- 1School of Energy and Environment, University of Phayao, Phayao, Thailand
- 2School of Medical Sciences, University of Phayao, Phayao, Thailand
- 3Department of Microbiology, Faculty of Liberal Arts and Science, Kasetsart University Kamphaeng Sean Campus, Nakorn Pathom, Thailand
- 4Department of Chemistry, Faculty of Science, Chiang Mai University, Chiang Mai, Thailand
- 5Environmental Science Research Center, Faculty of Science, Chiang Mai University, Chiang Mai, Thailand
Wide applications of glyphosate and 2,4-dichlorophenoxyacetic acid (2,4-D) in rice paddy fields could lead to their residues in environment, posing adverse effects on rice growth and primary producers in the rice ecosystem. This research aims to determine the effects of environmentally relevant concentrations of glyphosate and 2,4-D commercial formulations on Nostoc sp. N1 and rice seedlings. The effects of herbicides on Nostoc sp. N1 were measured from the growth and acute toxicity. The germination and growth were used to determine the effects of herbicides on rice seedlings by measuring their physical and biochemical characteristics. Results showed that while glyphosate had higher toxicity than 2,4-D, both herbicides could stimulate the growth of Nostoc sp. N1 as indicated by their increase in biomass and chlorophyll a content. In Petri dish experiments, Nostoc sp. N1 cells not only promoted the germination of rice seedlings when added alone, but they also alleviated the toxicity of both herbicides to the rice seedlings. In pot experiments, the addition of Nostoc sp. N1 cells combined with herbicides promoted the biochemical characteristics of the rice seedlings by increasing the total chlorophyll, carotenoid and total amino acid content. Our results suggested that environmentally relevant concentrations of glyphosate and 2,4-D formulations should not pose any adverse effects on Nostoc sp. N1. Also, with their toxicity-mitigating and growth-promoting effects on rice seedlings, Nostoc sp. N1 cells could be applied in the alleviation of herbicide residue toxicity in paddy fields.
Introduction
The massive application of herbicides in agricultural systems led to their detection in various environmental matrices of surface water, groundwater, sediment and soil (Aparicio et al., 2013; Xu et al., 2017). In 2050, worldwide pesticide production is predicted to be 2.7 times higher than that in 2000 (Oberemok et al., 2015). In Thailand, there was an increasing trend of top 10 pesticides imported from 100,405 tons in 2012 to ~115,720 tons in 2018, with herbicides taking the highest proportion of 62–79% (Department of Agriculture, 2020). Among the imported herbicides, glyphosate and 2,4-D are commonly applied in massive quantities to agricultural fields in Thailand. While glyphosate is a non-selective systemic herbicide formulated to control both broadleaf and grassy weeds, 2,4-D is a selective post-emergence herbicide that selectively kills many terrestrial and aquatic broadleaf weeds. Both herbicides have regularly been used to control weeds in rice cultivation areas, leading to their residues remaining in the field.
Different amounts of glyphosate ranging from 0.0 to 9.9 mg/kg soil have been reported worldwide (Paipard et al., 2014; Silva et al., 2018; Hagner et al., 2019; Helander et al., 2019). Residues of 2,4-D in soil and sediment were detected at much lower concentrations, which are ranging from <0.652 mg/kg of soil (Ismail et al., 2011; Baumgartner et al., 2017) to <10 μg/kg of soil (Haynes et al., 2000). However, these small amounts of herbicide residues can adversely affect living organisms in the rice ecosystem where there are more than 700 animal species per hectare presented (Clay, 2004). Moreover, they may accumulate in non-target rice fields inhabiting organisms such as the rice frog (Fejervarya limnocharis) and menwig frog (Physalaemus albonotatus) (Jantawongsri et al., 2015; Curi et al., 2019).
On the first level of the rice field tropic chain, cyanobacteria are one of the most important susceptible species that can be affected by the toxicity of pesticides (Dash et al., 2017). Filamentous cyanobacteria widely distributed in wetland rice ecosystems, especially the heterocystous filamentous cyanobacteria such as Nostoc spp. and Anabaena spp., are able to maintain the field fertility through their nitrogen fixation (Kim and Lee, 2006). Additionally, various strains of cyanobacteria can produce extracellular polymeric substances (EPSs) comprising mainly polysaccharides, which can increase soil aggregation and improve soil quality (Costa et al., 2018). Therefore, cyanobacteria have been considered as a promising alternative for reducing agrochemical uses and their adverse impacts. A previous study showed that applying cyanobacteria together with chemical fertilizers can reduce agrochemical costs and improve rice yields (Chittapun et al., 2017). It was also found that the toxicity of glyphosate and 2,4-D could be reduced by addition of the cyanobacteria. Nostoc punctiforme could take up and use glyphosate as a phosphorus source, exhibiting the high potential of its cells to metabolize phosphonate (Forlani et al., 2008). In the case of 2,4-D, Nostoc hatei and Anabaena lutea were shown to reduce 2,4-D contamination of water from rice paddy fields by 39.73% after 14 days of application (Kophimail and Bunnag, 2014).
The residue of herbicides possibly poses both direct and indirect adverse side effects on rice and cyanobacteria in the rice field. The toxicity of herbicides to paddy field cyanobacteria and rice has been previously investigated; however, most studies have been performed separately on cyanobacteria or rice (Chen et al., 2007; Sheeba et al., 2011; El-Nahhal et al., 2015) with the focus only on the active ingredients of herbicides. It has been proven that the presence of pure glyphosate [N-(phosphonomethyl)glycine] had a stimulating effect on the growth of several cyanobacteria including Nostoc muscorum (Drzyzga and Lipok, 2018). In contrast, it was found that glyphosate at 97% purity induced oxidative stress, cell apoptosis, and toxin release in cyanobacteria Microcystis aeruginosa (Wu et al., 2016). Similarly, the stress from a high concentration of 2,4-D at 98% purity could activate a harmful effect evidently as chlorosis occurrence in Nostoc hatei strain TISTR 8405 (Pimda and Bunnag, 2017). Nevertheless, the organic solvents and surfactants present in some of the herbicide formulations could alter the toxicity of those herbicides on cyanobacteria. Pereira et al. (2009) indicated higher antialgal toxicity of Spasors formulation than that of sole glyphosate, its active ingredient. Therefore, the study on the toxicity of glyphosate and 2,4-D should be conducted using the commercial formula rather than the pure chemicals. Moreover, there are relatively few data reported for the herbicide's toxicity at very low concentrations relative to the residue levels.
In this study, the effects of low dose exposure of commercial herbicide formulations of glyphosate and 2,4-D on the growth of Nostoc sp. N1 and Oryza sativa L. cv. San-Pah-Tawng 1 seedlings were investigated. Nostoc sp. N1 was chosen as a model organism based on its habitat and rice growth-promoting characteristics. By using the environmentally relevant concentrations of the commercial herbicide formulations of glyphosate and 2,4-D obtained from previous studies, the findings could be useful for the assessment of actual herbicide toxicity on rice and the rice-field cyanobacteria.
