- 1Department of Crop Sciences, Tshwane University of Technology, Pretoria, South Africa
- 2Department of Crop Science, University for Development Studies, Tamale, Ghana
- 3Chemistry Department, Tshwane University of Technology, Pretoria, South Africa
Here, we report on the symbiotic N2 fixation and water use efficiency (δ13C) of Kersting's groundnut, an important but underutilized grain legume in Africa, in response to inoculation with Bradyrhizobium strains 3267 and CB756. The 15N natural abundance and xylem ureide techniques were used to quantify N2 fixation. The landraces in this study derived variable levels of their N requirements from symbiosis, which translated into marked differences in the amounts of N-fixed by the landrace–inoculant combinations across the test locations. In most instances, the landrace-strain combinations that elicited higher shoot biomass also recorded greater N-fixed and/or ureide-N in xylem sap as well as grain yield. Although some landraces coupled increased grain yield with higher water use efficiency (shoot δ13C), a trait that could be tapped for crop improvement, others recorded lower yields despite eliciting relatively high shoot δ13C values, indicating genotypic variations in adaptation to the different environments. Grain yield of the test landraces showed marked variation and ranged from 131 to 1349.8 kg ha−1 depending on the landrace–strain combination used and the planting location. The high symbiotic dependence by landraces in this study could explain why Kersting's groundnut thrives in the low nutrient soils that are prevalent in its cultivation areas. These results provide more insights into the literature regarding the Kersting's groundnut–rhizobia symbiosis as well as the crop's water use efficiency.
Introduction
The N2-fixing trait of legumes offers them advantage over other species in N-limiting soils as they can reduce atmospheric N2 into useable forms for plants, through their symbiotic association with rhizobia (Menge and Chazdon, 2016). The symbiotic process can supply all or part of the legume's N needs, and even contribute to the soil N reserves for use by subsequent non-legume crops (Preissel et al., 2015). The Kersting's groundnut is an underutilized African legume that thrives in arid environments of Africa (Mergeai, 1993) and capable of N2 fixation when in symbiosis with soil rhizobia (Dakora, 1998). As with most tropical and sub-tropical legumes, the products of N2-fixation in the root nodules of Kersting's groundnut are exported as ureides (allantoin and allantoic acid) to the shoots via the xylem stream (Dakora et al., 1992; Dakora, 1998). Thus, the concentration of ureides relative to nitrate and amino-N in readily sampled xylem sap of Kersting's groundnut could be used to assess the crop's dependence on N2 fixation for its N nutrition (Herridge and Peoples, 1990; Unkovich et al., 2008). Like most neglected grain legumes, the crop can produce substantial grain yield without fertilization even in nutrient limiting environments (Bayorbor et al., 2010; Mohammed et al., 2018). The seeds of Kersting's groundnut contain a high proportion of protein (21.3%), carbohydrates (up to 73.3%), and essential amino acids (Ayenan and Ezin, 2016). The symbiotic, nutritional, and medicinal values of Kersting's groundnut make it an important food security crop (Amuti, 1980; Assogba et al., 2016; Akohoué et al., 2018). Since an earlier report that Kersting's groundnut can nodulate with Bradyrhizobium sp. strain CB756 and exhibit nitrate tolerant symbiosis in sand culture (Dakora, 1998), little has been done on the N2-fixing potential of Kersting's groundnut under field conditions (Mohammed et al., 2018). This is despite the current efforts at harnessing the benefits of symbiotic N2 fixation for agriculture as well as the renewed interest in rhizobial inoculant production and usage (Stephens and Rask, 2000; Catroux et al., 2001; Hungria et al., 2006; Rebah et al., 2007).
Field inoculation of legumes with rhizobia often aims at increasing plant growth and grain yield at a sustainable cost when compared to the use of fertilizers. A known example of inoculation success is the story of soybean in Brazil, where the government is reported to have saved about US$ 10 billion on fertilizers in one cropping season (Dwivedi et al., 2015). However, there has also been inoculation failures in the field due to the presence of native soil rhizobia with higher competitiveness for nodule occupancy (Ulzen et al., 2016; Mohammed et al., 2018). Legume inoculation in the field often does not yield the desired response in soils where the resident rhizobia are very competitive and not limited by numbers (Catroux et al., 2001). It is therefore necessary to test the response of legumes to inoculation with commercially available rhizobial inoculants in the presence of native soil rhizobia to check the quality of introduced strains in different agro-environments. Kersting's groundnut is an important food security crop commonly grown in rural Africa. There is therefore the need to assess its symbiotic performance in the field in response to inoculation with different rhizobial inoculants.
Although Kersting's groundnut is reported to be drought-tolerant (Mergeai, 1993; Ayenan and Ezin, 2016), little information exists on its water use efficiency measured using reliable techniques. During C3 plant photosynthesis, carbon isotope discrimination against 13CO2 in favor of 12CO2 (expressed as δ13C) is directly related to water use efficiency (Farquhar et al., 1989). Under water-limiting conditions when there is soil moisture deficit, plants with high water use efficiency exhibit reduced discrimination against 13CO2, resulting in higher δ13C values. Conversely, plants accessing adequate soil moisture tend to display low water use efficiency through increased 13C discrimination, which results in reduced δ13C values (Farquhar et al., 1989; Wang et al., 2016). Thus, carbon isotope discrimination has become a useful tool for assessing plant water use efficiency in nodulated legumes (Mohale et al., 2014; Mapope and Dakora, 2016), as well as in ecological settings (Wang et al., 2016). In this study, we measured symbiotic N2 fixation and water use efficiency in field-grown Kersting's groundnut in response to inoculation with Bradyrhizobium sp. strains CB756 and 3267. The ureide and 15N natural abundance techniques were used to assess N2 fixation in the Kersting's groundnut landraces, while the 13C/12C isotopic analysis was used to measure plant water use efficiency.
Materials and Methods
Experimental Site Description
The study was carried out at three locations (namely, Nyankpala, Savelugu, and Gbalahi) in the Northern Region of Ghana. Nyankpala is located on latitude 9.404 and longitude −0.982, while Gbalahi is located on latitude 9.437 and longitude −0.734. Savelugu, on the other hand, is located on latitude 9.569 and longitude −0.830. The Nyankpala site had been fallowed for over 5 years, while the Savelugu site was cultivated to okra in the previous year. The field at Gbalahi was planted to maize in the year before this experiment. Prior to planting, soil samples were cored at several points across plots, bulked, air-dried, and sieved (2 mm). Soil sub-samples were taken for analysis of pH (H2O), %C (Walkley and Black, 1934), P (Bray and Kurtz, 1945), B, Ca, Cu, Fe, K, Mg, Mn, Na, and S (ammonium acetate method) and total N using Kjeldahl digestion.
The Northern Region has a unimodal rainfall of 800–1200 mm distributed between April and October each year (Owusu and Waylen, 2013). The total annual rainfall during the study period in 2013 was 1081.5 mm at Nyankpala and 1009.7 mm at Savelugu. Due to broken equipment, rainfall data at Gbalahi (857.3 mm) were collected from January to October 2013. However, the total rainfall from planting till sampling (early podding stage of Kersting's groundnut) was 481 mm at Nyankpala, 471 mm at Savelugu, and 305 mm at Gbalahi. The mean weekly rainfall distribution at the three locations during the study period in 2013 is shown Figure 1.
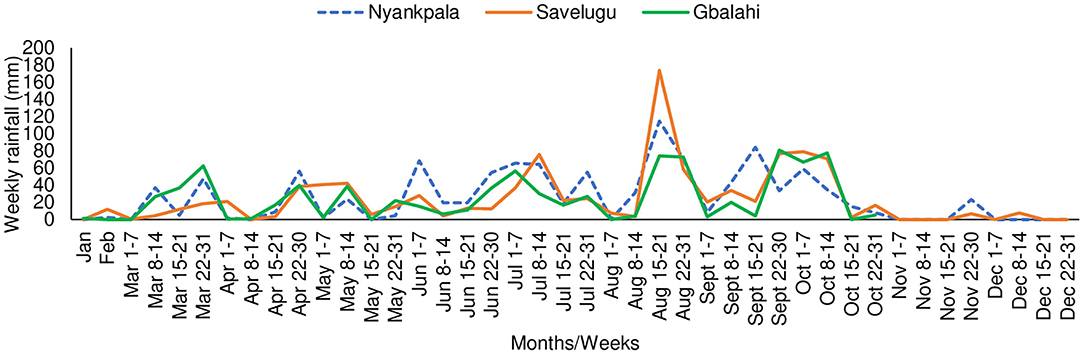
Figure 1. Weekly rainfall distribution at Nyankpala, Savelugu, and Tamale (Gbalahi) during the study in 2013.
Source of Seeds, Experimental Design, and Planting
The eight Kersting's groundnut landraces [Puffeun, Boli, Dowie, Funsi, Heng Milk Mottled (Heng MM), Heng Red Mottled (Heng RM), Nakori, and Sigiri] used in this study were sourced from the University for Development Studies, Tamale, Ghana. The seeds were originally collected from different villages within the Upper West Region of Northern Ghana (Bayorbor et al., 2010). The landraces together with two Bradyrhizobium inoculants (Bradyrhizobium sp. strains 3267 and CB756) and an uninoculated control, which gave a total of 24 treatment combinations, were laid in a randomized complete block design at each location with four replicate plots per treatment. Planting was done on July 2, 2013 at Nyankpala, July 10, 2013, at Savelugu, and July 16, 2013 at Gbalahi. Seeds were sown in plots measuring 2.4 m × 2 m at a spacing of 60 cm between rows and 20 cm between plants, with a 1-m path between plots and 1.5 m between blocks. Weeding was done when necessary, using a hand hoe.
Seed Inoculation With Bradyrhizobium Strains
Seed inoculation was done in the shade using 10 g inoculant per kg seed, which yielded 108 rhizobial cells g−1 of inoculant. To avoid cross contamination, uninoculated/control plots were planted first, followed by inoculated treatments with the individual Bradyrhizobium strains. Bradyrhizobium sp. strain CB756 is a commercial cowpea inoculant strain originally isolated from the root nodules of Macrotyloma africanum in Zimbabwe (Blumenthal and Staples, 1993) and was obtained from Stimuplant, South Africa. The Bradyrhizobium sp. strain BR 3267 is a commercial inoculant from Brazil, which was obtained from the CSIR-Savanna Agricultural Research Institute, Ghana.
Plant Sampling and Processing
Plants were sampled at early podding at 74 days after planting (DAP) at Nyankpala and Savelugu, and 75 DAP at Gbalahi. Five plants were randomly dug up from each plot at the experimental sites, and separated into shoots, roots, and nodules. The shoots were oven-dried at 65°C for 48 h and weighed to determine shoot biomass. The shoots were finely ground (0.50 mm sieve) for 15N and 13C isotopic analysis. The nodules were counted, similarly oven-dried, and weighed to determine nodule dry matter. Shoots of non-legume reference plants were concurrently sampled from each location, and similarly processed for 15N analysis (Supplementary Table 1).
15N/14N Isotopic Analysis of Plant Shoots
Ground shoot samples of both legume and reference plants were weighed (2.0–2.5 mg) and each sample was fed into a Carlo Erba NA1500 elemental analyzer (Fisons Instruments SpA, Strada, Rivoltana, Italy) coupled to a Finnigan MAT252 mass spectrometer (Fisons Instrument SpA, Strada, Rivoltana, Italy) via conflo II open-split device to measure 15N/14N isotopic composition, as described by Mapope and Dakora (2016). The δ15N of shoot samples was calculated as Unkovich et al. (2008):
where the 15N/14Nsample is the abundance ratio of 15N and 14N in the sample, and 15N/14Natm, the abundance ratio of 15N and 14N in the atmosphere.
Shoot N
The %N of shoot samples was obtained directly from the mass spectrometer, and shoot N content estimated as the product of shoot dry matter and %N (Pausch et al., 1996).
Percent N Derived From Fixation and N-Fixed
Shoot percent N derived from N2 fixation (%Ndfa) was calculated as Shearer and Kohl (1986):
where δ15Nref is the 15N natural abundance of reference plant, δ15Nleg is the 15N natural abundance of legume, and the B value is the 15N natural abundance of the shoot of Kersting's groundnut plants completely dependent on N2 fixation for their N nutrition. The B value (−3.84‰) used in this study was earlier determined by inoculating the Puffeun and Funsi landraces with Bradyrhizobium sp. strain CB756 in the glasshouse (Mohammed et al., 2018). The mean δ15N of all reference plants collected from each location (Supplementary Table 1) was used to calculate the %Ndfa of landraces per study site.
