Points with green circles indicate lines released as “biofortified.” Dashed line indicates the target value for biofortified beans. Adapted from Glahn et al. (2020).
The conclusions of this study are profound. First, it clearly showed that the average Fe concentration of 50 μg/g observed in the CIAT core collection was not representative of the countries of East Africa. Second, the biofortified varieties in this collection were similar in Fe concentration to the non-biofortified lines, indicating that the biofortified varieties were providing no additional dietary Fe. These observations also suggest that the organizations releasing the biofortified lines did not assess the Fe concentration of the marketplace in East Africa, and thus assumed that the 50 μg/g average Fe of the CIAT core collection similar for other regions (Andersson et al., 2017; Mulambu et al., 2017).
In a review of the literature, others studies also suggest that the 50 μg/g average cannot be applied globally. For example, mean Fe concentrations of beans have been found to be 68 μg/g in Central Africa (Blair et al., 2010) and 69 μg/g in Brazil and Latin America (Pereira et al., 2014). Collections from within the United States were observed to have Fe concentrations of 60–70 μg/g (Cichy et al., 2015; McClean et al., 2017; Wiesinger et al., 2018).
In summary, the above studies indicate that the first assumption of the high Fe approach, i.e., an average bean Fe concentration of 50 μg/g, cannot be applied globally, and certainly is not valid in East Africa, one of the key regions of the world for bean biofortification.
There appear to be no studies that specifically address soil and environmental factors that influence Fe concentration in beans as related to biofortification. Rather there are a multitude of studies on Fe uptake and homeostasis in plants demonstrating that Fe content can be influenced by genotype, weather, soil nutrient composition, uptake by the roots, translocation, and redistribution within the plant, followed by remobilization and mineral loading into the seeds (Kobayashi and Nishizawa, 2012; Dey et al., 2020). In general, it appears safe to say that assessment of genotype by environment and phenotype stability for seed Fe content across different agro-ecologies has not been adequately examined in common bean. Stability of this trait appears essential for this assumption to be valid.
Assumption #2: Higher Fe Concentration Results in More Bioavailable Fe Delivered for Absorption
This assumption depends on fractional bioavailability of a high Fe bean remaining sufficiently similar to that of a normal Fe variety. Programs using the high Fe approach are assuming that fractional Fe absorption is not decreased significantly when Fe concentration is increased. However, higher levels of Fe concentration have been associated with subsequently higher levels of Fe uptake inhibitors, and the effects of such higher levels of inhibitors have been demonstrated via comparison of bean varieties from the same harvests in two major human trials, with simultaneous studies conducted in vitro and in animals (Tako et al., 2014, 2015; Haas et al., 2016; Finkelstein et al., 2019). Measurement of seed coat polyphenol content and phytate concentration indicated that these inhibitors partially negated the benefit of higher Fe in one study (Tako et al., 2015; Haas et al., 2016) and almost completely negated the benefit in the other (Tako et al., 2014; Finkelstein et al., 2019). Another study exists where in vitro assessment coupled with an animal feeding trial compared a pair of carioca beans (Dias et al., 2018). In this study, a normal Fe (64 μg/g) was compared to a high Fe (85 μg/g) bean both alone and in the context of a Brazilian diet designed to optimize Fe bioavailability from the bean. The results showed low bioavailability from the beans alone, with no difference. However, in the context of the diet, the high Fe bean appeared to deliver slightly more Fe. This study coupled with the observations from the parallel studies conducted with the human efficacy studies mentioned above clearly show the importance of monitoring Fe bioavailability from beans both alone and in the context of a diet in a biofortification program. A protocol or plan to monitor Fe bioavailability does not appear to be in place by any organization or program producing high Fe beans. Such monitoring would have to be conducted via an in vitro approach as in vivo testing would be impractical due to cost and lack of throughput capability (Glahn et al., 1998).
Current knowledge demonstrates that bioavailability of Fe in beans is a function of 3 major factors: the seed coat polyphenolic profile, phytic acid, and the cotyledon cell wall. In general, phytic acid levels are consistently in high molar excess relative to Fe, usually at a 10:1 (phytic acid: Fe) or higher ratio, thus the inhibitory effect is near or at maximal. Similar to other organic acids such as citrate, the relative molar excess of phytic acid inhibits Fe uptake as it limits exchange of Fe with the intestinal uptake transporter (Glahn et al., 2002). The cotyledon cell wall is a major factor as 70–90% of the Fe in beans is housed within the cotyledon cells, and the cell wall is not broken down by cooking, mastication, or the digestive enzymes of the intestinal tract (Glahn et al., 2016). Polyphenolic compounds in the seed coat represent the third major factor that can influence Fe bioavailability. For most market classes of beans, the seed coat polyphenols are predominantly inhibitory in regard to Fe bioavailability; although recent studies have shown that varieties within the yellow bean market class exhibit seed coat polyphenolic profiles that enhance Fe bioavailability (Wiesinger et al., 2018, 2019).
