- 1Agroecology, Department of Crop Sciences, University of Göttingen, Göttingen, Germany
- 2Faculty of Sustainability Science, Institute of Ecology, Leuphana University, Lüneburg, Germany
Global agrobiodiversity is threatened by the replacement of traditional, locally adapted crop varieties with high-yielding and hybrid varieties during the past 60 years, resulting in associated losses of crop, variety, and allele diversity. Locally adapted, traditional varieties are known to perform equal or even better under environmental stress conditions and to be more resilient in unstable cultivation environments. Therefore, European organic vegetable breeding organizations conserve local, traditional varieties and breed new varieties in low-input organic environments, aiming to increase the range of varieties for sustainable cultivation under sub-optimal growing conditions. However, performance of organic vegetable varieties, in comparison to conventional high-yielding and hybrid varieties, under different environmental conditions has not been intensively researched. To contribute to this scientific field, we compared the agronomic and quality performance between hybrid, conventional, and organic tomato and sweet pepper varieties, two economically important species on the EU market under a) well-watered and b) drought stress conditions, using five different varieties (i.e., 30 varieties) as replicates in each of the six groups. Performance of both species was negatively affected by drought, regardless of the breeding background. Equally, for tomato and sweet pepper, hybrids produced higher amounts of individual fruits, however total yield in kg was comparable for hybrid, conventional and organic plants. Considering the agro-ecological importance of enlarging and securing variety diversity in light of changing environmental conditions, we show that the assumed benefits of the hybrids can also be delivered by the organic and conventional varieties. These varieties should be considered as an important source of genetic resources, supporting farmers to adapt to their local climate and environmental conditions in the future.
Introduction
Agrobiodiversity and the associated diversity of crop varieties is one of the global keystones in farming to secure stable harvest and livelihood under changing environmental and socio-economic conditions (FAO, 2018). A major ongoing threat to agrobiodiversity is the loss of traditional, locally adapted crop varieties equally for cereal, and vegetable species (MEA, 2005). One relevant reason for this loss is the replacement of traditional, open-pollinated varieties with commercial high-yielding and hybrid varieties during the past 60 years, resulting in a genetic bottleneck for crop, variety, and allele diversity (Gmeiner et al., 2018; Nemeskéri and Helyes, 2019). In the vegetable sector, the share of hybrid seeds on the market is increasing worldwide by about 8–10% per year (Da Silva Dias, 2010; Department of Agriculture Cooperation, 2012). At the same time, genetic resources in the form of vegetable variety diversity are declining all over the world at a rate 1–2% annually (Da Silva Dias, 2010).
This progressive concentration on a limited amount of commercial high-yielding and hybrid varieties is further promoted by usage restrictions via patents and variety protection laws and the privatization of seed material (Kotschi and Wirz, 2015; Messmer et al., 2015). Improvements in plant breeding techniques, such as field selection methods, use of inbred-lines creating F1-hybrids, laboratory techniques at the tissue or cell level, and techniques at DNA level have made high-yielding and hybrid varieties to a reliable source for high harvest quantity and quality under well-adjusted, optimal growing conditions, despite the often higher charged price for the seeds (Riggs, 1988; Ficiciyan et al., 2018). However, locally adapted, traditional varieties can deliver equal or even higher yields under suboptimal conditions and are known to be more resilient in unstable cultivation environments (Ficiciyan et al., 2018).
The increase of drought periods and their higher frequency is thereby currently one of the most critical environmental stress factors, lowering productivity in both, arable and vegetable production (Kumar et al., 2012; Kahiluoto et al., 2019). Especially, vegetable species are known for their high drought sensitivity during flowering and seed development (Kumar et al., 2012; Nemeskéri and Helyes, 2019), and yield reductions through drought occur in important species such as tomato, sweet pepper, bean, and sweet corn (Kumar et al., 2012). Thus, for agro-ecological and organic farming systems, a high diversity of crop varieties–including locally adapted, traditional varieties–is considered to support the resilience of vegetable production systems under unpredictable future weather conditions (Kumar et al., 2012; Conti et al., 2019).
Hence, more and more organic plant breeding organizations are scaling up on the European plant breeding sector, aiming to enlarge the diversity of vegetable varieties and to offer alternatives to high-yielding and hybrid varieties that still deliver reasonable yields, but are resistant against diseases and can cope with adverse local growing conditions such as limited water supply. Driven by a heightened awareness of consumers, these organizations conserve traditional varieties and develop new varieties under organic standards without synthetic pesticides and fertilizers (Gmeiner et al., 2018). Organic plant breeding rejects techniques such as genetically modified organisms, protoplast fusion, in vitro propagation, and induced mutation. Hybrid breeding is also avoided as it is not conducive to maintain genetic diversity (Lammerts van Bueren and Struik, 2004; Messmer et al., 2015). Another notable aspect of organic breeding is the rejection of plant variety protection via patents or private property protection. Most organic breeding organizations believe that commons-based rights, as opposed to private protection, on seeds and varieties are essential for a social-ecological transformation of plant production (Kotschi and Wirz, 2015; Gmeiner et al., 2018).
To our knowledge, no study has yet tested experimentally the performance of well-replicated sets of organic vegetable varieties in comparison to commercial high-yielding and hybrid varieties under different environmental conditions. To fill this knowledge gap, we used tomato and sweet pepper varieties as model species in this study and tested the following hypotheses:
I Compared to organic and commercial high-yielding varieties, hybrids perform best in terms of yield, yield stability and yield quality under optimal, well-watered conditions.
II The overall performance (yield, yield stability, yield quality) of organic varieties is higher under drought stress than that of commercial high-yielding and hybrid varieties.
We focused on tomato (Solanum lycopersicum L.) and sweet pepper (Capsicum spp.) as model species, since they are economically highly important for the EU vegetable market and popular among consumers. Moreover, tomatoes and sweet peppers are known to be sensitive to drought at all development stages, which underlines the necessity to identify drought tolerant varieties (Suguru et al., 2003; Wahb-Allah et al., 2011; Rossi, 2019).
Materials and Methods
This experiment was carried out in a greenhouse (180 m2, graveled area with water supply) with two open sides and a translucent Perspex roof of the experimental botanical garden of the University of Göttingen between March and October 2017. Cultivating the plants in the open-sided greenhouse allowed controlling soil moisture while the environmental parameters in the greenhouse stayed under nearly natural light and temperature conditions (detailed information on location and climate data see Supplementary Annex 2).The experiment took place as a pot experiment to investigate the performance of the plants under controlled, standardized conditions, minimizing potential confounding effects of heterogeneous biotic and abiotic factors (Schwarz et al., 2014; Figure 1).
