- 1Département de Biologie des Organismes, Faculté des Sciences, Université Libre de Bruxelles, Brussels, Belgium
- 2Department of Environment and Sustainability Sciences, Faculty of Natural Resources and Environment, University for Development Studies, Tamale, Ghana
- 3Department of Biology, Faculty of Science and Bioengineering Sciences, Vrije Universiteit Brussel, Brussels, Belgium
- 4Dipartimento di Biologia, Università degli Studi di Firenze, Firenze, Italy
- 5Advanced Material Science Division, CSIR-Building and Road Research Institute, Kumasi, Ghana
The current and projected warming of the earth is unequivocal with humans playing a strong role as both perpetrators and victims. The warming on the African continent is projected to be greater than the global average with an increased average temperature of 3–6°C by the end of the century under a high Representative Concentration Pathway. In Africa, the Sub-Saharan region is identified as the most vulnerable to the changing climate due to its very low capacity to adapt to or mitigate climate change. While it is common to identify studies conducted to assess how climate change independently impacts water, land, or food resources, very limited studies have sought to address the interlinkages, synergies, and trade-offs existing between climate change, water, land, and food (WLF) resources as a system in Sub-Saharan Africa (SSA). The climate change and WLF security nexus, therefore, seeks to address this shortfall in literature and subsequently serve as a relevant source of information for decision-making and policy implementation concerning climate change mitigation and adaptation. In this study, 41 relevant studies were selected from Web of Science, Google Scholar, ResearchGate, and institutional websites. We provide information on the independent relationships between climate change and WLF resources, and further discuss the existing inter-linkages between climate change and the WLF security in SSA using the nexus approach, with recommendations on how decision making and policy implementations should be done using the climate change and WLF security nexus approach.
Introduction
Climate change as defined by the Intergovernmental Panel on Climate Change (IPCC) is “a change in the state of the climate that can be identified (e.g. using statistical tests) by changes in the mean and/or the variability of its properties, and that persists for an extended period, typically decades or longer” [Intergovernmental Panel on Climate Change (IPCC), 2007]. The change in the global climate has been reported to be as a result of both human activities and natural variabilities. The United Nations Framework Convention on Climate Change (UNFCCC) in their definition emphasizes that the changing climate is directly or indirectly attributable to human activities (UNFCCC, 2011). The observed changes in climate at the global, continental, and sub-continental levels include an increase in air and ocean temperatures, sea-level rise, decrease in snow and ice extent, increase and decrease in precipitation, changes in terrestrial and marine biological systems, ocean acidification [Intergovernmental Panel on Climate Change (IPCC), 2007; UNFCCC, 2011].
These observed changes in the climatic system have been mainly induced by the warming process of the climate system. This warming process is described as global warming which involves the increase in the global temperature due to the increased emissions of Greenhouse Gases (GHGs) coming from human activities [Intergovernmental Panel on Climate Change (IPCC), 2014]. The major contributing GHGs assessed by the UNFCCC include carbon dioxide (CO2), methane (CH4), nitrous oxide (N2O), hydrofluorocarbons (HFCs), perfluorocarbons (PFCs), and sulphurhexafluoride (SF6) Intergovernmental Panel on Climate Change (IPCC), 2007; Bruhwiler et al., 2021. Notwithstanding the increased number of policies developed to mitigate climate change, there has been absolute increases in total anthropogenic GHGs over the period of 2000 to 2010, reaching a total of 49 ± 4.5 GtCO2-eq/yr3, with the major contribution (about 78%) being CO2 emissions from fossil fuel combustion and industrial processes.
From 1880 to 2012, there has been an observed increase in the average temperature of the global land and ocean surface by 0.85 [0.65–1.06]°C [Intergovernmental Panel on Climate Change (IPCC), 2014]. The global mean surface temperature by 2017 was ascertained to be around 1°C higher than the preindustrial values with an increase of 0.2°C/decade (Bruhwiler et al., 2021). It is projected that by the end of the twenty-first century, the global surface temperature will likely exceed 1.5°C with higher frequencies and longer durations of heatwaves based on RCP4.5, RCP6.0, and RCP8.5 scenarios [Intergovernmental Panel on Climate Change (IPCC), 2014]. Changes in precipitation will, however, be experienced differently across the different regions of the globe (Giorgi et al., 2018). An increase in annual precipitation will be experienced in regions in the high latitudes and the equatorial Pacific under the RCP8.5 scenario, whereas regions in the dry mid-latitude and subtropics will experience a decreased annual precipitation. The regions in the wet mid-latitude and wet tropics will also experience extreme annual precipitation [Intergovernmental Panel on Climate Change (IPCC), 2014; Giorgi et al., 2018].
From a continental view, the warming in the African continent is projected to be greater than the global average with an increased average temperature of 3–4°C over the twenty-second century (Thompson et al., 2010; Zewdie, 2014). The changes in precipitation to be experienced on the African continent at the end of the twenty-first century is however expected to be unique across the different regions (Niang et al., 2014). The West African region is expected to experience a slight or no change in heavy precipitation, while precipitation in the Eastern and Central African region is projected to increase (Niang et al., 2014; Girvetz et al., 2019). For the Southern and Northern African regions, there is a projected decrease in total precipitation with Southern Africa recording an increase in heavy precipitations (Niang et al., 2014).
In Africa, the Sub-Saharan region is identified as the most vulnerable to the changing climate (Ringler et al., 2010; Thompson et al., 2010). This is due to the region's very low adaptive capacity in connection to the acute levels of poverty and the limited facilities needed to mitigate and/or adapt to the changing climate (Ringler et al., 2010). Sub-Saharan Africa (SSA) is a region that greatly depends on precipitation to be able to provide the water, food, and energy needs of its people (Thompson et al., 2010). For instance, the prevalence of rain-fed agriculture in most of the region makes its food systems highly sensitive to the changing patterns in precipitation (Thompson et al., 2010).