Materials and Methods
Herbicides, Cyanobacteria, and Rice
Two commercial herbicide formulations were obtained from different companies: (1) Roundup [(N-(phosphonomethyl) 48% glycine (w/v) SL)] was purchased from Monsanto, USA, and (2) Keystone [84% 2-(2,4-dichlorophenoxy) acetic acid (w/v) SL] was purchased from Amada, Thailand. The herbicide concentrations used in the experiments were prepared by diluting with de-ionized (DI) water based on the percentage of their active ingredient in the formulations. Nostoc sp. N1 was isolated from paddy fields in Phayao Province (Tansai et al., 2018) and taxonomically identified as Nostoc sp. based on the morphological characteristics presented in the standard literatures (Desikachary, 1959; Komárek and Anagnostidis, 1989) (Supplementary Figure 1). Oryza sativa L. cv. San-Pah-Tawng 1 rice was obtained from the Phayao Rice Seed Center, Phayao, Thailand.
Growth of Nostoc sp. N1
The growth of Nostoc sp. N1 was determined based on the level of chlorophyll a. The chlorophyll a content was determined according to the method of Wintermans and De Mots (1965). For each treatment, Nostoc sp. N1 cells were grown in nitrogen-free BG-11 liquid medium before incubation at room temperature and illumination under fluorescent lamps with a 12:12 h light-dark photoperiod of 4,000 lux. The growth was then determined by measuring the chlorophyll a content every 3 days until the end of the experiment (30 days).
Effect of Herbicides on Nostoc sp. N1
Twelve-day cultivated Nostoc sp. N1 was used as an inoculum. The cells were grown in a 250 mL flask containing N-free BG-11 media supplemented with different concentrations of glyphosate (0.1 and 0.5 mg/L) and 2,4-D herbicides (0.05 and 0.1 mg/L) at room temperature and illuminated under fluorescent lamps with a 12:12 h light-dark photoperiod of 4,000 lux. The concentrations of herbicides used in this experiment were selected based on their environmentally relevant concentrations in water reported by other studies (Table 1 and Supplementary Table 1), and their minimum inhibitory concentration (MIC) on the growth of rice seedling was obtained from our preliminary test (Supplementary Table 2). Cell biomass (on a dry weight basis) was determined every 3 days for 12 days until the stationary phase was reached. Nostoc sp. N1 cultures were harvested by filtering through a Whatman GF/C glass microfiber filter (GE Healthcare, Leicestershire, UK), then oven-dried at 80°C overnight and weighed. The chlorophyll a content was determined as described above. The phycocyanin content was also investigated. Briefly, The Nostoc sp. N1 suspension was filtered with a GF/C filter paper to obtain cell pellet of 100 mg. Pellet cells were homogenized in 1 mL of 0.2 M phosphate buffer solution (pH 7). Five freeze–thaw cycles were carried out and then centrifuged (TD5, Yingtai Instrument, China) at 6,000 rpm and 4°C for 15 min. The supernatant was carefully separated from the sediment. The absorbance was measured at 615 and 652 nm (GENESYS™ 10S UV-Vis Spectrophotometer, Thermo Scientific™, Germany), and the phycocyanin concentration was calculated according to Bennett and Bogorad (1973).
Acute toxicity of the herbicides was determined from the median inhibitory concentrations (EC50) according to the modified method of Bérard et al. (1999). Nostoc sp. N1 cells in the log phase from stock culture were added to different dilutions of the herbicides. After the cells were exposed to the herbicides for 96 h, their chlorophyll a content was measured. The estimated EC50 value was calculated by plotting a dose-response curve between the herbicide concentrations and Nostoc sp. N1 chlorophyll a content using a linear regression equation.
Effects of Herbicides and Nostoc sp. N1 on the Germination of Rice Seedlings
Healthy rice seeds were surface sterilized with 10% sodium hypochlorite for 10 min and then washed in distilled water three times. The seeds were sown in sterile Petri dishes filled with filter paper; 10 mL of the test solutions was then added to each Petri dish. The different treatments were as follows: (1) Nostoc sp. N1 cells applied at concentrations of 5 and 10 g/L; (2) glyphosate and 2,4-D herbicides applied at different concentrations (0.5, 1.0, 2.5, and 5 mg/L glyphosate; 0.01, 0.1, 1.0, and 5.0 mg/L 2,4-D); (3) a mixture of Nostoc sp. N1 cells and the glyphosate or 2,4-D herbicides applied; and (4) neither Nostoc sp. N1 cells or herbicides was applied (control) (Supplementary Table 3). Germination was recorded at the interval of 24 h until 7 days after sowing, and then the seedling vigor index (SVI) was determined (Abdul-Baki and Anderson, 1973). Moreover, the physical parameters, which are root and shoot lengths and fresh and dry weights of the seedlings, were measured 7 days after sowing. The phytotoxicity index, which is the average of the phytotoxicity classes calculated based on the percent reduction in growth and physiological parameters, was calculated according to the method described by Aziz et al. (2015) (Supplementary Table 4).
Determination of Suitable Media and Application Methods for a Pot Experiment
To evaluate the suitability of culture media and the application method of Nostoc sp. N1 to the soil, BG-11 media and nitrogen-free BG-11 (N-free BG-11) media were separately used for culturing of Nostoc sp. N1. Experimental soil was excavated from the surface of a paddy field in Phayao Province (0–20 cm depth), after which it was air-dried, ground and sieved through a 6.00 mm sieve to remove rock and plant fragments. Plastic pots, each containing 500 g of the sieved and air-dried topsoil, were then prepared. Two application methods of Nostoc sp. N1 were compared: (1) mixing of Nostoc sp. N1 cells into the soil, and (2) applying Nostoc sp. N1 cells to the surface of the soil. The concentration of 10 g/kg of Nostoc sp. N1 was mixed into the soil or poured onto the surface of the soil.
Healthy rice seeds were surface sterilized with 10% sodium hypochlorite for 10 min, then rinsed 3 times with distilled water. The rice seeds were soaked in DI water overnight, then wrapped in a wet sheet cloth and incubated in the dark until germination. Consequently, ten germinated seeds were sown in the plastic pots containing the prepared soil and watered every 2 days with 20 mL of distilled water. Rice seedlings were grown under fluorescent lamps with a 12:12 h light-dark photoperiod of 4,000 lux. The experiment was arranged in a randomized complete block design with three replicates. The physical characteristics, i.e., root and shoot lengths along with fresh and dry weights, were measured after 15 days of application. The best application method was selected for subsequent experiments.
Effects of Herbicides and Nostoc sp. N1 on the Growth of 21-Day-Old Rice Seedlings
The germinated rice seeds and soil were prepared as previously described. The concentrations of glyphosate and 2,4-D herbicides used in this experiment were selected based on their environmentally relevant concentrations in soil described by other studies (Table 1). Sieved and air-dried soil was mixed with selected concentrations of glyphosate herbicide (1.0 and 10.0 mg/kg), 2,4-D herbicide (0.1 and 1.0 mg/kg) and fresh Nostoc sp. N1 (10 g/kg) for the pot experiments. Rice was grown under fluorescent lamps with a 12:12 h light-dark photoperiod of 4,000 lux. After 21 days of application, the physical characteristics of rice seedlings were determined. The chlorophyll a content was analyzed as described previously. The total sugar content was quantified according to the phenol-sulfuric acid method (Dubois et al., 1956) and the total free amino acid content was determined according to the ninhydrin carbon dioxide method (Hamilton and Van-Slyke, 1943), using leucine as a standard.