The N-fixed by Kersting's groundnut was calculated as Maskey et al. (2001):
The soil N uptake by legume plants was determined as the difference between shoot N content and the amount of N-fixed.
Shoot 13C/12C Isotopic Analysis
The ground shoot samples of Kersting's groundnut were similarly subjected to 13C/12C isotopic analysis, as described for 15N/14N. The 13C natural abundance (δ13C) was calculated as Farquhar et al. (1989):
where 13C/12Csample is the isotopic ratio of the plant sample and 13C/12Cstandard is the isotopic ratio of PDB, a universally accepted standard from Belemnite Pee Dee limestone formation (Craig, 1957).
Collection of Xylem Sap
Xylem sap was collected from the same plants that were sampled and processed for 15N and 13C isotopic analysis. After decapitating five plants at crown level for shoot samples from each plot, the root-bleeding xylem sap was collected into 2 ml Eppendorf tubes using clean glass Pasteur pipettes. The xylem sap samples were stored at −20°C until analysis for N solutes (ureide-N, nitrate-N, and amino-N).
Measurement of Xylem N Solutes
Ureide Concentration in Xylem Sap
The concentration of ureides in xylem sap was colorimetrically determined, as described by Young and Conway (1942) or Unkovich et al. (2008). Varying concentrations of allantoin (0.1, 0.02, 0.04, 0.1, and 0.15 mM) were prepared as standards for estimating ureides in xylem sap, after colorimetric assay (Young and Conway, 1942).
Nitrate Level in Xylem Sap
Nitrate-N in xylem sap was determined using the salicylic acid method (Cataldo et al., 1975), as described by Unkovich et al. (2008). Again, varying concentrations of KNO3 (0, 1.25, 2.5, 5, 10, and 15 mM) were used as standards to construct curves for extrapolating the concentrations of nitrate from xylem sap.
Total Amino Acid Determination
Amino acids in xylem sap was measured using the ninhydrin method (Yemm and Cocking, 1955; Unkovich et al., 2008). As with ureide and nitrate determinations, a standard curve was prepared from assaying different known concentrations of the amino acids glutamine and asparagine (0, 0.1, 0.2, 0.4, and 1 mM).
Relative Ureide Nitrogen
The relative ureide-N in xylem sap (or relative ureide abundance) was calculated as Herridge et al. (1990):
where a, b, and c are the molar concentrations of ureides (ureides contain four nitrogen atoms per molecule), nitrate-N, and amino-N, respectively (Unkovich et al., 2008).
Determination of Grain Yield
At maturity, which coincided with 125, 122, and 120 DAP at Nyankpala, Savelugu, and Gbalahi, respectively, 20 plants were harvested from the inner rows of each plot for determination of grain yield. The pods were detached from plants, air-dried to 15% moisture content, and threshed to obtain seeds. The seeds were weighed, and grain yield expressed per hectare.
Results
Chemical Properties of Soils
The bulk soils collected from Nyankpala, Savelugu, and Gbalahi before planting, respectively, had pH (H2O) 5.93, 6.08, and 6.3, and in that order contained 0.04, 0.01, and 0.08 mg kg−1 B, 0.40, 0.029, and 0.41% C, 250, 272, and 460 mg kg−1 Ca, 0.30, 0.20, and 0.78 mg kg−1 Cu, 42.0, 23.96, and 46.69 mg kg−1 Fe, 48, 29, and 58 mg kg−1 K, 55.2, 51.6, and 130.8 mg kg−1 Mg, 131.4, 66.46, and 65.51 mg kg−1 Mn, 7, 4, and 17 mg kg−1 Na, 7, 5, and 7 mg kg−1 P, 2.7, 2.1, and 2.2 mg kg−1 S, as well as 0.023, 0.016, and 0.031% N.
Shoot δ15N of Non-legume Reference Plants
The δ15N of reference plants used in estimating %Ndfa by Kersting's groundnut landraces is shown in Supplementary Table 1. The δ15N of reference plants ranged from +1.83‰ to +4.57‰ at Nyankpala (mean = +3.01‰), +2.52‰ to +3.51‰ at Savelugu (mean = +2.95‰), and +2.15‰ to 5.01‰ at Gbalahi (mean = +3.26‰). The mean δ15N of reference plants from each study site was used to calculate the %Ndfa of Kersting's groundnut plants from that location (Supplementary Table 1).
N2 Fixation and Water Use Efficiency of Kersting's Groundnut at Nyankpala
Main Effect of Landrace on Plant Growth, Symbiotic Parameters, Grain Yield and Shoot δ13C
A two-way ANOVA of data from Nyankpala showed that the main effect of landrace was significant (p ≤ 0.05) for nodule dry matter, shoot biomass, and N content at the site (Table 1). Landraces Heng RM, Boli, Dowie, and Heng MM recorded significantly higher nodule mass compared to the other landraces at Nyankpala (Table 1). The landraces Heng MM and Heng RM (with high nodule mass) together with Nakori and Sigiri (with lower nodule mass) recorded greater shoot biomass relative to the other landraces at that site (Table 1). The %N and C/N ratio of shoots were however similar for all the Kersting's groundnut landraces, with values ranging from 2.4 to 2.8% for N and 14.7 to 17.1 g g−1 for C/N ratio (Table 1). Shoot N accumulation (N content) was greater in landraces Heng RM and Nakori, followed by Heng MM, Dowie, and Sigiri (Table 1).
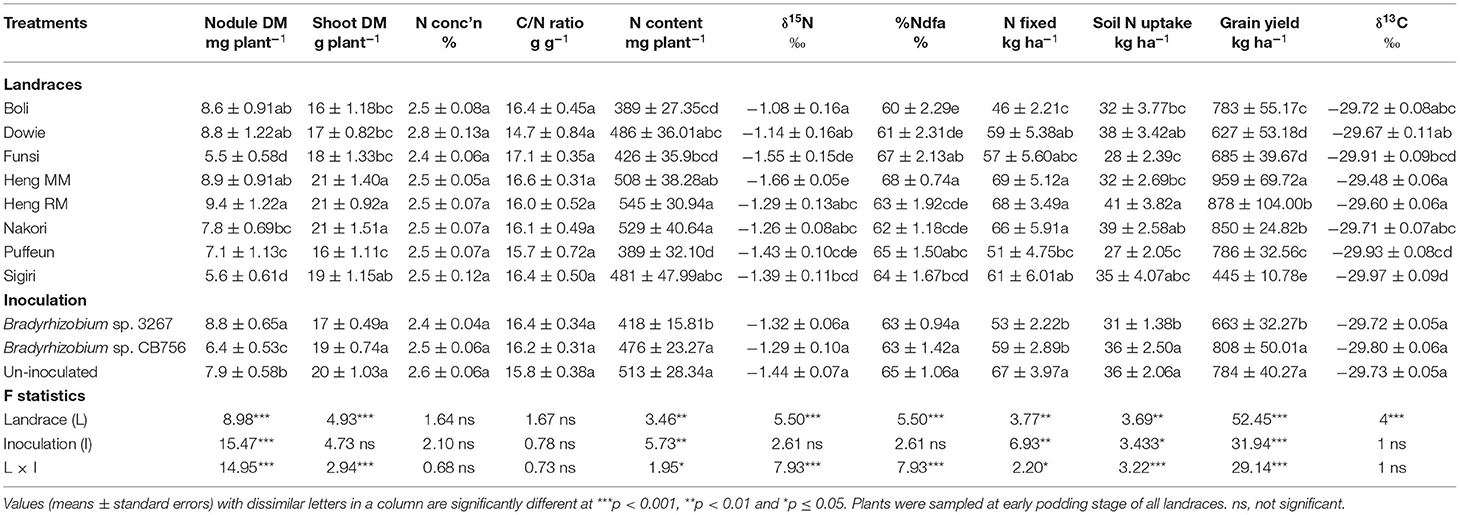
Table 1. Plant growth, N2-fixing parameters, grain yield, and δ13C of eight Kersting's groundnut landraces planted at Nyankpala in the northern Region of Ghana in 2013.
From the 15N analysis, the landraces Heng MM and Funsi had the least shoot δ15N values at Nyankpala, while Boli, Dowie, Heng RM and Nakori recorded higher δ15N values (Table 1). As a result, the landraces Heng MM and Funsi with lower shoot δ15N recorded relatively higher %Ndfa values (68 and 67%, respectively), followed by Puffeun (65%) and then Sigiri (64%) (Table 1). However, landraces Heng RM, Heng MM, and Nakori showed much higher amounts of N-fixed due to greater shoot biomass and N content at Nyankpala. Dowie, Sigiri, and then Funsi were next with high N-fixed values (Table 1), with landrace Boli showing the lowest N-fixed from its very high shoot δ15N and low %Ndfa at the Nyankpala site (Table 1).
Soil N uptake by landraces at Nyankpala also varied, with higher values found in Heng RM followed by Dowie, Nakori, and Sigiri (Table 1). Landraces Puffeun and Funsi recorded the least soil N uptake at Nyankpala. Here, grain yield was highest in landraces Heng MM (959 kg ha−1) followed by Heng RM (878 kg ha−1) and Nakori (850 kg ha−1), and least in Sigiri (445 kg ha−1) (Table 1).
The landraces Heng MM and Heng RM, which produced high grain yield, were also found to record greater shoot δ13C values (−29.48‰ and −29.60‰, respectively) at Nyankpala. Other landraces with high δ13C values at Nyankpala include Dowie (−29.67‰), Boli (−29.72‰) and Nakori (−29.71‰), while Sigiri had the least δ13C value (−29.97‰) at that site (Table 1).
Main Effect of Inoculation on Plant Growth, Symbiotic Parameters, Grain Yield and Shoot δ13C
The main effect of inoculation was also significant for nodule dry matter, N content, N-fixed, soil N uptake, and grain yield, while the overall shoot dry matter, %N, C/N ratio, and δ13C were unaltered by inoculation at Nyankpala (Table 1). Here, the highest nodule mass was obtained by bacterial inoculation of the landraces with strain 3267 followed by non-inoculation. The least nodule mass was recorded in plots inoculated with the Bradyrhizobium sp. strain CB756 (Table 1). Shoot N content was however greater in uninoculated plots as well as those inoculated with Bradyrhizobium sp. strain CB756 and lowest in plots inoculated with strain 3267. Overall, non-inoculation resulted in greater N-fixed compared to inoculation of the test landraces with either Bradyrhizobium strains at Nyankpala (Table 1). As observed with shoot N content, the average soil N uptake and grain yield of the plants at Nyankpala were higher in uninoculated plots or plots inoculated with strain CB756 relative to inoculation with strain 3267 at the site (Table 1). However, there was no overall effect of inoculation on shoot δ13C at Nyankpala.
Main Effect of Landrace on the Concentrations of Xylem N Solutes
There was a significant effect of landrace on the concentrations of N solutes in the xylem sap of the test Kersting's groundnuts grown at Nyankpala (Table 2). The landraces Heng MM and Nakori (with high N-fixed from 15N natural abundance) as well as Boli (with low N-fixed), all recorded higher concentrations of ureide and amino acids, but lower nitrate-N in xylem sap relative to the other landraces at the site (Table 2). As a result, those landraces exhibited higher RU-N in xylem sap at Nyankpala when compared to the other landraces at that site (Table 2).