In SSA, many studies have presented the current and future impacts of climate change on either water resources, land resources or food systems, and how these impacts further affect the lives of people who depend on them. Whenever we discuss the issue of climate change, it is a fact that nature will suffer from the consequences, but we need to realize that humans who are the main perpetrators are equally the main victims of climate change. This is because our well-being depends on nature and its services. The United Nations enlist food, clean water, and clean air as basic needs for our existence, indicating that at any point in time, these resources should be available at adequate quantity and quality, and accessible to all (UN Department of Economic Social Affairs, 2018).
In the past decade, scientists and policymakers have come to realize that the relationship between climate change and water resources, land, and food resources are not unidimensional but multidimensional. This situation therefore calls for a nexus approach that addresses the inter-connectedness, synergies, and trade-offs existing between climate change and the water, land, and food (WLF) resources, which in early times were considered independently. While studies have sought to present the climate change and WLF security nexus in Africa, efforts in realizing it in the policymaking process is less pronounced in SSA. This study seeks to bring to light the current and future impacts of climate change on WLF resources, as well as the relationship between climate change and the WLF security nexus in SSA, and further suggests strategies needed to address these issues from a multidimensional perspective in the policymaking process.
Methods
Studies published from the period of 2005 to 2021 were extracted from databases using the keywords: “Climate change in ‘country X”' where “country X” represents a country located in the SSA region. Databases used for the extraction of studies and reports included Web of science, Google Scholar, ResearchGate. Reports on the impacts of climate change on either water, land, and/or food resources in Africa were also extracted from websites of international organizations such as IPCC, UNFCC, and the FAO. Out of 136 studies which were initially extracted, 41 were selected as relevant for this study after their abstracts were screened. Out of the 41 studies which were selected, 28 of them were used in preparing the sections Impacts of Climate Change on Water, Land and Food Resources in SSA to Climate Change and the WLF Security Nexus in SSA of this study. Table 1 provides a summary of these 28 studies.
Impacts of Climate Change on Water, Land and Food Resources in SSA
Climate Change and Water Resources in SSA
Although anthropogenic activities such as changes in land use/cover, diversions, and hyper-withdrawal of water from rivers and lakes, and increased pollution and sedimentation directly impact freshwater ecosystems in SSA, climate change also contribute to their degradation. The contributing impacts of climate change on freshwater ecosystems in SSA are evidenced by elevated water temperatures reported in surface waters of Lakes Kariba, Kivu, Tanganyika, Victoria, and Malawi (Niang et al., 2014). The water capacity of some major water systems such as lakes and rivers in SSA is expected to reduce in response to the decrease in precipitation and an increase in evaporation (Bates et al., 2008). However, studies have suggested that climate change in the region will have an overall modest effect on future water scarcity when compared to other anthropogenic activities (Niang et al., 2014).
The projected changes in water resources in response to climate change and other drivers are different when considering the sub-regions of the SSA. For instance, under the A2 and B2 scenarios, it is projected that there will be a decrease in surface water discharge and seasonal runoff volumes in the Upper Nile Basin in Uganda and the Lake Tana Basin in Ethiopia, respectively, by the 2080s (Niang et al., 2014). Water shortages are also estimated for the Okavango Delta, the Breede River in South Africa, and the downstream of the Rozva dam in Zimbabwe (Niang et al., 2014). However, an increased flow is projected to occur in the Mara, Nyando, and Tana Rivers in Eastern Africa during the second half of this century (Niang et al., 2014).
The modeling of how climate change impacts water resources in Western Africa is constrained by the significant uncertainties in models used in reference to the future precipitation of the region. For instance, while higher future precipitation is estimated in the Niger River Basin (A1, A2, and B1 scenarios), a strong seasonal component with reduced precipitation in the basin is also projected during the rainy season and increased precipitation during the dry season (A1B scenario). The Volta Basin is projected to experience a slight mean increase in precipitation and the Bani River Basin in Mali is estimated to have significant decreases in runoff (A2 scenario) due to reduced precipitation. In the Congo Basin, the impact of climate change on total runoff is projected to be minimal under A2 scenario (Niang et al., 2014).
The occurrence of drought is another important environmental issue that is highly pronounced in some sub-regions of SSA. Drought is a component of the natural variability in climate on the African continent, with quite high intensities at monthly, yearly, decadal, or century timescales. Some studies have reported on how climate change, aerosol emissions, land use practices, and subsequent land-atmosphere interactions, have led to mechanisms inducing droughts (Masih et al., 2014; Bhaga et al., 2020). Moreover, El Niño–Southern Oscillation (ENSO) and sea surface temperatures (SSTs) are considered as key factors influencing drought across SSA (Masih et al., 2014). It is however important to note that predicting drought is a slow and difficult process due to the overarching complexities related to its causes, timescale, extent, and timing on different spatial and temporal scales (Ndehedehe et al., 2020).