Statistical Analysis
All experiments were performed in triplicate. The results are described in terms of the average ± standard deviation (SD). All the data were analyzed statistically by the analysis of variance followed by Duncan's multiple range test (DMRT) to verify the significant differences between the treatments at p ≤ 0.05.
Results
Effects of Herbicides on Nostoc sp. N1
The growth of Nostoc sp. N1, estimated from chlorophyll a content, was in the exponential phase from day 3 to day 12 before reaching the stationary phase and decreasing continuously after that (Supplementary Figure 2). Therefore, 12 days were chosen as the experimental time for subsequent experiments. After 12 days of exposure to the environmentally relevant concentrations of glyphosate and 2,4-D herbicides, the growth of Nostoc sp. N1 was significantly stimulated compared with the control condition (p < 0.05), and it was increased when the concentrations of the herbicides increased (Figure 1). The highest biomass and the maximum chlorophyll a content were observed at 0.5 mg/L of glyphosate and 0.05 mg/L of 2,4-D herbicides (Figures 1A–D). For the effects of herbicides on the content of phycocyanin, no difference was observed in the treatments compared with the control except at day 9 of the experiment where the amount of phycocyanin was significantly reduced by 0.5 mg/L of glyphosate (Figures 1E,F).
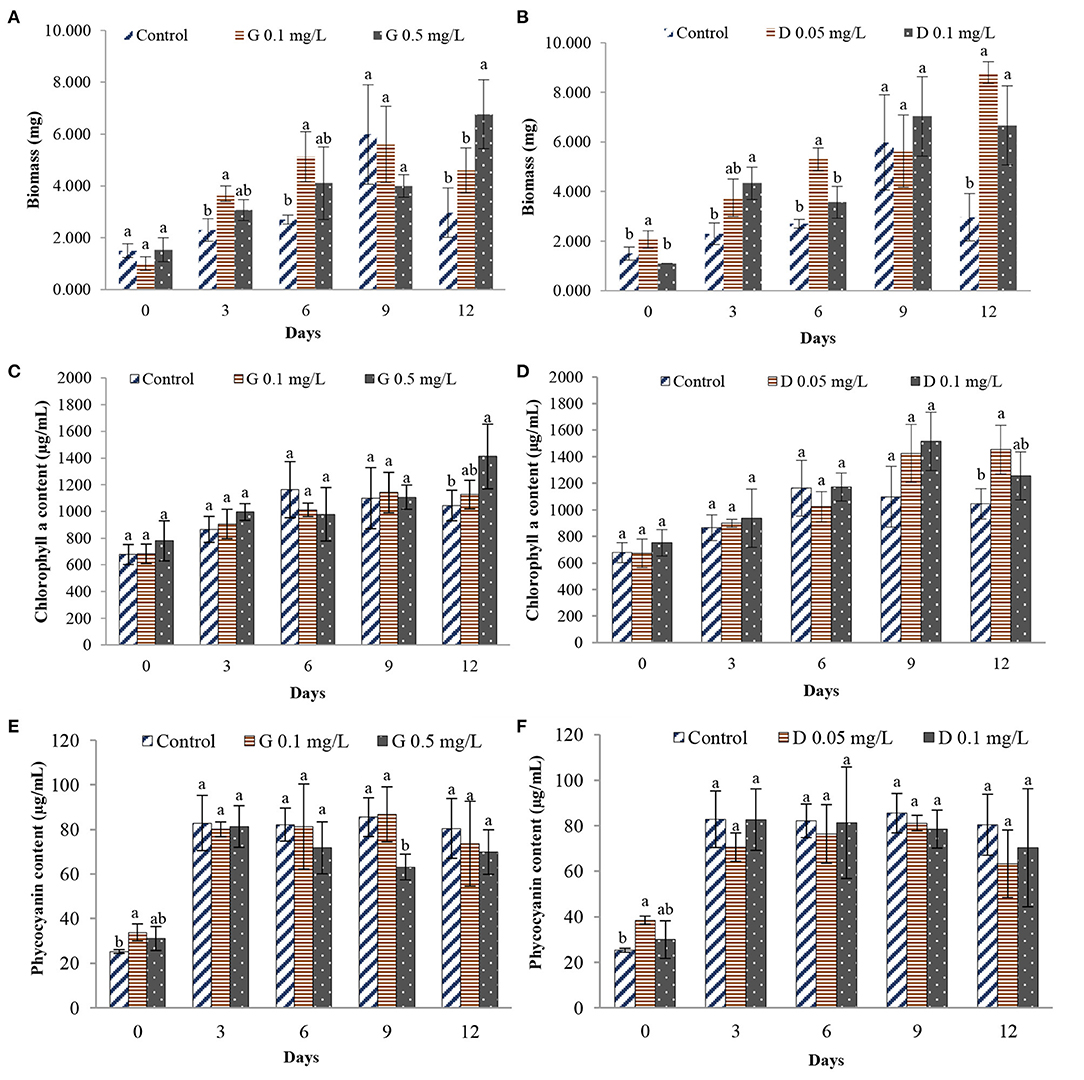
Figure 1. Effects of glyphosate and 2,4-D herbicides on the biomass and pigment contents of Nostoc sp. N1. (A) Effect of glyphosate herbicide on the biomass (B) Effect of 2,4-D herbicide on the biomass (C) Effect of glyphosate herbicide on the chlorophyll a content (D) Effect of 2,4-D herbicide on the chlorophyll a content (E) Effect of glyphosate herbicide on the phycocyanin content (F) Effect of 2,4-D herbicide on the phycocyanin content. G, Glyphosate herbicide; D, 2,4-D herbicide. The same letter are not significantly different at P = 0.05.
The median inhibitory concentrations (EC50) of glyphosate and 2,4-D herbicides were monitored for their acute toxicity to Nostoc sp. N1 cells. According to the results (Table 2), glyphosate was more toxic to Nostoc sp. N1 cells than 2,4-D with the EC50 values of 0.617 and 209.434 mg/L, respectively.
Effects of Herbicides and Nostoc sp. N1 on the Germination and Growth of Rice Seedlings
The effects of herbicides and Nostoc sp. N1 cells on the germination and growth of 7-day old rice seedlings were observed both separately and concurrently, and the results are shown in Table 3. The growth of rice seedlings was determined from the SVI and their physical characteristics. It was found that the addition of only Nostoc sp. N1 at 10 g/L promoted rice seedling growth with the highest SVI of 1,014.67 and 790 for both experiments conducted. Moreover, the growth-promoting efficiency of Nostoc sp. N1 could also be indicated by the positive value of percent increase of the treatment compared with that of the control (PIC). On the other hand, the addition of the herbicides tended to reduce the germination and growth of the rice seedlings (Supplementary Tables 5, 6). As the concentration of glyphosate herbicide increased from 0.5 to 5.0 mg/L, the PIC decreased from −10.44 to −79.20, respectively. Similar inhibitory effect was also found for 2,4-D herbicide at a concentration of 0.1–5.0 mg/L; however, a stimulatory effect was observed at the lowest concentration of 0.01 mg/L.