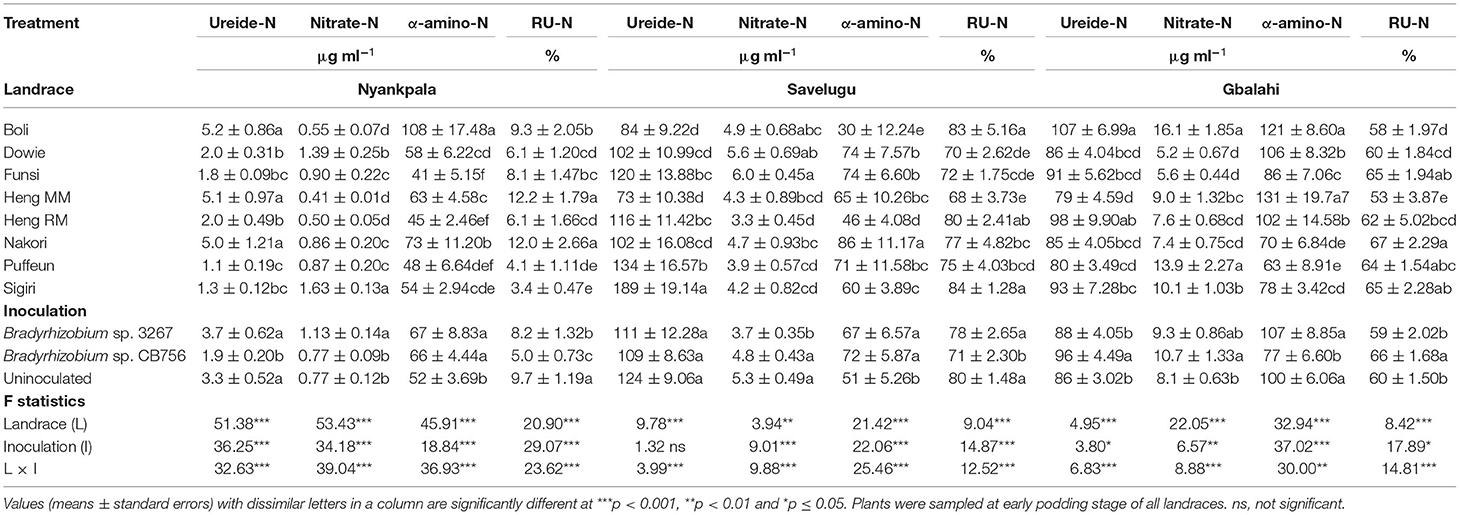
Table 2. Ureide, nitrate, α-amino-N concentrations and relative ureide-N (RU-N) in xylem sap of eight Kersting's groundnut landraces planted at Nyankpala, Savelugu, and Gbalahi in 2013.
Main Effect of Inoculation on the Concentrations of Xylem N Solutes
There was a significant effect of inoculation on the concentrations of ureide, nitrate, and amino acids in the xylem sap of the test Kersting's groundnuts grown at Nyankpala (Table 2). Here, non-inoculation as well as bradyrhizobial inoculation with strain 3267 increased the concentration of ureide in xylem sap relative to inoculation with strain CB756 (Table 2). The average concentration of nitrate in the xylem sap of the Kersting's groundnuts was higher with Bradyrhizobium strain 3267 inoculation relative to the control or inoculation with strain CB756. Bacterial inoculation with either strains significantly increased the overall concentration of amino acids in xylem sap relative to non-inoculation at Nyankpala (Table 2). The uninoculated control showed greater overall RU-N at Nyankpala, followed by plots inoculated with strain 3267 (Table 2).
Effects of Landrace × Inoculation Interactions at Nyankpala
The landrace × inoculation interaction effect was significant for all measured parameters at Nyankpala, except for %N, C/N ratio, and shoot δ13C values (Tables 1, 2). For brevity, the interaction effects are described for shoot biomass, N-fixed, and grain yield for landraces that showed consistent trends for those parameters. As shown in Figures 2D–F, non-inoculation led to greater shoot biomass in landraces Dowie, Heng MM, Nakori, and Puffeun relative to inoculation of those landraces with either Bradyrhizobium strains (Figure 2D). As a result, N-fixed was greater in uninoculated plants of those landraces (although not significant for Nakori) and resulted in higher grain yield relative to bacterial inoculation of those landraces with either strains (Figures 2E,F).
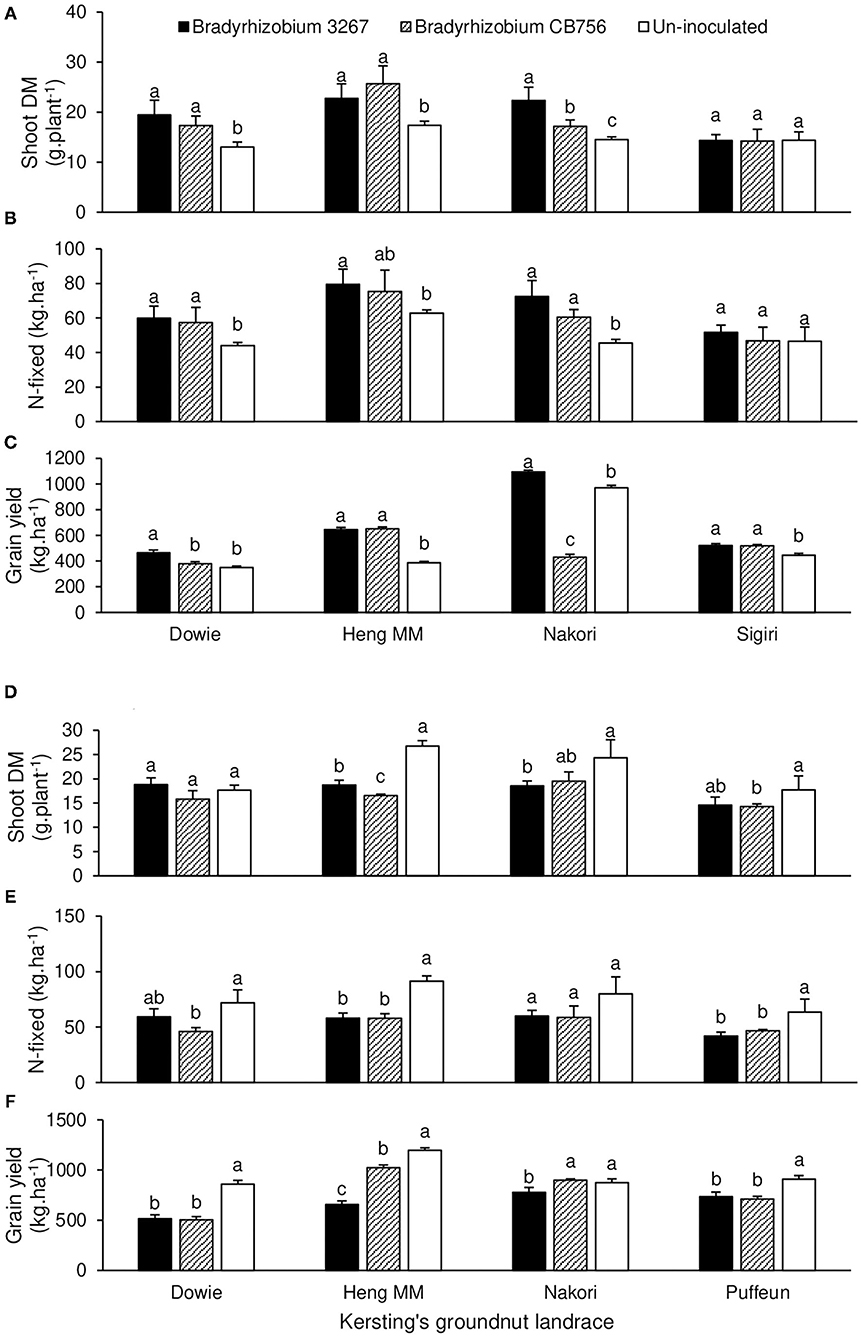
Figure 2. The interactive effect of landrace × inoculation on (A) shoot dry matter at Savelugu, (B) N-fixed as Savelugu, (C) grain yield at Savelugu as well as the interactive effect of landrace × inoculation on (D) shoot dry matter at Nyankpala, (E) N-fixed at Nyankpala, and (F) grain yield at Nyankpala in 2013. For each landrace, bars with dissimilar letters are significantly different (p ≤ 0.05).
N2 Fixation and Water Use Efficiency at Savelugu
Main Effect of Landrace on Plant Growth, Symbiotic Parameters, Grain Yield, and δ13C
At Savelugu, a two-way ANOVA revealed significant effect of landrace on nodule dry matter, shoot dry matter, N content, δ15N, %Ndfa, N-fixed, soil N uptake, grain yield, and shoot δ13C of Kersting's groundnut (Table 3). The landrace Sigiri recorded the highest dry nodule mass followed by Boli, Heng RM, and Puffeun, which recorded relatively lower but similar nodule mass. The landrace Dowie recorded the least nodule dry matter at the Savelugu site (Table 3).
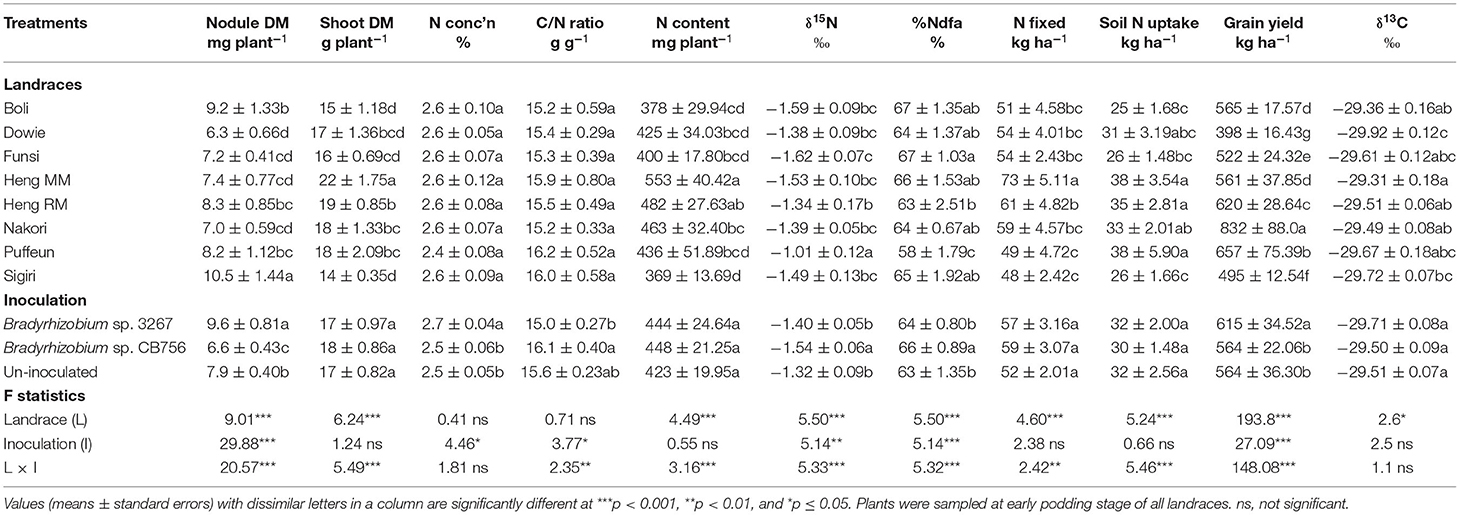
Table 3. Plant growth, N2-fixing parameters, grain yield, and δ13C of eight Kersting's groundnut landraces planted at Savelugu in the northern Region of Ghana in 2013.
The landrace Heng MM had the highest shoot biomass at Savelugu followed by Dowie, Heng RM, Nakori, and Puffeun, which recorded relatively lower but similar shoot biomass at that site. The least shoot biomass was recorded in Boli and Sigiri despite their relatively high nodule mass at the Savelugu site (Table 3).
The shoot N concentration (%N) and C/N ratio were each similar between and among the landraces grown at Savelugu, with values of %N ranging between 2.4 and 2.6%, while C/N ratio ranged between 15.2 and 16.2 g g−1 at that site. Shoot N content closely mirrored patterns of shoot biomass, with landraces Heng MM and Heng RM recording higher N content at the site and Sigiri the least. Other landraces with lower N content at Savelugu were Boli, Dowie, Funsi, and Puffeun (Table 3). Landrace Funsi recorded the least δ15N value, and hence greater %Ndfa (67%) at Savelugu while Puffeun recorded the highest δ15N and the least %Ndfa (58%) at that site (Table 3). N-fixed at Savelugu was highest in Heng MM due to its greater shoot biomass and high %Ndfa, followed by the landrace Heng RM. The landraces Sigiri and Puffeun recorded the least N-fixed at the site (Table 3). Together with Puffeun and Nakori, landraces Heng MM and Heng RM, which recorded higher N-fixed, also showed greater soil N uptake at Savelugu. Here, the lowest values of soil N uptake were recorded in landraces Boli and Sigiri (Table 3).
