- 1The Charles E. Via, Jr. Department of Civil and Environmental Engineering, Virginia Tech, Blacksburg, VA, United States
- 2National Socio-Environmental Synthesis Center (SESYNC), Annapolis, MD, United States
- 3Department of Civil Engineering, Kansas State University, Manhattan, KS, United States
- 4RTI International, Durham, NC, United States
Water scarcity is a pervasive threat to society that is expected to intensify alongside a growing and more affluent population and a changing climate. In this paper, we review the existing literature to assess the potential of lessening water scarcity by reducing food loss and waste. Existing studies reveal the scope of food loss and waste and its accompanying impact on water resources, thereby providing a foundation for policy action. We highlight existing or proposed food loss and waste reduction measures and review available evidence concerning their impact on water resources. Our review reveals that there is a deficit of research that can guide specific policy interventions aimed at mitigating water scarcity by reducing food loss and waste. Instead, the last decade of research has primarily focused on quantifying the current water footprint of food loss and waste for different locations, points within the supply chain, and food groups. Yet, the degree of uncertainty inherent in these estimates, their lack of precision, and several simplifying assumptions make it difficult to translate this research into robust policy measures to reduce the environmental burden of food loss and waste. We conclude by advancing a research agenda that will (i) quantify and reduce uncertainty through enhanced data collection and methods; (ii) holistically assess policy measures, including system level impacts and feedback; (iii) develop methods and technologies for transparent supply chain tracing. Together, advances in these areas will guide and ground food loss and waste policy toward reducing water scarcity.
Introduction
Over two-thirds of the global population lives under water scarcity (Mekonnen and Hoekstra, 2016). Limited water supplies inhibit economic growth, pose societal health risks, and endanger ecosystems. Measures to reduce water scarcity have primarily focused on developing new water supplies (World Commission on Dams, 2000) and improving water productivity (Marston et al., 2020), particularly in the agricultural sector, since it is responsible for over 90% of global consumptive freshwater use (Hoekstra and Mekonnen, 2012). Shifting to more efficient irrigation technologies (Jägermeyr et al., 2015), cultivating less water intensive crops (Marston and Konar, 2017), and growing crops best suited for a given hydroclimatic environment (Davis et al., 2017) can reduce the agricultural sector's water footprint and its contribution to water scarcity. However, the challenge of reducing water scarcity is ubiquitous and enduring, with no single remedy.
In an interconnected global economy, policy, and regulatory measures not directly aimed at reducing water scarcity can potentially go further in ameliorating water scarcity than traditional water conservation measures. System-level interventions—from farm to fork—are needed in the agricultural sector to address water scarcity. For instance, policies focused on shaping supply chain processes, including the public's consumption of water-intensive food goods, may offer similar levels of effectiveness in reducing system-wide water consumption as improvements in irrigation efficiency (Springmann et al., 2018). International food trade (Chapagain et al., 2006) and diets less reliant on water-intensive foods (Vanham et al., 2013, 2018) have been shown to reduce society's water footprint. One of the more promising means of reducing society's water footprint, however, is by reducing food loss and waste (Mekonnen and Fulton, 2018).
At least one-third of food is estimated to be lost or wasted globally (Gustavsson et al., 2011). Food loss and waste (FLW) occurs at all points along the food supply chain: food is lost on farms, at facilities, and during storage and distribution, and wasted within retail and households. Global food losses amount to 413 million metric tons at the agricultural production stage, 293 million metric tons in post-harvest handling and storage, 148 million metric tons in processing, 161 million metric tons in distribution, and 280 million metric tons wasted in consumption for a total of 1.3 billion metric tons of annual FLW (Gustavsson et al., 2011). In the United States alone, total annual FLW across all stages of the food supply chain is estimated to be 126 million tons, or about 10% of the world total (CEC, 2018). Across commodities, more FLW occurs for root crops (40–50%), fish (35%), and cereals (30%) compared to oilseeds, meat, and dairy products (20%) (Gustavsson et al., 2011).
The causes of FLW differ depending on the stage of the food supply chain. For example, at the farm production stage, the cost of harvesting a crop may be greater than the value of the crop due to changes in market conditions that result in lower commodity prices (Segrè et al., 2014). At the food processing stage, the costs of avoiding FLW may be greater than the revenue that could be potentially generated from alternative uses of ingredients or foods (Ellison et al., 2019). At the consumption stage, consumers may discard food past its use-by dates because they misunderstand that the dates represent quality rather than safety concerns (National Academies of Sciences Engineering Medicine, 2020). Furthermore, differences in food purchasing, storage, preparation, and serving practices between food-at-home and food-away-from-home (i.e., restaurants, cafeterias, and other types of foodservice operations) leads to different amounts of food wastage and prevention strategies (Aschemann-Witzel et al., 2015).
The causes of FLW also differ across low-, middle-, and high-income countries. Substantial post-harvest losses occur in low-income countries due to a lack of financial resources that limit the availability of harvesting and processing technologies and storage and transport infrastructure and because of climatic conditions that favor food spoilage (Gustavsson et al., 2011). In addition, producers in low-income countries often lack information and access to markets, leading to crops spoiling before they can be sold (Segrè et al., 2014). In higher-income countries, more FLW is estimated to occur at downstream stages (processing, distribution, and consumption) because of aesthetic preferences, lack of meal planning, overserving, and misinterpretation of date labels (Gustavsson et al., 2011; Xue et al., 2017). Differences in FLW at the consumption stage are particularly striking with estimates of 31–39% in middle- and high-income countries but only 4–16% percent in low-income countries (Gustavsson et al., 2011). Consumers in high-income countries can afford to waste food, because the cost of food is a much lower proportion of their budgets, while those in low-income countries cannot (Segrè et al., 2014). Regardless of supply chain stage, geographical location, or cause of FLW, the loss or waste of food amounts to exhausting or degrading natural resources with limited societal benefit.
In this paper, we provide a brief review of the literature that directly links FLW to water consumption and scarcity. Based on this foundation, we then discuss policies and other measures aimed at reducing FLW and the implications of these interventions on water consumption and scarcity. Because effective policy is rooted in sound science, we conclude by highlighting research gaps that need to be addressed to aid in policy development that meets the dual objectives of reducing FLW and water scarcity.
Wasted Food, Wasted Water
One-fourth of freshwater consumed in global food production is effectively wasted since the food produced with this water is never consumed (Kummu et al., 2012). The blue water footprint (i.e., consumed fresh surface water and groundwater) of global crop production is 723 km3/year, meaning uneaten plant-based food represents 174 km3 of wasted blue water each year (Kummu et al., 2012). If the waste of meat products is considered, FAO (2013) estimates 250 km3 of blue water was wasted due to FLW in 2007. Placing this in context, more than three times as much surface water and groundwater is wasted each year due to FLW than the average annual flow of the Nile River (Siam and Eltahir, 2017).
Global freshwater wasted in FLW per capita per year is ~21 m3 (Chen et al., 2020). However, the water embedded in FLW and its impact on water scarcity varies significantly depending on the person's diet and waste patterns, which is strongly influenced by where one lives (Chen et al., 2020) (Figure 1). High-income countries waste 43 m3 per capita each year, while low-income countries only waste a little over 4 m3 per capita each year (Chen et al., 2020). Food loss and waste is responsible for 7.1% of the freshwater planetary boundary (Chen et al., 2020), which denotes freshwater supplies that can be appropriated to society without causing continental-scale to planetary-scale harm (Rockström and Karlberg, 2010). If the world had a similar diet and pattern of food waste as North America, global FLW would constitute 20% of the freshwater planetary boundary (Chen et al., 2020).
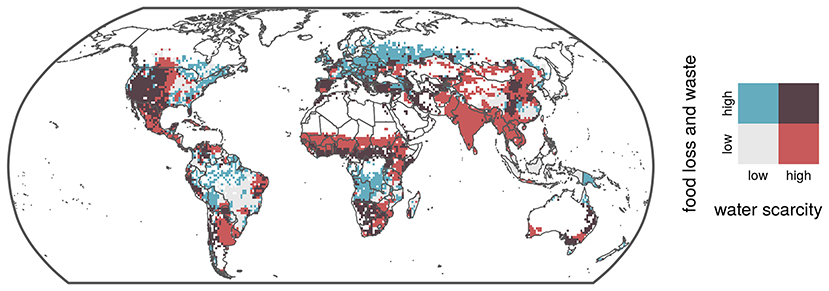
Figure 1. Map overlapping local water scarcity and crop-specific rates of food loss and waste within the region. Dark grid cells represent areas with high levels of water scarcity and high regional rates of loss and waste of the predominant crops grown within the grid cell. Water scarcity data are from Mekonnen and Hoekstra (2016), crop area data are from Monfreda et al. (2008), and food loss and waste rates are from Gustavsson et al. (2011).