Presoaking and cooking is an important factor to consider in regards to Fe bioavailability from beans. For polyphenolic compounds, the effects of cooking and presoaking on polyphenol-Fe binding have not been thoroughly studied. In vitro studies of Fe bioavailability indicate that presoaking and cooking has little effect on the polyphenolic effects on Fe bioavailability (Glahn et al., 2017; Wiesinger et al., 2020, 2021). For phytic acid, a study of Canadian pulse crops demonstrated that soaking and cooking had no effect on reducing phytic acid levels in beans (Shi et al., 2018). Cooking and soaking certainly have an effect on other “anti-nutrients” in the common bean, but aside from loss of Fe in cooking water these steps appear to have minimal effect on the net inhibitory role of phytate and the net inhibitory or promoting role of polyphenols on Fe bioavailability (Wiesinger et al., 2018, 2019, 2020, 2021).
It is important to note that most estimates of bean Fe bioavailability in biofortification have been done via extrinsic isotopic labeling. Extrinsic isotopic labeling is a method of Fe absorption measurement that has been used for over 50 years and is generally preferred because intrinsic labeling is time-consuming and expensive. With extrinsic labeling, the stable isotopic extrinsic label, usually 57Fe or 58Fe is simply added to the food in a known quantity, and extrinsic and intrinsic Fe are assumed to mix and equilibrate fully during the gastric phase of digestion. Both Fe sources are assumed to be absorbed by the upper small intestine in similar ways (Szabo et al., 1975). However, in a recent study on the extrinsic labeling of staple food crops, including beans, maize, and lentils, measurement of Fe solubility of the extrinsic label often did not match with the intrinsic Fe (Glahn et al., 2015). These observations clearly indicate that equilibration of the extrinsic with the intrinsic did not occur (Jin et al., 2008; Glahn et al., 2015). Therefore, the current bioavailability estimates that have guided the high Fe approach may be flawed as equilibration of extrinsic labels used in the guiding absorption studies was not assessed (Petry et al., 2012, 2014; Haas et al., 2016).
In summary, monitoring of Fe bioavailability from biofortified bean varieties has not been done in any distribution program. There appears to be only a few studies where bean Fe bioavailability was measured, and those were conducted via extrinsic labeling with no effort to determine if the equilibration assumption of extrinsic labeling was met. Moreover, analysis of extrinsic labeling in multiple staple food crops, including beans, indicates that equilibration of the extrinsic label with intrinsic Fe is often not achieved. Thus, there is little to no evidence that supports the assumption that higher bean Fe concentration equates to more Fe absorption. In fact, studies of this assumption demonstrate that bean Fe bioavailability is the primary factor that equates to enhanced Fe delivery.
Assumption #3: Breeding for High Fe Concentration Is a Trait That Can Be Achieved Through Traditional Breeding and Is Sustainable Once a High Fe Bean Sample Is Released to Farmers
As stated previously, The target value of 90–94 μg/g was selected based on the premise that the average bean Fe concentration is 50–55 μg/g, and that the 40 μg/g differential of biofortified vs. “normal” was calculated to be necessary to have nutritional benefit, with a fractional Fe bioavailability of the bean estimated at 7% (Mulambu et al., 2017; Beebe, 2020). While sufficient variability has been discovered in bean Fe concentration indicating that high Fe can be a trait to select for, recent studies also indicate a strong environment (E) and genotype by environment (G × E) influence on Fe concentration. It is also important to note that this target value for high Fe concentration appears to be at the high end of the range of Fe concentrations observed for beans.
To illustrate the E and GxE effect on Fe concentration, consider the results illustrated in Figure 3. In this study, Fe concentration of 10 bean varieties grown across 2 consecutive growing seasons in 9 different agroecologies in Uganda is summarized (Katuuramu et al., 2021). The results clearly show high variability across location for any given variety, ~30–40 μg/g. In addition, relevant to the discussion of the previous assumption of an average bean Fe concentration assumption of 50 μg/g, the results also indicate a much higher average of 60–75 μg/g. These results clearly indicate that Fe concentration is not a stable trait across multiple locations over a relatively small area such as Uganda. Moreover, the 30–40 μg/g range for these varieties suggests that Fe concentration is not a stable trait. Also, the observed average Fe concentration of 65 μg/g for all values would mean that a high Fe target value of 105 μg/g would be necessary to meet the assumptions of the high Fe biofortification approach. As shown in Figures 1, 2, values above 90 μg/g are uncommon.
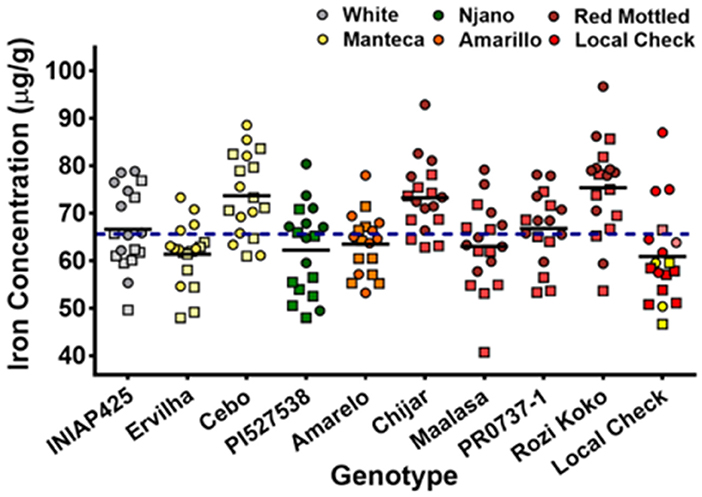
Figure 3. Iron concentrations of genotypes grown in nine locations across three agro-ecological zones in Uganda, field season 2015 (squares) and 2016 (circles). Adapted from Katuuramu et al. (2021). Dashed line indicates mean Fe concentration of all values.