The highest grain yield was recorded in landrace Nakori (832 kg ha−1), followed by Puffeun (657 kg ha−1), Heng RM (620 kg ha−1), Boli (565 kg ha−1), Heng MM (561 kg ha−1), and then Funsi (522 kg ha−1) in that order (Table 3). The least values for grain yield at Savelugu were recorded in landraces Sigiri (494.6 kg ha−1) and then Dowie (398 kg ha−1). Here, shoot δ13C was highest in Heng MM followed by landraces Boli, Heng RM, and Nakori with similar values, and least in Dowie (Table 3).
Main Effect of Inoculation on Plant Growth, Symbiotic Parameters, Grain Yield, and δ13C
The main effect of inoculation at Savelugu was also significant for nodule dry matter, %N, C/N ratio, δ15N, %Ndfa, and grain yield but not δ13C (Table 3). Here, plants inoculated with Bradyrhizobium sp. strain 3267 had the highest nodule mass followed by the control plants. Seed inoculation with Bradyrhizobium sp. strain 3267 increased overall %N in shoots of Kersting's groundnut and decreased C/N ratio relative to non-inoculation or inoculation with strain CB756 (Table 3). Non-inoculation or bacterial inoculation with strain 3267 increased shoot δ15N values and decreased overall %Ndfa of Kersting's groundnut at Savelugu relative to bacterial inoculation with strain CB756. Overall, N-fixed and soil N uptake were unaltered by inoculation relative to the control (Table 3). Grain yield was increased by inoculation with Bradyrhizobium sp. strain 3267 when compared to non-inoculation or inoculation with Bradyrhizobium sp. strain CB756 (Table 3).
Main Effect of Landrace on the Concentration of Xylem N Solutes
There were significant differences in the concentrations of N solutes in the xylem sap of the landraces grown at Savelugu (Table 2). The landraces Puffeun and Sigiri recorded higher concentrations of ureide-N in xylem sap and relatively lower nitrate-N at the site (Table 2). The landrace Nakori exhibited the highest concentration of amino-N in xylem sap at Savelugu despite its relatively lower ureide-N at the site. However, the landraces Sigiri, Boli, and Heng RM recorded greater RU-N at Savelugu relative to the other landraces (Table 2).
Main Effect of Inoculation on the Concentration of Xylem N Solutes
There was a significant effect of inoculation on the xylem ureide concentration at Savelugu (Table 2). However, together with non-inoculation, bacterial inoculation with Bradyrhizobium sp. strain CB756 increased xylem nitrates when compared to inoculation of the landraces with strain BR 3267. On the other hand, bradyrhizobial inoculation with either Bradyrhizobium strains resulted in a greater concentration of amino acids in the xylem sap of the Kersting's groundnuts compared to the uninoculated control (Table 2). Overall, RU-N was markedly greater in plants sampled from uninoculated plots and those from plots inoculated with Bradyrhizobium sp. strain 3267 (Table 2).
Effect of Landrace × Inoculation Interactions at Savelugu
At Savelugu, the landrace × inoculation interaction was significant for shoot biomass, N-fixed, grain yield, and all other measured parameters, except for shoot %N and δ13C values (Table 3). Here, bacterial inoculation with either Bradyrhizobium sp. strain BR 3267 or Bradyrhizobium sp. CB756 increased shoot biomass in landraces Dowie, Heng MM, and Nakori relative to non-inoculation (control), although the parameter was unaltered in landrace Sigiri (Figure 2A). As a result, N-fixed was markedly increased by inoculation of those landraces with either Bradyrhizobium strains at Savelugu relative to the control (Figure 2B). Despite the increased shoot biomass and N-fixed in plants of Dowie inoculated with either bacterial strains, grain yield was only increased in plants inoculated with Bradyrhizobium sp. strain BR 3267 (Figures 2A–C). However, inoculation with either bacterial strains increased grain yield in Heng MM when compared to non-inoculation. On the other hand, non-inoculation or bacterial inoculation with Bradyrhizobium sp. strain BR 3267 increased grain yield in Nakori when compared to inoculation with Bradyrhizobium sp. strain CB756 (Figure 2C). Although bradyrhizobial inoculation did not markedly alter shoot biomass and N-fixed in Sigiri, it resulted in higher grain yields than the control (Figures 2A–C).
N2 Fixation and Water Use Efficiency at Gbalahi
Main Effect of Landrace on Plant Growth, Symbiotic Parameters, Grain Yield, and δ13C
A two-way ANOVA of the data collected from Gbalahi revealed significant effect of landrace on nodule dry matter, shoot dry matter, N content, δ15N, %Ndfa, N-fixed, soil N uptake, grain yield, and shoot δ13C (Table 4). Here, landrace Sigiri recorded the highest nodule mass followed by Boli, while Funsi and Nakori recorded the least values of the parameter (Table 4).
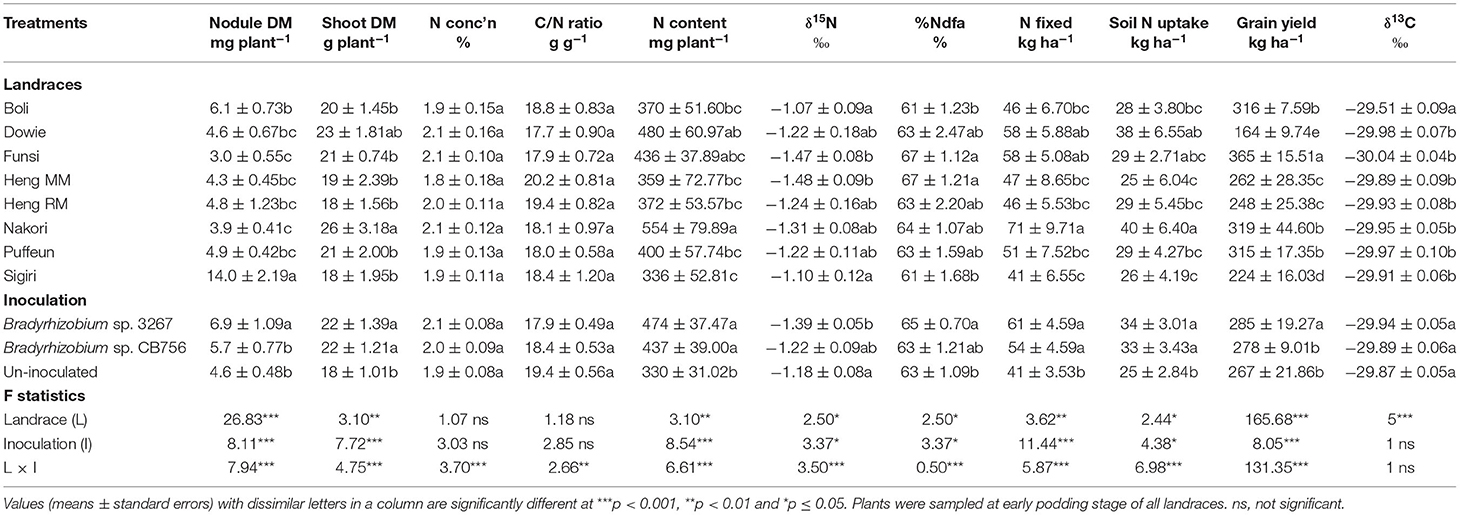
Table 4. Plant growth, N2-fixing parameters, grain yield, and δ13C of eight Kersting's groundnut landraces planted at Gbalahi in the northern Region of Ghana in 2013.
Shoot biomass was highest in the Nakori landrace followed by Dowie, while the remaining landraces had lower but similar shoot biomass at the Savelugu site. There were however no significant differences in shoot N and C/N ratio of Kersting's groundnut landraces at Gbalahi. The %N values ranged between 1.8 and 2.1% while C/N ratio ranged between 17.7 and 20.2 g g−1 (Table 4). Shoot N content was highest in the landrace Nakori followed by Dowie while the least N content was recorded in landrace Sigiri (Table 4).
The least δ15N values were recorded in the Funsi and Heng MM landraces, while Boli and Sigiri recorded the highest (Table 4). The remaining landraces recorded slightly higher but similar δ15N to Boli and Sigiri (Table 4). As a result, %Ndfa was greater in the Funsi and Heng MM landraces at Gbalahi, and lower in Boli and Sigiri at the site. The remaining landraces recorded relatively lower but similar %Ndfa to those of Funsi and Heng MM (Table 4). N-fixed was highest in Nakori followed by the landraces Dowie and Funsi. The least N-fixed at Gbalahi was recorded in landrace Sigiri despite its high nodule mass (Table 4).
The landrace Nakori recorded the highest soil N uptake followed by Dowie and Funsi, while Heng MM and Sigiri recorded the least (Table 4). Grain yield at Gbalahi was highest in Funsi (365 kg ha−1), followed by Boli (316 kg ha−1), Nakori (319 kg ha−1), and Puffeun (315 kg ha−1) with relatively lower grain yield. The least grain yield at Gbalahi was recorded in the landraces Dowie (164 kg ha−1) and Sigiri (224 kg ha−1). Other landraces with lower grain yield at Gbalahi were Heng MM and Heng RM (Table 4). Here, shoot δ13C was highest in the landrace Boli (−29.51‰) and least but similar in the remaining landraces (−29.89‰ to −30.04‰) (Table 4).
Main Effect of Inoculation on Plant Growth, Symbiotic Parameters, Grain Yield, and δ13C
As in the other locations, there was a significant effect of inoculation on nodule dry matter, shoot dry matter, N content, δ15N, %Ndfa, N-fixed, soil N uptake, and grain yield but not δ13C at Gbalahi (Table 4). Whereas inoculation with Bradyrhizobium sp. strain BR 3267 increased nodule mass over non-inoculation or inoculation with Bradyrhizobium sp. strain CB756, bacterial inoculation with either strains increased shoot biomass and N content over the control (Table 4). The overall δ15N value was however decreased by inoculation with Bradyrhizobium sp. strain BR 3267 relative to non-inoculation. As a result, bacterial inoculation with Bradyrhizobium sp. strain BR 3267 increased the overall %Ndfa compared to non-inoculation at Gbalahi (Table 4). Bacterial inoculation with either strains also increased N-fixed and soil N uptake compared to non-inoculation. There was no marked effect of inoculation on overall shoot δ13C at Gbalahi (Table 4). However, overall grain yield was only increased by bacterial inoculation with strain 3267 compared to non-inoculation or inoculation with Bradyrhizobium sp. strain CB756 (Table 4).
Main Effect of Genotype on the Concentrations of Xylem N Solutes
There was a marked effect of landrace on the concentrations of xylem N solutes (Table 2). The landrace Boli (with relatively lower N-fixed from 15N natural abundance) together with Heng RM recorded the highest concentration of ureide in xylem sap. However, the landrace Boli together with Puffeun recorded greater concentration of nitrate in xylem sap at the site relative to the other landraces (Table 2). However, the concentration of amino acids was greater in the xylem sap samples of the landraces Boli and Heng MM, followed by Dowie and Heng RM (Table 2). With high N-fixed values from 15N natural abundance, the landrace Nakori also recorded the highest RU-N, followed by landraces Funsi, Sigiri, and Puffeun (Table 2).
Main Effect of Inoculation on the Concentrations of Xylem N Solutes
Bradyrhizobial inoculation with strain CB756 increased the concentration of ureides and nitrate in xylem sap samples relative to non-inoculation (control) or inoculation with strain BR 3267 (Table 2). However, non-inoculation or bacterial inoculation with Bradyrhizobium sp. strain BR 3267 each increased the concentration of amino acids in xylem sap when compared to inoculation with Bradyrhizobium sp. strain CB756. Consequently, bacterial inoculation with strain CB756 resulted in greater RU-N due to increased concentration of ureides and reduced concentration of amino acids (Table 2).
Effect of Landrace × Inoculation Interactions at Gbalahi
At Gbalahi, there was a significant effect of landrace × inoculation interaction on shoot biomass, ureide concentration in xylem sap, relative ureide-N (RU-N), N-fixed, grain yield, and all other parameters, except for shoot δ13C (Tables 2, 4). Here, bradyrhizobial inoculation with strain BR 3267 or CB756 each increased shoot biomass relative to the control in landraces Heng MM and Sigiri (Figure 3A). However, bacterial inoculation with Bradyrhizobium sp. strain BR 3267 increased shoot biomass over inoculation with Bradyrhizobium sp. strain CB756 in Funsi, and over non-inoculation or inoculation with strain CB756 in landrace Puffeun (Figure 3A). Non-inoculation (control) or bacterial inoculation of Heng RM with Bradyrhizobium sp. strain CB756 each resulted in higher shoot biomass relative to inoculation of the same landrace with strain BR 3267 (Figure 3A). The landrace/inoculant strain combinations that elicited higher shoot biomass mostly recorded higher ureide concentration and RU-N in xylem sap, albeit a few exceptions (Figures 3A–C). For example, despite the lower shoot biomass of control plants of Puffeun and those inoculated with Bradyrhizobium sp. strain CB756, ureide-N and RU-N were unaltered by inoculation of the landrace (Figures 3A–C). Generally, N-fixed and grain yield by the landraces closely mirrored patterns of shoot biomass. Thus, landrace/inoculant strain combinations that elicited higher shoot biomass were found to record higher N-fixed and grain yield (Figures 3A–E).
