- 1Institute of Applied Science and Technology, College of Basic and Applied Sciences, University of Ghana, Accra, Ghana
- 2Department of Crop Science, School of Agriculture, College of Basic and Applied Sciences, University of Ghana, Accra, Ghana
Tomato is an important vegetable in Ghanaian diet and contributes enormously in livelihood improvement. Tomato production is threatened by a high prevalence of biotic and abiotic stresses as well as increased postharvest losses and poor agronomic practices, thereby resulting in massive importation of tomato and its products to meet the local demands. The recent introduction of greenhouse vegetable cultivation technology in Ghana is a sustainable attempt in addressing and ensuring year-round production of vegetables including tomato. However, research on agronomic practices targeted to improving yield and fruit quality under greenhouse conditions in Ghana is scarcely available. Therefore, this study seeks to evaluate the effect of plant spacing and topping on tomato yield and fruit quality under greenhouse conditions. A 3 ×3 factorial treatment arranged in a completely randomized design (CRD) with three replications was used. Two factors, plant spacing and topping with each having three levels, were used. Thus, the levels for plant spacing were 0.15 m × 1.3 m, 0.2 m × 1.3 m, and 0.3 m × 1.3 m while topping treatments at trusses 2, 3, and 4 (control) were done. The results showed that yield was significantly influenced by plant spacing in both experiments. The interaction effect of 0.2 m × 1.3 m plant spacing and topping at truss 2 showed significantly higher yields. Furthermore, juice volume was significantly increased by plant spacing. Again, 0.2 m × 1.3 m plant spacing by truss 2 topping interaction produced the highest juice volume. Therefore, these agronomic practices could be an essential and effective approach in achieving higher tomato production with improved fruit quality under greenhouse cultivation to ensure sustainable food security.
Introduction
Tomato (Solanum lycopersicum L.), belonging to the Solanaceae family, is among the most widely cultivated vegetables in the world. Its fruits are consumed fresh or cooked and processed into paste, juice, puree, and sauce. Due to its increasing dietary importance, the production of tomatoes worldwide has increased significantly. World tomato production is around 134 million tons, with Africa contributing about 11.9% [Food Agriculture Organization of the United Nations-Statistic Division (Food Agriculture Organization of the United Nations-Statistic Division (FAOSTAT)., 2019)]. In Ghana, tomato is one of the most important vegetable crops and essential ingredients for the daily food preparation in both urban and rural areas, which has contributed significantly to improving livelihoods. About 380,000 tons of tomatoes are grown annually [Food Agriculture Organization of the United Nations-Statistic Division (Food Agriculture Organization of the United Nations-Statistic Division (FAOSTAT)., 2019)], which is approximately USD 140 million in Ghana, of which 90% is consumed domestically (Osei et al., 2018). The demand for fresh tomato and its products in Ghana exceeds local production, leading to massive imports from other countries such as Burkina Faso, China, Italy, USA, and Spain (Awo, 2012). Ghana consumes more than 78,000 tons of tomato paste annually through imports, at a cost of about USD 100 million (Osei et al., 2018). The major contributors to the reduction in its production are attributed to the high prevalence of biotic (pest and diseases) and abiotic stresses (such as drought, heat stress) as well as increased postharvest losses and poor agronomic practices, posing a serious threat to food security. Also, due to population growth, lifestyle changes, and consumption, tomato production in Ghana is expected to increase in order to meet the rapid increase in its demand. Hence, the recent introduction of greenhouse vegetable cultivation technology in Ghana is a sustainable approach in addressing and ensuring year-round production of vegetables such as tomato, pepper, and cucumber. Likewise, this technology leads to an increase in productivity per unit area. The most commonly cultivated crop under greenhouse conditions in Ghana is tomato. To ensure sustainable tomato production, identifying adaptable and appropriate agronomic practices is highly recommended to improve yield and fruit quality, which could lead to increase in profit margin as well as meet consumer preferences (Maboko et al., 2017). Such agronomic practices include plant spacing, choice of cultivar (Maboko et al., 2017), and topping (Robinson and Kolavalli, 2010).
In Ghana, although both determinate and indeterminate tomato cultivars are grown under greenhouse conditions, the indeterminate type is commonly cultivated. Determinate cultivars have definite growth and as such are considered as self-topping. Thus, they grow, flower, and produce fruit at a specific stage. On the other hand, indeterminate cultivars grow indefinitely and are not self-topping, thereby producing fruits throughout the life of the plant (Rutledge et al., 1998; Panthee and Chen, 2010). Moreover, in self-topping or determinate cultivars, translocation of assimilates is targeted to the developing fruits at the reproductive stage, causing a decrease in harvest length compared to indeterminate types (Rutledge et al., 1998).
Topping is an agronomic practice that involves mechanical damage/wounding of the terminal bud to break the apical dominance of vegetable crops (Mohammed and Saeid, 2017). The technique of topping has been shown to offer advantages to yield and yield components increase in different crops (Tripathi et al., 2013) such as eggplant (Buczkowska, 2010), pepper (Adenle-Saheed et al., 2016), okra (Mohammed and Saeid, 2017), cotton (Aydin and Arslan, 2018), and jute (Das et al., 2014). The increase in yield and yield attributes promoted by the topping technique could be attributed to improved growth characteristics such as dry matter accumulation. For instance, in tobacco, at the maturity stage, topping caused a reduction in senescence-related metabolites and increased accumulation of secondary metabolites in mature leaves (Zhao et al., 2018). Hence, topping of indeterminate tomato cultivars could be a promising and effective agronomic technique in promoting fruit quality and yield through induced redistribution of sink-source assimilates (Kinet and Peet, 1997; Robinson and Kolavalli, 2010).
Furthermore, plant spacing is one of the agronomic techniques in achieving optimum yield. A recommended number of plants per unit area contributes to adequate utilization of the available planting space, thus ensuring an even distribution of water, nutrient, light, and air. However, an unregulated plant spacing may result in a relatively lower yield and poor fruit quality (Maboko et al., 2017). Tomato yield and yield components have been reported to be greatly affected by plant spacing (Balemi, 2008; Castoldi et al., 2010; Maboko et al., 2017). In Ghana, to the best of our knowledge, there is no report on planting spacing as well as topping for determinate tomato cultivars under greenhouse tomato cultivation. Hence, this study seeks to evaluate the effect of plant spacing and topping on tomato yield and fruit quality under greenhouse conditions.
Materials and Methods
Experimental Site and Condition
In this study, two experiments were carried out with the second one being a repetition of the first using tomato variety, Anna F1. The experiments were conducted under a greenhouse condition of a size of 270 m2 at the research farm of the University of Ghana Forest and Horticultural Crops Research Center (FOHCREC), Okumaning-Kade, in the Eastern Region of Ghana from September 2019 to May 2020. The research area lies on latitude 6°0854′N and longitude 0°5400′W, with an elevation of 114 m above sea level (Ofosu-Budu, 2003). The climatic conditions in the greenhouse are shown in Table 1. The temperature and relative humidity in the greenhouse ranged between 24 and 32°C and 63 and 80%, respectively, during the experimental period.
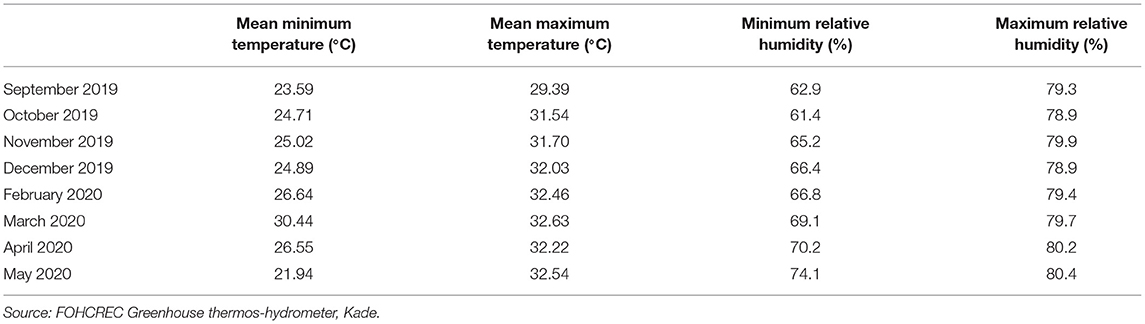
Table 1. Mean values of temperature and relative humidity in the greenhouse during the experimental period.