In the recent past, many extreme and extended droughts such as the 1999–2002 drought in North-western Africa, 1970s and 1980s droughts in Western Africa (Sahel), 2010–2011 drought in Eastern Africa (Horn of Africa), and 2001–2003 drought in Southern and South-Eastern Africa, were recorded (Masih et al., 2014). Since 2005, the rate of recurrence of drought in Eastern Africa has increased from one in every 6 years to one every 3 years. Between 2008 and 2010, over 13 million people in Eastern Africa have been affected by droughts. Moreover, the Horn of Africa which includes Djibouti, Eritrea, Ethiopia, and Somalia, have experienced extreme droughts from 2010 to 2011, caused issues of food insecurity that affected about 20 million people, leading to a significant loss of lives. Drought in Somalia, Kenya, and Ethiopia, contributed to socio-economic instabilities, where Somalia alone recorded 250,000 deaths during the same period (Bhaga et al., 2020). There is medium confidence that droughts will intensify in East and Southern Africa during the twenty-first twenty-first in some seasons, because of reduced precipitation and/or increased evapotranspiration (Niang et al., 2014).
Climate Change and Land Resources in SSA
Land use/cover change refers to any human activity and intervention that results in a change of a particular land use/cover into another land use/cover or the intensification of an existing land use/cover (Antwi-agyei et al., 2019). It has been presented that changes in land surface features such as albedo, evapotranspiration, and primary production contribute to changes in climate at different geographical scales, and the dynamics in atmospheric carbon concentrations (Linderman et al., 2005). A major consequence is when the livelihoods of people are negatively affected, and this is highly noticeable in SSA where most households rely on land-based activities for their well-being (Antwi-agyei et al., 2019).
Changes in land use/cover and climate have led to observed changes in the distribution and dynamics of different types of terrestrial ecosystems in SSA. Some of the terrestrial ecosystems include grasslands and shrublands, savannas and woodlands, and forests. The increasing intensity of anthropogenic activities in forested areas has led to a decrease in the areal extent of natural vegetations (Gonzalez et al., 2012). Observations have been made on a complex set of spatial shifts in the distribution of remaining natural vegetation types (Niang et al., 2014). Some plants and animal species which are specialist to certain ecological conditions in the SSA are identified to be vulnerable to limited levels of resources caused by climate change (Sintayehu, 2018). For instance, in Ethiopia, the Ethiopian wolf (Canis simensis) is said to be at risk of local extinction due to the longer dry periods with reduced availability of water (Sintayehu, 2018).
While there have been net decreases recorded in woody vegetation in Western Africa, the Central, Eastern, and Southern Africa have recorded net increases in their woody vegetation. Even though the principal driver of these changes is land use/cover change caused by human activities such as the expansion of crop production and livestock rearing activities, and fuelwood harvesting, climate variability, climate change, and interactions between them drivers have significant added and interacting effects (Niang et al., 2014). Rural livelihoods in SSA are frequently affected by changes in land use/cover. These impacts are commonly intensified by the dependence of rural households on agricultural activities that are significantly reliant on the climate (Antwi-agyei et al., 2019). Drought resulting from changes in land use/cover and climate is considered as a key cause of land degradation, aridity, and desertification, further producing immense socio-economic impacts such as large-scale migration, and famine in the semi-arid sub-region of SSA (Masih et al., 2014). Poor smallholder farmers in SSA are reliant on land to gain access to relevant input resources. However, acquiring access to arable lands for crop production has been on a diminishing trend due to the pressure from the growing population, exacerbating land degradation due to climate change, and more importantly land grabbing (Deen-Swarray et al., 2020).
Climate Change and Food Resources in SSA
In SSA, agricultural activities which depend on rain account for over 95% of the total agriculture which takes place on land (Mupangwa et al., 2016). Future climatic changes occurring in SSA may lead to the shortening of crop and fodder growing periods in Western and Southern Africa by an average of 20% by 2050, resulting in a 40% decrease in cereal yields and a drop in the biomass of cereal used for livestock rearing. Changes in length of the growing season with a propensity toward decreased growing season length are also projected, however, with some areas having the potential to have longer growing seasons (Niang et al., 2014). Estimated losses in crop yield resulting from climate change during the middle of the twenty-first-century range from 18% for Southern Africa to 22% totaled across SSA. However, in Eastern Africa, warming at high elevation locations could promote the production of maize (Niang et al., 2014).
The impacts of climate change on agriculture is not only limited to crop production but also includes livestock rearing and other activities related to agriculture. Climate variables such as air, temperature, humidity, wind speed and other climate factors are projected to directly influence the performance of livestock, such as growth, milk production, wool production, and reproduction. Chickens are largely at risk of climate change due to the low thermal window beyond which reproduction and growth are negatively affected. Moreover, projected increased warming can be highly pronounced within intensive poultry practices as animals are reared in enclosed spaces (Gezie, 2019). Changes in climate can also influence the quantity and quality of provender (pasture, forage, and grain) and also the occurrence and spread of livestock diseases and parasite (Ukpe et al., 2020). In the transition zones which include the West African Sahel and coastal and mid-altitude areas in Eastern and South-eastern Africa, it is projected that livestock rearing will replace mixed crop-livestock systems by 2050 (Niang et al., 2014).
Climate Change and the WLF Security Nexus in SSA
The use of the nexus approach in addressing issues of climate change helps to appreciate how WLF resources are interconnected in a two- and/or three-dimensional way. Some studies have indicated how water depletion in freshwater ecosystems and wetlands impacts crop production, fishing, and livestock rearing present in SSA (Leauthaud et al., 2013). Others have shown how improper agricultural practices can impact land and water resources (Barnhoorn and van Dyk, 2020; Olisah et al., 2020). Agriculture is known to be a major source of food and livelihoods in many local communities in SSA. With the majority of these communities depending on precipitation as a major ingredient for higher yields, crop production commonly takes place during wet seasons of the year (Thompson et al., 2010; Herrmann and Mohr, 2011). However, depending on the location and local weather conditions, an area within a country may experience either a unimodal, bimodal or multimodal wet season. For instance, most of the seasonal rainfall regimes (>90%) experienced by regions in SSA are single wet season regimes. However, the central Congo Basin, eastern Madagascar, and small areas of the Guinea coast do experience an all year round wet season, while dual wet-season regimes are most common in East Africa (Herrmann and Mohr, 2011).