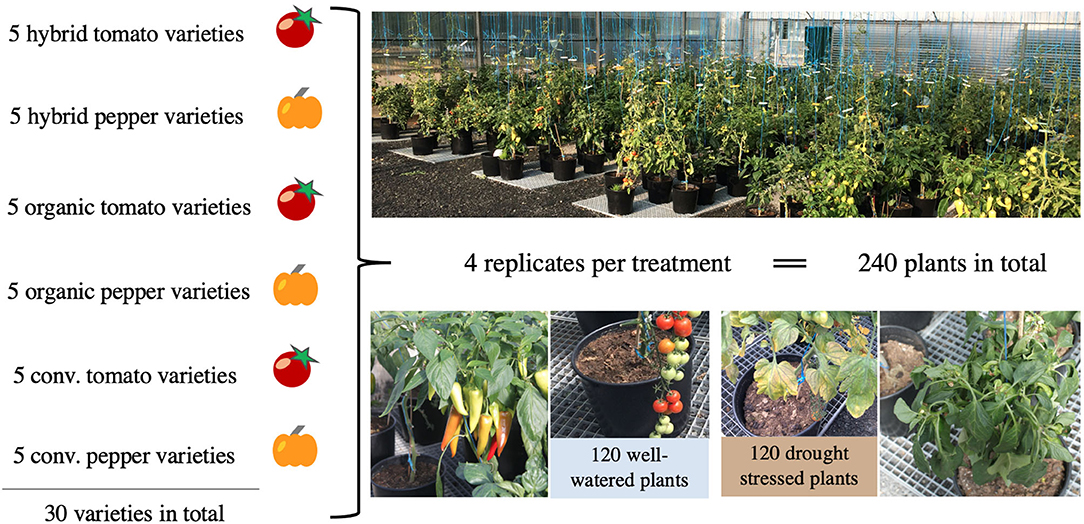
Figure 1. Experimental setup including 15 tomato and 15 sweet pepper varieties. In a randomized block design (above picture) with 4 replicates per treatment the whole experiment contained 120 well-watered plants (60 tomato and 60 sweet pepper plants, left pictures) and 120 drought stressed plants (also 60 tomato and 60 sweet pepper plants, right pictures).
We designed our study to experimentally compare variety groups from (i) hybrid breeding under conventional farming methods (hybrid group) (ii) open-pollinated high-yield breeding under conventional farming methods (conventional group), and (iii) open-pollinated organic breeding/conservation under organic farming methods (organic group) under well-watered as well as drought stress conditions.
Each of these six groups consisted of five varieties (i.e., all together 15 tomato and 15 sweet pepper varieties involved) as replicates. The groups were tested for agronomic performance in terms of yield (total fresh harvested weight and number of fruits), as well as yield stability (variability of yield between varieties of one breeding group), and quality performance expressed in fruit quality (commercial quality classes).
Organic varieties came from the German organic vegetable breeding and preservation association Kultursaat e.V. (sold by Bingenheimer Saatgut AG). Hybrid and conventional varieties came from the Bruno Nebelung GmbH, selling conventional varieties under the Kiepenkerl label. All varieties have a variety approval according to the German Seed Marketing Act (Verbraucherschutz, 2018). One dwarf variety of the conventional group (Vilma) was heavily infected by botrytis and thus had to be removed from the second half of the experiment, to avoid infestation of the other plants. Furthermore, the variety Pyros of the conventional tomato group turned out to be a hybrid instead of an open-pollinated variety and had to be excluded from the analysis. A detailed description of all varieties that were finally included in the analysis can be found in Supplementary Annex 1. The hybrid tomato and sweet pepper varieties were highest in retail price with 0.66 Euro and 0.75 euro per seed (Table 1). For tomato price per seed for varieties from the hybrid group was thus 3.5 times higher than that of the organic group and 5 times higher than that of the conventional group (Table 1). For sweet pepper price per seed for varieties from the conventional group was in the mid-range with 0.47 Euro per seed, followed by the price for organic sweet pepper seeds, which was lowest with 0.23 Euro (Table 1).
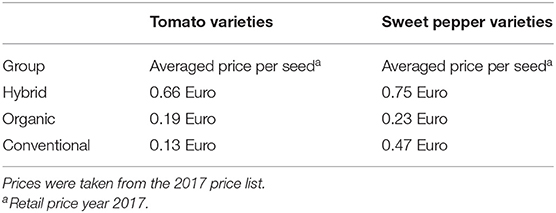
Table 1. Averaged retail seed price for hybrid, organic, and conventional tomato and sweet pepper varieties.
In a randomized block design with four repetitions, each variety was planted under well-watered conditions and under drought stress. Since the experiment was repeated four times, a total of eight plants (four drought stressed plants plus 4 well-watered plants) were tested for each variety, resulting in a total number of 240 pots (Figure 1). We used 15-liter plastic pots filled with 15 liters of Hawita Typ T [pH-value (CaCl2) = 5.9, salinity in g/KCl = 2.9, nitrogen (N) in mg/l (CaCl2) = 180, Phosphate (P2O5) in mg/l (CAL) = 180, Kalium (K2O) in mg/l (CAL) = 260, Magnesium (Mg) in mg/l (CaCl2) = 130]. Plants were watered by hand to treat well-watered and drought stress plants differently and in a standardized way. Drought stress was controlled manually in every corresponding pot using a HH2 Moisture Meter from Delta T Devices designed for field use. Water content in the substrate of 9 to 14% was taken to represent drought stress, and between 15 and 20% was considered optimal. Water content below 9% was considered under supply. Percentages were determined in a preliminary test. Plants with drought stress showed significantly lower turgor pressure (visible through soft leaves and stalks as well as hanging heads) and the substrate should be detached from the pot edge. However, plants were able to regain turgor after reapplication of water in order to survive. Plants were alternatingly fertilized with nitrogen (Wuxal Top N−12% N, 4% P, 6% K) and phosphorus (Wuxal Top P−5% N, 20% P, 5% K) liquid fertilizer, directly mixed into the water according to the mixing ratio recommended by the suppliers. All plants were tied with synthetic baler twine to the ceiling, and tomato plants were thinned out once a week to reduce branching. During the flowering period, two bumble bee colonies were installed between the plants to support pollination.
Harvest
The harvest period covered 9 weeks from the 12th of July to the 14th of September 2017 with fifteen harvest events. At each harvest event (15 harvest events in total), we only harvested individual, ripe tomato and sweet pepper fruits and no complete trusses from the tomato plants. The harvested fruits were collected separately for every plant in individual plastic boxes, marked with the plant and treatment information, and subsequently counted, weighted and subdivided into commercial classes by the same person. We used the regulations for marketing standards for tomatoes and sweet pepper provided by the Federal Office for Agriculture and Food in Germany, which are in accordance with the law in the European Union [Commission Implementing Regulation (EU) No 543/2011, 2011]. We identified 10 different types of deficiency symptoms for commercial class two and minus on the fruits: too small fruits, apical-blossom end rot, gold flecking, color defects, greenbacks, scab, shaping errors, sun burn, color errors, and botrytis (see Figure 2).