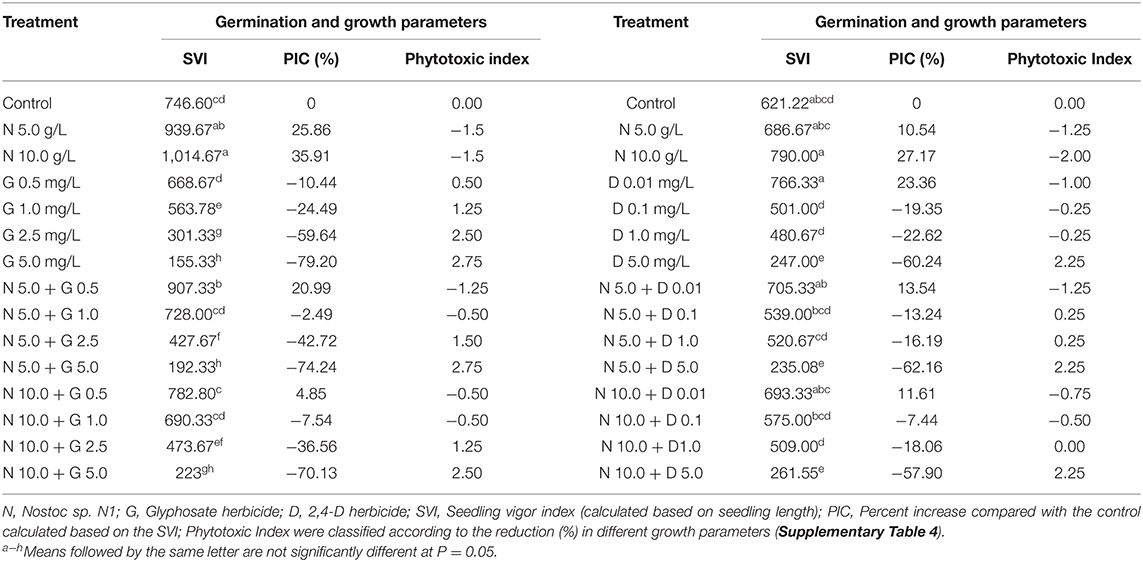
Table 3. Effects of glyphosate, 2,4-D herbicides and Nostoc sp. N1 cells on the germination and growth parameters of 7-day-old rice seedlings.
However, the addition of the Nostoc sp. N1 cells could alleviate the toxic effects of the herbicides on rice seedlings. When combined with either 5.0 and 10.0 g/L of Nostoc sp. N1, the treatments with glyphosate herbicide exhibited the increased PICs in all glyphosate concentrations (Table 3). Highest PICs were obtained at the lowest concentration of glyphosate herbicide (0.5 mg/L). Similarly, the addition of 2,4-D herbicide combined with Nostoc sp. N1 slightly increased the SVIs compared with those from the herbicide 2,4-D treatment alone.
The phytotoxicity index ranged between 0.00 (control) and 2.75 (5.0 mg/L of glyphosate herbicide). The higher value of phytotoxicity index demonstrated an adverse effect of both herbicides on physical and biochemical characteristics of rice seedling. Comparing the phytotoxicity index between two herbicides, glyphosate herbicide revealed higher toxicity than did 2,4-D herbicide. However, toxicity induced by those herbicides on rice seedling decreased with Nostoc sp. N1 addition as indicated by minimum phytotoxicity values (Table 3).
Effects of Herbicides and Nostoc sp. N1 on the Growth of Rice: A Pot Experiment
The physical characteristics of 15-day-old rice seedlings in response to the different types of BG-11 media and Nostoc sp. N1 application methods are shown in Table 4. From the results, both BG-11 and N-free BG-11 media caused no significant difference in any growth parameter compared with the control (p > 0.05). However, considering the Nostoc sp. N1 application methods, it was found that the application method of mixing Nostoc sp. N1 into the soil yielded higher numbers of all parameters than the method of applying Nostoc sp. N1 to the surface of the soil. Thus, the method of mixing Nostoc sp. N1 into the soil was selected for 21-day-old rice seedlings experiment.
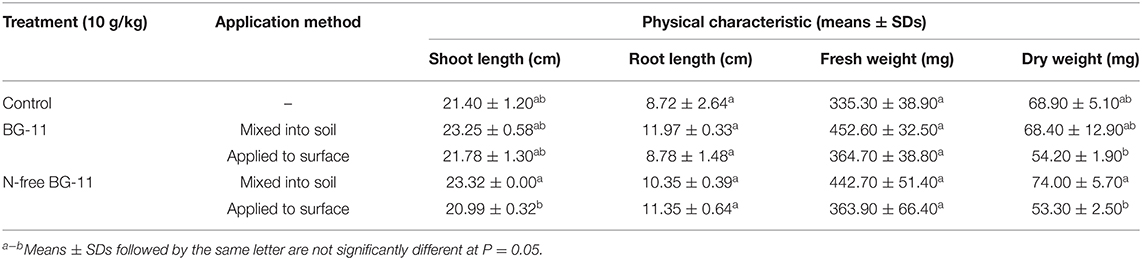
Table 4. Effects of different BG-11 media and application methods on the physical characteristics of rice seedlings.
For 21-day-old rice seedlings test (Table 5), it was found that both concentrations of glyphosate herbicide had no adverse effects on the dry weight of the seedlings. In contrast, rice seedlings were more strongly affected by 2,4-D herbicide than by glyphosate herbicide. The significantly lower dry weight was observed on the seedlings treated with both concentrations of 2,4-D herbicide compared with the seedlings in the other treatments (p < 0.05).
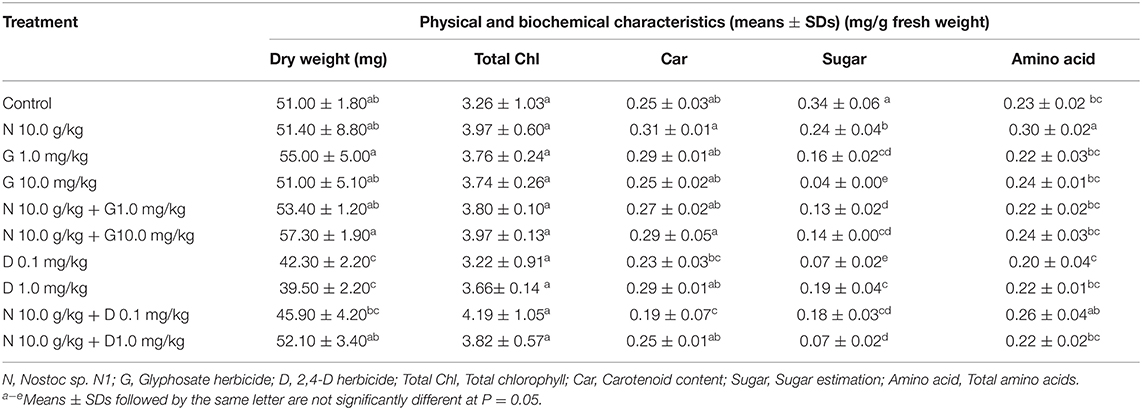
Table 5. Effects of glyphosate, 2,4-D herbicides and Nostoc sp. N1 on the physical and biochemical characteristics of 21-day-old rice seedlings.