Planting Material and Establishment
Seeds of tomato variety Anna F1 were obtained from Macrofertil Ghana Limited (MGL, Tema, Ghana). Seedlings were raised in plastic trays filled with buffered cocopeat obtained from Hortirite Ventures (Tema, Ghana). Recommended nursery practices were observed to ensure that vigorous and uniform seedlings were obtained. Before transplanting, chlorpyrifos-ethyl at a rate of 30 ml/15 l was applied to sanitize both the interior and exterior of the greenhouse. Seedlings were transplanted 4 weeks after sowing. Seedlings with an average height of 18 cm with 4–6 matured leaves were selected and transplanted into black polythene bags of size 9 × 14 inches which were half-filled with buffered cocopeat. A 3 × 3 factorial treatment arranged in a completely randomized design (CRD) with three replications was used. Two factors, plant spacing and topping with each having three levels, were used. Thus, the levels for plant intra–interspacing were 0.15 m × 1.3 m, 0.2 m × 1.3 m, and 0.3 m × 1.3 m while topping treatments at trusses 2, 3, and 4 (control) were done. Topping of plants was carried out when three leaves above the preceding truss were fully developed to ensure that the required numbers of trusses (i.e., two, three, and four trusses) were kept in each treatment. The topping was done using sterilized secateurs.
Agronomic Practices
Greenhouse cultural practices such as trellising and pruning were done when necessary. At the early vegetative stage, through drip irrigation, a phosphate fertilizer at 10 g per plant was supplied to ensure proper root and shoot development. Three weeks after transplanting (WAT), compound fertilizers N:P:K (10:5:20) at 12 g per plant at the reproductive stage was applied. Twenty-five milliliters per 15 l of emamectin benzoate 1.9 EC insecticide was applied at 2 weeks after transplanting through to harvesting while 10 ml/16 l of tribasic copper sulfate 8.4% fungicide was also applied immediately after transplanting to prevent pest and pathogen infestation. A mist foliar application of B-naphthoxyacetic acid at 3 ml/l was applied twice a week between 4 and 9 weeks after transplanting to enhance fruit set. At maturity, harvesting was done by handpicking.
Data Collection and Analysis
For each replication, data were taken on five record plants in both experiments. Plant height was recorded at 2, 4, and 6 weeks after transplanting using a meter rule. A measure from the substrate level to the shoot tip was considered as the plant height. The stem girth was taken 5 cm above the substrate level using Vernier calipers. A leaf area meter (Model CI-202, Germany) was used to determine the leaf area by measuring the area of five randomly detached leaves collected from the middle part of the plant. Shoot and root samples were kept in an oven at 70°C for 72 h prior to measurements using a digital scale. The shoot-to-root ratio was computed using Microsoft Excel. Measurements of relative growth rate (RGR) and net assimilation rate (NAR) were carried out by destructive sampling at 2, 4, and 6 WAT.
RGR and NAR were calculated using the formulae below as reported by Sunaryanti et al. (2018).
Where ln, natural log; W1, dry weight of plant/m2 recorded at time T1; W2, dry weight of plant/m2 recorded at time T2; T1 and T2, time interval.
Where A1 and A2 are total leaf area at T1 and T2, respectively. W1 and W2 are the total dry weight of plant/m2 recorded at time T1 and T2, respectively.
The numbers of flowers and fruits per plant were counted and recorded. The number of days to 50% flowering was recorded as the mean number of days from transplanting to 50% anthesis. At harvesting, fruit weight was measured using a weighing scale. Yield was calculated by multiplying the fruit weight (kg) by the total plant population per acre. Total soluble solids (TSS)/Brix and pH were measured using a refractometer (BEXCO Brix Handheld product) and pH meter, respectively. The juice volume of ground tomato fruits was measured using a graduated cylinder.
Statistical Analysis
Data were analyzed using GenStat statistical software version 19.0, and the least significant difference (LSD) at 5% was used in separating means.
Results and Discussion
Results
Plant height was significantly affected by spacing at 6 WAT in experiment 2 (Table 2). Plants grown at spacing of 0.15m × 1.3m were also significantly taller than plants planted at spacings of 0.2 m × 1.3 m, and 0.3 m × 1.3 m. However, plant height was not significantly influenced by topping in 2, 4, and 6 WAT in both experiments. The interaction effects of spacing and topping (S × T) on plant height were not significant in both experiments (Table 2).
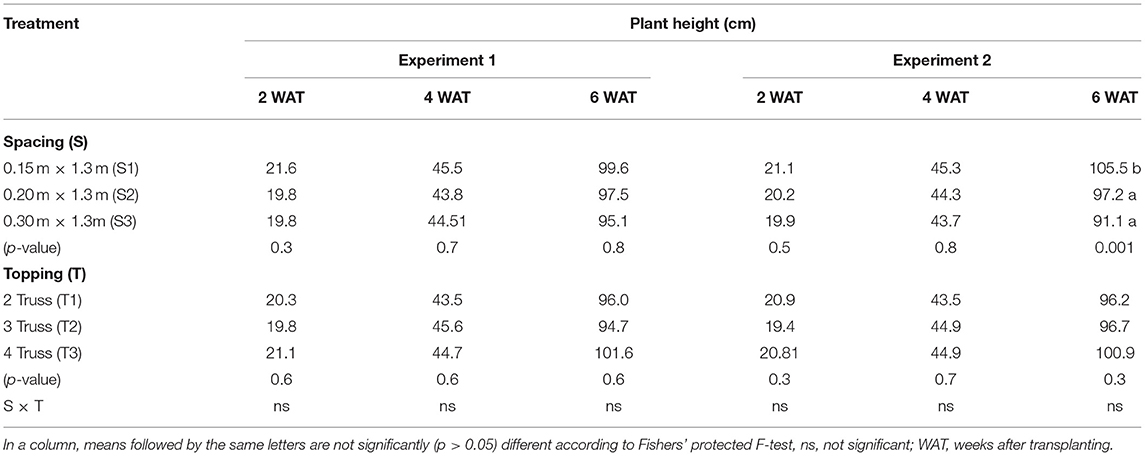
Table 2. Effect of spacing and topping on plant height of Solanum lycopersicum under greenhouse conditions.
Stem girth was significantly influenced by spacing at 2 WAT in experiment 2 (Table 3). Stems of plants planted at a spacing of 0.20m × 1.3m were significantly bigger (0.3mm) than stems collected from a plant spacing of 0.15m × 1.3m. In both experiments, however, stem girth was not significantly influenced by topping at 2, 4, and 6 WAT. Also, the interaction effects of spacing and topping on stem girth showed no significant influence in both experiments (Table 3).