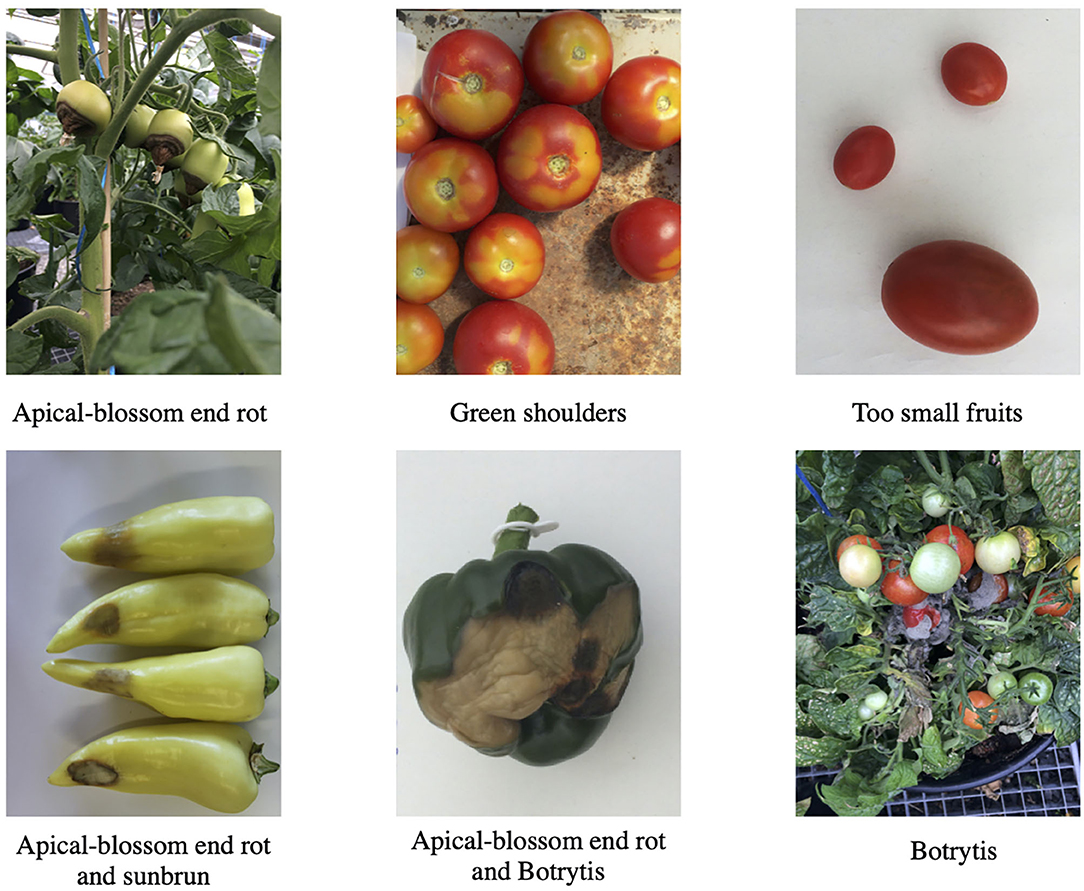
Figure 2. Selection of deficiency symptoms recorded on tomato and sweet pepper fruits. Affected fruits were categorized into commercial class 2 (green shoulders, too small fruits) or minus (botrytis, apical-blossom end rot). Symptoms were detected equally on hybrid, organic, and conventional varieties.
Statistical Analyses
All statistical analyses were performed using R version 3.6.1 (R Core Team, 2019). We analyzed the performance of the tomato and sweet pepper varieties separately.
For analyzing tomato and sweet pepper yield, we used fruit number and fresh fruit weight (in grams) per plant as the dependent variables in a zero-inflated negative binomial generalized, linear mixed-effect model, using Template Model Builder (“GlmmTMB” Package). Row (1–6), treatment (control or drought), and group (hybrid, organic, conventional), were set as fixed factors. Harvest date was set as a crossed random effect. “GlmmTMBs” fit linear and generalized linear mixed models with various extensions, including zero-inflation. The models were fitted using maximum likelihood estimation (Brooks et al., 2019). For the tomato varieties, fruit number and fruit weight data were not normally distributed. For fruit weight, breeding background caused zero-inflation. For fruit number, zero inflation was independent of predictor variables. For the sweet pepper varieties fruit number and weight were also not normally distributed. Zero-inflation was caused by the harvest event (p < 0.001) equally for fruit number and weight.
Zero-inflation was checked using the “DHARMa” package that provides a number of plot and test functions for overdispersion and zero-inflation. In “DHARMa,” zero-inflation is accounted for by running the model excluding all zeros caused by the corresponding predictor variable (Hartig, 2019). Final model selection was done by AIC comparison and visual plot analysis. Differences in means were controlled with post hoc-tests using the “emmean” package (Lenth et al., 2019). Correlation between fruit number and fruit weight was checked with Spearman-Rank Correlation.
Likewise, for tomato and sweet pepper fruit quality we used the number of fruits per class summed up over all harvest events as the dependent variable. Here too, row (1–6), treatment (control or drought), and breed (hybrid, organic, conventional), were set as fixed factors. Number of fruits was summed up over the whole harvest period. For the final model, we used a negative binomial generalized linear mixed (“lme4” package) because the data showed overdispersion. The negative binomial regression model adds a multiplicative random effect for unobserved heterogeneity (Rodríguez, 2013). Again, we controlled for the overdispersion using the “DHARMa” package. Final model selection was also done by AIC comparison and visual plot analysis. Differences in means were tested with post hoc-tests using “emmean” package (Lenth et al., 2019).
To evaluate yield stability for hybrid, organic, and conventional tomato and sweet pepper varieties under the control and drought treatment, we calculated and plotted the coefficient of variation for fruit weight and fruit number over the whole harvest period. We used the R package “cvequality” (Marwick and Krishnamoorthy, 2019) and run asymptotic tests to test for significant differences between the coefficients of variation from hybrid, organic, and conventional varieties.