Iron bioavailability measurements from this GxE study indicate that Fe bioavailability is a highly heritable trait (Figure 4). In addition, Fe concentration and Fe bioavailability were not correlated (Figure 5), with a yellow bean Manteca and white bean varieties consistently delivering more Fe regardless of concentration. These observations suggest that the high Fe bioavailability trait may already be established in certain market classes.
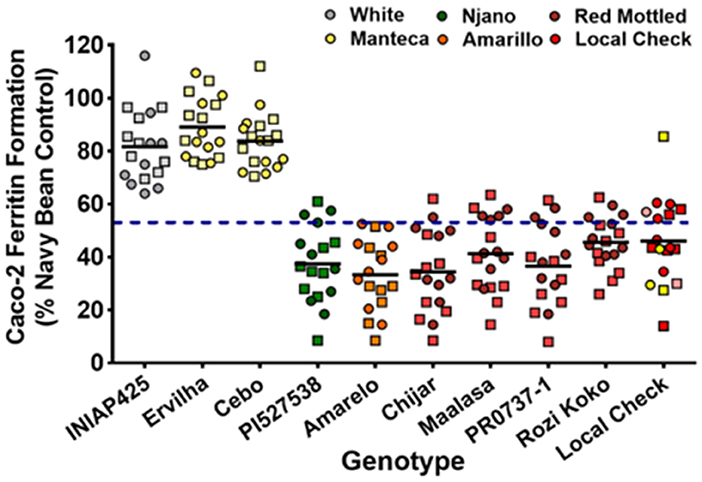
Figure 4. Iron bioavailability of genotypes grown in nine locations across three agro-ecological zones in Uganda, field season 2015 (squares) and 2016 (circles).
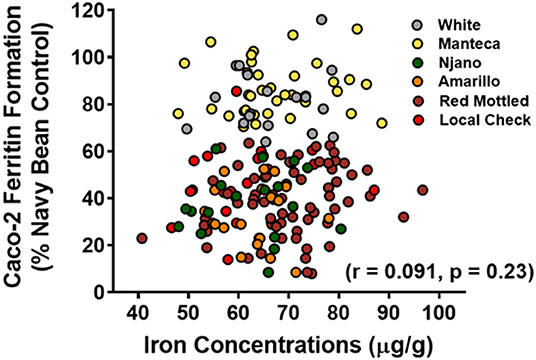
Figure 5. Scatter plots illustrating the relationship between cooked seed iron and iron bioavailability of the ten genotypes grown in nine locations across three agro-ecological zones in Uganda. Each value represents the mean of two field replicates per genotype from each location for field season 2015 and 2016 (n = 179). Adapted from Katuuramu et al. (2021).
In summary, the study by Katuuramu et al. (2021), appears to be the only published study demonstrating the GxE effect on bean Fe concentration. Most importantly this study is a 2 year “on farm” trial thus demonstrating the variance in Fe concentration that can occur once varieties are released to farmers. At present, the authors of this paper are aware of one other data set, currently under analysis, that investigates the GxE effect on bean Fe concentration that has been developed to examine this assumption as related to bean Fe biofortification. Thus, there appears to be very little evidence that the high Fe concentration can be a sustainable trait across a region of any defined size. This assumption is critical to the high Fe approach, as without fulfillment, the strategy fails.
Iron Delivery: an Alternative Approach to Iron Biofortification of Beans
In general, the high Fe approach to bean Fe biofortification relies on the belief that infusion of sufficient additional Fe into the diet from beans will result in more Fe absorbed and thus alleviation of Fe deficiency. Indeed, if all of the assumptions of this approach are met, then it would be reasonable to expect such effect to occur. However, as discussed previously, there is a multitude of evidence to indicate that the key assumptions of the high Fe approach are not met for bean Fe biofortification. Therefore, if beans are to be utilized as a vehicle to deliver more absorbable Fe into a diet, an alternative approach is needed.
Multiple studies now indicate that shifting to a strategy that focuses on “iron delivery” may be the best approach to improve the nutritional quality of Fe from beans. This approach utilizes knowledge of factors that affect Fe bioavailability and utilizing models that can measure and monitor Fe bioavailability at relatively low cost with high throughput of samples. Examples of such factors include disruption of the cotyledon cell wall in order to release intracellular Fe, reduction of phytic acid via activation of endogenous phytase via soaking prior to consumption, consideration of the influence of other components in the diet or in meals eaten with beans, and traits such as seed coat polyphenolic profiles that enhance Fe uptake rather than inhibit. It is important to remember that Fe is micronutrient, existing in relatively small quantity as compared to proteins, carbohydrates, fiber, organic acids, and phytochemicals. As a cation, it is easily complexed and exchanges with the components of a meal depending on pH and strength and nature of the bond to a compound. This dynamic interaction of Fe with food components means that assessment of Fe bioavailability within an individual food and within a meal must be a key feature of a crop biofortification program.