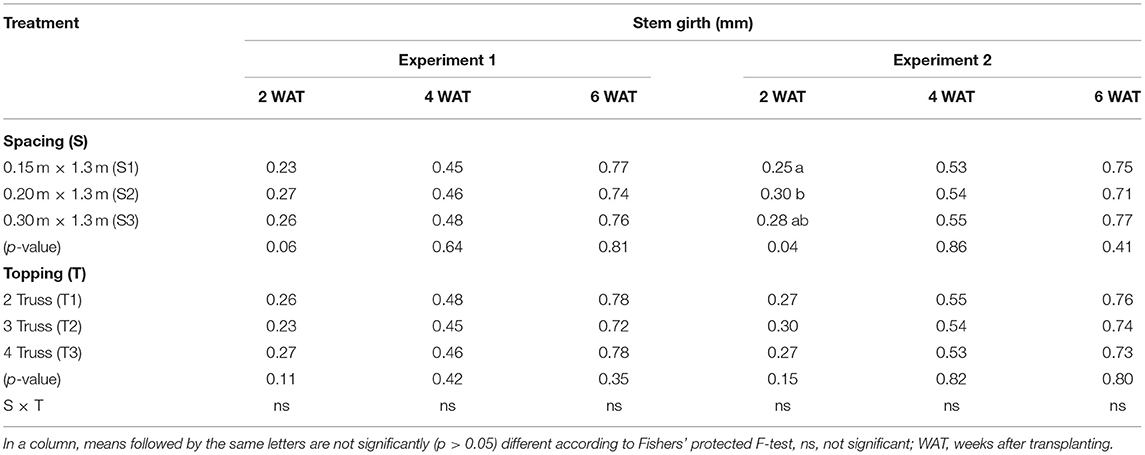
Table 3. Effect of spacing and topping on plant stem girth of Solanum lycopersicum under greenhouse conditions.
At 2, 4, and 6 WAT, shoot dry weight was significantly affected by spacing, topping, and its interaction in both experiments (Table 4). The interaction effects of 0.2 m × 1.3 m and 0.3 m × 1.3 m plant spacings and topping at the third truss showed a significantly higher shoot dry weight at 2 WAT in both experiments than the 0.3 m × 1.3 m plant spacing and topping at the second truss (Table 4). At 4 WAT, the interaction effects of the 0.3 m × 1.3 m plant spacing and topping at the third truss recorded a significantly higher shoot dry weight while the least was recorded at 0.15 m × 1.3 m plant spacing and topping at the second truss in experiment 1. However, at 6 WAT, the interaction effects of the 0.3 m × 1.3 m planting spacing and topping at the second and third trusses showed a significantly higher shoot dry weight in both experiments. On the other hand, the 0.15 m × 1.3 m plant spacing and topping at the second and fourth trusses recorded a smaller shoot dry weight in both experiments (Table 4). In experiment 2, the 0.20 m × 1.3 m plant spacing and topping at the fourth truss showed significantly higher shoot dry weight as compared with the 0.30 m × 1.3 m plant spacing and topping at the fourth truss (Table 4).
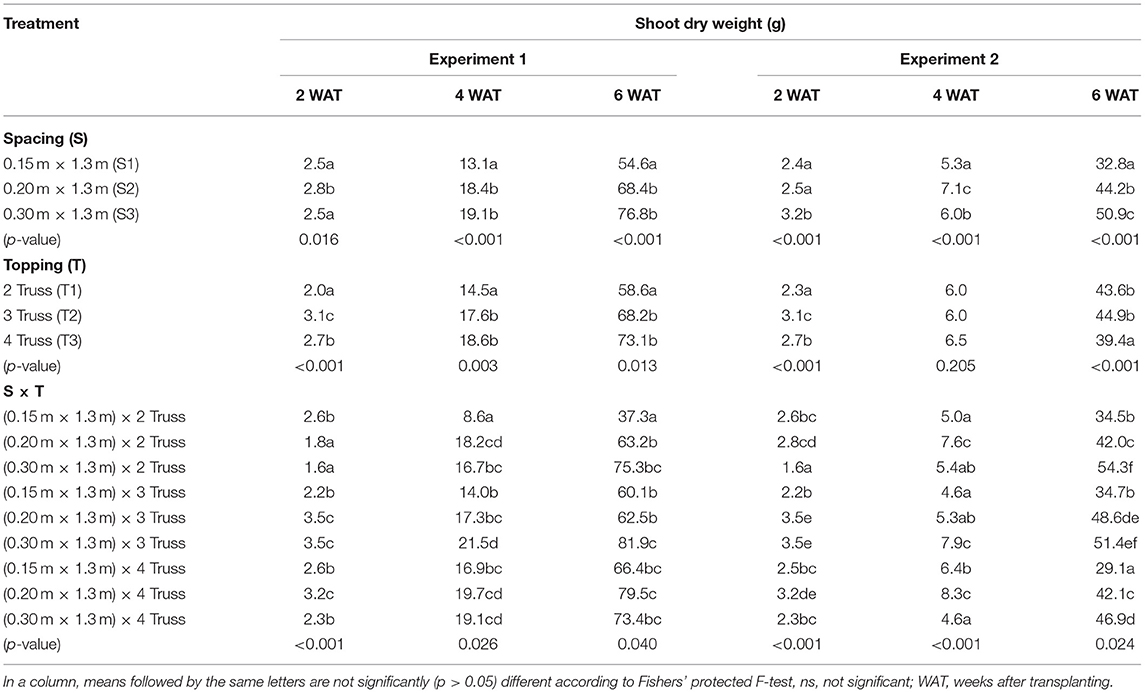
Table 4. Effect of spacing and topping on plant shoot dry weight of Solanum lycopersicum under greenhouse conditions.
Similarly, at 2, 4, and 6 WAT plant spacing, topping and its interaction caused significant differences in root dry weight in both experiments (Table 5). At 2 WAT, the interaction effects of 0.2 m × 1.3 m and 0.15 m × 1.3 m plant spacings and topping at the fourth truss recorded significantly higher root dry weight in experiments 1 and 2, respectively. In addition, 0.15 m × 1.3 m plant spacing and topping at the third truss recorded small shoot dry weight in experiment 1. Plant spacings of 0.30 m × 1.3 m and 0.15 m × 1.3 m with topping at the second and third trusses, respectively, also showed smaller shoot dry weight in experiment 2 (Table 5). At 4 WAT, the interaction effects of 0.2 m × 1.3 m and 0.15 m × 1.3 m plant spacings and topping at the second truss recorded significantly higher root dry weight in experiments 1 and 2, respectively. However, plant spacing of 0.15 m × 1.3 m and topping at the second and third trusses showed relatively smaller root dry weights in experiments 1 and 2, respectively (Table 5). At 6 WAT, interaction effects of 0.3 m × 1.3 m plant spacing and topping at the second truss showed significantly higher root dry weight in both experiments while smaller root dry weights were recorded for plant spacings of 0.15 m × 1.3 m and 0.2 m × 1.3 m and topping at the second truss in experiments 1 and 2, respectively (Table 5).
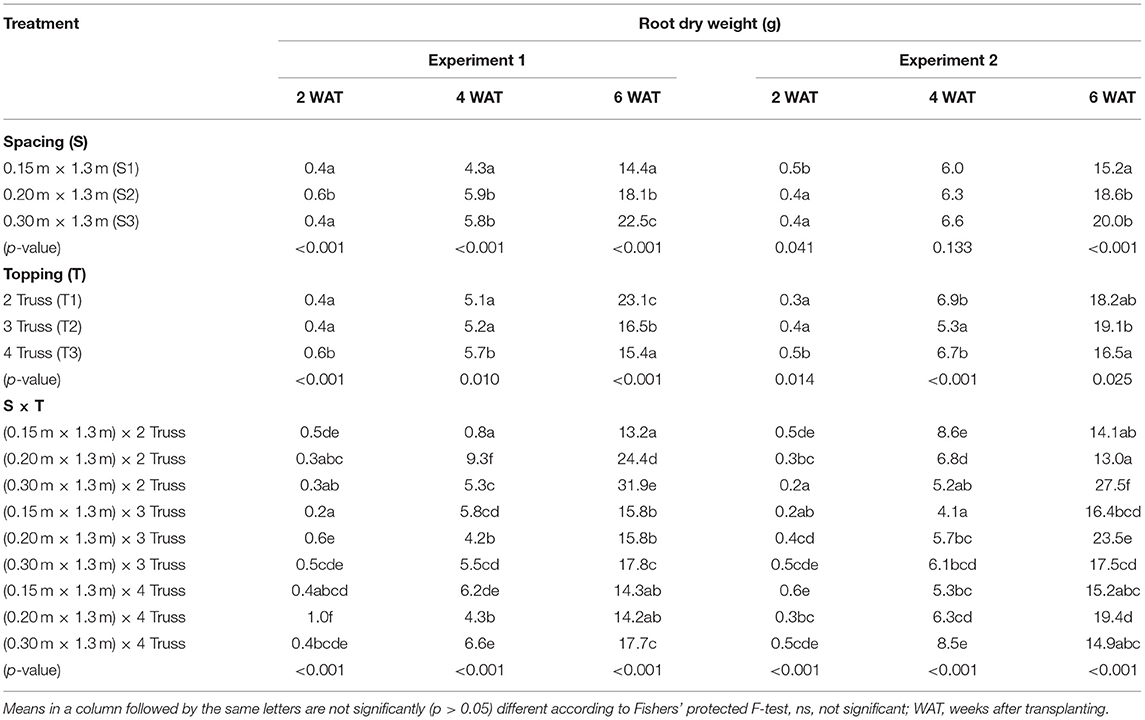
Table 5. Effect of spacing and topping on plant root dry weight of Solanum lycopersicum under greenhouse conditions.