Results
Tomato Results
Agronomic Performance–Yield and Yield Stability
Tomato plants under well-watered conditions (N = 56 plants) produced a total of 86 kg fresh tomatoes, and plants under drought stress (N = 56 plants) a total of 35 kg over the whole harvest period (see Table 3). The “GlmmTMB” model showed that drought stress resulted in significantly lower fruit weight and fruit number for the hybrid organic and the conventional group (p < 0.001, Table 2, see Figure 3A). Comparing the three groups revealed that the loss rate for fruit weight under drought stress was similar, with minus 58% for the hybrid, minus 64% for the organic, and minus 51% for the conventional group. For fruit number we found a lower productivity rate of minus 25% for the hybrid group, minus 31% for the organic, and minus 9% for the conventional group under drought stress (Table 3). Despite some plant failures within the conventional group (see methods section), the number of fruits of the conventional group under drought stress only decreased by 9%, and thus considerably less than the other two groups. However, fruit weight loss was equivalently high under drought stress. This means that the conventional group produced many, but relatively small fruits under drought stress.
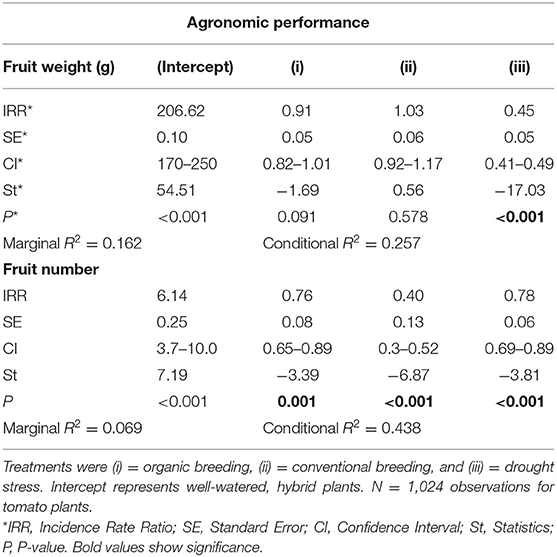
Table 2. Factors influencing agronomic performance via fruit weight and fruit number of tomato plants as dependent variables.
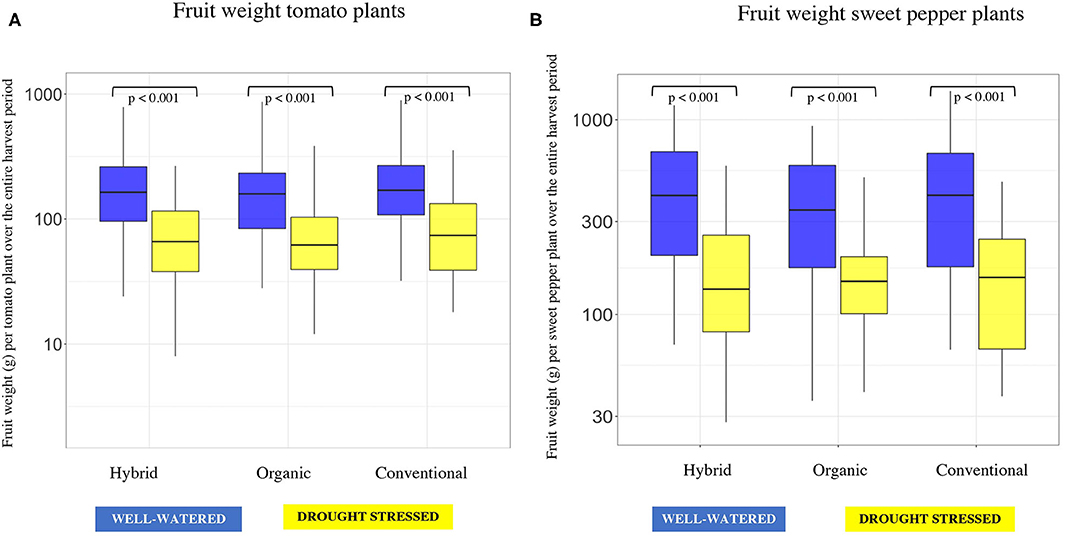
Figure 3. Comparison of fruit weight in grams per plant on average over the entire harvest period between the hybrid, organic, and conventional groups for tomato (A) and sweet pepper plants (B) under well-watered conditions and drought stress. Differences between treatments were significant equally for tomato and sweet pepper (p < 0.001). Each boxplot shows median (line within the box), lower and upper quartile (box), and range (whiskers).
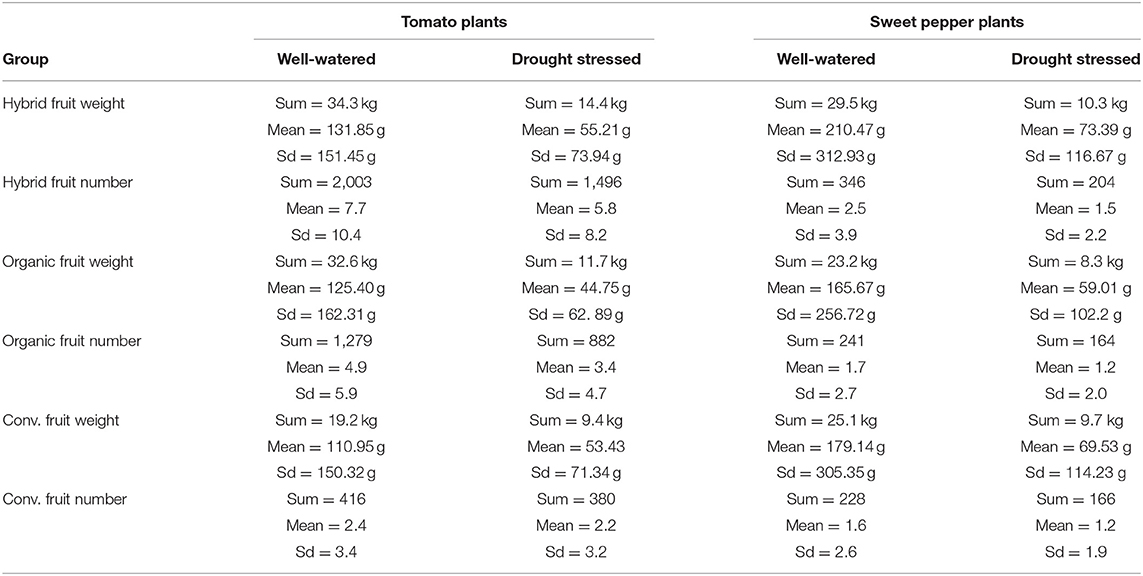
Table 3. Total sum, mean, and standard deviation (sd) of fruit weight and fruit number over the whole harvesting period (N = 15 harvest events) per hybrid, organic, and conventional = (Conv.) group under well-watered conditions and drought stress.