Interestingly, Nostoc sp. N1 cells demonstrated the potential to relieve the toxicity of both herbicides at environmentally relevant concentrations. The low dry weight of the rice seedlings, either resulted from glyphosate or 2,4-D, could be rescued by the addition of 10 g/kg Nostoc sp. N1 cells (Table 5). Also, the addition of Nostoc sp. N1 cells alone or combined with the herbicides tended to increase the total chlorophyll and carotenoid contents of the seedlings. A significantly higher total amino acid content was also found in the seedlings treated with Nostoc sp. N1 cells (p < 0.05) than in the seedlings in the other treatments.
Discussion
Stimulation of Nostoc sp. N1 Cell Growth by the Herbicides
From the results, the growth of Nostoc sp. N1 was significantly stimulated after being exposed to both glyphosate and 2,4-D herbicides. Also, the growth stimulation was positively related to the concentrations of the herbicides applied. At 0.5 mg/L glyphosate and 0.05 mg/L 2,4-D herbicides (Figures 1A–D), the maximum chlorophyll a content was found. This result was supported by Wong (2000), who reported that the photosynthesis and chlorophyll a synthesis in Scenedesmus quadricauda was increased at 0.02 mg/L of glyphosate and 0.02 or 0.2 mg/L of 2,4-D. Whereas, 2 mg/L of glyphosate and 20 mg/L of 2,4-D significantly decreased the chlorophyll-a contents. Saygideger and Okkay (2008) evidenced the same result in Chlorella vulgaris and Spirulina plantensis exposed to 2,4-D.
In this study, the phycocyanin content in both herbicide treatments was not significantly different from the controls (Figures 1E,F). In contrast, a previous study reported significantly higher concentrations of the light-harvesting pigments, phycobiliprotein, in the cyanobacteria exposed to 2,4-D (Pumas et al., 2011). This might be because of the low concentrations of glyphosate and 2,4-D used in this study and possibly the extracellular polysaccharides produced by Nostoc sp. N1 to enhance protection against these herbicides.
The EC50 of glyphosate and 2,4-D herbicides suggested that Nostoc sp. N1 was more tolerant to 2,4-D than to glyphosate herbicides (Table 2). Higher toxicity of glyphosate to Nostoc sp. N1 cells could be due to its mechanism to prevent the biosynthesis of amino acids by inhibiting 5-enol-pyruvylshikimate-3-phosphate (EPSP) synthase in the shikimate pathway (Smedbol et al., 2017) and inhibiting ammonium uptake (Singh et al., 1989). For 2,4-D, its plant growth regulator characteristics might stimulate the growth of Nostoc sp. N1, resulting in less toxicity. It was found that high concentrations of 2,4-D inhibited growth and photosynthesis in Chlorella elipsoidea, but low concentrations could stimulate the parameters (Chai and Chung, 1975), which could be the case in this study. It should be noted that this study demonstrated a low EC50 value of glyphosate (0.617 mg/L) while other studies have shown higher values up to 120 mg/L for Nostoc sp. and 20 mg/L for Nostoc muscorum (He et al., 2013; Anees et al., 2014). The variations of EC50 values depends on the species of Nostoc and the different market-available formulations of herbicides used in the experiments. For example, the presence of isopropylamine in commercial Roundup formulations is of vital importance with respect to the toxicity of this herbicide toward aquatic phytoplankton (Lipok et al., 2010).
Reduction of the Herbicide Toxic Effects to Rice Seedlings by Nostoc sp. N1
The addition of only Nostoc sp. N1 (10 g/L) promoted rice seedling growth as observed by SVI and PIC data; on the other hand, the addition of glyphosate or 2,4-D reduced the germination and growth of the rice seedlings (Table 3). However, a stimulatory effect of 2,4-D was found at its lowest concentration (0.01 mg/L). Since 2,4-D herbicide has a similar structure to indol-3-acetic acid (IAA), the herbicide at low concentrations might act as an auxin-like hormone to promote plant growth, while at high doses, it drives excessive overgrowth (Grossmann, 2000).
The toxic effects of both glyphosate and 2,4-D on the rice seedlings could be reduced by the combined addition with Nostoc sp. N1 as clearly observed via SVI (Table 3). This phenomenon might be explained by two reasons. Firstly, nitrogen-fixing cyanobacteria can produce phytohormones such as auxin, gibberellins and cytokinin that promote the growth of rice (Hashtroudi et al., 2013; Singh et al., 2014). Secondly, Nostoc application could enhance the organic carbon and nitrogen content of the surface soil and promote plant growth and iron uptake of plants (Obana et al., 2007). A previous study showed that Nostoc carneum and N. commune promoted Pathum Thani rice seedling growth and yield, resulting in a decrease in rice production costs without negatively affecting on the environment (Bhooshan et al., 2018).
According to the results from the pot experiments (21-day-old rice seedlings), glyphosate herbicide showed no adverse effects on the dry weight of the rice seedlings while 2,4-D herbicide significantly had a negative effect on this parameter (Table 5). Glyphosate tend to be bound tightly in most soils with a Koc of 24,000 mL/g (Wauchope et al., 1992; Duke and Powles, 2008) compared with 2,4-D with a lower Koc of 61.7 mL/g (U. S., Environmental Protection Agency, 2005). This could lead to more leaching of 2,4-D by water and more uptake by rice seedlings, resulting in more adverse effects. Moreover, glyphosate might benefit cyanobacteria by providing a source of nutritive carbon, nitrogen or phosphorus that is as beneficial as inorganic phosphate (Drzyzga and Lipok, 2018), leading to less toxicity than 2,4-D.
Similar to the Petri dish experiments, Nostoc sp. N1 cells could reduce the toxicity of both herbicides at environmentally relevant concentrations chosen in this study. Moreover, the biochemical parameters, i.e., total chlorophyll and carotenoid contents of the seedlings, were found to be increased by Nostoc sp. N1 cells. It has been known that Nostoc spp. has the ability to reduce the toxic stress of herbicides by producing polysaccharides to protect themselves from herbicide exposure or stress conditions (Arora et al., 2010). Cyanobacteria also play an essential role in the soil by fixing carbon and nitrogen, which increases the fertility of and water retention in the soil (Chamizo et al., 2018). Therefore, cyanobacteria in paddy fields like Nostoc spp. could potentially act as a biodegrading organism to eliminate various types of agrochemical residues, benefiting the ecosystem quality.
Glyphosate and 2,4-D account for a considerable herbicide market share worldwide. However, information concerning the effects of their residues at environmentally relevant concentrations on the growth of important cyanobacteria and rice seedlings is still limited. In this study, environmentally relevant concentrations of both glyphosate and 2,4-D herbicides did not significantly affect the growth of pigment contents of Nostoc sp. N1. Furthermore, Nostoc sp. N1 cells promoted plant germination and growth by increasing several physical and biochemical characteristics of the rice seedlings. The application of Nostoc sp. N1 combined with both herbicides in the soil did not cause adverse effects on the growth of rice. In contrast, it seemed to promote several growths and biochemical parameters of the rice seedlings. These findings suggested that the environmentally relevant concentration of herbicide formulations used in this work did not adversely affect either Nostoc sp. N1 or the rice seedlings. Thus, Nostoc spp. could play an important role in paddy fields in terms of primary production and could alleviate the toxicity of herbicides via growth-stimulating effects on rice seedlings.