The shoot-to-root ratio was significantly affected by topping in almost all the vegetative growth stages (Table 6) in both experiments. The interaction between plant spacing and topping significantly influenced the shoot-to-root ratio in both experiments except at 6 WAT in experiment 1. At 2 WAT, significantly higher shoot-to-root ratios were recorded for the interaction between plant spacing 0.15 m ×1.3 m with topping at truss 3 and 0.2 m ×1.3 m with topping at truss 4 in experiments 1 and 2, respectively (Table 6). At 4 WAT, the interaction effect of 0.15 m ×1.3m plant spacing with topping at truss 2 was significantly higher in experiment 1. At 6 WAT, the plant spacing of 0.2 m ×1.3 m with topping at the second truss showed a significantly higher shoot-to-root ratio while 0.15 m ×1.3 m plant spacing and topping at truss 4 showed a smaller shoot-to-root ratio in experiment 2 (Table 6).
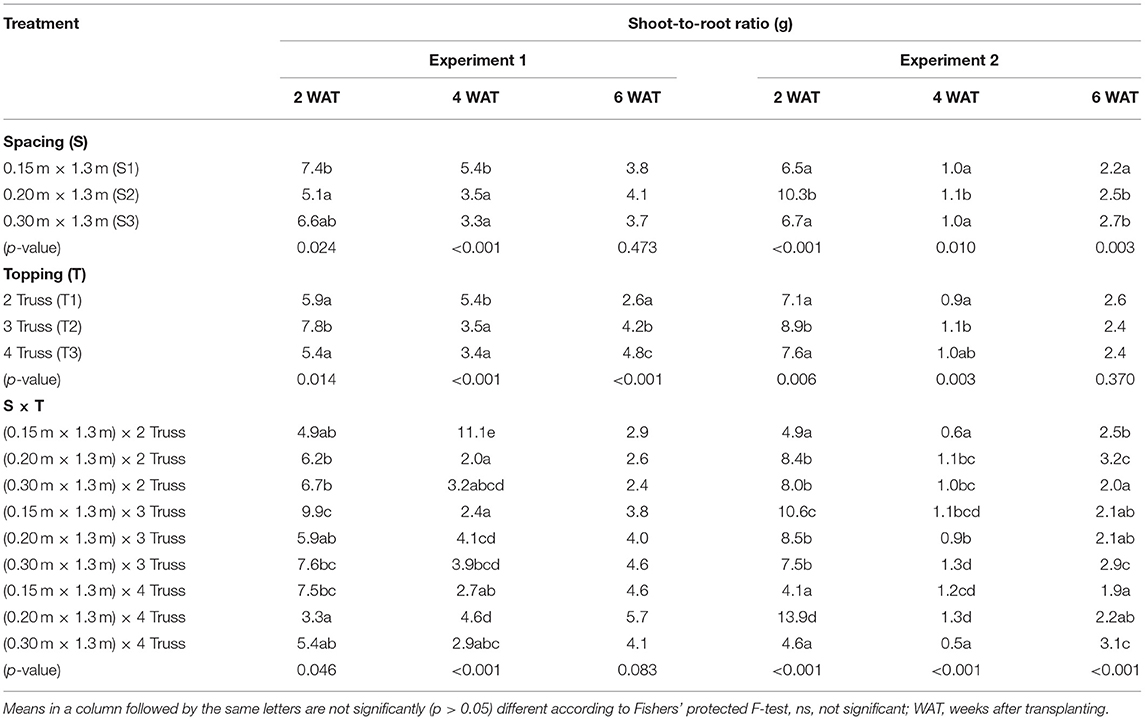
Table 6. Effect of spacing and topping on plant shoot-to-root ratio of Solanum lycopersicum under greenhouse conditions.
Plant spacing and topping significantly affected the leaf area in both experiments. At 2 WAT, the interaction effects of 0.2 m × 1.3 m plant spacing and topping at truss 3 as well as 0.3 m × 1.3 m spacing and topping at truss 2 showed significantly higher leaf area in experiments 1 and 2, respectively (Table 7). At 4 WAT, the interaction effect of plant spacing of 0.3 m × 1.3 m with topping at the third truss resulted in a significant increase in leaf area while 0.15 m × 1.3 m plant spacing with topping at truss 2 recorded a smaller leaf area in experiment 2 (Table 7). At 6 WAT, interaction effects of 0.15 m × 1.3 m plant spacing and toppings at trusses 4 and 3 recorded a significantly higher leaf area in experiments 1 and 2, respectively. However, the interaction effect of 0.3 m × 1.3 m plant spacing with topping at the third truss recorded the smallest leaf area in experiment 1 at 6 WAT (Table 7).
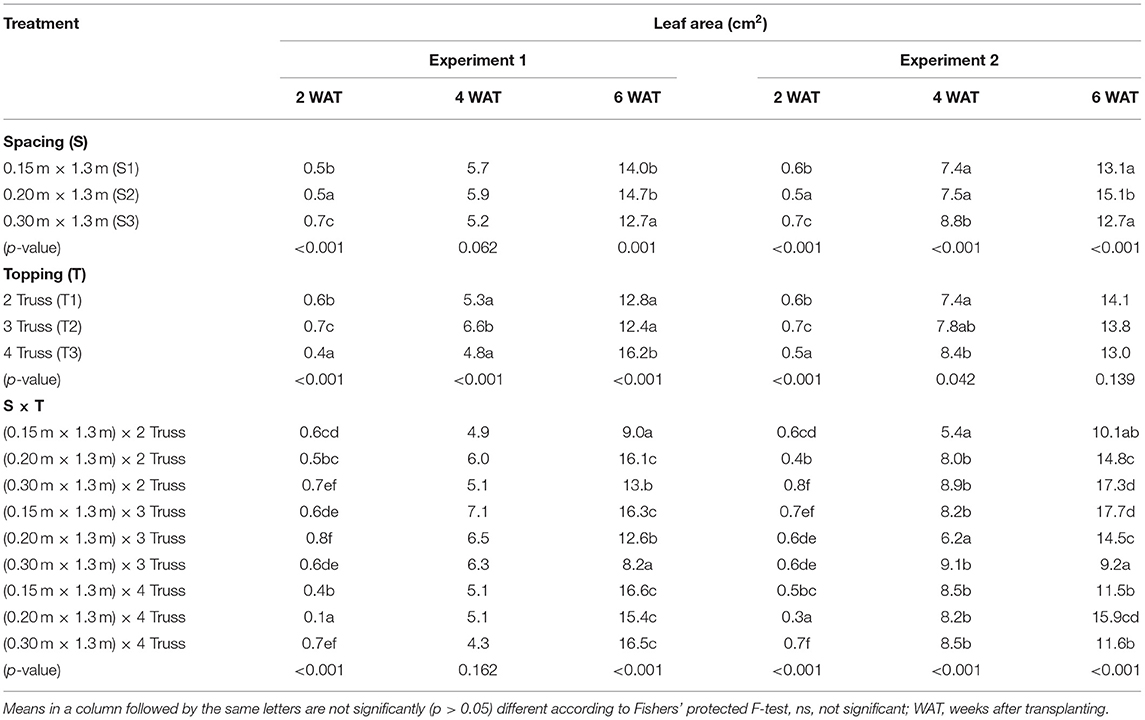
Table 7. Effect of spacing and topping on leaf area of Solanum lycopersicum under greenhouse conditions.