Differences in Wasted Food and Water Resources Across Countries
Table 1 provides a summary of studies that report the blue water footprint of FLW of different regions or countries. Relevant studies were identified using two methods. First, we searched for English language publications containing key terms “food loss and waste” and “water” in Web of Science between the years 2000–2020. This search returned 636 articles. We narrowed this list by eliminating papers unrelated to our review, such as those focused on using food waste as a feedstock for energy production. Second, we used citation chaining for key papers to identify additional papers that may have been missed in our initial search. The Connected Papers online tool (https://www.connectedpapers.com/) was used for citation chaining. Remaining papers that quantified the blue water footprint of food loss and/or waste were included in our study and found in Table 1, as well as the References section.
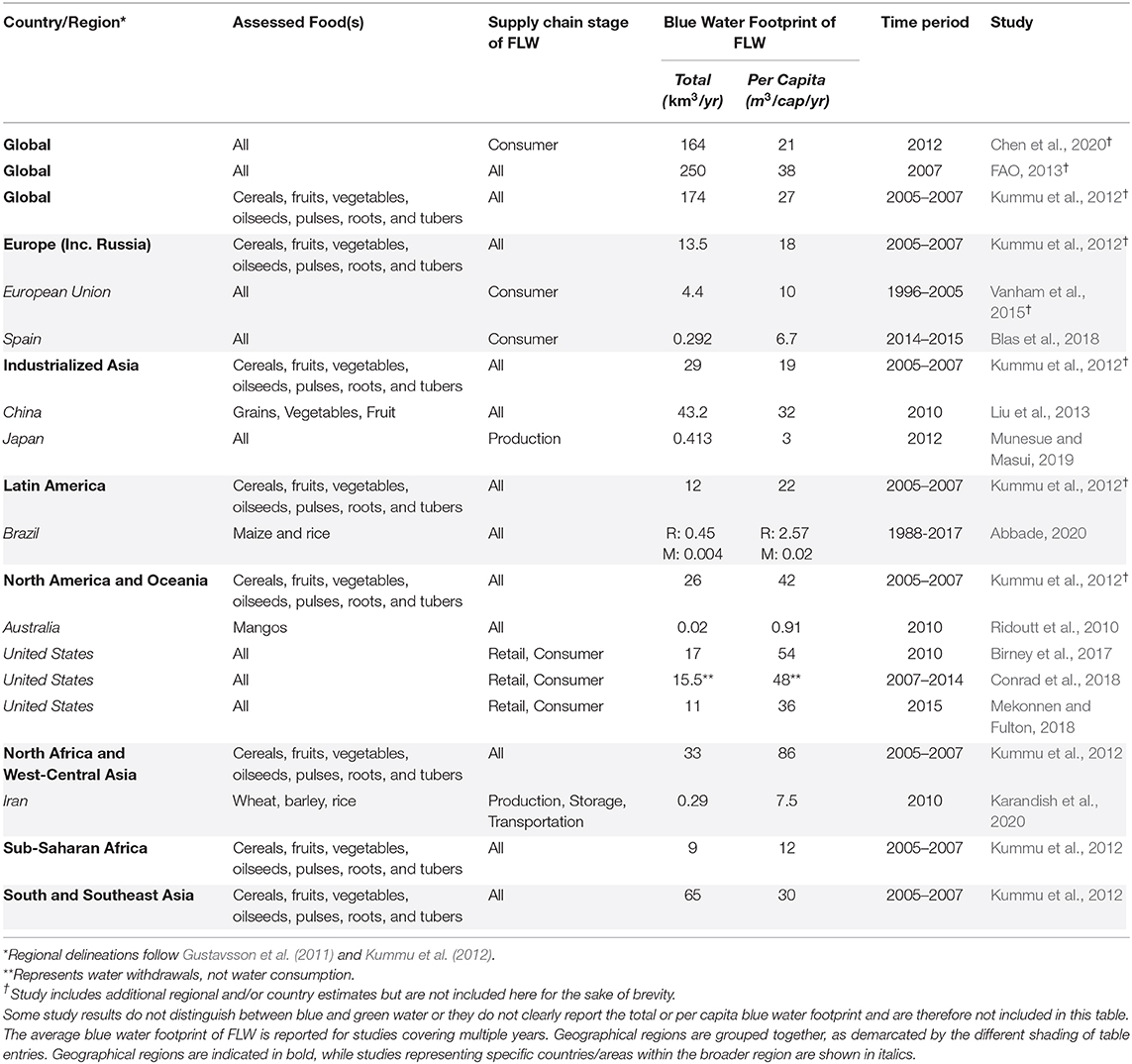
Table 1. Summary of studies that evaluate the blue water footprint of food loss and/or waste (FLW) by country or region.
Regional- and national-scale studies evaluating the freshwater implications of FLW are more prevalent for high-income countries. Total European Union (EU) food waste averages 123 kg/cap/year of food, 80% of which is avoidable (Vanham et al., 2015). This FLW amounts to 4 km3/year of blue water and 52 km3/year of green water (i.e., soil moisture available for plant transpiration; Vanham et al., 2015). In the United Kingdom, the water footprint of avoidable FLW comprises 5–6% of the nation's total water footprint of consumption (Chapagain and James, 2011, 2013). In 2012, Japan's blue water footprint of FLW was estimated as 0.4 km3 (Munesue and Masui, 2019). The United States has the highest blue water footprint associated with FLW per capita (54.9 m3/cap/year; Chen et al., 2020) and, along with India and China, the largest volume of blue water attributed to FLW (16–40 km3; Lundqvist et al., 2008; Birney et al., 2017; Conrad et al., 2018). Altogether, FLW is responsible for 25–34% of the United States consumptive freshwater used for food production (Hall et al., 2009; Birney et al., 2017; Mekonnen and Fulton, 2018).
Over one-third of the global population lives in India and China, meaning that current and future food consumption and waste of these countries will have major implications on global water consumption and scarcity. The FLW of China amounted to 62.8 million metric tons in 2010, which, according to Sun et al. (2018), constituted between 60.5 km3 of wasted blue and green water (~10% of the nation's total water consumption). Liu et al. (2013) estimated the blue water footprint of China's cereal, fruit, and vegetable FLW between 24.1 km3 and 62.3 km3. When factoring in green water, China's FLW water footprint is between 75.3 km3 and 194.7 km3 (Liu et al., 2013). If the waste of meat and animal products is also considered, an additional 18.6–92.6 km3 of blue and green water is wasted (though some of these losses overlap with losses attributed to wasted grain; Liu et al., 2013). The blue and green water footprint of FLW in India was 105 ± 3.8 km3 in 2013, with a little over half of the water footprint attributed to just one crop, sugarcane (Kashyap and Agarwal, 2020).
Data limitations, contrasting FLW definitions, and methodological differences underlie large variability in reported water footprints of FLW. Vanham et al. (2015) notes the considerable uncertainty in much of the underlying data used in all FLW analysis. For the EU, Vanham et al. attempt to partially account for this uncertainty by estimating a range of blue water and green water footprints of FLW of EU consumers, quantifying the FLW blue water footprint between 4.7 and 14.6 m3/cap/year and the FLW green water footprint between 46.4 and 163.9 m3/cap/years. Some distinction in FLW estimates between countries is expected due to differences in food production, processing, transportation, storage, retail, consumer diets, and cultural dining preferences (e.g., prevalence of dining out vs. dining at home). For instance, countries that rely more heavily on irrigated agriculture will typically have a larger blue water footprint of FLW, which may be more reflective of production or climatic conditions than higher rates of FLW. Data collection and reporting methods of FLW can also cause significant divergence in FLW estimates and are major sources of uncertainty in the water footprint of FLW. As an example, studies in Spain (Blas et al., 2018) and Japan (Munesue and Masui, 2019) calculate the blue water footprint of FLW using estimates of FLW from country-specific surveys that estimate FLW as much as an order of magnitude smaller than energy/mass balance or other approaches to estimate FLW for high-income countries (Chen et al., 2020) and Europe/Industrialized Asia (Kummu et al., 2012).