Data Availability Statement
The original contributions presented in the study are included in the article/Supplementary Material, further inquiries can be directed to the corresponding author/s.
Author Contributions
KI was primarily responsible for the project plan, experimental design, data analyses, and all manuscript drafts. ST, NN, OC, and PT designed and performed the experiments. ST contributed to the statistical data analyses and composing figures. This work was carried out in collaboration between all authors. All authors have read the manuscript and approved this submission.
Funding
This research was financially supported by the research grant of University of Phayao through National Research Council of Thailand (No. 352768) and partially supported by Chiang Mai University.
Conflict of Interest
The authors declare that the research was conducted in the absence of any commercial or financial relationships that could be construed as a potential conflict of interest.
Acknowledgments
The authors would like to thank the Phayao Rice Seed Center for supporting the rice seedlings in this experiment.
Supplementary Material
The Supplementary Material for this article can be found online at: https://www.frontiersin.org/articles/10.3389/fsufs.2021.661634/full#supplementary-material
References
Abdul-Baki, A. A., and Anderson, J. D. (1973). Vigor determination in soybean seed by multiple criteria. Crop. Sci. 13, 630–633. doi: 10.2135/cropsci1973.0011183X001300060013x
Al-Rajab, A. J., and Schiavon, M. (2010). Degradation of 14C-glyphosate and aminomethylphosphonic Acid (AMPA) in three agricultural soils. J. Environ. Sci. 22, 1374–1380. doi: 10.1016/S1001-0742(09)60264-3
Anees, S., Suhail, S., Pathak, N., and Zeeshan, M. (2014). Potential use of rice field cyanobacterium Nostoc muscorum in the evaluation of butachlor induced toxicity and their degradation. Bioinformation 10, 365–370. doi: 10.6026/97320630010365
Aparicio, V., De Gerónimo, E., Marino, D., Primost, J., Carriquiriborde, P., and Costa, J. (2013). Environmental fate of glyphosate and aminomethylphosphonic acid in surface waters and soil of agricultural basins. Chemosphere 93, 1866–1873. doi: 10.1016/j.chemosphere.2013.06.041
Arora, M., Kaushik, A., Rani, N., and Kaushik, C. P. (2010). Effect of cyanobacterial exopolysaccharides on salt stress alleviation and seed germination. J. Environ. Biol. 31, 701–704.
Aziz, H., Sabir, M., Ahmad, H. R., Aziz, T., Zia-ur-Rehman, M., Hakeem, K. R., et al. (2015). Alleviating effect of calcium on nickel toxicity in rice. CLEAN Soil Air Water. 43, 901–909. doi: 10.1002/clen.201400085
Baumgartner, D., Souza, E. G. D., Coelho, S. R. M., and Maggi, M. F. (2017). Correlation between 2,4-D herbicide residues and soil attributes in southern of Brazil. Rev. Ciênc. Agron. 48, 428–437. doi: 10.5935/1806-6690.20170050
Bennett, A., and Bogorad, L. (1973). Complementary chromatic adaptation in a filamentous blue-green alga. J. Cell Biol. 58, 419–435. doi: 10.1083/jcb.58.2.419
Bérard, A., Leboulanger, S., and Pelte, T. (1999). Tolerance of Oscillatoria limnetica Lemmermann to atrazine in natural phytoplankton populations and in pure culture: influence of season and temperature. Arch. Environ. Contam. Toxicol. 37, 472–479. doi: 10.1007/s002449900541
Bhooshan, N., Pabbi, S., Singh, A., Sharma, A., Verma, C., Jaiswal, A., et al. (2018). Impact of blue green algae (BGA) technology: an empirical evidence from northwestern Indo-Gangetic Plains. 3 Biotech 8, 324–324. doi: 10.1007/s13205-018-1345-5
Chai, I. K., and Chung, Y. S. (1975). Physiological effects of 2,4-D on Chlorella ellipsoidea. Misaenglum Hakhoe Chi. 13, 101–108.
Chamizo, S., Mugnai, G., Rossi, F., Certini, G., and De Philippis, R. (2018). Cyanobacteria inoculation improves soil stability and fertility on different textured soils: Gaining insights for applicability in soil restoration. Front. Environ. Sci. 6, 1–14. doi: 10.3389/fenvs.2018.00049
Chen, Z., Juneau, P., and Qiu, B. (2007). Effects of three pesticides on the growth, photosynthesis and photoinhibition of the edible cyanobacterium Ge-Xian-Mi (Nostoc). Aquat. Toxicol. 81, 256–265. doi: 10.1016/j.aquatox.2006.12.008
Chittapun, S., Limbipichai, S., Amnuaysin, N., Boonkerd, R., and Charoensook, M. (2017). Effects of using cyanobacteria and fertilizer on growth and yield of rice, Pathum Thani I: a pot experiment. J. Appl. Phycol. 30, 79–85. doi: 10.1007/s10811-017-1138-y
Clay, J. W. (2004). World Agriculture and the Environment: A Commodity-by-Commodity Guide to Impacts and Practices. Washington: Island Press.
Costa, O., Raaijmakers, J. M., and Kuramae, E. E. (2018). Microbial extracellular polymeric substances: ecological function and impact on soil aggregation. Front. Microbiol. 9:1636. doi: 10.3389/fmicb.2018.01636
Curi, L. M., Peltzer, P. M., Sandoval, M. T., and Lajmanovich, R. C. (2019). Acute toxicity and sublethal effects caused by a commercial herbicide formulated with 2,4-D on Physalaemus albonotatus tadpoles. Water Air Soil Pollut. 230, 1–15. doi: 10.1007/s11270-018-4073-x
Dash, N. P., Kaushik, M. S., Kumar, A., Abraham, G., and Singh, P. K. (2017). Agrochemicals influencing nitrogenase, biomass of N2 fixing cyanobacteria and yield of rice in wetland cultivation. Biocat. Agri. Biotechnol. 9, 28–34. doi: 10.1016/j.bcab.2016.11.001
Department of Agriculture, Ministry of Agriculture Co-operative. (2020). List of the Import of Agricultural Hazardous Substances (in Thai). Available online at: http://www.doa.go.th/ard/wp-content/uploads/2020/02/HASTAT62_03.pdf (accessed July 16, 2020).