The immediate tools that are available for an “iron delivery” approach are the Caco-2 cell bioassay for Fe bioavailability coupled with a poultry feeding model to identify, confirm, and monitor nutritional gains (Tako et al., 2016; Wiesinger et al., 2018, 2019; Glahn et al., 2020). These models have been thoroughly validated relative to human studies and represent an approach that is data-driven and cost-effective. Studies have also shown that the Caco-2 cell bioassay can be semi-quantitative in predicting the amount of Fe that could be taken up from a given food or meal (Yun et al., 2004).
Consider the following accomplishments in knowledge of Fe nutrition from beans, developed with the use of the Caco-2 cell bioassay alone, and in conjunction with animal feeding trials. First, the Caco-2 cell bioassay has been instrumental in defining the influence of seed coat polyphenols on Fe bioavailability. Initial studies demonstrated that the seed coat polyphenols play a strong role in bean Fe bioavailability by comparing white vs. red beans (Lung'aho and Glahn, 2010; Tako and Glahn, 2010). Subsequently, this approach dispelled the traditional belief that all seed coat polyphenols are inhibitors of Fe uptake. In recent years a series of experiments using the Caco-2 cell bioassay demonstrated that not all seed coat polyphenols are inhibitors, and some are clearly promoters of Fe bioavailability (Hart et al., 2015, 2017, 2020). In related work, studies of the yellow bean market class with the Caco-2 cell bioassay demonstrated that the relatively high Fe bioavailability found in certain varieties was due at least in part to the preponderance of Fe uptake promoting polyphenols relative to inhibitory polyphenols (Wiesinger et al., 2018, 2019). In similar work, the slow darkening trait of pinto beans was identified by the Caco-2 cell bioassay as a strong factor to promote Fe bioavailability, presumably due to polyphenolic profile, i.e., a preponderance of Fe uptake promoters relative to inhibitory compounds (Wiesinger et al., 2021). This was a significant discovery as slow darkening pinto varieties are already widely grown in the upper Midwest region of the US and are highly favored by growers and consumers; thus, the Caco-2 bioassay identified a popular trait with enhanced nutritional value.
Application of the Caco-2 cell bioassay demonstrated that the fast-cooking trait is also linked to improved Fe bioavailability (Wiesinger et al., 2016). Moreover, the fast cooking trait appears to be at least partially due to the preponderance of seed coat polyphenols that promote Fe bioavailability, as well as factors such as thinner cotyledon cell walls. Studies of the fast-cooking trait with the Caco-2 cell bioassay also lead to the observation that the cotyledon cell wall is a major barrier to Fe uptake from beans and other legumes (Glahn et al., 2016). Subsequent work demonstrating rupture of the cotyledon cell walls through processing techniques exposed the untapped resource of intracellular Fe in beans thus allowing for food science opportunities to generate products with enhanced Fe delivery (Wiesinger et al., 2020).
The strategy of enhancing Fe bioavailability via post-harvest processing to reduce PA or inhibitory polyphenols is a research area that shows promise to enhance Fe bioavailability. Multiple studies provide evidence that soaking, and method of cooking can alter these factors and enhance or reduce Fe delivery (Nakitto et al., 2015; Feitosa et al., 2018; Hummel et al., 2020). Also, reduction of phytate via transgenic expression of phytase shows promise in crops such as maize, wheat and peas but has not been published in the common bean (Drakakaki et al., 2005; Abid et al., 2017; Chouchene et al., 2018). These phytate reduction approaches have not been properly explored in the common bean via an established model such as the Caco-2 bioassay, but certainly could be easily addressed. Similar studies in lentils using the Caco-2 cell bioassay show promise as reduced phytate and enhanced Fe bioavailability can be achieved depending on location of harvest and post-harvest processing (DellaValle et al., 2013; DellaValle and Glahn, 2014). However, although reduction in phytate via bean genotype, or via phytase treatment has been shown to increase Fe absorption in humans, the results must be interpreted with caution (Petry et al., 2013, 2014). In these studies, extrinsic isotopic labeling was the method for measuring Fe absorption, and this approach is potentially flawed due to possible incomplete equilibration of the extrinsic label with intrinsic Fe of the beans (Glahn et al., 2015, 2017). In addition, low phytate beans have been shown to cause adverse gastrointestinal symptoms (Petry et al., 2016b); however, it should be noted that these studies on phytate reduction in beans could yield different results depending on the color class of beans. For example, low phytate white beans could yield high Fe bioavailability, as there would be no seed coat polyphenols to inhibit Fe bioavailability in the absence of phytate. Conversely, reduction of phytate in black beans or red beans could have no benefit as the seed coat polyphenols tend to be highly inhibitory of Fe bioavailability and thus the polyphenols would negate the benefit of lower phytate (Glahn et al., 2017). Such possibilities further justify an approach using a screening tool such as the Caco-2 cell bioassay to define these potential effects on Fe bioavailability.
