- 1Department of Food Science and Nutrition and Technology, University of Nairobi, Nairobi, Kenya
- 2Department of Plant Science and Crop protection, University of Nairobi, Nairobi, Kenya
Cassava roots, millet and cowpea leaves have short storage life thus the need for simple post-harvest handling and storage protocol to ensure prolonged availability to fully contribute toward food and nutrition security, a major challenge within Sub-Saharan Africa. The current study sought to investigate the effect of pre-treatment and processing on cyanide safety and nutrition composition of cassava roots, millet and cowpea leaves flours. The study used three popular cassava varieties grown along the Kenyan coast, cowpea leaves (M66) grown as vegetable and pearl millet. The study used analytical techniques as guided by AOAC standard methods, to determine the nutritional composition of the individual crops while subjecting them to pre-treatment processes (blanching, peeling, washing, drying, and fermentation) and optimizing for maximum nutrient composition. The cyanide content ranged 7.8–9.5, 3.4–5.0, and 2.2–2.8 ppb for raw, untreated, and fermented cassava flours, respectively. The carbohydrates content was in the range of 35–37, 81.73–83.49, and 70.28–71.20% for raw cowpea leaves, cassava roots, and millet, respectively; the carbohydrate content for untreated flours was in the range of 35.68–35.19, 66.07–83.49, and 66.07–68.89% for cowpea leaves, cassava roots, and millet, respectively; the carbohydrate content for the fermented flours was in the range of 29.06–28.01, 79.68–84.36, and 69.08–70.12% for cowpea leaves, cassava roots, and millet, respectively. The protein content was in the range of 25.69–26.01, 1.2–18, and 11.1–13.3% for untreated cowpea, cassava, and millet flours, respectively; fermented flours protein content was in the range of 25.7–29.3, 1.3–2.2, and 8.5–11.1% cowpea, cassava, and millet flours, respectively. Iron and zinc contents were in the range of 4.31–9.04, 1.0–1.3; 7.98–7.89, 1.21–1.25; 6.58–8.23, 0.99–1.22 (mg/100 g dwb) for raw, untreated, and fermented cowpea flours, respectively. Pre-treatment had significant effects (P ≤ 0.05) on cyanide content and nutritional composition of each of the flours. Farmers should be trained to utilize such simple processing techniques.
Introduction
Cassava roots are the third most utilized source of starch after maize and rice (Wanapat and Kang, 2015). Globally, the crop's utilization is on the rise due to the divergent product lines which include cassava flour, dried chips, animal feed, and industrial starch (Abong et al., 2016). Following the trending global drive for promotion of growth and utilization of indigenous crops as a means of dietary diversification and ensuring food security amongst the low income communities especially in the developing and third world countries (IITA, 2016), the growth and utilization of cassava is currently promoted with the aim of ensuring sufficient supply of carbohydrates and increased living standards of the farmers (Abong et al., 2016).
The crop, which is drought resistant, has been associated with regions that are afflicted with malnutrition (Wanapat and Kang, 2015). The crop is a highly valuable food crop for persons living in such zones. The cassava roots have a deficit of sulfur-containing amino acids, and certain micronutrients are not optimally distributed. The promotion of the crop is hindered, especially among consumers who fear the high levels of cyanogenic glucosides associated with the roots (Olapade et al., 2014). Additionally, there is limited documentation of the effect of processing on the nutritional composition of the fresh cassava roots and the level of retention of nutrients. The present study sought to device simple and efficient processing techniques to reduce the levels of cyanogenic glucosides.
Cowpea leaves is one of the traditionally consumed crops for their high protein content (Okonya and Maass, 2014). The indigenous vegetables are grown in small scale farms and even in urban gardens (Owade et al., 2019). The leaves are a good source of protein and vitamins (Animasaun et al., 2015). The leaves can be consumed boiled, fried or dried or fermented to increase their utilization, a practice that has gained a rising trend following an increased demand for nutritious foods (Gonçalves et al., 2016). Millet crop can survive under a varied range of soils and often does well even in areas of minimal rainfall and extreme temperatures (Chouhan et al., 2015). The crop is a good source of minerals especially the limiting zinc and iron (Sharma et al., 2016) which are needed especially by children of under 59 months and expectant women. Millet is often milled into flour for consumption. To increase the availability of the nutrients of the millet flour fermentation has primarily been used as a processing technique (Fayemi and Ojokoh, 2014). Cassava is deficient in minerals and vitamins; however, these are found in recommendable levels in cowpea leaves and millet. Cowpea leaves and cassava crops have short post-harvest life thus the need to subject the crops to pre-treatment processes while optimizing for maximum nutrient retention.
The present study sought to investigate the effect of pre-treatment and processing on cyanide safety and nutrition composition of cassava roots, millet, and cowpea leaves flours.