Drzyzga, D., and Lipok, J. (2018). Glyphosate dose modulates the uptake of inorganic phosphate by freshwater cyanobacteria. J. Appl. Phycol. 30, 299–309. doi: 10.1007/s10811-017-1231-2
Dubois, M., Gilles, K. A., Hamilton, J. K., Rebers, P. A., and Smith, F. (1956). Colorimetric method for determination of sugars and related substances. Anal. Chem. 28, 350–356. doi: 10.1021/ac60111a017
Duke, S. O., and Powles, S. B. (2008). Glyphosate: a once-in-a-century herbicide. Pest Manag. Sci. 64, 319–325. doi: 10.1002/ps.1518
El-Nahhal, Y., Kerkez, S., and Abu Heen, Z. H. (2015). Toxicity of diuron, diquat and terbutryn to cyanobacterial mats. Ecotoxicol. Environ. Contam. 10, 71–82. doi: 10.5132/eec.2015.01.11
Félix–Cañedo, T. E., Durán–Álvarez, J. C., and Jiménez–Cisneros, B. (2013). The occurrence and distribution of a group of organic micropollutants in Mexico City's water source. Sci. Total Environ. 454–455, 109–118. doi: 10.1016/j.scitotenv.2013.02.088
Fisher, R. S., Davidson, B., and Goodmann, P. T. (2007). Groundwater Quality in Watersheds of the Kentucky River, Salt River, Licking River, Big Sandy River, Little Sandy River, and Tygarts Creek. Kentucky Geological Survey, University of Kentucky. Available online at: https://kgs.uky.edu/kgsweb/olops/pub/kgs/water/RI16_12/RI16entire.pdf (accessed March 23, 2019).
Forlani, G., Pavan, M., Gramek, M., Kafarski, P., and Lipok, J. (2008). Biochemical bases for a widespread tolerance of cyanobacteria to the phosphonate herbicide glyphosate. Plant Cell Physiol. 49, 443–456. doi: 10.1093/pcp/pcn021
Giesy, J. P., Dobson, S., and Solomon, K. R. (2000). Ecotoxicological risk assessment for Roundup® Herbicide. Rev. Environ. Contam. Toxicol. 167, 120–135. doi: 10.1007/978-1-4612-1156-3_2
Grossmann, K. (2000). Mode of action of auxin herbicides: a new ending to a long, drawn out story. Trends Plant Sci. 5, 506–508. doi: 10.1016/S1360-1385(00)01791-X
Hagner, M., Mikola, J., Saloniemi, I., Saikkonen, K., and Helander, M. (2019). Effects of a glyphosate-based herbicide on soil animal trophic groups and associated ecosystem functioning in a northern agricultural field. Sci. Rep. 9, 1–13. doi: 10.1038/s41598-019-44988-5
Hamilton, P. B., and Van-Slyke, D. D. (1943). Amino acid determination with ninhydrin. J. Biol. Chem. 150, 231–233. doi: 10.1016/S0021-9258(18)51268-0
Hashtroudi, M. S., Ghassempour, A., Riahi, H., Shariatmadari, Z., and Khanjir, M. (2013). Endogenous auxins in plant growth-promoting cyanobacteria Anabaena vaginicola and Nostoc calcicola. J. Appl. Phycol. 25, 379–386. doi: 10.1007/s10811-012-9872-7
Haynes, D., Müller, J., and Carter, S. (2000). Pesticide and herbicide residues in sediments and seagrasses from the Great Barrier Reef world heritage area and Queensland Coast. Mar. Pollut. Bull. 41, 279–287. doi: 10.1016/S0025-326X(00)00097-7
He, H., Li, Y., Chen, T., Huang, X., Guo, Q., Li, S., et al. (2013). Butachlor induces some physiological and biochemical changes in a rice field biofertilizer cyanobacterium. Pestic. Biochem. Phys. 105, 224–230. doi: 10.1016/j.pestbp.2013.02.009
Helander, M., Pauna, A., Saikkonen, K., and Saloniemi, A. (2019). Glyphosate residues in soil affect crop plant germination and growth. Sci. Rep. 9:19653. doi: 10.1038/s41598-019-56195-3
Ismail, B. S., Sameni, M., and Muhamad, H. (2011). Evaluation of herbicide pollution in the kerian rice fields of Perak, Malaysia. World Appl. Sci. J. 15, 5–13. Available online at: http://www.aquarius-systems.com/userdata/documents/PesticideUseCausesUndesirableEffectstotheNaturalEnvironment.pdf (accessed 27 March 2021).
Jantawongsri, K., Thammachoti, P., Kitana, J., Khonsue, W., Varanusupakul, P., and Kitana, N. (2015). Altered immune response of the rice frog Fejervarya limnocharis living in agricultural area with intensive herbicide utilization at Nan Province, Thailand. EnvironmentAsia 8, 68–74. doi: 10.14456/ea.2015.9
Kim, J., and Lee, C. (2006). Antialgal effect of a novel polysaccharolytic Sinorhizobium kostiense AFK-13 on Anabaena flos-aquae causing water bloom. J. Microbiol. Biotechnol. 16, 1613–1621.
Kjaer, J., Olsen, P., Barlebo, H. C., Juhler, R. K., Plauborg, F., et al. (2004). The Danish Pesticide Leaching Assessment Programme: Monitoring Results 1999–2003. Available online at: https://www.researchgate.net/publication/216075829_The_Danish_Pesticide_Leaching_Assessment_Programme_Monitoring_results_1999-2003 (accessed November 6, 2020).
Kollman, W., and Segawa, R. (1995). Interim Report of the Pesticide Chemistry Database. Environmental Protection Agency Department of Pesticide Regulation. Available online at: http://citeseerx.ist.psu.edu/viewdoc/download?doi=10.1.1.432.8080&rep=rep1&type=pdf (accessed March 27, 2021).
Komárek, J., and Anagnostidis, K. (1989). Modern approach to the classification system of Cyanophytes 4. Nostocales. Arch. Hydrobiol. 82, 247–345.
Kophimail, W., and Bunnag, S. (2014). “Biodegradation of 2,4-D by Nostoc hatei and Anabaena lutea,” in proceedings of the 15th Khon Kaen University Graduate Research Conference. Khon Kaen, Thaialnd. Available online at: https://gsbooks.gs.kku.ac.th/57/grc15/files/bmp19.pdf (accessed July 8, 2020).
Laitinen, P., Ramo, S., and Siimes, K. (2007). Glyphosate translocation from plants to soil - Does this constitute a significant proportion of residues in soil? Plant Soil 300, 51–60. doi: 10.1007/s11104-007-9387-1
Lipok, J., Studnik, H., and Gruyaert, S. (2010). The toxicity of Roundup® 360 SL formulation and its main constituents: glyphosate and isopropylamine towards non-target water photoautotrophs. Ecotox. Environ. Safe. 73, 1681–1688. doi: 10.1016/j.ecoenv.2010.08.017
Obana, S., Miyamoto, K., Morita, S., Ohmori, M., and Inubushi, K. (2007). Effect of Nostoc sp. on soil characteristics, plant growth and nutrient uptake. J. Appl. Phycol. 19, 641–646. doi: 10.1007/s10811-007-9193-4
Oberemok, V. V., Laikova, K. V., Gninenko, Y. I., Zaitsev, A. S., Nyadar, P. M., and Adeyemi, T. A. (2015). A short history of insecticides. J. Plant Prot. Res. 55, 221–226. doi: 10.1515/jppr-2015-0033
Paipard, N., Supannatas, S., and Suttiprapa, T. (2014). Effects of pesticide use on farmer's health and the environment in Rong Kham district, Kalasin province. Khon Kaen Agr. J. 42, 301–310. Available online at: https://ag2.kku.ac.th/kaj/PDF.cfm?filename=05%20Natawut.pdf&id=1711&keeptrack=3 (accessed 25 January 2020).