Differences in Wasted Food and Water Resources Across the Supply Chain
Food's blue water footprint and contributions to water scarcity are largely attributed to irrigated crop production, as water consumption in other stages of the value chain is comparatively small for most foods (Hoekstra and Mekonnen, 2012; Marston et al., 2018). The degree to which water scarcity is attributed to FLW is a function of the underlying baseline water scarcity, the water requirements of the wasted crop, and the rate of loss and waste of the food produced (see Figure 1). Many studies ignore, or greatly simplify, the complex supply chains that connect the locations of food production, processing, consumption, and waste (e.g., Reynolds et al., 2015; Conrad et al., 2018; Cooper et al., 2018), which has implications on estimated water footprints and contributions to water scarcity of FLW.
It is often assumed that food production and consumption occur within the same country or region, yet this may not be true for some food types or countries. For instance, 41% of Spain's water footprint of food consumption and waste comes from outside the country (Blas et al., 2018). Much of Spanish consumer food waste is from imported dairy products, which have a high water footprint as well as a high consumer waste rate (Blas et al., 2018). Likewise, 79% of Japan's blue water footprint of food wastage is from food imports, with over half of the blue water coming from just one country, the United States, and primarily consisting of wasted cereal grains (Munesue and Masui, 2019). Over 90% of the water consumption and biodiversity impacts associated with Swiss FLW occur outside of Switzerland (Beretta et al., 2017).
The stage of the supply chain where loss or wastage occurs has implications on water resources. Detailed life cycle assessments from the UK (Tonini et al., 2018), US (Read et al., 2020), Switzerland (Beretta et al., 2017), Japan (Munesue and Masui, 2019), and Australia (Ridoutt et al., 2010; Reutter et al., 2017) point to water use and impacts of FLW largely occurring during the production stage, despite the majority of FLW occurring at the consumer level in high-income countries. In China, FLW is greatest at the consumer level and during storage (Liu et al., 2013). The lack of proper storage facilities, effective pesticides, and advanced technologies increase food losses during the storage stage relevant to higher income countries (Liu et al., 2013). Luo et al. (2020) isolated the on-farm grain storage losses in China and found that 2.66 km3 of water can be saved by reducing storage losses from the current loss rate (2.41%) to a loss rate typically found using more advanced storage technologies and techniques (1.00%). Using an environmental-extended input-output model (EEIO), Read et al. (2020) found that water use of FLW in the US could be minimized by first reducing FLW among household consumers, followed by targeted reductions in food processing, and then foodservice operations.
Differences in Wasted Food and Water Resources by Type of Food
The water scarcity implications of FLW can vary significantly between different food types and even for the same food product depending on where and how it was produced (e.g., irrigated vs. rainfed). One must consider not only the amount of water used to produce a food product but also the loss/wastage rate of that food product and its relative importance within consumers' diets. For instance, the blue water footprint of lost and wasted cereal grains is the primary contributor to the overall blue water footprint of FLW, despite its blue water footprint per unit of production and loss/wastage rate being relatively modest in relation to other food types (Gustavsson et al., 2011; Hoekstra and Mekonnen, 2012). The propensity for cereal grains to be grown in water-scarce areas (Karandish et al., 2020) and their place as a diet staple for much of the global population means that the loss/waste of cereals account for 40–50% of the global blue water footprint of all FLW (Kummu et al., 2012; Chen et al., 2020) and between 60 and 80% of the blue water footprint of FLW in Asia (Kummu et al., 2012).
Meat and dairy products are major contributors to the blue water footprint of FLW in high-income countries where meat and dairy products constitute a sizable portion of consumers' diets and have relatively large water footprints. In the US, the waste of meat and dairy represents 58% of FLW water footprint (Mekonnen and Fulton, 2018). The blue water footprint of meat waste in the EU is larger than any other food category and is 50% larger than fruit, which has the second largest wasted blue water footprint (Vanham et al., 2015). Waste of animal-derived foods was only 13% of FLW by weight in China, yet made up 44% of the water footprint of FLW (Song et al., 2015). However, in India animal products are the smallest contributor to the water footprint of FLW (<4%). Instead, sugarcane, rice, wheat, and potatoes make up over 70% of the water footprint associated with FLW (Kashyap and Agarwal, 2020). Regional differences in diets and waste rates mean that food waste reduction measures will have different outcomes in reducing water scarcity by region.
Does Reducing Food Loss and Waste Reduce Water Scarcity?
The last decade of research has established a clear link between wasted food and wasted water. More recently, several studies have investigated the effects of FLW reduction measures on water consumption and scarcity (e.g., Ma et al., 2019; Read et al., 2020). These studies do not typically focus on specific policy or interventions at reducing FLW (Read and Muth, 2021); instead, they test different scenarios of FLW reduction, often ranging from 10 to 50% reduction, and then assume a corresponding reduction in water consumption. The upper end of this range of FLW reduction (50%) aligns with the United Nations Sustainable Development Goal 12.3 of halving consumer FLW by 2030. Halving global FLW could reduce the water footprint of global food production by 12-13% (Jalava et al., 2016; Springmann et al., 2018). Moreover, reducing FLW by 50% would improve water scarcity for over 720 million people globally, and totally eliminate local water scarcity for 131 million of those people (Jalava et al., 2016).
Policies aiming to reduce FLW do not act in isolation and may involve tradeoffs or synergies with other policy measures and objectives. For instance, shifting the global population to a more nutritious and less water-intensive diet in parallel with FLW reduction measures reduces blue water footprints and green water footprints by 23 and 28%, respectively, which is more than the sum of the two strategies implemented independently (Jalava et al., 2016). However, policy measures and awareness campaigns that aim to improve diets without explicitly considering FLW may increase FLW and its associated water footprint (Birney et al., 2017; Min et al., 2020). To complicate matters further, differences in socio-econmic status and development may yield different FLW outcomes for similar policy measures. In China, an increase in dietary knowledge increased household food waste where community development was low but decreased household food waste where community development was high (Min et al., 2020).
While it is important to have a global understanding of how FLW reduction measures lessen society's environmental footprint (in line with Sustainable Development Goal 12.3), more localized studies typically provide richer analysis. For example, eliminating all losses and waste of cereal grains in Iran can reduce the blue water footprint of cereal grain production within the country by 0.54 km3 (5% of the total blue water footprint), with over 90% of these savings occurring in arid and semi-arid provinces where water is most scarce (Karandish et al., 2020). If FLW cannot be eliminated at the source, the next best alternative is to redirect it to feed hungry people (see Figure 2). An Australian study found that every US dollar invested in measures to redirect FLW to food insecure people saves 6.6 m3 of water by not requiring additional food production (Reynolds et al., 2015). Australian food rescue efforts saved 131 million m3 of water in 2008 alone (Reynolds et al., 2015). Birney et al. (2017) investigated the blue and green water impact of reducing FLW between 10 and 60% in the US and found that at the higher reduction goal each person in the US could reduce the blue water and green water footprint of their FLW by 36 and 220 m3 per year, respectively. After evaluating several specific FLW reduction and reuse measures in Switzerland, Beretta et al. (2017) conclude that avoiding food waste should be the highest priority, while selecting the optimal set of FLW reduction measures is less relevant.
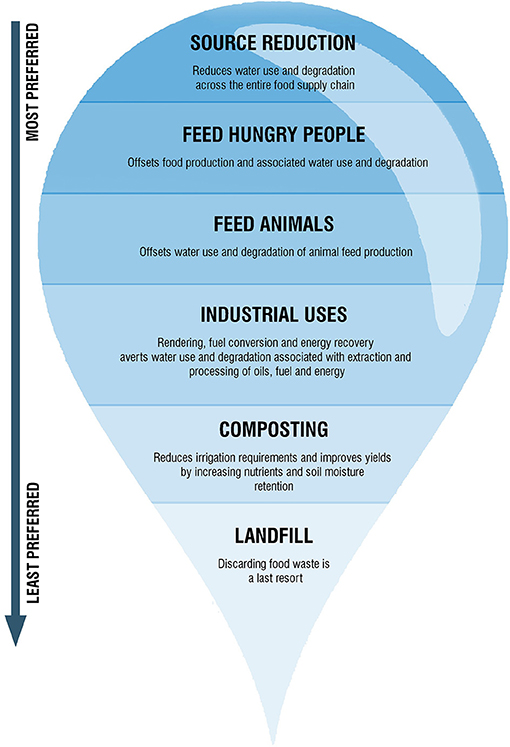
Figure 2. The EPA's Food Recovery Hierarchy prioritizes measures to reduce food loss and waste based on the benefits they bring to the environment, society, and economy. Here, we highlight each of these strategies' corresponding impact on water resources. Adapted from the EPA (https://www.epa.gov/sustainable-management-food).