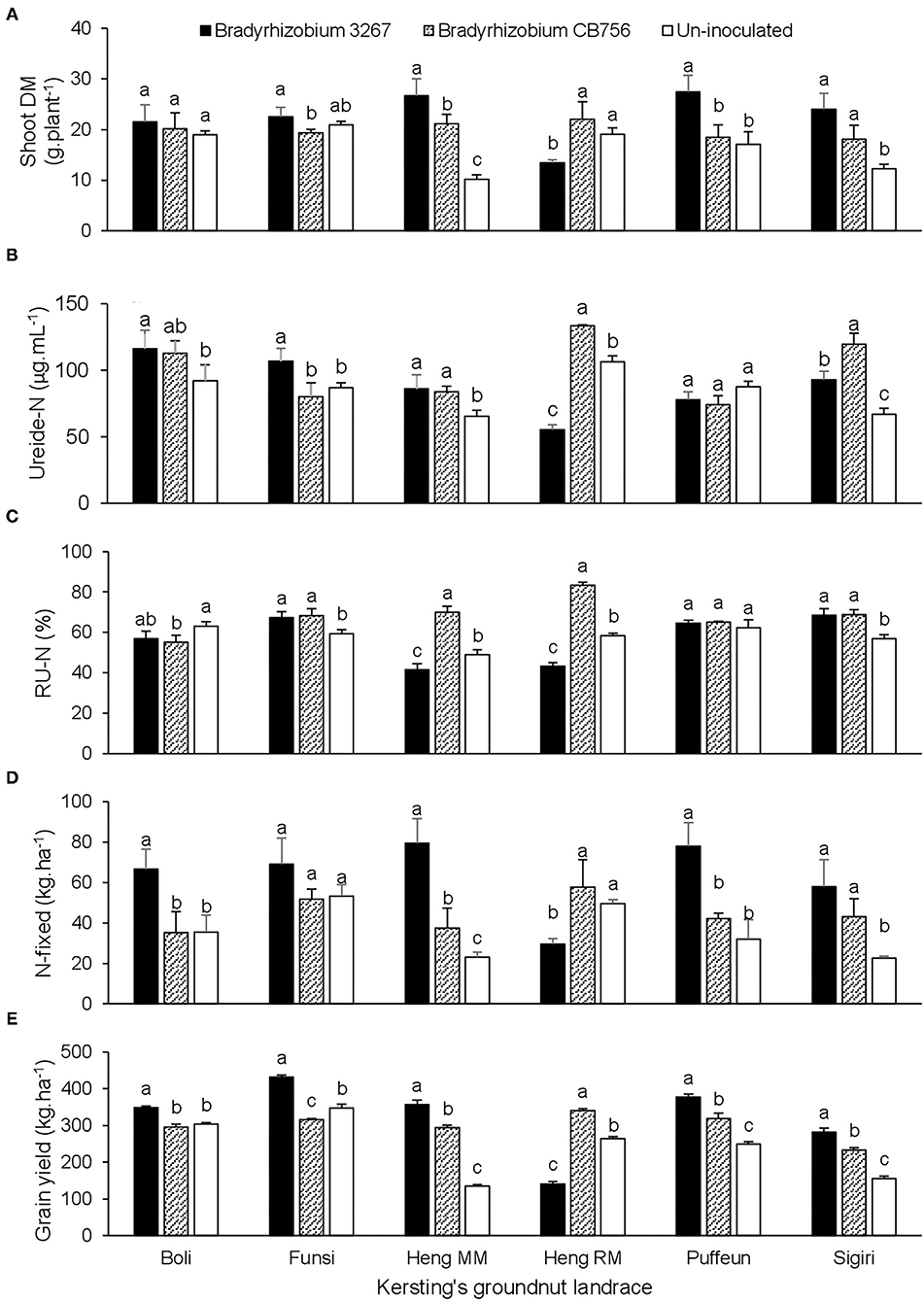
Figure 3. The interactive effect of landrace × inoculation on (A) shoot dry matter, (B) ureide-N in xylem sap, (C) relative ureide-N (RU-N), (D) N-fixed, and (E) grain yield of six Kersting's groundnut landraces planted at Gbalahi in 2013. For each landrace, bars with dissimilar letters are significantly different (p ≤ 0.05).
Three-Way ANOVA Comparison of Symbiotic Parameters, Grain Yield, and δ13C Between Locations
Except for %Ndfa, the main effect of location was significant for all growth and symbiotic parameters, grain yield, and shoot δ13C values (Supplementary Table 2). Although the overall nodule mass of plants was greater at Nyankpala and Savelugu, shoot biomass was markedly higher in the plants grown at Gbalahi followed by those grown at Nyankpala (Supplementary Table 2). The overall %N was higher in the plants grown at Nyankpala and Savelugu, resulting in much lower C/N ratio in those plants when compared to their counterparts grown at Gbalahi. The high shoot biomass and %N of plants at Nyankpala and Savelugu resulted in greater N content in those plants (Supplementary Table 2). Although average shoot δ15N value of plants was markedly lower at Savelugu, %Ndfa was similar between locations due to differences in the δ15N values of reference plants between those locations (Supplementary Table 1). However, N-fixed, soil N uptake as well as grain yield closely mirrored patterns in shoot biomass and N content between locations (Supplementary Table 2). Overall, shoot δ13C was greater in the plants grown at Savelugu, followed by those grown at Nyankpala and least in the plants at Gbalahi (Supplementary Table 2).
There was a marked effect of location on xylem N solutes of Kersting's groundnut in this study (Supplementary Table 3). The concentrations of ureides and nitrate were both higher in the xylem sap of the plants grown at Savelugu and Gbalahi, and unexpectedly least in the plants grown at Nyankpala despite their greater shoot biomass and N-fixed at the site. The concentration of amino acids in xylem sap was higher at Gbalahi, and lower but similar between Nyankpala and Savelugu (Supplementary Table 3). However, RU-N was higher in the plants at Savelugu followed by those at Gbalahi due to greater overall ureides in the plants grown in those locations (Supplementary Table 3).
Correlation Analysis
Correlation analysis revealed that the concentration of nitrate in xylem sap and %Ndfa measured from 15N natural abundance were negatively correlated in landraces Boli, Dowie, and Funsi at Nyankpala (Figure 4). The RU-N in xylem sap was also positively correlated with grain yield in all the test locations (Figure 4). Moreover, positive correlations were obtained when shoot biomass was plotted against the amount of N-fixed, soil N uptake, and shoot N content in this study (Figure 5). Furthermore, there was a positive correlation between %N and %C in shoots of the test landraces (Figure 6). On the other hand, shoot δ13C and grain yield were positively correlated at Nyankpala, although not statistically significant at Savelugu (Figure 6). The combined data from all the test locations also revealed a direct correlation between δ13C and grain yield (Figure 6).
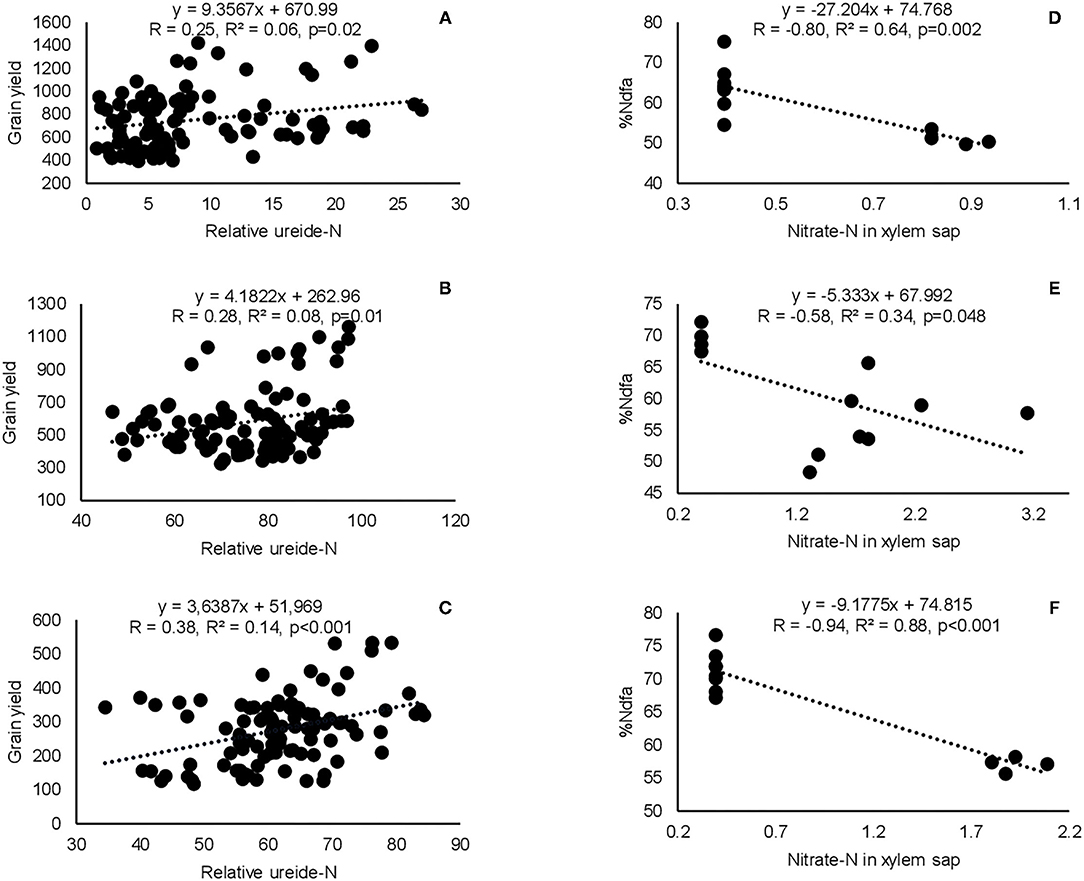
Figure 4. Correlation and regression analysis between xylem nitrate-N and relative ureide-N (RU-N) and grain yield of eight Kersting's groundnut landraces grown at (A) Nyankpala, (B) Savelugu, and (C) Gbalahi, and between xylem nitrate-N and %Ndfa from 15N natural abundance in landraces (D) Boli, (E) Dowie, and (F) Funsi planted at Nyankpala in 2013.
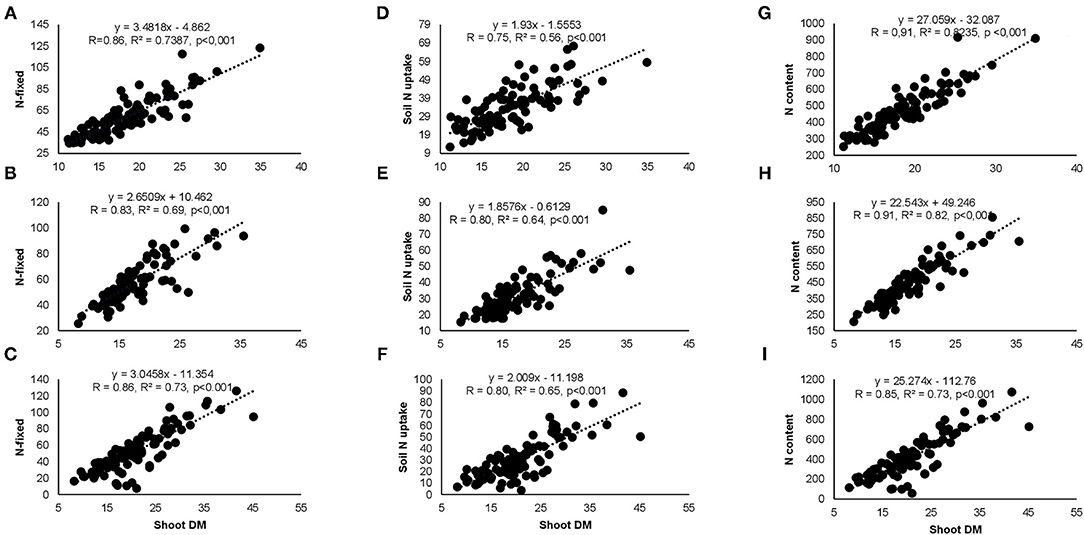
Figure 5. Correlation and regression analysis between shoot DM and N-fixed at (A) Nyankpala, (B) Savelugu, (C) Gbalahi, and between shoot DM and soil N uptake at (D) Nyankpala, (E) Savelugu, (F) Gbalahi as well as between shoot DM and N content at (G) Nyankpala, (H) Savelugu, and (I) Gbalahi.