Materials and Methods
Acquisition of Raw Materials
The current study utilized three popular cassava varieties (Kibandameno, Tajirika, and Kaleso) identified by farmers during focus group discussions done in April 2018. The sampling procedure targeted farmers with preferred varieties through a systematic sampling procedure. For each cassava variety three kilograms of fresh roots were picked from 3 farms in each sub county. For each county, sampling was done in 2 sub-counties that grew the preferred varieties. The sampled fresh roots were then stored in cooler boxes and transported overnight to the University of Nairobi Food Chemistry Laboratory for immediate analysis. Pearl millet, a cassava intercrop at the Kenyan coast was readily available at the local markets. Pearl millet grains were purchased in three different markets (Kongowea, Voi, and Taveta) and taken to the laboratory for analysis. Cowpea leaves were grown at the University of Nairobi field station following standard agronomic practices and harvested at varied times from weeks 2 to 10 after planting to optimize for protein levels. The leaves were sampled on a weekly basis and taken to the laboratory for immediate analysis.
Sample Preparation
Cassava Roots Preparation
The root samples were washed to remove any soils on the surface. The samples that were used to determine moisture content on wet weight basis were never washed but peeled immediately. The washed cassava samples were then peeled and sliced into chips of size 30 mm. The cassava chips were then oven dried at 60°C for 24 h. The dried chips were then milled into flour.
Preparation of Cowpea Leaves
Cowpea leaves were washed to remove any soil particles and sliced to ~3 mm. The samples were then blanched, and oven dried at 45°C for 24 h. The dried samples were then blended into fine powder for analysis.
Preparation of Millet
The dry pearl millet samples were sorted to remove chaffs, the millet grains were then milled into flour. The pearl millet flour was then subjected to proximate analysis and eventually used to formulate composite flours.
Fermentation of Cassava Roots, Cowpea Leaves, and Millet
The varied composite flours were traditionally fermented by adding water to the flours in the ratio of 3:2 (water:flour) to form a slurry and leaving to ferment at ambient temperature for 2 days as per focus group discussion. The fermented flours were then dried and packaged. Cowpea leaves were fermented through sauerkraut technology.
Analytical Methods
Determination of Moisture Content in an Air Oven
The moisture content of the raw samples and different flours were determined as per AOAC (2016) method number 934.01. About 5 g of each sample was weighed into a dish, the dish and its contents were then put in an air oven maintained at 105°C and left to dry for 4 h. The samples were then cooled in a desiccator and weighed. The dishes were then returned to the oven and dried until the moisture content variation was within 0.05%. The moisture content of the sample was then calculated as a percentage of the sample weight.
The moisture content of the raw cassava samples and different flours were determined as per AOAC (2016) method number 934.01. About 5 g of each of sample was taken and oven dried at 80°C for a period of 4 h. The dried samples were later re-weighed and the percentage weight loss determined. The percentage weight loss was expressed as the percentage moisture content of the respective flours.
Determination of Ash Content
Ash content was determined as per AOAC (2016) method number 923.03. About 2 g of each sample was weighed into a tared porcelain crucible; and ashing started with low burning Bunsen burner flame. The ashing process was then continued in a muffle oven at 500°C until a light gray ash of constant weight was obtained. The ash content of the sample was then calculated and expressed as a percentage of the sample weight.
Determination of Crude Protein Content
Crude protein content was determined as per AOAC (2016) method number 992.15. Approximately 0.5 g of each sample was put in a nitrogen-free filter paper, folded carefully, and placed in a Kjedahl flask together with anti-burning pumice, 1 Kjedahl catalyst tablet and concentrated sulphuric acid was added and the mixture heated slowly in a fume cupboard until a clear solution was obtained. The boiling continued for 1 h. The mixture was then cooled and a few drops of phenolphthalein added. A 400 ml conical flask containing 50 ml of 0.1N HCl solution with some added methyl orange indicator, was placed under the outlet of the distillation unit. Just enough 40% NaOH solution was added to change the color of the solution and the mixture distilled until a drop of distillate did not react with Nessler's reagent placed in a test-tube. Back titration was then done with 0.1N NaOH solution and the crude protein content of the sample calculated as a percentage of the sample weight.
Determination of Crude Fiber Content
Crude fiber content was determined according to AOAC (2016) method number 985.29. Approximately 2 g of the flours were weighed into a graduated 600 ml beaker and a small amount of boiling water and 25 ml of 2.04N sulphuric acid solution added. The volume of the solution was topped to 200 ml and maintained while boiling for 30 min. The contents of the beaker were then filtered using a Buchner funnel slightly packed with glass wool and the residue washed. The residue together with the glass wool were then transferred into the beaker and a small amount of distilled water and 25 ml of 1.73N KOH solution added, the volume was topped to 200 ml with boiling distilled water and heated for 30 min. The solution was again filtered using a glass wool. The residue was transferred quantitatively into a porcelain dish and dried in an air oven set at 105°C for 2 h. The desiccator was then cooled and weighed. The dish contents were then ignited at 550°C before cooling and weighing. The crude fiber content of the sample was then calculated as percentage of the sample weight.