Overall, the hybrid group produced with a total of 3,499 fruits significantly more fruits than the organic (2,161 fruits) or conventional (796 fruits) group, equally under well-watered conditions and drought stress (Table 2). Within all three groups, fruit number and fruit weight were positively correlated. The correlation was highest within the conventional group (r = 0.92, p < 0.001). The coefficients of variation showed no difference in the stability of fruit weight and fruit number between the hybrid, organic, or conventional group.
Quality Performance–Yield Quality
Of all 6,456 harvested fruits, 62% (4,024 fruits) were categorized as marketable for the retail sector, fulfilling the quality requirements for commercial class extra and one. The remaining 2,432 fruits (38%) were categorized here as non-marketable (commercial class two, for further processing, or minus, completely non-marketable).
Drought stress had no negative impact on the number of marketable fruits produced per group. However, the number of non-marketable fruits was lower in plants under drought stress (p = 0.017, Table 4). Within-group comparisons showed that the hybrid group produced 63% marketable and 37% non-marketable fruits and the organic group 66% marketable and 34% non-marketable fruits. The conventional group differed noticeable from the other two groups with only 49% marketable and 51% non-marketable fruits. Since the hybrid group produced significantly more fruits in general, this is also reflected in the higher share of marketable and non-marketable fruits in comparison to the organic (p = 0.025 for marketable fruits, p = 0.001 for non-marketable fruits, Table 4) or conventional group (p < 0.001 for marketable and non-marketable fruits, Table 4).
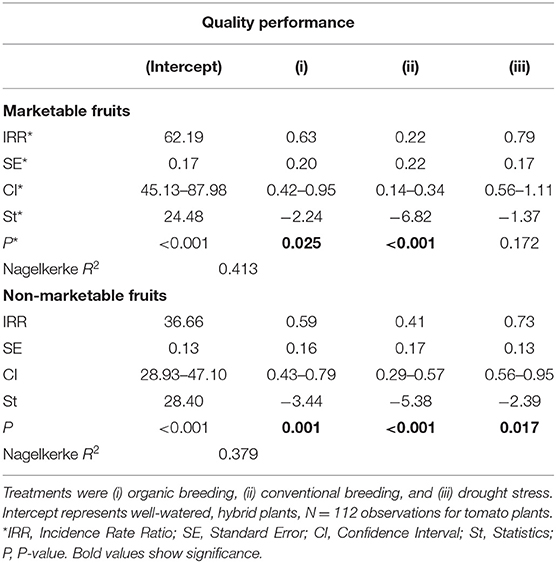
Table 4. Factors influencing on quality performance via the number of marketable and non-marketable fruits for tomato plants.
Sweet Pepper Results
Agronomic Performance–Yield and Yield Stability
Sweet pepper plants under well-watered conditions (N = 60 plants) produced a total of 78 kg fresh sweet peppers, and plants under drought stress (N = 60 plants) produced a total of 28 kg sweet peppers (see Table 3). The “GlmmTMB” model showed that drought stress resulted in significantly lower fruit weight for all three groups (p < 0.001, Table 5, see Figure 3B). The loss rate under drought stress for fruit weight was with minus 65% for the hybrid, minus 64% for the organic, and minus 61% for the conventional group similar in all three groups. Drought stress also lowered the number of fruits compared to well-watered plants (p < 0.001, Table 5). Comparing the three groups in terms of fruit number shows a loss rate of minus 41% for the hybrid group, minus 32% for the organic, and minus 27% for the conventional group (Table 3).
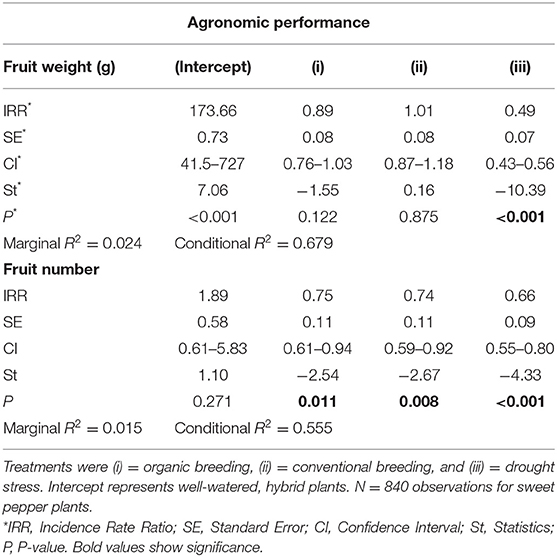
Table 5. Factors influencing agronomic performance via fruit weight and fruit number of sweet pepper plants as dependent variables.
Overall, the hybrid group produced with a total of 550 fruits significantly more fruits than the organic (405 fruits) or conventional (394 fruits) group, equally under well-watered conditions and drought stress (Table 5). For all three groups fruit number and fruit weight were positively correlated (R = 0.97–0.99, p < 0.001). Testing for the equality of coefficients of variation showed no difference in the stability of fruit weight and fruit number between the hybrid, organic, and conventional group.
Quality Performance–Yield Quality
From all 1,349 harvested peppers, 63% (853 fruits) were categorized as marketable for the retail sector, fulfilling the quality requirements for commercial class extra and one. The remaining 496 fruits (37%) were classified as non-marketable (commercial class two, for further processing, or minus, completely non-marketable). Drought stress lowered both the amount of marketable and non-marketable fruits to an equal extend (p < 0.001, Table 6). The hybrid group produced significantly more marketable fruits than the organic (p = 0.006, Table 6) or conventional group (p = 0.001, Table 6). The proportional distribution (in %) within the individual group reveals that the hybrid group produced the highest proportion of marketable fruits with 68 and 32% non-marketable fruits. The organic group produced 63% marketable and 37% non-marketable fruits. Comparable to the tomato results, the distribution was worst within the conventional group with 57% marketable and 43% non-marketable fruits.
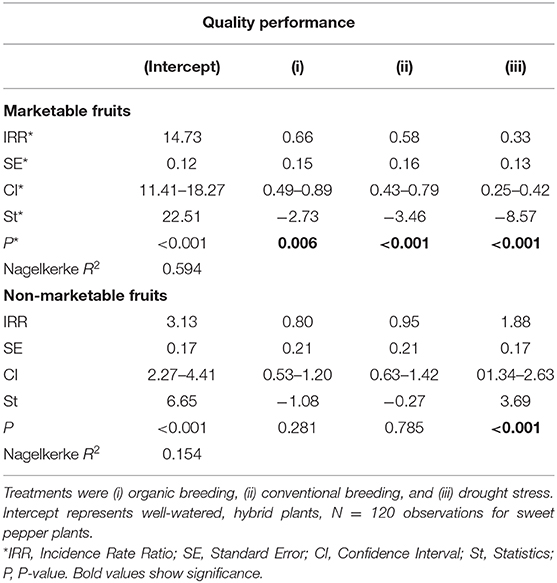
Table 6. Factors influencing on quality performance via the number of marketable and non-marketable fruits for sweet pepper plants.