Policy Measures to Reduce FLW and Water Scarcity
Food waste has received growing interest from local and national policymakers, international organizations, NGOs, and academics. Governments and international organizations have set policy targets to reduce food waste and its attendant environmental impacts. For example, both the European Union (European Commission, 2019) and the United States (US EPA, 2019) have instituted goals to reduce food waste at the retail and consumer levels by 50% by 2030, following the United Nations' Sustainable Development Goal 12.3 (Rosa, 2017).
Food loss and waste have a diversity of causes (Thyberg and Tonjes, 2016) and an equally diverse set of policies are needed to combat it (Muth et al., 2019). Food waste policies may have one or more of three potential goals: (1) waste prevention, (2) recovery of otherwise wasted food for consumption by humans (e.g., donation to food banks; repurposing manufacturing by-products) or (3) recycling of wasted food (e.g., using food waste to produce animal feed; generating bioenergy from waste) (Muth et al., 2019). Due to the environmental impacts averted if food is not produced in the first place, the US Environmental Protection Agency (EPA) Food Waste Recovery Hierarchy prioritizes prevention over all forms of recovery and recycling (Figure 2).
Typically, each policy intervention to reduce FLW targets only a single stage of the food supply chain: farms and agricultural producers, manufacturers, restaurants and retailers, or consumers (Aschemann-Witzel et al., 2017). In addition, each intervention takes a different approach to waste prevention, including improvements to technology, introduction of economic incentives to reduce waste or disincentives to produce waste, and campaigns to raise awareness or provide information to encourage waste reduction. Proposed or enacted FLW reduction policy interventions range in scale, from municipal to international (Shafiee-Jood and Cai, 2016), though most existing policies tend to be relatively local in scope.
Empirical evidence that FLW reduction policy leads to alleviation of water scarcity is limited. This is primarily because (1) policy interventions targeted at reducing FLW are still in their infancy, (2) those that have been implemented typically do not adequately monitor outcomes (Stöckli et al., 2018), and (3) even in the cases where FLW reduction can be attributed to the intervention, it is difficult to directly measure associated changes in resource use throughout the supply chain. Instead, reductions in water use are usually estimated indirectly from models. However, a review of environmental evaluations of food waste prevention showed that almost all decreases in water use attributed to FLW reduction come from avoided food production, with much smaller benefits from improved food waste management (Schott and Cánovas, 2015).
Regulatory approaches and institutional approaches may be more effective at reducing waste than financial incentives and promotion of technological innovations. While regulatory approaches to FLW reduction are relatively uncommon, there are examples of regulations aimed at reducing FLW that have been enacted at national and local scales. At the national scale, supermarkets in France are legally obliged to donate some portion of their unsold product to social institutions or convert it to animal feed, and violators may be assessed a fine. A similar law in Italy provides tax benefits for supermarkets and facilitates distribution of donations, without implementing the punitive approach of the French law (Schinkel, 2019). Locally, many municipalities in the United States, Canada, and Europe have enacted “pay-as-you-throw” ordinances, in which households are charged by the volume of waste (including organic waste) they generate. This incentivizes household waste reduction but assessments of their effectiveness have shown mixed results (Dahlén and Lagerkvist, 2010; van der Werf et al., 2020). A national-level correlational study indicates that countries with a regulatory approach to food waste reduction have lower per capita food waste rates than countries where fiscal incentives are the primary strategy to reduce FLW (Chalak et al., 2016); however, it is difficult to assess the direction of causality at such a coarse scale. When assessing the effectiveness of specific interventions at the consumption stage, institutional interventions such as updates to school nutritional guidelines aimed at waste reduction and changes in delivery methods of cafeteria food were found to be superior to interventions aimed at households (Reynolds et al., 2019).
Theory suggests a tax on food waste can reduce FLW and its accompanying environmental damage (Katare et al., 2017), yet there is little evidence of this in practice. Some argue that internalizing the environmental externalities of food production and waste would raise food prices and thereby spur FLW reduction (Cattaneo et al., 2020a). The evidence that financial incentives at the household level promote reduced food waste is mixed. Theory predicts that a combination of government incentives and pay-by-weight disposal tax will reduce household food waste (Katare et al., 2017). However, at the household level, approaches such as pay-by-weight taxes on food waste disposal may be counterproductive; financial incentives may actually undermine individuals' intrinsic motivation not to waste food (Dahlén and Lagerkvist, 2010). A recent survey of European Union citizens indicates that waste-reduction behaviors by households tend to be motivated by altruism rather than external factors (Cecere et al., 2014). U.S. households are even willing to pay for recycling services (Kinnaman, 2006), rendering financial incentives for households to reduce FLW superfluous. In general, to effectively reduce the environmental impact of FLW, policy initiatives should holistically target all actors along the supply chain, not just the final consumer (Schanes et al., 2018).
The private sector, particularly large transnational food corporations, plays a critical role in reducing FLW at all stages of the supply chain through their influence on both consumers and producers. Certain components of the food sector are highly concentrated, meaning that implementing sustainability standards aimed at reducing FLW by just a handful of companies can have substantial impacts throughout the supply chain, shaping the practices of both upstream and downstream actors. For example, eight companies control 54% of the global soybean market, three companies control 42% of the global banana market, and just three companies control 60% of the cocoa market (Folke et al., 2019). In the US, three companies control markets for three quarters of all cattle and over half of all pigs and chickens (Smith et al., 2017). These companies can influence production decisions and waste of upstream suppliers, spur on investments in food storage, transportation, and packaging to ensure more food reaches consumers, adjust portion sizes to align with household consumption patterns, promote closed-loop supply chains such that waste is utilized within the systems, and create uniformity in food date labeling to ensure food isn't thrown out prematurely (Springmann et al., 2018; Muth et al., 2019). However, social pressure and government regulations will likely be needed to move food companies to reduce FLW and implement sustainability practices throughout their supply chains given the private sectors priority of profit over environmental or societal goals (Grizzetti et al., 2013; Folke et al., 2019).
Recommendations for Future Research
The food demand of a growing and more affluent population is expected to require nearly 3,000 km3 of freshwater resources by the year 2050, a 65% increase from 2010 (Springmann et al., 2018). To meet the conflicting challenges of feeding the global population and environmental stewardship, producers, consumers, NGOs, international organizations, corporations, and governments will need to provide a portfolio of solutions working in unison to remain within planetary boundaries (Springmann et al., 2018). A single policy intervention will not be able to shift the complex food system to the desired level of efficiency at each stage of the supply chain. Unique cultural, regulatory, and economic contexts will determine the effectiveness of different intervention measures in different locations and stages of the supply chain. A decade's worth of research has established FLW reduction as a potential solution to improving food and water security. Yet, the degree of uncertainty inherent in these estimates, their lack of precision, and several simplifying assumptions make it difficult to translate this research into robust policy measures to reduce the environmental burden of FLW.
Additional research is needed to understand the nuances of different policy interventions on water consumption and scarcity, as well as resource use and environmental damage more broadly. More specifically, research needs to focus on i) quantifying and reducing uncertainty through enhanced data collection and methods; ii) integrated assessment of specific policy measures; iii) advancing geographically-specific supply chain tracing.
Quantifying and Reducing Uncertainty Through Enhanced Data Collection and Methods
Limited and poor-quality records of FLW for certain foods, stages of the supply chain, and geographic regions make policy design challenging. The global scope of the food supply chain means that the current dearth of FLW data for low- and medium-income countries (Schneider, 2013), as well as limited food loss data between farm fields and retailers (Reynolds et al., 2019), will lead to high levels of uncertainty that will propagate through most FLW studies. The high degree of uncertainty underlying most FLW research affects the interpretation of study findings and the conclusions that can be drawn from them with respect to shaping FLW reduction policy. When linking FLW to water use, the uncertainties associated with FLW estimates are amplified due to similar uncertainties associated with (often) unmeasured or validated estimates of water use (Konar and Marston, 2020) detail how uncertainty in water footprint estimates can be reduced).