Determination of Crude Fat Content
Crude fat content was determined as per AOAC (2016) method number 920.39. About 5 g of the flours were taken into an extraction thimble and covered with a cotton wool and placed in a soxhlet extractor. The tared flat bottomed flask with 200 ml of petroleum ether was then placed on a heating mantle and connected to the soxhlet extractor and the extraction let to run for 8 h. The solvent was then evaporated in a rotary evaporator, the residue dried in an air oven set at 105°C for 1 h and the crude fat content expressed as a percentage of the sample weight.
Determination of Carbohydrates Content
Carbohydrates content was determined as per AOAC (2016) by the difference, i.e., 100% minus the total sum of fat, moisture content, crude fiber, Ash, and proteins in 100 g of food.
Determination of Iron and Zinc Contents
The mineral content was determined as per AOAC (2016) by method number 985.27 for both zinc and iron. The mineral contents were read in an Atomic Absorption Spectrophotometer (buck Scientific 210 VGP, USA).
Determination of Cyanide Content in Cassava Roots
The cyanide content of the cassava roots was determined by distillation method as per AOAC (2016) raw cassava roots were peeled, washed, and dried. Fermented and milled samples were also evaluated. Ten grams of the samples were weighed and soaked in distilled water for 2 h, and then subjected to the distillation process. Using 25 ml of 2.5% NaOH solution and collected to 200 ml. The solution was then titrated with 0.02N silver Nitrate to a faint blue color and the cyanide content calculated as parts per billion (ppb).
Determination of the Contents of Vitamins A and C
Approximately 2 g of each sample was extracted and stabilized with 25 ml of TCA (trichloro-acetic acid) then titrated using 0.001 N N-Bromosuciinamide and starch as indicator. Titer volume of N-Bromosuciinamide was then used in calculation to determine vitamin C content using the formula:
V*C*(176/178)*100/weight of the sample = mg/100 g of Vitamin C, where V is titer volume, and C is Bromosuciinamide concentration (Abok et al., 2016).
Determination of Vitamin A
Approximately 2 grams of the samples were weighed and poured into a pestle. Using a motor and pestle color was extracted by adding small portions of acetone until the residual was colorless. The extracts were then all poured into a 100 ml volumetric flask. The contents were then evaporated in a rotary evaporator at about 60°C. To the evaporated sample, 1 ml of petroleum Spirit was added to dissolve the B-carotene. Through a packed column, B-Carotene was eluted. Depending on the sample, collect the orange or yellow pigment into a 25 ml volumetric flask. The absorbance was then read at 450 nm. The level of vitamin A was then calculated from the B-Carotene Standard curve.
Blanching
The fresh cowpea leaves were divided into two batches. One of the batches was blanched using steam at 95°C for 5 min using the pilot plant blancher at the Department of Food Science, Nutrition and Technology, University of Nairobi. The blanched leaves were then dried to remove free water before being fermented.
Energy Content
The energy content of the food samples in wet weight sample was determined using the conversion formula as described by FAO (2003) in a final energy conversion report. The formula entailed a conversion rate of 4 kcal g−1 for protein and carbohydrates and 9 kcal/g for fats.
Data Analysis
The data were analyzed using Statistical Package for Social Sciences (SPSS) software version 21 for the analysis of variance (ANOVA) and the means were separated by the least significant difference at P ≤ 0.05.
Results and Discussion
The Effect of Pre-treatment Processing on the Cyanide Content of Three Popular Cassava Varieties
The effect of pre-treatment and processing on cyanide content of cassava roots and flours is illustrated in Figure 1. The cyanide content of the cassava roots was significantly different (p < 0.05) among varieties and between pre-treatment processes. For the raw products, Kaleso had significantly higher (P < 0.05) amounts of cyanide (9.65 ppb). After drying and milling, Tajirika had significantly (P < 0.05) higher amounts (5.0 ppb) of cyanide. The flours were subjected to fermentation process and after fermentation Tajirika had significantly higher (P < 0.05) amounts (2.8 ppb) of cyanide compared to Kaleso (2.2 ppb) and Kibandameno (2.3 ppb). Peeling, drying, and milling of cassava roots reduces the cyanide contents by more than 45% (Figure 1). Kaleso had the highest cyanide content reduction of 64% while Tajirika had a loss of 46%. Peeling, drying and fermentation reduced the cyanide content in cassava flours by more than 70% (Figure 1). Fermentation reduces the cyanide content of cassava roots by ~35% (Figure 1).