Discussion
In this study we expected that drought stress generally lowers the agronomic and quality performance of all plants. We tested whether the organic tomato and sweet pepper group outperforms the conventional and hybrid group under drought stress, whereas hybrid plants were supposed to deliver highest yield and fruit quality under well-watered conditions. We show that the assumed benefits of the hybrid group can also be delivered by the organic and conventional group, emphasizing the value of securing the organic and conventional diversity in vegetable varieties in changing environmental conditions.
Equivalent Negative Effect of Drought Stress on Organic, Conventional, and Hybrid Varieties
We confirm the negative effect of drought stress on the agronomic and quality performance of all plants, regardless of the breeding background. In line with our results from the drought stress treatment, pepper is known to be highly at risk to limited water supply due to the large leaf surface and high stomata conductance (Alvino et al., 1994). In Nigeria, for e.g., exporting sweet pepper used to be highly important for farmers' incomes in the 1980s. However, due to rainfall limitations, sweet pepper yield in Nigeria decreased in recent decades up to 40% (Showemimo and Olarewaju, 2007). Mardani et al. (2017) tested the effect of different deficit irrigation levels (80, 60, 40%) and demonstrated a water supply below 80% of the optimum significantly reduced sweet pepper yield (fresh mass of fruit per plant) compared to fully irrigated treatment. Similar to our experimental drought stress, highest yield reduction was obtained when drought stress occurred continuously over the whole plant development phase until the time of harvesting (Mardani et al., 2017). Previous studies have also shown the negative effects of drought stress (combined with high air temperatures) on tomato fruit yield (Wahb-Allah et al., 2011; Cui et al., 2020). Santa Rosa et al. (2019) tested tomato hybrids against open-pollinated varieties under organic conditions and showed that hybrids in their study were more productive and resistant to biotic and abiotic stresses. They highlighted that for low-input systems the farmers' choice should not be between hybrids or open-pollinated varieties, but rather the most appropriate choice for the local growing conditions. Furthermore, hybrids which are more productive and resistant can thereby increase the economic output of organic tomato cultivation (Santa Rosa et al., 2019).
Organic and Conventional Varieties Deliver Similar Fruit Weight as Hybrids
The fact that hybrid varieties show a better agronomic and quality performance under optimal cultivation conditions was clearly proven in previous studies and is also shown under the well-watered conditions in our experiment. Similar to our results, Dowker and Gordon (1983) revealed that onion hybrids outperform commercial open-pollinated varieties by 14 to 67%. The same was demonstrated by Wehner (1999) for carrots, showing higher fruit quality for hybrids, leading to 25–30% higher marketable yield over open-pollinated varieties. Hybrid broccoli varieties over-yielded open-pollinated varieties by 40–90%, also pushed by the greater quality characteristics of the hybrids (Wehner, 1999). Also, tomato species are known to have a high hybrid potential, improving early ripening, uniformity, resistance against pest and diseases, as well as total yield (Cheema and Dhaliwal, 2005). However, comparing the total fruit weight of the hybrid group with the organic group in this study revealed that the organic group only produced 9% (4 kg) less total fruit weight. In relation to the total harvest period and the total harvested qualities, a plus of 9% from the hybrid group can be considered marginal. Moreover, since the effect of drought stress and the proportionate distribution of marketable and non-marketable fruits within each group was similar, we did not find that any of the three groups was better adapted to drought stress.
Differences became clear in the total number of fruits, as the hybrid group produced 38% more fruits than the organic group. Our findings showed a high market potential of the tested tomato hybrids. However, since fruit weight was similar, it means that the organic group produced bigger fruits compared to the hybrid group. Thus, the conventional tomato group did not offer an satisfactory alternative to the hybrid or organic group since it included varieties that were either highly susceptible to diseases or produced fruits with a high tendency to crack and burst.
Combining our results with previous findings from e.g., Santa Rosa et al. (2019), we highlight that increasing the diversity of crop varieties for vegetables supports resilient and sustainable vegetable cultivation. The greater the supply of genetic diversity in the form of different varieties, the greater the opportunities for farmers to adapt to their local climatic and environmental conditions. From an agro-ecological point of view, certain varieties (whether hybrid, conventional, or organic) should not be rated better or worse per se, but rather the overall supply of genetic diversity should be seen as an opportunity to simultaneously enlarge agrobiodiversity and maintain high agronomic and quality performance of a cultivation systems. However, vegetable farmers worldwide increasingly rely on high-yielding and hybrid varieties because of their typically higher provisioning services such as crop yield, and sometimes, also due to lack of variety alternatives (Lammerts van Bueren and Struik, 2004). This is because many seeds of local, traditional and organic varieties are often not available or not in sufficient quantities, despite their potential of adaptation to sub-optimal growing conditions.
Tomato Variety Conservation in Europe
In Europe, countries of the Mediterranean region have recently begun to promote and test local tomato varieties, including landraces in order to protect and increase genetic diversity with tomato varieties selected under severe drought conditions of the Mediterranean summer (Conti et al., 2019; Fullana-Pericàs et al., 2019). Fullana-Pericàs et al. (2019) tested 165 tomato genotypes from the Mediterranean basin and other diverse locations around the world, including landraces and modern varieties under well-watered and water-limited conditions. Their results highlighted that several landraces performed similarly or even better compared to modern varieties in terms of minimizing yield reduction under drought stress (Fullana-Pericàs et al., 2019). Italy promotes the protection and recovery of local tomato varieties on the national level (Conti et al., 2019). Analyses of the response of Italian varieties to drought stress indicated that locally, hot summer adapted varieties can include a “genotype-dependent response” to drought (Conti et al., 2019).
Techawongstien et al. (1992) found that pepper varieties from different sweet pepper types responded differently to drought stress. Drought tolerance was higher in genotypes of high leaf water potential, leading to early recovery of the corresponding “photosynthetic ability upon rehydration” and lower negative effect on final fruit yield (Techawongstien et al., 1992). Besides the studies of Santa Rosa et al. (2019) and Fullana-Pericàs et al. (2019) for tomato varieties and Techawongstien et al. (1992) for sweet pepper varieties, the number of previous studies comparing vegetable varieties from different breeding regimes under drought stress is low. Therefore, we are providing new information in an agronomically important field, the role of vegetable variety diversity under environmental stress like limited water supply.