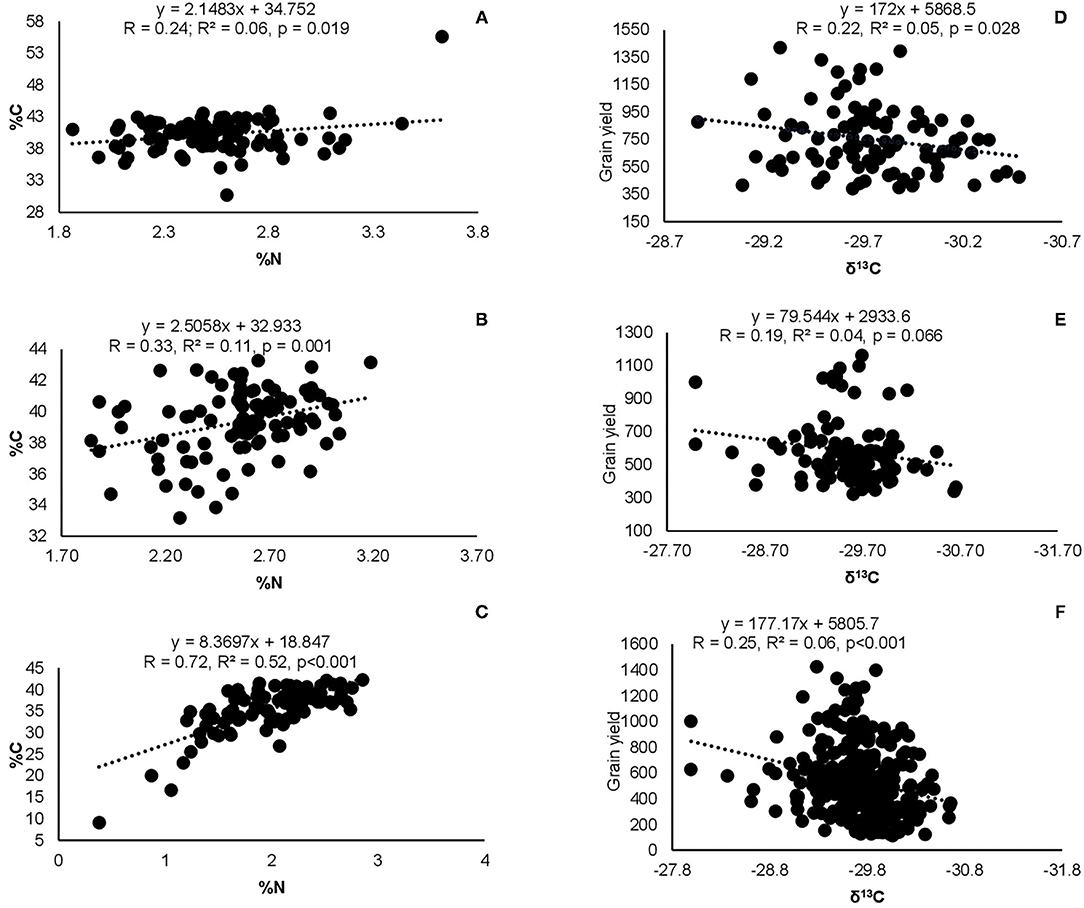
Figure 6. Correlation and regression analysis between shoot %N and %C at (A) Nyankpala, (B) Savelugu, (C) Gbalahi, and between δ13C and grain yield at (D) Nyankpala, (E) Savelugu, as well as (F) between δ13C and grain yield of the combined data from the three locations.
Discussion
Nodulation, Plant Growth, and Symbiotic N2 Fixation in Field-Grown Kersting's Groundnut Landraces
The ability of Kersting's groundnut to enter symbiosis with Bradyrhizobium sp. strain CB756 leading to N2 fixation was earlier demonstrated (Dakora, 1998). Since then, there has been little report of N2 fixation by this orphan grain legume (Mohammed et al., 2018), although such studies can provide information much needed for the crop's conservation and improvement. This study assessed symbiotic N2 fixation and water use efficiency (δ13C) of Kersting's groundnut landraces in response to inoculation with two Bradyrhizobium strains (Bradyrhizobium sp. BR 3267 and Bradyrhizobium sp. CB756) under field conditions in Ghana.
The findings revealed that increased nodulation in some Kersting's groundnut landraces resulted in greater plant growth, whereas the opposite was observed in some instances. For example, increased nodule mass in landraces Heng MM and Heng RM resulted in greater shoot biomass and N accumulation at Nyankpala as opposed to an observed lower shoot biomass in landraces Boli and Sigiri at Savelugu despite their higher nodule mass at the site. Moreover, the fact that the observed increases in plant growth associated with high nodulation at Nyankpala only decreased shoot δ15N and increased %Ndfa in Heng MM (Table 1) clearly suggests that the extent of nodulation alone does not always reflect symbiotic performance. However, whether differences in nodule size and/or nodule position on the root might have contributed to the observed trends in symbiotic performance of the test landraces was not assessed in this study. These results agree with earlier reports that enhanced symbiosis is linked to the N2-fixing efficiency of the nodule symbionts rather than increased nodulation only (Westhoek et al., 2017; Mohammed et al., 2019). The Kersting's groundnut landraces in this study exhibited marked variations in N2 fixation as evidenced by the differences in their shoot δ15N and %Ndfa values. As expected, lower δ15N by landraces were accompanied by higher %Ndfa in all the study locations, a finding consistent with previous reports that, lower δ15N values in N2-fixing legumes is due to their greater reliance on atmospheric N2 fixation (Unkovich et al., 2008; Szpak, 2014). With relatively lower δ15N values, therefore, symbiotic dependence (%Ndfa) was much higher in landraces Heng MM (68%) and Funsi (67%) at Nyankpala, Funsi (67%) at Savelugu, and in Heng MM (67%) and Funsi (67%) at Gbalahi. The high N2-fixing trait of these landraces is a desirable trait that can confer adaptation to low N environments such as those in this study. Owing to the observed differences in the percent N derived from symbiosis by the test landraces, the amounts of N-fixed also varied markedly, and closely mirrored the patterns of their shoot biomass and N accumulation. Moreover, symbiotic N and endogenous soil N both contributed to enhanced plant growth, a finding further supported by the positive correlations observed when shoot biomass was plotted against N-fixed, soil N uptake, and shoot N content (Figure 5). Due to the high symbiotic dependence by Kersting's groundnuts in this study, the amounts of N-fixed by landraces were generally greater than their soil N uptake values, with the amounts of N-fixed ranging from 46 to 69 kg ha−1 at Nyankpala, 48 to 73 kg ha−1 at Savelugu, and from 41 to 71 kg ha−1 at Gbalahi. Legumes exhibiting greater N2 fixation often show less dependence on soil N since the efficiency of the legume–rhizobia symbiosis diminishes with higher endogenous soil N concentrations (Zahran, 1999; Mbah and Dakora, 2017).
Across the board, the relatively lower overall δ15N values in the plants grown at Nyankpala and Savelugu resulted in higher %N and reduced C/N ratio in those plants probably due to enhanced symbiosis (Supplementary Table 2). Although C/N ratio did not markedly vary among the Kersting's groundnut landraces, the values were generally low and ranged between 14.4 and 20.2 g g−1 across the three sites, a finding that could be attributed to the reduction of atmospheric N2 to NH3 in their root nodules (Hobbie et al., 1998; Wolf et al., 2017). These results agree with earlier reports that legumes exhibit a characteristic low C/N ratio (<24 g g−1) due to their N2-fixing activities as opposed to higher values (>24 g g−1) often recorded in non-legumes (Hobbie et al., 1998). The legume–rhizobia symbiosis is such that, rhizobia in root nodules fix atmospheric N2 in exchange for plant photosynthates (Oldroyd et al., 2011), a functional link that was further illustrated by positive correlations between %N and %C in shoots of Kersting's groundnuts in this study. Thus, the increased supply of carbon compounds from photosynthesis stimulated greater N2 fixation in root nodules of the test landraces, such that higher shoot N concentration was accompanied by higher C concentration (Mohammed et al., 2018). Nevertheless, although the overall percent N derived from symbiosis was unaltered between locations, the shoot δ15N values of landraces showed marked variations, and closely mirrored the patterns in the endogenous soil N concentrations between the three sites (Supplementary Table 2). Thus, due to the relatively lower N content of soils at Savelugu (0.016%), the plants at the site recorded much lower overall δ15N value; conversely, the relatively higher endogenous soil N concentrations at Nyankpala (0.023%) and Gbalahi (0.031%) led to higher δ15N values in the plants at those sites due to a suppression of the symbiosis (Mbah and Dakora, 2017). However, although the observed lower δ15N in plants at Savelugu were accompanied by greater ureides biosynthesis and hence higher relative ureide abundance in the xylem sap, the RU-N in plants at Nyankpala were unexpectedly very low due to their reduced ureides concentration (Supplementary Table 3). Whether the dramatic reduction in the concentration of ureides in xylem sap at Nyankpala was influenced by the dynamics in soil moisture levels at the time of sampling was not assessed. Whatever the case, however, the ureide measure of symbiosis is instantaneous and can therefore be influenced by soil/environmental factors prevailing at the time of sampling as opposed to the more integrative 15N natural abundance technique (Unkovich et al., 2008).
Low grain yield of Kersting's groundnut on farmers' fields partly accounts for the decline in its cultivation (Ayenan and Ezin, 2016). In this study, average grain yield ranged between 445 and 959 kg ha−1 at Nyankpala, between 398 and 832 kg ha−1 at Savelugu, and between 164 and 365 kg ha−1 at Gbalahi. The relatively higher grain yield in the plants at Nyankpala and Savelugu could be attributed to adequate soil moisture during plant establishment and pod filling due to timely planting at the site relative to the cropping season (Figure 1). The fact that most landraces with greater shoot biomass coupled that with increased N accumulation and grain yield in some instances suggests complementary roles of symbiosis and soil N uptake in promoting the growth and yield of Kersting's groundnut in this study. The grain yield recorded by landraces in this study are generally lower than values (up to 1,876 kg ha−1) reported in earlier studies (Bayorbor et al., 2010; Mohammed et al., 2018). Although planting date was not reported in their study at Nyankpala (Bayorbor et al., 2010), earlier planting could have contributed to the higher yields obtained for the same landraces in that location. However, grain yield was reduced (192–688 kg ha−1) when planting was carried out in July in another experiment (Adu-gyamfi et al., 2012) possibly due to reduced soil moisture during crop development and/or pod filling. Variations in soil moisture resulting from differences in planting dates and/or rainfall distribution could possibly explain the patterns of grain yield across the three locations in this study (Figure 1).
Variations in Water Use Efficiency (δ13C) in Field-Grown Kersting's Groundnut Landraces
Even though Kersting's groundnut is reported to thrive well under drought conditions (Mergeai, 1993), there is no available report on the crop's water use efficiency. In this study, shoot δ13C was used as a measure of water use efficiency. The landraces Heng MM and Heng RM, both with high grain yield at Nyankpala also exhibited greater water use efficiency at that site as evidenced by their higher shoot δ13C (−29.48‰ and −29.60‰, respectively), when compared to other landraces. On the other hand, the landrace Sigiri coupled the least grain yield with the lowest water use efficiency at Nyankpala, recording a δ13C value of −29.97‰. These findings clearly indicate variations in water use efficiency among the tested Kersting's groundnut landraces in this study. Nevertheless, the fact that shoot δ13C values of the test landraces were much lower than values reported for some Bambara groundnut (−26.2‰) and soybeans (−25.1‰) elsewhere (Mohale et al., 2014; Mapope and Dakora, 2016) suggests that the plants in this study might have received adequate rainfall during the growth period, leading to an increase in C isotope discrimination and hence lower δ13C values. Among the landraces, Boli exhibited consistently high water use efficiency across the three locations followed by Heng MM and Heng RM, which also exhibited greater water use efficiency at Nyankpala and Savelugu. Interestingly, grain yield correlated positively with shoot δ13C at Nyankpala (r = 0.22, r2 = 0.09, p = 0.004), just as the combined data from all the test locations also revealed a positive correlation between the two parameters (r = 0.25, r2 = 0.06, p < 0.001), although these relationships were weak. These findings could explain why Kersting's groundnut is able to thrive and produce yield under water-limiting conditions in the African savanna (Mergeai, 1993; Mohammed et al., 2018). The fact that most landraces with higher grain yield in this study coupled that with relatively greater water use efficiency is a trait that could be exploited for increased production of Kersting's groundnut in water-limiting environments.