Targeted research investments are needed to identify critical regions, food types, and stages of the supply chain where measurements of FLW will be most helpful in shaping FLW reduction policy. Recent methodological advances and a convergence on shared definitions and data standards (e.g., Hanson et al., 2016; FAO, 2019) will help reduce existing uncertainty in FLW. However, investments need to be made in primary data collection efforts, particularly in understudied areas such as on-farm, storage, transportation, and processing food losses and FLW in the non-Western world. Where data and/or funding limits detailed surveys of FLW, energy or mass balance approaches (e.g., Buzby et al., 2014; Barrera and Hertel, 2021) can continue to be used but researchers should design their approach to harmonize with other FLW data (Cattaneo et al., 2020b). Researchers can identify types of wasted food most effective in reducing water scarcity so investments and policy measures can target foods that have a higher likelihood of leading to the desired environmental outcomes, even when accounting for underlying uncertainty [Kuiper and Cui (2020) provide an example of such research focused on GHG emissions].
Reducing sources of FLW data uncertainty, such as those attributed to systematic, methodological, or data-processing errors, should be prioritized. However, when uncertainty cannot be further reduced, researchers need to quantitatively assess and/or qualitatively describe the uncertainty associated with their findings. Qualitative descriptions of uncertainty can follow a rating scale [see Hanson et al. (2016) for examples and a listing of all sources of FLW data uncertainty], as well as provide discussion on validation measures, sample size, and critical assumptions underlying the data (e.g., groups left out of the study or that were out of scope and the impact of their exclusion on results). When possible, a quantitative assessment of uncertainty in FLW studies should be undertaken, which both helps decision-makers in forming FLW reduction measures and highlights areas where uncertainty is the largest so that target investments can be made to most effectively minimize uncertainty. Since FLW and water use estimates occur at multiple supply chain stages, parameter uncertainty propagates through all water footprint estimates of FLW, yet is seldom quantified. Calculating confidence intervals for all FLW (and associated water footprint) estimates will not alleviate uncertainty inherent in these studies but will aid policymakers in understanding the potential range of impacts and the degree to which proposed solutions may reduce FLW and associated water scarcity.
Integrated Assessment of Specific Policy Measures
An array of policy measures working in unison will likely be required to halve FLW as prescribed by Sustainable Development Goal 12.3. Yet, few studies evaluate specific FLW reduction policy measures or regulations and their interactions; instead, most research assumes a specified percentage reduction in FLW and that this reduction in FLW leads to an equivalent reduction in food production and associated water use. These studies greatly simplify the non-linear socio-economic system underpinning the food sector and ignore system feedback and cascading effects through the supply chain. Integrated assessments that consider interactions between demand and supply, substitution effects, and how changes in prices and costs are distributed throughout the economic system are needed to assess the effectiveness and incidental impacts of specific FLW reduction policy measures (Rutten, 2013; Kuiper and Cui, 2020).
Future research needs to build on recent FLW scholarship that uses partial equilibrium models (Barrera and Hertel, 2021), computable general equilibrium (CGE) models (Rutten et al., 2013; Philippidis et al., 2019), and environmentally-extended input-output models (Reutter et al., 2017; Read et al., 2020) to investigate the system-wide effects of FLW reduction measures. These models consider interactions between different segments of the economy that may lead to nonlinear dynamics. For example, Philippidis et al. (2019) found a 25–50% reduction in EU household food waste only led to 0.2–0.6% decrease in EU blue water consumption (121–316 million m3)—nearly an order of magnitude smaller than what simpler models that assume a reduction of FLW leads to an equivalent reduction in water consumption would suggest. This discrepancy calls for further investigation into how dynamics of the economic system shape FLW policy outcomes, particularly as they relate to environmental objectives.
An integrated assessment of specific FLW policy measures will aid policymakers in mitigating undesired outcomes of FLW reduction interventions, such as rebound effects and leakage. Targeted policy that reduces consumer waste of a certain food group may lead to lower effective food prices and increased consumer spending and waste of other, perhaps more water-intensive, food goods (Martinez-Sanchez et al., 2016). Thus, the net impact of reduced FLW may actually be an increase in water consumption and scarcity, not less—an example of a “rebound effect” or “Jevons Paradox.” The net effect of FLW reduction policy will be determined by the price elasticity of supply and demand (which dictate price adjustments) and how these price adjustments are transmitted between countries and supply chain stages (Barrera and Hertel, 2021). Further, lower food prices due to less FLW in one stage of the supply chain could make consumer food waste more likely. Martinez-Sanchez et al. (2016) highlight the importance of rebound effects by showing that FLW prevention measures may lead to welfare gains but could also lead to higher environmental impacts due to increased consumption of more environmentally damaging goods. Similarly, Salemdeeb et al. (2017) use a hybrid life-cycle assessment model paired with multi-regional environmentally-extended input-output model to show that rebound effects related to FLW prevention measures decrease potential reductions in GHG emissions by up to 60%. Additional research is needed to determine how much a rebound effect reduces potential water savings of specific FLW reduction policy.
Leakage can occur when FLW reduction policy is enacted in one location but leads to increased water consumption and scarcity elsewhere. While additional research is needed to determine the degree of leakage associated with particular policy interventions, initial research investigating leakage in the context of GHG emissions and food losses in livestock production suggest that displacement of environmental damages is a likely outcome of some FLW reduction policy (Kuiper and Cui, 2020). While national policymakers cannot directly control environmental conditions outside their borders, the local policies they enact can have second- and third-order impacts that can lead to environmental damage outside their jurisdiction. With greater understanding of how these systems work and how effects propagate through the system, policymakers can coordinate their efforts to mitigate these unintended environmental consequences.
Policy interventions can take considerable time to implement and the effects of these measures may not be seen until well into the future. Therefore, projections of future FLW are needed to refine assessments of medium- and long-term implications of FLW reduction policies. Trajectories of food production, consumption, loss/waste, and associated water use necessitate interdisciplinary efforts to assess how changes in food production, technology, water availability, supply chain and market integration, consumer incomes, demographics, and population together shape future FLW and its associated environmental impacts. Studies can build on the research of Barrera and Hertel (2021), who use a global partial equilibrium model of the agriculture sector to develop future trajectories of household food waste to 2050 and assess the environmental and food security implications associated with different pathways.
Advancing Geographically-Specific Supply Chain Tracing
If FLW reduction policy is to minimize the environmental impact of lost or wasted food, the scientific community needs to partner with the private sector to identify and link specific locations of environmental degradation or resource use to instances of FLW. Water use and scarcity are strongly contingent on the place of crop irrigation, though much of the food and embodied water is wasted at other stages of the supply chain, which are often displaced from the place of production. The impact of FLW reduction measures on water scarcity is difficult to assess due to heterogeneity in water use impacts and diversified sourcing across supply chains. Cattaneo et al. (2020a) found that consumer food waste reduction consistently improves environmental outcomes, but FLW reduction interventions at other stages of the food value chain provide varied results due to the aforementioned heterogeneity in environmental conditions and supply chains. From a policy perspective, the price signals caused by FLW reduction measures targeted downstream in the supply chain may be so weakened by the time they propagate through the supply chain and reach farmers that production and water use does not meaningfully change. Additional research is needed to determine how policy interventions in one place impact location-specific environmental outcomes.
To meaningfully assess the impact FLW has on water scarcity and specific water bodies, spatially detailed mapping of food supply chains is required. In the US, recent advances enabled the mapping of county-level food commodity flows (Lin et al., 2019) and spatial-linking of different stages of the supply chain (Garcia et al., 2020). In most regions, however, the private and public sectors will need to work together to chart out supply chains. The public sector can make supply chains more transparent by setting data and reporting standards and creating platforms for data sharing. Large food corporations can spur innovation and promote sustainability throughout the industry and prompt environmental stewardship throughout their supply chains as has been seen through the CDP Supply Chain program (https://www.cdp.net/en/research/global-reports/global-supply-chain-report-2019). However, these data must be made available to researchers and policymakers alike in order to create scientifically-grounded FLW reduction policy that is effective in reducing water scarcity.