In countries with a single wet season, crop production that takes place during the dry season is done with irrigation system interventions. The local communities build reservoirs or depend on riverine and lacustrine systems for irrigation of their crop fields. Nevertheless, this practice of irrigation does no prove to be sustainable as the reservoirs and freshwater ecosystems are prone to depletion with a projected increase in surface water and air temperatures and a decrease in precipitation in some regions of SSA (Niang et al., 2014). Such a situation is evident in Southern Africa where during 2015, 9 million ha which represents only 9% of the total cultivated land of 107 million ha was irrigated. However, the volume of water extracted for these irrigation activities represents over 70% of the available freshwater resources, of which countries like South Africa, Zimbabwe, Mozambique, and Tanzania are major contributors (Nhemachena et al., 2020). This issue is even more pronounced when the people have to depend on the limited water resources for other key uses such as drinking, cooking, or additional domestic activities.
In the 31st session of the Committee on World Food Security, the Food and Agriculture Organisation (FAO) (2005) indicated that in developing countries which includes countries in SSA, climate change would lead to an increase in lands that are arid and lands with moisture stress, thereby causing a decrease in cultivable rainfed land, with a consequent decline in cereal production. The potential impacts of climate change on lands and the growing population in SSA will eventually cause increased extraction of the limited freshwater and groundwater resources to meet the increased demands on food and water resources (Bonsor et al., 2010).
Countries in the north and east of the Southern Africa region is projected to experience a reduced potential output of cereal production by 50% or more by 2080 in response to the future events of increased water scarcity caused by climate change. Moreover, while countries in the west of the Southern Africa region are expected to experience a decrease of 15–50% in agricultural productivity, countries such as Angola, Democratic Republic of Congo (DRC), parts of Zambia and Mozambique, positioned in the further north will experience an increase of 25% or more (Nhemachena et al., 2020). Between the period of 2011 to 2020, countries like Mauritania, Mali, Cote d'Ivoire, Madagascar, and Lesotho have experienced poor rains and droughts leading to poor agricultural harvests, loss of livestock, an increase in food prices, starvation, food shortage, famine, drying up of reservoirs, and dams, affecting a great number of people. In Lesotho, it is projected that more than 30% of the population will experience acute food insecurity due to ongoing drought conditions (Bhaga et al., 2020).
Climate change resulting in the depletion of freshwater ecosystems with soil water over the years has caused the reduction in fishing and livestock-rearing activities in some countries in SSA. Several climate variables such as changes in air and water temperatures, precipitation, river flow, nutrient levels, storm frequency and intensity, and flooding occurring in freshwater ecosystems can influence fisheries through a range of direct and indirect pathways (Allison et al., 2009). Out of the 132 countries which are vulnerable to climate change-driven impacts on fisheries, Angola, DRC, Mauritania, and Senegal were found in the top five (Allison et al., 2009; Niang et al., 2014). Projected changes in temperature and precipitation will also lead to a reduction in the quantity and quality of biomass in pasturelands and drinking water needed for livestock rearing. This situation will be more obvious during prolonged dry seasons where the consequences could be increased reduction in livestock fitness and number with farmers shifting to more resilient breeds or crop farming (Descheemaeker et al., 2016).
Land deals, mostly in the form of leases or concessions provided on a long-term basis, have appeared as a significant component of land use/cover change across SSA over the last decade (Gomes, 2020). Among the total land area of about 50 million hectares (ha) in developing countries being targeted by external investors, SSA possesses the largest amount (Glover and Jones, 2018). In most cases, lands acquired by external investors in SSA are used to cultivate plants useful for biofuel production, which is identified as a strategy in mitigating climate change (Gomes, 2020). In Mozambique, the rush of land deals has generated controversies, pointing out its potential impacts on freshwater ecosystems and arable lands, restricting local communities of access to freshwater ecosystems on leased lands, thereby reinforcing their vulnerability to climate change (Glover and Jones, 2018; Gomes, 2020). When lands are available for the local communities located in resource-poor countries, it offers them a means to increase their limited natural resources for food production and may in turn act as a safeguard to potential future impacts of climate change on food security (Chiarelli et al., 2016).
Terrestrial ecosystems such as forests, riparian vegetation, grasslands, savannas, and woodlands are identified to contribute positively to the hydrological cycle. It is evident that the observed and projected changes in the distribution and dynamics of the different terrestrial ecosystems in SSA will add to the existing impacts of climate change on freshwater ecosystems. Sub-Saharan Africa has experienced rapid deforestation of about 3.4 million ha/year between 2000 and 2010 with Malawi having the highest deforestation rate (Mapulanga and Naito, 2019). The primary drivers of deforestation in SSA are the expansion of agriculture, livestock grazing, and fuelwood harvesting (Niang et al., 2014). With increasing rates of deforestation in SSA, the carbon storage and sequestration capacity of trees reduces, causing increased concentration of atmospheric CO2 and higher rates of warming events. The impacts of deforestation on freshwater ecosystems include reduced infiltration, increased sedimentation affecting water quality, increased soil erosion, and increased evaporation of neighboring surface water ecosystems (Mapulanga and Naito, 2019). This situation of depleted quantity and quality of freshwater ecosystems goes a long way to negatively affect crop and livestock-rearing activities, and further limits the available water for domestic use, all amounting to food and water insecurity.