RGR was significantly influenced by spacing at 4 WAT in both experiments but 6 WAT in experiment 2 (Table 8). NAR was significantly influenced by both spacing and topping at 4 and 6 WAT in both experiments. The interaction effects of plant spacing and topping on RGR at 4 WAT and NAR at 4 and 6 WAT in experiment 1 showed significant influence. Also, the interaction effects of plant spacing and topping (S × T) on RGR and NAR at 4 and 6 WAT showed a significant influence in experiment 2 (Table 8). The interaction effects of 0.2 m × 1.3 m plant spacing and topping at the fourth truss showed significantly higher RGR in experiments 1 and 2 at 4 WAT as compared with the 0.15 m × 1.3 m plant spacing and toppings at the second and fourth trusses with smaller RGR in both experiments at 4 WAT (Table 8). At 4 WAT, the interaction effects of 0.3 m × 1.3 m spacing and topping at the third truss showed significantly higher NAR in both experiments. However, the interaction effects of 0.15 m × 1.3 m spacing and topping at the third truss recorded smaller NAR both experiments at 4 WAT.
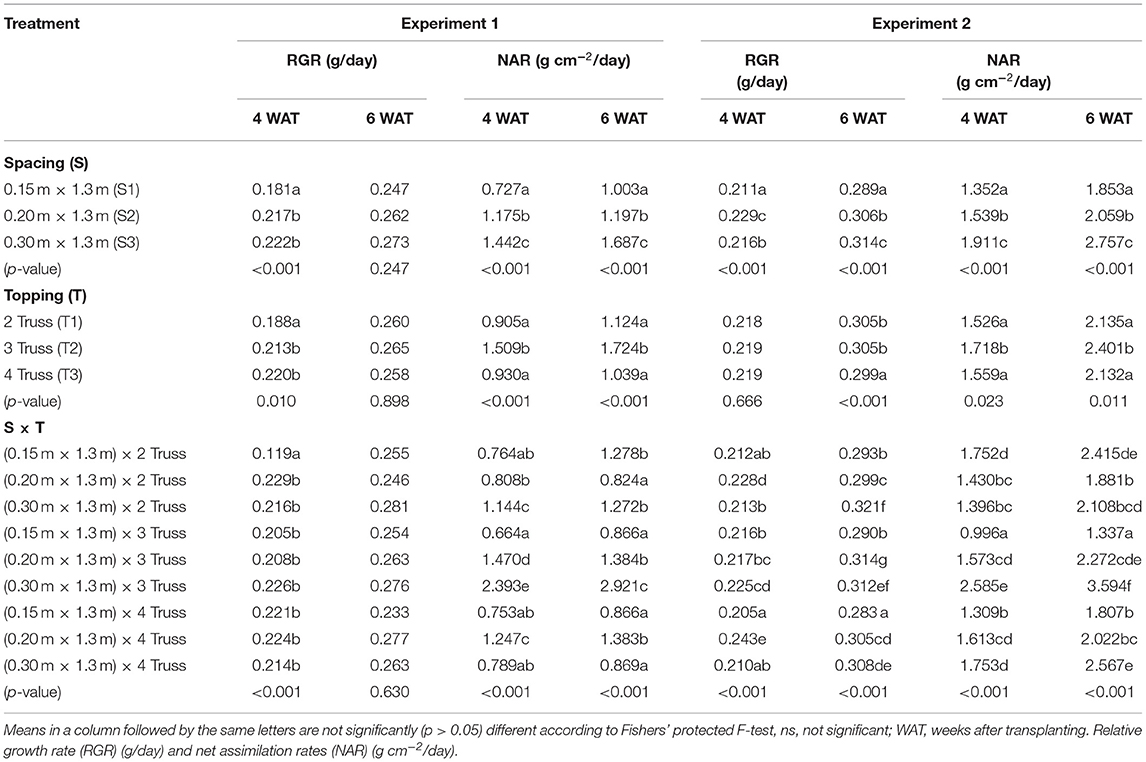
Table 8. Effect of spacing and topping on relative growth rate (RGR) and net assimilation rates (NAR) of Solanum lycopersicum under greenhouse conditions.
At 6 WAT, the interaction effect of 0.3 m × 1.3 m plant spacing and topping at the second truss showed significantly higher RGR as compared with 0.15 m × 1.3 m plant spacing and topping at the fourth truss with smaller RGR in experiment 2 (Table 8). However, the interaction effects of 0.3 m × 1.3 m plant spacing and topping at the third truss showed significantly higher NAR in both experiments. The interaction effects of 0.2 m × 1.3 m plant spacing and topping at the second truss showed significantly smaller NAR in experiment 1, and the interaction effects of 0.15 m × 1.3 m plant spacings and topping at the third truss also recorded smaller NAR in experiment 2 at 6 WAT (Table 8).
Days to 50% flowering and yield was significantly influenced by plant spacing in both experiments. However, fruits per plant and total fruit weight were significantly influenced by plant spacing in experiment 2 (Table 9). Again, number of flowers and days to 50% flowering were significantly affected by topping in both experiments; however, fruits per plant and weight per fruit were significantly affected by topping (Table 9). Days to 50% flowering, total fruit weight, and yield were significantly affected by the interaction between plant spacing and topping in experiment 1; however, weight per fruit, total fruit weight, and yield were significantly affected by plant spacing and topping interactions in experiment 2 (Table 9). The interaction effects of 0.2 m × 1.3 m and 0.3 m × 1.3 m plant spacings and topping at the second truss showed significantly more days to 50% flowering as compared with the 0.15 m × 1.3 m plant spacing and topping at the second truss with earlier days to 50% flowering in experiment 1 (Table 9). However, in experiment 2, the interaction effect of 0.2 m × 1.3 m plant spacing and topping at the second truss showed significantly higher weight per fruit as compared with the 0.2 m × 1.3 m plant spacing and topping at the fourth truss with smaller weight per fruit (Table 9). Also, the 0.2 m × 1.3 m plant spacing and topping at the second truss recorded significantly higher total fruit weight as compared with 0.15 m × 1.3 m plant spacing and topping at the second truss which recorded the smallest total fruit weight in experiments 1 and 2 (Table 9). The interaction effects of 0.2 m × 1.3 m plant spacing and topping at the second truss recorded significantly higher yields in both experiments. However, 0.3 m × 1.3 m plant spacing and topping at the fourth truss recorded a relatively lower yield in experiment 1. Likewise, the interaction effects of 0.3 m × 1.3 m plant spacings and topping at the third truss also recorded a lower yield in experiment 2 (Table 9).