Increasing supply chain transparency is critical for the global social and environmental impacts of food waste to be visible and to create a case for governmental intervention as well as social responsibility (Ridoutt et al., 2010). Many supply chain intermediaries are unaware of the geographical source, let alone water footprint, of their inputs. Spatially-explicit and traceable supply chains will require a private sector commitment toward transparency and wider adoption of blockchain and other novel technologies (e.g., www.trase.earth). Blockchain technology has the potential to track the provenance of supply chain inputs, thereby enabling the water footprint to be traced as well (Kamilaris et al., 2019). Furthermore, sustainability labels on food products would raise consumer awareness of the water footprint of the goods they consume, including those they waste. However, some research shows that consumers often do not fully understand these metrics, nor do they alter their behavior as a result (Grunert et al., 2014; Leach et al., 2016).
Discussion
Reducing food loss and waste has the potential to improve both food and water security. The last decade of research has shown the scope and scale of the problem of FLW and its accompanying environmental consequences. To address the global problem of FLW, policymakers need more complete and robust data on FLW and integrated assessments that better reflects the complex food system and its response to proposed policy measures. Beyond scientific advances and sound policy, public education programs aimed at increasing consumer awareness of FLW, its environmental implications, and ways they can prevent FLW is critical to spur FLW reductions at the consumer level, as well as drive further government response.
Here, we have narrowly focused on how FLW reduction policy measures can moderate water consumption and water scarcity. However, policymakers will likely have multiple objectives in reducing FLW beyond just lessening water scarcity (e.g., enhancing food security, improving social welfare, mitigating other environmental damage; see Muth et al., 2019 for a broader review of these other objectives of FLW reduction). While policymakers are charged with determining policy goals and priorities, the scientific community can aid by assessing tradeoffs between policy objectives and determine which combination of policies provide the most beneficial outcomes with respect to the priorities set forth by policymakers. Policy goals, such as improved farm welfare, a reduction in environmental impacts, and increased food security, are often conflicting, which necessitates a coherence between policy measures to ensure they do not counteract one another.
Author Contributions
LM conceived the study. All authors contributed to the writing of the manuscript.
Funding
This work was supported in part by the National Socio-Environmental Synthesis Center (SESYNC) under funding received from the National Science Foundation DBI-1639145. LM acknowledges support by the National Science Foundation Grant No. ACI-1639529 INFEWS/T1: Mesoscale Data Fusion to Map and Model the US Food, Energy, and Water (FEW) system). Any opinions, findings, and conclusions or recommendations expressed in this material are those of the author(s) and do not necessarily reflect the views of the National Science Foundation.
Conflict of Interest
The authors declare that the research was conducted in the absence of any commercial or financial relationships that could be construed as a potential conflict of interest.
References
Abbade, E. B. (2020). Land and water footprints associated with rice and maize losses in Brazil. Land Use Policy. 99:105106. doi: 10.1016/j.landusepol.2020.105106
Aschemann-Witzel, J., De Hooge, I., Amani, P., Bech-Larsen, T., and Oostindjer, M. (2015). Consumer-related food waste: causes and potential for action. Sustainability 7, 6457–6477. doi: 10.3390/su7066457
Aschemann-Witzel, J., Jensen, J. H., Jensen, M. H., and Kulikovskaja, V. (2017). Consumer behaviour towards price-reduced suboptimal foods in the supermarket and the relation to food waste in households. Appetite 116, 246–258. doi: 10.1016/j.appet.2017.05.013
Barrera, E. L., and Hertel, T. (2021). Global food waste across the income spectrum: Implications for food prices, production and resource use. Food Policy. 98:101874. doi: 10.1016/j.foodpol.2020.101874
Beretta, C., Stucki, M., and Hellweg, S. (2017). Environmental impacts and hotspots of food losses: value chain analysis of swiss food consumption. Environ. Sci. Technol. 51, 11165–11173. doi: 10.1021/acs.est.6b06179
Birney, C. I., Franklin, K. F., Davidson, F. T., and Webber, M. E. (2017). An assessment of individual foodprints attributed to diets and food waste in the United States. Environ. Res. Lett. 12:105008. doi: 10.1088/1748-9326/aa8494
Blas, A., Garrido, A., and Willaarts, B. (2018). Food consumption and waste in Spanish households: water implications within and beyond national borders. Ecol. Indic. 89, 290–300. doi: 10.1016/j.ecolind.2018.01.057
Buzby, J. C., Farah-Wells, H., and Hyman, J. (2014). The estimated amount, value, and calories of postharvest food losses at the retail and consumer levels in the United States. USDA-ERS Econ. Inform. Bull. 121. doi: 10.2139/ssrn.2501659
Cattaneo, A., Federighi, G., and Vaz, S. (2020a). The environmental impact of reducing food loss and waste: a critical assessment. Food Policy 98:101890. doi: 10.1016/j.foodpol.2020.101890
Cattaneo, A., Sánchez, M. V., Torero, M., and Vos, R. (2020b). Reducing food loss and waste: five challenges for policy and research. Food Policy 98:101974. doi: 10.1016/j.foodpol.2020.101974
CEC. (2018). Characterization and Management of Food Loss and Waste in North America – White Paper, North American Initiatives on Food and Organic Waste. Washington, DC.
Cecere, G., Mancinelli, S., and Mazzanti, M. (2014). Waste prevention and social preferences: the role of intrinsic and extrinsic motivations. Ecol. Econ. 107, 163–176. doi: 10.1016/j.ecolecon.2014.07.007
Chalak, A., Abou-Daher, C., Chaaban, J., and Abiad, M. G. (2016). The global economic and regulatory determinants of household food waste generation: a cross-country analysis. Waste Manag. 48, 418–422. doi: 10.1016/j.wasman.2015.11.040
Chapagain, A., and James, K. (2011). The Water and Carbon Footprint of Household Food and Drink Waste in the UK. Surrey: Waste & Resources Action Programme (WRAP), Banbury, Oxon, UK & WWF, Godalming.
Chapagain, A. K., Hoekstra, A. Y., and Savenije, H. H. G. (2006). Water saving through international trade of agricultural products. 10, 455–468. doi: 10.5194/hessd-2-2219-2005
Chapagain, A. K., and James, K. (2013). “Accounting for the impact of food waste on water resources and climate change,” in Food Industry Wastes, ed M. R. Kosseva and C. Webb (San Diego: Academic Press), 217–236. doi: 10.1016/B978-0-12-391921-2.00012-3
Chen, C., Chaudhary, A., and Mathys, A. (2020). Nutritional and environmental losses embedded in global food waste. Resour. Conserv. Recycling. 160:104912. doi: 10.1016/j.resconrec.2020.104912
Conrad, Z., Niles, M. T., Neher, D. A., Roy, E. D., Tichenor, N. E., and Jahns, L. (2018). Relationship between food waste, diet quality, and environmental sustainability. PLoS ONE. 13:e0195405. doi: 10.1371/journal.pone.0195405
Cooper, K. A., Quested, T. E., Lanctuit, H., Zimmermann, D., Espinoza-Orias, N., and Roulin, A. (2018). Nutrition in the bin: a nutritional and environmental assessment of food wasted in the UK. Front. Nutr. 5:19. doi: 10.3389/fnut.2018.00019
Dahlén, L., and Lagerkvist, A. (2010). Pay as you throw: Strengths and weaknesses of weight-based billing in household waste collection systems in Sweden. Waste Manage. 30, 23–31. doi: 10.1016/j.wasman.2009.09.022
Davis, K. F., Rulli, M. C., Seveso, A., and D'Odorico, P. (2017). Increased food production and reduced water use through optimized crop distribution. Nat. Geosci. 10, 919–924. doi: 10.1038/s41561-017-0004-5
Ellison, B., Muth, M. K., and Golan, E. (2019). Opportunities and challenges in conducting economic research on food loss and waste. Appl. Econo. Persp. Policy. 41, 1–19. doi: 10.1093/aepp/ppy035
European Commission. (2019). EU Platform on Food Losses and Food Waste Terms of Reference. Available at: https://ec.europa.eu/food/sites/food/files/safety/docs/fw_eu-actions_flw-platform_tor.pdf (accessed December 28, 2020).