Highest Seed Price for Hybrids—Balance Between Profitability and Equity
For both tomato and sweet pepper, the performance of the hybrid group compared to the organic and conventional group may be related to the total costs incurred in the breeding and cultivating process. Across all vegetable species, higher purchase prices for hybrid seeds are due to higher cost of variety development and seed production (Riggs, 1988). Also, in our study, retail price was highest for the hybrid tomato and sweet pepper seeds. Notably, there is no private property protection of organic seeds. These two factors, price and non-profit ownership, might justify slightly lower total yields of the organic group and emphasize the importance of variety alternatives for sustainable vegetable production. Thus, the inclusion of varieties without legal usage restrictions as a common pool resource on the market for seeds may contribute to the social-ecological balance between profitability and equity of different farming systems by increasing the sovereignty of the farmers (Kremen et al., 2012).
Conclusion
With our results we challenge the widely held assumption that conventional and organic varieties cannot be seen as equivalent alternatives to hybrids. Thus, these varieties should be considered as important for maintaining a diverse variety portfolio for local adaptation to unstable climate and water-supply conditions. Especially varieties of the organic group, which are not protected via private property rights, should be considered essential for the sustainability of vegetable production. A system, including all variety options will be the most efficient and equitable way to offer varieties for all kinds of farming and growing systems. Simultaneously, the use of open-pollinated varieties will expand genetic diversity in the vegetable breeding sector. In this sense, a wide range of varieties represents an important component of agrobiodiversity, and access to locally adapted varieties is and will be pivotal for crop production under expanding drought periods and further climate extremes.
Data Availability Statement
The raw data supporting the conclusions of this article will be made available by the authors, without undue reservation.
Author Contributions
AF, JL, and TT developed the methodology and the experimental design, wrote and edited the manuscript, and approved the final version of the manuscript. AF performed the experiment. All authors contributed to the article and approved the submitted version.
Funding
The Federal Ministry of Education and Research of Germany funded this research in the field of Research for Sustainable Development (Grant No. 01UU1602B).
Conflict of Interest
The authors declare that the research was conducted in the absence of any commercial or financial relationships that could be construed as a potential conflict of interest.
Acknowledgments
We thank the experimental botanical garden of the University of Göttingen and especially Regina Helbig and Gabi Kuchenbuch for knowledge and support cultivating the plants. Also, we are grateful to Julia Morley and Tristan Michaelis for assistance in cultivation and harvest. Furthermore, we are grateful for the collaboration and consultancy of the Agroecology Group and ZfS Statistical Consulting. We are grateful for the helpful comments of Johann Zaller and two reviewers. We acknowledge support by the Open Access Publication Funds of the Göttingen University.
Supplementary Material
The Supplementary Material for this article can be found online at: https://www.frontiersin.org/articles/10.3389/fsufs.2021.628537/full#supplementary-material
References
Alvino, A., Centritto, M., and De Lorenzi, F. (1994). Photosynthesis response of sunlit and shade pepper (Capsicum annuum) leaves at different positions in the canopy under two water regimes. Aust. J. Plant Physiol. 21, 377–391. doi: 10.1071/PP9940377
Brooks, M., Bolker, B., van Bentham, K., Maechler, M., Kristensen, K., Berg, C., et al (2019). Package “glmmTMB” Generalized Linear Mixed Models Using Template Model Builder. Available online at: https://cran.rproject.org/web/packages/glmmTMB/glmmTMB.pdf (accessed November 4, 2020).
Cheema, D. S., and Dhaliwal, M. S. (2005). Hybrid tomato breeding. J. New Seeds 6, 1–14. doi: 10.1300/J153v06n02_01
Commission Implementing Regulation (EU) No 543/2011 (2011). Marketing Standard for Tomatoes. Available online at: https://eurlex.europa.eu/LexUriServ/LexUriServ.do?uri=OJ:L:2011:157:0001:0163:EN:PDF (accessed November 4, 2020).
Conti, V., Mareri, L., Faleri, C., Nepi, M., Romi, M., Cai, G., et al. (2019). Drought stress affects the response of italian local tomato (Solanum lycopersicum L.) varieties in a genotype-dependent manner. Plants 8:E336. doi: 10.3390/plants8090336
Cui, J., Shao, G., Lu, J., Keabetswe, L., and Hoogenboom, G. (2020). Yield, quality and drought sensitivity of tomato to water deficit during different growth stages. Sci. agric. 77:e20180390. doi: 10.1590/1678-992x-2018-0390
Da Silva Dias, J. C. (2010). Impact of improved vegetable cultivars in overcoming food insecurity. Euphytica 176, 125–136. doi: 10.1007/s10681-010-0237-5
Department of Agriculture Cooperation Ministry of Agriculture Government of India. (2012). Checklist of Commercial Varieties of Vegetables. Available online at: http://shm.uk.gov.in/files/Books/Check_List_of_Commercial_Varieties_of_Vegetables_(pdf_8.22_MB).pdf (accessed November 4, 2020).
Dowker, B. D., and Gordon, G. H. (1983). “Heterosis and hybrid cultivars in onions,” in Heterosis, Reappraisal of Therory and Practice. Monographs on Theretical and Applied Genetic, ed R. Frankel (Heidelberg: Springer-Verlag), 220–231. doi: 10.1007/978-3-642-81977-3_8
FAO (2018). Agrobiodiversity. A Training Manual for Farmer Groups in East Africa. FAO. Available online at: http://www.fao.org/3/I9307EN/i9307en.pdf (accessed November 4, 2020).
Ficiciyan, A., Loos, J., Sievers-Glotzbach, S., and Tscharntke, T. (2018). More than yield: ecosystem services of traditional versus modern crop varieties revisited. Sustainability 10:2834. doi: 10.3390/su10082834
Fullana-Pericàs, M., Conesa, M., Douthe, C., Aou-Ouad, H., Ribas-Carbo, M., and Galmés, J. (2019). Tomato landraces as a source to minimize yield losses and improve fruit quality under water deficit conditions. Agr. Water Manage. 223:105722. doi: 10.1016/j.agwat.2019.105722
Gmeiner, N., Kliem, L., Ficiciyan, A., Sievers-Glotzbach, S., and Tschersich, J. (2018). “Gemeingüterbasierte rechte an saatgut und sorten als treiber für eine sozial-ökologische transformation des pflanzenbaus,” in Bundesamt für Naturschutz (Hrsg.), Treffpunkt Biologische Vielfalt, Interdisziplinärer Forschungsaustausch im Rahmen des Übereinkommens Über Die Biologische Vielfalt (Bonn: BfN Skripten), 487.