Deforestation exposes the land to soil erosion and the loss of available soil nutrients and moisture necessary for crop production and the production of pasturelands useful for livestock rearing. Deforestation occurring in riparian vegetation exposes freshwater ecosystems to direct sunlight, increasing the risk of surface evaporation as water and air temperature continues to increase in SSA. In regions of limited arable lands available for crop production, farmers will be obliged to adopt the use of fertilizers to improve crop yields. During events of heavy precipitation, as predicted to occur in Eastern African countries (Niang et al., 2014), increased runoffs from the farms reaching neighboring freshwater ecosystems pose the risk of water pollution, affecting water quality and aquatic biodiversity.
Climate change in connection with other environmental and production factors could increase crop loss generated by pests, weeds, and diseases. In the highland regions of Eastern Africa, warming could lead to crop pests extending their range into areas that are limited by cold temperatures (low confidence). For example, in highland areas of Eastern Africa where Arabica coffee is produced, trends in warming may cause the coffee berry borer (Hypothenemus hampei) to become a severe threat in regions of Ethiopia, Kenya, Uganda, Rwanda, and Burundi which are involved in coffee production (Jaramillo et al., 2011). Increases in temperature in highland areas of Eastern Africa which are involved in banana production may increase the risk of altitudinal range extension of the highly destructive burrowing nematode, Radopholus similis (Nicholls et al., 2008). Another study conducted by Zacarias (2020) projected that there is an enormous climatic potential for the spread of the fall armyworm (Spodoptera frugiperda), with potential increases in SSA, particularly the northern border of SSA.
To deal with the issue of pest, weeds and diseases in farms, farmers are forced to make use of pesticides, weedicides, herbicides, or fungicides. The improper handling of these chemicals by farmers and other end users have been responsible for the high level of occurrence in the freshwater and terrestrial ecosystems, hence posing threat to human health and the ecosystem at large (Olisah et al., 2020). In South Africa, significant concentrations of endosulfan, DDTs and other pesticides were reported to be present in Lourens River, Hex River, and Pongolo floodplain (Olisah et al., 2020). Barnhoorn and van Dyk (2020) also reported the occurrences of herbicides such as Dacthal, metribuzin, simazine, tebuthiuron, terbuthylazine, and the fungicides azoxystrobin, carbendazim, epoxiconazole, metalaxyl (Ridomil), propiconazole, pyrimethanil and thiabendazole in the Roodeplaat Dam of South Africa. Other studies conducted in Lake Bosomtwe and Lake Volta of Ghana, Tana and Sabaki of Kenya, Lake Victoria of Tanzania, Ogbese River of Nigeria, and Lake Ziway in the Ethiopian Rift Valley basin reported significant concentrations of pesticides in water and sediment samples (Merga et al., 2020; Olisah et al., 2020). In other instances, high levels of pesticides were recorded in soil samples collected from farmlands located in Upper Awash of Ethiopia and Ngarenanyuki, Vikuge Farm, and Lake Victoria of Tanzania (Olisah et al., 2020).
Conclusions And Recommendations
This study has presented the current and future trends of climate change in SSA. On one hand, it examines the relationship between climate change and the components of the WLF security nexus independently, and on the other hand, it provides an overview of how interconnected the components of the WLF security nexus are, and how when climate change impacts one of them, the others can also directly or indirectly suffer the consequences. The nexus approach which presents the interlinkages, trade-offs, and synergies existing between water, land and food resources suggests the need for developing mitigation and adaptation strategies toward climate change in a comprehensive manner. In other words, policies developed to tackle climate change impacts on either of the three components of the nexus must be able to have the other components in the view. For instance, water resource management strategies must make sure land resources such as forest ecosystems and food resources from agriculture are not negatively impacted in both present and future time, and vice versa.
This is a very complex system, and therefore calls for a trans-disciplinary team which is a diverse group of professionals and experts from the sectors of climate change, water resource management, natural resource management, biodiversity and ecosystem conservation, sustainable agricultural production, environmental protection, economics, sociology, and local communities (Figure 1), working together across their disciplines to tackle the issues relating to climate change and the WLF security nexus. The decision-making process among stakeholders must however be complemented by using models of systems approach. For instance, the development of the Climate, Land, Energy and Water (CLEWS approach) modeling framework by the International Atomic Energy Agency (IAEA) in 2009 helps to employ a combined use of modeling tools available for the components of the WLF security nexus and climate change (Welsch et al., 2014). The model which is interdisciplinary in nature helps stakeholders to quantify the existing interactions between the components of the WLF security nexus, and also provide a clear definition of data exchange between these components/stakeholders. The modeling tools are then calibrated using historical climatic data (preferably done singly by each model before assessing interlinkages). Future scenarios which are represented in the modeling tools are therefore used to assess implications by comparing current data with future development pathways and eventually the results are interpreted and implemented (Welsch et al., 2014).