Folke, C., Österblom, H., Jouffray, J. B., Lambin, E. F., Adger, W. N., Scheffer, M., et al. (2019). Transnational corporations and the challenge of biosphere stewardship. Nat. Ecol. Evol. 3, 1396–1403. doi: 10.1038/s41559-019-0978-z
Garcia, S., Rushforth, R., Ruddell, B. L., and Mejia, A. (2020). Full domestic supply chains of blue virtual water flows estimated for major US cities. Water Resour. Res. 56:e2019WR026190. doi: 10.1029/2019WR026190
Grizzetti, B., Pretato, U., Lassaletta, L., Billen, G., and Garnier, J. (2013). The contribution of food waste to global and European nitrogen pollution. Environ. Sci. Policy 33, 186–195. doi: 10.1016/j.envsci.2013.05.013
Grunert, K. G., Hieke, S., and Wills, J. (2014). Sustainability labels on food products: consumer motivation, understanding and use. Food Policy 44, 177–189. doi: 10.1016/j.foodpol.2013.12.001
Gustavsson, J., Cederberg, C., Sonesson, U., Otterdijk, R.v, and Meybeck, A. (2011). Global food losses and Food Waste: Extent, Causes and prevention. Rome: Food and Agriculture Organization of the United Nations (FAO), 29.
Hall, K. D., Guo, J., Dore, M., and Chow, C. C. (2009). The progressive increase of food waste in America and its environmental impact. PLoS ONE 4:e7940. doi: 10.1371/journal.pone.0007940
Hanson, C., Lipinski, B., Robertson, K., Dias, D., Gavilan, I., Gréverath, P., et al. (2016). Food Loss and Waste Accounting and Reporting Standard. Washington, DC: World Resources Institute.
Hoekstra, A. Y., and Mekonnen, M. M. (2012). The water footprint of humanity. Proc. Natl. Acad. Sci. U.S.A. 109, 3232–3237. doi: 10.1073/pnas.1109936109
Jägermeyr, J., Gerten, D., Heinke, J., Schaphoff, S., Kummu, M., and Lucht, W. (2015). Water savings potentials of irrigation systems: global simulation of processes and linkages. Hydrol. Earth Syst. Sci. 19, 3073–3091. doi: 10.5194/hess-19-3073-2015
Jalava, M., Guillaume, J. H., Kummu, M., Porkka, M., Siebert, S., and Varis, O. (2016). Diet change and food loss reduction: what is their combined impact on global water use and scarcity? Earth's Future 4, 62–78. doi: 10.1002/2015EF000327
Kamilaris, A., Fonts, A., and Prenafeta-Boldú, F. X. (2019). The rise of blockchain technology in agriculture and food supply chains. Trends Food Sci. Technol. 91, 640–652. doi: 10.1016/j.tifs.2019.07.034
Karandish, F., Hoekstra, A. Y., and Hogeboom, R. J. (2020). Reducing food waste and changing cropping patterns to reduce water consumption and pollution in cereal production in Iran. J. Hydrol. 586:124881. doi: 10.1016/j.jhydrol.2020.124881
Kashyap, D., and Agarwal, T. (2020). Food loss in India: water footprint, land footprint and GHG emissions. Environ. Dev. Susta. 22, 2905–2918. doi: 10.1007/s10668-019-00325-4
Katare, B., Serebrennikov, D., Wang, H. H., and Wetzstein, M. (2017). Social-optimal household food waste: taxes and government incentives. Am. J. Agric. Econ. 99, 499–509. doi: 10.1093/ajae/aaw114
Kinnaman, T. C. (2006). Policy watch: examining the justification for residential recycling. J. Econ. Persp. 20, 219–232. doi: 10.1257/jep.20.4.219
Konar, M., and Marston, L. (2020). The Water Footprint of the United States. Water 12:3286. doi: 10.3390/w12113286
Kuiper, M., and Cui, H. D. (2020). Using food loss reduction to reach food security and environmental objectives–A search for promising leverage points. Food Policy 98:101915. doi: 10.1016/j.foodpol.2020.101915
Kummu, M., De Moel, H., Porkka, M., Siebert, S., Varis, O., and Ward, P. J. (2012). Lost food, wasted resources: global food supply chain losses and their impacts on freshwater, cropland, and fertiliser use. Sci. Total Environ. 438, 477–489. doi: 10.1016/j.scitotenv.2012.08.092
Leach, A. M., Emery, K. A., Gephart, J., Davis, K. F., Erisman, J. W., Leip, A., et al. (2016). Environmental impact food labels combining carbon, nitrogen, and water footprints. Food Policy 61, 213–223. doi: 10.1016/j.foodpol.2016.03.006
Lin, X., Ruess, P. J., Marston, L., and Konar, M. (2019). Food flows between counties in the United States. Environ. Res. Lett. 14:084011. doi: 10.1088/1748-9326/ab29ae
Liu, J., Lundqvist, J., Weinberg, J., and Gustavsson, J. (2013). Food losses and waste in China and their implication for water and land. Environ. Sci. Technol. 47, 10137–10144. doi: 10.1021/es401426b
Lundqvist, J., de Fraiture, C., and Molden, D. (2008). Saving Water: From Field to fork: Curbing Losses and Wastage in the Food Chain. Stockholm: Stockholm International Water Institute.
Luo, Y., Huang, D., Li, D., and Wu, L. (2020). On farm storage, storage losses and the effects of loss reduction in China. Resour. Conserv. Recycling. 162:105062. doi: 10.1016/j.resconrec.2020.105062
Ma, L., Bai, Z., Ma, W., Guo, M., Jiang, R., Liu, J., et al. (2019). Exploring future food provision scenarios for China. Environ. Sci. Technol. 53, 1385–1393. doi: 10.1021/acs.est.8b04375
Marston, L., Ao, Y., Konar, M., Mekonnen, M. M., and Hoekstra, A. Y. (2018). High-resolution water footprints of production of the United States. Water Resour. Res. 54, 2288–2316. doi: 10.1002/2017WR021923
Marston, L., and Konar, M. (2017). Drought impacts to water footprints and virtual water transfers of the Central Valley of California. Water Resour. Res. 53, 5756–5773. doi: 10.1002/2016WR020251
Marston, L. T., Lamsal, G., Ancona, Z. H., Caldwell, P., Richter, B. D., Ruddell, B. L., et al. (2020). Reducing water scarcity by improving water productivity in the United States. Environ. Res. Lett. 15:094033. doi: 10.1088/1748-9326/ab9d39
Martinez-Sanchez, V., Tonini, D., Møller, F., and Astrup, T. F. (2016). Life-cycle costing of food waste management in Denmark: importance of indirect effects. Environ. Sci. Technol. 50, 4513–4523. doi: 10.1021/acs.est.5b03536
Mekonnen, M. M., and Fulton, J. (2018). The effect of diet changes and food loss reduction in reducing the water footprint of an average American. Water Int. 43, 860–870. doi: 10.1080/02508060.2018.1515571
Mekonnen, M. M., and Hoekstra, A. Y. (2016). Four billion people facing severe water scarcity. Sci. Adv. 2:e1500323.q. doi: 10.1126/sciadv.1500323
Min, S., Wang, X., and Yu, X. (2020). Does dietary knowledge affect household food waste in the developing economy of China? Food Policy 98:101896. doi: 10.1016/j.foodpol.2020.101896
Monfreda, C., Ramankutty, N., and Foley, J. A. (2008). Farming the planet: 2. Geographic distribution of crop areas, yields, physiological types, and net primary production in the year 2000, Global Biogeochem. Cycles 22:GB1022. doi: 10.1029/2007GB002947
Munesue, Y., and Masui, T. (2019). The impacts of Japanese food losses and food waste on global natural resources and greenhouse gas emissions. J. Industr. Ecol. 23, 1196–1210. doi: 10.1111/jiec.12863
Muth, M. K., Birney, C., Cuéllar, A., Finn, S. M., Freeman, M., Galloway, J. N., et al. (2019). A systems approach to assessing environmental and economic effects of food loss and waste interventions in the United States. Sci. Total Environ. 685, 1240–1254. doi: 10.1016/j.scitotenv.2019.06.230
National Academies of Sciences Engineering and Medicine. (2020). A National Strategy to Reduce Food Waste at the Consumer Level. Washington, DC: National Academies Press.