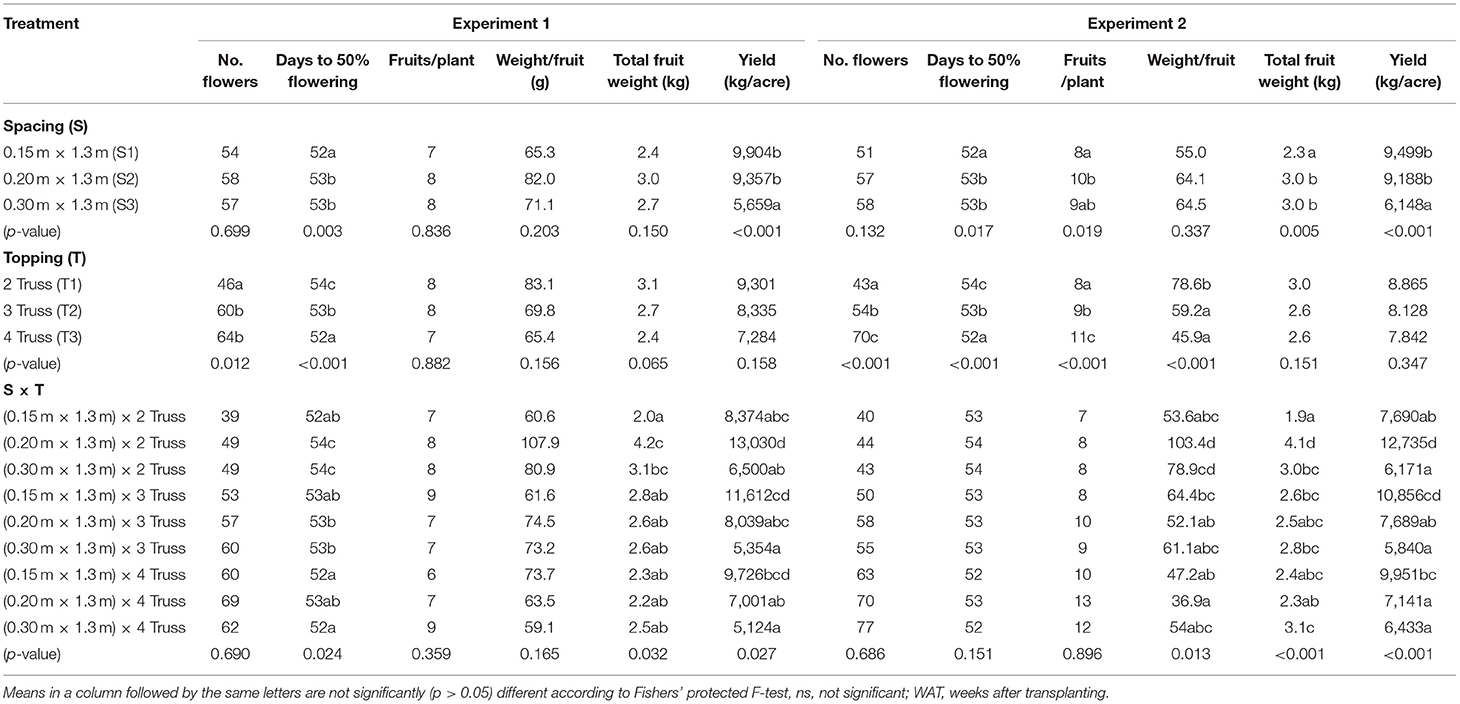
Table 9. Effect of spacing and topping on yield and yield components of Solanum lycopersicum under greenhouse conditions.
Furthermore, juice volume was significantly influenced by spacing in experiment 2 (Table 10). The juice volume of fruits from plants planted at a spacing of 0.20 m × 1.3 m was significantly higher than that of plants planted at 0.15 m × 1.3 m spacing. Topping did not significantly influence Brix, pH, and juice volume in both experiments (Table 10). However, in experiment 2, the interaction effects of spacing and topping significantly influence pH and juice volume (Table 10). Thus, interaction effects of 0.15 m × 1.3 m and 0.2 m × 1.3 m spacings and topping at the second truss showed significantly higher pH and juice volume as compared with the 0.2 m × 1.3 m and 0.15 m × 1.3 m spacings and topping at the second truss with lower pH and juice volume in experiment 2 (Table 10).
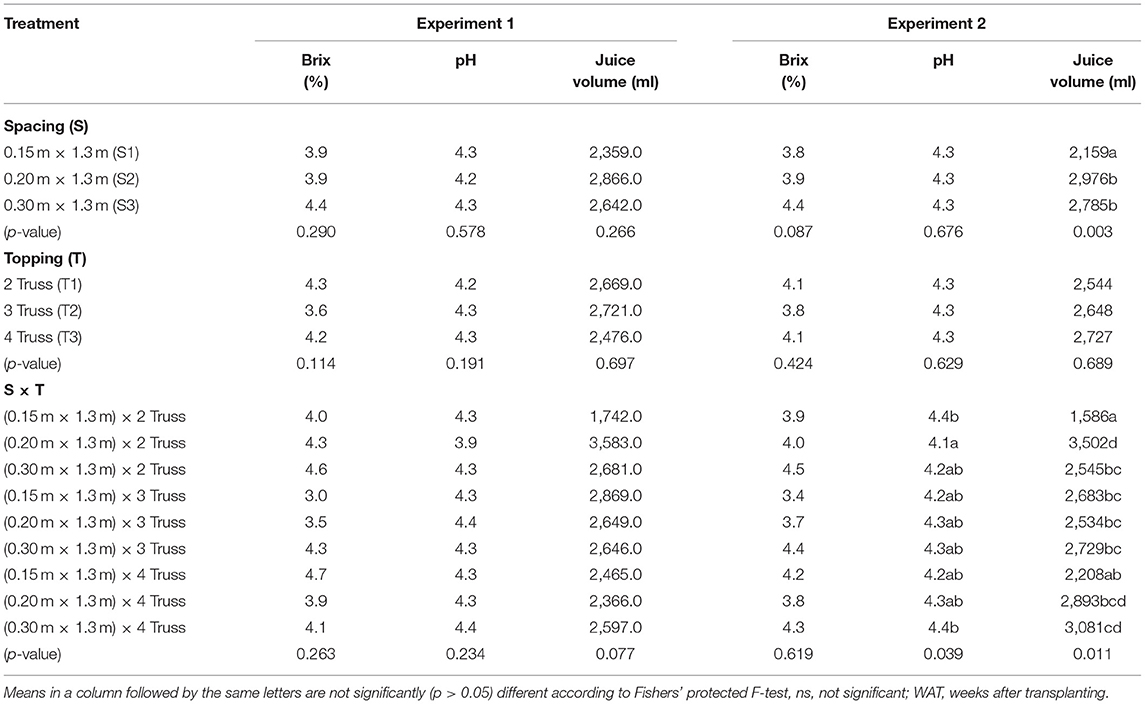
Table 10. Effect of spacing and topping on fruit quality of Solanum lycopersicum under greenhouse conditions.
Discussion
Improvement in the vegetative characters interacting with the genetic and optimum environmental conditions ensures the achievement of maximum yield of crop plants such as tomato. This study recorded significant differences among plant spacing and topping effects on the vegetative characters of tomato which include plant height, stem girth, and leaf area under greenhouse conditions. Plant height is an important vegetative character that enables the plant leaves to gain adequate access to full light when stems of plants are supported and maintained (Xu et al., 2020). Results from the study revealed that plant height increased with decreasing plant spacing. This could be as a result of increased plant competition for soil nutrients and sunlight between plants within the row and reduced competition between rows. The current result was in accord with the results of Ogundare et al. (2015) in tomatoes as well as in Crotalaria juncea L. (Tripathi et al., 2013). On the contrary, other researchers reported higher plant height in wider plant spacing than closer spacing (Ara et al., 2007 and Chernet et al., 2017). The highest truss number induced an increase in plant height which corroborates with studies by Ayarna et al. (2019) probably due to the increase in the length of the internode.
Stem girth increased with increasing plant spacing where the thickest stems recorded in plants spaced 0.2 m × 1.3 m could be due to greater biomass allocation to the stem which is in accordance with Olaniyi et al. (2010), Ogundare et al. (2015), and Ayarna et al. (2019). Plants grown under narrow spacing seemed to be crowded which resulted in some degree of etiolation. This could be as a result of such plants utilizing the photosynthates produced in increasing their height to compete for maximum light at the expense of stem growth (Maurya et al., 2013).