The Caco-2 cell bioassay fills a key role in studies of Fe nutrition due to its low cost and high throughput capability. As it is now thoroughly validated relative to human studies it can address research objectives of Fe nutrition, such as those mentioned previously, that would be impractical and cost-prohibitive to address via other models, especially human studies. Used appropriately, it has the potential to act as a monitoring tool of Fe delivery from foods such as beans and other staple food crops, with capability to provide a quantitative estimate of Fe uptake from foods (Yun et al., 2004). The Caco-2 cell bioassay has been shown to have the capability to assess components of a diet or meal plan, identifying foods consumed with beans with beans that can influence Fe bioavailability from bean Fe (Glahn et al., 2017; Dias et al., 2018). Such application can be particularly useful in planning for human efficacy trials of bean varieties targeted for a specific region or food system. Moreover, when coupled with the poultry model of Fe nutrition it can further refine conditions and objectives for human trials, thus enabling added confidence in success of costly human studies.
Summary
This paper presents the perspective that the current high Fe approach to bean Fe biofortification may not be effective at alleviating iron deficiency anemia as the major assumptions of this approach are not being met. The most profound evidence for this perspective is exemplified in a recent study of beans from countries of East Africa, where varieties released as “biofortified” were found to be no different than non-biofortified lines present in the marketplace (Glahn et al., 2020). Moreover, a recent “on farm” study in Uganda demonstrates the difficulty of consistently producing beans high in Fe that can deliver more bioavailable Fe (Katuuramu et al., 2021). Indeed, in this region it appears that the high rates of iron deficiency anemia have evolved and persisted in the presence of bean varieties that are substantially higher (~20 μg/g) than the assumed average Fe concentration of non-biofortified beans. This observation suggests a broad programmatic lack of assessment of the bean varieties present in the East Africa food system prior to or since the initiation of the high Fe approach ~18 years ago. Similar programs for bean Fe biofortification are in place in regions such as Central and South America, however a similar assessment has not yet been done or published of these regions.
The perspective of this paper also advocates for a redefinition of bean Fe biofortification. In contrast to what was known 18 years ago, when the high Fe approach was initiated, a body of work now exists to show that a strong focus on factors and traits that promote Fe bioavailability and enable delivery of absorbable Fe. This approach should be data driven and not based on assumptions, utilizing in vitro tools, animal trials and other analytical techniques to facilitate strategic human studies. Most importantly, the advances and factors that enable enhanced delivery of Fe from beans should be monitored to ensure that the nutritional benefits are sustainable.
Author Contributions
RG conceived the paper, and was primary author of the document. HN was a co-author and edited the paper for content.
Funding
This manuscript funded by the United States Department of Agriculture, Agricultural Research Service.
Conflict of Interest
The authors declare that the research was conducted in the absence of any commercial or financial relationships that could be construed as a potential conflict of interest.
References
Abid, N., Khatoon, A., Maqbool, A., Irfan, M., Bashir, A., Asif, I., et al. (2017). Transgenic expression of phytase in wheat endosperm increases bioavailability of iron and zinc in grains. Transgenic Res. 26, 109–122. doi: 10.1007/s11248-016-9983-z
Andersson, M. S., Saltzman, A., Virk, P. S., and Pfeiffer, W. H. (2017). Chapter 5: progress update: crop development of biofortified staple food crops under harvestplus. Afr. J. Food Agric. Nutr. Dev. 17, 11905–11935. doi: 10.18697/ajfand.78.HarvestPlus05
Beebe, S. (2020). Biofortification of common bean for higher iron concentration. Front. Sustain. Food Syst. 4:573449. doi: 10.3389/fsufs.2020.573449
Beebe, S., Gonzalez, A. V., and Rengifo, J. (2000). Research on trace minerals in the common bean. Food Nutr. Bull. 21, 387–391. doi: 10.1177/156482650002100408
Blair, M. W., Gonzalez, L. F., Kimani, P. M., and Butare, L. (2010). Genetic diversity, -gene pool introgression and nutritional quality of common beans (Phaseolus vulgaris L.) from Central Africa. Theor. Appl. Genet. 121, 237–248. doi: 10.1007/s00122-010-1305-x