Philippidis, G., Sartori, M., Ferrari, E., and M'Barek, R. (2019). Waste not, want not: a bio-economic impact assessment of household food waste reductions in the EU. Resour. Conserv. Recycl. 146, 514–522. doi: 10.1016/j.resconrec.2019.04.016
Read, Q. D., Brown, S., Cuéllar, A. D., Finn, S. M., Gephart, J. A., Marston, L. T., et al. (2020). Assessing the environmental impacts of halving food loss and waste along the food supply chain. Sci. Total Environ. 712:136255. doi: 10.1016/j.scitotenv.2019.136255
Read, Q. D., and Muth, M. K. (2021). Financial costs and environmental benefits of four food waste interventions: is food waste reduction a “win-win?” Resour. Conserv. Recycl. 168:105448. doi: 10.1016/j.resconrec.2021.105448
Reutter, B., Lant, P., Reynolds, C., and Lane, J. (2017). Food waste consequences: environmentally extended input-output as a framework for analysis. J. Clean. Prod. 153, 506–514. doi: 10.1016/j.jclepro.2016.09.104
Reynolds, C., Goucher, L., Quested, T., Bromley, S., Gillick, S., Wells, V. K., et al. (2019). Review: Consumption-stage food waste reduction interventions – What works and how to design better interventions. Food Policy 83, 7–27. doi: 10.1016/j.foodpol.2019.01.009
Reynolds, C. J., Piantadosi, J., and Boland, J. (2015). Rescuing food from the organics waste stream to feed the food insecure: an economic and environmental assessment of Australian food rescue operations using environmentally extended waste input-output analysis. Sustainability 7, 4707–4726. doi: 10.3390/su7044707
Ridoutt, B. G., Juliano, P., Sanguansri, P., and Sellahewa, J. (2010). The water footprint of food waste: case study of fresh mango in Australia. J. Clean. Prod. 18, 1714–1721. doi: 10.1016/j.jclepro.2010.07.011
Rockström, J., and Karlberg, L. (2010). The Quadruple Squeeze: Defining the safe operating space for freshwater use to achieve a triply green revolution in the Anthropocene. Ambio 39, 257–265. doi: 10.1007/s13280-010-0033-4
Rosa, W. (Ed.). (2017). Transforming Our World: The 2030 Agenda for Sustainable Development: A New Era in Global Health. New York, NY: Springer Publishing Company.
Rutten, M. M. (2013). What economic theory tells us about the impacts of reducing food losses and/or waste: implications for research, policy and practice. Agricult. Food Secur. 2:13. doi: 10.1186/2048-7010-2-13
Rutten, M. M., Nowicki, P. L., Bogaardt, M. J., and Aramyan, L. H. (2013). Reducing Food Waste by Households and in Retail in the EU; A prioritisation Using Economic, Land Use and Food Security Impacts (No. 2013-035). Wageningen: LEI, part of Wageningen UR.
Salemdeeb, R., Font Vivanco, D., Al-Tabbaa, A., and zu Ermgassen, E. K. H. J. (2017). A holistic approach to the environmental evaluation of food waste prevention. Waste Manag. 59, 442–450. doi: 10.1016/j.wasman.2016.09.042
Schanes, K., Dobernig, K., and Gözet, B. (2018). Food waste matters—A systematic review of household food waste practices and their policy implications. J. Clean. Prod. 182, 978–991. doi: 10.1016/j.jclepro.2018.02.030
Schinkel, J. (2019). Review of policy instruments and recommendations for effective food waste prevention. Procee. Instit. Civil Engin. Waste Resour. Manag. 172, 92–101. doi: 10.1680/jwarm.18.00022
Schneider, F. (2013). Review of food waste prevention on an international level. Procee. Instit. Civil Engin. Waste Resour. Manag. 166, 187–203. doi: 10.1680/warm.13.00016
Schott, A. B. S., and Cánovas, A. (2015). Current practice, challenges and potential methodological improvements in environmental evaluations of food waste prevention–A discussion paper. Resour. Conserv. Recycl. 101, 132–142. doi: 10.1016/j.resconrec.2015.05.004
Segrè, A., Falasconi, L., Politano, A., and Vittuari, M. (2014). Background Paper on the Economics of Food Loss and Waste (Working Paper). Rome: Food and Agriculture Organization of the United Nations.
Shafiee-Jood, M., and Cai, X. (2016). Reducing food loss and waste to enhance food security and environmental sustainability. Environ. Sci. Technol. 50, 8432–8443. doi: 10.1021/acs.est.6b01993
Siam, M. S., and Eltahir, E. A. (2017). Climate change enhances interannual variability of the Nile river flow. Nat. Clim. Chang. 7, 350–354. doi: 10.1038/nclimate3273
Smith, T. M., Goodkind, A. L., Kim, T., Pelton, R. E., Suh, K., and Schmitt, J. (2017). Subnational mobility and consumption-based environmental accounting of US corn in animal protein and ethanol supply chains. Proc. Natl. Acad. Sci. U.S.A. 114, E7891–E7899. doi: 10.1073/pnas.1703793114
Song, G., Li, M., Semakula, H. M., and Zhang, S. (2015). Food consumption and waste and the embedded carbon, water and ecological footprints of households in China. Sci. Total Environ. 529, 191–197. doi: 10.1016/j.scitotenv.2015.05.068
Springmann, M., Clark, M., Mason-D'Croz, D., Wiebe, K., Bodirsky, B. L., Lassaletta, L., et al. (2018). Options for keeping the food system within environmental limits. Nature 562, 519–525. doi: 10.1038/s41586-018-0594-0
Stöckli, S., Niklaus, E., and Dorn, M. (2018). Call for testing interventions to prevent consumer food waste. Resour. Conserv. Recycl. 136, 445–462. doi: 10.1016/j.resconrec.2018.03.029
Sun, S. K., Lu, Y. J., Gao, H., Jiang, T. T., Du, X. Y., Shen, T. X., et al. (2018). Impacts of food wastage on water resources and environment in China. J. Clean. Prod. 185, 732–739. doi: 10.1016/j.jclepro.2018.03.029
Thyberg, K. L., and Tonjes, D. J. (2016). Drivers of food waste and their implications for sustainable policy development. Resour. Conserv. Recycl. 106, 110–123. doi: 10.1016/j.resconrec.2015.11.016
Tonini, D., Albizzati, P. F., and Astrup, T. F. (2018). Environmental impacts of food waste: Learnings and challenges from a case study on UK. Waste Manag. 76, 744–766. doi: 10.1016/j.wasman.2018.03.032
US EPA. (2019). Winning on Reducing Food Waste (2019). Available online at: https://www.epa.gov/sustainable-management-food/winning-reducing-food-waste-federal-interagency-strategy (accessed August 22, 2019).
van der Werf, P., Larsen, K., Seabrook, J. A., and Gilliland, J. (2020). How neighbourhood food environments and a Pay-as-You-Throw (PAYT) waste program impact household food waste disposal in the city of Toronto. Sustainability 12:7016. doi: 10.3390/su12177016
Vanham, D., Bouraoui, F., Leip, A., Grizzetti, B., and Bidoglio, G. (2015). Lost water and nitrogen resources due to EU consumer food waste. Environ. Res. Lett. 10, 084008. doi: 10.1088/1748-9326/10/8/084008
Vanham, D., Comero, S., Gawlik, B. M., and Bidoglio, G. (2018). The water footprint of different diets within European sub-national geographical entities. Nature Sust. 1, 518–525. doi: 10.1038/s41893-018-0133-x
Vanham, D., Mekonnen, M. M., and Hoekstra, A. Y. (2013). The water footprint of the EU for different diets. Ecol. Indic. 32, 1–8. doi: 10.1016/j.ecolind.2013.02.020
World Commission on Dams. (2000). Dams and Development: A New Framework for Decision-Making: The Report of the World Commission on Dams. Cape Town: Earthscan.
Keywords: water footprint, water scarcity, policy, food loss, food waste
Citation: Marston LT, Read QD, Brown SP and Muth MK (2021) Reducing Water Scarcity by Reducing Food Loss and Waste. Front. Sustain. Food Syst. 5:651476. doi: 10.3389/fsufs.2021.651476
Received: 09 January 2021; Accepted: 04 March 2021;
Published: 01 April 2021.
Edited by:
Marta Tuninetti, Politecnico di Torino, ItalyReviewed by:
La Zhuo, Northwest A and F University, ChinaBarbara Anna Willaarts, International Institute for Applied Systems Analysis (IIASA), Austria
Copyright © 2021 Marston, Read, Brown and Muth. This is an open-access article distributed under the terms of the Creative Commons Attribution License (CC BY). The use, distribution or reproduction in other forums is permitted, provided the original author(s) and the copyright owner(s) are credited and that the original publication in this journal is cited, in accordance with accepted academic practice. No use, distribution or reproduction is permitted which does not comply with these terms.
*Correspondence: Landon T. Marston, bG1hcnN0b24mI3gwMDA0MDt2dC5lZHU=