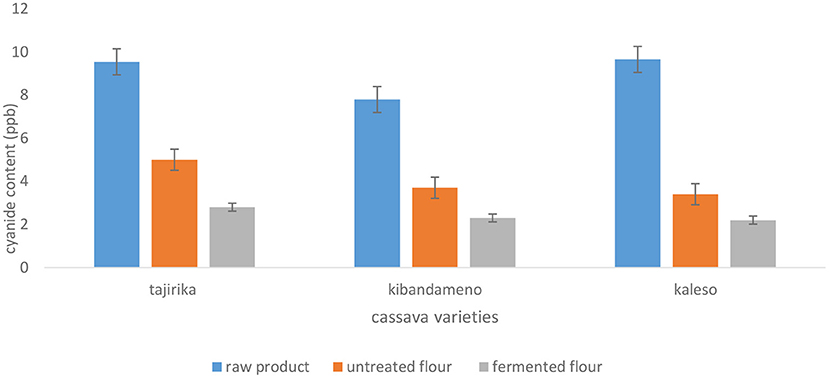
Figure 1. Effect of pre-treatment processes on the cyanide content of cassava roots. The bars are standard error bars.
The cyanide contents of cassava roots and flours reported in the current study (Figure 1) are significantly (P ≤ 0.05) below the maximum tolerated levels in consumable foods of 10 ppb. The highest cyanide content recorded in this study was in Kaleso (9.65 ppb) which is below the standards set by the World Health Organization (WHO) and regional organizations such as East African community, Kenya Bureau of Standards (KEBS) at 10 mg kg−1 (Abong et al., 2016). The findings in this study are in agreement with the study done by Guédé (2014) who recorded the cyanide content in fresh roots to be below 10 ppb. Tajirika, an improved high yielding cassava variety, preferred for high starch content and high yield per plant had cyanide levels of 9.54 ppb. The yield for this variety averages as high as 41 kg per plant.
Drying of cassava roots reduces the cyanide content by about 50%. The cyanide content for Tajirika, Kibandameno, and Kaleso reduced from 9.54, 7.79, and 9.65 ppb for fresh samples to 5, 3.7, and 3.4 ppb for dried samples respectively. For fermented and dried samples, the cyanide contents reported in the current study were 2.8, 2.3, and 2.2 ppb for Tajirika, Kibandameno, and Kaleso, respectively. Peeling, washing, drying, and fermentation reduces cyanide content by about 70%. The findings in this study are in agreement with the findings of Ismaila et al. (2018) who reported that fermentation reduces cyanide contents by more than 60%. The effect of processing on the cyanide content of cassava roots reported in this study are in agreement with Ismaila et al. (2018), Emmanuel et al. (2012), and Blanshard et al. (1994) who reported that a combination of processing procedures that involved fermentation significantly reduced the levels of cyanide contents to within 10 mg HCN per 100 g maximum tolerated level in consumable food. Processing of cassava roots into flours significantly reduces the cyanide content Muoki et al. (2015). Cyanide is one of the key toxicants in cassava roots and it occurs in both Sweet and bitter cassava varieties and acute cyanide toxicity can lead to death if not treated and thus the need to reduce the cyanide contents Gacheru et al. (2015).
The Effect of Pre-treatment Processes on the Nutritional Composition of Cowpea Leaves, Millet, and Cassava Roots
Drying as a pre-treatment process has a significant effect on the moisture content of cassava roots, millet and cowpea leaves (Table 1). The moisture content of the cassava roots significantly dropped from an average of 57.63% to an average of 8.08%. The moisture content of cowpea leaves was significantly reduced from moisture content 83.6% to an average of 8.37%. Drying involves removal of water from the food samples. A reduction in the water content of the food samples reduces the water activity of the food and thus a significant reduction in the growth of micro-organisms and thus inhibiting food spoilage due to microbial spoilage. The findings in the current study are in agreement with the findings of Emmanuel et al. (2012) that reported the moisture content to range from 6.96 to 9.66%. The moisture levels reported in the study showed no significant variation between the moisture content of the improved varieties (Kaleso and Tajirika) and the local cassava variety (Kibandameno). For better storage quality of flours, the moisture content should be below 12%, moisture content of above 12% would favor microbial growth and thus quick spoilage of the flours. The levels reported in the present study indicates that peeling, slicing, coupled with solar drying for 48 h, serves as an efficient method of lowering the water content of cassava roots. The dried flours would be used in formulation of blended composite flours. The values were slightly lower than levels reported by Shittu et al. (2007) who recorded an average of 11 and 16.5%, respectively (Emmanuel et al., 2012). The effect of drying on cowpea leaves reported in the current study agree with the findings of Gerrano et al. (2015) who reported that dying of cowpea leaves reduces significantly the moisture content of cowpea leaves. The nutritional composition of cassava roots depends on the age, the geographical region, the variety of the crops (FAOSTAT, 2011) and the part of the crop consumed. With the increased research on developing more nutritious varieties (the improved varieties), the nutritional value of the root tubers are expected to increase due to increased vitamin A (Abong et al., 2016).