Boy, E., Haas, J. D., Petry, N., Cercamondi, C. I., Gahutu, J. B., Mehta, S., et al. (2017). Efficacy of iron-biofortified crops. Afr. J. Food Agric. Nutr. Dev. 17, 11879–11892. doi: 10.18697/ajfand.78.HarvestPlus03
Chouchene, A., Micard, V., and Lullien-Pellerin, V. (2018). Evidence of a synergistic effect between pea seed and wheat grain endogenous phytase activities. J Agric Food Chem. 14, 12034–12041. doi: 10.1021/acs.jafc.8b03803
Cichy, K. A., Porch, T. G., Beaver, J. S., Cregan, P., Fourie, D., Glahn, R. P., et al. (2015). A Phaseolus vulgaris diversity panel for andean bean improvement. Crop Sci. 55, 2149–2160. doi: 10.2135/cropsci2014.09.0653
DellaValle, D. M., and Glahn, R. P. (2014). Differences in relative iron bioavailability in traditional Bangladeshi meal plans. Food Nutr. Bull. 35, 431–439. doi: 10.1177/156482651403500405
DellaValle, D. M., Vandenberg, A., and Glahn, R. P. (2013). Seed coat removal improves iron bioavailability in cooked lentils: studies using an in vitro digestion/Caco-2 cell culture model. J. Agric. Food Chem. 61, 8084–8089. doi: 10.1021/jf4022916
Dey, S., Regon, P., Kar, S., and Panda, S. K. (2020). Chelators of iron and their role in plant's iron management. Physiol. Mol. Biol. Plants 26, 1541–1549. doi: 10.1007/s12298-020-00841-y
Dias, D. M., Kolba, N., Binyamin, D., Ziv, O., Regini-Nutti, M., Martino, H. S. D., et al. (2018). Iron biofortified carioca bean (Phaseolus vulgaris L.)-based brazilian diet delivers more absorbable iron and affects the gut microbiota in vivo (Gallus gallus). Nutrients 10:1970. doi: 10.3390/nu10121970
Donahue, A. M., Berti, P., Siekmans, K., Tugirimana, P. L., and Boy, E. (2017). Prevalence of iron deficiency and iron deficiency anemia in the northern and southern provinces of Rwanda. Food Nutr. Bull. 38, 554–563. doi: 10.1177/0379572117723134
Drakakaki, G., Marcel, S., Glahn, R. P., Lund, E. K., Pariagh, S., Fischer, R., et al. (2005). Endosperm-specific co-expression of recombinant soybean ferritin and Aspergillus phytase in maize results in significant increases in the levels of bioavailable iron. Plant Mol. Biol. 59, 869–880. doi: 10.1007/s11103-005-1537-3
Feitosa, S., Greiner, R., Meinhardt, A. K., Müller, A., Almeida, D. T., and Posten, C. (2018). Effect of traditional household processes on iron, zinc and copper bioaccessibility in black bean (Phaseolus vulgaris L). Foods 7:123. doi: 10.3390/foods7080123
Finkelstein, J. L., Mehta, S., Villalpando, S., Mundo-Rosas, V., Luna, S. V., Rahn, M., et al. (2019). A randomized feeding trial of iron-biofortified beans in school children in Mexico. Nutrients 11:381. doi: 10.3390/nu11020381
Glahn, R. P., Cheng, Z., and Giri, S. (2015). Extrinsic labeling of staple food crops with isotopic iron does not consistently result in full equilibration: revisiting the methodology. J. Agric. Food Chem. 63, 9621–9628. doi: 10.1021/acs.jafc.5b03926
Glahn, R. P., Lee, O. A., Yeung, A., Goldman, M. I., and Miller, D. D. (1998). Caco-2 cell ferritin formation predicts nonradiolabeled food iron availability in an in vitro digestion/Caco-2 cell culture model. J. Nutr. 128:1555–61. doi: 10.1093/jn/128.9.1555
Glahn, R. P., Tako, E., Cichy, K., and Wiesinger, J. (2016). The cotyledon cell wall and intracellular matrix are factors that limit iron bioavailability of the common bean (Phaseolus vulgaris). Food Funct. 7, 3193–3200. doi: 10.1039/C6FO00490C
Glahn, R. P., Tako, E., Hart, J. J., Haas, J. D., Lung'aho, M., and Beebe, S. (2017). Iron bioavailability studies of the first generation of iron-biofortified beans released in Rwanda. Nutrients 9:787. doi: 10.3390/nu9070787
Glahn, R. P., Wiesinger, J. A., and Lung'aho, M. G. (2020). Iron concentrations in biofortified beans and nonbiofortified marketplace varieties in east africa are similar. J. Nutr. 150, 3013–3023. doi: 10.1093/jn/nxaa193
Glahn, R. P., Wortley, G. M., South, P. K., and Miller, D. D. (2002). Inhibition of iron uptake by phytic acid, tannic acid, and ZnCl2: studies using an in vitro digestion/Caco-2 cell model. J. Agric. Food Chem. 50, 390–395. doi: 10.1021/jf011046u
Haas, J. D., Luna, S. V., Lung'aho, M. G., Wenger, M. J., Murray-Kolb, L. E., Beebe, S., et al. (2016). Consuming iron biofortified beans increases iron status in Rwandan women after 128 days in a randomized controlled feeding trial. J. Nutr. 146, 1586–1592. doi: 10.3945/jn.115.224741
Habyarimana, F., Zewotir, T., and Ramroop, S. (2018). Spatial distribution and analysis of risk factors associated with anemia among women of reproductive age: case of 2014 rwanda demographic and health survey data. Open Public Health J. 11, 425–427. doi: 10.2174/1874944501811010425