Pereira, J. L., Antunes, S. C., Castro, B. B., Marques, C. R., Goncalves, A. M. M., Goncalves, F., et al. (2009). Toxicity evaluation of three pesticides on non-target aquatic and soil organisms: commercial formulation versus active ingredient. Ecotoxicology 18, 455–463. doi: 10.1007/s10646-009-0300-y
Peruzzo, P. J., Porta, A., and Ronco, A. E. (2018). Levels of glyphosate in surface waters, sediments and soils associated with direct sowing soybean cultivation in North Pampasic region of Argentina. Environ. Pollut. 156, 61–66. doi: 10.1016/j.envpol.2008.01.015
Pimda, W., and Bunnag, S. (2017). Effect of heavy metals and inorganic nutrients existing as co-contaminants on bioremoval of 2,4-dichlorophenoxyacetic acid (2,4-D) by Nostoc hatei TISTR 8405. Arab. J. Sci. Eng. 42, 2291–2301. doi: 10.1007/s13369-017-2492-4
Pumas, C., Vacharapiyasophon, P., Peerapornpisal, Y., Leelapornpisid, P., Boonchum, W., Ishii, M., et al. (2011). Thermostablility of phycobiliproteins and antioxidant activity from four thermotolerant cyanobacteria. Phycol. Res. 59, 166–174. doi: 10.1111/j.1440-1835.2011.00615.x
Saygideger, S. D., and Okkay, O. (2008). Effect of 2,4-dichlorophenoxyacetic acid on growth, protein and chlorophyll-a content of Chlorella vulgaris and Spirulina platensis cells. J. Environ. Biol. 29, 175–178.
Sheeba, S. V. P., Srivastava, P. K., and Prasad, S. M. (2011). Differential physiological and biochemical responses of two cyanobacteria Nostoc muscorum and Phormidium foveolarum against oxyfluorfen and UV-B radiation. Ecotoxicol. Environ. Saf. 74, 1981–1993. doi: 10.1016/j.ecoenv.2011.07.006
Silva, V., Montanarella, L., Jones, A., Fernandez-Ugalde, O., Mol, H. G. J., Ritsema, C. J., et al. (2018). Distribution of glyphosate and aminomethylphosphonic acid (AMPA) in agricultural topsoils of the European Union. Sci. Total Environ. 621, 1352–1359. doi: 10.1016/j.scitotenv.2017.10.093
Singh, A. K., Sailaja, M. V., and Singh, H. N. (1989). A class of glyphosate-selected mutants of the cyanobacterium Nostoc muscorum showing loss of ammonium transport activity (Amt–), heterocyst formation (Het–) and nitrogenase activity (Nif–). FEMS Microbiol. Lett. 60, 187–191. doi: 10.1016/0378-1097(89)90506-5
Singh, H., Khattar, J. I. S., and Ahluwalia, A. (2014). Cyanobacteria and agricultural crops. Vegetos 27, 37–44. doi: 10.5958/j.2229-4473.27.1.008
Smedbol, É., Lucotte, M., Labrecque, M., Lepage, L., and Juneau, P. (2017). Phytoplankton growth and PSII efficiency sensitivity to a glyphosate-based herbicide (Factor 540®). Aquat. Toxicol. 192, 265–273. doi: 10.1016/j.aquatox.2017.09.021
Tansai, S., Issakul, K., and Ngearnpat, N. (2018). “Toxicity of paraquat on growth of cyanobacteria (Nostoc sp. N1 and Anabaena sp. A1) and germination of rice seed (san-pah-twang 1),” in Proceedings of the 6th Biochemistry and Molecular Biology International Conference (6th BMB). Rayong, Thailand. Available online at: http://www.scisoc.or.th/BMBThailand/images/BMB2018/S7-P-09.pdf (accessed March 9, 2020).
U. S., Environmental Protection Agency (2005). Reregistration eligibility decision for2,4-D (EPA 738-R-05-002). EPA Archive document. Available online at: https://archive.epa.gov/pesticides/reregistration/web/pdf/24d_red.pdf (accessed January 15, 2020).
Wauchope, R. D., Buttler, T. M., Hornsby, A. G., Augustijn-Beckers, P. W. M., and Burt, J. P. (1992). The SCS/ARS/CES pesticide properties database for environmental decision-making. Rev. Enviro. Contam. Toxicol. 123, 1–155. doi: 10.1007/978-1-4612-2862-2_1
Wintermans, J. F., and De Mots, A. (1965). Spectrophotometric characteristics of chlorophylls a and b and their phenophytins in ethanol. Biochim. Biophys. Acta. 109, 448–453. doi: 10.1016/0926-6585(65)90170-6
Wong, P. K. (2000). Effects of 2,4-D, glyphosate and paraquat on growth, photosynthesis and chlorophyll–a synthesis of Scenedesmus quadricauda Berb 614. Chemosphere 41, 177–182. doi: 10.1016/S0045-6535(99)00408-7
Wu, L., Qiu, Z., Zhou, Y., Du, Y., Liu, C., Ye, J., et al. (2016). Physiological effects of the herbicide glyphosate on the cyanobacterium Microcystis aeruginosa. Aquat. Toxicol. 178, 72–79. doi: 10.1016/j.aquatox.2016.07.010
Xu, Y., Li, A. J., Li, K., Qin, J., and Li, H. (2017). Effects of glyphosate-based herbicides on survival, development and growth of invasive snail (Pomacea canaliculata). Aquat. Toxicol. 193, 136–143. doi: 10.1016/j.aquatox.2017.10.011
Keywords: herbicide toxicity, bio-fertilizers, paddy field cyanobacteria, plant growth-stimulating effects, pesticide residues
Citation: Tansay S, Issakul K, Ngearnpat N, Chunhachart O and Thuptimdang P (2021) Impact of Environmentally Relevant Concentrations of Glyphosate and 2,4-D Commercial Formulations on Nostoc sp. N1 and Oryza sativa L. Rice Seedlings. Front. Sustain. Food Syst. 5:661634. doi: 10.3389/fsufs.2021.661634
Received: 31 January 2021; Accepted: 15 March 2021;
Published: 12 April 2021.
Edited by:
Everlon Cid Rigobelo, Universidade Estadual Paulista, BrazilReviewed by:
Roberta Mendes, Universidade Estadual Paulista, BrazilAmarendra Narayan Misra, Central University of Jharkhand, India
Copyright © 2021 Tansay, Issakul, Ngearnpat, Chunhachart and Thuptimdang. This is an open-access article distributed under the terms of the Creative Commons Attribution License (CC BY). The use, distribution or reproduction in other forums is permitted, provided the original author(s) and the copyright owner(s) are credited and that the original publication in this journal is cited, in accordance with accepted academic practice. No use, distribution or reproduction is permitted which does not comply with these terms.
*Correspondence: Kritchaya Issakul, S2lzc2FrdWxAZ21haWwuY29t