Aside from the observed genotypic differences, there was also a marked effect of planting location on δ13C of Kersting's groundnut in this study, with plants at Savelugu eliciting the highest overall water use efficiency (shoot δ13C) followed by those planted at Nyankpala and Gbalahi. The observed variations in shoot δ13C values of plants between locations could be attributed to the variations in rainfall distribution and soil moisture during plant growth. Although grain yield at Gbalahi was reduced by decreased soil moisture due to late planting at the site relative to the rains (Figure 1), the stress happened at the onset of podding. As a result, the expected greater δ13C (water use efficiency) from water stress (Wang et al., 2016) was not noticed in plants at the site since sampling was done around the same time (early podding) that the water stress started. Nevertheless, the total rainfall from sowing till plant sampling was 481 mm at Nyankpala, 471 mm at Savelugu, and 305 mm at Gbalahi. The lower δ13C in plants at Gbalahi relative to those in the other locations was therefore unexpected since plants exposed to reduced soil moisture are known to exhibit greater water use efficiency (δ13C) (Farquhar et al., 1989; Wang et al., 2016). However, the field at Nyankpala was previously fallowed while the soils at Savelugu were shallow despite being under cultivation. The surprisingly lower δ13C in plants at Gbalahi might have been due to alterations in soil structure resulting from cultivation practices at the site that might have favored improved water retention capacity. The fact that δ13C was highest at Savelugu could however be attributed to both reduced rains at the site relative to Nyankpala coupled with lower water retention capacity due to an observed shallow nature of the soils at the site. An earlier study with soybeans also observed reduced δ13C in some areas with lower rainfall compared to those with higher rains due to differences in tillage systems (Mapope and Dakora, 2016).
The Response of Kersting's Groundnut Landraces to Bradyrhizobium Inoculation
Previous studies on legume inoculation in the field have reported variable response due to environmental differences, crop species, and/or genotypes as well as rhizobial strain differences in N2-fixing efficiency (Ulzen et al., 2016; Kyei-Boahen et al., 2017; Mohammed et al., 2018). In this study, plant growth, symbiotic performance, and grain yield of the test landraces showed variable response to inoculation with the two Bradyrhizobium strains. Inoculation response was markedly influenced by the crop landrace, location, and the strain of Bradyrhizobium used, indicating differences in host–soil microbiome compatibility. Although interaction effects within study sites were significant for several parameters (Tables 1–4), for brevity, the results are discussed for shoot biomass, N-fixed, and grain yield for landraces that showed consistent patterns of interactions for those parameters. The test landraces showed contrasting response to inoculation between the study locations, with bacterial inoculation of landraces Dowie, Heng MM, and Nakori with either Bradyrhizobium strains at Savelugu resulting in enhanced plant growth and N-fixed, leading to significant grain yield increases over non-inoculation in the case of Heng MM (Figures 2A–C). Despite the reduced shoot growth and N-fixed in uninoculated Nakori plants at Savelugu, however, grain yield was markedly increased when compared to inoculation of the landraces with Bradyrhizobium sp. strain CB756 (Figures 2A–C). In contrast to the plants at Savelugu that showed positive inoculation response, non-inoculation of Dowie, Heng MM, Nakori, and Puffeun at Nyankpala markedly enhanced plant growth and the amounts of N-fixed, resulting in significant grain yield increases when compared to Bradyrhizobium inoculation of those landraces with either inoculant strains at the site (Figures 2D–F). The observed contrasting response to inoculation by Kersting's groundnut landraces between the two locations could be attributed to variations in the N2-fixing efficacy of the native rhizobial populations between the sites. Whereas, the inoculated plants at Savelugu showed better symbiotic performance than their uninoculated counterparts at the site, the uninoculated plants at Nyankpala showed greater symbiotic performance than their inoculated counterparts. Similarly, the contrasting response to bacterial inoculation elicited by the plants at Gbalahi clearly highlights the complex effects of the crop landrace, inoculant strain, and location-specific factors on the Kersting's groundnut–rhizobia symbiosis under field conditions. Previous studies have highlighted the complex nature of legume inoculation response under field settings, a process governed by the N2-fixing efficiency of the inoculant strains as well as their competitive ability for nodule occupancy relative to the resident/indigenous strains (Catroux et al., 2001; Mohammed et al., 2018). Because the products of N2 fixation in root nodules of Kersting's groundnut are exported to the aerial portions as ureides via the xylem, the landrace/inoculant strain combinations that promoted higher shoot biomass a Gbalahi also recorded greater xylem ureides and amounts of N-fixed (Figure 3). Probably due to the instantaneous nature of the ureide measure of N2 fixation, however (Unkovich et al., 2008), some deviations were observed where higher xylem ureide levels did not translate into greater shoot biomass or N-fixed. Nevertheless, improved plant growth and symbiotic performance resulting from inoculation or non-inoculation at Gbalahi promoted significant grain yield increases, a finding that clearly demonstrates the importance of legume N2 fixation to plant growth and consequently grain yield production.
The trends in plant growth closely mirrored the patterns of N-fixed and was further illustrated by positive correlations between the two parameters (Figures 5A–C). The observed direct link between plant growth and N nutrition as observed with Kersting's groundnut in this study was earlier reported in field-grown cowpea and Kersting's groundnut (Belane and Dakora, 2010; Mohammed et al., 2018). The amounts of N-fixed by Kersting's groundnut in this study also varied interactively, with values ranging from 42 to 91 kg ha−1 at Nyankpala, from 44 to 80 kg ha−1 at Savelugu, and from 23 to 80 kg ha−1 at Gbalahi depending on the landrace/inoculant combinations used in those locations. These N-fixed values clearly indicate the potential role of Kersting's groundnut in sustainable agriculture, where their N contribution to soils from shoot residues after harvest could be substantial. The contribution of legume N2 fixation to plant growth and grain yield was further evidenced by positive correlations between RU-N in xylem sap and grain yield (Figure 4). It is this N2-fixing trait of nodulated legumes such as Kersting's groundnut that gives them advantage over non-legumes in low-N environments (Menge and Chazdon, 2016). The fact that shoot biomass correlated positively with N-fixed, soil N-uptake, and shoot N content shows that both symbiosis and soil N uptake complemented each other in enhancing the growth of Kersting's groundnuts in this study. Nevertheless, lower soil N uptake values were largely associated with greater dependence on symbiotic N, thus agreeing with known reports that low endogenous soil N stimulates greater symbiotic N2 fixation and vice versa (Zahran, 1999; Mbah and Dakora, 2017; Mohammed et al., 2018).
Depending on the landrace/inoculant strain combinations used, grain yield of the test Kersting's groundnuts varied between 515 and 1,198 kg ha−1 at Nyankpala, between 350 and 1,095 kg ha−1 at Savelugu, and between 135 and 432 kg ha−1 at Gbalahi. In most instances, the landrace/inoculant combinations that elicited greater plant growth and N-fixed also had high grain yield. Thus, whereas bacterial inoculation with either Bradyrhizobium strains generally improved symbiotic performance and grain yield of Kersting's groundnuts at Savelugu and Gbalahi, non-inoculation markedly enhanced N-fixed and grain yield over bacterial inoculation of the plants at Nyankpala, a clear indication of variations in the plant growth-promoting effects of the soil microbiomes between the test sites (Figures 2, 3).
Conclusion
This study has explored the N2-fixing capacity and water use efficiency (δ13C) of the orphan Kersting's groundnut in response to inoculation with two bradyrhizobial strains. The findings revealed high symbiotic dependence by Kersting's groundnut, which was influenced by the landrace/inoculant strain combination and planting location, indicating differences in the N2-fixing efficacy of native rhizobia between locations. Moreover, the differential performance of the inoculant strains between and among locations indicate possible variations in their competitiveness for nodule occupancy and their ability to persist and fix N2 in symbiosis with their host in the studied environments. Whereas, symbiosis accounted for a greater part of the N demand by Kersting's groundnut in this study, soil N uptake complemented the symbiotic process in enhancing plant growth, shoot N accumulation, and, in some instances, grain yield. Given that the overall symbiotic dependence by landraces in this study ranged between 58 and 68%, there is huge potential to increase the efficiency of the Kersting's groundnut–rhizobia symbiosis through manipulation of the host legume and its nodule symbionts. This effort could commence through exploring indigenous rhizobia within and without the crop's cultivation areas that are capable of higher N2 fixation in diverse environments.
Data Availability Statement
The original contributions presented in the study are included in the article/Supplementary Material, further inquiries can be directed to the corresponding author/s.
Author Contributions
MM conducted field experiments, analyzed and interpreted data, and prepared the manuscript. GM took part in xylem N solute analyses. ES helped in field experiments. FD funded and coordinated the research and helped in manuscript preparation. All authors contributed to the article and approved the submitted version.
Conflict of Interest
The authors declare that the research was conducted in the absence of any commercial or financial relationships that could be construed as a potential conflict of interest.
Publisher's Note
All claims expressed in this article are solely those of the authors and do not necessarily represent those of their affiliated organizations, or those of the publisher, the editors and the reviewers. Any product that may be evaluated in this article, or claim that may be made by its manufacturer, is not guaranteed or endorsed by the publisher.
Acknowledgments
We are grateful to the South African Research Chair in Agrochemurgy and Plant Symbioses, the National Research Foundation, and the Tshwane University of Technology (TUT) for financial support to FD's research, and for a competitive TUT Doctoral bursary to MM. The authors also appreciate support from Dr. Benjamin D. K. Ahiabor of the CSIR-Savanna Agricultural Research Institute at Nyankpala, Ghana for the supply of inoculant and support during field experimentation.
Supplementary Material
The Supplementary Material for this article can be found online at: https://www.frontiersin.org/articles/10.3389/fsufs.2021.672247/full#supplementary-material
References
Adu-gyamfi, R., Dzomeku, I. K., and Lardi, J. (2012). Evaluation of growth and yield potential of genotypes of kersting's groundnut (Macrotyloma geocarpum Harms) in Northern Ghana. Int. Res. J. Agric. Sci. Soil Sci. 2, 509–515. Available online at: http://www.interesjournals.org/IRJAS
Akohoué, F., Sibiya, J., and Achigan-Dako, E. G. (2018). On-farm practices, mapping, and uses of genetic resources of Kersting's groundnut [Macrotyloma geocarpum (Harms) Maréchal et Baudet] across ecological zones in Benin and Togo. Genet. Resour. Crop Evol. 66, 195–214. doi: 10.1007/s10722-018-0705-7
Amuti, K. (1980). Geocarpa groundnut (Kerstingiella geocarpa) in Ghana. Econ. Bot. 34, 358–361. doi: 10.1007/BF02858310
Assogba, P., Ewedje, E. E. B. K., Dansi, A., Loko, Y. L., Adjatin, A., Dansi, M., et al. (2016). Indigenous knowledge and agro-morphological evaluation of the minor crop Kersting's groundnut (Macrotyloma geocarpum (Harms) Marechal et Baudet) cultivars of Benin. Genet. Resour. Crop Evol. 63, 513–529. doi: 10.1007/s10722-015-0268-9
Ayenan, M. A. T., and Ezin, V. A. (2016). Potential of Kersting's groundnut [Macrotyloma geocarpum (Harms) Maréchal & Baudet] and prospects for its promotion. Agric. Food Secur. 5:10. doi: 10.1186/s40066-016-0058-4
Bayorbor, T., Dzomeku, I., Avornyo, V., and Opoku-Agyeman, M. (2010). Morphological variation in Kersting ' s groundnut (Kerstigiella geocarpa Harms) landraces from northern Ghana. Agric. Biol. J. North Am. 1, 290–295. doi: 10.5251/abjna.2010.1.3.290.295
Belane, A. K., and Dakora, F. D. (2010). Symbiotic N2 fixation in 30 field-grown cowpea (Vigna unguiculata L. Walp.) genotypes in the Upper West Region of Ghana measured using 15N natural abundance. Biol. Fertil. Soils 46, 191–198. doi: 10.1007/s00374-009-0415-6
Blumenthal, M. J., and Staples, I. B. (1993). Origin, evaluation and use of Macrotyloma as forage - a review. Trop. Grasslands 27, 16–29.