Hartig, F. (2019). Package “DHARMa” Residual Diagnostics for Hierarchial (Multi Level/Mixed) Regression Models. Regensburg: Theoretical Ecology, University of Regensburg. Available online at: https://cran.r-project.org/web/packages/DHARMa/vignettes/DHARMa.html (accessed November 4, 2020).
Kahiluoto, H., Kaseva, J., Balek, J., Olesen, J. E., Ruiz-Ramos, M., Gobin, A., et al. (2019). Decline in climate resilience of european wheat. PNAS 116, 123–128. doi: 10.1073/pnas.1804387115
Kotschi, J., and Wirz, J. (2015). Wer Zahlt Für Das Saatgut? Gedanken zur Finanzierung Ökologischer Pflanzenzüchtung. Arbeitspapier. Marburg and Dornach: AGRECOL und 1097 Sektion für Landwirtschaft.
Kremen, C., Iles, A., and Bacon, C. (2012). Diversified farming systems: an agroecological, system-based alternative to modern industrial agriculture. Ecol. Soc. 17:44. doi: 10.5751/ES-05103-170444
Kumar, R., Solankey, S. S., and Singh, M. S.S. (2012). Breeding for drought tolerance in vegetables. Veg. Sci. 39, 1–15.
Lammerts van Bueren, E. T., and Struik, P. C. (2004). The consequences of the concept of naturalness for organic plant breeding and propagation. Wageningen J. Life Sci. 52, 85–95. doi: 10.1016/S1573-5214(04)80031-9
Lenth, R., Singmann, H., Love, J., Buerkner, P., and Herve, M. (2019). Package “Emmeans”. R topics documented, 216–221.
Mardani, S., Tabatabaei, S. H., Pessarakli, M., and Zareabyaneh, H. (2017). Physiological responses of pepper plant (Capsicum annuum L.) to drought stress. J. Plant Nutr. 40, 1453–1464. doi: 10.1080/01904167.2016.1269342
Marwick, B., and Krishnamoorthy, K. (2019). cvequality: Test for the Equality of Coefficients of Variation From Multiple Groups. R software package version 0.1.3.
MEA (2005). Millennium Ecosystem Assessment: Synthesis. Ecosystems and Human Well-Being. Washington, DC: Island Press.
Messmer, M., Wilbois, K.-P, Baier, C., Schaefer, F., Arncken, C., Drexler, D., et al (2015). Plant Breeding Techniques: An Assessment for Organic Farming. FiBL–Research Institute of Organic Agriculture, Crop Production. Available onlinw at: https://shop.fibl.org/CHen/mwdownloads/download/link/id/155/?ref=1 (accessed November 4, 2020).
Nemeskéri, E., and Helyes, L. (2019). Physiological responses of selected vegetable crop species to water stress. Agronomy 9:447. doi: 10.3390/agronomy9080447
R Core Team (2019). R: A Language and Environment for Statistical Computing. Vienna: R Foundation for Statistical Computing. Available online at: http://www.R-project.org/ (accessed November 4, 2020).
Riggs, T. J. (1988). Breeding F1 hybrid varieties of vegetables. J. Horti. Sci. 63, 369–382. doi: 10.1080/14620316.1988.11515871
Rodríguez, G. (2013). Models for Count Data With Overdispersion. Available online at: https://data.princeton.edu/wws509/notes/c4addendum.pdf (accessed November 4, 2020).
Rossi, R. (2019). The EU Fruit and Vegetable Sector: Main Features, Challenges and Prospects. European Parliamentary Research Service. Available online at: http://www.europarl.europa.eu/RegData/etudes/BRIE/2019/635563/EPRS_BRI(2019)635563_EN.pdf (accessed November 4, 2020).
Santa Rosa, A. J., Sala, F. C., and Cardoso, J. C. (2019). Performance and selection of tomato cultivars for organic cultivation in greenhouse. Rev. Ceres. 66, 94–101. doi: 10.1590/0034-737x201966020003
Schwarz, D., Thompson, A., and Kläring, H.-P. (2014). Guidelines to use tomato in experiments with a controlled environment. Front. Plant Sci. 5:625. doi: 10.3389/fpls.2014.00625
Showemimo, F. A., and Olarewaju, J. D. (2007). Drought tolerance indices in sweet pepper (Capsicum annuum L.). Int. J. Plant Breed. Genet. 1, 29–33. doi: 10.3923/ijpbg.2007.29.33
Suguru, S., Moreshet, S., Takagaki, M., Shinohara, Y., and Ito, T. (2003). Effects of drought stress on sap flow, stomatal conductance, leaf water potential of pepper cultivars (Capsicum annuum L.). Jpn. J. Trop. Agri. 47, 61–69. doi: 10.11248/jsta1957.47.61
Techawongstien, S., Nawata, E., and Shigenaga, S. (1992). After-effect of short-term water stress at the pre-anthesis stage on physiological characteristics in four chilli pepper cultivars. Jpn. J. Trop. Agr. 36, 88–93.
Verbraucherschutz (2018). Verordnung Über Den Verkehr Mit Saatgut Landwirtschaftlicher Arten und Von Gemüsearten (Saatgutverordnung). Available online at: https://www.gesetze-im-internet.de/saatv/SaatV.pdf (accessed November 4, 2020).
Wahb-Allah, M. A., Alsadon, A., and Ibrahim, A. A. (2011). Drought tolerance of several tomato genotypes under greenhouse conditions. World Appl. Sci. J. 15, 933–940.
Keywords: agrobiodiversity, drought stress, genetic diversity, seed commons, sustainable vegetable production, variety comparison, vegetable breeding
Citation: Ficiciyan AM, Loos J and Tscharntke T (2021) Similar Yield Benefits of Hybrid, Conventional, and Organic Tomato and Sweet Pepper Varieties Under Well-Watered and Drought-Stressed Conditions. Front. Sustain. Food Syst. 5:628537. doi: 10.3389/fsufs.2021.628537
Received: 12 November 2020; Accepted: 27 January 2021;
Published: 19 February 2021.
Edited by:
Johann G. Zaller, University of Natural Resources and Life Sciences Vienna, AustriaReviewed by:
Rong Zhou, Aarhus University, DenmarkSara Sestili, Council for Agricultural and Economics Research (CREA), Italy
Copyright © 2021 Ficiciyan, Loos and Tscharntke. This is an open-access article distributed under the terms of the Creative Commons Attribution License (CC BY). The use, distribution or reproduction in other forums is permitted, provided the original author(s) and the copyright owner(s) are credited and that the original publication in this journal is cited, in accordance with accepted academic practice. No use, distribution or reproduction is permitted which does not comply with these terms.
*Correspondence: Anoush Miriam Ficiciyan, YW5vdXNoLmZpY2ljaXlhbkB1bmktZ29ldHRpbmdlbi5kZQ==