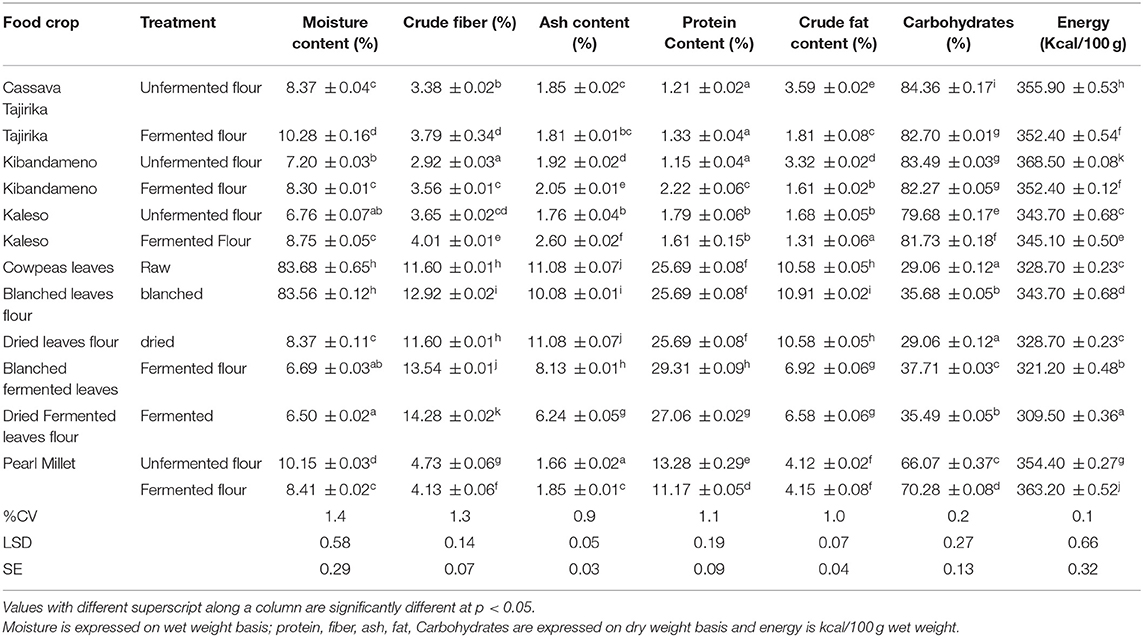
Table 1. Proximate composition of unfermented flours and fermented flours of cassava roots, cowpea leaves, and pearl millet.
Drying and fermentation significantly increases the crude fiber content of cassava roots, millet and cowpea leaves. The fiber content of cassava root was significantly increased from an average of 3.32–3.78%. The fiber contents of cowpea leaves was equally increased from 11.60 to 14.28%. The fiber content of millet was significantly reduced from 4.73 to 4.13%. Drying and fermentation concentrates the nutritional components of the foods. The protein content of cowpea leaves was significantly increased through drying and fermentation. Fermentation significantly increased the protein content from 25.69 to 29.31%. The levels reported in this study are within the range of the levels that were reported by Okonya and Maass (2014) of 28.02–31.84%. The nutritional composition reported by Animasaun et al. (2015) has a lower protein level of 23.42–26.78%, the levels reported in the present study however are relatively high, which can be attributed to the soils, variety of the crop and the age of harvesting. The present study optimized the use of cowpea leaves by pinching every week, the levels of protein were not significantly different between the plants harvested in the 2nd week to the 8th week as the protein levels averaged ~28%, however from week 9 the levels of protein significantly dropped to 26%. According the study done by Kulwa et al. (2015) one of the recommendations to ensure nutritional security is to improve on the feeding practices and nutrient content of complimentary meals through the incorporation of plant leaf protein in the protein deficient meals such as enriching cassava flour with both cowpea leaves and millet as source of protein and micronutrients such as iron and zinc and vitamins. The cowpea leaves had a significant level of protein (Salvador et al., 2014; Wanapat and Kang, 2015; Oresegun et al., 2016) all reported that the plant leaves could be a good source of protein in studies done on cassava leaves.
The findings of the current study are in agreement with a study conducted by Kumar et al. (2016) who reported the nutritional composition of 4 varieties of millet to range as follows: protein 6.8–12.5%, fiber 2.4–15.6%, fat 0.5–6.9%, energy 329–389 kcal/100 g wet weight, carbohydrates 60.9–72.6% and moisture 11.9–13.1%. The findings in the present study report levels of fat, protein, carbohydrates, moisture, energy, and fiber as 4.1%, 13.3%, 70.2%, 10.14%, 348.8 kcal/100 g wet weight, and 4.73% respectively. The protein levels reported are quite low as compared to the levels reported by Singh (2016) which ranged 7.3–12.5% and the protein levels reported by Kumar et al. (2016) who reported 6.8–12.5% on average from 4 millet varieties.