The highest plant shoot dry weight was recorded in the wider plant spacing which is in line with a report by Naik et al. (2018). The presence of sufficient space, concomitant with reduced interplant competition, as well as the availability of sufficient moisture, nutrients, and light enhanced the growth and development of the plants. Thus, increased light interception by widely spaced plants with increased carbon assimilates for photosynthesis and efficient use of resources resulted in increased dry matter accumulation. Ibeawuchi et al. (2008) also reported similar results. On the contrary, a study by Makinde and Alabi (2002) reported higher shoot dry weight in closely spaced plants. The high dry matter accumulation at the third and fourth trusses could be a result of increased carbon and nitrogen metabolism, photosynthesis, and secondary metabolism (Zhao et al., 2018). Wider plant spacing promoted root dry weight accumulation. This finding is in agreement with reports by Jimba and Adedeji (2003), Moosavi et al. (2012), and Legwaila et al. (2014). On the other hand, narrower spacing contributed to an increase in root dry matter accumulation which was reported in sorghum (Snider et al., 2012) and soybean (De Bruin and Pedersen, 2008). The higher shoot-to-root ratio observed in narrow spacing may be attributed to plants channeling more nutrients and resources into the shoot development, thereby enhancing the manufacturing of photosynthates (Ayarna et al., 2019).
Wider spacing contributed to an increase in RGR and NAR, which may be as a result of less competition for nutrients and light. This finding is in contrast with a report by Law-Ogbomo and Egharevba (2008). Topping influenced the number of flowers in truss 4 which may be attributed to translocation of assimilates for flower development (Pék and Helyes, 2004) and may result in an increase in fruit number. In addition, moderate plant spacing of 0.20 m × 1.3 m influenced increased fruit number per plant as a similar pattern was observed by Jovicich et al. (2004) and Adenle-Saheed et al. (2016). In contrast, Ara et al. (2007) reported that narrow spacing gave the maximum marketable number of fruits per plant. This could be caused by differences in crop varieties as early- to late-maturing crop cultivars do well in different plant spacing.
Moreover, topping at truss 2 also produced the maximum number of fruits per plant. This might be due to an increase in the accumulation of photosynthates for fruit development which is in line with a study by Ayarna et al. (2019). Wider plant spacing had a positive effect on fruit weight due to less competition for water, nutrients, and light as well as maximum photosynthetic area, thereby enhancing high assimilation and accumulation of photosynthates in tomato fruits as observed in study by Ayarna et al. (2019). This finding also corroborates with reports by Kultur et al. (2001) in muskmelon, Ibeawuchi et al. (2008) in maize, Naik et al. (2018) in clusterbean, and Aminifard et al. (2012) in sweet pepper. The higher yields observed in the narrow (0.15 × 1.3 m) and moderate (0.2 m × 1.3 m) spacing confirms maximum/efficient utilization of space under greenhouse cultivation of vegetables, which ensures an even supply and distribution of water and nutrients for efficient plant growth and development. Thus, the higher number of plants per unit area resulted in higher fruit number, thereby recompensing for the slight reduction of fruit weight as seen in wider spacing (Law-Ogbomo and Egharevba, 2008). Additionally, topping at truss 2 boosted yield, which could be attributable to the allocation of photosynthates and metabolites produced by leaves to strong carbohydrate sinks, such as fruit.
Moreover, spacing at 0.20 m × 1.3 m showed a positive effect on juice volume. This might be due to adequate reception of solar radiation, resulting in high leaf assimilates concomitant with increase in metabolite accumulation in the fruits. Furthermore, the less competition for light, space, and nutrient contributed to larger-sized fruits potentially enhancing more juice accumulation (Kirimi et al., 2011; Assefa et al., 2015).
Conclusion
Plant spacing and topping are useful agronomic practices that can be adopted to improve tomato yield and fruit quality under greenhouse conditions. In both experiments, the plant spacing 0.2 m × 1.3 m with topping at truss 2 produced higher yields as well as fruit juice volume. These agronomic practices could be an essential and effective approach in achieving multiple cultivations accompanied with higher tomato productivity and improved fruit quality under greenhouse cultivation to ensure sustainable food security.
Data Availability Statement
The raw data supporting the conclusions of this article will be made available by the authors, without undue reservation.
Author Contributions
GN contributed to the funding acquisition, conceptualization of project, supervision, and manuscript preparation. CA contributed to the experimental design and supervision. MZ conducted the experiment and data collection. SO-N contributed to the writing, reviewing, and editing of the manuscript. PO contributed to the writing and reviewing of the original draft of the manuscript. FO-A contributed in data analyses and writing of the manuscript. All authors contributed to the article and approved the submitted version.
Conflict of Interest
The authors declare that the research was conducted in the absence of any commercial or financial relationships that could be construed as a potential conflict of interest.
Publisher's Note
All claims expressed in this article are solely those of the authors and do not necessarily represent those of their affiliated organizations, or those of the publisher, the editors and the reviewers. Any product that may be evaluated in this article, or claim that may be made by its manufacturer, is not guaranteed or endorsed by the publisher.
References
Adenle-Saheed, V. O., Oso, Y. A. A., Adebanwo, I. T., and Adetayo, O. O. (2016). Effect of Apex Removal and Different Spacing on Growth and Yield of Capsicum Frutescence. Lagos State Polytechnic School of Agriculture, 65.
Aminifard, M. H., Aroiee, H., Ameri, A., and Fatemi, H. (2012). Effect of plant density and nitrogen fertilizer on growth, yield and fruit quality of sweet pepper (Capsicum annum L.). Afr. J. Agric. Res. 7, 859–866.
Ara, N., Bashar, M. K., Begum, S., and Kakon, S. S. (2007). Effect of spacing and stem pruning on the growth and yield of tomato. Int. J. Sustain. Crop Prod. 2, 35–39.
Assefa, W., Buzuayehu, T., and Dessalegn, L. (2015). Influence of inter and intra-rows spacing on yield and yield components of tomato cultivars. Ethiopian J. Agric. Sci. 25, 71–81.
Awo, M. A. (2012). Marketing and Market Queens: A Study of Tomato Farmers in the Upper East Region of Ghana (Vol. 21). Münster: LIT Verlag.
Ayarna, A. W., Tsukagoshi, S., Nkansah, G. O., and Maeda, K. (2019). Effect of spacing and topping on the performance of hydroponically grown tomato under tropical conditions. J. Agric. Crops 5, 23–30. doi: 10.32861/jac.52.23.30
Aydin, I., and Arslan, S. J. I. C. (2018). Mechanical properties of cotton shoots for topping. Ind. Crops Prod. 112, 396–401. doi: 10.1016/j.indcrop.2017.12.036
Balemi, T. (2008). Response of tomato cultivars differing in growth habit to nitrogen and phosphorus fertilizers and spacing on vertisol in Ethiopia. Acta Agric. Slovenica 91, 103–119. doi: 10.2478/v10014-008-0011-8
Buczkowska, H. (2010). Effect of plant pruning and topping on yielding of eggplant in unheated foil tunnel. Acta Sci. Pol. Hortorum Cultus 9, 105–115.
Castoldi, R., Faveri, L. A., Souza, J. O., Braz, L. T., and Charlo, H. C. O. (2010). Productivity characteristics of endive as a function of spacing,” in XXVIII International Horticultural Congress on Science and Horticulture for People (IHC2010): International Symposium, Vol. 936 (Lisbon), 305–309.
Chernet, S., Belay, F., Tekle, G., Kahsay, Y., Weldu, N., and Zerabruk, G. (2017). Response of yield and yield components of tomato (Solanum lycopersicon L.) to different inter and intra-row spacing at Merebleke, Northern Ethiopia. Afr. J. Agric. Res. 12, 2614–2619. doi: 10.5897/AJAR2016.11116
Das, H., Poddar, P., Haque, S., Pati, S., Poddar, R., and Kundu, C. K. (2014). Seed yield and economics of white jute as influenced by different dates of sowing, spacing and topping schedule in Terai region of West Bengal. Int. J. Farm Sci. 4, 51–58.
De Bruin, J. L., and Pedersen, P. (2008). Effect of row spacing and seeding rate on soybean yield. Agron. J. 100, 704–710. doi: 10.2134/agronj2007.0106
Food Agriculture Organization of the United Nations-Statistic Division (FAOSTAT). (2019). Available online at: https://www.fao.org/faostat/en/#data (accessed January 31, 2020).