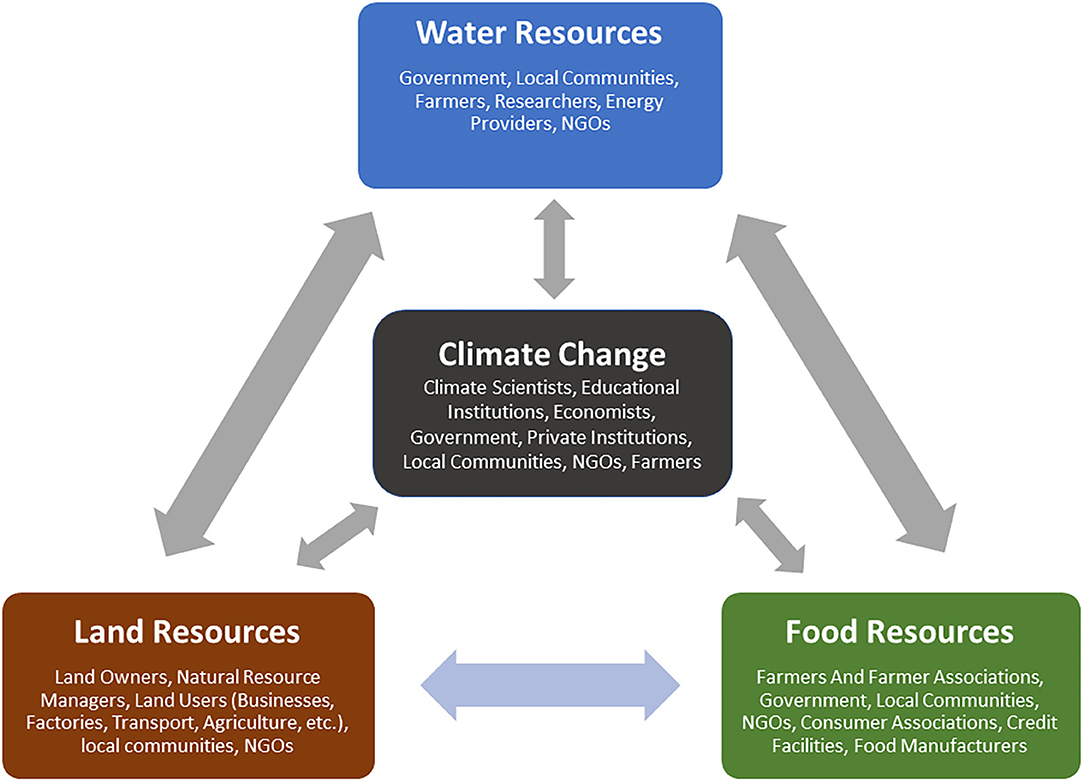
Figure 1. A flowchart representing the climate change and WLF security nexus and the key stakeholders involved in the policymaking process.
One of the strengths of the CLEWS approach is that it is not inherently system-biased, indicating that no system is given priority over the other (Ramos et al., 2021). Over the years, the drive of the CLEWS approach by institutions through multiple applications has contributed to closing the gap between science and policy (Ramos et al., 2021). Amidst the benefits being derived from the CLEWS approach, it possesses some limitations. It is only able to promote more interactions between the components of the nexus when used at a larger scale (regional and national level studies). At smaller scales (local level studies), the interactions between the components of the nexus appear to be limited and rather promotes intra-linkages between them. Moreover, mechanisms are not available to promote the transfer of methods and approaches across the regional, national, and/or local scales (Ramos et al., 2021).
To achieve the WLF security nexus in a changing climate, sufficient attention must be given to both national and international organizations/institutions in SSA. They must be able to integrate the outcomes WLF security nexus generated from the multidisciplinary teams into adaptation and mitigation plans toward climate change. Considering the novelty of the WLF security nexus approach in the policymaking process of SSA region, efforts must be taken to deepen the knowledge hub of the stakeholders which will help to foster the ease of interactions between them. Finally, governments and private institutions must be ready to invest or provide institutional support to attract investments for promoting the WLF security nexus (Rasul and Sharma, 2016).
Author Contributions
SAO: writing and editing of manuscript. SC and SO: editing and review of manuscript. All authors contributed to the article and approved the submitted version.
Conflict of Interest
The authors declare that the research was conducted in the absence of any commercial or financial relationships that could be construed as a potential conflict of interest.
Acknowledgments
The authors wish to extend their gratitude to all climate change scientists for their immense efforts in providing information on the impacts of climate change in the Sub-Saharan African region.
References
Allison, E. H., Perry, A. L., Badjeck, M., Adger, W. N., and Brown, K. (2009). Vulnerability of national economies to the impacts of climate change on fisheries. Fish Fish. 10, 173–196. doi: 10.1111/j.1467-2979.2008.00310.x
Antwi-agyei, P., Kpenekuu, F., Hogarh, J. N., Obiri-danso, K., Abaidoo, R. C., Jeppesen, E., et al. (2019). Land use and land cover changes in the Owabi reservoir catchment, Ghana: implications for livelihoods and management. Geosciences 9:286. doi: 10.3390/geosciences9070286
Barnhoorn, I., and van Dyk, C. (2020). The first report of selected herbicides and fungicides in water and fish from a highly utilized and polluted freshwater urban impoundment. Environ. Sci. Pollut. Res. 27, 33393–33398. doi: 10.1007/s11356-020-09930-7
Bates, B. C., Kundzewicz, Z. W., Wu, S., and Palutikof, J. P. (2008). Climate Change and Water. Technical Paper of the Intergovernmental Panel on Climate Change, IPCC Secretariat, Geneva. p. 210.
Bhaga, T. D., Dube, T., Shekede, M. D., and Shoko, C. (2020). Impacts of climate variability and drought on surface water resources in Sub-Saharan Africa using remote sensing: a review. Remote Sens. 12:4184. doi: 10.3390/rs12244184
Bonsor, H. C., Macdonald, A. M., and Calow, R. C. (2010). “Potential impact of climate change on improved and unimproved water supplies in Africa,” in Sustainable Water, eds R. E. Hester and R. M. Harrison (Cambridge: Royal Society of Chemistry), 1–23. doi: 10.1039/9781849732253-00025
Bruhwiler, L., Basu, S., Butler, J. H., Chatterjee, A., Dlugokencky, E., Kenney, M. A., et al. (2021). Observations of greenhouse gases as climate indicators. Clim. Change 165, 1–18. doi: 10.1007/s10584-021-03001-7
Chiarelli, D. D., Davis, K. F., and Rulli, M. C. (2016). Climate change and large-scale land acquisitions in Africa: quantifying the future impact on acquired water resources. Adv. Water Resour. 94, 231–237. doi: 10.1016/j.advwatres.2016.05.016
Deen-Swarray, M., Odularu, G., and Adekunle, B. (2020). “Introduction: nutrition, sustainable agriculture and climate change issues in Africa,” in Nutrition, Sustainable Agriculture and Climate Change in Africa Issues and Innovative Strategies. ed G. Odularu (Cham: Palgrave Macmillan), 1–11.