Bray, R. H., and Kurtz, L. T. (1945). Determination of total, organic, and available forms of phosphorus in soils. Soil Sci. 59, 39–46. doi: 10.1097/00010694-194501000-00006
Cataldo, D., Haroon, M., Schrader, L. E., and Youngs, V. L. (1975). Rapid colorimetric determination of nitrate in plant tissue by nitration of salicylic acid. Commun. Soil Sci. Plant Anal. 6, 71–80. doi: 10.1080/00103627509366547
Catroux, G., Hartmann, A., and Revellin, C. (2001). Trends in rhizobial inoculant production and use. Plant Soil 230, 21–30. doi: 10.1023/A:1004777115628
Craig, H. (1957). Isotopic standards for carbon and oxygen and correction factors for mass-spectrometric analysis of carbon dioxide. Geochim. Cosmochim. Acta 12, 133–149. doi: 10.1016/0016-7037(57)90024-8
Dakora, F. D. (1998). Nodule function in symbiotic Bambara groundnut (Vigna subterranea L.) and Kersting's bean (Macrotyloma geocarpum L.) is tolerant of nitrate in the root medium. Ann. Bot. 82, 687–690. doi: 10.1006/anbo.1998.0720
Dakora, F. D., Atkins, C. A., and Pate, J. S. (1992). Effect of NO3 on N2 fixation and nitrogenous solutes of xylem in two nodulated West African geocarpic legumes, Kersting's bean (Macrotyloma geocarpum L.) and Bambara groundnut (Vigna subterranea L.). Plant Soil 140, 255–262. doi: 10.1007/BF00010602
Dwivedi, S. L., Sahrawat, K. L., Upadhyaya, H. D., Mengoni, A., Galardini, M., Bazzicalupo, M., et al. (2015). “Advances in host plant and rhizobium genomics to enhance symbiotic nitrogen fixation in grain legumes,” in Advances in Agronomy, Vol. 29, ed Donald L. Spark (San Diego, CA: Elsevier Ltd), 1–116. doi: 10.1016/bs.agron.2014.09.001
Farquhar, G. D., Ehleringer, J. R., and Hubick, K. T. (1989). Carbon isotope discrimination and photosynthesis. Annu. Rev. Plant Biol. 40, 503–537. doi: 10.1146/annurev.pp.40.060189.002443
Herridge, D. F., Bergersen, F. J., and Peoples, M. B. (1990). Measurement of nitrogen fixation by soybean in the field using the ureide and natural N abundance methods. Plant Physiol. 93, 708–716. doi: 10.1104/pp.93.2.708
Herridge, D. F., and Peoples, M. B. (1990). Ureide assay for measuring nitrogen fixation by nodulated soybean calibrated by N methods. Plant Physiol. 93, 495–503. doi: 10.1104/pp.93.2.495
Hobbie, E. A., MacKo, S. A., and Shugart, H. H. (1998). Patterns in N dynamics and N isotopes during primary succession in Glacier Bay, Alaska. Chem. Geol. 152, 3–11. doi: 10.1016/S0009-2541(98)00092-8
Hungria, M., Franchini, J., Campo, R., and Graham, P. (2006). “The importance of nitrogen fixation to soybean cropping in South America,” in Nitrogen Fixation in Agriculture, Forestry, Ecology, and the Environment, eds D. Werner and W. E. Newton (Netherlands: Springer Netherlands).
Kyei-Boahen, S., Savala, C. E. N., Chikoye, D., and Abaidoo, R. (2017). Growth and yield responses of cowpea to inoculation and phosphorus fertilization in different environments. Front. Plant Sci. 8:646. doi: 10.3389/fpls.2017.00646
Mapope, N., and Dakora, F. D. (2016). N2 fixation, carbon accumulation, and plant water relations in soybean (Glycine max L. Merrill) varieties sampled from farmers' fields in South Africa, measured using 15N and 13C natural abundance. Agric. Ecosyst. Environ. 221, 174–186. doi: 10.1016/j.agee.2016.01.023
Maskey, S. L., Bhattarai, S., Peoples, M. B., and Herridge, D. F. (2001). On-farm measurements of nitrogen fixation by winter and summer legumes in the hill and terai regions of nepal. F. Crop. Res. 70, 209–221. doi: 10.1016/S0378-4290(01)00140-X
Mbah, G. C., and Dakora, F. D. (2017). Nitrate inhibition of N2 fixation and its effect on micronutrient accumulation in shoots of soybean (Glycine max L. Merr.), Bambara groundnut (Vigna subterranea L. Vedc) and Kersting's groundnut (Macrotyloma geocarpum Harms.). Symbiosis 2, 1–12. doi: 10.1007/s13199-017-0531-2
Menge, D. N. L., and Chazdon, R. L. (2016). Higher survival drives the success of nitrogen-fixing trees through succession in Costa Rican rainforests. New Phytol. 209, 965–977. doi: 10.1111/nph.13734
Mergeai, G. (1993). Influence des facteurs sociologiques sur la conservation des ressources phytogénétiques. Le cas de la lentille de terre (Macrotyloma geocarpum (Harms) Marechal & Baudet) au Togo. Bull. des Rech. Agron. Gembloux 28, 487–500.
Mohale, K. C., Belane, A. K., and Dakora, F. D. (2014). Symbiotic N nutrition, C assimilation, and plant water use efficiency in Bambara groundnut (Vigna subterranea L. Verdc.) grown in farmers ' fields in South Africa, measured using 15N and 13C natural abundance. Boil. Fertil. Soils 50, 307–319. doi: 10.1007/s00374-013-0841-3
Mohammed, M., Jaiswal, S. K., and Dakora, F. D. (2019). Insights into the phylogeny, nodule function and biogeographic distribution of microsymbionts nodulating the orphan Kersting's groundnut [Macrotyloma geocarpum (Harms) Marechal & Baudet] in African soils. Appl. Environ. Microbiol. 85, e00342–e00319. doi: 10.1128/AEM.00342-19
Mohammed, M., Jaiswal, S. K., Sowley, E. N. K. K., Ahiabor, B. D. K. K., and Dakora, F. D. (2018). Symbiotic N2 fixation and grain yield of endangered Kersting's groundnut landraces in response to soil and plant associated Bradyrhizobium inoculation to promote ecological resource-use efficiency. Front. Microbiol. 9:2105. doi: 10.3389/fmicb.2018.02105
Oldroyd, G. E. D. D., Murray, J. D., Poole, P. S., and Downie, J. A. (2011). The rules of engagement in the legume-rhizobial symbiosis. Annu. Rev. Genet. 45, 119–144. doi: 10.1146/annurev-genet-110410-132549
Owusu, K., and Waylen, P. R. (2013). The changing rainy season climatology of mid-Ghana. Theor. Appl. Climatol. 112, 419–430. doi: 10.1007/s00704-012-0736-5
Pausch, R. C., Mulchi, C. L., Lee, E. H., and Meisinger, J. J. (1996). Use of 13C and 15N isotopes to investigate O3 effects on C and N metabolism in soybeans. Part II. Nitrogen uptake, fixation, and partitioning. Agric. Ecosyst. Environmen. 60, 61–69. doi: 10.1016/S0167-8809(96)01062-6
Preissel, S., Reckling, M., Schläfke, N., and Zander, P. (2015). Field crops research magnitude and farm-economic value of grain legume pre-crop benefits in Europe: A review. F. Crop. Res. 175, 64–79. doi: 10.1016/j.fcr.2015.01.012
Rebah, F., Ben Prévost, D., Yessa, A., and Tyagi, R. (2007). Agro-industrial waste materials and wastewater sludge for rhizobial inoculant production : A review. Bioresour. Technol. 98, 3535–3546. doi: 10.1016/j.biortech.2006.11.066
Shearer, G., and Kohl, D. H. (1986). N2-fixation in field settings: estimations based on natural 15N abundance. Aust. J. Plant Physiol. 13, 699–756. doi: 10.1071/PP9860699
Stephens, J. H. G., and Rask, H. M. (2000). Inoculant production and formulation. F. Crop. Res. 65, 249–258. doi: 10.1016/S0378-4290(99)00090-8
Szpak, P. (2014). Complexities of nitrogen isotope biogeochemistry in plant-soil systems: implications for the study of ancient agricultural and animal management practices. Front. Plant Sci. 5:288. doi: 10.3389/fpls.2014.00288
Ulzen, J., Abaidoo, R. C., Mensah, N. E., Masso, C., and Abdel Gadir, A. H. (2016). Bradyrhizobium inoculants enhance grain yields of soybean and cowpea in Northern Ghana. Front. Plant Sci. 7:1770. doi: 10.3389/fpls.2016.01770
Unkovich, M., Herridge, D., Peoples, M., Cadisch, G., Boddey, B., Giller, K., et al. (2008). Measuring Plant-Associated Nitrogen Fixation in Agricultural Systems. Canberra: Australian Centre for International Agricultural Research.
Walkley, A., and Black, I. A. (1934). An examination of the degtjareff method for determining soil organic matter, and a proposed modification of the chromic acid titration method. Soil Sci. 37, 29–38. doi: 10.1097/00010694-193401000-00003
Wang, C., Liu, D., Luo, W., Fang, Y., and Bai, E. (2016). Variations in leaf carbon isotope composition along an arid and semi-arid grassland transect in northern China. J. Plant Ecol. 9, 576–585. doi: 10.1093/jpe/rtw006
Westhoek, A., Field, E., Rehling, F., Mulley, G., Webb, I., Poole, P. S., et al. (2017). Policing the legume-Rhizobium symbiosis: A critical test of partner choice. Sci. Rep. 7, 566–576. doi: 10.1038/s41598-017-01634-2
Wolf, A. A., Funk, J. L., and Menge, D. N. L. (2017). The symbionts made me do it: legumes are not hardwired for high nitrogen concentrations but incorporate more nitrogen when inoculated. New Phytol. 213, 690–699. doi: 10.1111/nph.14303
Yemm, E., and Cocking, R. R. (1955). The determination of amino-acids with ninhydrin. Analyst 80, 209–204. doi: 10.1039/an9558000209
Young, G. B. E., and Conway, C. F. (1942). On the estimation of allantoin by the Rimini-Schryver reaction. J. Biol. Chem. 142, 839–853. doi: 10.1016/S0021-9258(18)45082-X
Keywords: legume-rhizobium symbiosis, N-fixed, grain yield, δ13C, water use efficiency, Kersting's groundnut
Citation: Mohammed M, Mbah GC, Sowley ENK and Dakora FD (2021) Bradyrhizobium Inoculation of Field-Grown Kersting's Groundnut [Macrotyloma geocarpum (Harms) Marechal & Baudet] Increased Grain Yield and N2 Fixation, Measured Using the Ureide, and 15N Natural Abundance Techniques. Front. Sustain. Food Syst. 5:672247. doi: 10.3389/fsufs.2021.672247
Received: 25 February 2021; Accepted: 23 September 2021;
Published: 28 October 2021.
Edited by:
E. O. Deedi Sogbohossou, Wageningen University and Research, NetherlandsReviewed by:
Mark Paul Running, University of Louisville, United StatesKedir Woliy, Hawassa University, Ethiopia
Zaiya Arlette, Institute for Agricultural Research for Development (IRAD), Cameroon
Copyright © 2021 Mohammed, Mbah, Sowley and Dakora. This is an open-access article distributed under the terms of the Creative Commons Attribution License (CC BY). The use, distribution or reproduction in other forums is permitted, provided the original author(s) and the copyright owner(s) are credited and that the original publication in this journal is cited, in accordance with accepted academic practice. No use, distribution or reproduction is permitted which does not comply with these terms.
*Correspondence: Felix D. Dakora, RGFrb3JhRkRAdHV0LmFjLnph