Hart, J. J., Tako, E., and Glahn, R. P. (2017). Characterization of polyphenol effects on inhibition and promotion of iron uptake by caco-2 cells. J. Agric. Food Chem. 65, 3285–3294. doi: 10.1021/acs.jafc.6b05755
Hart, J. J., Tako, E., Kochian, L. V., and Glahn, R. P. (2015). Identification of black bean (Phaseolus vulgaris L.) polyphenols that inhibit and promote iron uptake by caco-2 cells. J. Agric. Food Chem. 63, 5950–5956. doi: 10.1021/acs.jafc.5b00531
Hart, J. J., Tako, E., Wiesinger, J., and Glahn, R. P. (2020). Polyphenolic profiles of yellow bean seed coats and their relationship with iron bioavailability. J Agric Food Chem. 68, 769–778. doi: 10.1021/acs.jafc.9b05663
HarvestPlus (2019). Iron Beans in Rwanda: A Nutrition Success Story. Available online at: www.harvestplus.org/rwandasuccess: HarvestPlus
Hummel, M., Talsma, E. F., Taleon, V., Londoño, L., Brychkova, G., Gallego, S., et al. (2020). Iron, zinc and phytic acid retention of biofortified, low phytic acid, and conventional bean varieties when preparing common household recipes. Nutrients 28:658. doi: 10.3390/nu12030658
Islam, F. M. A., Basford, K. E., Redden, R. J., Gonzalez, A. V., Kroonenberg, P. M., and Beebe, S. (2002). Genetic variability in cultivated common bean beyond the two major gene pools. Genet. Resour. Crop Evolu. 49, 271–83. doi: 10.1023/A:1015567513005
Jin, F., Cheng, Z., Rutzke, M. A., Welch, R. M., and Glahn, R. P. (2008). Extrinsic labeling method may not accurately measure Fe absorption from cooked pinto beans (Phaseolus vulgaris): comparison of extrinsic and intrinsic labeling of beans. J. Agric. Food Chem. 56, 6881–6885. doi: 10.1021/jf800658s
Katuuramu, D. N., Wiesinger, J. A., Luyima, G. B., Nkalubo, S. T., Glahn, R. P., and Cichy, K. A. (2021). Investigation of genotype by environment interactions for seed zinc and iron concentration and iron bioavailability in common bean. Front. Plant Sci. 12:670965. doi: 10.3389/fpls.2021.670965
Kobayashi, T., and Nishizawa, N.K. (2012). Iron uptake, translocation, and regulation in higher plants. Annu. Rev. Plant Biol. 63, 131–152. doi: 10.1146/annurev-arplant-042811-105522
Lung'aho, M., and Glahn, R. P. (2010). Use of white beans instead of red beans may improve iron bioavailability from a Tanzanian complementary food mixture. Int. J. Vitam. Nutr. Res. 80, 24–31. doi: 10.1024/0300-9831/a000003
McClean, P. E., Moghaddam, S. M., Lopez-Millan, A. F., Brick, M. A., Kelly, J. D., Miklas, P. N., et al. (2017). Phenotypic diversity for seed mineral concentration in North American dry bean germplasm of middle American ancestry. Crop Sci. 57, 3129–3144. doi: 10.2135/cropsci2017.04.0244
Mulambu, J., Andersson, M., Palenberg, M., Pfeiffer, W., Saltzman, A., Birol, E., et al. (2017). Iron beans in Rwanda: crop development and delivery experience. Afr. J. Food Agric. Nutr. Dev. 17, 12026–12050. doi: 10.18697/ajfand.78.HarvestPlus10
Nakitto, A. M., Muyonga, J. H., and Nakimbugwe, D. (2015). Effects of combined traditional processing methods on the nutritional quality of beans. Food Sci Nutr. 3, 233–41. doi: 10.1002/fsn3.209
Ngesa, O., and Mwambi, H. (2014). Prevalence and risk factors of anaemia among children aged between 6 months and 14 years in Kenya. PLoS ONE 9:e113756. doi: 10.1371/journal.pone.0113756
PABRA (2017). PABRA Annual Report 2016/2017 - 20th Anniversary Special Edition. Nairobi: Pan-Africa Bean Research Alliance–PABRA.
Pasricha, S. R. (2014). Anemia: a comprehensive global estimate. Blood 123, 611–612. doi: 10.1182/blood-2013-12-543405
Pereira, H. S., Del Peloso, M. J., Bassinello, P. Z., Guimaraes, C. M., Melo, L. C., and Faria, L. C. (2014). Genetic variability for iron and zinc content in common bean lines and interaction with water availability. Genet. Mol. Res. 13, 6773–6785. doi: 10.4238/2014.August.28.21
Petry, N., Egli, I., Campion, B., Nielsen, E., and Hurrell, R. (2013). Genetic reduction of phytate in common bean (Phaseolus vulgaris L.) seeds increases iron absorption in young women. J. Nutr. 143, 1219–1224. doi: 10.3945/jn.113.175067
Petry, N., Egli, I., Gahutu, J. B., Tugirimana, P. L., Boy, E., and Hurrell, R. (2012). Stable iron isotope studies in Rwandese women indicate that the common bean has limited potential as a vehicle for iron biofortification. J. Nutr. 142: 492–497. doi: 10.3945/jn.111.149286
Petry, N., Egli, I., Gahutu, J. B., Tugirimana, P. L., Boy, E., and Hurrell, R. (2014). Phytic acid concentration influences iron bioavailability from biofortified beans in Rwandese women with low iron status. J. Nutr. 144:1681–1687. doi: 10.3945/jn.114.192989