Ibeawuchi, I. I., Matthews-Njoku, E., Ofor, M. O., Anyanwu, C. P., and Onyia, V. N. (2008). Plant spacing, dry matter accumulation and yield of local and improved maize cultivars. J. Am. Sci. 4, 545–1003.
Jimba, S. C., and Adedeji, A. A. (2003). Effect of plant spacing in the nursery on the production of planting materials for field establishment of Vetiver grass. Tropicultura 21, 199–203.
Jovicich, E., Cantliffe, D. J., and Stoffella, P. J. (2004). Fruit yield and quality of greenhouse-grown bell pepper as influenced by density, container, and trellis system. HortTechnology 14, 507–513.
Kirimi, J. K., Itulya, F. M., and Mwaja, V. N. (2011). Effects of nitrogen and spacing on fruit yield of tomato. Afr. J. Horticult. Sci. 5, 50–60.
Kultur, F., Harrison, H. C., and Staub, J. E. (2001). Spacing and genotype affect fruit sugar concentration, yield, and fruit size of muskmelon. HortScience 36, 274–278.
Law-Ogbomo, K. E., and Egharevba, R. K. A. (2008). Effects of planting density and NPK fertilizer on growth and fruit yield of tomato (Lycospersicon esculentus Mill). Res. J. Agric. Biol. Sci. 4, 265–272.
Legwaila, G. M., Mathowa, T., Makopola, P., Mpofu, C., and Mojeremane, W. (2014). The growth and development of two pearl millet landraces as affected by intra-row spacing. Int. J. Curr. Microbiol. Appl. Sci. 3, 505–515.
Maboko, M. M., Du Plooy, C. P., and Chiloane, S. (2017). Yield of determinate tomato cultivars grown in a closed hydroponic system as affected by plant spacing. Horticult. Brasileira 35, 258–264. doi: 10.1590/s0102-053620170217
Makinde, E. A., and Alabi, B. S. (2002). Influence of spatial arrangement on the growth and yield of maize-melon intercrop. Moor J. Agric. Res. 3, 59–63.
Maurya, R. P., Bailey, J. A., and Chandler, J. S. A. (2013). Impact of plant spacing and picking interval on the growth, fruit quality and yield of okra (Abelmoschus esculentus (L.) Moench). Am. J. Agric. For. 1, 48–54. doi: 10.11648/j.ajaf.20130104.11
Mohammed, G. H., and Saeid, A. I. (2017). Effect of topping, humic acid, mulching color and their interactions on vegetative growth and seed yield of Okra (Abelmoschus esculentus L.). J. Univ. Duhok 20, 41–49. doi: 10.26682/avuod.2017.20.1.7
Moosavi, S. G., Seghatoleslami, M. J., and Moazeni, A. (2012). Effect of planting date and plant density on morphological traits, LAI and forage corn (Sc. 370) yield in second cultivation. Int. Res. J. Appl. Basic Sci. 3, 57–63.
Naik, M. T., Srihari, D., Dorajeerao, A. V. D., Sasikala, K., Umakrishna, K., and Suneetha, D. R. S. (2018). Yield and biochemical quality in relation to spacing and nutrition interactions in seed guar varieties. Plant Arch. 18, 199–206.
Naik, M. T., Srihari, D., Dorajeerao, A. V. D., Sasikala, K., Umakrishna, K., and Suneetha, D. R. S. (2018). Yield and biochemical quality in relation to spacing and nutrition interactions in seed guar varieties. Plant Arch. 18, 199–206.
Ofosu-Budu, K. G. (2003). Performance of citrus rootstocks in the forest zone of Ghana. Ghana J. Hortic. 3, 1–9.
Ogundare, S. K., Oloniruha, J. A., Ayodele, F. G., and Bello, I. A. (2015). Effect of different spacing and urea application rates on fruit nutrient composition, growth and yield of tomato in derived Savannah vegetation of Kogi State, Nigeria. Am. J. Plant Sci. 6:2227. doi: 10.4236/ajps.2015.614225
Olaniyi, J. O., Akanbi, W. B., Adejumo, T. A., and Ak, O. G. (2010). Growth, fruit yield and nutritional quality of tomato varieties. Afr. J. Food Sci. 4, 398–402.
Osei, R. D., Okyere, C., Kubi, J. W. A., Onumah, J., Ofori-Appiah, Y. A. W., Asem, F., et al (2018). Cost-Benefit Analysis of Prioritizing: Agriculture Growth in Ghana. Available online at: https://www.copenhagenconsensus.com/sites/default/files/gp_a4_agriculture.pdf (accessed July 31, 2020).
Panthee, D. R., and Chen, F. (2010). Genomics of fungal disease resistance in tomato. Curr. Genomics 11, 30–39. doi: 10.2174/138920210790217927
Pék, Z., and Helyes, L. (2004). The effect of daily temperature on truss flowering rate of tomato. J. Sci. Food Agric. 84, 1671–1674.
Robinson, E. J., and Kolavalli, S. L. (2010). The Case of Tomato in Ghana: Productivity (No. 19). International Food Policy Research Institute (IFPRI).
Rutledge, A. D., Wills, J. B., and Bost, S. (1998). Commercial Tomato Production. University of Tennessee Agricultural Extension Service Publication. Available online at: https://extension.tennessee.edu/publications/Documents/pb737.pdf (accessed December 19, 2020).
Snider, J. L., Raper, R. L., and Schwab, E. B. (2012). The effect of row spacing and seeding rate on biomass production and plant stand characteristics of nonirrigated photoperiod-sensitive sorghum (Sorghum bicolor (L.) Moench). Ind. Crops Prod. 37, 527–535. doi: 10.1016/j.indcrop.2011.07.032
Sunaryanti, D. P., Chozin, M. A., and Aziz, S. A. (2018). Growth analysis and physiological characteristics of several tomato genotypes under the low light intensity. J. Int. Soc. Southeast Asian Agric. Sci. 24, 129–140.
Tripathi, M. K., Chaudhary, B., Singh, S. R., and Bh, H. R. (2013). Growth and yield of sunnhemp (Crotalaria juncea L.) as influenced by spacing and topping practices. Afr. J. Agric. Res. 8, 3744–3749. doi: 10.5897/AJAR12.6919
Xu, F., Chu, C., and Xu, Z. (2020). Effects of different fertilizer formulas on the growth of loquat rootstocks and stem lignification. Sci. Rep. 10, 1–11.
Keywords: tomato, topping, plant spacing, fruit quality, yield, greenhouse
Citation: Nkansah GO, Amoatey C, Zogli MK, Owusu-Nketia S, Ofori PA and Opoku-Agyemang F (2021) Influence of Topping and Spacing on Growth, Yield, and Fruit Quality of Tomato (Solanum lycopersicum L.) Under Greenhouse Condition. Front. Sustain. Food Syst. 5:659047. doi: 10.3389/fsufs.2021.659047
Received: 26 January 2021; Accepted: 03 November 2021;
Published: 10 December 2021.
Edited by:
Patrick Meyfroidt, Catholic University of Louvain, BelgiumReviewed by:
Ajit Singh, University of Nottingham Malaysia Campus, MalaysiaJuan Pablo Martinez, Instituto de Investigaciones Agropecuarias, Chile
Copyright © 2021 Nkansah, Amoatey, Zogli, Owusu-Nketia, Ofori and Opoku-Agyemang. This is an open-access article distributed under the terms of the Creative Commons Attribution License (CC BY). The use, distribution or reproduction in other forums is permitted, provided the original author(s) and the copyright owner(s) are credited and that the original publication in this journal is cited, in accordance with accepted academic practice. No use, distribution or reproduction is permitted which does not comply with these terms.
*Correspondence: George Oduro Nkansah, gonkansah@ug.edu.gh; gonkansah2013@gmail.com; Christiana Amoatey, camoatey@ug.edu.gh