Descheemaeker, K., Oosting, S. J., Tui, S. H., Masikati, P., Falconnier, G. N., and Giller, K. E. (2016). Climate change adaptation and mitigation in smallholder crop – livestock systems in Sub-Saharan Africa: a call for integrated impact assessments. Region. Environ. Change 16, 2331–2343. doi: 10.1007/s10113-016-0957-8
Food and Agriculture Organisation (FAO) (2005). 31st Session of the Committee on World Food Security 23-26 May 2005: Special Event on Impact of Climate Change, Pests and Diseases on Food Security and Poverty Reduction.
Gezie, M. (2019). Farmer's response to climate change and variability in Ethiopia: a review. Cogent Food Agric. 5:1613770. doi: 10.1080/23311932.2019.1613770
Giorgi, F., Raffaele, F., and Coppola, E. (2018). The response of precipitation characteristics to global warming from global and regional climate projections. Earth Syst. Dyn. 10, 73–89. doi: 10.5194/esd-10-73-2019
Girvetz, E., Ramirez-Villegas, J., Claessens, L., Lamanna, C., Navarro-Racines, C., Nowak, A., et al. (2019). “Future climate projections in Africa: where are we headed?,” in The Climate-Smart Agriculture Papers, eds T. Rosenstock, A. Nowak, and E. Girvetz (Cham: Springer), 15–27. doi: 10.1007/978-3-319-92798-5_5
Glover, S., and Jones, S. (2018). Can commercial farming promote rural dynamism in Sub-Saharan Africa? Evidence from Mozambique. World Dev. 114, 110–121. doi: 10.1016/j.worlddev.2018.09.029
Gomes, C. (2020). Trusted land: land deals, climate vulnerability and adaptation in Northern Mozambique. Clim. Dev. 2020:1–12. doi: 10.1080/17565529.2020.1852909
Gonzalez, P., Tucker, C. J., and Sy, H. (2012). Tree density and species decline in the African Sahel attributable to climate. J. Arid Environ. 78, 55–64. doi: 10.1016/j.jaridenv.2011.11.001
Herrmann, S. M., and Mohr, K. I. (2011). A continental-scale classification of rainfall seasonality regimes in Africa based on gridded precipitation and land surface temperature products. J. Appl. Meteorol. Climatol. 50, 2504–2513. doi: 10.1175/JAMC-D-11-024.1
Intergovernmental Panel on Climate Change (IPCC) (2007). Climate Change 2007: Synthesis Report. Contribution of Working Groups I, II and III to the Fourth Assessment Report of the Intergovernmental Panel on Climate Change [Core Writing Team, eds R. K. Pachauri and L. A. Meyer]. Geneva: IPCC, p. 104.
Intergovernmental Panel on Climate Change (IPCC) (2014). Climate Change 2014: Synthesis Report. Contribution of Working Groups I, II and III to the Fifth Assessment Report of the Intergovernmental Panel on Climate Change [Core Writing Team, eds R. K. Pachauri and L. A. Meyer]. Geneva: IPCC, p. 151.
Jaramillo, J., Muchugu, E., Vega, F. E., Davis, A., Borgemeister, C., and Chabi-Olaye, A. (2011). Some like it hot: the influence and implications of climate change on coffee berry borer (Hypothenemus hampei) and coffee production in East Africa. PLoS ONE 6:e24528. doi: 10.1371/journal.pone.0024528
Leauthaud, C., Duvail, S., Hamerlynck, O., Paul, J.-L., Cochet, H., Nyunja, J., et al. (2013). Floods and livelihoods: the impact of changing water resources on wetland agro-ecological production systems in the Tana River Delta, Kenya. Global Environ. Change 23, 252–263. doi: 10.1016/j.gloenvcha.2012.09.003
Linderman, M., Rowhani, P., Benz, D., Serneels, S., and Lambin, E. F. (2005). Land-cover change and vegetation dynamics across Africa. J. Geophys. Res. 110, 1–15. doi: 10.1029/2004JD005521
Mapulanga, M. A., and Naito, H. (2019). Effect of deforestation on access to clean drinking water. Proc. Natl. Acad. Sci. U.S.A. 116, 8249–8254. doi: 10.1073/pnas.1814970116
Masih, I., Maskey, S., and Trambauer, P. (2014). A review of droughts on the African continent: a geospatial and long-term perspective. Hydrol. Earth Syst. Sci., 18, 3635–3649. doi: 10.5194/hess-18-3635-2014
Merga, L. B., Mengistie, A. A., Faber, J. H., Brink, P. J., and Van, D.en, Group, F. (2020). Trends in chemical pollution and ecological status of Lake Ziway, Ethiopia: a review focussing on nutrients, metals and pesticides. Afr. J. Aquat. Sci. 45, 386–400. doi: 10.2989/16085914.2020.1735987
Mupangwa, W., Walker, S., Masvaya, E., Magombeyi, M., and Munguambe, P. (2016). Rainfall risk and the potential of reduced tillage systems to conserve soil water in semi-arid cropping systems of southern Africa. AIMS Agric. Food 1, 85–101. doi: 10.3934/agrfood.2016.1.85
Ndehedehe, C. E., Agutu, N. O., Ferreira, V. G., and Getirana, A. (2020). Evolutionary drought patterns over the Sahel and their teleconnections with low frequency climate oscillations. Atmos. Res. 233:104700. doi: 10.1016/j.atmosres.2019.104700
Nhemachena, C., Nhamo, L., Matchaya, G., Nhemachena, C. R., Muchara, B., Karuaihe, S. T., et al. (2020). Climate change impacts on water and agriculture sectors in southern Africa: threats and opportunities for sustainable development. Water 12:2673. doi: 10.3390/w12102673
Niang, I., Ruppel, O. C., Abdrabo, M. A., Essel, A., Lennard, C., Padgham, J., et al. (2014). Africa. Climate Change 2014: Impacts, Adaptation and Vulnerability: Part B: Regional Aspects: Working Group II Contribution to the Fifth Assessment Report of the Intergovernmental Panel on Climate Change, p. 1199–1266. doi: 10.1017/CBO9781107415386.002
Nicholls, T., Norgrove, L., and Masters, G. (2008). “Innovative solutions to new invaders: managing agricultural pests, diseases and weeds under climate change,” in Proceedings of “Agriculture in a Changing Climate: The New International Research Frontier,” The ATSE Crawford Fund Fourteenth Annual Development Conference, 3 September 2008, Parliament House, Canberra, Australia, ed A. G. Brown. (Deakin, ACT: The Crawford Fund), 9–14.