Fermentation increased significantly (P ≤ 0.05) the moisture content of the cassava varieties (Tajirika Kaleso and Kibandameno). The fiber content of the fermented products indicated a slight increase (Table 1). The protein content of fermented cassava roots, millet, and cowpea leaves increased in the present study. Wanapat and Kang (2015) and (Ismaila et al., 2018) reported that fermentation of the cassava roots increases the protein levels by increasing the bioavailability of proteins. Apart from millet, the energy contents of the samples decreased after fermentation. Processing of the fresh cassava roots, millet and cowpea leaves serves the key role of increasing the shelf-life of the roots. However, along with the processing, there is a significant drop in the energy (kcal/100 g), and this can be attributed to the significant drop of the levels of carbohydrates.
Effect of Pre-treatment Processes on Mineral and Vitamin Content of Cowpea Leaves, Millet, and Cassava Roots
Pre-treatment processing had significantly different effects on the mineral content of cowpea leaves, cassava roots and millet (Table 2). The iron content of cassava roots was significantly reduced from as high as 0.99 mg kg−1 to as low as 0.23 mg kg−1. Drying and fermentation reduced the iron content of cowpea leaves from 9.04 mg kg−1 to 6.58 mg kg−1. The iron content of millet was equally significantly reduced. Drying and fermentation had a significant different effect on the zinc content between the samples and between treatments. The zinc content of cassava roots was significantly increased by a combination of drying and fermentation (Table 2). The levels of iron reported are in agreement with the levels reported by Salvador et al. (2014) and Okonya and Maass (2014) on the protein and iron levels of cowpea leaves grown in local farms within Dodoma. The mineral and iron contents were also within the range of values reported by Singh (2016) who reported that millets had a relatively high amount of iron. The zinc levels in Tajirika, an improved cassava variety, significantly reduced with processing. From Table 1 above the level of zinc generally reduces with processing for all the samples except for millet whose zinc levels increased. The level of vitamin C was significantly reduced by pre-treatment processing. Vitamin A was not detected in cassava roots and millet.
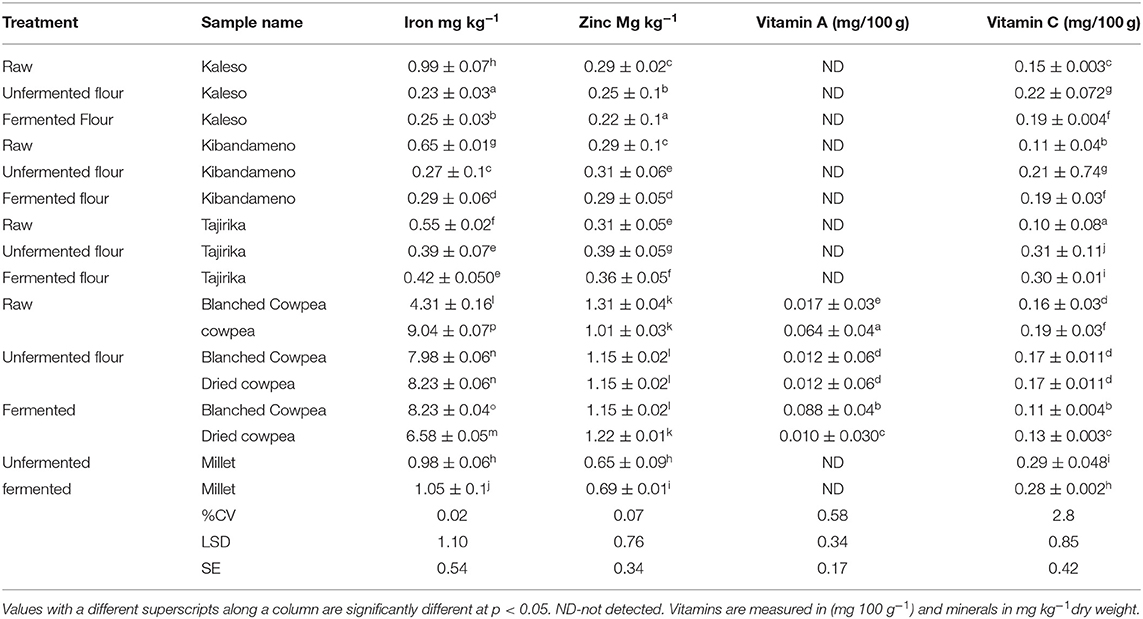
Table 2. Effect of pre-treatment processing on the mineral and vitamin content of raw, unfermented flour and fermented flours of cowpea leaves, millet, and cassava roots.