Petry, N., Olofin, I., Hurrell, R. F., Boy, E., Wirth, J. P., Moursi, M., et al. (2016a). The proportion of anemia associated with iron deficiency in low, medium, and high human development index countries: a systematic analysis of national surveys. Nutrients 8:693. doi: 10.3390/nu8110693
Petry, N., Rohner, F., Gahutu, J. B., Campion, B., Boy, E., Tugirimana, P. L., et al. (2016b). In rwandese women with low iron status, iron absorption from low-phytic acid beans and biofortified beans is comparable, but low-phytic acid beans cause adverse gastrointestinal symptoms. J. Nutr. 146, 970–975. doi: 10.3945/jn.115.223693
Shi, L., Arntfield, S. D., and Nickerson, M. (2018). Changes in levels of phytic acid, lectins and oxalates during soaking and cooking of Canadian pulses. Food Res. Int. 107, 660–668. doi: 10.1016/j.foodres.2018.02.056
Szabo, A. J., Grirnaldi, R. D., and Jung, W. F. (1975). Absorption of extrinsic and intrinsic iron labels. Nutr. Rev. 33, 238–240. doi: 10.1111/j.1753-4887.1975.tb05226.x
Tako, E., Bar, H., and Glahn, R. P. (2016). The combined application of the Caco-2 cell bioassay coupled with in vivo (Gallus gallus) feeding trial represents an effective approach to predicting Fe bioavailability in humans. Nutrients 8, 732–757. doi: 10.3390/nu8110732
Tako, E., Beebe, S., Reed, S., Hart, J. J., and Glahn, R. P. (2014). Polyphenolic compounds appear to limit the nutritional benefit of biofortified higher iron black bean (Phaseolus vulgarisL.). Nutr. J. 13:28. doi: 10.1186/1475-2891-13-28
Tako, E., and Glahn, R. P. (2010). White beans provide more bioavailable iron than red beans: studies in poultry (Gallus gallus) and an in vitro digestion/Caco-2 model. Int. J. Vitam. Nutr. Res. 80, 416–429. doi: 10.1024/0300-9831/a000028
Tako, E., Reed, S., Anandaraman, A., Beebe, S., Hart, J. J., and Glahn, R. P. (2015). Studies of cream seeded carioca beans (Phaseolus vulgaris L.) from a Rwandan efficacy trial: in vitro and in vivo screening tools reflect human studies and predict beneficial results from iron biofortified beans. PLoS ONE 10:e0138479. doi: 10.1371/journal.pone.0138479
Wiesinger, J. A., Cichy, K. A., Glahn, R. P., Grusak, M., Brick, M. A., Thompson, H. J., et al. (2016). Demonstrating a nutritional advantage to the fast-cooking dry bean (Phaseolus vulgaris L.). J. Agric. Food Chem. 64, 8592–8603. doi: 10.1021/acs.jafc.6b03100
Wiesinger, J. A., Cichy, K. A., Hooper, S. D., Hart, J. J., and Glahn, R. P. (2020). Processing white or yellow dry beans (Phaseolus vulgaris L.) into a heat treated flour enhances the iron bioavailability of bean-based pastas. J. Funct. Foods 71:104018. doi: 10.1016/j.jff.2020.104018
Wiesinger, J. A., Cichy, K. A., Tako, E., and Glahn, R. P. (2018). The fast cooking and enhanced iron bioavailability properties of the manteca yellow bean (Phaseolus vulgaris L.). Nutrients 10:1609. doi: 10.3390/nu10111609
Wiesinger, J. A., Glahn, R. P., Cichy, K. A., Kolba, N., Hart, J. J., and Tako, E. (2019). An in vivo (Gallus gallus) feeding trial demonstrating the enhanced iron bioavailability properties of the fast cooking manteca yellow bean (Phaseolus vulgaris L.). Nutrients 11:1768. doi: 10.3390/nu11081768
Wiesinger, J. A., Osorno, J. M., McClean, P. E., Hart, J. J., and Glahn, R. P. (2021). Faster cooking times and improved iron bioavailability are associated with the down regulation of procyanidin synthesis in slow-darkening pinto beans (Phaseolus vulgaris L.). J. Funct. Foods 82:104444. doi: 10.1016/j.jff.2021.104444
Keywords: beans, iron, biofortification, anemia, bioavailability
Citation: Glahn RP and Noh H (2021) Redefining Bean Iron Biofortification: A Review of the Evidence for Moving to a High Fe Bioavailability Approach. Front. Sustain. Food Syst. 5:682130. doi: 10.3389/fsufs.2021.682130
Received: 17 March 2021; Accepted: 31 May 2021;
Published: 05 July 2021.
Edited by:
Carol Anne Wham, Massey University, New ZealandReviewed by:
Jayashree Arcot, University of New South Wales, AustraliaSapna Langyan, National Bureau of Plant Genetic Resources (ICAR), India
Copyright © 2021 Glahn and Noh. This is an open-access article distributed under the terms of the Creative Commons Attribution License (CC BY). The use, distribution or reproduction in other forums is permitted, provided the original author(s) and the copyright owner(s) are credited and that the original publication in this journal is cited, in accordance with accepted academic practice. No use, distribution or reproduction is permitted which does not comply with these terms.
*Correspondence: Raymond P. Glahn, cmF5bW9uZC5nbGFobkB1c2RhLmdvdg==