Olisah, C., Okoh, O. O., and Okoh, A. I. (2020). Occurrence of organochlorine pesticide residues in biological and environmental matrices in Africa: a two-decade review. Heliyon 6:e03518. doi: 10.1016/j.heliyon.2020.e03518
Ramos, E. P., Howells, M., Sridharan, V., Engström, R. E., Taliotis, C., Mentis, D., et al. (2021). The climate, land, energy, and water systems (CLEWs) framework: a retrospective of activities and advances to 2019. Environ. Res. Lett. 16:033003. doi: 10.1088/1748-9326/abd34f
Rasul, G., and Sharma, B. (2016). The nexus approach to water – energy – food security: an option for adaptation to climate change an option for adaptation to climate change. Clim. Policy 16, 682–702. doi: 10.1080/14693062.2015.1029865
Ringler, C., Zhu, T., Cai, X., Koo, J., and Wang, D. (2010). Climate Change Impacts on Food Security in Sub-Saharan Africa: Insights from Comprehensive Climate Change Scenarios. International Food Policy Research Institute.
Sintayehu, D. W. (2018). Impact of climate change on biodiversity and associated key ecosystem services in Africa: a systematic review. Ecosystem Health Sustainability 4, 225–239. doi: 10.1080/20964129.2018.1530054
Thompson, H. E., Berrang-Ford, L., and Ford, J. D. (2010). Climate change and food security in Sub-Saharan Africa: a systematic literature review. Sustainability 2, 2719–2733. doi: 10.3390/su2082719
Ukpe, U. H., Djomo, C. R. F., Ngo, V. N., Oben, N. E., and Gbadebo, O. (2020). “Crop and livestock production responses to rainfall and temperature variation in West Africa,” in Nutrition, Sustainable Agriculture and Climate Change in Africa Issues and Innovative Strategies, ed: G. Odularu. (Cham: Palgrave Macmillan), 13–28. doi: 10.1007/978-3-030-47875-9_2
UN Department of Economic Social Affairs (2018). Sustainable Development. Available online at: https://sdgs.un.org/goals (accessed March 11, 2021)
UNFCCC (2011). Climate Change Science - The Status of Climate Change Science Today. United Nations Framework Convention on Climate Change, 1–7. Available online at: https://unfccc.int/files/press/backgrounders/application/pdf/press_factsh_science.pdf (accessed November 17, 2020).
Welsch, M., Hermann, S., Howells, M., Rogner, H. H., Young, C., Ramma, I., et al. (2014). Adding value with CLEWS – modelling the energy system and its interdependencies for Mauritius adding value with CLEWS – modelling the energy system and its interdependencies for Mauritius. Appl. Energy 113, 1434–1445. doi: 10.1016/j.apenergy.2013.08.083
Zacarias, D. A. (2020). Global bioclimatic suitability for the fall armyworm, Spodoptera frugiperda (Lepidoptera: Noctuidae), and potential co-occurrence with major host crops under climate change scenarios. Clim. Change 161, 555–566. doi: 10.1007/s10584-020-02722-5
Keywords: climate change, water depletion, land degradation, food security, nexus approach, multidisciplinary approach
Citation: Ofori SA, Cobbina SJ and Obiri S (2021) Climate Change, Land, Water, and Food Security: Perspectives From Sub-Saharan Africa. Front. Sustain. Food Syst. 5:680924. doi: 10.3389/fsufs.2021.680924
Received: 15 March 2021; Accepted: 31 May 2021;
Published: 01 July 2021.
Edited by:
Henry Bikwibili Tantoh, University of South Africa, South AfricaReviewed by:
Olanrewaju Lawal, University of Port Harcourt, NigeriaDessalegn Gemeda, Jimma University, Ethiopia
Copyright © 2021 Ofori, Cobbina and Obiri. This is an open-access article distributed under the terms of the Creative Commons Attribution License (CC BY). The use, distribution or reproduction in other forums is permitted, provided the original author(s) and the copyright owner(s) are credited and that the original publication in this journal is cited, in accordance with accepted academic practice. No use, distribution or reproduction is permitted which does not comply with these terms.
*Correspondence: Samuel Appiah Ofori, ofori496@gmail.com; Samuel Jerry Cobbina, scobbina@uds.edu.gh