Conclusion and Recommendation
Pre-treatment processing has significant effects on the nutritional composition of cassava roots, millet, and cowpea leaves. Pre-treatment processing by peeling, washing, solar drying, and fermentation reduces significantly the perishability of the foods while retaining much of the nutrients. Fermentation reduces HCN content to levels that are safe for human consumption. After processing cowpea leaves have 28% protein and thus serves as a good source for blending a composite flour. Millet and cowpea leaves had significantly higher levels of zinc and iron and vitamin A and C than cassava. The dried cassava roots, millet and cowpea leaves flours can be used in formulation of blended nutritious flours while optimizing for proteins, and minerals zinc and iron.
Data Availability Statement
The raw data supporting the conclusions of this article will be made available by the authors, without undue reservation.
Author Contributions
The primary author of the manuscript is SO. GA, MO, DK, and AM contributed through provision of Literature materials to clinically enable the student to discuss the results. All the authors further reviewed the article while giving advise on the best approach to efficiently communicate the findings of the research work.
Funding
This work was supported by MasterCard Foundation through the Regional University Forum for Capacity Building in Agriculture (RUFORUM) under the Cassava Project RU/2018/CARP+/04. We also acknowledge the support of the University of Nairobi's Food Science laboratory and Seed Enterprise Management Institute (SEMIs) for the success of this work.
Conflict of Interest
The authors declare that the research was conducted in the absence of any commercial or financial relationships that could be construed as a potential conflict of interest.
References
Abok, E., Abong, G., and Okoth, M. (2016). Cassava chips quality as influenced by cultivar, blanching time and slice thickness. Afr. J. Food Agric. Nutr. Dev. 16, 11457–11476. doi: 10.18697/ajfand.76.16855
Abong, G. O., Shibairo, S., Okoth, M., Lamuka, P., Katama, C., and Ouma, J. (2016). Quality and safety characteristics of cassava crisps sold in urban Kenya. Afr. Crop Sci. J. 24:89. doi: 10.4314/acsj.v24i1.9S
Animasaun, D. A., Oyedeji, S., Mustapha, O. T., and Azeez, M. A. (2015). Genetic variability study among ten cultivars of cowpea (Vigna unguiculata L. Walp) using morpho-agronomic traits and nutritional composition. J. Agric. Sci. 10:119. doi: 10.4038/jas.v10i2.8057
Blanshard, A. F. J., Dahniya, M. T., Poulter, N. H., and Taylor, A. J. (1994). Fermentation of cassava into foofoo: effect of time and temperature on processing and storage quality. J. Sci. Food Agric. 66, 485–492. doi: 10.1002/jsfa.2740660410
Chouhan, M., Gudadhe, N. N., Kumar, D., Kumawat, A. K., and Kumar, R. (2015). Transplanting dates and nitrogen levels influences on growth, yield attributes, and yield of summer pearl. Bioscan 10, 1295–1298.
Emmanuel, O. A., Clement, A., Agnes, S. B., Chiwona-Karltun, L., and Drinah, B. N. (2012). Chemical composition and cyanogenic potential of traditional and high yielding CMD resistant cassava (Manihot esculenta crantz) varieties. Int. Food Res. J. 19, 175–181.
FAO (2003). FAO Food and Nutrition Paper 77. Food Energy - Methods of Anlysis and Conversion Factors.
FAOSTAT (2011). Retrieved from: http://www.fao.org/faostat/en/#data/QC
Fayemi, O. E., and Ojokoh, A. O. (2014). The effect of different fermentation techniques on the nutritional quality of the cassava product (FUFU). J. Food Process. Preserv. 38, 183–192. doi: 10.1111/j.1745-4549.2012.00763.x
Gacheru, P., Abong, G., Okoth, M., Lamuka, P., Shibairo, S., and Katama, C. (2015). Cyanogenic content, aflatoxin level and quality of dried cassava chips and flour sold in nairobi and coastal regions of Kenya. Curr. Res. Nutr. Food Sci. J. 3, 197–206. doi: 10.12944/CRNFSJ.3.3.03
Gerrano, A. S., Adebola, P. O., van Rensburg, W. S. J., and Venter, S. L. (2015). Genetic variability and heritability estimates of nutritional composition in the leaves of selected cowpea genotypes [Vigna unguiculata (L.) Walp.]. HortScience 50, 1435–1440. doi: 10.21273/HORTSCI.50.10.1435
Gonçalves, A., Goufo, P., Barros, A., Domínguez-Perles, R., Trindade, H., Rosa, E. A. S., et al. (2016). Cowpea (Vigna unguiculata L. Walp), a renewed multipurpose crop for a more sustainable agri-food system: nutritional advantages and constraints. J. Sci. Food Agric. 96, 2941–2951. doi: 10.1002/jsfa.7644
Guédé, S. S. (2014). Assessment of cyanide content in cassava (Manihot esculenta Crantz) varieties and derived products from Senegal. Int. J. Nutr. Food Sci. 2:225. doi: 10.11648/j.ijnfs.20130205.12
Ismaila, A. R., Alakali, J. S., and Atume, T. G. (2018). Effect of local processing techniques on the nutrients and anti-nutrients content of bitter cassava (Manihot Esculenta Crantz). Am. J. Food Sci. Technol. 6, 92–97. doi: 10.12691/ajfst-6-3-1
Kulwa, K. B. M., Mamiro, P. S., Kimanya, M. E., Mziray, R., and Kolsteren, P. W. (2015). Feeding practices and nutrient content of complementary meals in rural central Tanzania: implications for dietary adequacy and nutritional status. BMC Pediatr. 15, 1–11. doi: 10.1186/s12887-015-0489-2
Kumar, A., Metwal, M., Kaur, S., Gupta, A. K., Puranik, S., Singh, S., et al. (2016). Nutraceutical value of finger millet [Eleusine coracana (L.) Gaertn.], and their improvement using omics approaches. Front. Plant Sci. 7, 1–14. doi: 10.3389/fpls.2016.00934
Muoki, P. N., Kinnear, M., Emmambux, M. N., and de Kock, H. L. (2015). Effect of the addition of soy flour on sensory quality of extrusion and conventionally cooked cassava complementary porridges. J. Sci. Food Agric. 95, 730–738. doi: 10.1002/jsfa.6820
Okonya, J. S., and Maass, B. L. (2014). Protein and iron composition of cowpea leaves: an evaluation of six cowpea varieties grown in Eastern Africa. Afr. J. Food Agric. Nutr. Dev. 14, 2129–2140.
Olapade, A. A., Babalola, Y. O., and Aworh, O. C. (2014). Quality attributes of fufu (fermented cassava) flour supplemented with bambara flour. Int. Food Res. J. 21, 2025–2032.
Oresegun, A., Fagbenro, O. A., Ilona, P., and Bernard, E. (2016). Nutritional and anti-nutritional composition of cassava leaf protein concentrate from six cassava varieties for use in aqua feed. Cogent Food Agric. 2, 1–6. doi: 10.1080/23311932.2016.1147323
Owade, J., George, A., Okoth, M., and Mwang'ombe, A. (2019). “Utilization of traditional processed and preserved cowpea leaves in the Coastal Region of Kenya,” in Conference on International Research on Food Security, Natural Resource Management and Rural Development (Kassel), 2017–2020.
Salvador, E. M., Steenkamp, V., and McCrindle, C. M. E. (2014). Production, consumption and nutritional value of cassava (Manihot esculenta, Crantz) in Mozambique: an overview. J. Agric. Biotechnol. Sustain. Dev. 6, 29–38. doi: 10.5897/JABSD2014.0224
Sharma, S., Saxena, D. C., and Riar, C. S. (2016). Nutritional, sensory and in-vitro antioxidant characteristics of gluten free cookies prepared from flour blends of minor millets. J. Cereal Sci. 72, 153–161. doi: 10.1016/j.jcs.2016.10.012
Shittu, T. A., Raji, A. O., and Sanni, L. O. (2007). Bread from composite cassava-wheat flour: I. Effect of baking time and temperature on some physical properties of bread loaf. Food Res. Int. 40, 280–290. doi: 10.1016/j.foodres.2006.10.012
Singh, E. S. (2016). Potential of millets: nutrients composition and health benefits. J. Sci. Innov. Res. 5, 46–50. Available online at: www.jsirjournal.com (accessed July 27, 2019).
Keywords: cassava roots, pre-treatment, millet, iron, zinc, cowpea leaves
Citation: Onyango SO, Abong GO, Okoth MW, Kilalo DC and Mwang'ombe AW (2021) Effect of Pre-treatment and Processing on Nutritional Composition of Cassava Roots, Millet, and Cowpea Leaves Flours. Front. Sustain. Food Syst. 5:625735. doi: 10.3389/fsufs.2021.625735
Received: 03 November 2020; Accepted: 30 April 2021;
Published: 02 June 2021.
Edited by:
Selena Ahmed, Montana State University, United StatesReviewed by:
Shalini Gaur Rudra, Indian Agricultural Research Institute (ICAR), IndiaTânia Gonçalves Albuquerque, Instituto Nacional de Saúde Doutor Ricardo Jorge (INSA), Portugal
Copyright © 2021 Onyango, Abong, Okoth, Kilalo and Mwang'ombe. This is an open-access article distributed under the terms of the Creative Commons Attribution License (CC BY). The use, distribution or reproduction in other forums is permitted, provided the original author(s) and the copyright owner(s) are credited and that the original publication in this journal is cited, in accordance with accepted academic practice. No use, distribution or reproduction is permitted which does not comply with these terms.
*Correspondence: Samwel Ochieng Onyango, c2Ftd2Vsb255YW5nbzI3QGdtYWlsLmNvbQ==